- 1Instituto do Cancer do Estado de São Paulo, Hospital das Clínicas da Faculdade de Medicina da Universidade de São Paulo, São Paulo, Brazil
- 2LIM-62/Division of Neurosurgery, Department of Neurology, Faculdade de Medicina da Universidade de São Paulo, São Paulo, Brazil
- 3Service of Interdisciplinary Neuromodulation, Instituto de Psiquiatria do Hospital das Clínicas da Faculdade de Medicina da Universidade de São Paulo, São Paulo, Brazil
- 4School of Medicine - Universidade da Cidade de São Paulo UNICID, São Paulo, Brazil
Background: This prospective study aimed to evaluate the cortical excitability (CE) of patients with brain tumors surrounding or directly involving the corticospinal tract (CST) using navigated transcranial magnetic stimulation (nTMS).
Methods: We recruited 40 patients with a single brain tumor surrounding or directly involving the CST as well as 82 age- and sex-matched healthy controls. The patients underwent standard nTMS and CE evaluations. Single and paired pulses were applied to the primary motor area (M1) of both affected and unaffected cerebral hemispheres 1 week before surgery. The CE parameters included resting motor threshold (RMT), motor evoked potential (MEP) ratio for 140 and 120% stimulus (MEP 140/120 ratio), short-interval intracortical inhibition (SICI), and intracortical facilitation (ICF). Motor outcome was evaluated on hospital discharge and on 30-day and 90-day postoperative follow-up.
Results: In the affected hemispheres of patients, SICI and ICF were significantly higher than in the unaffected hemispheres (p=0.002 and p=0.009, respectively). The 140/120 MEP ratio of patients' unaffected hemispheres was lower than that in controls (p=0.001). Patients with glioblastomas (GBM) had a higher interhemispheric RMT ratio than patients with grade II and III gliomas (p = 0.018). A weak correlation was observed among the RMT ratio and the preoperative motor score (R2 = 0.118, p = 0.017) and the 90-day follow-up (R2 = 0.227, p = 0.016).
Conclusion: Using preoperative nTMS, we found that brain hemispheres affected by tumors had abnormal CE and that patients with GBM had a distinct pattern of CE. These findings suggest that tumor biological behavior might play a role in CE changes.
Introduction
Developed in 1985 by Barker and colleagues (1), transcranial magnetic stimulation (TMS) is a non-invasive, economical, accurate, and well-tolerated method of adjuvant intervention utilized in various neuropsychiatric disorders including major depression (2), Alzheimer's disease (3), diffuse axonal injury (4, 5), schizophrenia (6), and anxiety (7). In neuro-oncology, navigated TMS (nTMS) has been useful in studying electrophysiology in patients with tumors located in eloquent areas to assess motor tract integrity. Brain mapping with nTMS has been associated with a decreased risk of new postoperative neurological deficits and an increased extent of resection (EOR) (8), which are essential for achieving better progression-free survival and quality of life (9, 10).
It has been suggested that preoperative nTMS results could be used as a predictor of motor outcome in patients with lesions involving the primary motor cortex (M1) and corticospinal tract (CST). For example, an abnormal interhemispheric resting motor threshold (RMT) ratio was found to be a high-risk criterion for early poor postoperative motor outcome (7 days), but not for late outcome (3 months) (11). Recent reports also indicate the correlation of the absence of intraoperative motor evoked potential (MEP), detected by postoperative nTMS, with poor motor prognosis (12). In addition, several parameters of cortical excitability (CE), such as short-interval intracortical inhibition (SICI) and intracortical facilitation (ICF), have been described in patients with traumatic brain injury (4) and stroke (13); however, they have not been evaluated in patients with brain tumors involving the M1. Further, the association of abnormal values obtained by nTMS with motor dysfunction is not yet clear.
The aim of the current study was to characterize the CE of patients with brain tumors surrounding the rolandic area and to compare it with those of healthy controls. This would aid in the understanding of how neoplasm behavior affects the neurophysiology of the perilesional motor cortex, using preoperative nTMS.
Methods
Setting
For this exploratory prospective study, we recruited 40 adult patients (age ≥18 years old), both genders, with a single brain tumor surrounding or directly involving the CST—a convenience sample—and 82 age- and gender-matched healthy controls. All participants underwent nTMS and CE evaluations at a tertiary referral hospital of São Paulo, Brazil.
Preoperative Clinical Evaluation
Muscle strength and performance scales were assessed preoperatively, at hospital discharge, and 30-day and 90-day postoperatively. Motor score was defined as upper plus lower extremity strengths of each hemibody according to the Medical Research Council (MRC) (14, 15) grade scale, with 0 indicating no muscle activation and five indicating total muscle strength. Performance status was evaluated using the Karnofsky Performance Scale (KPS) (16). Although the use of antiepileptic drugs (AED) and antidepressants had been previously associated with alterations in neuroexcitability, these drugs could not be withdrawn before nTMS sessions. Instead, we studied the interference of these drugs on CE.
Brain Tumor Management
Brain tumor diagnosis was established based on clinical history, preoperative magnetic resonance imaging (MRI) analysis, and histopathologic study of each lesion, as per the latest World Health Organization Classification of Tumors of the Central Nervous System (17). Surgical resection was aimed at achieving the best possible EOR.
We used an axial T2-weighted fluid attenuated inversion recovery (FLAIR) MRI sequence to assess the distance (mm) between cortical lesions and the posterior border of the “omega,” correspondent to the area of the hand on the pre-central gyrus (M1). For subcortical lesions, we calculated the distance between the lesion and the posterior limb of the internal capsule.
nTMS Evaluation
Up to 1 week before surgery, neuronavigation was performed using a frameless stereotaxic system, combining preoperative structural MRI and a sensor-based navigation system (Brainsight TMS version 1.7, Canada) for the guidance of coil placement and visualization of the angle of impact for the magnetic impulse onto the cortical surface (18, 19). Both single- and paired-pulse TMS were applied to M1 of affected and unaffected hemispheres using a circular coil connected to an offline electromyography amplifier of a one-channel, three-surface electrode output (Magventure Tonika Elecktronic, Denmark). The MEP response curve amplitudes were recorded in microvolts (μV) for the first interosseous muscle of the contralateral hand. All evaluations were performed by the same examiner.
CE assessed RMT (%), defined as the lowest stimulus provoking a MEP of at least 50 μV in five out of 10 consecutive trials using single-pulse TMS (20). To assess the amplitude of the input/output curve, we used the MEP obtained with 120 and 140% of RMT stimulus, the most varied range of this curve, and calculated the MEP 140/120 ratio (21–24). With the conditioning stimulus set at 80% of RMT and the test stimulus set at 120% of RMT, we applied paired-pulse TMS and measured SICI by taking the ratio between the amplitude of MEP response curves at 2 and 4 ms inter-stimulus intervals (ISI), while the ICF ratio was calculated taking the ratio between the amplitudes of MEP response curves at ISI 10 ms and 15 ms, for each hemisphere (4, 21, 25). All parameters were classified as low, normal, or high, based on normative values obtained by Cueva et al. (25). The ratios between affected and unaffected hemispheres for each parameter were calculated, considering the normal reference range as 90–110%.
Ethical Standard
This study was approved by the Ethics and Research Committee of the University of São Paulo Medical School, and all individuals provided written informed consent, following the Declaration of Helsinki guidelines.
Statistical Analysis
Continuous variable normality was verified using the asymmetry and kurtosis values. We performed a Wilcoxon test to compare CE between the affected and unaffected hemispheres. Additionally, to compare the patients with controls, we calculated the mean scores for the controls' hemispheres and compared them with scores for both affected and unaffected hemispheres of all patients using the Mann–Whitney U test. Comparisons among the subgroups of patients according to brain tumor histopathology diagnosis (primary central nervous system [CNS] tumor vs. metastasis; World Health Organization [WHO] grade II and III gliomas vs. glioblastomas [GBMs]) were performed using the Mann–Whitney test. We studied the association between motor score and neurophysiological parameters using Spearman's correlation coefficients for quantitative data (absolute value) and Pearson's Chi-square test for qualitative data: classification normal × altered (high + low). Finally, we compared pre- and postoperative muscle strength and KPS using ANOVA for repeated measures. The analyses were performed using the Statistical Package for Social Sciences, version 24.0 (IBM Statistics, Armonk, New York, USA). The data were considered significant when p was < 0.05.
Results
Forty patients underwent nTMS analysis. One patient underwent a new nTMS session before undergoing an additional surgery for recurrent GBM resection a year after the first resection, totalizing 41 CE evaluations. The general characteristics of the patients are presented in Table 1. The mean age of the patients was 50.00 ± 15.34 years, 15 females and 25 males. Similarly, the control group had a mean age of 49.72 ± 15.37 (33 females and 50 males). The mean preoperative KPS score was 83.90 ± 13.01 (range, 50–100). The frequent clinical manifestations that were observed included seizures (27 patients, 73.0%) and hemiparesis (10 patients, 31.3%). The median motor score was 10 (8–10). Around 44% of the patients were taking dexamethasone and 80% antiepileptic drugs when submitted to nTMS session. Three patients were using antidepressants, and only one was using a neuroleptic drug at the time of nTMS session. Thirty-one patients presented cortical tumors while nine patients had subcortical lesions. Thirty-five patients had primary CNS tumors, 23 patients had WHO grade II or III gliomas, 11 had GBMs, and six had secondary brain tumors (originating from the lungs, skin, and gastrointestinal tract).
Assessing CE, we found that SICI and ICF values were significantly higher in the patients' affected hemispheres than in the unaffected hemispheres (1.12 ± 0.60 vs. 0.80 ± 0.59, p = 0.002; 2.30 ± 1.14 vs. 1.83 ± 1.20, p = 0.009, respectively; Table 2, Figure 1). RMT and MEP interhemispheric ratios exhibited normal distributions in patients, while SICI and ICF interhemispheric ratios had significant interindividual variations. We observed a high frequency of altered (outside the 90–110% range) interhemispheric ratios in the group of patients: 51% of patients had abnormal RMT ratio; 89%, MEP 140/120 ratio; 86%, ICF ratio; and 94%, SICI ratio.
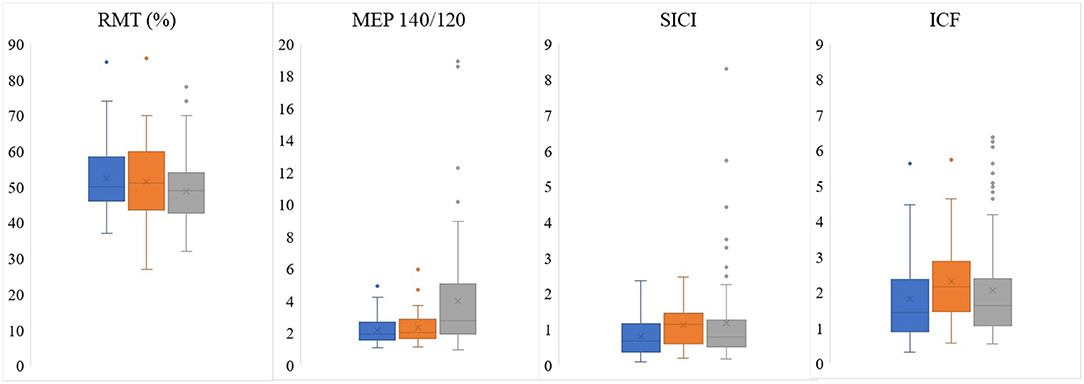
Figure 1. Comparison of electrophysiological parameters obtained by preoperative TMS between unaffected (blue) and affected (orange) patients' hemispheres and the mean between controls' hemispheres (gray). RMT, resting motor threshold; MEP, motor evoked potential; SICI, short-interval intracortical inhibition; ICF, intracortical facilitation.
When the patients were compared to the controls, it was found the MEP 140/120 ratio was lower in patients' both unaffected and affected hemispheres than in those of the controls (3.98 ± 3.41 vs. 2.15 ± 0.86, p = 0.001; 3.98 ± 3.41 vs. 2.33 ± 1.04, p = 0.008, respectively; Table 2, Figure 1).
The use of antidepressants was not associated with a different CE pattern. Preoperative use of AED seemed not to significantly influence CE in the total population. However, when we studied only the subgroup with CNS tumors, patients who used antiepileptic drugs had significant lower ratio MEP 140/120 and ICF in the affected hemisphere (2.14 vs. 3.54 for MEP ratio, p = 0.045, 2.21 vs. 3.42 for ICF, p = 0.022, respectively, Supplementary Material 1).
Preoperative clinical and neurophysiologic data of each patient are detailed in Table 3. Thirty-one patients presented abnormal RMT on the unaffected hemisphere and 33 patients presented abnormal RMT on the affected hemisphere. For the other CE parameters, altered MEP 140/120 ratio, SICI, and ICF were more frequent on unaffected hemisphere than the affected one (30 vs. 27 for MEP140/120 ratio, 31 vs. 29 for SICI, and 32 vs. 25 patients for ICF, respectively).
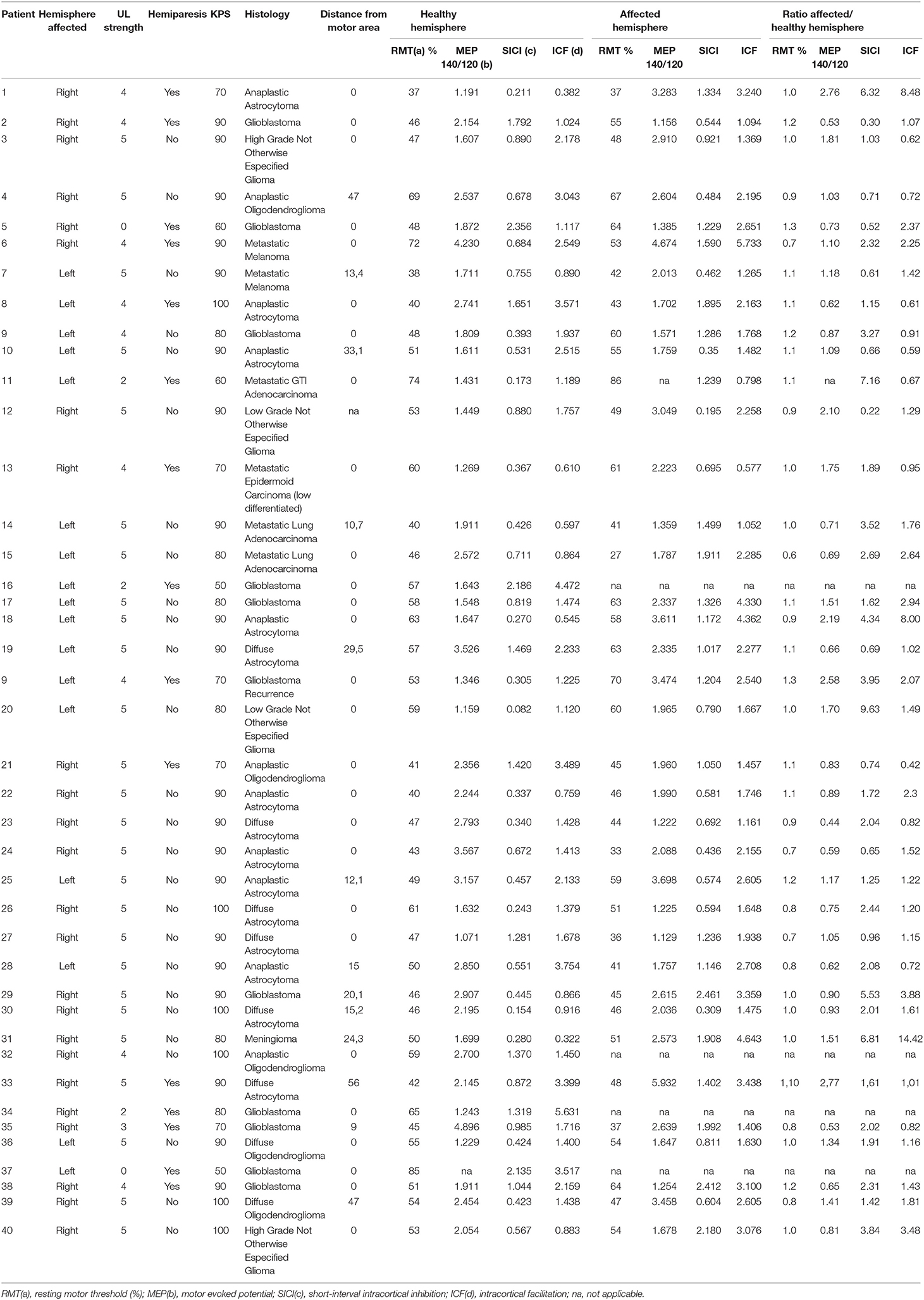
Table 3. Preoperative clinical presentation and cortical excitability obtained by navigated transcranial magnetic stimulation of the patients.
When we compared patients according to the presence of hemiparesis, the only difference found was a higher SICI in the unaffected hemisphere of patients with hemiparesis (p = 0.013). However, there was no difference in interhemispheric SICI ratio (Table 4). Comparing the subgroups of patients according to their histopathological diagnoses revealed no significant difference in CE between patients with primary and secondary tumors. However, comparisons of patients with GBMs with patients with WHO grade II and III gliomas indicated that patients with GBMs had a higher interhemispheric RMT ratio (p = 0.018; Table 4). Table 5 shows a detailed analysis of CE in each group of patients, according to their tumor diagnosis.
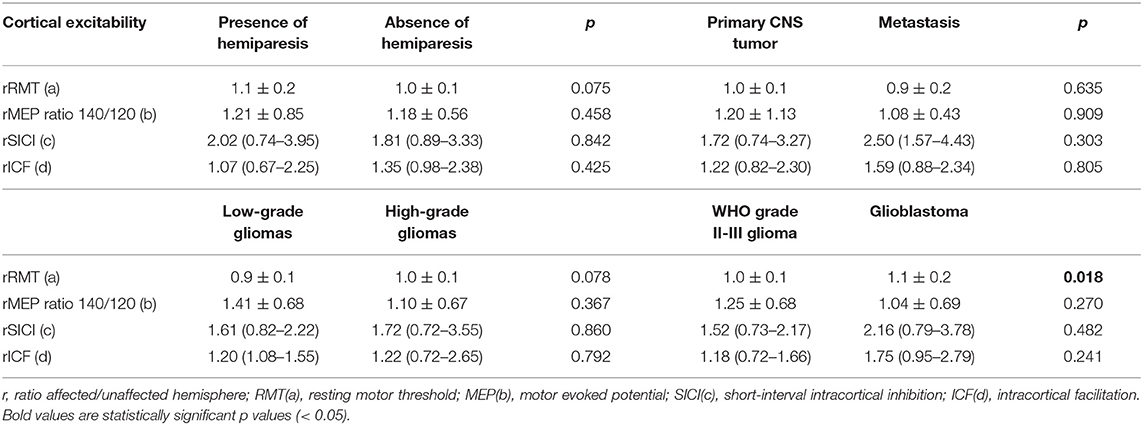
Table 4. Ratio affected/unaffected hemisphere according to the presence of hemiparesis and histology.
We compared the preoperative and the three postoperative motor evaluations. Patients presented the highest motor score at the preoperative moment and the lowest at the hospital discharge (Table 6, p = 0.030). A weak correlation was observed among the RMT ratio and the preoperative motor score (R2 = 0.118, p = 0.017), and the 90-day follow-up (R2 = 0.227, p = 0.016), and between unaffected hemisphere SICI and the pre- and postoperative motor scores (R2 = 0.255, p = 0.009 for preoperative motor score, R2 = 0.271, p = 0.018 for hospital discharge, R2 = 0.321, p = 0.013 for 30-day follow-up, and R2 = 0.396, p = 0.059 for 90-day follow-up, Table 5). However, preoperative RMT ratio and unaffected hemisphere SICI were not associated with motor score change (p = 0.938 for RMT and p = 0.470 for SICI, ANOVA for repeated measures, Figure 2).
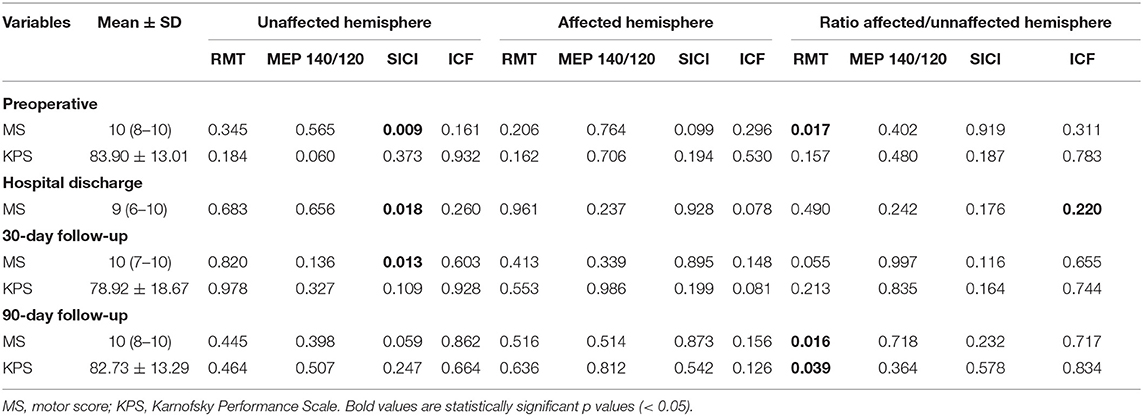
Table 6. Correlation of spearman between cortical excitability and motor and performance scale outcomes.
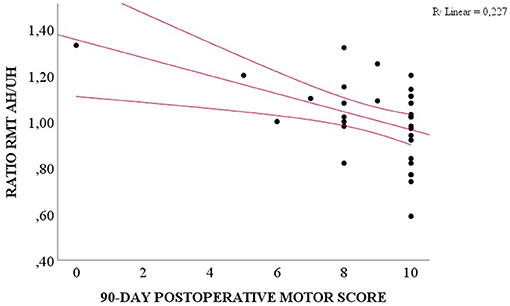
Figure 2. Correlation between preoperative RMT ratio AH/UH and 90-day postoperative motor score (Spearman, p = 0.016).
A correlation was observed between distance (in millimeters) from motor area on MRI and the MEP 140/120 ratio of both hemispheres (p = 0.030, R2 = 0.348 in the unaffected hemisphere and p = 0.032, R2 = 0.363 in the affected hemisphere).
Discussion
This study contributes to the knowledge about the neurophysiology of patients with tumors within M1. It evaluates distinct parameters that have been previously reported, RMT and MEP, as well as describes the values of SICI and ICF for the first time. The first two parameters refer to the integrity of both upper and lower motor neurons, from the cerebral cortex to the neuromuscular junction. RMT was similar between patients' hemispheres, which contradicts previous studies that reported higher RMT in the hemisphere affected by the tumor (26). SICI and ICF, the only two parameters found to be significantly higher in patients' affected hemispheres, are exclusively mediated by circuits located in the cortex (27, 28) and, therefore, have a higher specificity for cortical alterations than RMT and MEP. SICI is mediated by GABAA receptors, which are ligand-gated ion channels, while long-interval intracortical inhibition is associated with GABAB. These are G-protein-coupled receptors and are, therefore, slower than GABAA (28–30). Varrasi et al. found abnormal intracortical inhibition in patients with partial epilepsy, which was attributed to weakness of the GABA receptors, thereby provoking an imbalance between excitatory and inhibitory circuits (31). SICI was also found to be reduced in movement disorders, such as dystonia (32) and Tourette's syndrome (33). Conversely, ICF is mediated by glutamate and is associated with excitatory cortical circuits (34). SICI was found to be lower with a concomitant increase in ICF values in patients with Parkinson's disease (35). In our study, the patients' unaffected hemisphere's excitability was lower compared to that of the controls and compared to the affected hemisphere. Since observations of significant interhemispheric differences in healthy individuals are unexpected (36), the differences found in our study might be associated with dysfunction of motor neurons in the patients' hemispheres affected by the tumor as well as an interhemispheric imbalance between excitatory and inhibitory circuits, which might be related to the greater prevalence of preoperative seizures in our study (73%). The mechanism by which the CE parameters of the contralateral hemisphere is altered as well is still unclear.
It has been reported that the use of antiepileptic, neuroleptic, and antidepressant drugs can affect neuroexcitability (37–41). Voltage-gated sodium channels blockers, such as phenytoin, carbamazepine, and lamotrigine, were previously found to increase motor threshold (41, 42), with carbamazepine associated with decreased ICF (42). In our series, the influence of AED on CE was only observed in the subgroup of patients with CNS tumors, composed of 29 patients who used AED and 6 patients who did not use it. Although the findings of lower ratio MEP 140/120 and lower ICF in the group using AED might agree with previous studies, they also might reflect a type 1 error.
It has been reported that MEP may have high interindividual variability and that the interhemispheric ratio has a more reliable value in assessing CE (26). In our sample, we found high rates of abnormal ratios (51% for RMT, 89% for MEP 140/120 ratio, 86% for ICF, and a remarkable 94% for SICI). However, only half of the patients presented with motor deficits, which led us to two main hypotheses: The first is that the alteration of these values may coexist with a normal motor function because tumor growth is not an acute process, requiring some time to progressively affect the tissue surrounding it. This conclusion applies especially to patients with low-grade gliomas, which have a relatively slower evolution, giving the unaffected brain some time to try to compensate by neuroplasticity. The second hypothesis is that pathologic neurophysiology may predict a poor motor outcome in these patients in the future. Picht et al. speculated that patients initially without hemiparesis but with high RMT and low MEP interhemispheric ratios were at a higher risk of a decline in motor function in the future (26). These authors also suggested that patients with no previous deficits but with high MEP ratios probably have a perilesional tissue more adapted to tumor growth. Additionally, they highlighted the finding that the patients had low RMT ratios, suggesting that in these individuals, tumors might have infiltrated the inhibitory tracts of the secondary motor cortex, thereby accounting for the lack of motor deficits (26). In our study, we found six patients [patients 2, 5, 9, 19, 22, 38] with simultaneously high RMT and low MEP interhemispheric ratios, two of whom had no motor deficits prior to the resection (19, 22). Four patients had worse motor scores during the follow-up (2, 5, 22, 38), one patient remained stable (9), and one of the two patients who initially did not have any motor deficit displayed normal motor function (19). This patient is also the only one in the subgroup with a low-grade glioma, as all the others were diagnosed with high-grade gliomas. These data are consistent with Picht et al.'s speculation about poor outcome prediction and our second hypothesis. In our study, 10 patients (3, 7, 12, 17, 18, 20, 25, 31, 36, 39) had high MEP ratios with normal preoperative motor status, and six remained with no motor deficit (7, 18, 20, 25, 31, 39), which again is consistent with the findings of Picht et al. and fits our first hypothesis of adaptation of the normal tissue. The direct correlation between greater tumor's distance from motor area and higher MEP also reinforces this hypothesis.
Lastly, we observed eight patients (6, 15, 24, 26–28, 35, 39) with a low RMT ratio, and, contrary to our expectations, six of them had a high SICI ratio (6, 15, 26, 28, 35, 39). Indeed, the only difference between patients concerning hemiparesis presentation was a lower SICI in patients without this motor deficit. Concerning clinical presentation, however, the presence of preoperative motor deficit was not associated with more CE abnormalities.
The idea that preoperative TMS findings might predict motor outcome is not new. Rosenstock et al. studied abnormal RMT interhemispheric ratio as one criterion for high risk of poor motor outcome and found that a high RMT ratio was associated with worst motor score 7 days postoperatively, but that there was no association 3 months postoperatively (11). Therefore, more analysis is necessary to determine whether preoperative SICI and ICF are associated with presence of motor deficit at the time of CE evaluation and might predict patients' prognoses.
Tumor growth rate influences the surrounding cortex adaptation, and this could explain another finding of our study, the higher values of RMT ratio in patients with GBMs compared with patients with WHO grade II and III gliomas. It is well-known that GBM rapidly infiltrates parenchyma, hindering motor function recovery. Therefore, GBM affects CE in a way closer to the changes seen in acute/subacute brain injuries. In their meta-analysis, McDonnel et al. found that RMT was already higher in affected hemispheres at the early phase after stroke and continued to be altered during the chronic phase (13). As discussed previously, high RMT ratio might be a sign of a decline in motor function, even in those who do not have clinical manifestations.
This is an exploratory study whose findings contribute to the knowledge of how neuroexcitability might be affected by a tumor. However, some results require further studies to be well-understood. One of the limitations of our study is tumor heterogeneity: We included a majority of patients with gliomas (of both low and high grades and, therefore, different rates of normal tissue infiltration), a minority of patients with brain metastases (which typically provoke mass-effect alterations), and one patient with a grade I meningioma, an extra-axial tumor related to alterations due to the tumor's expansion. It is impressive that most CE parameters studied had a normal distribution considering different biological behaviors of different tumors. We had a glance on how CE in each subgroup of diagnosis is. However, focusing our attention on small subgroups increases the risk of a type 1 error. Therefore, these specific data should be considered only for descriptive purposes.
Another potential bias in the study is that the MEPs were only measured on the hands and not also on the lower extremities; more than 63% of patients presented no motor deficit or had a mild deficit preoperatively (motor score of 8–10), reflecting a possible selection bias and hindering the correlation of CE data with motor outcome. The final limitation is the lack of data on cognition and quality of life. Only minor adverse events were observed, such as light pinch on scalp and light headache during nTMS.
This study provides a detailed description of the CE of patients with tumors located in the eloquent areas of the brain. Brain hemispheres affected by tumors had abnormal CE, but further studies are needed to determine if CE is associated with loss of motor function integrity. GBMs showed a discrete pattern when compared with grade II and III gliomas, suggesting that tumor biological behavior might play a role in CE changes observed in patients with gliomas.
Data Availability Statement
The original contributions generated for this study are included in the article/Supplementary Material, further inquiries can be directed to the corresponding author/s.
Ethics Statement
The studies involving human participants were reviewed and approved by Comitê de Ética e Pesquisa (Ethics and Research Committee, CEP) of University of São Paulo Medical School. The patients/participants provided their written informed consent to participate in this study.
Author Contributions
IN, RG, DA, AB, MT, and WP: conception and design. IN: patients recruitment. IN, AG, CA, and CH: data collection. IN, AG, and DS: data analysis and interpretation. IN and AG: drafting the manuscript for important intellectual content. DS, RG, CH, DA, AB, MT, and WP: text review. IN, AG, CA, CH, DS, RG, DA, AB, MT, and WP: final approval. All authors contributed to the article and approved the submitted version.
Funding
This work was supported by Fundação de Amparo à Pesquisa do Estado de São Paulo (São Paulo Research Foundation—FAPESP) (scholarship funding to AGS # 2019/14687-4).
Conflict of Interest
The authors declare that the research was conducted in the absence of any commercial or financial relationships that could be construed as a potential conflict of interest.
Acknowledgments
We would like to acknowledge the support of the secretary Sandra Falcon from the Service of Interdisciplinary Neuromodulation for organizing patient schedule.
Supplementary Material
The Supplementary Material for this article can be found online at: https://www.frontiersin.org/articles/10.3389/fneur.2020.582262/full#supplementary-material
References
1. Barker AT, Jalinous R, Freeston I. Non-invasive magnetic stimulation of human motor cortex. Lancet. (1985) 1:1106–7. doi: 10.1016/S0140-6736(85)92413-4
2. Sonmez AI, Camsari DD, Nandakumar AL, Voort JLV, Kung S, Lewis CP, et al. Accelerated TMS for depression: a systematic review and meta-analysis. Psychiatry Res. (2019) 273:770–81. doi: 10.1016/j.psychres.2018.12.041
3. Dong X, Yan L, Huang L, Guan X, Dong C, Tao H, et al. Repetitive transcranial magnetic stimulation for the treatment of Alzheimer's disease: a systematic review and meta-analysis of randomized controlled trials. PLoS ONE. (2018) 13:1–13. doi: 10.1371/journal.pone.0205704
4. Hayashi CY, Neville IS, Rodrigues PA, Galhardoni R, Brunoni AR, Zaninotto AL. Altered intracortical inhibition in chronic traumatic diffuse axonal injury. Front Neurol. (2018) 9:1–8. doi: 10.3389/fneur.2018.00189
5. Neville IS, Zaninotto AL, Hayashi CY, Rodrigues PA, Galhardoni R, De Andrade DC. Repetitive TMS does not improve cognition in patients with TBI: a randomized double-blind trial. Neurology. (2019) 93:E190–9. doi: 10.1212/WNL.0000000000007748
6. Marzouk T, Winkelbeiner S, Azizi H, Malhotra AK, Homan P. Transcranial magnetic stimulation for positive symptoms in schizophrenia: a systematic review. Neuropsychobiology. (2020) 79:384–96. doi: 10.1159/000502148
7. Rodrigues PA, Zaninotto AL, Neville IS, Hayashi CY, Brunoni AR, Teixeira MJ. Transcranial magnetic stimulation for the treatment of anxiety disorder. Neuropsychiatr Dis Treat. (2019) 15:2743–61. doi: 10.2147/NDT.S201407
8. Raffa G, Scibilia A, Conti A, Ricciardo G, Rizzo V, Morelli A, et al. The role of navigated transcranial magnetic stimulation for surgery of motor-eloquent brain tumors: a systematic review and meta-analysis. Clin Neurol Neurosurg. (2019) 180:7–17. doi: 10.1016/j.clineuro.2019.03.003
9. Frey D, Schilt S, Strack V, Zdunczyk A, Sler JR, Niraula B, et al. Navigated transcranial magnetic stimulation improves the treatment outcome in patients with brain tumors in motor eloquent locations. Neuro Oncol. (2014) 16:1365–72. doi: 10.1093/neuonc/nou110
10. Picht T. Current and potential utility of transcranial magnetic stimulation in the diagnostics before brain tumor surgery. CNS Oncol. (2014) 3:299–310. doi: 10.2217/cns.14.25
11. Rosenstock T, Grittner U, Acker G, Schwarzer V, Kulchytska N, Vajkoczy P, et al. Risk stratification in motor area-related glioma surgery based on navigated transcranial magnetic stimulation data. J Neurosurg. (2017) 126:1227–37. doi: 10.3171/2016.4.JNS152896
12. Seidel K, Häni L, Lutz K, Zbinden C, Redmann A, Consuegra A, et al. Postoperative navigated transcranial magnetic stimulation to predict motor recovery after surgery of tumors in motor eloquent areas. Clin Neurophysiol. (2019) 130:952–9. doi: 10.1016/j.clinph.2019.03.015
13. McDonnell MN, Stinear CM. TMS measures of motor cortex function after stroke: a meta-analysis. Brain Stimul. (2017) 10:721–34. doi: 10.1016/j.brs.2017.03.008
14. Council MR. Aids to the Examination of Peripheral Nerves System. London: Memo no 45 Her Majesty's Station Off (1981).
15. Paternostro-Sluga T, Grim-Stieger M, Posch M, Schuhfried O, Vacariu G, Mittermaier C, et al. Reliability and validity of the Medical Research Council (MRC) scale and a modified scale for testing muscle strength in patients with radial palsy. J Rehabil Med. (2008) 40:665–71. doi: 10.2340/16501977-0235
16. Karnofsky AW, Craver LBJ. The use of nitrogen mustard in the palliative treatment of cancer. Cancer. (1948) I:634–56.
17. Louis DN, Perry A, Reifenberger G, von Deimling A, Figarella-Branger D, Cavenee WK, et al. The 2016 World Health Organization classification of tumors of the central nervous system: a summary. Acta Neuropathol. (2016) 131:803–20. doi: 10.1007/s00401-016-1545-1
18. Herwig U, Schönfeldt-Lecuona C, Wunderlich AP, Von Tiesenhausen C, Thielscher A, Walter H, et al. The navigation of transcranial magnetic stimulation. Psychiatry Res - Neuroimaging. (2001) 108:123–31. doi: 10.1016/S0925-4927(01)00121-4
19. Lefaucheur JP. Why image-guided navigation becomes essential in the practice of transcranial magnetic stimulation. Neurophysiol Clin. (2010) 40:1–5. doi: 10.1016/j.neucli.2009.10.004
20. Groppa S, Oliviero A, Eisen A, Quartarone A, Cohen LG, Mall V, et al. A practical guide to diagnostic transcranial magnetic stimulation: report of an IFCN committee. Clin Neurophysiol. (2012) 123:858–82. doi: 10.1016/j.clinph.2012.01.010
21. Lefaucheur JP, Drouot X, Ménard-Lefaucheur I, Keravel Y, Nguyen JP. Motor cortex rTMS restores defective intracortical inhibition in chronic neuropathic pain. Neurology. (2006) 67:1568–74. doi: 10.1212/01.wnl.0000242731.10074.3c
22. Han TR, Kim JH, Lim JY. Optimization of facilitation related to threshold in transcranial magnetic stimulation. Clin Neurophysiol. (2001) 112:593–9. doi: 10.1016/S1388-2457(01)00471-0
23. Davey NJ, Smith HC, Savic G, Maskill DW, Ellaway PH, Frankel HL. Comparison of input-output patterns in the corticospinal system of normal subjects and incomplete spinal cord injured patients. Exp Brain Res. (1999) 127:382–90. doi: 10.1007/s002210050806
24. Rossini PM, Burke D, Chen R, Cohen LG, Daskalakis Z, Di Iorio R, et al. Non-invasive electrical and magnetic stimulation of the brain, spinal cord, roots and peripheral nerves: basic principles and procedures for routine clinical and research application: an updated report from an I.F.C.N. Committee. Clin Neurophysiol. (2015) 126:1071–107. doi: 10.1016/j.clinph.2015.02.001
25. Cueva AS, Galhardoni R, Cury RG, Parravano DC, Correa G, Araujo H, et al. Normative data of cortical excitability measurements obtained by transcranial magnetic stimulation in healthy subjects. Neurophysiol Clin. (2016) 46:43–51. doi: 10.1016/j.neucli.2015.12.003
26. Picht T, Strack V, Schulz J, Zdunczyk A, Frey D, Schmidt S, et al. Assessing the functional status of the motor system in brain tumor patients using transcranial magnetic stimulation. Acta Neurochir (Wien). (2012) 154:2075–81. doi: 10.1007/s00701-012-1494-y
27. Di Lazzaro V, Restuccia D, Oliviero A, Profice P, Ferrara L, Insola A, et al. Magnetic transcranial stimulation at intensities below active motor threshold activates intracortical inhibitory circuits. Exp Brain Res. (1998) 119:265–8. doi: 10.1007/s002210050341
28. Nakamura H, Kitagawa H, Kawaguchi Y, Tsuji H. Intracortical facilitation and inhibition after transcranial magnetic stimulation in conscious humans. J Physiol. (1997) 498:817–23. doi: 10.1113/jphysiol.1997.sp021905
29. Keller A. Intrinsic synaptic organization of the motor cortex. Cereb Cortex. (1993) 3:430–41. doi: 10.1093/cercor/3.5.430
30. Cash RFH, Noda Y, Zomorrodi R, Radhu N, Farzan F, Rajji TK, et al. Characterization of glutamatergic and GABA A-mediated neurotransmission in motor and dorsolateral prefrontal cortex using paired-pulse TMS-EEG. Neuropsychopharmacology. (2017) 42:502–11. doi: 10.1038/npp.2016.133
31. Varrasi C, Civardi C, Boccagni C, Cecchin M, Vicentini R, Monaco F, et al. Cortical excitability in drug-naive patients with partial epilepsy: a cross-sectional study. Neurology. (2004) 63:2051–5. doi: 10.1212/01.WNL.0000145770.95990.82
32. Simonetta-Moreau M, Lourenço G, Sangla S, Mazieres L, Vidailhet M, Meunier S. Lack of inhibitory interaction between somatosensory afferent inputs and intracortical inhibitory interneurons in focal hand dystonia. Mov Disord. (2006) 21:824–34. doi: 10.1002/mds.20821
33. Orth M, Münchau A. Transcranial magnetic stimulation studies of sensorimotor networks in Tourette syndrome. Behav Neurol. (2013) 27:57–64. doi: 10.1155/2013/349137
34. Chen R. Interactions between inhibitory and excitatory circuits in the human motor cortex. Exp Brain Res. (2004) 154:1–10. doi: 10.1007/s00221-003-1684-1
35. Shirota Y, Ohminami S, Tsutsumi R, Terao Y, Ugawa Y, Tsuji S, et al. Increased facilitation of the primary motor cortex in de novo Parkinson's disease. Park Relat Disord. (2019) 66:125–9. doi: 10.1016/j.parkreldis.2019.07.022
36. Säisänen L, Julkunen P, Niskanen E, Danner N, Hukkanen T, Lohioja T. Motor potentials evoked by navigated transcranial magnetic stimulation in healthy subjects. J Clin Neurophysiol. (2008) 25:367–72. doi: 10.1097/WNP.0b013e31818e7944
37. Lefaucheur JP, Aleman A, Baeken C, Benninger DH, Brunelin J, Di Lazzaro V, et al. Evidence-based guidelines on the therapeutic use of repetitive transcranial magnetic stimulation (rTMS): an update (2014–2018). Clin Neurophysiol. (2020) 131:474–528. doi: 10.1016/j.clinph.2019.11.002
38. Lefaucheur JP, Aleman A, Baeken C, Benninger DH, Brunelin J, Di Lazzaro V, et al. Corrigendum: “Evidence-based guidelines on the therapeutic use of repetitive transcranial magnetic stimulation (rTMS): An update (2014–2018)” (Clinical Neurophysiology. (2020) 131(2) (474–528), (S1388245719312799), (10.1016/j.clinph.2019.11.002)). Clin Neurophysiol. (2020) 131:1168–9. doi: 10.1016/j.clinph.2020.02.003
39. Darmani G, Bergmann TO, Zipser C, Baur D, Müller-Dahlhaus F, Ziemann U. Effects of antiepileptic drugs on cortical excitability in humans: a TMS-EMG and TMS-EEG study. Hum Brain Mapp. (2019) 40:1276–89. doi: 10.1002/hbm.24448
40. Ziemann U. TMS and drugs. Clin Neurophysiol. (2004) 115:1717–29. doi: 10.1016/j.clinph.2004.03.006
41. Ziemann U, Reis J, Schwenkreis P, Rosanova M, Strafella A, Badawy R, et al. TMS and drugs revisited 2014. Clin Neurophysiol. (2015) 126:1847–68. doi: 10.1016/j.clinph.2014.08.028
Keywords: motor outcome, glioblastoma, neuromodulation, brain tumor, transcranial magnetic stimulation, cortical excitability
Citation: Neville IS, Gomes dos Santos A, Almeida CC, Hayashi CY, Solla DJF, Galhardoni R, de Andrade DC, Brunoni AR, Teixeira MJ and Paiva WS (2021) Evaluation of Changes in Preoperative Cortical Excitability by Navigated Transcranial Magnetic Stimulation in Patients With Brain Tumor. Front. Neurol. 11:582262. doi: 10.3389/fneur.2020.582262
Received: 11 July 2020; Accepted: 14 December 2020;
Published: 22 January 2021.
Edited by:
Thomas Picht, Charité – Universitätsmedizin Berlin, GermanyReviewed by:
Yasuo Terao, Kyorin University, JapanPetro Julkunen, Kuopio University Hospital, Finland
Copyright © 2021 Neville, Gomes dos Santos, Almeida, Hayashi, Solla, Galhardoni, de Andrade, Brunoni, Teixeira and Paiva. This is an open-access article distributed under the terms of the Creative Commons Attribution License (CC BY). The use, distribution or reproduction in other forums is permitted, provided the original author(s) and the copyright owner(s) are credited and that the original publication in this journal is cited, in accordance with accepted academic practice. No use, distribution or reproduction is permitted which does not comply with these terms.
*Correspondence: Alexandra Gomes dos Santos, YWxleGFuZHJhLmdvbWVzJiN4MDAwNDA7Zm0udXNwLmJy