- Department of Neurology, Peking University First Hospital, Beijing, China
Vascular endothelial cell (EC) and blood–brain barrier (BBB) dysfunction is the core pathogenesis of cerebral small vessel disease (CSVD). Moreover, animal experiments have shown the importance of connexin (Cx)-43 in EC and BBB function. In this study, we recruited 200 patients diagnosed with sporadic CSVD. Initially, we examined imaging scores of white matter hyperintensities (WMH), lacunar infarction (LI), and cerebral microbleeds (CMB). Additionally, we performed next-generation sequencing of the GJA1 gene (Cx43 coding gene) to examine correlation between these single-nucleotide polymorphisms and the burden and distribution of CSVD. Fourteen target loci were chosen. Of these, 13 loci (92.9%) contributed toward risk for cerebellar LI, one locus (7.1%) was shown to be a protective factor for lobar CMB after FDR adjustment. In conclusion, single-nucleotide polymorphisms in the GJA1 gene appear to affect the distribution but not severity of CSVD.
Introduction
Cerebral small vessel disease (CSVD) affects the small arteries, arterioles, venules, and capillaries of the brain, and describes a group of clinical, pathological, and neuroimaging processes of heterogeneous etiologies (1). White matter hyperintensities (WMH), lacunar infarctions (LI), and cerebral microbleeds (CMB) are the three major neuroimaging features of CSVD (2). The topography of the cranial lesions (such as the incidence, severity, and location) differ depending on the disease origin. For example, in the most common inherited CSVD, cerebral autosomal dominant arteriopathy with subcortical infarcts and leukoencephalopathy (CADASIL), WMH tend to be located in the capsula externa and temporal pole (3). Contrarily, with age-related WMH, the lesions tend to involve the frontal lobes. These findings suggest that spatial specificity may provide insight into the underlying vascular pathology and disease mechanisms (4).
Dysfunction of vascular endothelial cells (ECs) and the blood–brain barrier (BBB), chronic ischemia/hypoperfusion and enlarged perivascular spaces play an important role in the pathogenesis of CSVD (5). Dysfunction of ECs may impact upon formation and repair of myelin (6), while breakdown of the BBB may lead to leakage of blood products, both of which can result in thickening and sclerosis of the arteriolar wall. The resultant loss of myelin and gliosis manifests as WMH on brain magnetic resonance imaging (MRI). Severe ischemia of the small vessel territory results in small subcortical infarctions. Leakage of blood products from a microaneurysm results in CMB (7). Therefore, the neuroimaging features of CSVD are closely related to its pathogenicity.
There are two types of vascular endothelial cell–cell junctions: the tight junction and adherens junction, with both playing key roles in the barrier function of the BBB (8, 9). Recently, researchers identified a third category, gap junction channels, that interact with other cell–cell junctions in maintenance of barrier function. Gap junctions appear as plaques at the cell plasma membrane surface and are formed by the interaction of two hemichannels. Each hemichannel is composed of six connexins (Cx), which are transmembrane proteins consisting of a cytoplasmic loop, two extracellular loops, a cytoplasmic C-terminal tail, and a cytoplasmic N-terminal tail. More than 20 different Cx species have been identified in humans. Cx43 is fully expressed across the central nervous system, especially in ECs, vascular smooth muscle cells, pericytes, and astrocytes, which are important components of the BBB (10). An increasing number of in vivo and in vitro experiments suggest that Cx43 is necessary for maintaining BBB function from several aspects including ECs (11, 12), astrocytes (13, 14), and hemichannels (15–17). Further, single-nucleotide polymorphisms (SNPs) of the GJA1 gene (Cx43 coding gene) correlate with primary hypertension (locus rs1925223) (18), congenital heart disease (locus rs2071166) (19), and arrhythmia (locus rs1925223) (20). However, there is currently no clinical evidence showing a relationship between CSVD and Cx43, or any other Cx. In the present study, we performed next-generation sequencing of the GJA1 gene to examine correlation between GJA1 gene SNPs and brain MRI topography of WMH, LI, and CMB in CSVD. Our study provides clinical evidence for the role of Cx43 in the pathogenesis of CSVD.
Materials and Methods
Study Population
The Quanto 1.2 software will measure the sample size. Patients from the Neurology Department of Peking University First Hospital (Beijing, China) were recruited between 2013 and 2019. The inclusion criteria were: age ≥ 40 years-old; neuroimaging changes confirming CSVD, including at least one of the following changes—a recent small subcortical infarct, lacunae of presumed vascular origin, WMH of presumed vascular origin, enlarged perivascular space or CMB (2). Patients with large vessel stenosis [defined as stenosis > 50% detected by carotid ultrasound/transcranial doppler sonography (TCD) or magnetic resonance angiography (MRA) in any large or medium artery supplying brain tissue] or suspicion of cerebral amyloid angiopathy or Alzheimer's disease (based on clinical course or imaging changes) were excluded. Informed consent was obtained from all participants. This research was approved by the Ethics Committee of Peking University Health Science Center (IRB00001052-17018).
Clinical Data
Demographic information of the patients (e.g., age, sex) was collected. Common vascular risk factors including hypertension, diabetes mellitus, dyslipidaemia, coronary heart disease, atrial fibrillation, renal function, serum homocysteine levels, smoking, family history of stroke, and use of antithrombotic drugs were documented. Color Doppler carotid ultrasound, TCD, and MRA were used to determine changes in extracranial and intracranial arteries. Venous blood was collected in EDTA anticoagulant tubes and stored at −80°C.
Magnetic Resonance Scanning and Measuring
Each patient had undergone a head MR scan with sequences including T1-weighted, T2-weighted, fluid attenuated inversion recovery (FLAIR), diffusion weighted imaging (DWI), and T2*-weighted gradient-recalled echo (GRE). The MR scanners used were the General Electric Medical Systems 1.5 T (Chicago, IL, USA) and Philips Medical Systems 3.0 T (Amsterdam, the Netherlands). Axial slices at a slice thickness of 6.0 mm were obtained. One specifically trained rater analyzed the MRI data twice, then the second result was adopted. Key neuroimaging markers including WMH, LI (recent small subcortical infarcts and lacunae of presumed vascular origin), and CMB were examined, following the definition of STandards for ReportIng Vascular changes on nEuroimaging (STRIVE) (2). Perivascular spaces were not estimated in our study. The severity and distribution of WMH were assessed qualitatively and quantitatively. The age-related white matter changes (ARWMC) scale (21) was used to rate the severity and distribution of the lesions. The severity scale included mild (ARWMC scale < 7) and severe (ARWMC ≥ 7) (22). The number and location of LI were recorded. To count the number of lacunae, different areas were examined separately in the right and left hemispheres, that is, frontal, parietooccipital, temporal, corpus callosum, internal capsule, external capsule, basal ganglia, thalamus, brainstem, and cerebellum. The severity included single lacuna and multiple lacunae (number of LI ≥ 2). For microbleeds, the Microbleed Anatomical Rating Scale (MARS) (23) was used to record their number and distribution. The severity scale included mild (MARS < 5) and severe (MARS ≥ 5) (24).
Next-Generation Sequencing and Functional Annotation
Genomic DNA was extracted from peripheral blood of all participants and next-generation sequencing of the GJA1 gene was performed. DNA extraction, Ion Torrent Proton Library Preparation and Sequencing: Genomic DNA was extracted from anticoagulated whole blood of each sample using a commercial Blood Genomic DNA Miniprep kit (Axygen, Union City, CA, USA). The Qubit dsDNA HS Assay kit was used to quantify DNA with the QubitFluorometer (Thermo Fisher Scientific, Inc., Waltham, MA, USA), according to the manufacturer's instructions. 20 ng gDNA for each participant was amplified in two separate primer pool wells using Ion AmpliSeq Custom panel including 14.13kb Cx43 gene target region and AmpliSeq HiFi mix (Thermo Fisher Scientific,Inc, Carlsbad, CA, USA). All two PCR pools were combined in one well and subjected to primer digestion performing incubation with FuPa reagent (Thermo Fisher Scientific, Inc). Amplified Cx43 gene targets were ligated with Ion P1 and Ion Xpress Barcode adapters. After purification libraries were quantified using quantitative PCR with the Ion Library Quantification Kit (Thermo Fisher Scientific, Inc, Cat.no.446880). Sample emulsion PCR and enrichment were performed using the Ion PI HI-Q Template OT2 200 Kit (Thermo Fisher Scientific, Inc, Cat.no.A26434), according to the manufacturer's instructions. Template-positive ISPs were enriched and sequencing was undertaken using Ion PI HI-Q Sequencing 200 Kit (Termofisher, Cat.no.A26433) on the Ion Torrent Proton according to the manufacturer's instructions.
Tag loci were selected based on the allele frequency of variant nucleotides higher than the 1,000 Genomes Project East Asian allele frequency (25) and with a minor allele frequency (MAF) > 1% (26). Association of gene expression levels with genotype was further investigated using the online Genotype-Tissue Expression (GTEx) Portal expression quantitative trait locus (eQTL) browser (http://www.broadinstitute.org/gtex/). An eQTL represents a locus in the genome in which variation between individuals is correlated with a quantitative gene expression trait, often measured as mRNA abundance. The RegulomeDB database (https://regulomedb.org/regulome-search/) determines whether SNPs are located within known or predicted regulatory elements within intergenic regions of the human genome. Functional annotation data for SNPs were then obtained from HaploReg V4 (http://pubs.broadinstitute.org/mammals/haploreg/haploreg_v4.php).
Statistical Analysis
Statistical analyses were performed using the Statistical Package for Social Sciences version 25.0 (IBM SPSS Statistics, Armonk, NY, USA). Depending on distribution of the values, quantitative data are expressed as mean ± standard deviation (SD) or median. Categorical data are described as frequencies and ratios. One-way analysis of variance (ANOVA) was used for comparison of age. Pearson's chi-squared test or Fisher's exact test were used to examine differences in categorical data. Multivariable regression analysis was performed to determine whether there is a significant difference after correcting for the effects of confounding factors such as age. Variables with P < 0.15 in univariable analyses and well-known risk factors of CSVD (age and hypertension) were included in multivariable analyses. P-values were controlled for multiple testing using FDR adjustment. P < 0.05 was considered significant.
Results
Baseline Information
Of 299 patients with sporadic CSVD, 93 patients were excluded: 4 patients for deficient vascular examinations, 62 patients for large vessel stenosis, 14 patients for cardiogenic embolism, 2 patients for demyelinating diseases, and 11 patients for rejecting participation. Overall, 206 patients conformed to the inclusion and exclusion criteria. However, a further 4 patients were excluded for data shortage and 2 patients for contaminated blood. Therefore, data of 200 patients were included in the final analysis (The power using Quanto for testing association using 200 samples is 0.81). Baseline information and neuroimaging features of the patients are shown in Supplementary Table 1. Altogether, 136 (68%) patients were male and the average age was 62.7 ± 11.0 years-old. Regarding disease status, 167 patients were diagnosed with hypertension, 64 (32.0%) with diabetes, 123 (61.5%) with dyslipidaemia, 28 (14.0%) with coronary heart disease, 61 (30.5%) with hyperhomocysteinemia, and 17 (8.5%) with chronic kidney disease. Moreover, 94 (47.0%) patients had a smoking history and 70 (35.0%) patients had a family history of cerebral vessel disease. Further, 67 (33.5%) patients were taking antiplatelet drugs, while 2 (1.0%) patients were taking anticoagulant drugs.
In total, 185 (92.5%) patients presented with WMH, with 97 (52.4%) patients exhibiting severe WMH. Of these WMH lesions, 177 (95.7%) were located in the frontal lobe, 143 (77.3%) in the parietooccipital lobe, 78 (42.2%) in the temporal lobe, 38 (20.5%) in the subtentorium, and 81 (43.8%) in the basal ganglia. Regarding LI, 157 (78.5%) patients presented with LI and 132 (84.1%) patients had multiple LI. Of the lacuna lesions, 103 (65.6%) were located in the frontal lobe, 32 (20.4%) in the parietooccipital lobe, 27 (17.2%) in the temporal lobe, 47 (29.9%) in the hippocampus, 125 (79.6%) in the basal ganglia, 8 (5.1%) in the cerebellum, and 39 (24.8%) in the brainstem. Regarding CMB, 138 patients had brain MRI performed with T2*-weighted GRE. Consequently, 45 (32.6%) patients had CMB, with severe CMB in 23 (51.1%). Of the CMB lesions, 28 (62.2%) were located in lobes, 33 (73.3%) in deep regions, and 21 (46.7%) in infratentorial regions.
Target SNPs of the GJA1 Gene
Overall, gene polymorphisms were detected in 44 loci. Subsequently, we selected 14 of them as target loci (Table 1).
Hardy–Weinberg Equilibrium in GJA1 Gene SNPs
For the 14 target loci, we calculated the genotype frequency of each case group and control group in WMH, LI, and CMB cohorts. We found no significance between the observed value and expected value for genotype distribution using the Hardy–Weinberg equilibrium test. Therefore, all SNPs of target loci can be assumed to represent the population.
Correlation Between SNPs and CSVD
Correlation Between SNPs and WMH
The patients were classified into three groups based upon Cx43 genotype, namely, wild-type homozygous genotype, variant homozygous genotype, and variant heterozygous genotype. We then compared the severity and distribution of WMH among the three groups. There were no significant differences among the three genotypes in the severity or spatial distribution of all 14 loci. We then set the risk factors [P < 0.15 in univariable analyses and well-known risk factors of CSVD (age and hypertension)] as confounding factors (shown in Table 2). After adjusting for confounding factors (multivariable analysis shown in Table 2), we found that WMH tended to be located in the temporal lobe with the variant homozygous genotype compared with the wild-type homozygous genotype for loci rs78125885, rs76430488/rs75464514 (genotype distribution was the same), rs3805788, rs386705335, rs72548744, and rs79597379. Moreover, for locus rs73532215, WMH tended to be located in the infratentorial region for the variant homozygous genotype compared with the wild-type homozygous genotype. There were no significant differences in the severity of WMH among the three genotypes. However, after FDR adjustment, SNPs of all 14 loci were not associated with distribution or severity of WMH (P > 0.05).
Correlation Between SNPs and LI
Again, the patients were distributed into three groups based upon Cx43 genotype, and the severity and distribution of LI compared among the three groups. Before adjusting for confounding factors, we detected significant differences in cerebellar distribution of LI with loci rs79597379, rs72548744, rs34782269, rs76430488/rs75464514 (genotype distribution was the same), rs78125885, rs73532215, rs386705335, rs3840372, rs3805786/rs3805785/rs17083633 (genotype distribution was the same), rs3805788, and rs14962265 (P < 0.05). Further, there were significant differences in parietooccipital lobe distribution of LI with loci rs72548744 and rs73532215 (P < 0.05). There were no significant differences in the severity of LI. Positive results are shown in Table 3.
After adjusting for confounding factors (multivariable analysis shown in Table 4). Moreover, LI tended to be cerebellar in location with the variant homozygous genotype compared with wild-type homozygous genotype at loci rs79597379, rs72548744, rs76430488/rs75464514, rs78125885, rs73532215, rs386705335, rs3840372, rs3805786/rs3805785/rs17083633, rs3805788, and rs149622654. Meanwhile, for loci rs72548744 and rs73532215, LI tended to be located in the parietooccipital lobe with the variant homozygous genotype compared with the wild-type homozygous genotype. There were no significant differences in severity of LI among the three Cx43 genotypes. After FDR adjustment, SNPs of locus rs79597379, rs72548744, rs76430488/rs75464514, rs78125885, rs386705335, rs3840372, rs3805786/rs3805785/rs17083633, rs3805788, and rs149622654 (P = 0.023) and rs73532215 (P = 0.043) was still associated with cerebellar LI.
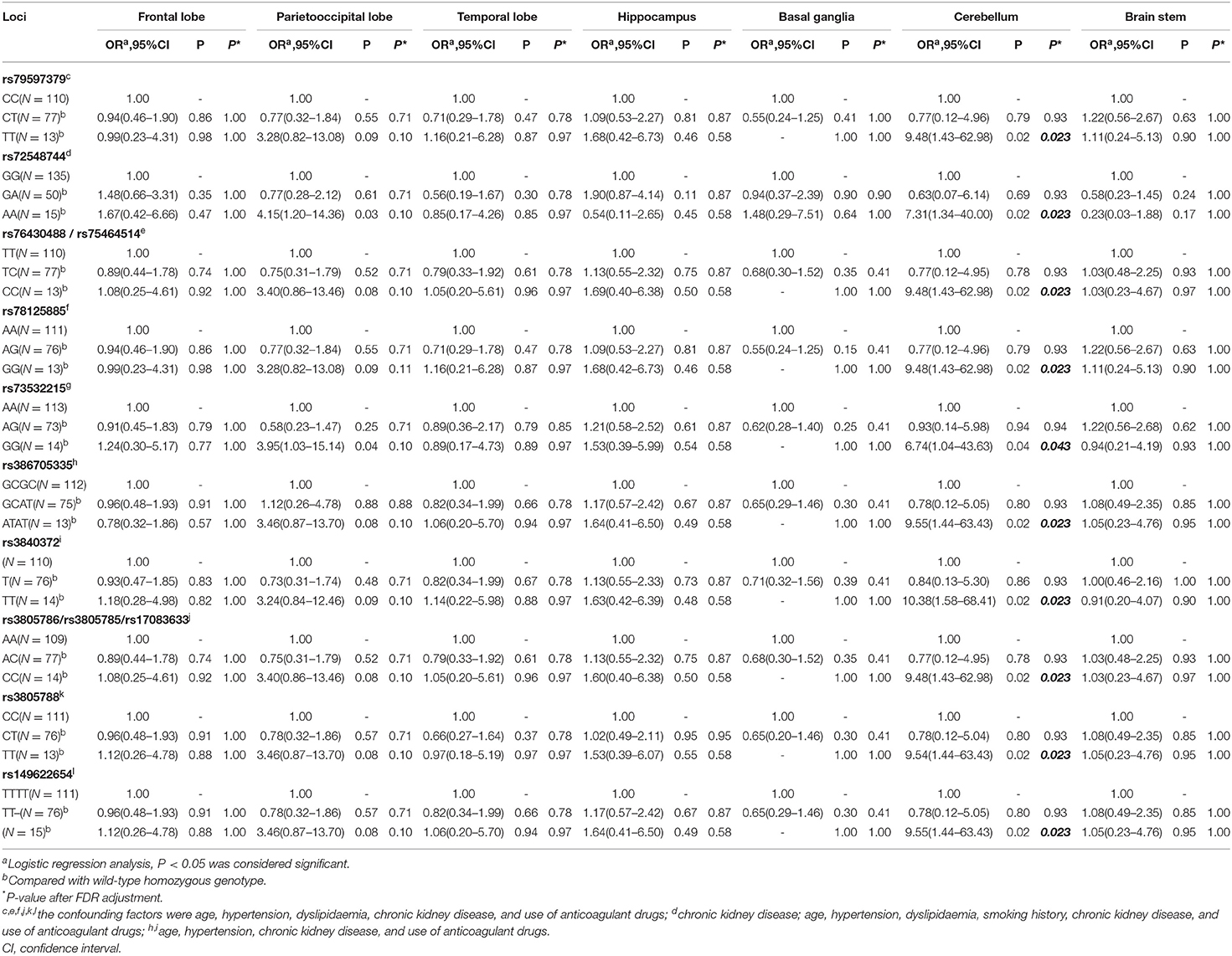
Table 4. Association of GJA1 gene SNPs and distribution of lacunar infarctions (multivariable analysis).
Correlation Between SNPs and CMB
Overall, 138 patients underwent brain MRI with T2*-weighted GRE. Similarly, the patients were classified into three groups based upon Cx43 genotype, and the severity and distribution of CMB compared among the three groups. Before adjusting for confounding factors, there were significant differences in lobar region distribution of CMB with loci rs76430488/rs75464514 and rs73532215 (P < 0.05). However, there were no significant differences in the severity of CMB (P > 0.05). Positive results are shown in Table 5.
After adjusting for confounding factors (multivariable analysis shown in Table 6), we found that CMB tended not to be located in the lobar region with the variant homozygous genotype compared with wild-type homozygous genotype at locus rs73532215 (P = 0.03, OR = 0.06, 95%CI, 0.01–0.75). There were no significant differences in the severity of CMB among the three genotypes. After FDR adjustment, SNP of locus 73532215 was still negative associated with lobar CMB (P = 0.04).
Thus, in summary, homozygosis of 13 (13/14, 92.9%) loci in the GJA1 gene were probably the risk factor for cerebellar LI. Homozygosis of one (1/14, 7.1%) loci was probably the protective factor of lobar CMB. There were SNPs of each loci that did not correlate with severity of WMH, LI, or CMB.
Functional Annotation of GJA1 Gene SNPs
In the GTEx database, there were no significant differences between Cx43 genotype and gene expression in brain tissue (P > 0.05) with very small size of 20 heterozygosis variation and 0 or 1 homozygosis variation. This suggests that gene expression of the GJA1 gene in brain tissue does not correlate with genotype. No evidence was detected for expression in cerebral vessels.
Data in the HaploReg and Regulome database are shown in Table 7. Annotation in HaploReg showed that SNPs of all 14 loci might influence gene expression of the GJA1 gene. Overall, 12 loci overlapped the promotor modifying zone of histone methylation and acetylation, 13 loci overlapped the enhancer modifying zone of histone, and 10 loci overlapped hypersensitive DNase sites (which can be cut by DNase, suggesting they are located in transcription factor active zones). By restricting the tissue to the brain or cerebral vessels, we still identified 11 loci overlapping the promotor modifying zone of histone methylation and acetylation, 10 loci overlapping the enhancer modifying zone of histone, and 3 loci overlapping hypersensitive DNase sites. Five loci were predicted to bind protein and 11 loci could cause motif changes. Predicting outcomes of RegulomeDB showed that loci rs3805786 and rs3805788 may influence the binding of transcription factors.
Discussion
Our study shows that SNPs of Cx43 correlate with the burden of CSVD. We focused on the impact of Cx43 in CSVD mainly because Cx43 is highly expressed in vascular ECs and ECs in the BBB, as well as astrocytes and pericytes forming the BBB (10). GJA4 (Cx37 coding gene) gene SNPs correlate with large vessel disease, such as ischemic stroke and coronary heart disease (27, 28). Similarly, GJA1 gene SNPs correlate with primary hypertension, congenital heart disease, and arrhythmia (18–20). Although there is no clear clinical evidence suggesting that GJA1 gene SNPs correlate with CSVD, its biodistribution supports this hypothesis. In the present study, we show that GJA1 gene SNPs mainly influence the distribution of LI and CMB lesions, with less effect on WMH.
GJA1 gene SNPs mostly influenced lesion distribution and there was no correlation with lesion severity. First, heterogeneity of spatial distribution may be caused by uneven BBB regulation in different brain areas (29). Using a mouse model, Villasenor found region-specific differences in permeability upon loss of pericytes, with marked increases in permeability in the cortex, hippocampus, and corpus striatum. This might be caused by region-specific intracellular information delivery (30). Therefore, the influence of Cx43 on localization of CSVD is probably because of differing regulation of information delivery leading to altered permeability in different brain regions. Second, specific spatial distribution might be caused by differences in anatomical structures. Zhao et al. found differing densities of blood capillaries in different brain regions, being highest in quadrigeminal bodies, followed by the thalamus, parietooccipital cortex, frontal cortex, hippocampus, corpus striatum, midbrain, and cerebellum. Densities in the pons and medulla were lowest (31). This suggests that region-specific permeability of the BBB might correlate with different expression of Cx. Third, different structures exhibit different susceptibility to BBB dysfunction. Using an osmotic demyelination syndrome model of mice, Bouchat et al. found that central nervous system demyelination might be caused by regional vulnerability of oligodendrocytes and astrocytes, and refuted BBB disruption as a primary cause of demyelination, especially in the thalamus and occipital cortex (32). Consequently, GJA1 gene SNPs might influence localization of CSVD via differing expression of Cx43 and differential regulation of information delivery in different brain regions. Our findings still need further experiments to investigate the expression of Cx43 mRNA and protein in the cerebellar region.
Cx43 might differentially influence multiple pathways of CSVD. Our research suggests that GJA1 gene SNPs mostly affect LI, followed by CMB. These opposing actions might be caused by different pathogenic pathways in various types of CSVD. Dysfunction of ECs leads to stenosis of cerebral vessels and acute ischemia, further promoting the likelihood of LI. Breakdown of the BBB causes leakage of plasma substances, while chronic hypoperfusion inhibits the generation and repair of myelin, thereby injuring the axon and causing WMH. CMB are caused by BBB breakdown or amyloid β (Aβ) deposition during cerebral amyloid angiopathy (CAA) (3, 33). Our findings suggest that GJA1 gene SNPs are mainly associated with EC pathways or Aβ deposition.
Our results suggest a key role of Cx43 in development of cranial lesions in CSVD. We also provide clinical evidence to support a critical function of Cx43 on ECs. Li et al. found that downregulation of Cx43 inhibited differentiation of endothelial progenitor cells and neointima formation, and ultimately inhibited vessel repair (11). Meanwhile, another study found that inhibition of Cx43 activity inhibited proliferation and migration by inhibiting extracellular signal-regulated kinase 1/2 (12). Further, Chen et al. showed that Cx43/Zo1 complex (specifically, a tight junction) maintained the balance of directional migration control to a more linear movement, which enhanced the rate of wound healing (34). In cell experiments, Saez et al. found that reduction of hemichannel activity may represent a strategy against the activation of deleterious pathways leading to endothelial dysfunction (16). When Cx43 activities are disrupted, regulation of EC function is lost, and LI occurs. Cx43 can also affect BBB function by both protein expression and opening of hemichannels. Ezan et al. found that decreased Cx43 expression weakened BBB function (13). Corroboratively, another two studies have shown that opening of Cx43 hemichannels can cause dysfunction of information delivery in the BBB by leakage of intracellular substances and ATP (17, 35). Subsequently, impairment of BBB permeability leads to WMH and CMB. Unfortunately, in our study, there is no correlation between GJA1 gene and WMH. It is worth noting that, the GG genotype of rs73532215 is negative associated with CMB. Yi et al. found that connexin43 gene knocking-out mice allowed to reduce oxidative stress and neuritic dystrophies in hippocampal neurons associated to Aβ plaques (36). This suggested that GG genotype of rs73532215 might reduce the impairment of vessels caused by Aβ deposition to lower the risk of lobar CMB. Large sample researches are needed.
In GTEx database, we found no significant differences between Cx43 genotype and gene expression in brain tissue. However, the sample size in GTEx database was small, the significance was limited. In HaploReg database, we found that 13 SNPs were proximal to known regulatory regions that might influence gene expression of the GJA1 gene. Large sample size and further experiments in vivo and vitro are needed.
Conclusion
In summary, we have shown that GJA1 gene SNPs correlate with MRI features of CSVD. GJA1 gene SNPs mainly influence the distribution of LI in cerebellum, a single locus might be a protective factor for lobar CMB. Thus, the GJA1 gene might regulate the distribution of CSVD to some extent. Our study provides the first clinical evidence of an association between GJA1 gene SNPs and CSVD. Based on previous experiments, we further show that Cx43 correlates with clinical destruction of EC function. Moreover, we investigated region-specific differences among different genotypes of GJA1 gene SNPs and show the impact of Cx43 on spatial heterogeneity of CSVD.
Data Availability Statement
The original contributions presented in the study are publicly available. This data can be found at: www.ncbi.nlm.nih.gov, PRJNA672247.
Ethics Statement
The studies involving human participants were reviewed and approved by the Ethics Committee of Peking University (PU IRB), No. IRB00001052-17018. The patients/participants provided their written informed consent to participate in this study. Written informed consent was obtained from the individual(s) for the publication of any potentially identifiable images or data included in this article.
Author Contributions
JZ participated in data collection, performed the imaging evaluation and statistical analysis, interpreted the results, and drafted the manuscript. QY and WS participated in data collection in Beijing. JS and HJ contributed to the statistical analysis. QG and MY provided guidance and advice for genetic analysis. JS, WZ, and YH were involved in design of the study, supervised the data collection process, analysis, and interpretation of data, and revised the manuscript for intellectual content. All authors contributed to the article and approved the submitted version.
Funding
This research was funded by the University of Ulm and Peking University Health Science Center (ULM–PUHSC) Joint Institute for Translational and Clinical Research (Grant No. PKU2017ZC001-5) and Natural Science Funds for Cluster Tibet Aid in Tibet Autonomous Region (XZ2017ZR-ZY020).
Conflict of Interest
The authors declare that the research was conducted in the absence of any commercial or financial relationships that could be construed as a potential conflict of interest.
Acknowledgments
We thank Rachel James, Ph.D., from Liwen Bianji, Edanz Group China (www.liwenbianji.cn/ac), for editing the English text of a draft of this manuscript.
Supplementary Material
The Supplementary Material for this article can be found online at: https://www.frontiersin.org/articles/10.3389/fneur.2020.583974/full#supplementary-material
References
1. Pantoni L. Cerebral small vessel disease-from pathogenesis and clinical characteristics to therapeutic challenge. Lancet Neurol. (2010) 9:689–701. doi: 10.1016/S1474-4422(10)70104-6
2. Wardlaw JM, Smith EE, Biessels GJ, Cordonnier C, Fazekas F, Frayne R, et al. Neuroimaging standards for research into small vessel disease and its contribution to ageing and neurodegeneration. Lancet Neurol. (2013) 12:822–38. doi: 10.1016/s1474-4422(13)70124-8
3. Giau VV, Bagyinszky E, Youn YC, An SSA, Kim SY. Genetic factors of cerebral small vessel disease and their potential clinical outcome. Int J Mol Sci. (2019) 20:4298. doi: 10.3390/ijms20174298
4. Schirmer MD, Giese AK, Fotiadis P, Etherton MR, Cloonan L, Viswanathan A, et al. Spatial signature of white matter hyperintensities in stroke patients. Front Neurol. (2019) 10:208. doi: 10.3389/fneur.2019.00208
5. Wardlaw JM, Smith C, Dichgans M. Small vessel disease: mechanisms and clinical implications. Lancet Neurol. (2019) 18:684–96. doi: 10.1016/s1474-4422(19)30079-1
6. Rajani RM, Quick S, Ruigrok SR, Graham D. Reversal of endothelial dysfunction reduces white matter vulnerability in cerebral small vessel disease in rats. Sci Transl Med. (2018) 10:eaam9507. doi: 10.1126/scitranslmed.aam9507
7. Cannistraro RJ, Badi M, Eidelman BH, Dickson DW, Middlebrooks EH, Meschia JF. CNS small vessel disease: a clinical review. Neurology. (2019) 92:1146–56. doi: 10.1212/WNL.0000000000007654
8. Bechmann I, Galea I, Perry VH. What is the blood-brain barrier (not)? Trends Immunol. (2007) 28:5–11. doi: 10.1016/j.it.2006.11.007
9. Lippoldtb HWA. Tight junctions of the blood–brain barrier-development, composition and regulation. Vascul Pharmacol. (2002) 38:323–37. doi: 10.1016/s1537-1891(02)00200-8
10. Willebrords J, Crespo Yanguas S, Maes M, Decrock E, Wang N, Leybaert L, et al. Connexins and their channels in inflammation. Crit Rev Biochem Mol Biol. (2016) 51:413–39. doi: 10.1080/10409238.2016.1204980
11. Li L, Liu H, Xu C, Deng M, Song M, Yu X, et al. VEGF promotes endothelial progenitor cell differentiation and vascular repair through connexin 43. Stem Cell Res Ther. (2017) 8:237. doi: 10.1186/s13287-017-0684-1
12. Arshad M, Conzelmann C, Riaz MA, Noll T, Gündüz D. Inhibition of Cx43 attenuates ERK1:2 activation, enhances the expression of Cav-1 and suppresses cell proliferation. Int J Mol Med. (2018) 42:2811–18. doi: 10.3892/ijmm.2018.3828
13. Ezan P, Andre P, Cisternino S, Saubamea B, Boulay AC, Doutremer S, et al. Deletion of astroglial connexins weakens the blood-brain barrier. J Cereb Blood Flow Metab. (2012) 32:1457–67. doi: 10.1038/jcbfm.2012.45
14. Nielsen S, Nagelhus EA, Amiry-Moghaddam M, Bourque C. Specialized membrane domains for water transport in glial cells- high-resolution immunogold cytochemistry of aquaporin-4 in rat brain. J Neurosci. (1997) 17:171–80.
15. Retamal MA, Froger N, Palacios-Prado N, Ezan P, Saez PJ, Saez JC, et al. Cx43 hemichannels and gap junction channels in astrocytes are regulated oppositely by proinflammatory cytokines released from activated microglia. J Neurosci. (2007) 27:13781–92. doi: 10.1523/JNEUROSCI.2042-07.2007
16. Saez JC, Contreras-Duarte S, Gomez GI, Labra VC, Santibanez CAR. Gajardo-Gomez, et al. Connexin 43 hemichannel activity promoted by pro-inflammatory cytokines and high glucose alters endothelial cell function. Front Immunol. (2018) 9:1899. doi: 10.3389/fimmu.2018.01899
17. Kaneko Y, Tachikawa M, Akaogi R, Fujimoto K, Ishibashi M, Uchida Y, et al. Contribution of pannexin 1 and connexin 43 hemichannels to extracellular calcium-dependent transport dynamics in human blood-brain barrier endothelial cells. J Pharmacol Exp Ther. (2015) 353:192–200. doi: 10.1124/jpet.114.220210
18. Wang LJ, Zhang WW, Zhang L, Shi WY, Wang YZ, Ma KT, et al. Association of connexin gene polymorphism with essential hypertension in Kazak and Han Chinese in Xinjiang, China. J Huazhong Univ Sci Technolog Med Sci. (2017) 37:197–203. doi: 10.1007/s11596-017-1715-y
19. Gu R, Sheng W, Ma X, Huang G. Association of Cx43 rs2071166 polymorphism with an increased risk for atrial septal defect. Cardiol Young. (2018) 28:397–402. doi: 10.1017/S1047951117002001
20. Wang P, Qin W, Wang P, Huang Y, Liu Y, Zhang R, et al. Genomic variants in NEURL, GJA1 and CUX2 significantly increase genetic susceptibility to atrial fibrillation. Sci Rep. (2018) 8:3297. doi: 10.1038/s41598-018-21611-7
21. Wahlund LO, Barkhof F, Fazekas F, Bronge L. A new rating scale for age-related white matter changes applicable to MRI and CT. Stroke. (2001) 32:1318–22. doi: 10.1161/01.str.32.6.1318
22. Ederle J, Davagnanam I, van der Worp HB, Venables GS, Lyrer PA, Featherstone RL, et al. Effect of white-matter lesions on the risk of periprocedural stroke after carotid artery stenting versus endarterectomy in the International Carotid Stenting Study (ICSS): a prespecified analysis of data from a randomised trial. Lancet Neurol. (2013) 12:866–72. doi: 10.1016/s1474-4422(13)70135-2
23. Gregoire SM, Chaudhary UJ, Brown MM, Yousry TA, Kallis C, Jäger HR, et al. The Microbleed Anatomical Rating Scale (MARS):Reliability of a tool to map brain microbleeds. Neurology. (2009) 73:1759–66. doi: 10.1212/WNL.0b013e3181c34a7d
24. Lioutas V.-A, Goyal N, Katsanos AH, Krogias C. Microbleed prevalence and burden in anticoagulant associated intracerebral bleed. Ann Clin Trans Neurol. (2019) 6:1546–51. doi: 10.1002/acn3.50834
25. Genomes Project C, Abecasis GR, Altshuler D, Auton A, Brooks LD, Durbin RM, et al. A map of human genome variation from population-scale sequencing. Nature. (2010) 467:1061–73. doi: 10.1038/nature09534
26. Clarke GM, Anderson CA, Pettersson FH, Cardon LR, Morris AP, Zondervan KT. Basic statistical analysis in genetic case-control studies. Nat Protoc. (2011) 6:121–33. doi: 10.1038/nprot.2010.182
27. Li H, Yu S, Wang R, Sun Z, Zhou X, Zheng L, et al. Polymorphism of connexin37 gene is a risk factor for ischemic stroke in Han Chinese population. Lipids Health Dis. (2018) 17:72. doi: 10.1186/s12944-018-0727-3
28. Wen D, Du X, Nie SP, Dong JZ, Ma CS. Association of connexin37 C1019T with myocardial infarction and coronary artery disease: a meta-analysis. Exp Gerontol. (2014) 58:203–7. doi: 10.1016/j.exger.2014.06.011
29. Noumbissi ME, Galasso B, Stins MF. Brain vascular heterogeneity: implications for disease pathogenesis and design of in vitro blood-brain barrier models. Fluids Barriers CNS. (2018) 15:12. doi: 10.1186/s12987-018-0097-2
30. Villasenor R, Kuennecke B, Ozmen L, Ammann M, Kugler CF, Gruninger, et al. Region-specific permeability of the blood-brain barrier upon pericyte loss. J Cereb Blood Flow Metab. (2017) 37:3683–94. doi: 10.1177/0271678X17697340
31. Zhao R, Pollack GM. Regional differences in capillary density, perfusion rate, and P-glycoprotein activity: a quantitative analysis of regional drug exposure in the brain. Biochem Pharmacol. (2009) 78:1052–9. doi: 10.1016/j.bcp.2009.06.001
32. Bouchat J, Couturier B, Marneffe C, Gankam-Kengne F, Balau B, De Swert K, et al. Regional oligodendrocytopathy and astrocytopathy precede myelin loss and blood-brain barrier disruption in a murine model of osmotic demyelination syndrome. Glia. (2018) 66:606–22. doi: 10.1002/glia.23268
33. Petrault M, Casolla B, Ouk T, Cordonnier C, Berezowski V. Cerebral microbleeds: beyond the macroscope. Int J Stroke. (2019) 14:468–75. doi: 10.1177/1747493019830594
34. Chen C.-H, Mayo JN, Gourdie RG, Johnstone SR. The connexin 43:ZO-1 complex regulates cerebral endothelial F-actin architecture and migration. Am J Physiol Cell Physiol. (2015) 309:C600–7. doi: 10.1152/ajpcell.00155.2015
35. Tachikawa M, Murakami K, Akaogi R, Akanuma SI, Terasaki T, Hosoya KI. Polarized hemichannel opening of pannexin 1/connexin 43 contributes to dysregulation of transport function in blood-brain barrier endothelial cells. Neurochem Int. (2020) 132:104600. doi: 10.1016/j.neuint.2019.104600
Keywords: CSVD, distribution, MRI, connexins, gene polymorphism
Citation: Zhang J, You Q, Shu J, Gang Q, Jin H, Yu M, Sun W, Zhang W and Huang Y (2020) GJA1 Gene Polymorphisms and Topographic Distribution of Cranial MRI Lesions in Cerebral Small Vessel Disease. Front. Neurol. 11:583974. doi: 10.3389/fneur.2020.583974
Received: 16 July 2020; Accepted: 02 November 2020;
Published: 25 November 2020.
Edited by:
Giorgio B. Boncoraglio, Carlo Besta Neurological Institute (IRCCS), ItalyReviewed by:
Ganesh Chauhan, Indian Institute of Science, IndiaJohnathan Cooper-Knock, The University of Sheffield, United Kingdom
Copyright © 2020 Zhang, You, Shu, Gang, Jin, Yu, Sun, Zhang and Huang. This is an open-access article distributed under the terms of the Creative Commons Attribution License (CC BY). The use, distribution or reproduction in other forums is permitted, provided the original author(s) and the copyright owner(s) are credited and that the original publication in this journal is cited, in accordance with accepted academic practice. No use, distribution or reproduction is permitted which does not comply with these terms.
*Correspondence: Wei Zhang, neurozw@163.com