- 1Department of Rehabilitation Medicine, Pohang Stroke and Spine Hospital, Pohang-si, South Korea
- 2Department of Radiology, Pohang Stroke and Spine Hospital, Pohang-si, South Korea
- 3Department of Neurosurgery, Pohang Stroke and Spine Hospital, Pohang-si, South Korea
- 4Department of Neurology, Pohang Stroke and Spine Hospital, Pohang-si, South Korea
Background: Spinal cord infarction (SCI) is a rare disease and its early diagnosis is challenging. Here, we described the clinical features and imaging findings of SCI, and assessed the results of evoked potential (EP) studies to elucidate their diagnostic role in the early stage of SCI.
Methods: We retrospectively analyzed 14 patients who had spontaneous SCI. The demographic, neurological, and temporal profiles of the SCI patients were identified. We reviewed the imaging findings and assessed the changes in them over time. To review EP, central motor conduction time (CMCT) and somatosensory evoked potential (SEP) values were obtained. We also enrolled 15 patients with transverse myelitis (TM), and compared the clinical, radiological and electrophysiological features between SCI and TM patients.
Results: The ages of the SCI patients ranged from 54 to 73 years. Nine patients (64.3%) showed nadir deficits within 6 h. The most common type of clinical visit was via the emergency center. Nine patients (64.3%) presented with peri-onset focal pain. The median initial modified Rankin scale score was 3. For 9 patients (64.3%), initial T2 imaging findings were negative, but subsequent diffusion weighed imaging (DWI) showed diffusion restriction. Vertebral body infarction was observed in 5 patients (35.7%). EP data were available for 10 SCI patients. All 8 patients who had their CMCT measured showed abnormalities. Among them, motor evoked potentials were not evoked in 6 patients at all. SEP was measured in 10 patients, and 9 of them showed abnormalities; one of them showed no SEP response. For 5 patients, the EP studies were done prior to DWI, and all the patients showed definite abnormalities. The abnormalities in the EP findings of the SCI patients were more profound than those of the TM patients, even though the duration from the onset to the start of the study was much shorter for SCI patients.
Conclusion: SCI can be diagnosed based on typical clinical manifestations and appropriate imaging studies. Our study also indicates that immediate sensory and motor EP study can have an adjuvant diagnostic role in the hyperacute stage of SCI, and can improve the accuracy of diagnosis.
Introduction
Spinal cord infarction (SCI) is an uncommon cause of spinal cord injury, and has been reported to account for ~1.0% of all stroke cases (1, 2). SCI patients present with severe and diverse neurologic deficits depending on the anatomical location and the extent of the infarction. SCI patients generally experience an abrupt onset of motor weakness, loss of senses of pain and temperature, altered proprioception, and bowel and bladder dysfunctions. SCI has also been reported to have generally unfavorable outcomes (3, 4).
Early recognition of SCI immediately after the onset of symptoms is difficult; thus, SCI is considered a challenging disease (5). Differential diagnosis of SCI should exclude diseases such as acute inflammatory myelitis, multiple sclerosis, intra or extramedullary tumor, infectious conditions, and hematoma, which are characterized by features of spinal cord disorders; brain lesions and peripheral nerve lesions should be excluded as well (6). Transverse myelitis (TM) in particular should be excluded, as previous studies have reported that about 15% of patients diagnosed with TM were actually SCI patients (7, 8).
Although imaging has been an important tool for diagnosing SCI, studies have shown that early detection of SCI by imaging alone is difficult (9). Often, conventional magnetic resonance imaging (MRI) done after the onset of symptoms does not show any positive findings. Diffusion weighted imaging (DWI) is not generally performed together with the initial conventional MRI. Consequently, SCI is often masked in the hyperacute stage. Even if DWI is initially performed in cases of suspected SCI, it is possible that a diffusion MRI performed during the hyperacute stage will show false negative findings (10, 11). A standardized imaging protocol for the initial diagnosis of SCI has not been proposed yet. Thus, SCI is a disease with a high probability of delayed diagnosis or misdiagnosis in its early stage.
Evoked potential (EP) study has proven to be a useful diagnostic tool for evaluating the motor and sensory tracts of the spinal cord, and for diagnosing myelopathy (12–14). Central motor conduction time (CMCT) can mainly assess the corticospinal tract from the primary motor cortex to the spinal cord, and reflects the functions of not only the pyramidal tracts but the extrapyramidal tracts of the adjacent anterolateral columns (15). CMCT can be obtained by subtracting the peripheral motor conduction time from the onset latency of the motor evoked potential (MEP) induced by transcranial magnetic stimulation (16, 17). For the measurement of somatosensory evoked potential (SEP), stimuli originating from the peripheral nerve are mainly transmitted along the sensory pathway of the dorsal column of the spinal cord and the medial lemniscus of the brain stem, and are then finally recorded in the thalamus. SEP is a useful tool that takes the overall perfusion state into account, and is also useful for evaluating the function of the posterior column of the spine, which is difficult to detect using MEP alone (18, 19). Multimodal EP study, which utilizes both MEP and SEP simultaneously, is known to be a highly sensitive and specific testing method with a rapid response to abnormalities in the central neural pathway. However, research on how EP could play a distinct role in the early diagnosis of SCI is yet to be carried out.
In this study, we described the clinical features and the imaging findings of SCI patients admitted to our hospital. In addition, we analyzed the role of immediate sensory and motor EP study in the early diagnosis of SCI. We additionally elucidated the usefulness of EP study by comparing the EP results of TM patients with those of SCI patients, and we also identified the clinical and radiological differences between SCI and TM patients.
Materials and Methods
Study Design and Patient Inclusion
We conducted a retrospective observational study based on patient medical records, imaging findings, and electrodiagnostic test records. Out of the patients that visited our hospital from October 2011 to March 2020, we selected 16 patients who were diagnosed with spontaneous SCI. All patients underwent conventional spinal and brain MRI immediately after their visit according to their clinical symptoms. Following the MRI, patients suspected of SCI underwent spinal DWI and apparent diffusion coefficient (ADC) study. For SCI diagnosis, we utilized the diagnostic criteria proposed by Zalewski et al. (20) in 2019. Thereafter, we enrolled patients with definite or probable spontaneous SCI into our study. For this study, the diagnostic criteria for definite spontaneous SCI included: (1) no preceding myelopathy or traumatic lesion of the spinal cord; progression from onset to nadir within 12 h or less (onset to nadir); or severe deficits that rapidly developed within 12 h or less; (2) no spinal cord compression; (3) intramedullary high signal intensity (HSI) in T2 image; (4) one of the following: presence of diffusion restriction in DWI/ADC study, vertebral body infarction, arterial dissection, or occlusion of adjacent artery; and (5) differentiation from other similar diseases. The criteria for probable spontaneous SCI are similar to the aforementioned criteria; however, (4) is replaced with non-inflammatory findings of the CSF study. Two of the patients enrolled as SCI patients were excluded because they did not meet the criteria for definite or probable SCI. Thus, a total of 14 patients were finally selected for the study, and all of them were diagnosed with definite SCI.
To compare the clinical, radiological, and electrophysiological findings of SCI and TM patients, we enrolled 15 patients diagnosed with TM within the same sampling period. Our diagnostic criteria for inflammatory myelopathy were mainly based on the proposal released by the Transverse Myelitis Consortium Working Group in 2002 (21). The final diagnoses of both SCI and TM were made by experienced neurologists, neurosurgeons, and radiologists based on clinical features, imaging findings, and CSF study results.
Observation Items
To identify the clinical features of SCI, we reviewed demographic factors and vascular risk factors such as hypertension, diabetes mellitus, hyperlipidemia, and smoking. For each patient, we checked the motor, sensory, and autonomic symptoms at nadir, and checked for peri-onset focal adjacent pain. To describe functions and outcomes, we used the modified Rankin scale (mRS). To identify the temporal profiles of the clinical features, we categorized the duration from onset of symptoms to nadir into the following four categories: <6, 6–24, 24–72, and 72 h or more. We also determined the type of visit and the duration from the onset of symptoms to the time of the hospital visit. Initial serum inflammatory indexed–white blood cell (WBC) count, erythrocyte sedimentation rate (ESR), and C-reactive protein (CRP) level were also verified.
To check the characteristics and findings of imaging studies, we investigated the duration from the onset of symptoms to the completion of the first spinal MRI study and the presence of HSI in the initial T2 image. Subsequently, we determined the duration from the onset of symptoms to the completion of the DWI/ADC study, the presence of diffusion restriction, and the involved spinal levels. We also investigated the presence of vertebral body infarction.
To check the EP study, we adopted the values of CMCTs recorded from the abductor pollicis brevis muscle (APB-CMCT) and the tibialis anterior muscle (TA-CMCT). We also used the value of median SEP and tibial SEP. For patients who had saddle symptoms or hypo-reflexic bowel and bladder, we also conducted a pudendal SEP. Based on previous reports, the cut-off value was set at 8.8 ms for APB-CMCT and 17.2 ms for TA-CMCT (22). The cut-off value for latencies of median SEP, tibial SEP, and pudendal SEP were 20.3, 41.3, and 41.1 ms, respectively (23). All patients who underwent the EP study also underwent a routine nerve conduction study (NCS) and electromyography (EMG) to rule out other peripheral neuropathies, polyneuropathy or polyradiculopathy. We also measured the duration from the onset of symptoms to the completion of the electrodiagnostic study. To compare the EP results of the SCI and TM patients, of the 15 TM patients, we selected 10 who underwent EP testing.
We expressed continuous variables as median values (interquartile range, IQR), and categorical variables were expressed as number of patients and percentage values. The Mann-Whitney test was used to compare SCI and TM group data.
Results
Clinical Features
A total of 14 SCI patients were enrolled in this study. The ages of the patients ranged from 54 to 73 years. There were 8 male patients (57.1%). Eleven patients (78.6%) had one or more vascular risk factors, and hypertension, which 6 patients presented with, was the most common. Eleven patients (78.6%) had autonomic dysfunctions. The median score of the initial mRS test was 3 (2,4), indicating a relatively profound neurologic deficit. We were able to conduct a follow-up test with the mRS for 10 patients. Among them, 4 patients scored 3 or higher, indicating that they had limited functional independency in their daily life (Table 1).
The temporal profiles of the SCI patients were as follows: regarding the duration from the onset of symptoms to nadir, 9 patients (64.3%) presented with the hyperacute type (<6 h), making it the most common type recorded; for the type of hospital visit, 10 patients (71.4%) visited the hospital via the emergency center; and for the duration from the onset to the hospital visit, the first visit was made within 4 h [0.17 day [0.10, 1.00]]. The time it took to obtain the first T2 image was 8 h (0.33 day [0.14, 1.00]), and the duration from the onset to the completion of the diffusion MRI study was 2.75 days (1.26, 3.00). The duration from the onset to the acquisition of EP results was 1.17 days (0.92, 3.00) (Table 2).
On the other hand, the TM group showed significant differences compared to the SCI group, especially in terms of the temporal profile. In 13 patients (86.7%), it took more than 72 h to the nadir deficit. It took 5 days (3, 7) from the onset to the first hospital visit, and 10 patients (66.7%) visited the hospital out-patient clinic. Regarding the initial serum inflammatory indexes, there were no significant differences in ESR and WBC counts between the two groups. Meanwhile, CRP values were significantly higher in the TM group−0.08 (0.07, 0.13) in the SCI group and 0.21 (0.10, 0.59) in the TM group (P = 0.017) (Supplementary Table 1). All 14 SCI patients showed negative inflammatory findings in the CSF study.
MRI Findings
Table 3 shows the summary of the imaging characteristics of the SCI patients. HSI was present in the initial T2 images of 5 patients (35.7%). For these patients, their T2 images were taken 24 h or more after the onset. Nine patients (64.3%) did not have HSI in their initial T2 images; the T2 images of these patients were taken within 12 h from the onset. Gadolinium enhancement was not observed in any of the patients. All patients showed diffusion restriction in the DWI/ADC study (Figure 1). In our SCI patient group, the earliest time for a positive finding from the diffusion MRI study was 11 h from the onset (case 10). Vertebral body infarction was observed in 5 patients (35.7%) (Figure 2). Regarding the involved spinal levels, the lower thoracic level (T7–12) was the most invaded level, followed by the cervical level. The median value for the length of the invaded level in SCI group was 2 levels (1, 2). Meanwhile, the lesion length in the TM group was 3 levels (2, 4), which was significantly longer than that in the SCI group (P < 0.001) (Figure 3). Adjacent vascular evaluations for determining infarction etiology revealed aortic atherosclerosis with calcification in 2 cases and intercostal artery occlusion in 1 case. However, most patients had no accompanying vascular lesions.
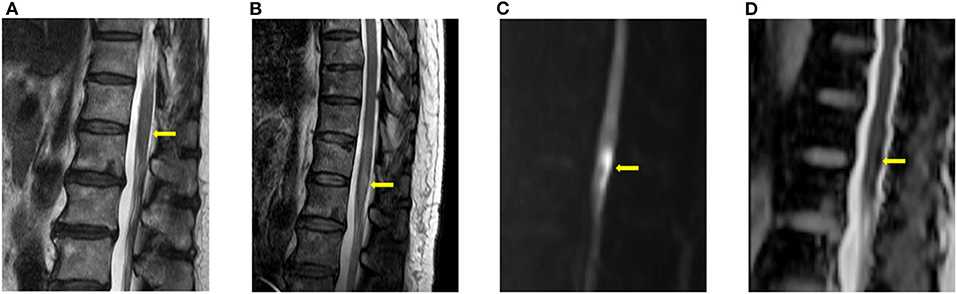
Figure 1. Typical imaging appearances of acute spinal cord infarction. These were images of a patient with conus medullaris infarction (case 4). (A) T2 image obtained 3 h after the onset of symptoms showed no high signal intensity around the conus level. (B) Follow-up T2 image obtained on the fourth day after the onset of symptoms showed high signal intensity around the conus level. Diffusion restriction was also observed in the diffusion weighted imaging (C) and the apparent diffusion coefficient (D) study.
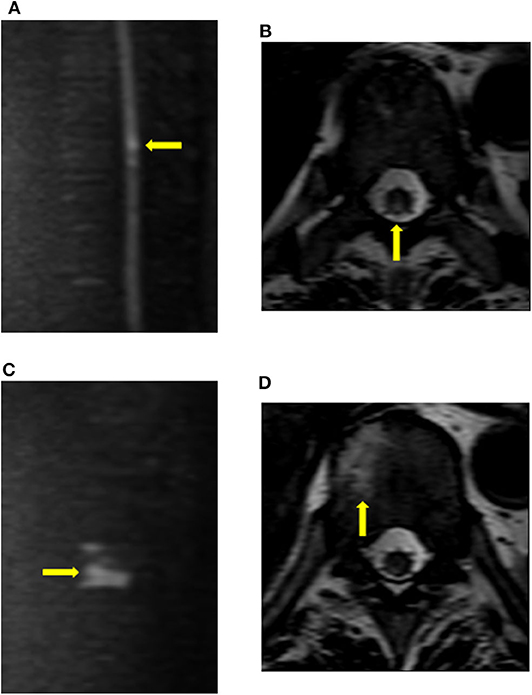
Figure 2. Findings of vertebral body infarction. The patient (case 9) underwent diffusion imaging on the fourth day after the onset of symptoms. (A,B) Diffusion restriction was mainly observed in the posterior column of the T9 cord level. (C,D) Abnormal signals, which were consistent with vertebral body infarction, were observed within the right vertebral body of T10.
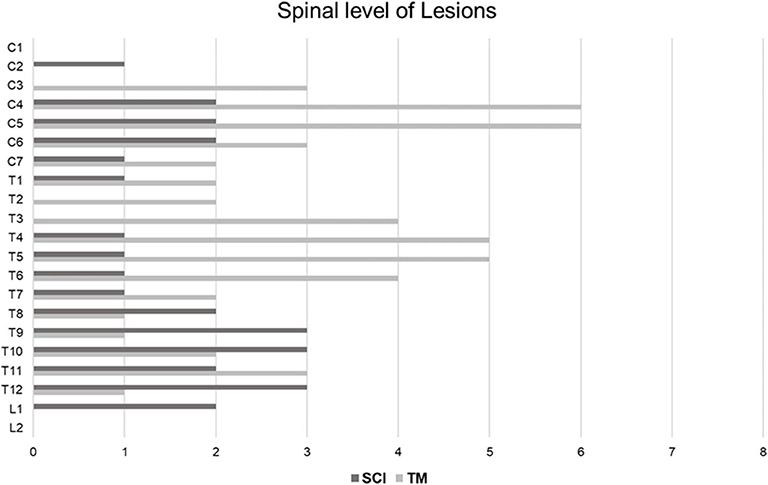
Figure 3. Distribution of SCI and TM at the spinal levels. In the SCI group, a total of 28 spinal levels of all 14 patients were involved. On the other hand, a total of 52 spinal levels of all 15 patients were involved in the TM group. Therefore, the lesion length of the SCI group was shorter than that of the TM group (P = 0.005). SCI occurred most frequently in the lower thoracic level, followed by the mid-cervical level. TM was mainly distributed across the cervical and upper thoracic levels. SCI, spinal cord infarction; TM, transverse myelitis.
Evoked Potentials
Table 4 summarizes the EP results of all patients. Initial electrodiagnostic data including EP, NCS, and EMG values were available for 10 patients. All of them showed one or more EP abnormalities, such as delayed values or no EP response.
All 8 patients who were measured for CMCT had abnormal results. The APB-CMCT results of 3 patients were available, and all of them had prolonged APB-CMCTs. The TA-CMCT results of 7 patients were available; among them, 1 patient had a prolonged TA-CMCT, and 6 patients showed no tibial MEP response. SEP was measured in 10 patients, and 8 of them showed prolonged latencies. The median SEP of case 1 was within the normal range, but her APB-CMCT was delayed. Case 6 had no response for both TA-CMCT and tibial SEP measured on the tenth day from the onset. Three patients underwent the pudendal SEP study, and all of them had values within the normal range of latency.
Regarding the time frames, 7 patients completed the EP study within 48 h from the onset; all of them had abnormal findings. For 5 patients, electrodiagnostic studies were completed prior to the diffusion MRI study, and all of them showed pronounced EP abnormalities. Among them, 3 patients showed both prolonged SEP latency and no TA-MEP responses (case 2, 4, 8). For case 4, TA-MEP was not evoked despite the examination being done just 16 h after the onset. The patient had no peroneal nerve lesion or any L5 root lesion confirmed with NCS and EMG. For 3 patients, though their EP findings were obtained after DWI, both studies were conducted the same day and within a short period. Among these patients, 2 showed both CMCT and SEP abnormalities (case 5, 12). For case 13, the electrodiagnostic test was performed only 4 h after the onset. Out of all the patients, the patient in this case had the shortest duration before the EP study was performed, and the results showed prolonged tibial SEP latency.
On the other hand, for TM patients, it took more than 10 days (5.50, 18.25) from the onset to perform the electrodiagnostic testing; this duration was longer than that of SCI patients. Time of testing also varied widely from patient to patient. We were able to obtain routine NCS, EMG, and EP results for 10 TM patients. Among them, 9 patients had delayed EP values. Abnormalities in both CMCT and SEP were observed in 4 patients. Two patients showed abnormalities only in CMCT. There were no cases in which the EP was not evoked. We also compared the tibial SEP latency between SCI and TM patients. Data for 8 SCI patients and 9 TM patients were available for the analysis. The median value in the SCI group and the TM group was 43.35 ms (41.45, 45.83) and 42.50 ms (38.10, 43.15), respectively. Thus, tibial SEP latency in the SCI group was slightly more prolonged than that of the TM group; however, the difference was not statistically significant (P = 0.370).
Discussion
A distinctive feature of the present study is that it suggests the possibility of using EP study as an additional diagnostic tool for identifying acute SCI. Our results indicate that the immediate sensory and motor EP test can be a useful diagnostic tool for identifying SCI in its early stage. We also aimed to elucidate the usefulness of EP by comparing the EP results of TM patients with those of SCI patients.
By assessing the findings of SCI patients who underwent EP studies within a relatively short time from the onset of symptoms, we observed the following: (1) some MEPs were not evoked; (2) definite abnormalities were noted in both CMCT and SEP in many cases. These findings indicate that a spinal cord lesion triggered by SCI could be detected in a relatively short time using EP testing (17). Five patients even showed clearly abnormal EP results after electrodiagnostic testing, which was done prior to the diffusion MRI study. Although the electrodiagnostic study was done after DWI, 3 patients underwent both tests on the same day, and they too had abnormal EP findings. This means that the likelihood of SCI is higher if, in addition to clinical suspicion, profound EP abnormalities are observed in early electrodiagnostic testing. With an EP study, clinicians can provide an additional basis for conducting a diffusion MRI study for patients suspected to have SCI. Consequently, delays in both diagnosis and misdiagnosis can possibly be reduced.
In the TM group, most patients presented with delays in EP; however, there were no cases in which EP was not evoked. Although the duration from the onset to the completion of testing was shorter for SCI patients than for TM patients, the EP abnormalities of SCI patients were more severe than those of TM patients. Therefore, the early EP findings were also useful for differential diagnosis.
In previous animal studies, histologic changes were generally observed 48 h after induction of cord infarction. Profound gray matter (GM) and white matter (WM) injuries accompanied by neuronal cell damage and a decline in cell numbers were observed, and the boundary between the two areas also became steadily unclear (24, 25). The abnormal spinal cord conduction velocity findings may be primarily related to WM injuries accompanied by myelinated axonal damage, which is known to be eventually correlated with the extent of GM injuries (26, 27). Ultimately, such findings indicate that CMCT could be adopted for the early detection of not only WM injuries but also GM lesions of the spinal cord, which is relatively more sensitive to ischemia. In addition, previous studies have reported that CMCT abnormalities could reflect early microscopic ischemic changes that are not identified by MRI (14, 28). Experimental studies have also demonstrated that MEP responses to ischemia are sensitive and immediate. Tsuda et al. (25) induced spinal infarction in dogs using aortic balloon occlusion and checked for changes in MEP. They discovered that transcranial MEP amplitude reduced by 50% in about 6 min, and completely disappeared in around 7 min. Moreover, apart from MEP providing real-time feedback, it has already been revealed in animal and clinical studies that these changes are correlated with functional outcomes (29–31). Therefore, MEP study can be viewed as a significantly useful tool for the immediate diagnosis of spinal cord injuries.
SEP response to ischemic damage has been shown to be less sensitive than that of MEP, and it is also affected by other factors such as hypotension and low temperature (15, 32, 33). Therefore, it has been often viewed as being less useful than MEP. Additionally, one study reported that the correlation of SEP with motor function was poor, because it mainly reflects the function of the sensory pathway of the posterior column (34). However, from an anatomical perspective, the ascending sensory pathway of the spinal cord is adjacent to the pyramidal tracts. Moreover, other studies have demonstrated that SEP is correlated with motor outcomes related to ischemic injury (35). This is also supported by the distribution of the vascular supply of the spinal cord. The anterior and lateral regions of the spinal cord are mainly supplied by the central and radicular arteries derived from the anterior spinal artery. Meanwhile, the posterior spinal arteries (PSAs) mainly supply not only the posterior column but also the posterior part of the lateral column where the descending motor tracts pass through (36). In addition, the distributions of vascular territories partially overlap, and approximately one third of the entire transection area of the spinal cord is considered to be an overlapping area (37). Therefore, an adjacent motor tract lesion may be identified based on SEP abnormalities. In cases of SCI with pure PSA infarction accompanied by severe sensory symptoms, abnormalities may be observed only in the SEP. Therefore, it is critical to perform both SEP and MEP studies at the same time during the initial assessment of SCI.
As has been described thus far, since MEP and SEP responses to ischemia and reperfusion are rapid, both tests have been applied as key modalities of intraoperative neurophysiological monitoring (IONM) during spinal surgeries (15, 32). IONM requires neural insults of the spinal cord to be assessed in real-time; therefore, complementary MEP and SEP monitoring is suitable for this. Regarding the early diagnosis of SCI, performing sensory and motor EP studies prior to checking for imaging changes in the spinal cord has the advantage of not only having a diagnostic role, but of also being a useful way to assess the extent of spinal cord injury.
For early diagnosis of SCI using EP, it is also important to perform the electrodiagnostic test quickly and report the results immediately. With regard to the time of onset to hospital visit and onset to initial T2 imaging, it was indicated that an MRI was primarily considered in most patients who visited with acute spinal cord syndrome. In our institute, for patients with symptoms of acute spinal cord syndrome, electrodiagnostic tests are generally requested after MRI for verifying neurophysiologic integrity and differential diagnosis. Based on our results, the time difference between the initial MRI and the EP test was within 24 h. Moreover, it can be considered that EP study is highly useful, because it is relatively easy and quick to perform.
In this study, we also described the clinical features and imaging findings of SCI patients. Similar to the findings of previous studies, the SCI patients in this study presented with acute clinical symptoms that worsened rapidly (20, 37). Of the 14 patients in our study, 9 had a rapid progression time of <6 h. A previous report suggests that, in order to diagnose patients with SCI, the duration from the onset of symptoms to nadir should be <72 h (10). All patients in the present study showed a nadir deficit within 72 h from the onset.
SCI occurs most frequently in cases of mid to lower thoracic lesions, and 2 patients in our study developed infarction in their conus medullaris. This area is supplied by the artery of Adamkiewicz and is vulnerable not only to ischemia caused by aortic lesions but also to spontaneous infarctions. It has been known to have a higher metabolic demand than other spinal levels, and thus is more susceptible to ischemia (38–40). Previous studies have also reported that SCI is mainly distributed across the lower thoracic, cervical, and conus levels (8). Thus, the results of the SCI group in the present study are in line with the findings of previous studies. TM on the other hand, is mainly distributed across the cervical and upper thoracic levels. Therefore, the distribution pattern has been emphasized as a feature that could help distinguish SCI from acute inflammatory myelitis, which has a longer lesion length than SCI (5).
In the present study, peri-onset focal adjacent pain was observed in 64.3% of the patients. This rate was similar to or slightly higher than those reported in previous studies (5, 41). Focal adjacent pain is mainly caused by infarction of the associated nerve root or plexus; other causes are vertebral body or meningeal ischemia (42, 43). Mostly, focal adjacent pain manifests as radicular pain around the involved spinal level. In the present study, patients who had thoracic level infarction complained of vague abdominal pain or chest pain. Due to these anatomical concerns, focal pain was not necessarily consistent with the abnormalities of median or tibial SEP in our patient group. Though more than half of the patients with SCI had focal adjacent pain, it was also accompanied with not only inflammatory myelitis, but degenerative spinal disease, arthritis or underlying peripheral nerve diseases (38). Thus, caution needs to be taken before reaching a definite diagnosis.
All our SCI patients showed diffusion restriction in the DWI/ADC study. The majority of the initial T2 studies were performed within 12 h of onset, and all the initial T2 images showed no HSI. Since all patients showed diffusion restriction in the subsequent diffusion MRI study, it can be concluded that it is not easy to diagnose SCI based on an initial T2 image. Vertebral body infarction is known as a confirmatory sign of SCI, and it was observed in 35.7% of the patients in the present study. This rate was similar to those of previous reports (5, 44).
In the present study, 2 patients received delayed diagnoses. In case 1, the abrupt onset of symptoms started with right arm weakness; however, the patient had chronic pain in the right shoulder and mistakenly attributed the weakness of the right arm to the aggravation of a shoulder lesion. Thus, the patient did not visit the hospital on time; the hospital visit was made 3 days after the onset of symptoms. We diagnosed the patient with SCI after conducting T2 MRI and DWI/ADC study at the same time. In case 9, the patient had a chronic right multilevel herniated lumbar disc. At the time of the visit, the only complaint was of radiating pain, but subtle dorsiflexor weakness of the right ankle developed on the second day of admission. We diagnosed the patient with exacerbation of L5 root lesion after performing NCS and EMG; thereafter, we proceeded with a diskectomy, but the patient did not show any subsequent signs of recovery. On the fourth day of admission, the patient suddenly complained of paresthesia, voiding difficulty, hip flexion, and abduction weakness that was rapidly progressing on both sides. We subsequently performed MRI and a DWI/ADC study and diagnosed the patient with SCI at the T9-10 level. CSF analysis conducted immediately after the MRI also presented no evidence of inflammatory changes. This patient experienced focal adjacent pain first, and then focal weakness for a while, after which there was a sudden progression to severe neurologic symptoms. On the MRI performed after the onset of nadir symptoms, we discovered diffusion restriction at the lower thoracic cord level, accompanied by vertebral body infarction.
A proven effective acute treatment for SCI is yet to be established (45). Antiplatelets and anticoagulants have been reported to be administered, but the focus has been on secondary prevention rather than revascularization (46–48). In many cases, steroids were administered simultaneously. The main reason for the administration of steroids is that it is difficult to completely exclude inflammatory myelitis in the early stages of SCI (10, 49). Unlike the case of cerebral infarctions, an acute phase thrombolytic treatment protocol has not been standardized for SCI. Several case reports and ongoing clinical trials have been released, and a common emphasis in the reports is that acute thrombolytic treatment could be performed in a timely manner only if an accurate diagnosis is made prior to treatment (50–53). Therefore, should a systematic acute phase treatment process be established in the future, the importance of an accurate early diagnosis of SCI will be emphasized even more. Based on our results, an immediate sensory and motor EP study performed together with a review of clinical symptoms can provide clues to facilitate the diagnosis and confirmation of SCI prior to the performance of a diffusion MRI study. Furthermore, an EP study can be a useful base study for well-established therapeutic decisions in the future.
This study has a few limitations. This was a retrospective study based on the experience of a single center, and was conducted with a small sample size. In addition, because our sampling period was over 8 years, not all patients underwent the same standardized examination. Furthermore, as seen in the study results, not all patients underwent EP testing. In each patient group, only 10 patients completed electrodiagnostic tests, including EP. Therefore, we were not able to prove whether the results of the EP test had any significant statistical meaning. In the future, systematic researches should be conducted using well-controlled cohorts.
In conclusion, although SCI is rare and difficult to identify immediately, it can be diagnosed through the review of typical characteristics of clinical features and imaging findings. From our observations, we were also able to identify severe EP abnormalities in SCI patients, even in the hyperacute stage of the disease. Therefore, we suggest that for suspected SCI cases, conducting an immediate sensory and motor EP study in a timely manner can improve diagnostic accuracy and speed.
Data Availability Statement
The raw data supporting the conclusions of this article will be made available by the authors, without undue reservation.
Ethics Statement
The studies involving human participants were reviewed and approved by the Institutional Review Board of Pohang Stroke and Spine Hospital reviewed and approved this study (Approval No. PSSH0475-202004-HR005). Written informed consent for participation was not required for this study in accordance with the national legislation and the institutional requirements. Written informed consent was obtained from the individual(s) for the publication of any potentially identifiable images or data included in this article.
Author Contributions
DP and SEL: writing the manuscript, data collection, and interpretation. BHK, JKP, and JMC: data analysis, diagnosis, and procedures of patients. HDK and SYL: editing the manuscript and supervising the entire study. All authors reviewed the manuscript and were in full agreement to submission.
Conflict of Interest
The authors declare that the research was conducted in the absence of any commercial or financial relationships that could be construed as a potential conflict of interest.
Supplementary Material
The Supplementary Material for this article can be found online at: https://www.frontiersin.org/articles/10.3389/fneur.2020.563553/full#supplementary-material
References
1. Romi F, Naess H. Spinal cord infarction in clinical neurology: a review of characteristics and long-term prognosis in comparison to cerebral infarction. Eur Neurol. (2016) 76:95–8. doi: 10.1159/000446700
2. Salvador de la Barrera S, Barca-Buyo A, Montoto-Marques A, Ferreiro-Velasco ME, Cidoncha-Dans M, Rodriguez-Sotillo A. Spinal cord infarction: prognosis and recovery in a series of 36 patients. Spinal Cord. (2001) 39:520–5. doi: 10.1038/sj.sc.3101201
3. Sandson TA, Friedman JH. Spinal cord infarction. Report of 8 cases and review of the literature. Medicine. (1989) 68:282–92. doi: 10.1097/00005792-198909000-00003
4. Hanson SR, Romi F, Rekand T, Naess H. Long-term outcome after spinal cord infarctions. Acta Neurol Scand. (2015) 131:253–7. doi: 10.1111/ane.12343
5. Hsu JL, Cheng MY, Liao MF, Hsu HC, Weng YC, Chang KH, et al. A comparison between spinal cord infarction and neuromyelitis optica spectrum disorders: clinical and MRI studies. Sci Rep. (2019) 9:7435. doi: 10.1038/s41598-019-43606-8
7. Zalewski NL, Flanagan EP, Keegan BM. Evaluation of idiopathic transverse myelitis revealing specific myelopathy diagnoses. Neurology. (2018) 90:e96–e102. doi: 10.1212/WNL.0000000000004796
8. Barreras P, Fitzgerald KC, Mealy MA, Jimenez JA, Becker D, Newsome SD, et al. Clinical biomarkers differentiate myelitis from vascular and other causes of myelopathy. Neurology. (2018) 90:e12–21. doi: 10.1212/WNL.0000000000004765
9. Pawar NH, Loke E, Aw DC. Spinal cord infarction mimicking acute transverse myelitis. Cureus. (2017) 9:e1911. doi: 10.7759/cureus.1911
10. Yadav N, Pendharkar H, Kulkarni GB. Spinal cord infarction: clinical and radiological features. J Stroke Cerebrovasc Dis. (2018) 27:2810–21. doi: 10.1016/j.jstrokecerebrovasdis.2018.06.008
11. Pikija S, Mutzenbach JS, Kunz AB, Nardone R, Leis S, Deak I, et al. Delayed hospital presentation and neuroimaging in non-surgical spinal cord infarction. Front Neurol. (2017) 8:143. doi: 10.3389/fneur.2017.00143
12. Misra UK, Kalita J. Transverse myelitis–neurophysiological and MRI correlation. Paraplegia. (1994) 32:593–6. doi: 10.1038/sc.1994.94
13. MacDonald DB, Dong C, Quatrale R, Sala F, Skinner S, Soto F, et al. Recommendations of the international society of intraoperative neurophysiology for intraoperative somatosensory evoked potentials. Clin Neurophysiol. (2019) 130:161–79. doi: 10.1016/j.clinph.2018.10.008
14. Chen R, Cros D, Curra A, Di Lazzaro V, Lefaucheur JP, Magistris MR, et al. The clinical diagnostic utility of transcranial magnetic stimulation: report of an IFCN committee. Clin Neurophysiol. (2008) 119:504–32. doi: 10.1016/j.clinph.2007.10.014
15. Sloan TB, Jameson LC. Electrophysiologic monitoring during surgery to repair the thoraco-abdominal aorta. J Clin Neurophysiol. (2007) 24:316–27. doi: 10.1097/WNP.0b013e31811ebc66
16. Imajo Y, Kanchiku T, Suzuki H, Funaba M, Nishida N, Taguchi T. Utility of the central motor conduction time recorded from the abductor pollicis brevis and the abductor digiti minimi muscles in patients with C6-7 myelopathy. J Spinal Cord Med. (2018) 41:182–91. doi: 10.1080/10790268.2017.1322673
17. Nardone R, Holler Y, Thomschewski A, Holler P, Bergmann J, Golaszewski S, et al. Central motor conduction studies in patients with spinal cord disorders: a review. Spinal Cord. (2014) 52:420–7. doi: 10.1038/sc.2014.48
18. Elmore JR, Gloviczki P, Harper CM Jr, Murray MJ, Wu QH, Boer TC, et al. Spinal cord injury in experimental thoracic aortic occlusion: investigation of combined methods of protection. J Vasc Surg. (1992) 15:789–98. doi: 10.1016/0741-5214(92)90713-I
19. Laschinger JC, Cunningham JN Jr, Cooper MM, Baumann FG, Spencer FC. Monitoring of somatosensory evoked potentials during surgical procedures on the thoracoabdominal aorta. I. Relationship of aortic cross-clamp duration, changes in somatosensory evoked potentials, and incidence of neurologic dysfunction. J Thorac Cardiovasc Surg. (1987) 94:260–5. doi: 10.1016/S0022-5223(19)36291-9
20. Zalewski NL, Rabinstein AA, Krecke KN, Brown RD Jr, Wijdicks EFM, Weinshenker BG, et al. Characteristics of spontaneous spinal cord infarction and proposed diagnostic criteria. JAMA Neurol. (2019) 76:56–63. doi: 10.1001/jamaneurol.2018.2734
21. Transverse Myelitis Consortium Working Group. Proposed diagnostic criteria and nosology of acute transverse myelitis. Neurology. (2002) 59:499–505. doi: 10.1212/WNL.59.4.499
22. Jaiser SR, Barnes JD, Baker SN, Baker MR. A multiple regression model of normal central and peripheral motor conduction times. Muscle Nerve. (2015) 51:706–12. doi: 10.1002/mus.24427
23. Dumitru D, Amato AA, Zwarts MJ. Electrodiagnostic Medicine. 2nd Edn. Philadelphia, PA: Hanley & Belfus (2002). p. 357–408.
24. Basoglu H, Kurtoglu T, Cetin NK, Bilgin MD, Kiylioglu N. Assessment of in vivo spinal cord conduction velocity in rats in an experimental model of ischemic spinal cord injury. Spinal Cord. (2013) 51:616–22. doi: 10.1038/sc.2013.40
25. Tsuda K, Shiiya N, Takahashi D, Ohkura K, Yamashita K, Kando Y, et al. Transesophageal versus transcranial motor evoked potentials to monitor spinal cord ischemia. J Thorac Cardiovasc Surg. (2016) 151:509–17. doi: 10.1016/j.jtcvs.2015.08.120
26. Stys PK. Anoxic and ischemic injury of myelinated axons in CNS white matter: from mechanistic concepts to therapeutics. J Cereb Blood Flow Metab. (1998) 18:2–25. doi: 10.1097/00004647-199801000-00002
27. Kurita N, Kawaguchi M, Horiuchi T, Inoue S, Sakamoto T, Nakamura M, et al. An evaluation of white matter injury after spinal cord ischemia in rats: a comparison with gray matter injury. Anesth Analg. (2005) 100:847–54. doi: 10.1213/01.ANE.0000146523.56647.5E
28. Cohen-Adad J, Buchbinder B, Oaklander AL. Cervical spinal cord injection of epidural corticosteroids: comprehensive longitudinal study including multiparametric magnetic resonance imaging. Pain. (2012) 153:2292–9. doi: 10.1016/j.pain.2012.07.028
29. Lu Y, LV B, Song Q. Transcranial electrical stimulation motor-evoked potentials in a spinal cord ischaemia rabbit model. Chin Neurosurg J. (2019) 5:1–8. doi: 10.1186/s41016-019-0174-7
30. Hattori K, Yoshitani K, Kato S, Kawaguchi M, Kawamata M, Kakinohana M, et al. Association between motor-evoked potentials and spinal cord damage diagnosed with magnetic resonance imaging after thoracoabdominal and descending aortic aneurysm repair. J Cardiothorac Vasc Anesth. (2019) 33:1835–42. doi: 10.1053/j.jvca.2018.12.004
31. Lee WH, Lee SU, Jung SH. Ischemic cervical myelopathy caused by vertebral artery dissection: the clinical utility of a motor-evoked potential study. Neurologist. (2016) 21:8–10. doi: 10.1097/NRL.0000000000000066
32. Stecker MM. A review of intraoperative monitoring for spinal surgery. Surg Neurol Int. (2012) 3:S174–87. doi: 10.4103/2152-7806.98579
33. Kang H, Gwak HS, Shin SH, Woo MK, Jeong IH, Yoo H, et al. Monitoring rate and predictability of intraoperative monitoring in patients with intradural extramedullary and epidural metastatic spinal tumors. Spinal Cord. (2017) 55:906–10. doi: 10.1038/sc.2017.43
34. Gonzalez-Fajardo JA, Toledano M, Alvarez T, Vaquero C. Monitoring of evoked potentials during spinal cord ischaemia: experimental evaluation in a rabbit model. Eur J Vasc Endovasc Surg. (1998) 16:320–8. doi: 10.1016/S1078-5884(98)80051-8
35. Aminoff MJ. The clinical role of somatosensory evoked potential studies: a critical appraisal. Muscle Nerve. (1984) 7:345–54. doi: 10.1002/mus.880070502
36. Gailloud P. Spinal vascular anatomy. Neuroimaging Clin N Am. (2019) 29:615–33. doi: 10.1016/j.nic.2019.07.007
37. Ogawa K, Akimoto T, Hara M, Morita A, Fujishiro M, Suzuki Y, et al. Clinical study of thirteen patients with spinal cord infarction. J Stroke Cerebrovasc Dis. (2019) 28:104418. doi: 10.1016/j.jstrokecerebrovasdis.2019.104418
38. Cheng MY, Lyu RK, Chang YJ, Chen RS, Huang CC, Wu T, et al. Spinal cord infarction in Chinese patients. Clinical features, risk factors, imaging and prognosis. Cerebrovasc Dis. (2008) 26:502–8. doi: 10.1159/000155988
39. Yuh WT, Marsh EE 3rd, Wang AK, Russell JW, Chiang F, Koci TM, et al. MR imaging of spinal cord and vertebral body infarction. AJNR Am J Neuroradiol. (1992) 13:145–54.
41. Nelson JA, Ho CY, Golomb MR. Spinal cord stroke presenting with acute monoplegia in a 17-year-old tennis player. Pediatr Neurol. (2016) 56:76–9. doi: 10.1016/j.pediatrneurol.2015.11.007
42. Monteiro L, Leite I, Pinto JA, Stocker A. Spontaneous thoracolumbar spinal cord infarction: report of six cases. Acta Neurol Scand. (1992) 86:563–6. doi: 10.1111/j.1600-0404.1992.tb05487.x
43. Cheshire WP Jr. Spinal cord infarction mimicking angina pectoris. Mayo Clin Proc. (2000) 75:1197–9. doi: 10.4065/75.11.1197
44. Suzuki T, Kawaguchi S, Takebayashi T, Yokogushi K, Takada J, Yamashita T. Vertebral body ischemia in the posterior spinal artery syndrome: case report and review of the literature. Spine. (2003) 28:E260–4. doi: 10.1097/01.BRS.0000067285.39466.FB
45. Nasr DM, Rabinstein A. Spinal cord infarcts: risk factors, management, and prognosis. Curr Treat Options Neurol. (2017) 19:28. doi: 10.1007/s11940-017-0464-3
46. Mathias E, Sethuraman U. Ischemic stroke of the spinal cord: a pediatric emergency in an otherwise healthy child. J Emerg Med. (2016) 51:73–6. doi: 10.1016/j.jemermed.2016.03.001
47. Nance JR, Golomb MR. Ischemic spinal cord infarction in children without vertebral fracture. Pediatr Neurol. (2007) 36:209–16. doi: 10.1016/j.pediatrneurol.2007.01.006
48. Fortuna A, Ferrante L, Acqui M, Trillo G. Spinal cord ischemia diagnosed by MRI. Case report and review of the literature. J Neuroradiol. (1995) 22:115–22.
49. Beh SC, Greenberg BM, Frohman T, Frohman EM. Transverse myelitis. Neurol Clin. (2013) 31:79–138. doi: 10.1016/j.ncl.2012.09.008
50. Restrepo L, Guttin JF. Acute spinal cord ischemia during aortography treated with intravenous thrombolytic therapy. Tex Heart Inst J. (2006) 33:74–7.
51. Sakurai T, Wakida K, Nishida H. Cervical posterior spinal artery syndrome: a case report and literature review. J Stroke Cerebrovasc Dis. (2016) 25:1552–6. doi: 10.1016/j.jstrokecerebrovasdis.2016.02.018
52. Muller KI, Steffensen LH, Johnsen SH. Thrombolysis in anterior spinal artery syndrome. BMJ Case Rep. (2012) 2012:bcr2012006862. doi: 10.1136/bcr-2012-006862
Keywords: spinal cord infarction, early diagnosis, motor evoked potential (MEP), somatosensory evoked potential (SEP), diffusion MRI, transverse myelitis
Citation: Park D, Kim BH, Lee SE, Park JK, Cho JM, Kwon HD and Lee SY (2020) Spinal Cord Infarction: A Single Center Experience and the Usefulness of Evoked Potential as an Early Diagnostic Tool. Front. Neurol. 11:563553. doi: 10.3389/fneur.2020.563553
Received: 19 May 2020; Accepted: 14 August 2020;
Published: 27 October 2020.
Edited by:
Ping Zhou, University of Texas Health Science Center at Houston, United StatesReviewed by:
Antonio Currà, Sapienza University of Rome, ItalyNicholas Lawrence Zalewski, Mayo Clinic, United States
Terrence Thomas, KK Women's and Children's Hospital, Singapore
Copyright © 2020 Park, Kim, Lee, Park, Cho, Kwon and Lee. This is an open-access article distributed under the terms of the Creative Commons Attribution License (CC BY). The use, distribution or reproduction in other forums is permitted, provided the original author(s) and the copyright owner(s) are credited and that the original publication in this journal is cited, in accordance with accepted academic practice. No use, distribution or reproduction is permitted which does not comply with these terms.
*Correspondence: Su Yun Lee, c3VueXU4MDRAZ21haWwuY29t