- 1Division of Biokinesiology and Physical Therapy, University of Southern California, Los Angeles, CA, United States
- 2Department of Neurology, Keck School of Medicine, University of Southern California, Los Angeles, CA, United States
There is growing evidence that after a stroke, sensorimotor deficits in the ipsilesional hand are related to the degree of impairment in the contralesional upper extremity. Here, we asked if the relationship between the motor capacities of the two hands differs based on the side of stroke. Forty-two pre-morbidly right-handed chronic stroke survivors (left hemisphere damage, LHD = 21) with mild-to-moderate paresis performed distal items of the Wolf Motor Function Test (dWMFT). We found that compared to RHD, the relationship between contralesional arm impairment (Upper Extremity Fugl-Meyer, UEFM) and ipsilesional hand motor capacity was stronger ( 0.42; < 0.01; z = 2.12; p = 0.03) and the slope was steeper (t = −2.03; p = 0.04) in LHD. Similarly, the relationship between contralesional dWMFT and ipsilesional hand motor capacity was stronger ( 0.65; = 0.09; z = 2.45; p = 0.01) and the slope was steeper (t = 2.03; p = 0.04) in LHD compared to RHD. Multiple regression analysis confirmed the presence of an interaction between contralesional UEFM and side of stroke (β3 = 0.66 ± 0.30; p = 0.024) and between contralesional dWMFT and side of stroke (β3 = −0.51 ± 0.34; p = 0.05). Our findings suggest that the relationship between contra- and ipsi-lesional motor capacity depends on the side of stroke in chronic stroke survivors with mild-to-moderate impairment. When contralesional impairment is more severe, the ipsilesional hand is proportionally slower in those with LHD compared to those with RHD.
Introduction
It is now well-known that unilateral stroke not only results in weakness of the opposite half of the body, i.e., contralateral to the lesion or contralesional limb, but also significant motor deficits in the same half of the body, i.e., ipsilateral to the lesion or ipsilesional limb (1–4). Previous work suggests that deficits in the ipsilesional arm and hand varies with the severity of contralesional deficits, especially in the sub-acute and chronic phase after stroke (5–8). More interestingly, the unilateral motor deficits observed for contralesional and ipsilesional limbs seem to be hemisphere-specific and thus depend on side of stroke lesion (9–15). For predominantly right-handed cohorts, contralesional deficits appear to be more severe in those with right hemisphere damage (RHD), in whom the contralesional limb is non-dominant. For example, using clinical motor assessments of grip strength and hand dexterity, Harris and Eng (11) showed that contralesional motor impairments were less severe in chronic stroke survivors who suffered damage in the dominant (i.e., left) hemisphere (LHD) compared to those who suffered damage in the non-dominant (right) hemisphere (11, 15).
In contrast, considering ipsilesional motor deficits, the evidence is mixed concerning hemisphere-specific effects. For instance, some studies reported that individuals with LHD exhibited more severe ipsilesional arm and hand deficits compared to those with RHD (4, 15–17) while others have reported no difference in ipsilesional hand motor capacity between LHD and RHD (2). In acute stroke survivors, Nowak et al. demonstrated that deficits in grip force of the ipsilesional hand were significantly associated with clinical measures of function of the contralesional hand only in LHD (12). Contrary to this, de Paiva Silva et al. (14) found that compared to controls and LHD, the ipsilesional hand in chronic stroke survivors was significantly slower and less smooth in RHD especially when contralesional impairment was relatively more severe (UEFM < 34).
Taken together, there is converging evidence regarding the relationship between motor deficits of the contralesional and ipsilesional upper extremity, such that ipsilesional deficits are worse when contralesional impairment is greater (Figure 1A); however, it is uncertain whether the relationship between the two limbs depends on which hemisphere is damaged. In particular, motor deficits of the two limbs are most prominent for tasks that require dexterous motor control (e.g., grip force, tapping, tracking). For predominantly right-handed cohorts (as is the case in most studies), contralesional deficits appear to be more severe in those with RHD, in whom the contralesional limb is non-dominant; whereas ipsilesional deficits are more severe in those with LHD. An exception to this observation for those with RHD seems to be in the case when contralesional impairment is most severe (i.e., UEFM < 34) (14). Thus, one might predict that as contralesional impairment worsens, individuals with LHD would have proportionally worse ipsilesional deficits, but individuals with RHD (especially if say UEFM > 34) would not; see Figures 1B,C for two alternative hypotheses. To our knowledge, this prediction has not before been explicitly tested.
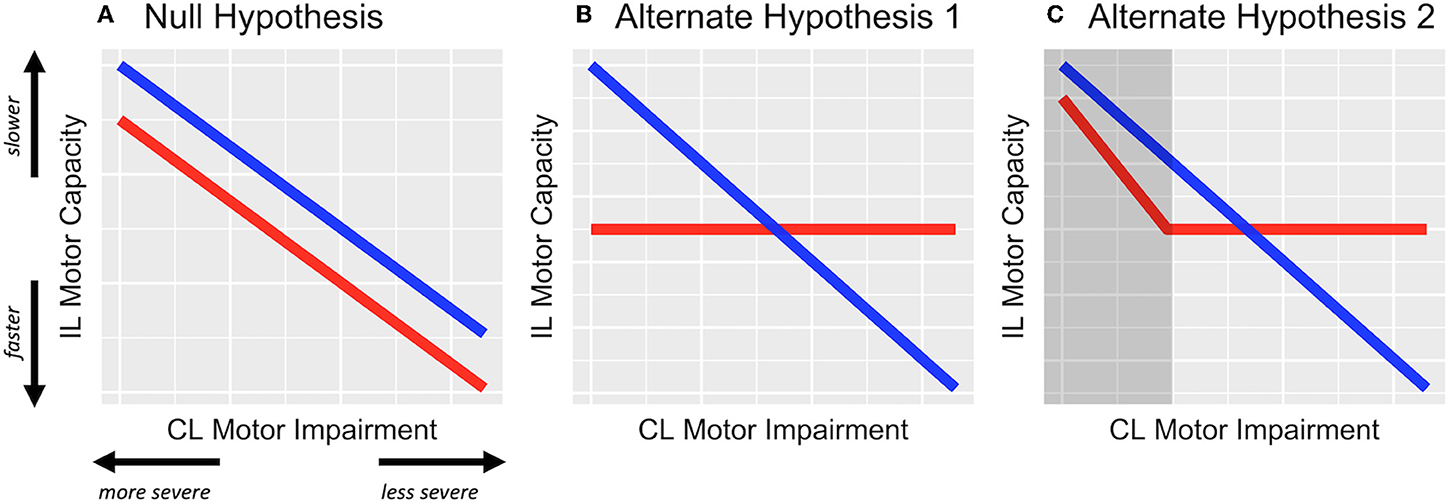
Figure 1. Hypothesized effects represented in schematic figure. (A) The null hypothesis, wherein the relationship between contralesional (CL) impairment and ipsilesional (IL) motor capacity is not modified by the side of stroke lesion, i.e., β1 ≠ 0 but β3 = 0. (B) Alternative hypothesis 1, wherein ipsilesional deficits are related to contralesional impairment but only in LHD (blue) and not in RHD (red). (C) Alternate hypothesis 2, wherein ipsilesional deficits are related to contralesional impairment but only in LHD and in RHD with severe impairment (represented in the shaded dark-gray area). For both alternate hypotheses, β1 and β3 ≠ 0.
One reason that this prediction remains untested might be methodological in that in these previous studies participants were categorically classified based on the degree of contralesional motor impairment (e.g., mild, moderate, severe) (6, 8, 14). Categorization (or worse, dichotomization) of a continuous variable presents several concerns, including loss of measurement resolution, an assumption of discontinuity in the underlying construct (in this case motor impairment), unequal subgroup sizes (or biased sampling), and large unexplained residuals in regression models (18–20). Overall, if the objective is to understand the nature and extent of critical response-predictor relationships, then a categorical approach is particularly problematic.
Our primary objective was to determine if the severity of deficits in the ipsilesional hand varies directly with that of the contralesional hand and if this relationship differs based on the side of stroke lesion (i.e., an interaction effect). To accomplish this objective, we conducted a retrospective regression analysis of an existing dataset taken from mild-to-moderately impaired chronic stroke survivors. Although the range of contralesional impairment was limited in this dataset, we preserved its continuity and tested the prediction that the dexterous motor capacity of the ipsilesional hand varies directly with the severity of the contralesional motor impairment in individuals with LHD, but not in individuals with RHD (see Figure 1).
Methods
Participants
A retrospective analysis of data from 42 chronic stroke survivors (n = 21 left-hemisphere damage, LHD) was conducted. Participants were enrolled as part of a larger phase-IIb clinical trial (Dose Optimization for Stroke Evaluation, ClinicalTrials.gov ID: NCT01749358) (21) and provided informed consent in accordance with the 1964 Declaration of Helsinki and the guidelines of the Institutional Review Board for the Health Science Campus of the University of Southern California. Participants were at least 150 days post-stroke, pre-morbidly right-handed with mostly resolved upper extremity paresis. For a complete description of the inclusionary and exclusionary criteria, please refer to Supplementary Materials.
Outcome Measures
Motor Component of the Upper Extremity Fugl-Meyer (UEFM)
The UEFM (22) is an assessment of motor impairment of the contralesional arm and hand after stroke and includes tests of strength and independent joint control. Item-wise scoring of the UEFM ranges from 0 (unable to perform) to 2 (able to perform completely) while total score ranges from 0 to 66, with a higher score indicating lesser impairment. The UEFM score was modeled as a continuous variable for statistical analysis purposes.
Wolf Motor Function Test (WMFT)
The WMFT assesses upper extremity motor capacity through timed functional task performance (e.g., lifting a can, pencil, or paper clip). Originally designed for patients with moderate to severe upper extremity motor deficits, the test was later modified by Morris, Crago, and Taub to accommodate individuals with mild motor impairments (23). Hand motor capacity was assessed for both the contralesional and ipsilesional hands using the distal task battery of the WMFT (dWMFT) (24) which consisted of the following 8 tasks: lift can, lift pencil, lift paper clip, stack checkers, flip cards, turn a key in a lock, fold towel, and lift basket. A Principal Component Analysis of WMFT time scores revealed two clusters: one consisting of the proximal (#1–8, except 6, i.e., lifting weight to box), the other consisting of the distal (#9–17, except 14, i.e., grip strength) (Kim et al., unpublished, personal communication).
Statistical Analyses
All analyses were conducted in the R statistical computing package version 3.5.1 (25). To test the hypothesis that the inter-limb relationship of motor capacity is modified by the side of stroke lesion, we used the coefficient of determination (R2) and compared the covariances between LHD and RHD using the Fisher's Z-test. We then performed a simple linear regression to determine the slope of the relationship between contralesional (CL) UEFM and ipsilesional (IL) dWMFT (Model 1), and, CL dWMFT and IL dWMFT (Model 2). We used t-tests to compare slope between LHD and RHD.
To supplement these primary analyses and as a more robust assessment of the interaction between the side of lesion and contralesional motor capacity, we used multiple linear regression of the following form:
In both models, y is the average time score on the distal WMFT of the ipsilesional hand. Using this multiple model, our hypotheses were that β1 ≠ 0 and β3 ≠ 0 (see Figure 1). Any statistically significant interaction was resolved post-hoc using a t-test comparison of estimated marginal trends between LHD and RHD.
All continuous variables were assessed for normality using Lilliefors test (modified Kolmogorov–Smirnov test). Distributions for chronicity and average time-score for the distal WMFT were positively skewed and were therefore log-transformed. Welch's t-tests were used to compare age, chronicity, and Upper Extremity Fugl-Meyer scores between LHD and RHD, whereas Chi-square test was used to compare the proportion of females and males between the two groups. Each group was standardized to its own unit variance (z-scored) to equalize range and for subsequent linear regression analysis. Outliers were identified by visual inspection of scatterplots. Any value of IL dWMFT more extreme than ±1.5 log-SD was examined carefully for their influence on interlimb covariance and slope. If removal of these observations did not change the direction or significance of the effect in the simple model, we included them in the final model. Residuals of the final model were analyzed to confirm that all necessary assumptions for multiple regression were met. Significance level (α) was set at p < 0.05.
In order to select the predictor variables that best explain the response, we used a backward selection approach, in which we began by adding all predictor variables in each of the two above models to explain the response variable y. This included our hypothesized predictors, CL UEFM (or CL dWMFT) and the side of stroke lesion (LHD or RHD), and, potential known confounders (age, chronicity, and sex). In a combined full model, those predictors that met a liberal cut-off of p = 0.2 were preserved in the final reduced model. Based on this selection process, we found sex to be a significant confounder (p = 0.08) in Model 1, and therefore included it as a predictor in the reduced Model 1. For Model 2, none of the confounders met the cut-off p-value, except our hypothesized predictors. Additional information on model selection and model diagnostics is included as Supplementary Materials. Standard errors and 95% CI of the estimates of regression coefficients were confirmed by performing 1,000 bootstrap replicates.
Results
Descriptive statistics for all participants are provided in Table 1. On average, the 42 stroke survivors had moderate arm impairment (UEFM = 41.6), were ~60 years of age, 5.75 years post-stroke, and were predominantly male (74%). There were no significant differences between LHD and RHD in the level of impairment, chronicity, or the number of males. Individuals with RHD were younger compared to LHD (median age difference 8.7 years) but not statistically different.
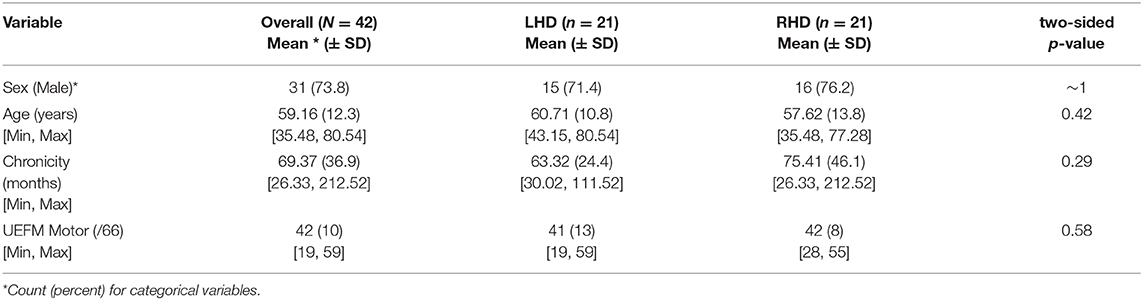
Table 1. Descriptive Statistics for the full sample (N = 42), and for the two groups of interest, left hemisphere damage, LHD (n = 21), and right hemisphere damage, RHD (n = 21).
Model 1: Side of lesion Modifies the Relationship Between CL UEFM and IL Motor Capacity
Contralesional UEFM explained 42% of the variance in ipsilesional hand motor capacity in LHD (p = 0.001), but <1% in RHD (p > 0.05). The slope of this relationship was −0.65 ± 0.17 (p = 0.001) in LHD and −0.066 ± 0.23 (p = 0.78) in RHD. Compared to RHD, the covariance between contralesional UEFM and ipsilesional hand motor capacity was significantly stronger (Fisher's z = 2.12, p = 0.03) and the slope was steeper in LHD (t = −2.03, p = 0.04).
Four observations (two each in LHD and RHD) were identified as potential outliers. After removal of these outliers, contralesional UEFM explained 44.3% of the variance in ipsilesional hand motor capacity in LHD (p = 0.001), and 2.26% in RHD (p > 0.05). The slope of this relationship changed to −0.42 ± 0.11 (p = 0.001) in LHD and 0.13 ± 0.20 (p = 0.54) in RHD. Again, a comparison of the covariances and slope between the groups revealed that compared to RHD, the relationship between contralesional UEFM and ipsilesional hand motor capacity was significantly stronger (Fisher's z = 2.7, p = 0.006) and the slope was steeper in LHD (t = −2.41, p = 0.02).
Since these observations did not significantly change the strength of covariance nor the slope of the relationship, they were preserved in the final multiple model. Analysis of residuals of the final model did not indicate violations of necessary assumptions in multiple regression in terms of linearity, equality of variance, independence and normality of errors, and multicollinearity of independent variables, nor the presence of unduly influential observations. Nonetheless, estimates below are reported both with and without suspected outliers.
After adjusting for main effects and significant confounders using multiple regression, the final reduced form of Model 1 was statistically different from a null model (F = 3.47, p = 0.016, adjusted R2 = 0.19). Based on estimates from Model 1, CL impairment (UEFM) was significantly associated with IL hand motor capacity, i.e., dWMFT (β1 = −0.72 ± 0.21, p = 0.001; without outliers: −0.44 ± 0.17, p = 0.01) (Figure 2A). There was no significant effect of the side of lesion (β2 = 0.026 ± 0.27, p = 0.92; without outliers: 0.22 ± 0.23, p = 0.33). There was a significant interaction between the side of lesion and CL impairment (β3 = 0.66 ± 0.30, p = 0.024; without outliers: 0.56 ± 0.24, p = 0.024). Post-hoc contrasts of estimated marginal trends indicated that the slope of the relationship between CL UEFM and IL dWMFT was significantly more negative in LHD compared to RHD (t = −2.34, p = 0.02; without outliers: −2.37, p = 0.02). Figures 2B,C illustrates the interaction.
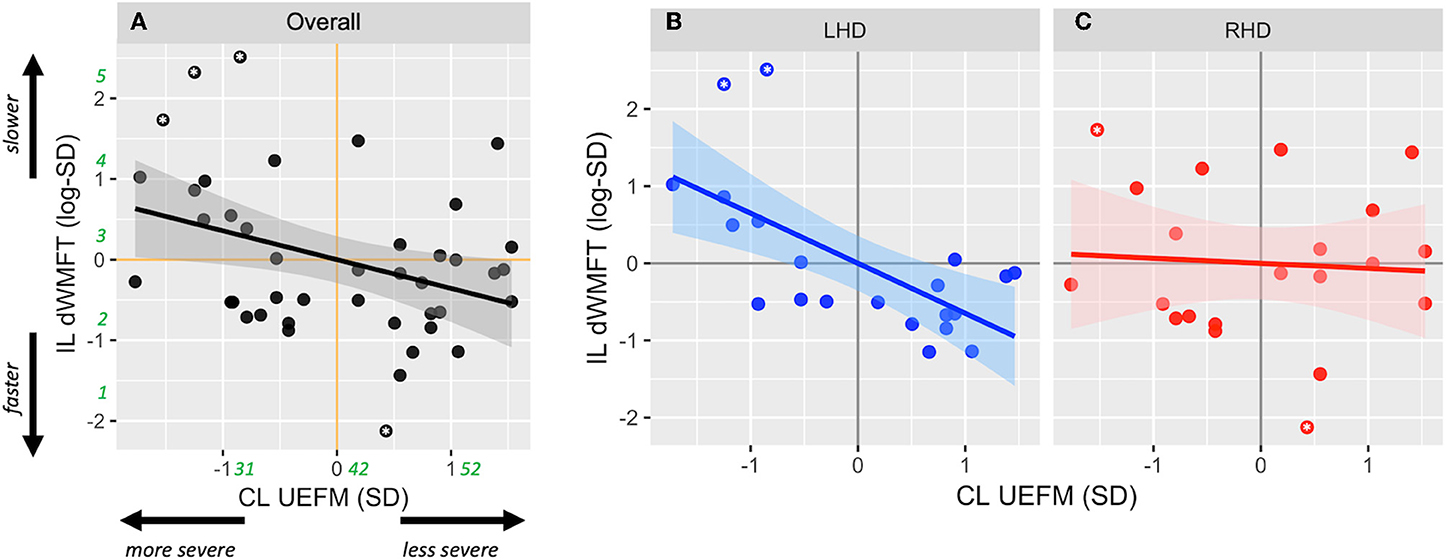
Figure 2. Scatterplots show the relationship between contralesional motor impairment (CL UEFM) and ipsilesional distal motor performance (IL dWMFT) for (A) the full sample, (B) LHD, and (C) RHD. Solid lines represent the linear prediction and shaded areas represent the 95% confidence interval. For ease of interpretation, rounded estimates of raw values (in seconds for dWMFT and points for UEFM) have been provided in green in (A). Asterisks indicate values evaluated as outliers.
Model 2: Side of lesion Modifies the Relationship Between CL dWMFT and IL Motor Capacity
Contralesional dWMFT explained 65% of the variance in ipsilesional hand motor capacity in LHD (p < 0.001), but only 9% in RHD (p > 0.05). The slope of this relationship was 0.81 ± 0.13 (p < 0.001) in LHD and 0.29 ± 0.22 (p = 0.19) in RHD. A comparison of the covariances and slope between LHD and RHD revealed that compared to RHD, the relationship between CL dWMFT and IL motor capacity was significantly stronger (Fisher's z = 2.45, p = 0.01) and the slope was steeper in LHD (t = 2.03, p = 0.04).
After removing the outlying observations, contralesional dWMFT explained 62% of the variance in ipsilesional hand motor capacity in LHD (p < 0.001), and <1% in RHD (p > 0.05). The slope of this relationship changed to 0.54 ± 0.1 (p < 0.001) in LHD and 0.05 ± 0.21 (p = 0.81) in RHD. Compared to RHD, the relationship between CL dWMFT and IL motor capacity was significantly stronger (Fisher's z = 2.85, p = 0.004) and the slope was steeper in LHD (t = 2.11, p = 0.04).
Since these observations did not significantly change the strength of covariance or the slope of the relationship, they were preserved in the final multiple model. Once again, analysis of residuals did not indicate violations of necessary assumptions in multiple regression nor the presence of unduly influential observations. Nonetheless, estimates below are reported both with and without suspected outliers.
After adjusting for main effects and significant confounders using multiple regression, the final reduced form of Model 2 was statistically different from a null model (F = 7.48, p < 0.001, adjusted R2 = 0.32). Based on estimates from Model 2, CL hand motor capacity was significantly associated with IL hand motor capacity (β1 = −0.81± 0.16, p < 0.001; without outliers: 0.54 ± 0.17, p = 0.003) (Figure 3A). There was no significant effect of the side of lesion (β2 = 0.12 ± 0.26, p = 0.66; without outliers: 0.19 ± 0.22, p = 0.39). There was an interaction between the side of lesion and CL hand motor capacity, but it only approached statistical significance (β3 = −0.51 ± 0.34, p = 0.05; without outliers: −0.49 ± 0.24, p = 0.046). Figures 3B,C illustrates the interaction.
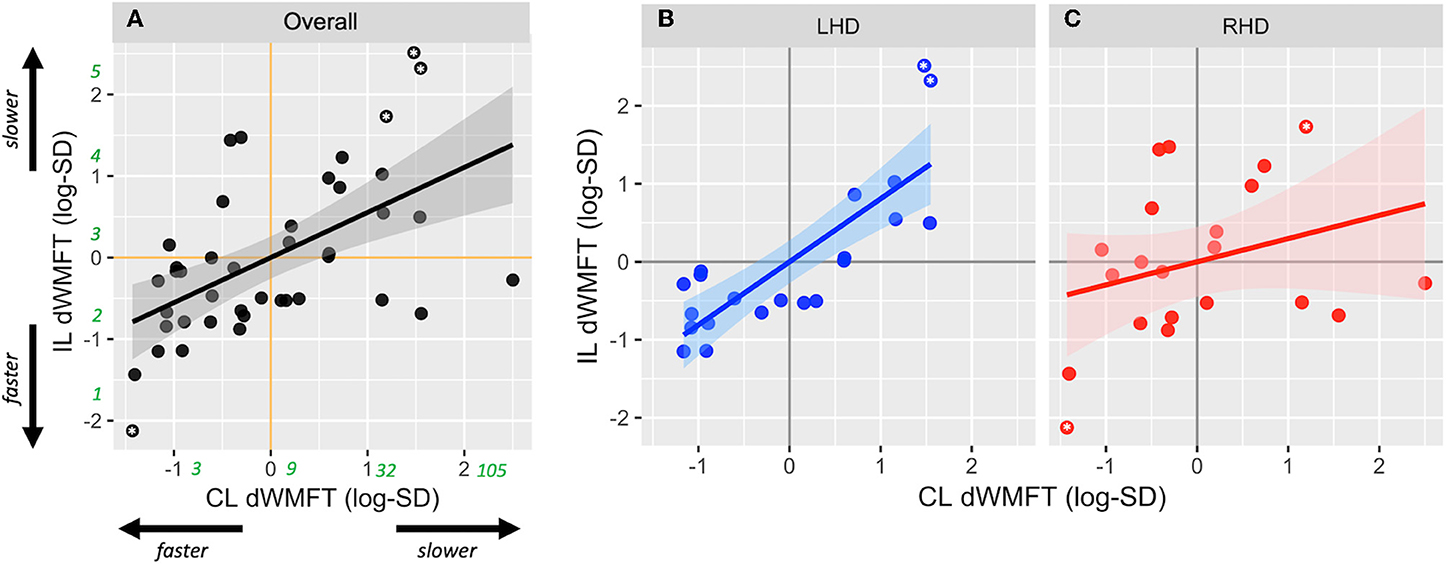
Figure 3. Scatterplots show relationship between contralesional distal motor performance (CL dWMFT) and ipsilesional distal motor performance (IL dWMFT) for (A) the full sample, (B) LHD, and (C) RHD. Solid lines represent the linear prediction and shaded areas represent the 95% confidence interval. For ease of interpretation, rounded estimates of raw values (in seconds) have been provided in green in (A). Asterisks indicate values evaluated as outliers.
Finally, we note here that we conducted similar analyses for the proximal component of the WMFT (not reported) but did not observe the same relationships (model adj. R2 = ~3% for both models, p > 0.25). One possibility is that unlike the distal component, the proximal WMFT is a much less sensitive metric of motor performance, especially in individuals with mild-to-moderate impairment.
Discussion
For the first time, we explicitly tested the hypothesis that motor capacity of the ipsilesional hand is influenced by an interaction between the severity of contralesional deficits and the side of stroke lesion. Using retrospective analysis of an existing dataset, we found that ipsilesional motor capacity co-varies with contralesional impairment to a significantly greater degree in individuals with LHD compared to RHD.
Analysis of the Interaction Effect
Hints of this interaction were implicit in a few previous studies (2, 3, 14); however, categorical reporting of the Upper Extremity Fugl-Meyer (UEFM) masked this interesting effect. By preserving the continuity of the UEFM and utilizing continuous standardized z-scores, our statistical approach allowed a direct comparison of our regression estimates, thus reflecting effect sizes of each of the candidate predictors. Unlike previous studies, we did not observe a significant effect of the side of lesion on ipsilesional motor capacity (16, 17) or on contralesional UEFM or dWMFT (11). One reason might be that the effect of the side of lesion observed in those previous studies may have arisen from its interaction with contralesional impairment. However, because an interaction effect was not explicitly tested and because contralesional impairment was either collapsed across the groups (2, 3) or categorical (8, 14), variance in the ipsilesional capacity may have been conflated with the effect of the side of lesion, or, remained unexplained, especially for UEFM scores that fell at the boundaries of the pre-defined categories. Previously, various cut-off scores for the UEFM have been used to define impairment categories (8, 14). Of these, our data suggest that a UEFM score of 42, which occurs at the intersection of the linear fits for LHD and RHD, would best reflect the change in the direction of effect—that is, for UEFM scores <42, the ipsilesional limb would be slower in LHD compared to RHD whereas for UEFM scores >42, those with LHD would be slightly faster compared to RHD. Interestingly, at this score of 42, there would appear to be no differences in motor capacity of either hand between LHD and RHD, which might explain why a number of large clinical trials [e.g., EXCITE (26), ICARE (27)], designed for mild to moderately impaired stroke survivors (mean UEFM scores in these studies were 42.5 and 41.6, respectively) may not have observed, on average, any differences in motor capacity based on the side of stroke.
On a related note, in Model 1, we observed that the relationship between contralesional UEFM and ipsilesional motor performance was (mildly) confounded by sex. Specifically, males showed slightly faster performance times (mean difference = 0.3 s, β = −0.56, p = 0.08) compared to females. Given that this difference was very small and may have been exaggerated by the unequal sample sizes, i.e., there were about 3 times more males than females, in LHD, RHD and overall, we suspect this was an artifact of the unequal sample sizes rather than a true difference between males and females.
Insights From the Type of Task
A common link between our study and past reports is that ipsilesional deficits were found to be most pronounced for distal (dexterous) tasks. These tasks—lift can, lift pencil, lift paper clip, stack checkers, flip cards, turn a key in a lock, fold towel, and lift basket—nearly always involve object manipulation and inherently require dexterous motor control of the hands. Sunderland et al. (4) demonstrated that early on after a stroke, spatial accuracy in dexterity tasks performed with the ipsilesional hand correlated with cognitive deficits, such as apraxia, in individuals with LHD. While individuals included in this study did not exhibit severe apraxia and were ~5 years post-stroke, it is possible that mild cognitive deficits, including apraxia, may have impacted dexterous task performance in those with LHD, especially in the more severe ranges of UEFM. Furthermore, we note that our evaluation of dexterous task performance was through timed tests, and not quality of movement or accuracy. It has been suggested that the left hemisphere plays an important role in regulating the timing and speed of movements (13), and thus, injury to the left hemisphere, such as to premotor and fronto-parietal networks (28, 29) may impair planning and sequencing required for smooth and rapid performance of dexterous motor tasks.
The Role of the Left Hemisphere in the Control of Both Hands
Our main observation that deficits in ipsilesional hand motor capacity scale with contralesional impairment only in LHD is qualitatively similar to previous clinico-behavioral [e.g., (16, 17, 30, 31)] and phenomenological evidence [e.g., (32–34)]. These findings are consistent with a rather simplified organizational model of the nervous system in which certain aspects of motor and/or cognitive control are lateralized to the left (or dominant) hemisphere, such that damage to the left hemisphere results in deficits in skilled motor actions of both upper extremities. For example, using EMG recordings of homologous muscles in the arm, Cernacek (35) demonstrated that the frequency of motor irradiations, i.e., unintended motor output in the ipsilateral hand, were significantly higher from the dominant to the non-dominant extremity. Similarly, Wyke (17) reported that while individuals with left-sided cerebral lesions exhibited bilateral motor deficits in speed and limb postural control, deficits in those with right cerebral lesions were restricted to the contralateral limb. Even within the LHD patient group, the nature and extent of ipsilesional deficits has been shown to be modulated by the degree of (clinical) paresis. For example, Haaland et al. (36) demonstrated that deficits in ipsilesional torque amplitude specification were statistically significant in LHD patients with contralesional upper extremity paresis compared to those with no paresis, despite all other features of the movement and lesion (e.g., error, speed, lesion volume) being similar.
Lastly, in one of the earliest experiments using functional MRI, Kim et al. (37) showed that the task-evoked activation of the left hemisphere was substantially greater for ipsilateral movements compared to the right hemisphere. In later years, a number of neuroimaging (30, 31) and neurophysiologic (32–34) studies have provided confirmatory evidence for the role of the dominant hemisphere in organizing motor outputs to both hands. Our results of co-varying deficits between the contralesional and ipsilesional hand in LHD provides further empirical support for the role of the left hemisphere (in our pre-morbidly right-handed group) in the control of both hands.
Limitations and Future Considerations
In interpreting our findings, it is important to consider that this study is a retrospective analysis of a relatively small dataset. Therefore, sample size, and characteristics such as the range of impairment, chronicity, age, were limited to what was available. To this point, we conducted analyses with and without outliers, and found that while exclusion of outliers affected the strength of the overall model, it did not affect the probability associated with rejecting the null hypothesis. A prospective study or independent validation in a separate cohort would be ideal, if larger samples were available. A larger sample would render more robust findings that are less sensitive to distortions from outlying values.
Along this line, UEFM scores for the RHD group were restricted toward the more severe range, with the most severely impaired individual's score being 28. This restriction, however, was less so in the LHD group (min. UEFM = 19). Although this limitation in range was circumvented by using group-wise z-scores, we are cautious in generalizing our observations regarding the interaction effect to more severe ranges of motor impairment in RHD. This is quite apparent in the variability around our estimated linear fits especially toward the extreme ranges of predictor values for RHD. Indeed, it is possible that for the more severe range in RHD, there exists a linear relationship between contralesional and ipsilesional motor deficits as illustrated in Figure 1C. Thus, while we can, with some confidence, reject the null hypothesis, our data are insufficient to differentiate between the two alternate hypotheses, and warrant a follow-up study.
Finally, it must be emphasized that the absence of a relationship with contralesional impairment in RHD should not be taken to mean that ipsilesional deficits are absent in this group. In fact, there is substantial evidence to the contrary (38–41). Comparison with an appropriate control group would be necessary to demonstrate the presence of ipsilesional deficits in RHD and the functional implications of these deficits. As alluded to earlier, measuring the speed of performance, as in the case of timed functional tasks assessed here, does not provide specific information about perceptual errors, spatial accuracy or visuomotor deficits, which, based on previous evidence (42, 43), might be a more important component of motor performance in RHD.
Conclusion
In summary, our results suggest that ipsilesional motor deficits co-vary with the degree of impairment in LHD, but this relationship is less pronounced in RHD. This observation further underscores the extensive motor experiences of the pre-morbidly dominant ipsilesional limb and the importance of the left hemisphere in the control of timed tasks for both hands. For the future, we propose that a hypothetical model of bilateral deficits in LHD is readily testable through a prospective study that uses a bimanual experimental paradigm with sensitive kinematic measures. Such a paradigm could offer important insights into the role and organization of each hemisphere for the control of uni- and bi-manual movements.
Data Availability Statement
The complete raw dataset along with a codebook for analysis is available through the first author's OSF repository: https://osf.io/pbtk9.
Ethics Statement
The studies involving human participants were reviewed and approved by Institutional Review Board for the Health Science Campus of the University of Southern California. The participants provided their written informed consent to participate in this study.
Author Contributions
RV conceptualized the hypothesis, analyzed the data, and drafted the manuscript. CW provided critical review and interpretation of the findings and was involved in manuscript revision.
Funding
This research study is supported by the NICHD National Center for Medical Rehabilitation Research of the National Institutes of Health under award numbers: F31HD098796-01 to RV and R01HD065438 to Nicolas Schweighofer and CW. The Article Processing Charge is supported by the Division of Biokinesiology and Physical Therapy.
Conflict of Interest
The authors declare that the research was conducted in the absence of any commercial or financial relationships that could be construed as a potential conflict of interest.
Acknowledgments
This manuscript has been released as a preprint on bioRxiv at https://doi.org/10.1101/635136 (44).
Supplementary Material
The Supplementary Material for this article can be found online at: https://www.frontiersin.org/articles/10.3389/fneur.2019.01340/full#supplementary-material
References
1. Winstein CJ, Pohl PS. Effects of unilateral brain damage on the control of goal-directed hand movements. Exp Brain Res. (1995) 105:163–74. doi: 10.1007/BF00242191
2. Wetter S, Poole JL, Haaland KY. Functional implications of ipsilesional motor deficits after unilateral stroke. Arch Phys Med Rehabil. (2005) 86:776–81. doi: 10.1016/j.apmr.2004.08.009
3. Chestnut C, Haaland KY. Functional significance of ipsilesional motor deficits after unilateral stroke. Arch Phys Med Rehabil. (2008) 89:62–8. doi: 10.1016/j.apmr.2007.08.125
4. Sunderland A, Bowers MP, Sluman SM, Wilcock DJ, Ardron ME. Impaired dexterity of the ipsilateral hand after stroke and the relationship to cognitive deficit. Stroke. (1999) 30:949–55. doi: 10.1161/01.STR.30.5.949
5. Rinne P, Hassan M, Fernandes C, Han E, Hennessy E, Waldman A, et al. Motor dexterity and strength depend upon integrity of the attention-control system. Proc Natl Acad Sci USA. (2017) 115:E536–45. doi: 10.1073/pnas.1715617115
6. Noskin O, Krakauer JW, Lazar RM, Festa JR, Handy C, Brien KAO, et al. Ipsilateral motor dysfunction from unilateral stroke : implications for the functional neuroanatomy of hemiparesis. J Neurol Neurosurg Psychiatry. (2008) 79:401–6. doi: 10.1136/jnnp.2007.118463
7. Metrot J, Froger J, Hauret I, Mottet D, Van Dokkum L, Laffont I. Motor recovery of the ipsilesional upper limb in subacute stroke. Arch Phys Med Rehabil. (2013) 94:2283–90. doi: 10.1016/j.rehab.2014.03.647
8. Bustrén EL, Sunnerhagen KS, Alt Murphy M. Movement kinematics of the ipsilesional upper extremity in persons with moderate or mild stroke. Neurorehabil Neural Repair. (2017) 31:376–86. doi: 10.1177/1545968316688798
9. Mani S, Mutha PK, Przybyla A, Haaland KY, Good DC, Sainburg RL. Contralesional motor deficits after unilateral stroke reflect hemisphere-specific control mechanisms. Brain. (2013) 136:1288–303. doi: 10.1093/brain/aws283
10. Schaefer SY, Haaland KY, Sainburg RL. Ipsilesional motor deficits following stroke reflect hemispheric specializations for movement control. Brain. (2007) 130:2146–58. doi: 10.1093/brain/awm145
11. Harris JE, Eng JJ. Individuals with the dominant hand affected following stroke demonstrate less impairment than those with the nondominant hand affected. Neurorehabil Neural Repair. (2006) 20:380–9. doi: 10.1177/1545968305284528
12. Nowak DA, Grefkes C, Dafotakis M, Küst J, Karbe H, Fink GR. Dexterity is impaired at both hands following unilateral subcortical middle cerebral artery stroke. Eur J Neurosci. (2007) 25:3173–84. doi: 10.1111/j.1460-9568.2007.05551.x
13. de Paiva Silva FP, De Freitas SMSF, Silva PV, Banjai RM, Alouche SR. Ipsilesional arm motor sequence performance after right and left hemisphere damage. J Mot Behav. (2014) 46:407–14. doi: 10.1080/00222895.2014.924473
14. de Paiva Silva FP, Freitas SMSF, Banjai RM, Alouche SR. Ipsilesional arm aiming movements after stroke: influence of the degree of contralesional impairment. J Mot Behav. (2018) 50:104–15. doi: 10.1080/00222895.2017.1306479
15. Hanna-Pladdy B, Mendoza JE, Apostolos GT, Heilman KM. Lateralised motor control: hemispheric damage and the loss of deftness. J Neurol Neurosurg Psychiatry. (2002) 73:574–7. doi: 10.1136/jnnp.73.5.574
16. Heap M, Wyke M. Learning of a unimanual motor skill by patients with brain lesions: an experimental study. Cortex. (1972) 8:1–18. doi: 10.1016/S0010-9452(72)80023-6
17. Wyke M. The effect of brain lesions in the performance of an arm-hand precision task. Neuropsychologia. (1968) 6:125–34. doi: 10.1016/0028-3932(68)90054-7
18. Collins GS, Ogundimu EO, Cook JA, Manach YLe, Altman DG. Quantifying the impact of different approaches for handling continuous predictors on the performance of a prognostic model. Stat Med. (2016) 35:4124–35. doi: 10.1002/sim.6986
19. Royston P, Altman DG, Sauerbrei W. Dichotomizing continuous predictors in multiple regression: a bad idea. Stat Med. (2006) 25:127–41. doi: 10.1002/sim.2331
20. Naggara O, Raymond J, Guilbert F, Roy D, Weill A, Altman DG. Analysis by categorizing or dichotomizing continuous variables is inadvisable: an example from the natural history of unruptured aneurysms. Am J Neuroradiol. (2011) 32:437–40. doi: 10.3174/ajnr.A2425
21. Winstein C, Kim B, Kim S, Martinez C, Schweighofer N. Dosage matters. Stroke. (2019) 50:1831–7. doi: 10.1161/STROKEAHA.118.023603
22. Fugl-Meyer AR, Jääskö L, Leyman I, Olsson S, Steglind S. The post-stroke hemiplegic patient. 1. A method for evaluation of physical performance. Scand J Rehabil Med. (1975) 7:13–31.
23. Wolf SL, Catlin PA, Ellis M, Archer AL, Morgan B, Piacentino A. Assessing Wolf Motor Function Test as outcome measure for research in patients after stroke. Stroke. (2001) 32:1635–9. doi: 10.1161/01.STR.32.7.1635
24. Woodbury M, Nichols-Larsen DS, Wolf SL, Thompson PA, Blanton S, Morris D, et al. Measurement structure of the Wolf Motor Function Test: Implications for motor control theory. Neurorehabil Neural Repair. (2010) 24:791–801. doi: 10.1177/1545968310370749
25. R Core Team. R: A Language and Environment for Statistical Computing. Vienna: R Foundation for Statistical Computing (2012).
26. Wolf SL, Winstein CJ, Miller JP, Taub E, Uswatte G, Morris D, et al. Nichols-Larsen D, EXCITE Investigators for the. Effect of Constraint-Induced Movement Therapy on upper extremity function 3 to 9 months after stroke. JAMA. (2006) 296:2095. doi: 10.1001/jama.296.17.2095
27. Winstein CJ, Wolf SL, Dromerick AW, Lane CJ, Nelsen MA, Lewthwaite R, et al. Effect of a task-oriented rehabilitation program on upper extremity recovery following motor stroke the ICARE randomized clinical trial. JAMA. (2016) 315:571–81. doi: 10.1001/jama.2016.0276
28. Harrington DL, Haaland KY. Hemispheric specialization for motor sequencing: abnormalities in levels of programming. Neuropsychologia. (1991) 29:147–63. doi: 10.1016/0028-3932(91)90017-3
29. Harrington DL, Haaland KY, Knight RT. Cortical networks underlying mechanisms of time perception. J Neurosci. (1998) 18:1085–95. doi: 10.1523/JNEUROSCI.18-03-01085.1998
30. Serrien DJ, Cassidy MJ, Brown P. The importance of the dominant hemisphere in the organization of bimanual movements. Hum Brain Mapp. (2003) 18:296–305. doi: 10.1002/hbm.10086
31. Bundy DT, Szrama N, Pahwa M, Leuthardt EC. Unilateral, 3D arm movement kinematics are encoded in ipsilateral human cortex. J Neurosci. (2018) 38:10042–56. doi: 10.1523/JNEUROSCI.0015-18.2018
32. Hess CW, Mills KR, Murray NMF. Magnetic stimulation of the human brain: facilitation of motor responses by voluntary contraction of ipsilateral and contralateral muscles with additional observations on an amputee. Neurosci Lett. (1986) 71:235–40. doi: 10.1016/0304-3940(86)90565-3
33. Sohn YH, Jung HY, Kaelin-Lang A, Hallett M. Excitability of the ipsilateral motor cortex during phasic voluntary hand movement. Exp Brain Res. (2003) 148:176–85. doi: 10.1007/s00221-002-1292-5
34. Ganguly K, Secundo L, Ranade G, Orsborn A, Chang EF, Dimitrov DF, et al. Cortical representation of ipsilateral arm movements in monkey and man. J Neurosci. (2009) 29:12948–56. doi: 10.1523/JNEUROSCI.2471-09.2009
35. Cernacek J. Contralateral motor irradiation-cerebral dominance: Its changes in hemiparesis. Arch Neurol. (1961) 4:165–72. doi: 10.1001/archneur.1961.00450080047005
36. Haaland KY, Schaefer SY, Knight RT, Adair J, Magalhaes A, Sadek J, et al. Ipsilesional trajectory control is related to contralesional arm paralysis after left hemisphere damage. Exp Brain Res. (2009) 196:195–204. doi: 10.1007/s00221-009-1836-z
37. Kim SG, Ashe J, Hendrich K, Ellermann JM, Merkle H, Ugurbil K, et al. Functional magnetic resonance imaging of motor cortex: hemispheric asymmetry and handedness. Science. (1993) 261:615–6. doi: 10.1126/science.8342027
38. Wyke M. The effects of brain lesions on the learning performance of a bimanual co-ordination task. Cortex. (1971) 7:59–72. doi: 10.1016/S0010-9452(71)80022-9
39. Wyke M. Postural arm drift associated with brain lesions in man. Arch Neurol. (1966) 15:329–34. doi: 10.1001/archneur.1966.00470150107016
40. Giuliani CA, Purser JL, Light KE, Genova PA. Impairments in arm control in subjects with left and right hemisphere stroke. NeuroRehabilitation. (1997) 9:71–87. doi: 10.3233/NRE-1997-9107
41. Freitas SMSF, Gera G, Scholz JP. Timing variability of reach trajectories in left versus right hemisphere stroke. Brain Res. (2011) 1419:19–33. doi: 10.1016/j.brainres.2011.08.039
42. Boll TJ. Right and left cerebral hemisphere damage and tactile perception: Performance of the ipsilateral and contralateral sides of the body. Neuropsychologia. (1974) 12:235–8. doi: 10.1016/0028-3932(74)90008-6
43. Bracewell RM, Husain M, Stein JF. Specialization of the right hemisphere for visuomotor control. Neuropsychologia. (1990) 28:763–75. doi: 10.1016/0028-3932(90)90001-5
Keywords: ipsilesional deficits, stroke, hemispheric differences, upper limb, motor capacity
Citation: Varghese R and Winstein CJ (2020) Relationship Between Motor Capacity of the Contralesional and Ipsilesional Hand Depends on the Side of Stroke in Chronic Stroke Survivors With Mild-to-Moderate Impairment. Front. Neurol. 10:1340. doi: 10.3389/fneur.2019.01340
Received: 13 September 2019; Accepted: 04 December 2019;
Published: 08 January 2020.
Edited by:
Yves Vandermeeren, CHU Dinant Godinne UCL Namur, BelgiumReviewed by:
Sydney Y. Schaefer, Arizona State University, United StatesSheng Li, University of Texas Health Science Center at Houston, United States
Katharina Stibrant Sunnerhagen, University of Gothenburg, Sweden
Copyright © 2020 Varghese and Winstein. This is an open-access article distributed under the terms of the Creative Commons Attribution License (CC BY). The use, distribution or reproduction in other forums is permitted, provided the original author(s) and the copyright owner(s) are credited and that the original publication in this journal is cited, in accordance with accepted academic practice. No use, distribution or reproduction is permitted which does not comply with these terms.
*Correspondence: Rini Varghese, rinivarg@usc.edu