- 1Department of Neurology, Tianjin Neurological Institute, Tianjin Medical University General Hospital, Tianjin, China
- 2Department of Neurology, The First Affiliated Hospital of Hainan Medical University, Haikou, China
- 3Department of Clinical Laboratory Center, Tianjin Medical University General Hospital, Tianjin, China
Uric acid (UA) is a natural scavenger for peroxynitrite and can reflect antioxidant activity and oxidative stress in several neurological disorders. Changes in serum and cerebrospinal fluid (CSF) levels of UA have been reported in patients with multiple sclerosis and neuromyelitis optica spectrum disorders. The levels of UA in CSF are relatively poorly understood in patients with Guillain–Barré syndrome (GBS). It remains unclear whether UA can play an antioxidant role and reflect oxidative stress in GBS. The purpose of this study is to investigate CSF and serum UA levels in patients with GBS and their relationship with clinical characteristics. The CSF and serum UA levels were detected in 43 patients with GBS, including 14 acute inflammatory demyelinating polyneuropathy (AIDP), 6 acute motor axonal neuropathy (AMAN), 13 with acute motor and sensory axonal neuropathy (AMSAN), 7 Miller Fisher syndrome (MFS), and 3 unclassified, and 25 patients with non-inflammatory neurological disorders (NIND) as controls. Moreover, serum UA levels were also detected in 30 healthy controls. The levels of UA were measured using uricase-based methods with an automatic biochemical analyzer. CSF UA levels were significantly increased in patients with GBS (p = 0.011), particularly in patients with AIDP (p = 0.004) when compared with NIND. Among patients with GBS, CSF UA levels were higher in those with demyelination (p = 0.022), although the difference was not significant after multiple testing correction. CSF UA levels in GBS were positively correlated with serum UA levels (r = 0.455, p = 0.022) and CSF lactate (r = 0.499, p = 0.011). However, no significant correlations were found between CSF UA levels and GBS disability scores. There were no significant differences in serum UA levels among GBS, NIND, and healthy controls. These results suggest that CSF UA may be related to the pathogenesis of demyelination in patients with GBS and may be partially determined by serum UA and the impaired blood–nerve barrier.
Introduction
Uric acid (UA) is the final metabolite of purine degradation in the human. As a natural scavenger for peroxynitrite and oxygen radicals, UA is thought to be one of the most important antioxidants and responsible for nearly two-thirds of the total plasma antioxidant activity (1, 2). The role of UA in the nervous system has attracted widespread attention during the past 30 years (3–5). The neuroprotective effect of UA in the central nervous system (CNS) has been identified by many investigations in animal models of multiple sclerosis (MS), ischemic brain injury, and spinal cord injury (3, 4, 6, 7). By diminishing oxidative damage in brain, UA may play a potential therapeutic role in the CNS diseases (8–11). Several studies have shown inconsistent UA concentrations in neurological diseases. The UA levels in MS and other neurological diseases have been reported either increased or decreased when compared with controls in different studies (8, 12–17). It has been reported that reduced serum UA levels are linked to the development and progression of neurological diseases, including MS, neuromyelitis optica (NMO), Alzheimer's disease, and Parkinson's disease (8, 12–14). On the other hand, increased levels of UA and purine compounds in cerebrospinal fluid (CSF) and serum of MS patients were reported to be associated with increased purine and adenosine triphosphate (ATP) catabolism and energy imbalance (15). A study in neuromyelitis optica spectrum disorders (NMOSDs) discovered increased CSF UA levels during relapse, suggesting oxidative stress and excitotoxicity in these patients (16). Therefore, UA levels may also reflect oxidative stress and ATP metabolism in neurological diseases (17). These inconsistent findings point to a more complex relationship between UA and neurological disorders. On the one hand, it remains unclear whether the reduced concentration of serum UA is a cause or a consequence of antioxidant in neurological diseases (12). On the other hand, further studies are required to discuss whether the elevation of UA levels in neuroinflammatory diseases is related to oxidative stress and ATP degradation (15–17).
Guillain–Barré syndrome (GBS) is an acute onset autoimmune inflammatory peripheral neuropathy. The clinical features of GBS are characterized by rapid progressive symmetrical muscle weakness, decreased tendon reflexes or areflexia, and neuropathic pain (18). Abnormal immune attacks against peripheral nerves and spinal nerve roots are usually associated with precursor infections or other immune stimulation, which often lead to demyelination or axonal damage (19). There are several clinical variants and subtypes in GBS including mainly demyelinating form: acute inflammatory demyelinating polyneuropathy (AIDP); mainly axonal damage form: acute motor axonal neuropathy (AMAN) or acute motor and sensory axonal neuropathy (AMSAN), and Miller Fisher syndrome (MFS) variant, which is characterized by sensory ataxia, oculomotor weakness, and areflexia (20). Antibodies against gangliosides are generally accepted immunopathogenesis in axonal and MFS variants, whereas unknown antigens, complement activation, and macrophage scavenging are considered to be involved in demyelinating form of GBS (20). In addition, oxidative stress and free radical toxicity have been proven to contribute to the pathogenic mechanism of GBS (21–24). However, compared with MS, NMO, and other diseases in CNS, there are relatively less research focusing on the levels of UA in GBS. Patients with GBS showed reduced serum UA levels compared with healthy controls in the study of Peng et al., but the statistical test of serum UA levels between patients with GBS and healthy controls was not performed (25). Su et al. demonstrated reduced serum levels of UA in GBS patients, but without data for CSF, and proposed UA as a protective factor for GBS (26). However, in the study of Becker et al. (27), urate levels of 18 patients with GBS were higher in CSF samples but did not differ significantly in the serum compared with controls. Unfortunately, in their study, patients with GBS lacked clinical information and were not divided into subtypes of GBS probably due to the small sampling size (27). In the present study, we hypothesized that UA levels in CSF may be increased in patients with GBS, reflecting oxidative stress and ATP metabolism. In addition, it was assumed that CSF UA levels might vary among subtypes of GBS. This study investigated CSF and serum UA levels in 43 patients with GBS and its subtypes and estimated the relationship between CSF UA levels and clinical features.
Materials and Methods
Patients
This study involved 43 patients with GBS who were hospitalized in the Neurology Department of Tianjin Medical University General Hospital from March 2018 to October 2019. Patients with GBS were diagnosed according to the Brighton criteria (28) by experienced neurologist, and the level of diagnostic certainty is shown in Table 1. According to Ho's criteria (29) and Brighton criteria (28), all the GBS patients were divided into four subtypes based on electrophysiological and clinical characteristics: AIDP (n = 14), AMAN or AMSAN (n = 19, including 6 with AMAN and 13 with AMSAN), MFS (n = 7), and unclassified (n = 3). Twenty-five age- and sex-matched patients with non-inflammatory neurological disorders (NINDs) were enrolled as controls, including seven patients with subacute combined degeneration, four patients with mental disorders, four patients with cerebrovascular disease, two patients with cerebral venous sinus thrombosis, two patients with myasthenia gravis, two patients with nutritional and vitamin deficiency neuropathy, two patients with cervical spondylosis, one patient with migraine, and one patient with spinal cavernous hemangioma. Thirty healthy controls from the Health Center of Tianjin Medical University General Hospital were also enrolled in this study. Cases including patients with GBS, NIND controls, and healthy controls who had been treated with acetylsalicylic acid, antitubercular drugs, thiazide diuretics, or other drugs that could affect UA levels (30) within the last 12 weeks, as well as cases with gout, diabetes mellitus, and hepatic or renal disorders were excluded from this study.
Data and Sample Collection
The demographic features, preceding infections, history of disease, GBS disability scores, antiganglioside (GM1/GQ1b) antibodies status, electrophysiology, and CSF parameters of enrolled GBS patients were obtained from our GBS database. The preceding infections of the GBS patients were determined based on the influenza-like syndrome (such as fever and respiratory signs) and gastrointestinal tract infection (usually diarrhea) within previous 4 weeks before the onset of weakness. The severity of disease was evaluated using the GBS disability score (31) at nadir by at least two experienced neurologists independently. CSF samples from the 43 patients with GBS were collected during the acute phase, and CSF samples from 25 patients with NIND were also collected as controls. All CSF samples were immediately aliquoted and stored at −80°C after lumbar puncture until further analysis. Serum samples from 43 patients with GBS, 25 patients with NIND, and 30 healthy controls were collected and stored at −80°C until analysis. All the samples from patients and controls were obtained undisturbedly after an overnight fasting in order to minimize the effects on uric acid.
Measurement of UA Levels
Serum and CSF UA levels were measured using uricase-based methods with a LABOSPECT 008 α Hitachi Automatic Analyzer (Hitachi High-Technologies, Tokyo, Japan) according to the manufacturer's instructions. The normal range of serum UA levels in our hospital is 140–414 μmol/L.
Statistical Analysis
All analyses were performed using SPSS, version20 (IBM Corp., Armonk, NY, USA) and GraphPad Prism, version 7 (GraphPad Software Inc., San Diego, CA, USA). The quantitative data were presented as mean ± standard deviation (SD) or median with interquartile range (IQR) depending on whether the data were normally distributed. In summary, age was normally distributed. The UA levels in CSF and serum, GBS disability scores, and CSF parameters were not normally distributed. A p < 0.05 was considered statistically significant. Mann–Whitney U-tests were conducted for quantitative data analysis between two groups and Kruskal–Wallis H-tests for three or more groups. Correlation between CSF UA levels and age, serum UA levels, CSF parameters, and GBS disability scores were evaluated by Spearman's rank correlation analysis. The one-way ANOVA was used to compare age or other normally distributed continuous data among three or more groups. The chi-square tests or Fisher exact tests were conducted to compare qualitative variables. The Bonferroni correction was performed to adjust p-values for multiple testing.
Results
Demographic and Clinical Features of Subjects
Demographic and clinical features of enrolled cases in the present study are summarized in Table 1. There were no differences in age or gender among patients with GBS, NIND, and healthy controls (p = 0.284 for age and p = 0.906 for gender). In patients with GBS, 55.8% had preceding infections, 44.2% had cranial nerve involvement, 65.1% had antiganglioside antibodies positive, and 90.7% had albuminocytologic dissociation.
Increased CSF UA Levels in GBS and AIDP Compared With NIND
In the present study, CSF UA levels were significantly higher in patients with GBS compared with NIND [12.10 (4.00, 20.80) μmol/L vs. 7.00 (2.30, 10.50) μmol/L, p = 0.011, Figure 1A]. In subtypes of GBS, patients with AIDP, but not other subtypes, had increased CSF UA levels compared with NIND [16.35 (8.90, 28.00) μmol/L vs. 7.00 (2.30, 10.50) μmol/L, p = 0.004, Figure 1A]. However, no differences in CSF UA levels were found among the four subtypes of GBS [16.35 (8.90, 28.00) μmol/L for AIDP, 10.40 (4.00, 17.00) μmol/L for AMAN/AMSAN, 17.70 (2.40, 22.20) μmol/L for MFS, 8.20 (3.00, 36.00) μmol/L for unclassified, p = 0.285].
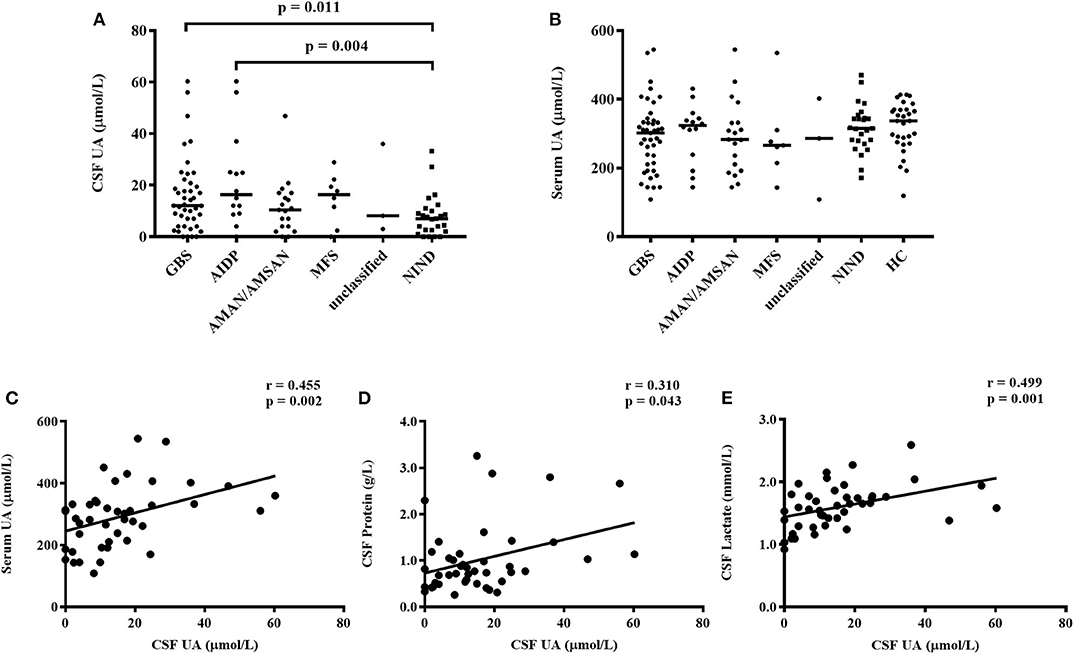
Figure 1. CSF and serum UA levels in subtypes of GBS, NIND, and HC. (A) CSF UA levels were increased significantly in patients with GBS (p = 0.011) and AIDP subtype (p = 0.004) compared with NIND. (B) The difference of serum UA levels among patients with GBS, NIND, and HC were not statistically significant (p = 0.175). (C) Correlation between levels of CSF UA and serum UA, adjusted p = 0.022 after the Bonferroni correction. (D) Correlation between CSF UA and CSF protein, adjusted p = 0.473 after the Bonferroni correction. (E) Correlation between CSF UA and CSF lactate, adjusted p = 0.011 after the Bonferroni correction. GBS, Guillain–Barré syndrome; AIDP, acute inflammatory demyelinating polyneuropathy; AMAN, acute motor axonal neuropathy; AMSAN, acute motor and sensory axonal neuropathy; MFS, Miller Fisher syndrome; NIND, non-inflammatory neurological disorders; HC, healthy control; CSF, cerebrospinal fluid; UA, uric acid.
No Significant Differences in Serum UA Levels Among GBS, NIND, and Healthy Controls
The serum UA levels in GBS, NINID, and healthy controls were 302.00 (210.60, 338.00) μmol/L, 315.00 (274.50, 349.50) μmol/L, and 337.00 (274.00, 370.00) μmol/L, respectively. However, there were no significant differences in serum UA levels among these three groups (p = 0.175, Figure 1B), neither among four subtypes of GBS (p = 0.650, Figure 1B). No significant differences were found in serum UA levels when comparing each subtype of GBS with NIND or healthy controls separately.
UA Levels and Different Clinical Characteristics of Patients With GBS
To investigate the UA levels in GBS patients with different clinical characteristics, 43 patients with GBS were further divided into two subgroups by gender, preceding infections, antiganglioside antibodies, GBS disability scores, cranial nerve involvement, and nerve conduction study (NCS) results (excluded two patients whose NCS results were not available). As shown in Table 2, the CSF UA levels in patients with demyelination were significantly higher than in patients without demyelination (p = 0.022). The serum UA levels of female GBS patients were significantly lower than male patients (p = 0.011). However, after multiple testing corrections (adjusted p-value = p-value*7 characteristics), neither of these differences remained significant. The differences in UA levels were not statistically significant in other clinical characteristics in patients with GBS.
Correlations Between CSF UA Levels and Age, Serum UA Levels, GBS Disability Scores, and CSF Parameters in Patients With GBS
In the present study, Spearman's rank correlation analysis was conducted to investigate the relationship between CSF UA levels and age, serum UA levels, GBS disability scores, and CSF parameters in patients with GBS. CSF UA levels were positively correlated with serum UA levels (r = 0.455, p = 0.002; Figure 1C), CSF protein (r = 0.310, p = 0.043; Figure 1D), and CSF lactate (r = 0.499, p = 0.001; Figure 1E). After using the Bonferroni correction for multiple testing (adjusted p-value = p-value*11 characteristics), the correlations with serum UA levels (adjusted p = 0.022) and CSF lactate (adjusted p = 0.011) were still significant. However, no significant correlations were found between CSF UA levels and age (r = 0.210, p = 0.176), GBS disability scores (r = −0.013, p = 0.934), CSF white blood cells (r = 0.292, p = 0.057), CSF lactate dehydrogenase (r = 0.275, p = 0.074), CSF adenosine deaminase (r = 0.259, p = 0.094), CSF high-sensitive C-reactive protein (r = 0.060, p = 0.705), CSF glucose (r = 0.133, p = 0.394), or CSF chloride (r = −0.147, p = 0.348).
Discussion
There has been a growing interest in the role of UA in the nervous system due to the potential for antioxidant therapy and reflection of oxidative stress and purine catabolism in neurological diseases (15, 17, 32, 33). The results of this study showed that the UA levels in the CSF were significantly elevated in patients with GBS, especially in AIDP, when compared with NIND, but no differences in serum UA levels among GBS, NIND, and healthy controls. These findings were consistent with those of Becker et al. who found markedly increased CSF urate in GBS and bacterial meningitis (27). Unlike Su et al. (26), we did not observe the reduced serum UA levels in patients with GBS. We speculated that the increasing CSF UA levels in patients with GBS may be related to oxidative stress and metabolism of purine and ATP.
UA is considered a natural antioxidant and believed to have neuroprotective functions by counteracting the peroxynitrite-mediated oxidative stress (4, 32). Oxidative stress and impaired antioxidant defense have been established to be involved in the pathogenesis of neuroinflammatory diseases (21–24). Peroxynitrite, a highly reactive oxidant molecule, is notorious for causing cell damage in various conditions including inflammation and neurodegeneration (34). Reactive oxygen species and peroxynitrite may result in cell and tissue damage directly by the oxidation of DNA, proteins, and lipids (35). Activation of glia and macrophages in neuroinflammation may induce oxidative stress by generation of more oxygen and nitrogen free radicals (36). The function of the mitochondrial respiratory chain may be affected by peroxynitrite and other reactive species, which may contribute to the spread of oxidative damage and impaired ATP metabolism (35). As the end metabolite of purine catabolism, UA is related to nucleic acid catabolism and assumed to be associated with adenosine and ATP metabolism, which may be a potential biomarker of energy condition (15, 37).
The CSF UA levels in CNS neuroinflammatory diseases were inconsistent in different studies. Some studies found no significant difference in CSF UA levels between MS patients and controls (27, 38, 39), while one study found lower CSF UA levels in patients with MS (40). Elevated CSF UA levels were observed in patients with MS and NMOSD, which may reflect oxidative stress and excitotoxicity (16, 17). In experimental autoimmune encephalomyelitis, an animal model of MS, UA plays a therapeutic role through inhibiting peroxynitrite-mediated toxicity, maintaining the integrity of the blood–brain barrier, and preventing immune cell invasion (37, 41, 42). Therefore, in view of compensatory mechanisms, UA might be recruited as the antioxidant in CSF under disease condition (27). In the study of Amorini et al. (15), the elevated levels of UA, purine metabolites, and creatinine in both CSF and serum in patients with MS were interpreted as enhanced purine catabolism and energy imbalance, which questioned the view that UA mainly acted as an antioxidant in neurological diseases. However, taking into account the damage of mitochondria and the impaired ATP metabolism in oxidative stress (35), reflecting purine and energy metabolism is not contradictory with UA as an antioxidant. The inconsistent results of different studies might be related to the difference in controls, sample sizes, and measurement methods.
Little attention has been paid to UA in peripheral nerves system diseases such as GBS. In this study, the elevated CSF UA levels in patients with GBS may be interpreted as the compensatory reaction of antioxidant activity against the increasing oxidative stress. In the study of Tang et al. (23), lipophilic antioxidants in GBS patients were decreased, which indicating a reduced resistance to oxidative damage. Kumar et al. (21) found that free radical toxicity in GBS could lead to a compensatory increase in antioxidants such as plasma vitamin E and erythrocyte glutathione. In addition, in experimental autoimmune neuritis, the animal model of GBS, enhanced glycolysis has been proven (43). As a product of glycolysis, lactate in CSF is believed to represent energy metabolism (44). In the present study, UA levels in CSF were positively correlated with CSF lactate, indicating an association between the energy or ATP metabolism and UA levels in patients with GBS. Thus, it is plausible to presume that increased CSF UA levels may also reflect purine metabolism and energy requirements in GBS. Furthermore, the impaired blood–CSF or blood–nerve barrier may also be part of the reason for the increase in CSF UA levels (10, 27). Albuminocytologic dissociation, a characteristic manifestation in CSF of patients with GBS, has been supposed to be caused by the disruption of the blood–nerve barrier on account of inflammation at the nerve root (45). Reactive oxygen species were reported to account for changes in tight junction of endothelium and loss of integrity in the blood–CSF barrier (36). In this study, the UA levels in CSF were positively correlated with serum UA, indicating that CSF UA may be partially determined by serum UA and the impaired blood–CSF or blood–nerve barrier in GBS, which is consistent with previous studies in MS and NMOSD (16, 38). Female GBS patients had lower serum UA levels in the present study, but there was no gender difference in CSF, which is in line with the study of Shu et al. in NMOSD (16). Lower serum UA levels in female have been identified in MS, NMOSD, and healthy controls (25, 46). The reason for the gender differences in UA levels was not clear (46). It was speculated that the lower levels of UA in female were due to the increase in fractional excretion of UA induced by estrogen (47).
Notably, unlike other subtypes of GBS, patients with AIDP showed significant increase in CSF UA levels compared with NIND. Within GBS patients, cases whose NCS results indicted demyelination had higher levels of CSF UA than cases without demyelination, although the difference was not significant after multiple testing correction. The pathophysiological differences in subtypes of GBS have been extensively studied (19). Antibodies against gangliosides (mainly GM1 and GD1a) in exposed nerve membranes at nodes of Ranvier and nerve terminals are specific biomarkers for AMAN and axonal loss type. Antibodies against GQ1b ganglioside are most common in MFS. However, antibody biomarkers have not been identified in AIDP. It is believed that nerve-specific T cell and a wider range of antinerve autoantibodies, as well as complement activation and macrophage scavenging may play an important role in demyelination of AIDP (19). The myelin sheath, largely composed of lipids, is vulnerable to oxidative stress (23, 24). The multiple layers of lipid membranes in myelin sheath could be easily impaired by lipid peroxidation (36). It has been proven that demyelination can be aggravated by oxidative stress and lipid peroxidation (21). Activated macrophages can produce more oxygen and nitrogen free radicals. The inflammatory environment of demyelinating might result in an increase in reactive oxygen and nitrogen species (36). In turn, lipid peroxidation of myelin may contribute to immune pathogenesis of GBS by generation of lipid-derived inflammatory mediators and proinflammatory cytokines (21, 36). The vulnerability to lipid peroxidation in lipid-rich myelin sheath and the increased compensatory antioxidant activity may be the reason for elevated CSF UA levels in AIDP. High levels of CSF UA were also observed in patients of MFS, although this was not statistically significant. MFS is mainly implicated in axolemma rather than the myelin sheath, which is associated with anti-GQ1b antibodies (48). Antibody-mediated inflammation may also lead to oxidative stress and lipid peroxidation of axolemma in MFS. The antioxidant activity against lipid peroxidation in the reticular formation and cerebellum, which possibly expressed GQ1b, may account for the high CSF UA levels in MFS (36, 48).
CSF UA levels are affected by multiple factors (27), and the role of UA in GBS remains uncertain. This study has revealed increased CSF UA levels in patients with GBS and AIDP and showed a prospect that elevated CSF UA may be associated with demyelination. However, no correlations with disease severity limited the CSF UA as a biomarker in GBS. Based on the above previous research findings and assumptions and the results of the present study, we speculated that increased UA concentration in CSF may reflect oxidative stress and enhanced purine or ATP metabolism in patients with GBS.
Several limitations to this study need to be acknowledged. First, the sample size is small in the present study, especially when considering the subtypes of GBS, which may be the limit for firm conclusions. Future research requires a larger sample size and a sufficient number of cases in subtypes of GBS. Second, allantoin, a product of the oxidation of UA, and other products of purine, were not detected in this study. Future work should therefore include allantoin and purine metabolites such as hypoxanthine and xanthine to evaluate oxidative stress and purine catabolism in patients with GBS. Third, although the relationship between CSF UA and demyelination has been proposed with evidence in this study, further studies are needed to verify the lipid peroxidation in GBS probably through estimating malondialdehyde, a biomarker of lipid peroxidation and oxidative stress (49). Fourth, the GBS patients included in this study were all in the acute phase; future research should focus on CSF UA levels in patients with different disease stages.
Conclusion
In summary, this study has shown that the levels of UA in CSF were significantly increased in patients with GBS and AIDP, suggesting that CSF UA may be related to the pathogenesis of demyelination in patients with GBS and may be partially determined by serum UA and the impaired blood–nerve barrier. As a natural antioxidant and the end metabolite of purine catabolism, elevated UA levels in CSF may reflect oxidative stress and enhance purine or ATP metabolism in GBS.
Data Availability Statement
The raw data supporting the conclusions of this article will be made available by the authors, without undue reservation.
Ethics Statement
The studies involving human participants were reviewed and approved by the Medical Research Ethics Committee at Tianjin Medical University General Hospital. The patients/participants provided their written informed consent to participate in this study.
Author Contributions
LY, X-BT, and S-HC contributed to the design and data interpretation. S-HC and X-BT contributed to the drafting of the manuscript. S-HC, JW, and L-JZ contributed to data analysis. M-QL, YQ, C-NH, and C-LG contributed to data collection, case diagnosis, and confirmation. L-SS contributed to sample analysis. LY, X-BT, and D-QZ contributed to critical review. All authors contributed to the article and approved the submitted version.
Funding
This study was financially supported by the National Natural Science Foundation of China (grant numbers 81771363 and 81701239) and the Natural Science Foundation of Tianjin (grant number 18JCQNJC81700).
Conflict of Interest
The authors declare that the research was conducted in the absence of any commercial or financial relationships that could be construed as a potential conflict of interest.
Acknowledgments
We would like to thank the study participants and the clinical care staff at the Tianjin Medical University General Hospital.
References
1. Ames BN, Cathcart R, Schwiers E, Hochstein P. Uric acid provides an antioxidant defense in humans against oxidant- and radical-caused aging and cancer: a hypothesis. Proc Natl Acad Sci USA. (1981) 78:6858–62. doi: 10.1073/pnas.78.11.6858
2. Sautin YY, Johnson RJ. Uric acid: the oxidant-antioxidant paradox. Nucleosides Nucleotides Nucleic Acids. (2008) 27:608–19. doi: 10.1080/15257770802138558
3. Hooper DC, Bagasra O, Marini JC, Zborek A, Ohnishi ST, Kean R, et al. Prevention of experimental allergic encephalomyelitis by targeting nitric oxide and peroxynitrite: implications for the treatment of multiple sclerosis. Proc Natl Acad Sci USA. (1997) 94:2528–33. doi: 10.1073/pnas.94.6.2528
4. Yu ZF, Bruce-Keller AJ, Goodman Y, Mattson MP. Uric acid protects neurons against excitotoxic and metabolic insults in cell culture, and against focal ischemic brain injury in vivo. J Neurosci Res. (1998) 53:613–25. doi: 10.1002/(SICI)1097-4547(19980901)53:5<613::AID-JNR11>3.0.CO;2-1
5. Kanemitsu H, Tamura A, Kirino T, Oka H, Sano K, Iwamoto T, et al. Allopurinol inhibits uric acid accumulation in the rat brain following focal cerebral ischemia. Brain Res. (1989) 499:367–70. doi: 10.1016/0006-8993(89)90786-5
6. Cutler RG, Camandola S, Feldman NH, Yoon JS, Haran JB, Arguelles S, et al. Uric acid enhances longevity and endurance and protects the brain against ischemia. Neurobiol Aging. (2019) 75:159–68. doi: 10.1016/j.neurobiolaging.2018.10.031
7. Scott GS, Cuzzocrea S, Genovese T, Koprowski H, Hooper DC. Uric acid protects against secondary damage after spinal cord injury. Proc Natl Acad Sci USA. (2005) 102:3483–8. doi: 10.1073/pnas.0500307102
8. Koch M, De Keyser J. Uric acid in multiple sclerosis. Neurol Res. (2006) 28:316–9. doi: 10.1179/016164106X98215
9. Peng F, Yang Y, Liu J, Jiang Y, Zhu C, Deng X, et al. Low antioxidant status of serum uric acid, bilirubin and albumin in patients with neuromyelitis optica. Eur J Neurol. (2012) 19:277–83. doi: 10.1111/j.1468-1331.2011.03488.x
10. Bowman GL, Shannon J, Frei B, Kaye JA, Quinn JF. Uric acid as a CNS antioxidant. J Alzheimers Dis. (2010) 19:1331–6. doi: 10.3233/JAD-2010-1330
11. Parkinson Study Group SURE-PD Investigators, Schwarzschild MA, Ascherio A, Beal MF, Cudkowicz ME, Curhan GC, et al. Inosine to increase serum and cerebrospinal fluid urate in Parkinson disease: a randomized clinical trial. JAMA Neurol. (2014) 71:141–50. doi: 10.1001/jamaneurol.2013.5528
12. Kutzing MK, Firestein BL. Altered uric acid levels and disease states. J Pharmacol Exp Ther. (2008) 324:1–7. doi: 10.1124/jpet.107.129031
13. Min J-H, Waters P, Vincent A, Lee S, Shin HY, Lee KH, et al. Reduced serum uric acid levels in neuromyelitis optica: serum uric acid levels are reduced during relapses in NMO. Acta Neurol Scand. (2012) 126:287–91. doi: 10.1111/j.1600-0404.2012.01643.x
14. Cutler RG, Camandola S, Malott KF, Edelhauser MA, Mattson MP. The role of uric acid and methyl derivatives in the prevention of age-related neurodegenerative disorders. Curr Top Med Chem. (2015) 15:2233–8. doi: 10.2174/1568026615666150610143234
15. Amorini AM, Petzold A, Tavazzi B, Eikelenboom J, Keir G, Belli A, et al. Increase of uric acid and purine compounds in biological fluids of multiple sclerosis patients. Clin Biochem. (2009) 42:1001–6. doi: 10.1016/j.clinbiochem.2009.03.020
16. Shu Y, Li H, Zhang L, Wang Y, Long Y, Li R, et al. Elevated cerebrospinal fluid uric acid during relapse of neuromyelitis optica spectrum disorders. Brain Behav. (2017) 7:e00584. doi: 10.1002/brb3.584
17. Stover JF, Lowitzsch K, Kempski OS. Cerebrospinal fluid hypoxanthine, xanthine and uric acid levels may reflect glutamate-mediated excitotoxicity in different neurological diseases. Neurosci Lett. (1997) 238:25–8. doi: 10.1016/S0304-3940(97)00840-9
18. Esposito S, Longo MR. Guillain-Barré syndrome. Autoimmun Rev. (2017) 16:96–101. doi: 10.1016/j.autrev.2016.09.022
19. Willison HJ, Jacobs BC, van Doorn PA. Guillain-Barré syndrome. Lancet. (2016) 388:717–27. doi: 10.1016/S0140-6736(16)00339-1
20. Wijdicks EFM, Klein CJ. Guillain-Barré syndrome. Mayo Clin Proc. (2017) 92:467–79. doi: 10.1016/j.mayocp.2016.12.002
21. Kumar KT, Chandrika A, Sumanth KN, Sireesha P, Rao S, Rao A. Free radical toxicity and antioxidants in guillain-barre syndrome, a preliminary study. Clin Chim Acta. (2004) 346:205–9. doi: 10.1016/j.cccn.2004.03.032
22. Ghabaee M, Jabedari B, Al-E-Eshagh N, Ghaffarpour M, Asadi F. Serum and cerebrospinal fluid antioxidant activity and lipid peroxidation in guillain-barre syndrome and multiple sclerosis patients. Int J Neurosci. (2010) 120:301–4. doi: 10.3109/00207451003695690
23. Tang H-Y, Ho H-Y, Chiu DT-Y, Huang C-Y, Cheng M-L, Chen C-M. Alterations of plasma concentrations of lipophilic antioxidants are associated with guillain-barre syndrome. Clin Chim Acta. (2017) 470:75–80. doi: 10.1016/j.cca.2017.05.001
24. Gümüşyayla S, Vural G, Yurtogullari Çevik S, Akdeniz G, Neselioglu S, Deniz O, et al. Dynamic thiol-disulphide homeostasis in patients with guillain-barre syndrome. Neurol Res. (2019) 41:413–8. doi: 10.1080/01616412.2019.1573955
25. Peng F, Zhang B, Zhong X, Li J, Xu G, Hu X, et al. Serum uric acid levels of patients with multiple sclerosis and other neurological diseases. Mult Scler. (2008) 14:188–96. doi: 10.1177/1352458507082143
26. Su Z, Chen Z, Xiang Y, Wang B, Huang Y, Yang D, et al. Low serum levels of uric acid and albumin in patients with guillain-barre syndrome. Medicine. (2017) 96:e6618. doi: 10.1097/MD.0000000000006618
27. Becker BF, Kastenbauer S, Ködel U, Kiesl D, Pfister HW. Urate oxidation in CSF and blood of patients with inflammatory disorders of the nervous system. Nucleosides Nucleotides Nucleic Acids. (2004) 23:1201–4. doi: 10.1081/NCN-200027469
28. Sejvar JJ, Kohl KS, Gidudu J, Amato A, Bakshi N, Baxter R, et al. Guillain-Barré syndrome and fisher syndrome: case definitions and guidelines for collection, analysis, and presentation of immunization safety data. Vaccine. (2011) 29:599–612. doi: 10.1016/j.vaccine.2010.06.003
29. Ho TW, Mishu B, Li CY, Gao CY, Cornblath DR, Griffin JW, et al. Guillain-Barré syndrome in northern China. relationship to campylobacter jejuni infection and anti-glycolipid antibodies. Brain. (1995) 118:597–605. doi: 10.1093/brain/118.3.597
30. Ben Salem C, Slim R, Fathallah N, Hmouda H. Drug-induced hyperuricaemia and gout. Rheumatology. (2017) 56:679–88. doi: 10.1093/rheumatology/kew293
31. Hughes RA, Newsom-Davis JM, Perkin GD, Pierce JM. Controlled trial prednisolone in acute polyneuropathy. Lancet. (1978) 2:750–3. doi: 10.1016/S0140-6736(78)92644-2
32. Scott GS, Hooper DC. The role of uric acid in protection against peroxynitrite-mediated pathology. Med Hypotheses. (2001) 56:95–100. doi: 10.1054/mehy.2000.1118
33. Kastenbauer S, Koedel U, Becker BF, Pfister HW. Oxidative stress in bacterial meningitis in humans. Neurology. (2002) 58:186–91. doi: 10.1212/WNL.58.2.186
34. Szabó C, Ischiropoulos H, Radi R. Peroxynitrite: biochemistry, pathophysiology and development of therapeutics. Nat Rev Drug Discov. (2007) 6:662–80. doi: 10.1038/nrd2222
35. Lassmann H, van Horssen J. Oxidative stress and its impact on neurons and glia in multiple sclerosis lesions. Biochimica Et Biophysica Acta. (2016) 1862:506–10. doi: 10.1016/j.bbadis.2015.09.018
36. Ortiz GG, Pacheco-Moisés FP, Bitzer-Quintero OK, Ramírez-Anguiano AC, Flores-Alvarado LJ, Ramírez-Ramírez V, et al. Immunology and oxidative stress in multiple sclerosis: clinical and basic approach. Clin Dev Immunol. (2013) 2013:708659. doi: 10.1155/2013/708659
37. Hooper DC, Spitsin S, Kean RB, Champion JM, Dickson GM, Chaudhry I, et al. Uric acid, a natural scavenger of peroxynitrite, in experimental allergic encephalomyelitis and multiple sclerosis. Proc Natl Acad Sci USA. (1998) 95:675–80. doi: 10.1073/pnas.95.2.675
38. Dujmovic I, Pekmezovic T, Obrenovic R, Nikolić A, Spasic M, Mostarica Stojkovic M, et al. Cerebrospinal fluid and serum uric acid levels in patients with multiple sclerosis. Clin Chem Lab Med. (2009) 47:848–53. doi: 10.1515/CCLM.2009.192
39. Kastenbauer S, Kieseier BC, Becker BF. No evidence of increased oxidative degradation of urate to allantoin in the CSF and serum of patients with multiple sclerosis. J Neurol. (2005) 252:611–2. doi: 10.1007/s00415-005-0697-z
40. Zamani A, Rezaei A, Khaeir F, Hooper DC. Serum and cerebrospinal fluid uric acid levels in multiple sclerosis patients. Clin Neurol Neurosurg. (2008) 110:642–3. doi: 10.1016/j.clineuro.2008.03.002
41. Kean RB, Spitsin SV, Mikheeva T, Scott GS, Hooper DC. The peroxynitrite scavenger uric acid prevents inflammatory cell invasion into the central nervous system in experimental allergic encephalomyelitis through maintenance of blood-central nervous system barrier integrity. J Immunol. (2000) 165:6511–8. doi: 10.4049/jimmunol.165.11.6511
42. Spitsin SV, Scott GS, Mikheeva T, Zborek A, Kean RB, Brimer CM, et al. Comparison of uric acid and ascorbic acid in protection against EAE. Free Radic Biol Med. (2002) 33:1363–71. doi: 10.1016/S0891-5849(02)01048-1
43. Liu R-T, Zhang M, Yang C-L, Zhang P, Zhang N, Du T, et al. Enhanced glycolysis contributes to the pathogenesis of experimental autoimmune neuritis. J Neuroinflammation. (2018) 15:51. doi: 10.1186/s12974-018-1095-7
44. Albanese M, Zagaglia S, Landi D, Boffa L, Nicoletti CG, Marciani MG, et al. Cerebrospinal fluid lactate is associated with multiple sclerosis disease progression. J Neuroinflammation. (2016) 13:36. doi: 10.1186/s12974-016-0502-1
45. Brooks JA, McCudden C, Breiner A, Bourque PR. Causes of albuminocytological dissociation and the impact of age-adjusted cerebrospinal fluid protein reference intervals: a retrospective chart review of 2627 samples collected at tertiary care centre. BMJ Open. (2019) 9:e025348. doi: 10.1136/bmjopen-2018-025348
46. Peng F, Zhong X, Deng X, Qiu W, Wu A, Long Y, et al. Serum uric acid levels and neuromyelitis optica. J Neurol. (2010) 257:1021–6. doi: 10.1007/s00415-010-5455-1
47. Yahyaoui R, Esteva I, Haro-Mora JJ, Almaraz MC, Morcillo S, Rojo-Martínez G, et al. Effect of long-term administration of cross-sex hormone therapy on serum and urinary uric acid in transsexual persons. J Clin Endocrinol Metabol. (2008) 93:2230–3. doi: 10.1210/jc.2007-2467
48. Shahrizaila N, Yuki N. Bickerstaff brainstem encephalitis and fisher syndrome: anti-GQ1b antibody syndrome. J Neurol Neurosurg Psychiatry. (2013) 84:576–83. doi: 10.1136/jnnp-2012-302824
Keywords: Guillain-Barré syndrome, acute inflammatory demyelinating polyneuropathy, uric acid, cerebrospinal fluid, purine metabolism
Citation: Chang S-H, Tian X-B, Wang J, Liu M-Q, Huang C-N, Qi Y, Zhang L-J, Gao C-L, Zhang D-Q, Sun L-S and Yang L (2020) Increased Cerebrospinal Fluid Uric Acid Levels in Guillain–Barré Syndrome. Front. Neurol. 11:589928. doi: 10.3389/fneur.2020.589928
Received: 31 July 2020; Accepted: 21 October 2020;
Published: 12 November 2020.
Edited by:
Marcello Moccia, University of Naples Federico II, ItalyReviewed by:
Timothy Michael Ellmore, City College of New York (CUNY), United StatesThomas Langerak, Erasmus Medical Center, Netherlands
Copyright © 2020 Chang, Tian, Wang, Liu, Huang, Qi, Zhang, Gao, Zhang, Sun and Yang. This is an open-access article distributed under the terms of the Creative Commons Attribution License (CC BY). The use, distribution or reproduction in other forums is permitted, provided the original author(s) and the copyright owner(s) are credited and that the original publication in this journal is cited, in accordance with accepted academic practice. No use, distribution or reproduction is permitted which does not comply with these terms.
*Correspondence: Li Yang, eWFuZ2xpMjAwMUB0bXUuZWR1LmNu
†These authors have contributed equally to this work