- 1Department of Neurology and Stroke Center, The First Affiliated Hospital, Jinan University, Guangzhou, China
- 2Clinical Neuroscience Institute, The First Affiliated Hospital, Jinan University, Guangzhou, China
- 3Department of Central Nervous System Regeneration, Guangdong-Hongkong-Macau Institute of Central Nervous System (CNS) Regeneration (GHMICR), Jinan University, Guangzhou, China
- 4Shenzhen Key Laboratory of Ophthalmology, Shenzhen Eye Hospital, Shenzhen University School of Medicine, Shenzhen, China
- 5Department of Neurology, The Affiliated Shunde Hospital of Jinan University, Guangzhou, China
Aims: Retinal microvasculature shares prominent similarities with the brain vasculature. We aimed to assess the association between retinal microvasculature and subtypes of ischemic stroke.
Method: We consecutively enrolled ischemic stroke patients within 7 days of onset, who met the criteria of subtype of atherothrombosis (AT), small artery disease (SAD), or cardioembolism (CE) according to a modified version of the Trial of Org 10172 in Acute Stroke Treatment (NEW-TOAST). Digital fundus photographs were taken within 72 h of hospital admission using a digital camera (Topcon TRC-50DX), and fundus photographs were semi-automatically measured by software (Canvus 14 and NeuroLucida) for retinal vasculature parameters.
Results: A total of 141 patients were enrolled, including 72 with AT, 54 with SAD, and 15 with CE. AT subtype patients had the widest mean venular diameter within 0.5–1.0 disk diameter (MVD0.5−1.0DD) followed by SAD and CE subtypes (86.37 ± 13.49 vs. 83.55 ± 11.54 vs. 77.90 ± 8.50, respectively, P = 0.047); CE subtype patients had the highest mean arteriovenous ratio within 0.5–1.0 disk diameter (MAVR0.5−1.0DD) followed by the AT and SAD subtype groups (0.97 ± 0.03 vs. 0.89 ± 0.99 vs. 0.89 ± 0.11, respectively, P = 0.010); SAD subtype patients were found with the highest mean venular tortuosity within 0.0–2.0 disk diameter (MVT0.0−2.0DD) followed by the AT and CE subtypes (1.0294 ± 0.0081 vs. 1.0259 ± 0.0084 vs. 1.0243 ± 0.0066, respectively, P = 0.024). After adjusting for clinic characteristics, MVD0.5−1.0DD was significantly different among AT, SAD, and CE subtypes (P = 0.033). By receiver operating characteristic curve analysis, MVD0.5−1.0DD predicted the AT subtype (area 0.690, 95% confidence interval, 0.566–0.815), with a cutoff value of 82.23 μm (sensitivity 61.1%, specificity 73.3%).
Conclusion: Retinal MVD0.5−1.0DD (>82.23 μm) might be associated with the AT stroke subtype; however, we need large-scale prospective studies in future to explore the underlying mechanism and causal explanation for this finding.
Introduction
The retinal microvasculature shares common features with the cerebral circulation (1). Studying the retinal microvasculature contributes to our understanding of pathological process of ischemic stroke. Evidence of retinal microvascular signs (such as microaneurysms, hemorrhages, arteriovenous nicking, and focal arteriolar narrowing) were associated with transient ischemic attack (TIA) as well as stroke (2–4) and could predict progression of brain microvascular diseases (leukoaraiosis and lacunar infarcts) (5). Studies had shown that variation in retinal arteriolar diameter was associated with intracranial arterial stenosis (6), and larger retinal venular diameters were associated with an increased risk of stroke (7).
These findings further supported that retinal microvascular abnormalities were associated with stroke, but more data were required to clarify associations between specific types of retinal microvascular abnormality and subtypes of stroke (8). A few studies had investigated the relationship between retinal vascular parameters and subtypes of ischemic stroke with inconsistent conclusions. Doubal et al. (9) found that patients with lacunar infarction had wider venular diameter and narrower arterial diameter than patients with cortex infarction. Some other studies also suggested that retinal vascular abnormalities (narrower arterial diameter, wider venular diameter, arteriovenous nicking, and focal arteriolar narrowing) were associated with lacunar infarction (10–13). In contrast, Ong et al. (14) found no difference in retinal vascular parameters between stroke subtypes using a modified version of the Trial of Org 10172 in Acute Stroke Treatment (NEW-TOAST) classification. The different methods of stroke classification in previous studies may be one of the reasons for the inconsistent results.
The NEW-TOAST classification is the most widely used and approved form of etiologic subtyping with high inter-rater reliability (kappa = 0.82) (15). Clinical therapies and secondary prevention strategies vary among stroke subtypes and have significant impacts on patient outcomes (16).
However, stroke subtyping relies on detailed history taking and complementary investigations, which are not conducive to screening. This study aimed to assess the association between retinal microvascular parameters and subtypes of ischemic stroke defined by NEW-TOAST classification.
Methods
Study Subjects
From October 2015 to March 2017, we consecutively included patients with ischemic stroke admitted to our stroke center within 7 days of onset. We recorded patient demographics, vascular risk factors, past medical history, neurological deficit, the National Institutes of Health Stroke Scale (NIHSS) scores, and acute vascular recanalization therapies after admission, as well as results of stroke-related investigation (detailed information in examination flow chart, Figure 1). We determined the patient's stroke subtype according to the NEW-TOAST criteria based on the patient's clinical findings. We included all patients over 18 years with New-TOAST stroke subtypes of atherothrombosis (AT), small artery disease (SAD), or cardioembolism (CE) and excluded patients who had not undergone fundus photograph examination or with ocular diseases that affected the visualization of fundus photography.
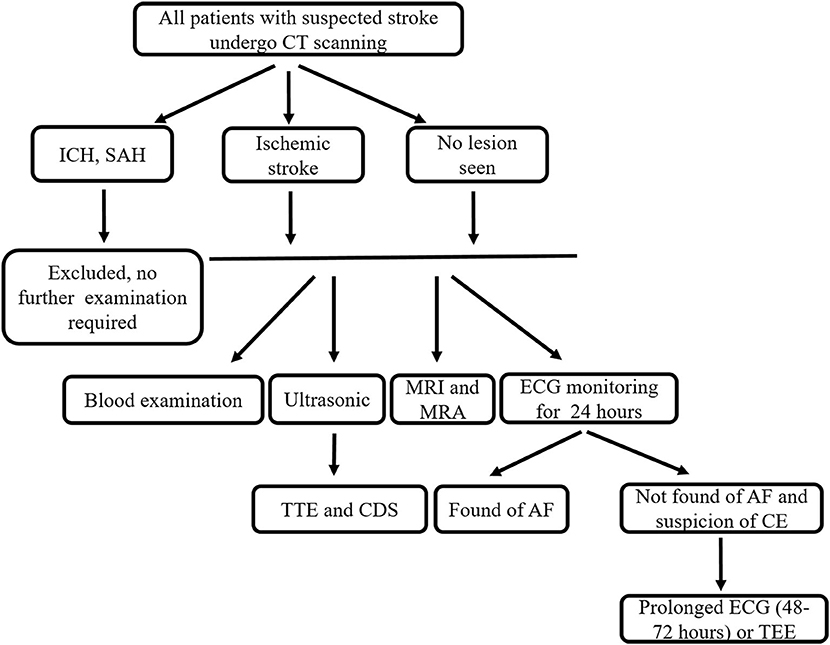
Figure 1. Examination flow chart. CT, computer tomography; ICH, intracerebral hemorrhage; SAH, subarachnoid hemorrhage; MRI, magnetic resonance imaging; MRA, magnetic resonance angiography; ECG, electrocardiogram; TTE, transthoracic echocardiography; CDS, carotid duplex sonography; AF, atrial fibrillation; CE, cardioembolism; TEE, transesophageal echocardiography.
Assessment of Retinal Vascular Parameters
Digital fundus photographs were taken within 72 h of hospital admission by dilating with 1% tropicamide eye drops as standardized protocol. We used a camera (Topcon TRC-50DX) to photograph bilateral retinal vasculature. Photographs were centered on the region of the optic disc and were taken in 50° range. We took the average of each retinal vasculature parameter values on both sides as the final values. We drew a scaleplate in the fundus camera to get the actual size of diameter and measured the scaleplate in NeuroLucida program.
All fundus photographs were graded at the Guangdong-Hongkong-Macau Institute of Central Nervous System (CNS) Regeneration (GHMICR) of Jinan University using software Canvus 14 (Advanced Cell Diagnostics, Inc.) and NeuroLucida (MicroBrightField, Inc.). Trained graders, without knowledge of the identification of participants, used the Canvus 14 to draw concentric circles within the scope of 0.0–2.0 disc diameters (DD) from the optic disk margin per interval distance 0.5 DD (Figures 2A,B). Vessels were traced according to the diameter and trajectory by software NeuroLucida (Figure 2C). Prior to tracing the vessels, we labeled arteries and veins according to their different characteristics (thin and pink for arteries, thick and purple for veins). For better observation of the morphological characteristic of target vessels, we extracted vascular images from the background of fundus (Figure 2D). Software Neurolucida automatically calculated the diameter, tortuosity, and branching angle of the vessels. We measured retinal vascular parameters (vascular diameter, arteriovenous ratio, tortuosity, and branching angle) and analyzed them.
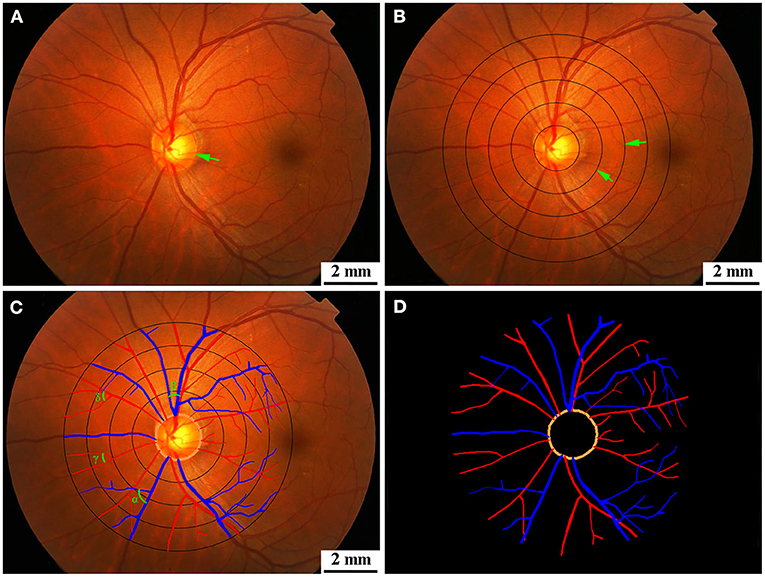
Figure 2. (A) Original diagram. The green arrow showed optic disk. (B) Retinal photographs graded by Canvus 14, drew concentric circles within the scope of 0.0–2.0 DD from the optic disk margin per interval distance 0.5 DD. The green arrows showed concentric circles. (C) Retinal photographs graded by NeuroLucida. Arteries are traced as red line, and veins are traced as blue line. The branching angles of vessels are shown as α, β, γ, and δ in (C). (D) Segmentation of retina vessels. DD, disk diameter.
Definition and Automatic Calculation of Retinal Vascular Parameters
The DD was measured by drawing a circle along the optic disk margin, which was calculated by software NeuroLucida. Retinal vascular diameters (the internal size of the vessel) were calculated as the average diameter of arteries or veins coursing from 0.5 to 1.0 DD by software NeuroLucida and expressed as mean arterial diameter (MAD) or mean venular diameter (MVD). The mean arteriovenous ratio (MAVR) from 0.5 to 1.0 DD was defined as the ratio of MAD and MVD (MAD/MVD). Retinal vascular tortuosity was defined as the integral of the curvature square along the vessel path, normalized by the total path length (17). The tortuosity of retinal arteries or veins from 0.0 to 2.0 DD were expressed as mean arterial tortuosity (MAT) or mean venular tortuosity (MVT) and automatically calculated by software NeuroLucida. Smaller values of MAT or MVT represent less tortuosity of the retinal vessels. The retinal vascular branching angle was the first angle subtended between two daughter vessels at each vascular bifurcation (18). In this study, the average branching angle of the arteries and veins in 0.0–2.0 DD were automatically calculated by software NeuroLucida and expressed as mean arterial branching angle (MABA) and mean venular branching angle (MVBA), respectively.
Assessment of Ischemic Stroke Subtypes
The classification of stroke subtype was based on the results of routine hospital investigations including blood examination, transthoracic echocardiography (TTE), carotid duplex sonography (CDS), 24 h electrocardiogram (ECG) monitoring and brain imaging [computer tomography (CT), magnetic resonance imaging (MRI), and MR angiography (MRA)] (see examination flow chart, Figure 1). In order to screen patients for CE, patients with clinical suspicion of CE underwent prolonged ECG (48–72 h) or transesophageal echocardiography (TEE) according to our routine hospital protocol. Two senior neurologists with over 10 years of experience (Z.T. and Y.Z.) classified the different stroke subtypes according to the NEW-TOAST classification (15, 19). Discrepancies between the two evaluators were decided after consultation with another neurologist A.X. (more than 15 years of experience). The specific standards and methods of classification were performed as described previously (15).
Statistical Analysis
All data were analyzed with the statistical software program IBM SPSS Statistics 25. One-way analysis of variance (ANOVA) followed by the Student–Newman–Keuls (SNK) multiple comparison tests was used for continuous variables. Pearson chi-square test comparing multiple sample rates was used for categorical variables. We used one-way ANOVA and Pearson chi-square test for assessing the differences in retinal vascular parameters among different subtypes of ischemic stroke. The analysis of covariance was used for adjusting demographic and clinic characteristics [age, sex, current cigarette smoker, hypertension, diabetes, dyslipidemia, stroke history, coronary disease, peripheral artery disease, and body mass index (BMI)]. We applied receiver operating characteristic (ROC) curve to evaluate the diagnostic value of MVD0.5−1.0DD for AT stroke subtype. P < 0.05 were considered as significant.
Results
From October 2015 to March 2017, 141 acute ischemic stroke (AIS) patients were enrolled in this study with qualified retinal microvascular images (see screening flow chart, Figure 3). Among them, 72 patients were AT, 54 patients belonged to SAD, and 15 were geared to CE. The demographic and clinic characteristics of the subjects are shown in Table 1. No significant differences were found among AT, SAD, and CE subtype in gender, current smoking, diabetes, stroke history, coronary disease, peripheral artery disease, BMI, Modified Rankin Scale (mRS), Barthel Index (BI), and NIHSS. Among three subtype groups, the SAD subtype was the youngest (P = 0.001). The CE subtype had the least proportion of hypertension (P = 0.009) and hyperlipidemia (P = 0.000) and the highest rate of acute recanalization treatment (P = 0.005).
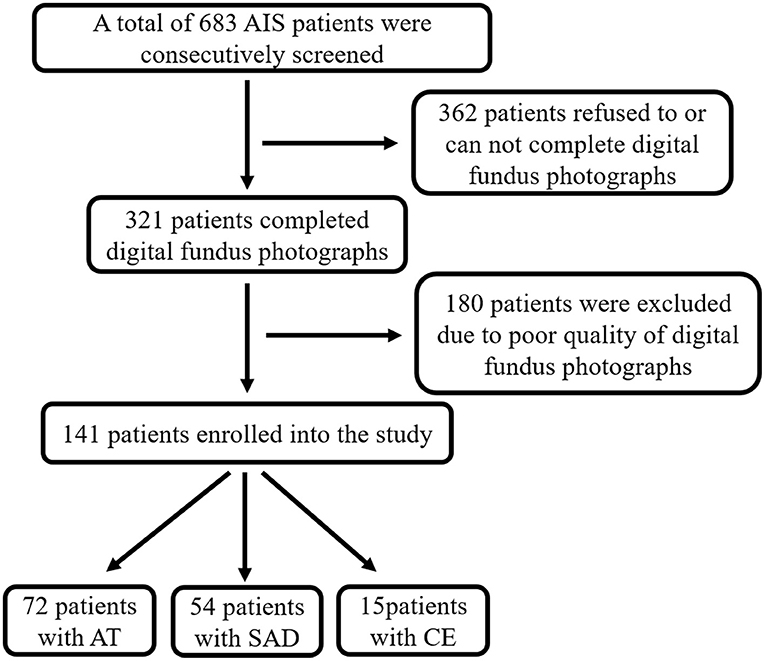
Figure 3. Screening flow chart. AIS, acute ischemic stroke; AT, atherothrombosis; SAD, small artery disease; CE, cardioembolism.
As shown in Table 2, AT subtype had the widest MVD0.5−1.0DD (P = 0.047, Figure 4A), CE subtype had the highest MAVR0.5−1.0DD (P = 0.010, Figure 4B), and the SAD subtype had the largest MVT0.0−2.0DD (P = 0.024, Figure 4C).
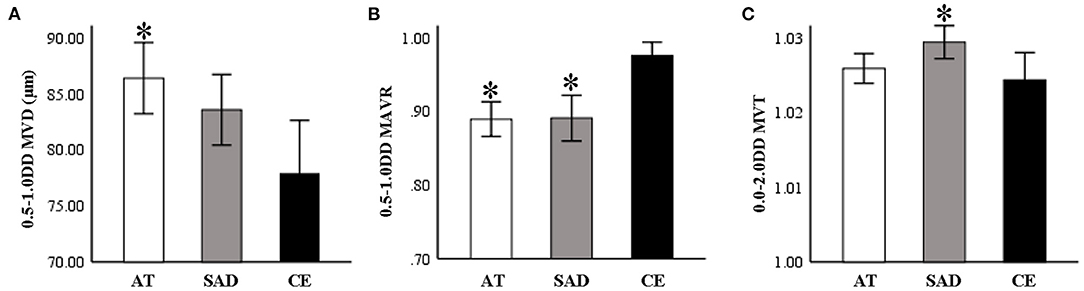
Figure 4. (A) AT subtype group had the widest MVD0.5−1.0DD (P = 0.047). (B) CE subtype group had the highest MAVR0.5−1.0DD (P = 0.010). (C) The SAD subtype group had the largest MVT0.0−2.0DD (P = 0.024). AT, atherothrombosis; SAD, small artery disease; CE, cardioembolism; DD, disk diameter; MVD, mean venular diameter; MAVR, mean arteriovenous ratio; MVT, mean venular tortuosity. *P < 0.05, compared to CE.
Comparison of MVD0.5−1.0DD, MAVR0.5−1.0DD, and MVT0.0−2.0DD among three subtypes is shown in Table 3 after adjusting for age, sex, current cigarette smoker, hypertension, diabetes, dyslipidemia, stroke history, coronary disease, peripheral artery disease, and BMI. MVD0.5−1.0DD was significantly different among the three stroke subtypes (Figure 5). Nevertheless, there were no differences in MAVR0.5−1.0DD and MVT0.0−2.0DD after adjusting for clinic characteristics.
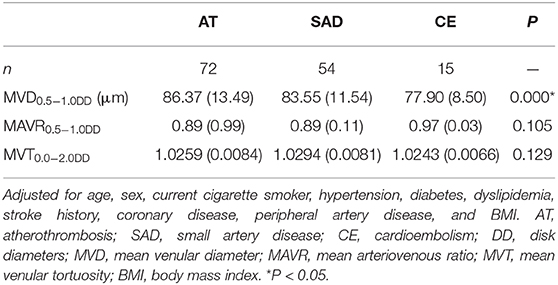
Table 3. Comparison of MVD0.5−1.0DD, MAVR0.5−1.0DD, and MVT0.0−2.0DD among AT, SAD, and CE subtypes after adjusting for clinic characteristics.
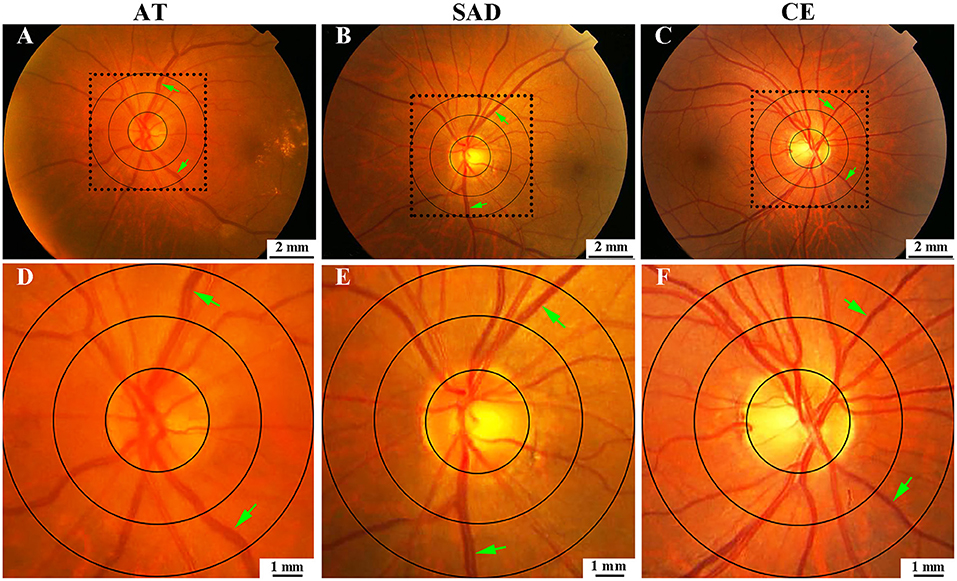
Figure 5. Comparison of MVD0.5−1.0DD among AT, SAD, and CE subtypes. (A) was for AT, (B) was for SAD, (C) was for CE. (D–F) were the magnified images of the selected areas in (A–C), respectively. AT, atherothrombosis; SAD, small artery disease; CE, cardioembolism; DD, disk diameter; MVD, mean venular diameter.
The ROC curve was used to evaluate the diagnostic value of MVD0.5−1.0DD between subtype group AT and CE. As shown in Figure 6, area under ROC curve (AUC) for prediction of AT subtype by MVD0.5−1.0DD was 0.690 [95% confidence interval (CI): 0.566–0.815, P = 0.021]. The cutoff value of MVD0.5−1.0DD for the AT subtype classification was 82.23 μm with a sensitivity of 61.1% and specificity of 73.3%.
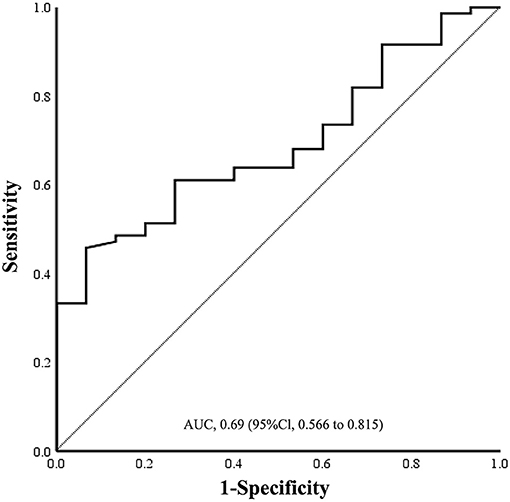
Figure 6. Sensitivity and specificity of ROC curve in the assessment of prediction of AT by MVD0.5−1.0DD. Cutoff value was 82.23 μm. Sensitivity was 61.1%, and specificity was 73.3%. ROC, receiver operating characteristic; AUC, area under curve; AT, atherothrombosis; MVD, mean venular diameter.
Discussion
In this study, we found significant differences in MVD0.5−1.0DD among different stroke subtypes. AT subtype had the widest MVD0.5−1.0DD, and MVD0.5−1.0DD (>82.23 μm) was associated with the classification of AT stroke subtype.
Previous research has shown that retinal vessel diameter was associated with stroke risk (8, 20). A meta-analysis showed that wider retinal venular diameter predicted stroke (pooled hazard ratio = 1.15, 95% CI: 1.05–1.25 per 20 μm increase in diameter) but not the diameter of retinal arterioles (20). Similar results were reported in another systematic review that wider retinal venular diameter increased the risk of incident stroke by 40% (summary risk ratios = 1.4, 95% CI: 1.1–1.7) (8). These provided shreds of evidence that wider retinal venular diameter was associated with stroke. The mechanisms linking wider retinal venules and stroke were unclear. Concurrent circulatory phenomena in both the retina and brain, such as altered vessel wall stresses, cerebral hypoxia, or venous insufficiency might be the underlying causes (21, 22). Other studies suggested that a wider retinal venular caliber was a marker of endothelial dysfunction and inflammation, as well as an indicator of impaired cerebral oxygen perfusion (23, 24).
Our study found that AT subtype was associated with wider venular diameter. However, we still lack an explanation of the mechanism and causal relationship in this phenomenon. There were few studies that investigated the relationship between retinal vascular parameters and subtypes of ischemic stroke. Consistent with our study, Ikram et al. (25) found an association between atherosclerosis and larger venular diameters. The present study was one of the few studies that found the association between AT subtype and retinal venular diameter in the Asian population.
One of the possible underlying mechanisms of the association between retinal venules and AT subtype may be due to their sharing risk factors for atherosclerosis. Wider retinal venules were more common in diabetic retinopathy (26), and diabetes mellitus was a risk factor for atherosclerosis. Patients with retinal venous thrombosis or deep venous thrombosis were more often associated with atherosclerosis (27, 28). Both atherosclerosis and venous thrombosis were linked to similar risk factors, such as hyperlipidemia, platelet activation, and blood coagulation (28). However, this trend was found controversial in other studies. One study showed that lacunar stroke subtype was associated with wider retinal venular caliber than other stroke subtypes (10, 11), and some other studies showed there were no significant differences in retinal vascular parameters among stroke subtypes (14, 29). One of the reasons for the inconsistency may be the different methods of stroke classification used in different studies.
Software Neurolucida was initially used to perform morphometric measurements of neurons and dendrites (30). The neuron bodies morphologically resemble the optic disc, and the axons and dendrites resemble the retinal vasculature; therefore, we used software Neurolucida for the first time to track the retinal vascular morphology and obtain retinal vascular parameters. We used software to measure all target vessels' actual mean values within the detection range, rather than to measure the relative mean values of some selected vessels as in previous studies. The accuracy and consistency of the software Neurolucida had been demonstrated by the DD we obtained being consistent with the actual diameter in previous studies (9, 31). Similar to our study, some studies used the other computer-assisted program for the measurement of retinal vascular parameters (such as computer monitor or computer imaging program of retinal analysis) (11, 32, 33). In a few studies, retinal photographs were graded by semi-automated computer software, Singapore I Vessel Assessment (SIVA). By selecting the diameters of a main vessel and two branch vessels, the central retinal arteriolar equivalent (CRAE) and central retinal venular equivalent (CRVE) were calculated as the revised Knudtson–Parr–Hubbard formula (14, 34), and selection bias was unavoidable by this approach. This study measured the average diameter of all arterioles and venules coursing through an area of 0.5–1.0 DD from the optic disc margin, which provides us a global reflection of the retinal micro-circulation.
There were some limitations to the present study. Firstly, the present study had a relatively small sample size. Second, the proportion of CE subtype in the present study was relatively lower than other studies, even though most of the CE subtypes can be identified by routine examination. Patients with massive infarction or in serious condition were excluded due to incompetent to complete digital fundus photography examination, especially CE subtype patients with large vessel occlusion. So the present study was not a full reflection of stroke subtypes in the Asian population. Thirdly, we did not set healthy people as control group in this study. In this study, we mainly focused on the difference of retinal vascular parameters in various ischemic stroke subtypes, rather than comparison between healthy and ischemic stroke patients. Furthermore, previous studies also suggested that retinal vascular parameters were associated with ischemic stroke after adjusting for age, sex, race, and cerebrovascular risk factors (2, 8, 14, 35). At last, stroke of other demonstrated etiology (SOE) and stroke of other undemonstrated etiology (SUE) were not included in this study because of their unclear causes of stroke. We could not rule out the possibility that part of them might be AT, CE, or SAD subtypes. Therefore, we looked forward to large-scale longitudinal cohort study focused on observing the association between retinal microvascular changes and followed up ischemic stroke subtype in the near future.
Conclusion
Retinal MVD0.5−1.0DD (>82.23 μm) might be associated with the AT stroke subtype; however, we need large-scale prospective studies in future to explore the underlying mechanism and causal explanation for this finding.
Data Availability Statement
The raw data supporting the conclusions of this article will be made available by the authors, without undue reservation.
Ethics Statement
The studies involving human participants were reviewed and approved by The ethics committees of the First Affiliated Hospital of Jinan University. The patients/participants provided their written informed consent to participate in this study. Written informed consent was obtained from the individual(s) for the publication of any potentially identifiable images or data included in this article.
Author Contributions
Z-FT and H-LH conceived and designed the study. YZ, BY, Y-WR, and YX performed the study. YZ and BY analyzed the data. YZ, BY, Z-FT, and H-LH wrote the paper. A-DX gave suggestions how to design the study, edit the results, and write the manuscript. All authors contributed to the article and approved the submitted version.
Funding
This study was supported by the Cultivating Fund of the First Affiliated Hospital of Jinan University (No. 2015208) and received funding from the Medical Science and Technology Research Council of Guangdong province (No. A2015461); Science and Technology Plan Project of Tianhe District, Guangzhou City, Guangdong Province, China (No. 2018YT006); and the National Nature Science Foundation of China (No. 81671167).
Conflict of Interest
The authors declare that the research was conducted in the absence of any commercial or financial relationships that could be construed as a potential conflict of interest.
Acknowledgments
The authors are most grateful to all patients participating in the study and appreciate colleagues of the department for the support to the enrollment.
References
1. Patton N, Aslam T, Macgillivray T, Pattie A, Deary IJ, Dhillon B. Retinal vascular image analysis as a potential screening tool for cerebrovascular disease: a rationale based on homology between cerebral and retinal microvasculatures. J Anat. (2005) 206:319–48. doi: 10.1111/j.1469-7580.2005.00395.x
2. Wong TY, Klein R, Couper DJ, Cooper LS, Sharrett AR. Retinal microvascular abnormalities and incident stroke: the atherosclerosis risk in communities study. Lancet. (2001) 358:1134–40. doi: 10.1016/S0140-6736(01)06253-5
3. De Silva DA, Manzano JJF, Liu EY, Woon FP, Wong WX, Chang HM, et al. Retinal microvascular changes and subsequent vascular events after ischemic stroke. Neurology. (2011) 77:896–903. doi: 10.1212/WNL.0b013e31822c623b
4. Jie JW, Baker ML, Hand PJ, Hankey GJ, Mitchell P. Transient ischemic attack and acute ischemic stroke associations with retinal microvascular signs. Stroke. (2011) 42:404–8. doi: 10.1161/STROKEAHA.110.598599
5. Hanff TC, Sharrett AR, Mosley TH, Shibata D, Knopman DS, Klein R, et al. Retinal microvascular abnormalities predict progression of brain microvascular disease: an ARIC MRI study. Stroke. (2014) 45:1012. doi: 10.1161/STROKEAHA.113.004166
6. Rhee EJ, Chung PW, Wong TY, Song SJ. Relationship of retinal vascular caliber variation with intracranial arterial stenosis. Microvasc Res. (2016) 108:64–8. doi: 10.1016/j.mvr.2016.08.002
7. Ikram MK, De Jong FJ, Bos MJ, Vingerling JR, Hofman A, Koudstaal PJ, et al. Retinal vessel diameters and risk of stroke: the rotterdam study. Neurology. (2006) 66:1339–43. doi: 10.1212/01.wnl.0000210533.24338.ea
8. Doubal FN, Hokke PE, Wardlaw JM. Retinal microvascular abnormalities and stroke: a systematic review. J Neurol Neurosurg Psychiatry. (2009) 80:158–65. doi: 10.1136/jnnp.2008.153460
9. Doubal FN, MacGillivray TJ, Hokke PE, Dhillon B, Dennis MS, Wardlaw JM. Differences in retinal vessels support a distinct vasculopathy causing lacunar stroke. Neurology. (2009) 72:1773–8. doi: 10.1212/WNL.0b013e3181a60a71
10. Lindley RI, Wang JJ, Wong M-C, Mitchell P, Liew G, Hand P, et al. Retinal microvasculature in acute lacunar stroke: a cross-sectional study. Lancet Neurol. (2009) 8:628–34. doi: 10.1016/S1474-4422(09)70131-0
11. Yatsuya H, Folsom AR, Wong TY, Klein R, Sharrett AR. Retinal microvascular abnormalities and risk of lacunar stroke atherosclerosis risk in communities study. Stroke. (2010) 41:1349–55. doi: 10.1161/STROKEAHA.110.580837
12. Cheung N, Liew G, Lindley RI, Liuc EY, Wang JJ, Hand P, et al. Retinal fractals and acute lacunar stroke. Ann Neurol. (2010) 68:107–11. doi: 10.1002/ana.22011
13. Gobron C, Erginay A, Massin P, Lutz G, Tessier N, Vicaut E, et al. Microvascular retinal abnormalities in acute intracerebral haemorrhage and lacunar infarction. Rev Neurol. (2014) 170:13–8. doi: 10.1016/j.neurol.2013.07.029
14. Ong YT, De Silva DA, Cheung CY, Chang HM, Chen CP, Wong MC, et al. Microvascular structure and network in the retina of patients with ischemic stroke. Stroke. (2013) 44:2121–7. doi: 10.1161/STROKEAHA.113.001741
15. Han SW, Kim SH, Lee JY, Chu CK, Yang JH, Shin HY, et al. A new subtype classification of ischemic stroke based on treatment and etiologic mechanism. Eur Neurol. (2007) 57:96–102. doi: 10.1159/000098059
16. Powers WJ, Rabinstein AA, Ackerson T, Adeoye OM, Bambakidis NC, Becker K, et al. Guidelines for the early management of patients with acute ischemic stroke: 2019 update to the 2018 guidelines for the early management of acute ischemic stroke: a guideline for healthcare professionals from the American heart association/American stroke association. Stroke. (2019) 50:344–418. doi: 10.1161/STR.0000000000000211
17. Cheung YL, Zheng Y, Hsu W, Lee ML, Wong TY. Retinal vascular tortuosity, blood pressure, and cardiovascular risk factors. Ophthalmology. (2010) 118:812–8. doi: 10.1016/j.ophtha.2010.08.045
18. Zamir M, Medeiros JA, Cunningham TK. Arterial bifurcations in the human retina. J Gen Physiol. (1979) 74:537–48. doi: 10.1085/jgp.74.4.537
19. Adams HP, Bendixen BH, Kappelle LJ, Biller J, Love BB, Gordon DL, et al. Classification of subtype of acute ischemic stroke. Definitions for use in a multicenter clinical trial. TOAST. Trial of Org 10172 in acute stroke treatment. Stroke. (1993) 24:35–41. doi: 10.1161/01.STR.24.1.35
20. McGeechan K, Liew G, Macaskill P, Irwig L, Klein R, Klein BEK, et al. Prediction of incident stroke events based on retinal vessel caliber: a systematic review and individual-participant meta-analysis. Am J Epidemiol. (2009) 170:1323–32. doi: 10.1093/aje/kwp306
21. Black S, Gao F, Bilbao J. Understanding white matter disease: imaging-pathological correlations in vascular cognitive impairment. Stroke. (2009) 40(Suppl. 1):48–52. doi: 10.1161/STROKEAHA.108.537704
22. Sun C, Wang JJ, Mackey DA, Wong TY. Retinal vascular caliber: systemic, environmental, and genetic associations. Surv Ophthalmol. (2009) 54:74–95. doi: 10.1016/j.survophthal.2008.10.003
23. Cheung N, Islam F, Jacobs D Jr, Sharrett A, Klein R, Polak J, et al. Arterial compliance and retinal vascular caliber in cerebrovascular disease. Ann Neurol. (2007) 62:618–24. doi: 10.1002/ana.21236
24. Jong FJD, Ikram MK, Witteman JCM, Hofman A, Breteler MMB. Retinal vessel diameters and the role of inflammation in cerebrovascular disease. Ann Neurol. (2010) 61:491–5. doi: 10.1002/ana.21129
25. Ikram MK, De Jong FJ, Vingerling JR, Witteman JCM, Hofman A, Breteler MMB, et al. Are retinal arteriolar or venular diameters associated with markers for cardiovascular disorders? the rotterdam study. Invest Ophthalmol Vis Sci. (2004) 45:2129–34. doi: 10.1167/iovs.03-1390
26. Klein R, Sharrett AR, Klein BEK, Moss SE, Couper D. The association of atherosclerosis, vascular risk factors, and retinopathy in adults with diabetes: the atherosclerosis risk in communities study. Ophthalmology. (2002) 109:1225–34. doi: 10.1016/S0161-6420(02)01074-6
27. Williamson TH. Central retinal vein occlusion: what's the story. Br J Ophthalmol. (1997) 81:698704. doi: 10.1136/bjo.81.8.698
28. Duggirala MK, Cook DA, Mauck KF. An association between atherosclerosis and venous thrombosis. N Engl J Med. (2003) 349:401–2. doi: 10.1056/NEJM200307243490418
29. Luijckx GJ, Boiten J, Kroonenburgh MPMV, Kitslaar P, Lodder J. Systemic small-vessel disease is not exclusively related to lacunar stroke. A pilot study. J Stroke Cerebrovasc Dis. (1998) 7:52–7. doi: 10.1016/S1052-3057(98)80021-9
30. Abate G, Colazingari S, Accoto A, Conversi D, Bevilacqua A. Dendritic spine density and EphrinB2 levels of hippocampal and anterior cingulate cortex neurons increase sequentially during formation of recent and remote fear memory in the mouse. Behav Brain Res. (2018) 344:120–31. doi: 10.1016/j.bbr.2018.02.011
31. Rao HL, Puttaiah NK, Babu JG, Maheshwari R, Senthil S, Garudadri CS. Agreement among 3 methods of optic disc diameter measurement. J Glaucoma. (2010) 19:650–4. doi: 10.1097/IJG.0b013e3181ca7f9a
32. Kifley A, Liew G, Wang JJ, Kaushik S, Smith W, Wong TY, et al. Long-term effects of smoking on retinal microvascular caliber. Am J Epidemiol. (2007) 166:1288–97. doi: 10.1093/aje/kwm255
33. Wong TY, Kamineni A, Klein R, Sharrett AR, Klein BE, Siscovick DS, et al. Quantitative retinal venular caliber and risk of cardiovascular disease in older persons: the cardiovascular health study. Arch Intern Med. (2006) 166:2388–94. doi: 10.1001/archinte.166.21.2388
34. Shalev I, Moffitt TE, Wong TY, Meier MH, Houts RM, Ding J, et al. Retinal vessel caliber and lifelong neuropsychological functioning: retinal imaging as an investigative tool for cognitive epidemiology. Psychol Sci. (2013) 24:1198–207. doi: 10.1177/0956797612470959
Keywords: ischemic stroke, subtypes, retinal microvascular changes, venular diameter, neurolucida
Citation: Zhao Y, Yang B, Xu A-D, Ruan Y-W, Xu Y, Hu H-L and Tan Z-F (2021) Retinal Microvascular Changes in Subtypes of Ischemic Stroke. Front. Neurol. 11:619554. doi: 10.3389/fneur.2020.619554
Received: 20 October 2020; Accepted: 23 December 2020;
Published: 27 January 2021.
Edited by:
Simona Sacco, University of L'Aquila, ItalyReviewed by:
Lina Palaiodimou, University General Hospital Attikon, GreeceMichele Romoli, University of Perugia, Italy
Copyright © 2021 Zhao, Yang, Xu, Ruan, Xu, Hu and Tan. This is an open-access article distributed under the terms of the Creative Commons Attribution License (CC BY). The use, distribution or reproduction in other forums is permitted, provided the original author(s) and the copyright owner(s) are credited and that the original publication in this journal is cited, in accordance with accepted academic practice. No use, distribution or reproduction is permitted which does not comply with these terms.
*Correspondence: Ze-Feng Tan, tanzaister@gmail.com; Hui-Ling Hu, huiling_hu@qq.com
†These author share first authorship