- 1VA Boston Healthcare System, Boston, MA, United States
- 2Department of Neurology, School of Medicine, Boston University, Boston, MA, United States
- 3Department of Radiology and Biomedical Imaging, University of California, San Francisco, San Francisco, CA, United States
- 4Department of Biostatistics, School of Public Health, Boston University, Boston, MA, United States
- 5VA Boston Healthcare System, National Center for Posttraumatic Stress Disorder, Boston, MA, United States
- 6Wellman Center for Photomedicine, Massachusetts General Hospital, Boston, MA, United States
- 7Laser Research Center, Faculty of Health Sciences, University of Johannesburg, Johannesburg, South Africa
Introduction: Approximately 25–30% of veterans deployed to Kuwait, 1990-91, report persistent multi-symptom Gulf War Illness (GWI) likely from neurotoxicant exposures. Photobiomodulation (PBM) in red/near-infrared (NIR) wavelengths is a safe, non-invasive modality shown to help repair hypoxic/stressed cells. Red/NIR wavelengths are absorbed by cytochrome C oxidase in mitochondria, releasing nitric oxide (increasing local vasodilation), and increasing adenosine tri-phosphate production. We investigated whether PBM applied transcranially could improve cognition, and health symptoms in GWI.
Materials and Methods: Forty-eight (40 M) participants completed this blinded, randomized, sham-controlled trial using Sham or Real, red/NIR light-emitting diodes (LED) applied transcranially. Fifteen, half-hour transcranial LED (tLED) treatments were twice a week (7.5 weeks, in-office). Goggles worn by participant and assistant maintained blinding for visible red. Pre-/Post- testing was at Entry, 1 week and 1 month post- 15th treatment. Primary outcome measures were neuropsychological (NP) tests; secondary outcomes, Psychosocial Questionnaires, including PTSD.
Results: Primary Analyses (all participants), showed improvement for Real vs. Sham, for Digit Span Forwards (p < 0.01); and a trend for Trails 4, Number/Letter Sequencing (p < 0.10). For secondary outcomes, Real group reported more improvement on the SF-36V Plus, Physical Component Score (p < 0.08). Secondary Analyses included only subjects scoring below norm (50%ile) at Entry, on specific NP test/s. Real and Sham improved at 1 week after 15th treatment; however, at 1 month, only those receiving Real improved further: Digit Span Total, Forwards and Backwards; Trails 4, Number/Letter Sequencing; California Verbal Learning Test-II, long delay free recall; Continuous Performance Test-II, False Alarm Rate; and Color-Word Interference, Stroop, Trial 3, Inhibition; Sham group worsened, toward Entry values. Only those with more post-traumatic stress disorder (PTSD) symptomatology at Entry, receiving Real, continued to have additional PTSD reduction at 1 month; Sham regressed.
Conclusion: This study was underpowered (n = 48), with large heterogeneity at Entry. This likely contributed to significance or trend to significance, for only two of the NP tests (Digit Span Forwards; Trails 4, Number/Letter Sequencing) and only one general health measure, the SF-36V Plus, Physical Component Score. More subjects receiving Real, self-reported increased concentration, relaxation and sleep. Controlled studies with newer, transcranial LED home treatment devices are warranted; this is expected to increase enrollment.
Clinical Trial Registration: www.ClinicalTrials.gov, identifier: NCT01782378.
Introduction
An estimated 25–30% of 700,000 veterans deployed to the Kuwait Theater, 1990-91, have developed a persistent multi-symptom illness related to neurotoxicant exposures (1, 2). To date, treatments developed for Gulf War Illness (GWI) have been insufficient. Interventions addressing underlying pathologies are needed. Photobiomodulation (PBM) is a plausible therapy to address GWI symptoms. PBM consisting of red and near-infrared (NIR) wavelengths of light may impart beneficial effects on mitochondrial and cellular function, immune function, and inflammation, all of which are affected in GWI.
Gulf War Illness
Gulf War veterans have presented with Chronic Multi-symptom Illness which includes one or more chronic symptoms (lasting >6 months) from at least two of three symptom categories: (1) musculoskeletal (muscle or joint pain, stiffness); (2) mood-cognition; (3) fatigue. Headaches and/or gastro-intestinal problems may also be present (1, 3). Symptoms have been found to worsen over time, consistent with chronic inflammation (2, 4). GWI veterans' symptoms are heterogeneous. However, the persistent presence of impairments can have profound negative effects on veterans' daily functioning.
Cognitive Dysfunction in Veterans With GWI
Research on cognitive and behavioral changes associated with GWI has been mixed (5). Although GWI symptoms are heterogeneous, a meta-analysis of published studies regarding neuropsychological/cognitive functioning in GWI veterans, has found evidence of significantly decreased performance within three cognitive domains: attention/executive function, learning/memory, and visuospatial skills (5).
Mitochondrial Dysfunction
Neurotoxicant exposures including low levels of sarin, cyclosarin, smoke, pollutants from oil well-fires, organophosphate pesticides and pyridostigmine bromide pills are thought to be the main causes of symptoms associated with GWI (4, 6, 7). The latter two can cause CNS tissue loss from direct damage to mitochondria, leading to oxidative stress and mitochondrial dysfunction (4, 8–12). Mitochondrial dysfunction occurring in the brain can produce cognitive dysfunction; while in the muscles it causes chronic pain and fatigue (2, 13–16).
Immunological and Inflammatory Dysfunction in GWI
Immune dysfunction may also be a factor to consider, regarding therapeutics for GWI (4, 17, 18). Georgopoulos and colleagues observed an abnormal synchronous neural interaction pattern in GWI using magnetoencephalography (MEG), reflecting in part, altered brain function in genetically vulnerable individuals (19). Similar immune system disruption occurs in relapsing-remitting multiple sclerosis, Sjogren's syndrome and rheumatoid arthritis. Additionally, blood biomarkers of inflammation - e.g., lymphocytes, monocytes and C-reactive protein were 90% predictive of GWI in a cohort of veterans (20).
Photobiomodulation (PBM)
PBM therapy is a safe, non-invasive, painless, non-thermal modality based on a strong body of research dating back to the 1960's (21). Light-emitting diode (LED) or low-level laser/light therapy (LLLT), uses either visible red light (600–700 nm) or near-infrared (NIR) wavelengths of light (usually 810, 830, up to 1,100 nm) to repair damaged or dying cells and tissue (22–24).
Detailed Cellular Effects of PBM
PBM has produced beneficial cellular effects in controlled trials (25). Red/NIR wavelength photons are absorbed within the mitochondrial membrane by the last complex (IV) of the electron transport chain, cytochrome C oxidase (CCO), particularly in hypoxic/stressed cells (26). This promotes increased adenosine tri-phosphate (ATP) production (24, 27) and increased local vasodilation due to release of nitric oxide from CCO. Along with improved mitochondrial and cellular function (23, 24, 28), reduced oxidative damage and reduced inflammation have been reported (29).
Approximately 3% of NIR LLLT photons applied to the head have been observed to penetrate through skin, scalp, bone and dura mater in human cadavers, enough to reach surface brain cortex (30). NIR (808 nm) photons from laser PBM have been detected at 4–5 cm beneath the site of scalp application (31). PBM to the ear canals has been observed to penetrate to the base of the skull in a cadaver and to reach the mesial temporal lobes, altering emotional modulation on a visual attention task (32). PBM applied to points on the ears has also been reported to reduce pain and insomnia (33).
Effect of Transcranial PBM in Animals
Positive results from application of transcranial PBM (tPBM) have been observed in animal models of traumatic brain injury (TBI) (34, 35); neurodegenerative diseases such as Alzheimer's Disease (AD) (36–39) and Parkinson's Disease (40–42).
Brain Imaging and Transcranial PBM in Humans
Application of transcranial light-emitting diodes (tLED) in humans has been shown to increase regional cerebral blood flow (rCBF) in normals (43, 44), in depression (45), severe TBI (46), and chronic mild-moderate TBI (47). Cognitive/behavioral improvements have been reported in chronic TBI (47–52), severe depression (45), acute and chronic stroke (53–55), and dementia (56, 57).
Improved functional connectivity on resting-state functional-connectivity MRI scans (rs-fcMRI) has been observed within intrinsic neural networks, including the default mode network (DMN), following tLED treatments in various patient populations. Chao (57) observed increased functional connectivity within the three posterior nodes of the DMN in chronic dementia patients, along with significant improvements in cognition. The dementia patients were treated at home, using a NIR LED device designed to treat only the cortical node areas of the DMN. Case studies have shown improved functional connectivity in the DMN in chronic stroke/aphasia, along with improved language following a series of tLED treatments (55); likewise, improved cognition and functional connectivity in athletes with repetitive head impacts who may develop chronic traumatic encephalopathy (CTE) – e.g., pro-football (51, 58), and ice hockey (59).
Increased functional connectivity has been observed within 1 min during NIR laser application (1 cm diameter, 808 nm, 250 mW, 318 mW/cm2) to the right forehead/frontal pole in adults (ages 18–40) (60). Immediate EEG changes have also been shown in healthy adults (ages 61–74) after one, 20-min tLED treatment applied only to cortical node areas of the DMN (61).
Intranasal delivery of PBM also may have beneficial effects. NIR intranasal LED (pulsed at 10 Hz) is hypothesized to deliver photons to the olfactory bulbs (on orbito-frontal cortex), where olfactory inputs activate medial entorhinal cortex via the hippocampus (62). In a small study, five dementia patients who used a NIR intranasal, pulsed at 10 Hz, daily for 12 weeks (with once weekly, headframe NIR tLED treatments to nodes of the DMN), improved significantly in cognition (56). Four dementia patients using NIR intranasal plus tLED at home, three times per week for 12 weeks, also observed cognitive improvement (57). Application of red intranasal PBM has been observed to improve blood rheology (blood viscosity, plasma viscosity, redox viscosity and red blood cell aggregation), and improve cholesterol levels (blood lipid total cholesterol and low/high-density lipoprotein cholesterol) in patients with coronary heart disease and cerebral infarction (63, 64).
Some benefits for GWI have been found from exercise, cognitive-behavioral therapies, co-enzyme Q10 supplements and individualized acupuncture treatments (9, 65). Two GWI case reports showed symptom reduction in the mood-cognitive domain, pain, sleep and fatigue after a 12-week, at-home transcranial plus intranasal NIR LED treatment series, treating every other day (66).
PBM offers a potentially safe and effective treatment option for veterans with GWI. This current study reports the results of a sham-controlled clinical trial that examined whether a 7.5-week series of red/NIR tLED treatments (plus LEDs applied over the ears, and intranasal LED in each nostril simultaneously) could improve cognition and other health symptoms in veterans with GWI.
Methods
Participants
Participants were recruited from subjects in a Department of Defense (DoD) study of a longitudinal cohort of Gulf War Veterans who returned from their deployment to Kuwait (1990-91) through Fort Devens, MA. This cohort has been followed at multiple time points since the end of the GW (67); and through the VA Informatics and Computing Infrastructure/Corporate Data Warehouse (VINCI/CDW) database, with approval from the Veteran's Health Administration (VHA) National Data System (NDS). The San Francisco VA Medical Center (SF VAMC) was a second site on this study (36 Veterans participated in Boston, and 12, in San Francisco on the same protocol). Those recruited from the VINCI/CDW database, resided within a 25-mile radius of the VA Boston Healthcare System (VABHS) or the SF VAMC. The Institutional Review Board at the VABHS and the SF VAMC (University of California, San Francisco) approved the study. In accordance with the Declaration of Helsinki, Informed Consent and HIPAA authorization were obtained. The ClinicalTrials.gov reference is NCT01782378.
Inclusion Criteria
Participants answered “Yes” during a telephone screening regarding: (1) Difficulty concentrating; and/or (2) Difficulty remembering recent information. Additional inclusion criteria were the following: Must be a veteran deployed in the 1990-91 Gulf War, to the Kuwait Theater; meets criteria for GWI as defined by “Symptom Questions used to identify Gulf War Illness by Kansas Case Definition, and Chronic Multi-symptom Illness by Fukuda Case Definition” (1, 3) – i.e., must have presence of one or more chronic symptoms (lasting >6 months) from at least two of three symptom categories from Fukuda 1998: (1) musculoskeletal (muscle pain, or joint pain, stiffness); (2) mood/cognition; (3) fatigue. Participants filled out the symptom questionnaire (3) at Entry to determine if they met these criteria. The veterans must have exhibited symptoms after deployment (with exposures) and have current symptomatology as noted above, fulfilling the Kansas Case Definition and Chronic Multi-symptom Illness definition of GWI.
In addition, the age range was 38–65 years; must be physically able to travel to the VABHS, Jamaica Plain campus or SF VAMC for Neuropsychological (NP) testing and tLED treatments; and must meet screening criteria from the Eligibility Screening, NP tests (administered at in-office Screening visit): Trail Making Test A & B (68, 69); Controlled Oral Word Association Test [COWAT (FAS) (70, 71); California Verbal Learning Test (CVLT) - II (72); Color-Word Interference Test, Stroop (73). For inclusion, participants were required to score at least 2 SD below the standardized norm (age, education) on at least one of the above-mentioned NP screening tests, or 1 SD below the standardized norm on at least two of them.
In addition, the Word Reading Subtest from the Wide Range Achievement Test-4 (74) was used to estimate premorbid level of cognitive functioning. The SD for each participant on each NP screening test was adjusted by his/her estimated premorbid cognitive level. A symptom validity measure, the Test of Memory Malingering (75) was also administered at screening. Participants who failed Trial 2, or Trial 1 and 2 were excluded from the study. If a participant failed Trial 1, but did not fail Trial 2, he/she was not excluded if he/she showed evidence of poor learning on other NP screening tests, such as the CVLT (76).
Additional Screening questionnaires were administered: Short Form McGill Pain Questionnaire (77); VAS pain rating (0–10); and the PTSD Checklist- Civilian (PCL-C) (78). Participants were included only if the VAS pain rating was ≤ 7/10, and ≤ 38/50 on the Short Form McGill Pain Questionnaire, as pain has been shown to influence cognition (79).
Exclusion Criteria
Exclusion criteria were the following: Less than age 38, or greater than age 65; presence of a neurodegenerative disease such as Amyotrophic Lateral Sclerosis, Parkinson's Disease, dementia; presence of a life-threatening disease such as cancer; presence of a severe mental disorder such as schizophrenia, or bipolar depression (not associated with PTSD); current substance abuse or active treatment within the last 6 months.
Randomization
A total of 63 eligible participants were randomized to the Sham or Real LED treatment groups (computer-assigned randomization with GraphPad.com, in blocks of 10). Three in each group dropped out before any treatment visits; and nine of the remaining 57 who started treatment visits dropped out (five from Sham, and four from Real) without any Post- testing, leaving a total of 48 participants (Sham group, 23 participants; Real group, 25 participants). See Consort Chart, Figure 1. Only the study coordinator had the code for the groups.
The demographics for each group (Sham and Real) are provided in Table 1; there were no significant differences (Pearson Chi-Square) between the two groups on any of the demographic variables.
Blinding
Each subject was assigned a random ID number and blinding was maintained by the study coordinator throughout the study. None of the other staff were aware of group assignment. This included the Neuropsychologist performing the Pre-/Post- testing, the principal investigator, the participant and family members, as well as those performing the LED treatments (Sham or Real), explained further under the subsection, Blinding at Each LED Treatment Session.
NP Tests and Questionnaires, Pre-/Post- Sham or Real LED Intervention Series
The NP testing was performed within 1 week before the intervention series, at 1 week after completion, and at 1 month later. Each NP Testing session was completed in 1.5 h. The NP tests (primary outcome measures), and the Psychosocial, Self-Report Measures/Questionnaires (secondary outcome measures) are listed in Table 2.
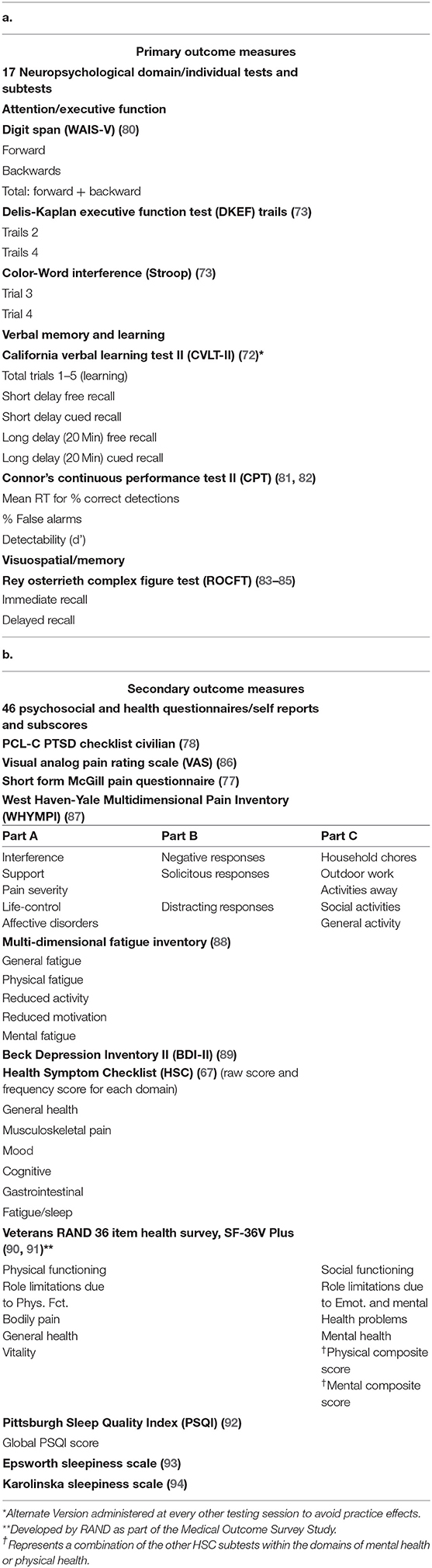
Table 2. a,b. Primary outcome measures, neuropsychological tests and secondary outcome measures, psychosocial and health questionnaires.
NP Tests, Domain Scores
Domain Scores were also calculated as a composite score to represent different areas of cognition: Attention and Executive Function (AF); Learning and Memory (LM); and Psychomotor/Visual Spatial (P/VS). AF included 8 subtests: Digit Span Total; Trail-Making Test conditions 2 and 4; reaction time for correct detections, false alarm rate and delta prime measures on the Continuous Performance Test (CPT); and Color-Word Interference Test, conditions 3 and 4. LM included 7 subtests: CVLT-II, total; short and long delay - free and cued recall; and Rey-Osterreith Complex Figure Test (ROCFT), immediate and delayed recall. P/VS included 2 subtests: CPT, reaction time for correct detections; and the ROCFT, copy.
LED Intervention Series (Sham or Real)
Schedule of Visits
The half-hour LED treatments (Sham or Real) were scheduled for twice a week, with at least 48 h between treatments, for 7.5 weeks (15 treatments).
Real LED Devices
Treatments were administered using three types of LED devices, simultaneously at each visit – this included (1) an LED-lined helmet (Photomedex or Thor); (2) LED cluster heads for ear placements (except when the larger, Thor helmet was used which covered the ears); and (3) two intranasal LED applicators. All participants received treatment with all three types of devices applied at the same time during each treatment, as described below. The Real LED devices are shown in Figure 2. The LED parameters and equipment specifications for each Real LED device are provided in Table 3. The VABHS Safety Subcommittee of the IRB approved all LED devices.
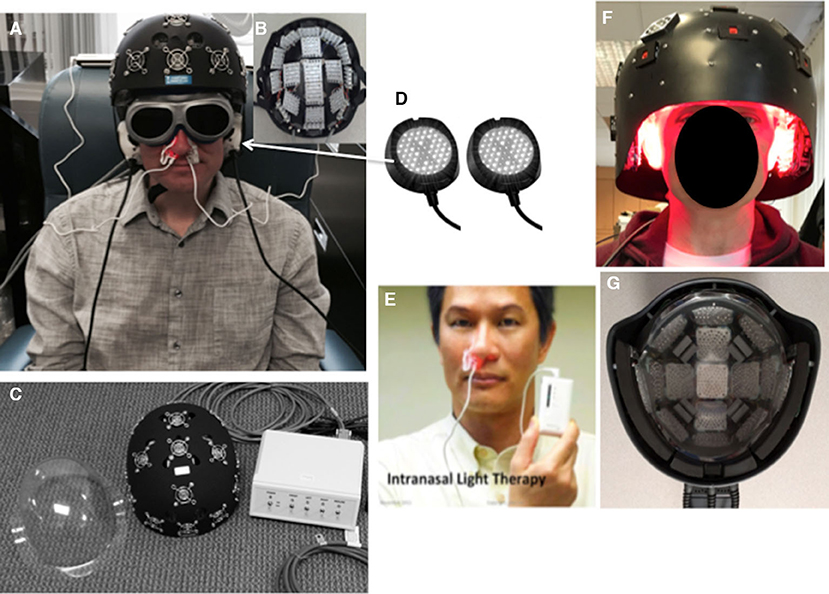
Figure 2. LED devices. (A) NIR, LED-lined helmet (PhotoMedex, Horsham, PA). (B) LED cluster head placement configuration inside PhotoMedex helmet. (C) Separate clear plastic liner assigned to each participant to fit inside the PhotoMedex helmet (to protect diodes and for hygiene), and control box where each row could be turned on and off separately. (D) Two NIR, LED cluster heads for placement over the ears (MedX Health, Toronto). (E) Red intranasal LED applicator (Vielight, Toronto; Hayward, CA); note, an identical NIR intranasal was also used in the other nostril. (F) NIR, LED-lined helmet (Thor Photomedicine, London, UK; Hampstead, MD), used if the participant's head had a circumference greater than 24 inches. (G) LED cluster head placement configuration inside the Thor helmet, with both red and NIR diodes in each cluster head. Only the NIR diodes were turned on for this study. During all helmet treatments, the midline row was first treated, then turned off, and then the left and right sides were treated. The fans for each LED cluster head inside the helmet (for cooling) were always turned on. Sham and real treatments felt identical. Participants and assistant wore goggles to block the red intranasal light. NIR, near-infrared wavelength; LED, light-emitting diodes. See Table 3 for LED specifications and treatment parameters. (E) Printed with permission from the Vielight Co.
Real LED Device 1, LED-Lined Helmet
A Real, NIR LED-lined helmet was manufactured by PhotoMedex, Inc., Horsham, PA (Figures 2A,B); or by Thor Photomedicine, Inc., London, UK; Hampstead, MD (Figures 2F,G). If a participant's head circumference was larger than 24 inches, the larger LED-lined helmet (Thor Photomedicine helmet) was used. Each LED-lined helmet contained previously-approved, FDA-cleared LED cluster heads which were considered non-significant risk. Each participant wore a clear plastic helmet liner (assigned to that participant only) to protect the LEDs from hair, and for hygiene reasons (Figure 2C).
Real LED Device 2, LED Cluster Heads for Ear Placements
Two NIR LED cluster heads (each, 2-inch diameter) were manufactured by MedX Health, Mississauga, ON, Canada (Figure 2D). The smaller, Photomedex helmet did not cover the ears, thus these LEDs were used simultaneously on the left and right ears for 4 min, toward the end of each treatment visit when the Photomedex helmet was used. They were lower in power (90 mW) than the previously-approved, FDA-cleared (500 mW), non-significant risk MedX Health LED cluster heads. The larger, Thor Photomedicine LED Helmet contained LEDs that covered the ears.
Real LED Device 3, Intranasal LED Applicators
Two intranasal LEDs (red and NIR single-diode applicators) were manufactured by Vielight, Inc., Toronto, Canada; Hayward, CA. One intranasal LED was placed into each nostril, held in place with a plastic clip. Placement of the red intranasal LED (Figure 2E), and the NIR intranasal LED (not shown) was alternated by side (left/right), at each session. Due to the very low power of each intranasal LED, 8 mW and 14.2 mW (red and NIR, respectively), each was considered to be within the low-risk device, FDA category of General Wellness, no medical claims made.
Sham LED Devices
Sham LED devices that looked and felt identical to the Real LED devices were also manufactured by each LED company listed above. No red or NIR photons were emitted from any of the Sham LED devices. The fans in a LED-lined Sham helmet were turned on during the entire half-hour Sham LED treatment session, as was also done during a Real LED session. The helmet fans were used for cooling.
Blinding at Each LED Treatment Session (Sham or Real)
For the purpose of blinding during a treatment session (Sham or Real), the participant and the assistant administering the treatment wore goggles (Diode Laser Safety Goggles – Model 55, LS-DIO-55, Phillips Safety Products, Inc., Middlesex, NJ). The goggles blocked 600–900 nm wavelengths, used particularly to block visual perception of the red, 633 nm photons emitted from the red intranasal diode. The NIR photons are beyond the visible spectrum. Two LED treatment rooms were used; the study coordinator instructed the assistant regarding which treatment room to use for each LED treatment (The LED equipment could also be moved randomly, from one treatment room to another). All LED devices looked and felt identical (Sham and Real).
To address the possible effect of skin pigmentation on photon absorption, the Fitzpatrick Skin Type Scale (95) was used to determine skin type (pigmentation level), ranging from 1 (very fair skin) to 6 (darkest skin). The dose was adjusted when treating participants who had the lightest pigmentation, 1; or the darkest pigmentation, 6. Four participants had levels 1 or 6, and all others, in between. For those with the lightest skin, the treatment time/dose was reduced by 25%, and for those with the darkest, increased by 25%.
Possible Changes in Medications During Participation
Participants were encouraged not to change medications or dosages, if possible, during participation in the study. At each treatment visit (twice a week), they answered questions regarding possible changes in medications, sleep, pain or other changes/complaints since the previous treatment.
Treatment Compliance and Safety
Forty-eight participants completed all 15 treatments, however, only 25% of group 1 (Sham) and 21% of group 2 (Real) adhered to the intervention schedule of in-office visits twice per week for 7.5 weeks. If a participant missed a treatment, that treatment was added at the end of the series. Participants were withdrawn from the study if they had only one treatment within a 2-week period. An average total of 8.1 weeks was required for participants to complete 15 treatments (mean 8.35, Sham; mean 7.9, Real). Four participants required > 10 weeks to complete the 15 treatments (3 Sham; 1 Real). Nine of the 57 participants who began treatments dropped out before any Post- testing was performed (15.7%). There were no adverse events or negative side effects.
Statistical Analyses
Primary Analyses described below were performed on data for all 48 participants. Secondary Analyses described below were performed on data for only those participants who scored below norm at Entry, on a specific NP test. Not all participants scored below average on all NP tests at Entry due to large heterogeneity among scores at Entry.
Primary Analyses (All Participants)
Each NP test was modeled as a two-factor ANOVA with factors, time (Pre-Tx Entry, 1 week Post- Tx, and 1 month Post- Tx) and treatment (Sham, Real). Without correction for multiple comparisons, each test outcome was first tested as a fixed effects model. The fixed effects model was re-run adjusting for baseline demographic factors. These additional analyses showed little effect on the results and are not shown.
We recruited 63 participants, six dropped out, and 57 began treatments. However, nine cases had only Entry/baseline data; hence, no subsequent measurements of response to treatment. The 48 remaining cases provided little statistical power, even without correction for multiple comparisons. Besides having p <5% indicate potentially useful predictors, we also displayed factors with a p-value between 5 and 10%, which might also prove useful predictors for future studies. We designated as potentially useful, those factors falling between 5 and 10%, with the smallest alternative p-values. We re-ran the fixed effects ANOVA model, adding the assumption that the factor “subject” was a random effect. This gave us a random effects p-value and a fixed effects p-value for each factor.
In summary, our method used a set of two p-values to identify potentially useful factors; a measure of relative consistency rather than an absolute measure of significance. We kept the threshold small, p <10%, out of respect for the level of Type I error.
Primary Analyses for the secondary outcome measures (Psychosocial, Self-Report Measures/Questionnaires) were performed in the same manner. Baseline demographic factors did not affect the results. All analyses were run using SAS version 9.4.
Secondary Analyses (Only Participants Who Scored Below Norm at Entry, on a Specific Test)
As mentioned, there was large heterogeneity within the scores for the cognitive tests at Entry, and not all participants had scores that were below the norm on all NP tests. This is consistent with the known heterogeneity of GWI symptomology. Therefore, for a single NP test, the data for only those participants who scored at, or below, a z-score of −0.5 (below 50th percentile for the norm) on that specific NP test at Entry, were included in the Secondary Analyses. This resulted in a varied number of subjects for each NP test, reducing the power due to fewer participants per group. Every participant was included for some of the NP tests. A fixed effects ANOVA model and a mixed-design random effects analysis with the same factors as for the Primary Analyses, were performed for each separate NP test.
For the secondary outcome measures/Questionnaires, cutoff scores were used to classify participants. On the PCL-C, analyses were completed separately for those who scored <36 at Entry (mild or no PTSD symptomatology), and for those who scored >36 at Entry (evidence of PTSD symptomatology) (96, 97). For the Beck Depression Inventory (BDI) (89, 98), analyses were completed for subgroups based on Entry scores: Little to no depression, scores of 0–12; mild depression, 13–19; moderate, 20–29; and severe, >30. Analyses were completed using SPSS v.26.
Intent-to-Treat (ITT) Analyses
Of 63 participants enrolled, six dropped out before treatments, and nine only completed a few treatments; never returning for evaluation of treatment effects (Post- testing). Excluding these nine participants without Post- testing, constitutes modified ITT (99). The modified ITT analysis covers the 48 remaining participants. Dropouts per group (Sham and Real) were approximately equivalent. See Consort Chart, Figure 1. We included only those remaining 48 subjects with Pre- and at least most Post- testing data, because our goal was to explore the data to detect measures sensitive to treatments effects.
Results
Primary Analyses (All Participants)
Primary Outcome Measures
The p-values for Sham compared to Real for the primary outcome measures are shown in Table 4a (fixed effects results shown only for p < 0.10). Only one primary outcome measure showed significant improvement at the p < 0.10 level, on the mixed design random effects model analysis in favor of Real compared to Sham over time, Digit Span Forwards (Working Memory, Executive Function), p < 0.01, Est Value −0.55, Std Err 0.33. A trend to significance was present for Trails 4, Number/Letter Sequencing (Executive Function), p < 0.10, Est. Value −0.36, Std Err 0.22. See Figures 3A,B.
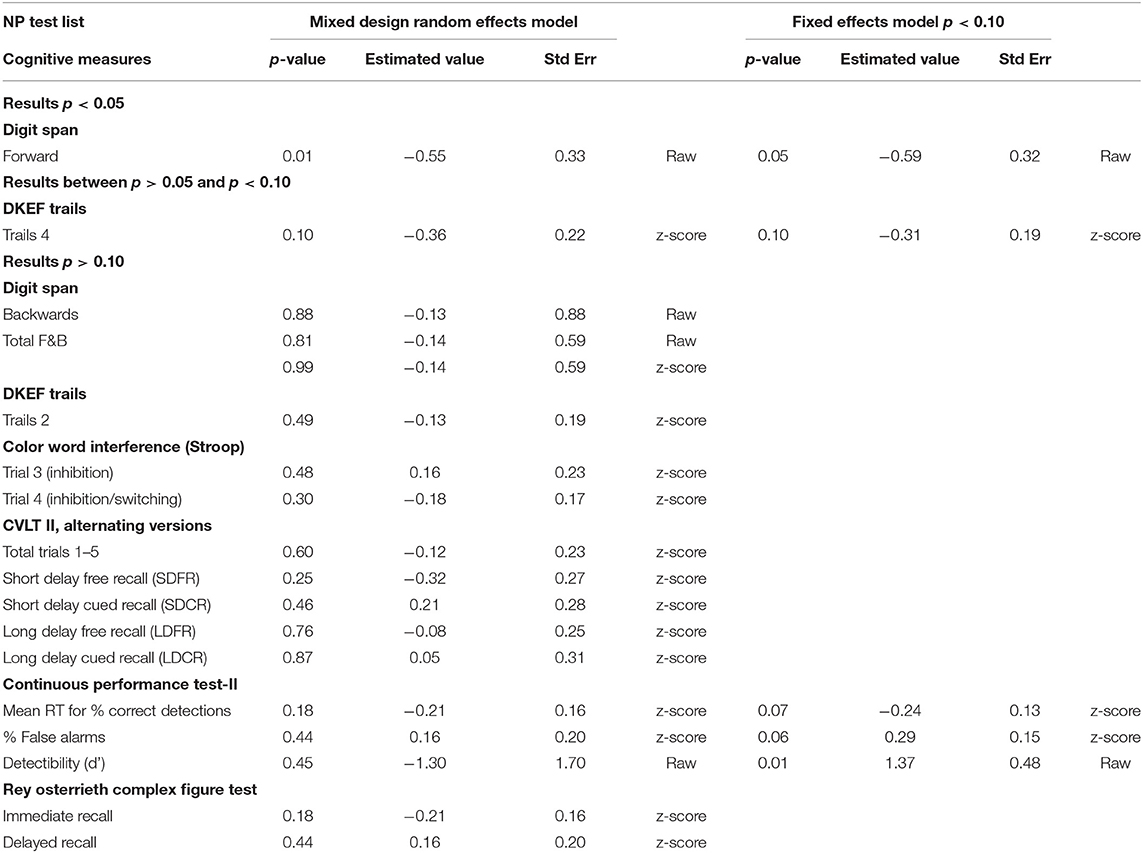
Table 4a. Primary analyses (all participants), for fixed effects model and mixed design random effects model, for primary outcome measures/cognitive measures.
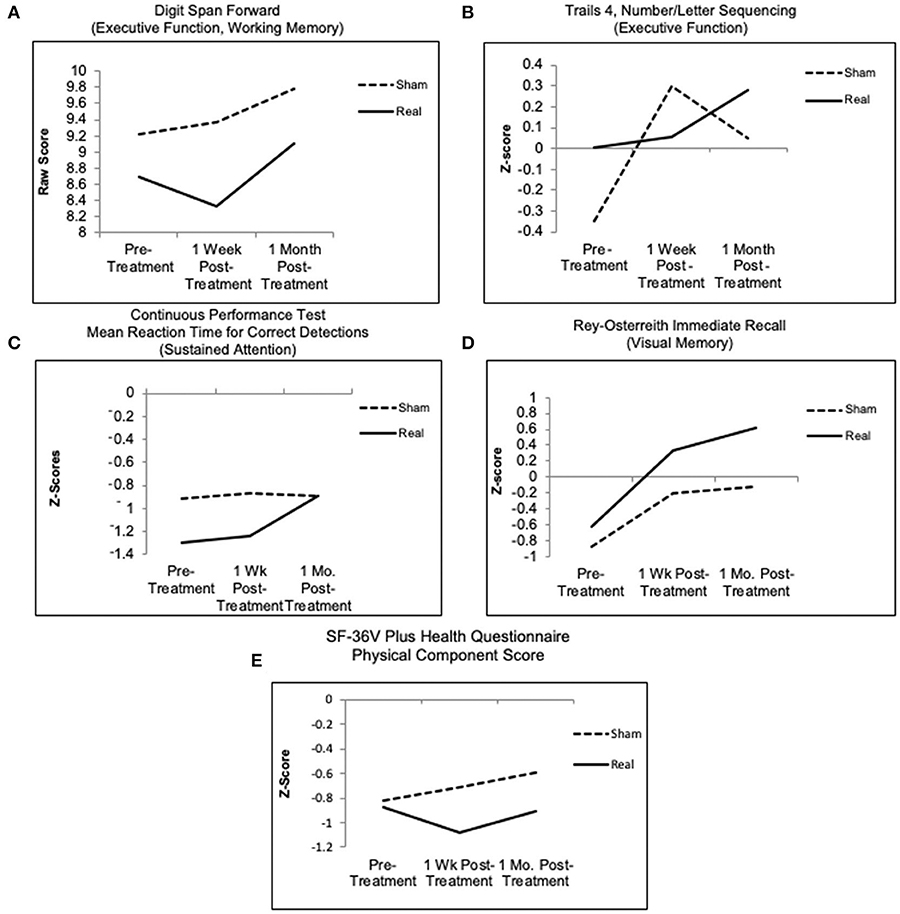
Figure 3. Graphed results for primary analyses (all participants), mixed design random effects model for primary outcome measures/cognitive measures, for four NP tests over time. (A,B,D) Higher scores indicate better outcome. (C) Continuous performance test, only the real group improved, showing shorter reaction time at 1 month post- treatment; the sham group showed no change. (E) SF-36V plus health questionnaire, physical component score (a secondary outcome measure), only the real group endorsed fewer symptoms at 1 week post- LED, relative to entry; whereas the sham group endorsed more symptoms at 1 week and 1 month. See Tables 4A,B for p-values.
Additionally, Figures 3C,D shows the Real group improved on the CPT, mean reaction time for correct detections (Sustained Attention), while the Sham group remained flat; and both groups improved on the Rey-Osterreith Complex Figure Test (ROCFT), immediate recall (Visual Memory), with a trend toward greater improvement for the Real group. These two NP tests, however, and other cognitive measures did not show significance between the groups.
Secondary Outcome Measures
The p-values for Sham compared to Real for the secondary outcome measures, Psychosocial, Self-Report Measures/Questionnaires are shown in Table 4b (fixed effects results shown only for p < 0.10). On only one Secondary Outcome measure, the SF-36V Plus - Physical Component Score (PCS), those in the Real group endorsed fewer physical complaints over time, compared to those in the Sham group, p < 0.08, Est Value −0.31, Std Err 0.16. See Figure 3E. A trend to significance was present for Short Form McGill Pain Questionnaire, p < 0.08, Est. Value 3.36, Std Err 1.86. No change was observed in the Real group, while there was a slight decrease in pain in the Sham group. No differences were found for other secondary outcome measures including fatigue, sleep, or mood.
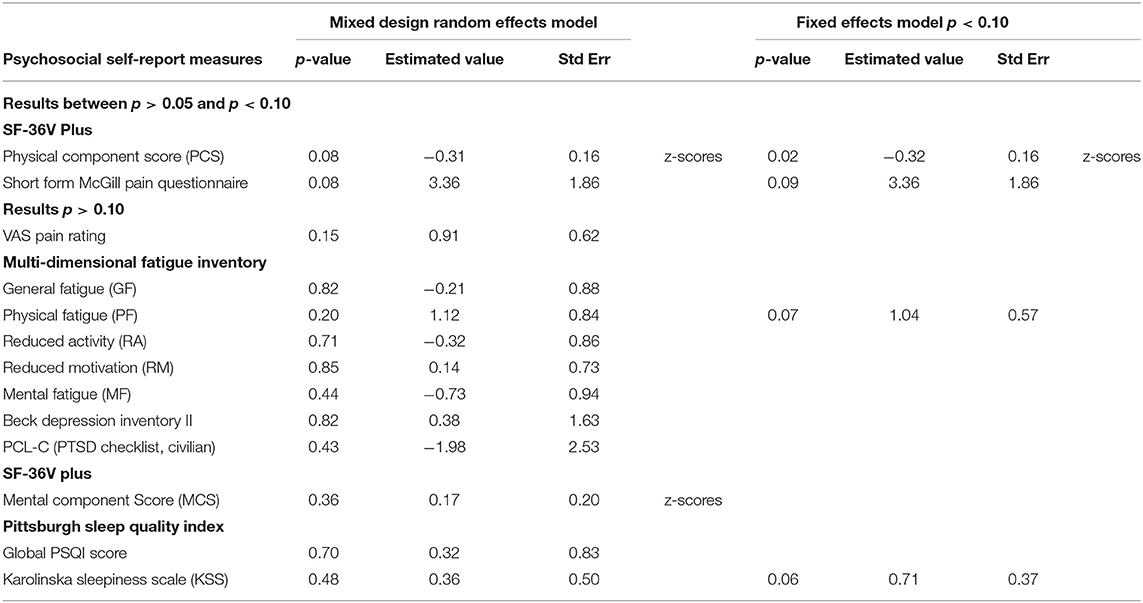
Table 4b. Primary analyses (all participants), for fixed effects model and mixed design random effects model, for secondary outcome measures/psychosocial self-report measures.
Secondary Analyses (Only Participants Who Scored Below Norm at Entry, on a Specific Test)
Primary Outcome Measures
After filtering to include only participants whose scores were below norm (50%ile) at Entry, on a specific NP test, a distinct, similar pattern of results was observed for the primary outcome/cognitive measures – i.e., both the Sham LED group and the Real LED group improved at 1 week after the final, 15th LED treatment, suggesting a placebo effect. However, at 1 month after the final, 15th LED treatment, only those in the Real LED group continued to improve, while those in the Sham LED group worsened, and their NP test scores returned toward Entry values. See Figures 4A–F. There was no significant group x time interaction.
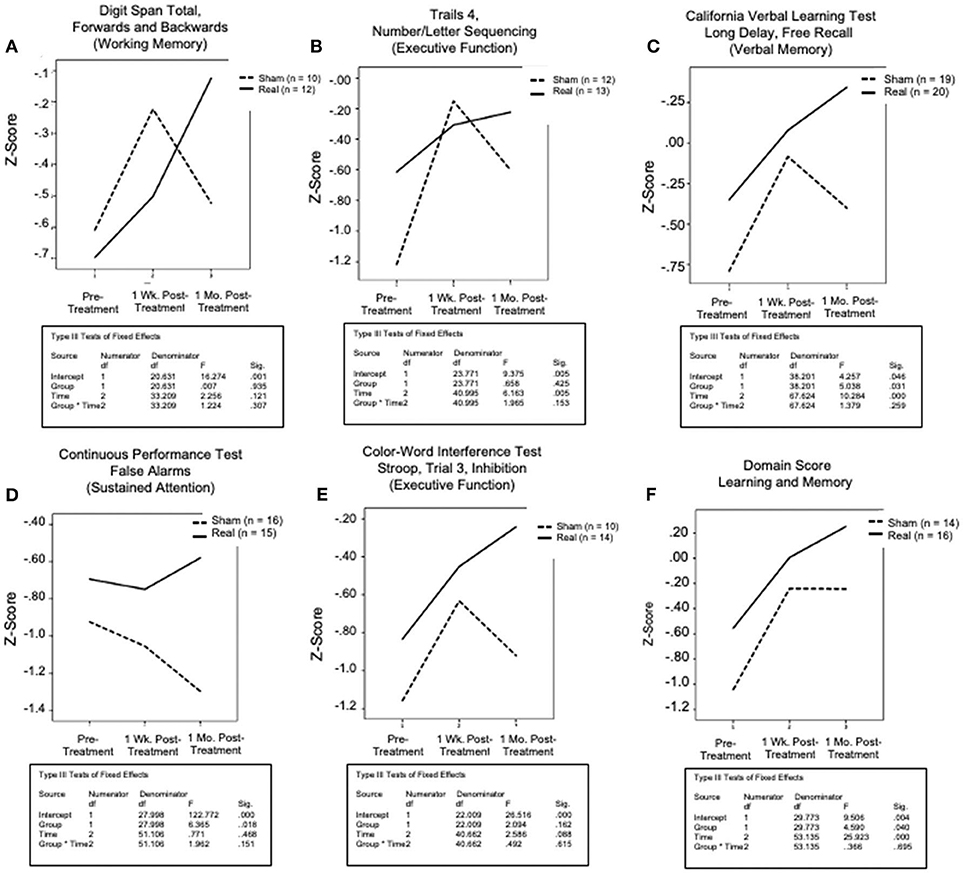
Figure 4. Graphed results for secondary analyses (only subjects who scored below norm at entry, on a specific test), primary outcome/cognitive measures for five NP tests and one domain score. These reflect the same factors as the primary analyses [time (pre-Tx entry, 1-Wk post- Tx, 1-Mo post- Tx); and treatment (sham, real), and their interaction], for each. The domain score for learning and memory reflects a composite score for seven NP tests: the CVLT (total; short and long delay - free and cued recall); and the rey-osterreith complex figure test (immediate and delayed recall). There was a consistent pattern across all graphs, where both groups showed improvement at 1 week post- the final, sham or real LED treatment, but only those who received real LED continued to improve at the 1-month, post- testing time point. Those who received sham, regressed at 1 month, approaching entry values.
The five cognitive tests that showed continued, increased performance at 1 month after the final, 15th Real LED treatment included: (1) Digit Span Total, Forwards and Backwards (Working Memory, Executive Function); (2) Trails 4, Number/Letter Sequencing (Executive Function); (3) CVLT-II, long-delay, free recall (Verbal Memory); (4) CPT, False Alarm Rate (Sustained Attention); and (5) Color-Word Interference Test, Stroop, Trial 3, Inhibition (Executive Function). A similar post- LED result pattern was observed for the Domain Score for Learning and Memory. The LM Domain Score included 7 subtests: CVLT-II (total; short- and long- delay, free and cued recall); and the ROCFT (immediate and delayed recall). See Figure 4F.
Secondary Outcome Measures
Results for measures of PTSD and depression are shown in Figures 5A–E. Participants who had scores <36 on the PCL-C at Entry (mild symptomatology) showed little or no change over time in either the Sham LED or the Real LED groups (Figure 5A). However, those with scores >36 on the PCL-C at Entry showed the same pattern as that observed for the cognitive tests mentioned above – i.e., a reduction in PTSD at 1 week post- the Sham or the Real LED series, but at 1 month, only those who had received the Real LEDs continued to improve even more, with reduced PTSD scores at that time (Figure 5B). Those who had received the Sham LEDs regressed, toward PTSD Entry scores. One participant did not complete the PCL-C at Entry (declined to complete it).
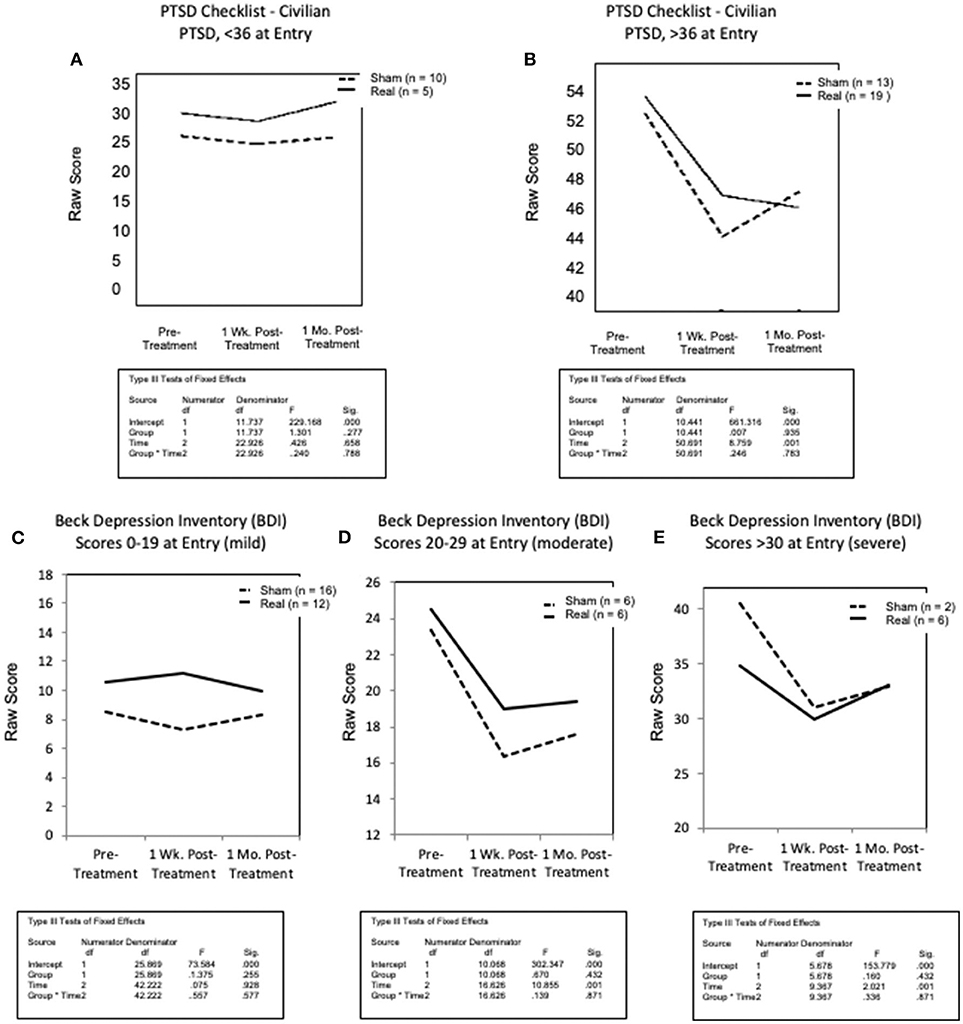
Figure 5. Graphed results for secondary analyses, for secondary outcome measures/psychosocial self-report/questionnaires for PTSD (A,B); and depression (C–E). All participants were studied for each secondary outcome measure, but only in subgroups as stratified by level of severity at entry [mild, moderate, severe]. (A) Scores less than 36 (mild or no PTSD) showed little change post-treatment in either group, or at either post- testing time point. (B) In contrast to that, for those entering with PCL-C scores greater than 36 (indicative of PTSD symptoms reported at the clinical level), both groups reported reduced PTSD at 1-week post-treatment. However, at 1-month, only the real LED group continued to report even fewer PTSD symptoms, while those in sham, reported increased symptoms, toward entry values. (C) For depression, on the beck depression inventory, there was no change for those with mild or no depression (BDI scores of only 0–19, at entry) in either treatment condition at any time of post- testing. (D,E) However, for those with moderate or severe depression (BDI scores of 20–29, or >30, respectively), those in the sham and real LED groups both reported less depression at 1 week post- treatment. At 1 month post- treatment, however, both groups began to return slightly toward entry values. No difference between sham and real LED was observed, likely due to small numbers in the subgroups.
On the BDI (Figures 5C–E), veterans in the mild group (scores of 0–19 at Entry) showed no change over time, in either treatment group. Veterans with moderate depression (scores 20–29), and severe depression (scores > 30) improved at 1 week Post- treatment, in both the Sham and the Real treatment groups. At 1 month Post- treatment, scores in both groups started back toward Entry, but did not return to Entry levels. Small numbers, with only 2 to 6 subjects in the moderate or severe, Sham or Real groups, likely affected the minimal group differences.
After all post- testing was completed, participants were provided the opportunity to comment on the study. They reported on psychosocial changes noticed outside the laboratory setting. A total of 10 participants offered comments, post- Sham or Real. There were 13 categories of comments from the Real LED group, and 6 categories of comments from the Sham LED group. Four participants who received Real, commented that they noticed increased concentration; one participant from Sham, mentioned increased concentration. See Table 5.
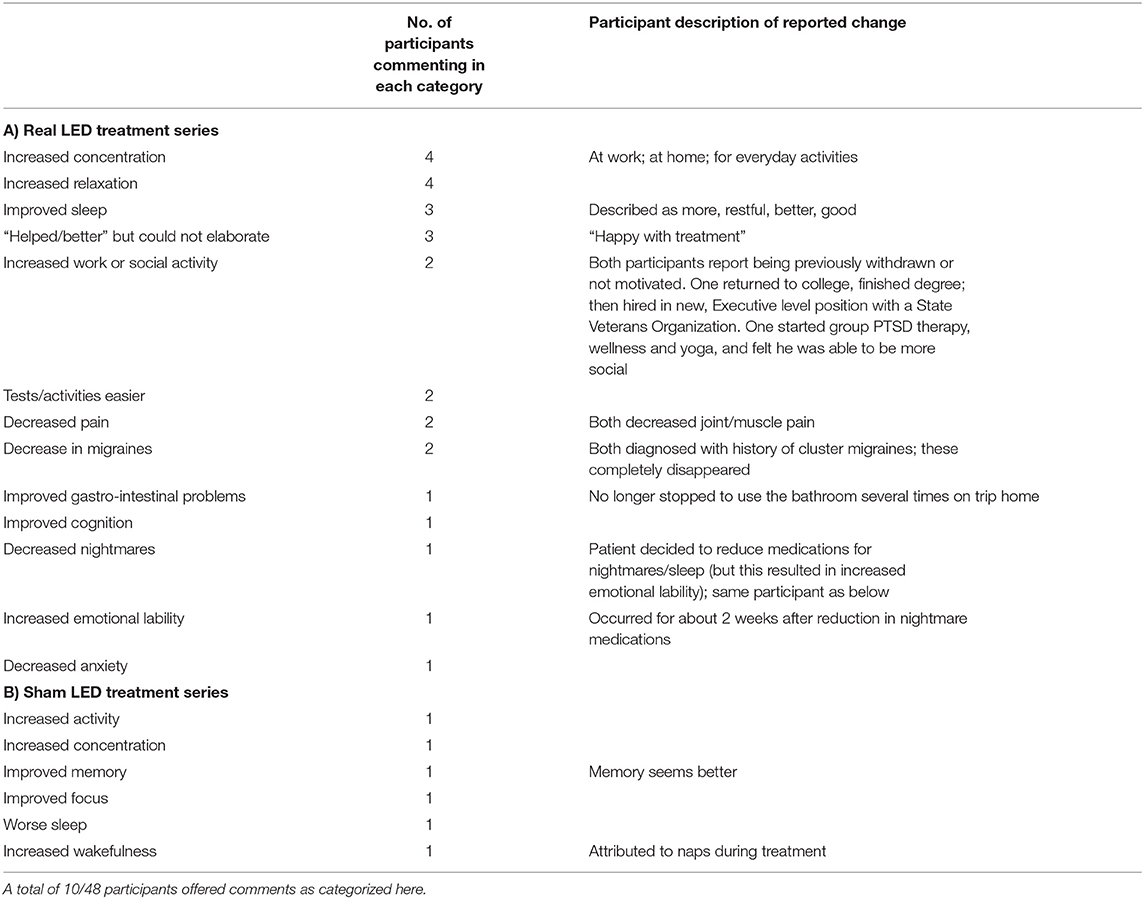
Table 5. List of open-ended comments/changes noticed by participants outside the laboratory setting, during or after the study.
Discussion
To our knowledge, this is the first Sham-controlled study to examine effects of tPBM therapy on cognition and health symptoms in veterans with GWI. In the Primary Analyses, which included data for all participants, results showed a significant effect in the Real LED group compared to Sham over time, for only one out of 17 NP tests, Digit Span Forwards (Working Memory, Executive Function) (p < 0.01), and a trend to significance for Trails 4, Number/Letter Sequencing (Executive Function) (p < 0.10).
Significant improvement was also observed in favor of Real compared to Sham, for only one secondary outcome measure SF-36V Plus, Physical Component Score (p < 0.08). Significant improvement on this test was previously reported in an acupuncture study with GWI veterans (65).
Under the null hypothesis we would expect false positives 5% of the time, a Type I error of 5%. This would yield around three false positives among 63 tests, including subtests. In fact, we observed three: (1) Digit Span Forwards; (2) Trails 4, Number/Letter Sequencing; (3) SF-36V Plus, Physical Component Score. Had we used a Bonferroni correction, then no results would have been significant and no implications could be drawn. Chance could not be ruled out. Thus, we performed Secondary Analyses to identify possible future predictors.
The Secondary Analyses were performed to include only participants for each NP test who scored below the norm (<50th percentile) at Entry, due to large heterogeneity in scores at Entry, consistent with literature describing the heterogeneity of GWI. A separate and distinct pattern of results was observed. When tested at 1 week after the final, 15th LED treatment, there was improvement on cognitive testing in both groups. When tested at 1 month, however, only those who had received Real, continued to show improvement, whereas those who received Sham, worsened with scores returning toward Entry value. Although, due to small numbers, there was no significant group x time interaction, five out of 17 NP tests showed improvement for the Real group at 1 month including: (1) Digit Span Total, Forwards and Backwards (Working Memory, Executive Function); (2) Trails 4, Number/Letter Sequencing (Executive Function); (3) CVLT, long delay, free recall (Verbal Memory); (4) CPT, False Alarm Rate (Sustained Attention); and (5) Color-Word Interference Test, Stroop, Trial 3, Inhibition (Executive Function).
A meta-analysis of GWI studies recommending which NP tests to use in GWI treatment efficacy studies (5) included the first four of the five NP tests mentioned above. That meta-analysis had also suggested a Visuospatial Memory Test, Block Design. Results from the NP improvements observed in the secondary analyses of this study suggest that the tests listed above may be useful as future predictors for inclusion in treatment efficacy studies, including for tPBM treatment studies.
Regarding the secondary outcome measures/Psychosocial Self-Report/Questionnaires in the Secondary Analyses, improvement occurred only when there was a higher level of symptomatology at Entry. When participants endorsed more PTSD symptomatology on the PCL-C (scores >36), there was reduced PTSD after both the Sham and Real LED series at 1 week. At 1 month, however, only those who had received Real, had additional improvement; whereas Sham worsened, toward Entry value. A somewhat similar pattern was present for the BDI. There was reduced depression for those with moderate or severe depression in both the Sham and Real groups at 1 week post- treatment, but slight increase in depression at 1 month post- treatment in both groups. Studies focusing on depression should include a greater number of subjects, even with moderate-severe depression, to better determine possible changes related to tPBM. Schiffer et al. (45), had reported a reduction in severe depression after only one tLED treatment to F3 and F4 (10–20 EEG system), however, after 1 month, the level of depression began to rise. Continued tPBM treatments may be necessary with depression.
Regarding a placebo effect, research in various treatment studies has shown there are three main components that can affect a placebo response (100): (1) assessment and observation, (2) therapeutic ritual, and (3) a supportive patient-practitioner relationship, with the latter being the most robust. The present study contained all three components, and in particular, the patient-practitioner relationship could have played a role in treatment response. There were bi-weekly interactions for at least a half hour, during each of the 15 treatment visits (Sham and Real), and this could have affected the 1-week post- testing. At the 1-month post- testing time point, however, there had been no bi-weekly visits for the previous 4 weeks. At the 1-month post- testing visit, scores in the Sham group declined toward Entry values, but scores in the Real group continued to improve, possibly separating out a placebo response that had occurred in the Sham group at 1 week.
An additional factor to consider regarding a placebo effect at 1-week Post- treatment, is the color green, which was visually perceived by all participants when wearing the goggles. Functional MRI research with migraine patients has reported that cortical hyperactivation associated with pain, can be reduced in migraine with tinted lenses (101); green light via cone-driven retinal pathways has been observed to have therapeutic effects (102, 103). The goggles may have indirectly had a beneficial, cortical effect for up to 1 week in the present study. This is unknown. The effect may have worn off at the 1-month post- testing time point resulting in a regression of scores toward Entry in the Sham LED group, whereas those in the Real LED group had scores that continued to improve. Two participants who received the Real LEDs commented after completion of the study that their migraine headaches had completely disappeared (Table 5). Since all subjects in each group wore the goggles, it is possible that the green color perceived through the goggles had a modulating effect on the cortical activation associated with pain in migraine, in accordance with the literature (101–103). In addition, the red photons from the red intranasal may have improved blood circulation, improving their migraines (63, 64).
Although small, the observed improvements following Real tPBM could have been associated with decreased inflammation (29), increased focal rCBF (45, 46), improved functional connectivity (51, 55, 57–59), as well as increased ATP production in hypoxic/stressed cells (26), all factors associated with PBM application.
This study was unique, in that it combined transcranial, intranasal, plus ear application of the LEDs to allow coverage of additional regions. While the relative contribution of any specific placement location of the LEDs is unknown, it is possible that a synergistic effect was obtained from all three. Specifics of LED placements need to be studied to understand how to obtain optimal effects. Future studies could also include blood work, and brain imaging studies such as rs-fcMRI, magnetic resonance spectroscopy, or arterial spin labeling to examine some of these factors.
Future tLED research could also include additional follow-up time points in order to better evaluate a long-term response. In the present study, the improvements observed in the Real LED group, which increased 1 month later, suggests a possible long-term effect of tPBM. A long-term pattern of improvement out to 2 months after a 6-week, Real tLED treatment series was observed in chronic, TBI/PTSD cases (49).
Limitations
The sample size was small (48 cases), making it difficult to observe significant improvement, when considering the number of NP tests examined. Thus, chance cannot be ruled out. However, small effects were observed that may inform future studies. Although our study examined 17 NP tests in the Primary Analyses, our Secondary Analyses support testing GWI veterans, within three cognitive domains: attention/executive function, learning/memory, and visuospatial skills, which have been identified as areas of difficulty in GWI veterans (5). Including GWI veterans who perform poorly at Entry, in at least one or more of these three domains may be important in future studies, especially since there is large heterogeneity among the GWI veterans in cognitive abilities and symptomatology. This would improve the design, and statistical power, requiring fewer participants to determine significance.
In future tLED studies, using only NIR wavelengths (beyond the visible spectrum) and omitting any red LEDs, could avoid potential confound from goggles necessary to block visible red. Optimum tLED placement, treatment dose and treatment schedules are yet to be determined. In-office treatments at a VAMC (twice per week for almost 2 months) was difficult. An at-home tLED intervention would ease the burden of on-site visits, increasing enrollment and reducing dropouts. Treating at home could improve treatment compliance and allow for treating more often - e.g., three times per week (as in our previous TBI and stroke studies). In the present study, only 21% of those receiving Real tLED kept to the treatment schedule of twice per week (and 25% of Sham). Home treatments may also remove the patient-practitioner confounding interaction.
Technical improvements in PBM equipment since the time this study was designed (2012) have now made tLED home treatments feasible (57, 58, 66). The positive changes observed in the present study in cognition and reduced PTSD, support previous findings from the two case reports of GWI veterans treated at home with NIR tLED where symptom reduction was present in the mood-cognitive domain after 12 weeks of treatments, applied every other day (66). That study also observed reduced pain, sleep and fatigue; these improvements may have been associated with more frequent treatments (three times per week) and a longer treatment period (12 weeks), compared to the present study. Weekly telehealth phone calls could establish treatment compliance.
Conclusions
This study was underpowered (n = 48), with large heterogeneity at Entry, which likely contributed to significance or trend to significance, for only two of the NP tests (Digit Span Forwards; and Trails 4, Number/Letter Sequencing) and the SF-36V Plus, Physical Component Score. GWI veterans who exhibit cognitive impairments, particularly in the domains of Executive Function, Working Memory, Sustained Attention and/or Verbal Memory are likely good candidates for future tPBM studies. Transcranial PBM is a safe therapy without negative side effects or adverse events. Controlled studies with newer, transcranial LED home treatment devices are warranted; this is expected to increase enrollment.
Data Availability Statement
The datasets presented in this article are not readily available because Datasets are available by written request to the PI, MN, with approval by the VA Boston Healthcare System IRB, Privacy and Security Offices. Requests to access the datasets should be directed to MN, bW5hZXNlciYjeDAwMDQwO2J1LmVkdQ==.
Ethics Statement
The studies involving human participants were reviewed and approved by The Institutional Review Board at the VA Boston Healthcare System and the San Francisco VA Medical Center (University of California, San Francisco). In accordance with the Declaration of Helsinki, Informed Consent and HIPAA authorization were obtained. The VA Boston Healthcare System, Safety Subcommittee of the IRB approved all LED devices. The patients/participants provided their written informed consent to participate in this study. Written informed consent was obtained from the individual(s) for the publication of any potentially identifiable images or data included in this article.
Author Contributions
PM: study coordinator, study design and implementation, recruitment and screening, data management, collection, scoring, statistics, and manuscript writing/preparation. MN: Principal Investigator (PI)- Boston, study design and implementation, study oversight, manuscript writing/preparation, PBM oversight, and training. LC: PI- San Francisco, study implementation and oversight (SF), and manuscript preparation. MK: study design and implementation, neuropsychologist, data collection, and manuscript preparation. MDH: study design and implementation, treatment application, and manuscript preparation. MY: recruitment, screening, data collection, scoring, and statistics. RL: statistics. JK: neuropsychologist, study design, data collection, and manuscript writing/preparation. MRH: PBM expertise/safety consultation and study design. All authors contributed to the article and approved the submitted version.
Funding
Department of Veterans Affairs, Office of Research and Development, Merit Review Grant Project ID: IO1CX000524 MRH was supported by US NIH Grants R01AI050875 and R21AI121700.
Conflict of Interest
MRH declares the following potential conflicts of interest: Scientific Advisory Boards: Transdermal Cap Inc, Cleveland, OH; BeWell Global Inc, Wan Chai, Hong Kong; Hologenix Inc. Santa Monica, CA; LumiThera Inc, Poulsbo, WA; Vielight, Toronto, Canada; Bright Photomedicine, São Paulo, Brazil; Quantum Dynamics LLC, Cambridge, MA; Global Photon Inc, Bee Cave, TX; Medical Coherence, Boston MA; NeuroThera, Newark DE; JOOVV Inc, Minneapolis-St. Paul MN; AIRx Medical, Pleasanton CA; FIR Industries, Inc. Ramsey, NJ; UVLRx Therapeutics, Oldsmar, FL; Ultralux UV Inc, Lansing MI; Illumiheal & Petthera, Shoreline, WA; MB Lasertherapy, Houston, TX; ARRC LED, San Clemente, CA; Varuna Biomedical Corp. Incline Village, NV; Niraxx Light Therapeutics, Inc, Boston, MA. Consulting; Lexington Int, Boca Raton, FL; USHIO Corp, Japan; Merck KGaA, Darmstadt, Germany; Philips Electronics Nederland B.V. Eindhoven, Netherlands; Johnson & Johnson Inc, Philadelphia, PA; Sanofi-Aventis Deutschland GmbH, Frankfurt am Main, Germany. Stockholdings: Global Photon Inc, Bee Cave, TX; Mitonix, Newark, DE.
The remaining authors declare that the research was conducted in the absence of any commercial or financial relationships that could be construed as a potential conflict of interest.
Acknowledgments
The authors thank Alfred Intintoli, David Cellucci, Bruce Richie, Ken Snyder, Jim Tracey for engineering work on the first LED-lined helmets; James Carroll, the second helmets; and Eugen Kiss, the ear LEDs. We also thank Lew Lim, Ph.D. and Anita Saltmarche, MHSc for assistance with LED devices; Beatrice Golomb, MD, Ph.D., Marc Goldstein MD, R. Rox Anderson MD for early medical/safety consultation; Kimberly Sullivan, Ph.D., Carole L. Palumbo, Ph.D. for study design; Yelena Bogdanova, Ph.D. for testing; Errol H. Baker, Ph.D., for statistics; Ethan Treglia, MS, Angela R. Berlingeri, MSW, Andrea E. Fedoruk, Nina Alpert as Research Assistants. The authors have obtained written permission from all persons named in the acknowledgments.
References
1. Steele L. Prevalence and patterns of Gulf War illness in kansas veterans: association of symptoms with characteristics of person, place, and time of military service. Am J Epidemiol. (2000) 152:992–1002. doi: 10.1093/aje/152.10.992
2. Research Advisory Committee on Gulf War Veterans Illnesses. Gulf War Illness and the Health of Gulf War Veterans: Research Update and Recommendations, 2009-2013. Boston, MA: US Government Printing Office (2014).
3. Fukuda K, Nisenbaum R, Stewart G, Thompson WW, Robin L, Washko RM, et al. Chronic multisymptom illness affecting air force veterans of the gulf war. JAMA. (1998) 280:981. doi: 10.1001/jama.280.11.981
4. White RF, Steele L, O'Callaghan JP, Sullivan K, Binns JH, Golomb BA, et al. Recent research on Gulf War illness and other health problems in veterans of the 1991 Gulf War: effects of toxicant exposures during deployment. Cortex. (2016) 74:449–75. doi: 10.1016/j.cortex.2015.08.022
5. Janulewicz PA, Krengel MH, Maule A, White RF, Cirillo J, Sisson E, et al. Neuropsychological characteristics of Gulf War illness: a meta-analysis. PLoS ONE. (2017) 12:e0177121. doi: 10.1371/journal.pone.0177121
6. Chao LL, Zhang Y, Buckley S. Effects of low-level sarin and cyclosarin exposure on white matter integrity in Gulf War Veterans. NeuroToxicol. (2015) 48:239–48. doi: 10.1016/j.neuro.2015.04.005
7. Abu-Qare AW, Abou-Donia MB. Combined Exposure To Deet (N,N -Diethyl- M -Toluamide) and permethrin-induced release of rat brain mitochondrial cytochrome C. J Toxicol Env Health A. (2001) 63:243–52. doi: 10.1080/15287390151143640
8. Kaur P, Radotra B, Minz R, Gill K. Impaired mitochondrial energy metabolism and neuronal apoptotic cell death after chronic dichlorvos (OP) exposure in rat brain. NeuroToxicol. (2007) 28:1208–19. doi: 10.1016/j.neuro.2007.08.001
9. Golomb BA, Allison M, Koperski S, Koslik HJ, Devaraj S, Ritchie JB. Coenzyme Q10 benefits symptoms in Gulf War veterans: results of a randomized double-blind study. Neural Comp. (2014) 26:2594–651. doi: 10.1162/NECO_a_00659
10. Shetty GA, Hattiangady B, Upadhya D, Bates A, Attaluri S, Shuai B, et al. Chronic oxidative stress, mitochondrial dysfunction, Nrf2 Activation and inflammation in the hippocampus accompany heightened systemic inflammation and oxidative stress in an animal model of Gulf War Illness. Front Mol Neurosci. (2017) 10:182. doi: 10.3389/fnmol.2017.00182
11. Abdullah L, Evans JE, Joshi U, Crynen Reed J, Mouzon B, et al. Translational potential of long-term decreases in mitochondrial lipids in a mouse model of Gulf War Illness. Toxicol. (2016). 372:22–33. doi: 10.1016/j.tox.2016.10.012
12. Chen Y, Meyer JN, Hill HZ, Lange G, Condon MR, Klein JC, et al. Role of mitochondrial DNA damage and dysfunction in veterans with Gulf War Illness. PLoS ONE. (2017) 12:e0184832. doi: 10.1371/journal.pone.0184832
13. Sullivan K. Cognitive functioning in treatment seeking gulf war veterans: pyridostigmine bromide use and PTSD. J Psychopath Behl Assess. (2003) 25:95–103. doi: 10.1023/A:1023342915425
14. Golomb BA. Acetylcholinesterase inhibitors and Gulf War illnesses. Proc Natl Acad Sci USA. (2008) 105:4295–300. doi: 10.1073/pnas.0711986105
15. Koslik HJ, Hamilton G, Golomb BA. Mitochondrial dysfunction in Gulf War Illness revealed by 31 phosphorus magnetic resonance spectroscopy: a case-control study. PLoS ONE. (2014) 9:e92887. doi: 10.1371/journal.pone.0092887
16. Rayhan RU, Raksit MP, Timbol CR, Adewuyi O, Vanmeter JW, Baraniuk JN. Prefrontal lactate predicts exercise-induced cognitive dysfunction in Gulf War Illness. Am J Transl Res. (2013) 5:212–23.
17. O'Callaghan JP, Kelly KA, Locker AR, Miller DB, Lasley SM. Corticosterone primes the neuroinflammatory response to DFP in mice: potential animal model of Gulf War Illness, J. Neurochem. (2015) 133:708–21. doi: 10.1111/jnc.13088
18. O'Callaghan JP, Miovicz LT, Kelly KA. Supporting a neuroimmune basis of Gulf War illness. EBioMed. (2016) 13:5–6. doi: 10.1016/j.ebiom.2016.10.037
19. Georgopoulos AP, James LM, Carpenter AF, Engdahl BE, Leuthold AC, Lewis SM. Gulf War illness (GWI) as a neuroimmune disease. Brain Res. (2017) 235:3217–25. doi: 10.1007/s00221-017-5050-0
20. Johnson GJ, Slater BC, Leis LA, Rector TS, Bach RR. Blood biomarkers of chronic inflammation in gulf war illness. PLoS ONE. (2016) 11:e0157855. doi: 10.1371/journal.pone.0157855
21. Mester E, Spiry T, Szende B, Tota JG. Effect of laser rays on wound healing. Am J Surg. (1971) 122:532–5. doi: 10.1016/0002-9610(71)90482-X
22. Ferraresi C, Parizotto NA, de Sousa MVP, Kaippert B, Huang Y-Y, Koiso T, et al. Light-emitting diode therapy in exercise-trained mice increases muscle performance, cytochrome c oxidase activity, ATP and cell proliferation. J Biophot. (2015) 8:740–54. doi: 10.1002/jbio.201400087
23. Karu TI, Pyatibrat LV, Afanasyeva NI. Cellular effects of low power laser therapy can be mediated by nitric oxide. Laseurg Med. (2005) 36:307–14. doi: 10.1002/lsm.20148
24. Wong-Riley MT, Liang HL, Eells JT, Chance B, Henry MM, Buchmann E, et al. Photobiomodulation directly benefits primary neurons functionally inactivated by toxins: role of cytochrome C oxidase. J Biol Chem. (2005) 280:4761–71. doi: 10.1074/jbc.M409650200
25. Desmet KD, Paz DA, C JJ, Eells JT, Wong-Riley MT, Henry MM, et al. Clinical and experimental applications of NIR-LED photobiomodulation. Photomed Laser Surg. (2006) 24:121–8. doi: 10.1089/pho.2006.24.121
26. Ayuk SM, Houreld NN, Abrahamse H. Effect of 660 nm visible red light on cell proliferation and viability in diabetic models in vitro under stressed conditions. Lasers Med Sci. (2018) 33:1085–93. doi: 10.1007/s10103-017-2432-2
27. Karu T, Pyatibrat L, Kalendo G. Irradiation with He-Ne laser increases ATP level in cells cultivated in vitro. Photochem Photobiol B Biol. (1995) 27:219–23. doi: 10.1016/1011-1344(94)07078-3
28. Eells JT, Henry MM, Summerfelt P, Wong-Riley MT, Buchmann EV, Kane M, et al. Therapeutic photobiomodulation for methanol-induced retinal toxicity. Proc Natl Acad Sci USA. (2003) 100:3439–44. doi: 10.1073/pnas.0534746100
29. Hamblin MR. Mechanisms and applications of the anti-inflammatory effects of photobiomodulation. AIMS Biophys. (2017) 4:337–61. doi: 10.3934/biophy.2017.3.337
30. Wan S, Anderson RR, Parrish JA. Analytical modeling for the optical properties of the skin with in vitro and in vivo applications. PhotochemPhotobiol. (1981) 34:493–9. doi: 10.1111/j.1751-1097.1981.tb09391.x
31. Tedford CE, DeLapp S, Jacques S, Anders J. Quantitative analysis of transcranial and intraparenchymal light penetration in human cadaver brain tissue: transcranial and intraparenchymal light penetration. Lasers Surg Med. (2015) 47:22. doi: 10.1002/lsm.22343
32. Sun L, Peräkylä J, Kovalainen A, Ogawa KH, Karhunen PJ, Hartikainen KM. Human brain reacts to transcranial extraocular light. PLoS ONE. (2016) 11:e0149525. doi: 10.1371/journal.pone.0149525
33. Round R, Litscher G, Bahr F. Auricular Acupuncture with Laser. Comp Alt Med. (2013) 2013:984763. doi: 10.1155/2013/984763
34. Oron A, Oron U, Streeter J, de Taboada L, Alexandrovich A, Trembovler V, et al. Low-level laser therapy applied transcranially to mice following traumatic brain injury significantly reduces long-term neurological deficits. J Neurotrauma. (2007) 24:651–6. doi: 10.1089/neu.2006.0198
35. Xuan W, Vatansever F, Huang L, Wu Q, Xuan Y, Dai T, et al. Transcranial low-level laser therapy improves neurological performance in traumatic brain injury in mice: effect of treatment repetition regimen. PLoS ONE. (2013) 8:e53454. doi: 10.1371/journal.pone.0053454
36. De Taboada L J, El-Amouri S, Gattoni-Celli S, Richieri S, McCarthy T, et al. Transcranial laser therapy attenuates amyloid-β peptide neuropathology in amyloid-β protein precursor transgenic Mice. J Alz Dis. (2011) 23:521–35. doi: 10.3233/JAD-2010-100894
37. Grillo SL, Duggett NA, Ennaceur A, Chazot PL. Non-invasive infra-red therapy (1072nm) reduces β-amyloid protein levels in the brain of an Alzheimers disease mouse model, TASTPM. J Photochem Photobiol B Biol. (2013) 123:13–22. doi: 10.1016/j.jphotobiol.2013.02.015
38. Purushothuman S, Johnstone DM, Nandasena C, Mitrofanis J, Stone J. Photobiomodulation with near infrared light mitigates Alzheimers disease-related pathology in cerebral cortex – evidence from two transgenic mouse models. Alz Res Therapy. (2014) 6:2. doi: 10.1186/alzrt232
39. Purushothuman S, Johnstone DM, Nandasena C, van Eersel J, Ittner LM, Mitrofanis J, et al. Near infrared light mitigates cerebellar pathology in transgenic mouse models of dementia. Neurosci Lett. (2015) 591:155–9. doi: 10.1016/j.neulet.2015.02.037
40. Reinhart F, Massri NE, Darlot F, Torres N, Johnstone DM, Chabrol C, et al. 810 nm near-infrared light offers neuroprotection and improves locomotor activity in MPTP-treated mice. Neurosci Res. (2015) 92:86–90. doi: 10.1016/j.neures.2014.11.005
41. Johnstone DM, Moro C, Stone J, Benabid AL, Mitrofanis J. Turning on lights to stop neurodegeneration: the potential of near infrared light therapy in Alzheimers and Parkinsons disease. Front Neurosci. (2016) 9:500. doi: 10.3389/fnins.2015.00500
42. Darlot F, Moro C, El Massri N, Chabrol C, Johnstone DM, Reinhart F, et al. Near-infrared light is neuroprotective in a monkey model of Parkinson disease: neuroprotection. Ann Neurol. (2016) 79:59–75. doi: 10.1002/ana.24542
43. Wang X, Tian F, Soni S, Gonzalez-Lima F, Liu H. Interplay between up-regulation of cytochrome-c-oxidase and hemoglobin oxygenation induced by near-infrared laser. Sci Rep. (2016) 6:30540. doi: 10.1038/srep30540
44. Tian F, Hase SN, Gonzalez-Lima F, Liu H. Transcranial laser stimulation improves human cerebral oxygenation: human cerebral oxygenation by laser stimulation. Lasers Surg Med. (2016) 48:343–9. doi: 10.1002/lsm.22471
45. Schiffer F, Johnston AL, Ravichandran C, Poi A, Teicher MH, Webb RH, et al. Psychological benefits 2 and 4 weeks after a single treatment with near infrared light to the forehead: a pilot study of 10 patients with major depression and anxiety. Beh Brain Funct. (2009) 5:46. doi: 10.1186/1744-9081-5-46
46. Nawashiro H, Wada K, Nakai K, Sato S. Focal Increase in cerebral blood flow after treatment with near-infrared light to the forehead in a patient in a persistent vegetative state. Photomed Laser Surg. (2012) 30:231–3. doi: 10.1089/pho.2011.3044
47. Hipskind SG, Grover FL, Fort TR, Helffenstein D, Burke TJ, Quint SA, et al. Pulsed transcranial red/near-infrared light therapy using light-emitting diodes improves cerebral blood flow and cognitive function in veterans with chronic traumatic brain injury: a case series. Photobiomod Photomed Laser Surg. (2019) 37:77–84. doi: 10.1089/photob.2018.4489
48. Naeser MA, Saltmarche A, Krengel MH, Hamblin MR, Knight JA. Improved cognitive function after transcranial, light-emitting diode treatments in chronic, traumatic brain injury: two case reports. Photomed Laser Surg. (2011) 29:351–8. doi: 10.1089/pho.2010.2814
49. Naeser MA, Zafonte R, Krengel MH, Martin PI, Frazier J, Hamblin MR, et al. Significant improvements in cognitive performance post-transcranial, red/near-infrared light-emitting diode treatments in chronic, mild traumatic brain injury: open-protocol study. J Neurotrauma. (2014) 31:117. doi: 10.1089/neu.2013.3244
50. Naeser MA, Martin PI, Ho MD, Krengel MH, Bogdanova Y, Knight JA, et al. Transcranial, red/near-infrared light-emitting diode therapy to improve cognition in chronic traumatic brain injury. Photomed Laser Surg. (2016) 34:610–26. doi: 10.1089/pho.2015.4037
51. Martin PI, Ho MD, Bogdanova Y, Krengel MH, Knight JA, Hamblin MR, et al. LED therapy improves functional connectivity and cognition in professional football player with TBI: case study. Arch Phys Med Rehab. (2018) 99:e104–5. doi: 10.1016/j.apmr.2018.07.374
52. Bogdanova Y, Martin PI, Ho MD, Yee MK, Ho VT, Knight JA, et al. Improved sleep and cognition post transcranial or intranasal, red/near-infrared LED treatments in chronic TBI: Pilot case series. J Head Trauma Rehab. (2015) 30:e101–2.
53. Lampl Y, Zivin JA, Fisher M, Lew R, Welin L, Dahlof B, et al. Infrared laser therapy for ischemic stroke: a new treatment strategy: results of the NeuroThera Effectiveness and Safety Trial-1 (NEST-1). Stroke. (2007) 38:1843–9. doi: 10.1161/STROKEAHA.106.478230
54. Zivin JA, Albers GW, Bornstein N, Chippendale T, Dahlof B, Devlin T, et al. Effectiveness and safety of transcranial laser therapy for acute ischemic stroke. Stroke. (2009) 40:1359–64. doi: 10.1161/STROKEAHA.109.547547
55. Naeser MA, Ho MD, Martin PI, Hamblin MR, Koo BB. Increased functional connectivity within intrinsic neural networks in chronic stroke following treatment with red/near-infrared transcranial photobiomodulation: case series with improved naming in aphasia. PhotobiomodPhotomed Laser Surg. (2020) 38:115–31. doi: 10.1089/photob.2019.4630
56. Saltmarche AE, Naeser MA, Ho KF, Hamblin MR, Lim L. Significant improvement in cognition in mild to moderately severe dementia cases treated with transcranial plus intranasal photobiomodulation: case series report. Photomed Laser Surg. (2017) 35:432–41. doi: 10.1089/pho.2016.4227
57. Chao LL. Effects of home photobiomodulation treatments on cognitive and Behavioral function, cerebral perfusion, and resting-state functional connectivity in patients with dementia: a pilot trial. Photobiomod Photomed Laser Surg. (2019) 37:133–41. doi: 10.1089/photob.2018.4555
58. Naeser MA, Martin PI, Ho MD, Krengel MH, Bogdanova Y, Knight JA, et al. Transcranial, near-infrared photobiomodulation to improve cognition in two, retired professional football players possibly developing CTE (0386) abstract from The international brain injury associations 13th world congress on brain injury: march 13 – 16, 2019 Toronto, Canada. Brain Inj. (2019) 33(Supp.1): 1–337. doi: 10.1080/02699052.2019.1608749
59. Chao LL, Barlow C, Karimpoor M, Lim L. Changes in brain function and structure after self-administered home photobiomodulation treatment in a concussion case. Front Neurol. (2020) 11:952. doi: 10.3389/fneur.2020.00952
60. Dmochowski GM, Shereen AD, Berisha D, Dmochowski JP. Near-infrared light increases functional connectivity with a non-thermal mechanism. Cereb. Cortex Comm. (2020) 1:tgaa004. doi: 10.1093/texcom/tgaa004
61. Zomorrodi R, Loheswaran G, Pushparaj B, Lim L. Pulsed Near Infrared Transcranial and Intranasal Photobiomodulation Significantly Modulates Neural Oscillations: a pilot exploratory study. Sci. Rep. (2019) 9:6309. doi: 10.1038/s41598-019-42693-x
62. Biella G, de Curtis M. Olfactory inputs activate the medial entorhinal cortex via the hippocampus. J Neurophysiol. (2000) 83:1924–31. doi: 10.1152/jn.2000.83.4.1924
63. Liu TC-Y, Cheng L, Su W-J, Zhang Y-W, Shi Y, Liu A-H, et al. Randomized, double-blind, and placebo-controlled clinic report of intranasal low-intensity laser therapy on vascular diseases. Int J Phot. (2012) 2012:489713. doi: 10.1155/2012/489713
64. Saour F, Gholipour-Khalili S, Farajdokht F, Kamari F, Walski T, Hamblin MR, et al. Therapeutic potential of intranasal photobiomodulation therapy for neurological and neuropsychiatric disorders: a narrative review. Rev Neurosci. (2020) 31:269–86. doi: 10.1515/revneuro-2019-0063
65. Conboy L, Gerke T, Hsu KY, St John M, Goldstein M, Schnyer R. The effectiveness of individualized acupuncture protocols in the treatment of gulf war illness: a pragmatic randomized clinical trial. PLoS ONE. (2016) 11:e0149161. doi: 10.1371/journal.pone.0149161
66. Chao LL. Improvements in Gulf War illness symptoms after near-infrared transcranial and intranasal photobiomodulation: two case reports. Mil Med. (2019) 184:e568–74. doi: 10.1093/milmed/usz037
67. Proctor SP, Heeren T, White RF, Wolfe Jrgos MS, Davis JD, et al. Health status of Persian Gulf War veterans: self-reported symptoms, environmental exposures and the effect of stress. Int J Epidemiol. (1998) 27:1000–10. doi: 10.1093/ije/27.6.1000
68. Reynolds C. R. Comprehensive Trail-Maiking Test (CTMT). Examiners Manual. Austin, TX: PRO-E (2002).
69. Tombaugh TN. Trail making test A and B: normative data stratified by age and education. Arch Clin Neuropsychol. (2004) 19:203–14. doi: 10.1016/S0887-6177(03)00039-8
70. Spreen O, Benton A. Neurosensory Centre Comprehensive Examination for Aphasia: Manual of Instructions. Victoria, BC: University of Victoria (1977).
71. Benton AL, Hamsher K. Multilingual Aphasia Examination. Iowa City, IA: AJA Associates, Inc (1989).
72. Delis DC, Kramer JH, Kaplan E, Ober BA. California Verbal Learning Test – Second Edition. Adult Version. 2nd Edn. San Antonio, TX: Psychological Corporation (2007).
73. Delis DC, Kaplan E, Kramer JH. Delis-Kaplan Executive Function System: Technical Manual. San Antonio, TX: Harcourt Assessment Company (2001).
74. Wilkinson G, Robertson G. WRAT4 Wide Range Achievement Test. Lutz, FL: Psychological Assessment Resources (2006).
76. Schroeder RW, Buddin WH, Hargrave DD, VonDran EJ, Campbell EB, Brockman CJ, et al. Efficacy of test of memory malingering trial 1, trial 2, the retention trial, and the albany consistency index in a criterion group forensic neuropsychological sample. Arch Clin Neuropsych. (2013) 28:21–9. doi: 10.1093/arclin/acs094
77. Melzack R. The short-form McGill pain questionnaire. Pain. (1987) 30:191–7. doi: 10.1016/0304-3959(87)91074-8
78. Weathers FW, Litz BT, Herman D, Huska J, Keane T. The PTSD Checklist-Civilian Version (PCL-C). Boston, MA: National Center for PTSD (1994).
79. Moriarty O, McGuire BE, Finn DP. The effect of pain on cognitive function: a review of clinical and preclinical research. Prog Neurobiol. (2011) 93:385–404. doi: 10.1016/j.pneurobio.2011.01.002
80. Weschler D. WAIS-IV-Wechsler Adult Intelligence Scale-Fourth Edition. 4th Edn. San Antonio, Pearson Education, Inc. (2008). doi: 10.1037/t15169-000
81. Rosvold HE, Mirsky AF, Sarason I, Bransome ED, Beck LH. A continuous performance test of brain damage. J Consult Psych. (1956) 20:343–50. doi: 10.1037/h0043220
82. Letz R, Baker E. L. Neurobehavioral Evaluation System: NES Users Manual. Winchester, MA: Neurobehavioral Systems, Inc. (1988).
83. Rey A. L'examen psychologique dans les cas dencephalopathie traumatique (Les problems). Arch Psychol. (1941) 28:215–85.
84. Knight JA, Kaplan E. (eds). Handbook of Rey-Osterrieth Complex Figure Usage: Clinical and Research Applications. Odessa, FL: Psychological Assessment Resources (2004).
85. Osterrieth PA. Filetest de copie dune figure complex: Contribution a letude de la perception et de la memoire [The test of copying a complex figure: a contribution to the study of perception and memory]. Arch Psychol. (1944) 30:286–395.
86. Farrar JT, Young JP, LaMoreaux L, Werth JL, Poole MR. Clinical importance of changes in chronic pain intensity measured on an 11-point numerical pain rating scale: Pain. (2001) 94:149–58. doi: 10.1016/S0304-3959(01)00349-9
87. Kerns RD, Turk DC, Rudy TE. The west haven-yale multidimensional pain inventory (WHYMPI). Pain. (1985) 23:345–56. doi: 10.1016/0304-3959(85)90004-1
88. Smets EM, Garssen B, Bonke B, De Haes JC. The Multidimensional Fatigue Inventory (MFI) psychometric qualities of an instrument to assess fatigue. J Psychosom Res. (1995) 39:315–25. doi: 10.1016/0022-3999(94)00125-O
89. Beck AT, Steer RA, Brown GK. (1996). Manual for Beck Depression Inventory. 2nd Edn. San Antonio, TX: The Psychological Corp.
90. Ware J, Kosinski M, Dewey JE. How to Score Version 2 of the SF-36 Health Survey. Lincoln, NE: Quality Metrics, Inc (2001).
91. Jones D, Kazis L, Lee A, Rogers W, Skinner K, Cassar L, et al. Health status assessments using the Veterans SF-36 and SF-12. Methods for evaluating outcomes in the Veterans Health administration. J Ambul Care Manag. (2001) 24:68–86. doi: 10.1097/00004479-200107000-00011
92. Buysse DJ, Reynolds CF, Monk TH, Berman SR, Kupfer DJ. The Pittsburgh sleep quality index: a new instrument for psychiatric practice and research. Psych Res. (1989) 28:193–213. doi: 10.1016/0165-1781(89)90047-4
93. Johns MW. A new method for measuring daytime sleepiness. the epsworth sleepiness scale. J Sleep Res. (1991) 14:540–5 doi: 10.1093/sleep/14.6.540
94. Shahid A, Shen J, Shapiro CM. Measurements of sleepiness and fatigue. J Psychosom Res. (2010) 69:81–9. doi: 10.1016/j.jpsychores.2010.04.001
95. Fitzpatrick TB. The validity and practicality of sun-reactive skin types I through VI. Arch Derm. (1988) 124:869–71. doi: 10.1001/archderm.124.6.869
96. Monson CM, Gradus JL, Young-Xu Y, Schnurr PP, Price JL, Schumm JA. Change in posttraumatic stress disorder symptoms: do clinicians and patients agree? Psychol Assess. (2008) 20:131–8. doi: 10.1037/1040-3590.20.2.131
97. Using the PTSD Checklist (PCL). National Center for PTSD. (2012). Available online at: https://www.ptsd.va.gov (accessed November 29, 2020).
98. Beck A. T. Depression: Causes and Treatment. Philadelphia, PA: University of Pennsylvania Press (2006).
99. Gupta SK. Intention-to-treat concept: a review. Pers Clin Res. (2011) 2:109–12. doi: 10.4103/2229-3485.83221
100. Kaptchuk TJ, Kelley JM, Conboy LA, Davis RB, Kerr CE, Jacobson EE, et al. Components of placebo effect: randomised controlled trial in patients with irritable bowel syndrome. BMJ. (2008) 336:999–1003. doi: 10.1136/bmj.39524.439618.25
101. Huang J, Zong X, Wilkins A, Jenkins B, Bozoki A, Cao Y. fMRI evidence that precision ophthalmic tints reduce cortical hyperactivation in migraine. Cephal. (2011) 31:925–36. doi: 10.1177/0333102411409076
102. Noseda R, Bernstein CA, Nir RR, Lee AJ, Fulton AB, Bertisch SM, et al. Migraine photophobia originating in cone-driven retinal pathways. Brain. (2016) 139:1971–86. doi: 10.1093/brain/aww119
Keywords: photobiomodulation (PBM), light-emitting diode (LED), cognitive function, Gulf war illness (GWI), LLLT (low-level laser/light therapy)
Citation: Martin PI, Chao L, Krengel MH, Ho MD, Yee M, Lew R, Knight J, Hamblin MR and Naeser MA (2021) Transcranial Photobiomodulation to Improve Cognition in Gulf War Illness. Front. Neurol. 11:574386. doi: 10.3389/fneur.2020.574386
Received: 19 June 2020; Accepted: 09 November 2020;
Published: 21 January 2021.
Edited by:
Eliane Correa Miotto, University of São Paulo, BrazilReviewed by:
Farzad Salehpour, Tabriz University of Medical Sciences, IranLuca Sebastianelli, Hospital of Vipiteno, Italy
Copyright © 2021 Martin, Chao, Krengel, Ho, Yee, Lew, Knight, Hamblin and Naeser. This is an open-access article distributed under the terms of the Creative Commons Attribution License (CC BY). The use, distribution or reproduction in other forums is permitted, provided the original author(s) and the copyright owner(s) are credited and that the original publication in this journal is cited, in accordance with accepted academic practice. No use, distribution or reproduction is permitted which does not comply with these terms.
*Correspondence: Paula I. Martin, cGF1bGFrJiN4MDAwNDA7YnUuZWR1