- 1Department of Neurology, The First Affiliated Hospital of Guangxi University of Chinese Medicine, Guangxi University of Chinese Medicine, Nanning, China
- 2Department of Nuclear Medicine, Minzu Hospital of Guangxi, Nanning, China
- 3Department of Rehabilitation Medicine, The First Affiliated Hospital of Guangxi University of Chinese Medicine, Guangxi University of Chinese Medicine, Nanning, China
Summary of Background Data: Depression is one of the most common comorbidities in patients with chronic low back pain. However, the mechanisms of depression in chronic low back pain patients and the effect of antidepressants on the comorbidity of pain and depression need to be further explored. The establishment of the appropriate animal models and of more effective therapies is critical for this comorbidity. Lumbar disc herniation (LDH) is the most common disease that causes low back pain. The current study examined whether an LDH model shows behavioral and biochemical alterations that are in accordance with the characteristics of the comorbidity of pain and depression and tested the effect of fluoxetine (FLX) on these measures.
Objective: The current study examined whether an LDH model showed the behavioral and biochemical alterations that were in accordance with the characteristics of the comorbidity of pain and depression and tested the effect of FLX on these measures.
Methods: The LDH animal model was generated by the implantation of the autologous nucleus pulposus on the left L5 nerve root just proximal to the dorsal root ganglion in Wistar rats. Pain intensity was evaluated by mechanical allodynia and thermal hyperalgesia, and changes in depressive behavior were examined by the taste preference and forced swim tests. Hippocampal serotonin (5-HT) levels were measured by liquid chromatography-mass spectrometry, and tumor necrosis factor-α (TNF-α) mRNA was quantified using real-time reverse transcriptase PCR.
Results: LDH resulted in chronic pain, which further induced depressive behavior that persisted for 6 weeks after surgery. There were decreased 5-HT concentrations and upregulated TNF-α mRNA levels that were accompanied by behavioral changes. FLX treatment improved depressive behavior and moderately alleviated pain through increased 5-HT concentrations, and inhibited TNF-α mRNA expression.
Conclusions: In summary, our studies provide initial evidence that the LDH chronic pain model might serve as a model of the comorbidity of low back pain and depression. The finding that FLX improved depressive behavior and pain through normalized 5-HT concentrations and TNF-α mRNA expression establishes the initial mechanism of the comorbidity of pain and depression.
Introduction
Depression represents one of the most common comorbidities in patients with chronic low back pain (LBP) (1). LBP contributes to low health-related quality of life (HRQoL) scores and higher pain scores, lower labor productivity and increased healthcare use (2), and the psychological symptoms, and pain-related disability may further reduce the probability of recovery from chronic LBP (3). Currently, antidepressants are suggested for symptomatic management (4). However, the mechanisms of depression in chronic LBP patients are not clear. Establishing and evaluating animal models of this comorbidity is the basis for both understanding the mechanisms and identifying appropriate treatments. However, we did not find any reports of lumbar disc herniation (LDH) animal models as a model of the comorbidity of pain and depression. The current study examined whether the LDH model was in accordance with the characteristics of the comorbidity of pain and depression and tested the effect of fluoxetine (FLX).
Comorbidity of pain and depression has long been recognized. But the underlying basis for this comorbidity is complex. Most studies have focused on hypothalamic-pituitary-adrenal (HPA) axis, neurogenesis, various neurotransmitters/neuromodulators, including serotonin (5-HT), norepinephrine (NA), dopamine (DA), glutamate (GLU), and GABA, the endocannabinoid system, brain-neurotrophic factor, opioids, neuroinflammatory factors (5, 6). Among this molecular neurobiology of the comorbidity, neuroinflammatory pathogenic mechanisms (7, 8), and 5-HT deficiency (9, 10) were widely investigated.
Central 5-HT deficiency is not only a mechanism of depression but also a mechanism of pain. 5-HT is involved in nociceptive pain signaling via ascending and descending modulatory pathways (11). The dysfunction of serotonergic transmission urges the use of particular drugs, namely, selective serotonin reuptake inhibitors (SSRIs). FLX was the first SSRI on the market; when it is administered, the inhibition of presynaptic serotonin transporters (5-HTT) causes less 5-HT to be transported from the synapse into pre-synaptic neurons, which increases the amount of 5-HT present in the synapse (12). It was used to uncover a pathophysiological link between pain and depression (13). Besides 5-HT deficiency, procytokines are an important pathogenic mechanism for depression through the activation of oxidative and nitrosative stress pathways, and the induction of indoleamine 2,3-dioxygenase (IDO) expression, which contributes to depression (14). Hippocampal procytokines are also an important pathogenic mechanism for pain through the induction of IDO and the inhibition of the melatoninergic system (15). Furthermore, the effect of FLX on the above-mentioned pathogenic mechanisms of comorbidity was tested. Previous studies concluded that FLX was effective to normalize BDNF (16, 17), enhance neurogenesis (18, 19), and inhibit GLU transmission (20, 21). Besides these, the effects of FLX on DA, NE, and 5-HT neurotransmission (22, 23) as well as neuroinflammatory factors (7, 8) were widely verified. Therefore, in this study, we also investigated the relationship between 5-HT levels, TNF-α mRNA expression, and the comorbidity of pain and depression and the effect of FLX on these measures.
Materials and Methods
Animals
Sixty- to sixty-five-day-old male Wistar rats (Shanghai Laboratory Animal Center, Shanghai) were housed and handled in strict accordance with the guidelines of the institutional and national Committees of Animal Use and Protection. Rats were housed in a temperature— (23 to 25°C) and humidity— (45 to 55%) controlled environment with a 12/12-h modified dark-light cycle (lights on from 7.00 a.m. to 7.00 p.m.). The protocol was approved through the Committee on the Ethics of Animal Experiments of the Guangxi University of Chinese Medicine (Permit Number: SCXK 2012–0002).
Lumbar Disc Herniation
LDH was conducted as previously described (24). A midline dorsal incision was made in pentobarbital-anesthetized (intraperitoneal injection, 50 mg/kg) rats, the midline dorsal muscles were separated along the L4–S1 spinous processes and the left L5 nerve roots and dorsal root ganglion (DRG) were exposed through laminectomy. Nucleus pulposus incised from the Co2–3 intervertebral disc was implanted next to the left L5 nerve root proximal to the DRG. Surgery was performed in sham rats without the nucleus pulposus implantation.
Pain Behavior
The thermal withdrawal threshold was assessed as previously described (25); the assessment used a painful heat stimulus with a Model BME-410A Paw Stimulator Analgesia Meter (BME, Tianjin) that had a default latency period set at 5 min, and the maximum latency at the light intensity used was set at 20 s to avoid tissue damage. Each rat received five trials, with 10 min between each trial, in which each hindpaw was tested 30 s apart. The average latency until paw withdrawal was calculated.
The mechanical withdrawal threshold was tested as previously described (26), and it was assessed using a mechanical stimulation of the hindpaw with von Frey filaments (BME-403, Tianjin). Rats were placed in a clear box with a metal mesh floor for 15 min to habituate the rats to mechanical stimulation, and the plantar surface of the hindpaw was stimulated by adjusting the stiffness of the measurement probe beginning with 1 g of pressure (the stimulus intensities were 1, 2, 4, 6, 8, 10, 15, 26 g). Each stimulation persisted for 5–6 s, and 5 min intervals were used between stimulations to allow the animal to cease possible responding. Whenever, three positive responses to a given filament occurred with five stimulations, 50% probability thresholds of mechanical paw withdrawal were calculated. A positive sign of a withdrawal response was characterized as a rapid pulling back, biting, licking, or shaking of the stimulated hind limb.
Thermal withdrawal thresholds and mechanical withdrawal thresholds were assessed at 0, 2, 4, and 6 weeks after surgery by an investigator blinded to the treatment conditions.
Depressive Behavior
A taste preference test (TPT) and a forced swim test (FST) were used to evaluate depressive behavior. The TPT was used to examine the behavior of anhedonia, and the FST was used to evaluate the state of despair, as previously described (27, 28). To examine chronic LBP-induced depressive behavior, we performed the FST and TPT at 0, 2, and 6 weeks after surgery.
The TPT was performed as previously described (27, 28). The animals were given free access to a standard rodent diet. Two bottles of 250 ml water were offered in their home cage on the first day of the experiment (habituation), but one of the bottles was replaced with 0.1% saccharin (#109185, Sigma) diluted in tap water on the next day (test). The test was initiated at 6.00 pm and ran for 24 h. TPT was expressed as a percentage of the volume of saccharin solution intake relative to the total liquid intake (saccharin solution plus regular water) over 24 h. Lower levels of preference for saccharin were indicative of anhedonia.
Subsequently, a modified version (27, 28) of the classic FST was conducted; the time was reduced to a single 5-min test, and higher immobility times indicated a state of despair. The rat was placed for 5 min in a glass container (60 cm in height and 30 cm in width) filled with tap water to a height of 45 cm and maintained at 22 to 25°C. The swimming behavior was videotaped and analyzed offline by an investigator blinded to the treatment conditions, and the total immobility time was calculated. Immobility was defined as not engaging in escape or exploratory behavior and consisted in moving the limbs just enough to keep the head above water. Rats were habituated in water for 5 min on the day before the formal test.
FLX and Distilled Water Treatment
Fluoxetine hydrochloride (Sigma-Aldrich) was dissolved in distilled water and put on a shaker which kept the temperature at 37°C and was shaken gently for 30 min. It was administered at 20 mg/kg through gavage twice daily at 12-h intervals for 2 weeks starting from 4 weeks after surgery. Previous studies have demonstrated FLX effectively attenuated pain and depressive behavior at 18 mg/kg (29). Animals in distilled water treatment groups received the same volume of distilled water.
Real-Time Reverse Transcriptase PCR
Total RNA was extracted from the hippocampus using TRIzol reagent (TaKaRa Bio, Dalian, China) according to the manufacturer's instructions. RNA was reverse transcribed to cDNA by using a reverse transcriptase kit (TaKaRa Bio, Dalian, China) on a Stratagene Robocycler Gradient 96 Thermal Cycler (Stratagene, California, USA). Real-time PCR assays were performed using a SYBR green Master Mix kit (Thermo Scientific, California, USA; Cat. No. #K0251) on an Mx3005 system (Stratagene). The following primers were used in this study: β-actin, (forward) 5′-GCA GGA GTA CGA TGA GTC CG-3′ and (reverse) 5′-ACG CAG CTC AGT AAC AGT CC-3′; TNF-α, (forward) 5′-GAC ACC ATG AGC ACG GAA AGC A-3′, and (reverse) 5′-CGC CAC GAG CAG GAA TGA GAA G-3′. The mRNA expression levels of the target gene were normalized to those of β-actin. Real-time PCR was done 6 weeks after surgery.
Liquid Chromatography-Mass Spectrometry
Chemicals
Serotonin hydrochloride (#H9523) and high-performance liquid chromatography-grade methanol were purchased from Sigma.
Apparatus
Liquid chromatography-mass spectrometry (LC-MS) comprised a Prominence 20 A series UFLC System (Shimadzu, Kyoto, Japan) with a 100 × 2.1-mm Restek C18 Aqueous column (Restek, Pennsylvania, USA) and an API 4,000 Qtrap MS System (ABSciex, Massachusetts, USA). The LC-MS detection parameters and mobile phases were prepared as previously described (30).
Sample Preparation
The brain samples were prepared as previously described (28). Briefly, the hippocampus was dissected and crushed on ice and then homogenized in a 10-fold volume of a 0.1 M formic acid solution. The homogenates were centrifuged at 18,000 × g for 20 min at 4°C. The supernatants were collected and stored at −80°C until chromatographic analysis. The LC-MS detection was done 6 weeks after surgery.
Statistical Analysis
All the results are expressed as the means ± SEM. Statistical analysis was performed using Statistical Package for the Social Sciences (SPSS 19.0, Chicago, USA) software (independent samples t-test was used to compare the means of two independent samples, one-way analysis of variance was used for multiple comparisons). P < 0.05 was accepted as an index of statistically significant differences.
Results
Rats With LDH Shows Persistent Pain Behavior
Compared to sham rats, the experimental group showed a significant drop on the ipsilateral foot and a moderate drop on the contralateral foot in mechanical withdrawal threshold at 2 and 4 weeks after surgery, and persisted for 6 weeks after surgery (Figure 1A, P < 0.01 or P < 0.05). Compared to sham rats, the experimental group showed drops on the bilateral feet in thermal withdrawal latency at 2 and 4 weeks after surgery, and persisted for 6 weeks after surgery (Figure 1B, P < 0.05).
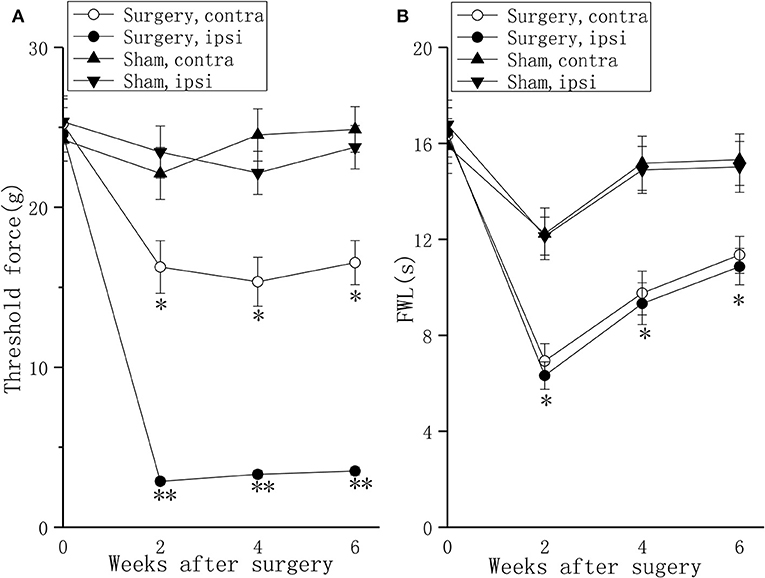
Figure 1. Behavioral responses in the mechanical withdrawal threshold test and thermal withdrawal latency test in a lumbar disc herniation model. The mechanical withdrawal threshold test (A) and the thermal withdrawal latency (B) on the ipsilateral foot and the contralateral foot were used to evaluate pain behavior at 2, 4, and 6 weeks after surgery. The data are presented as the means ± SEM, n = 10; *P < 0.05; **P < 0.01. FWL, foot withdrawal latency; ipsi, ipsilateral; contra, contralateral.
Consistent With the Persistent Pain, Rats With LDH Showed Chronic Depressive Behavior
Compared with sham rats, persistent pain induced depressive-like behavior in the FST (Figure 2A, P < 0.01) and the TPT (Figure 2B, P < 0.01) in rats with LDH at 2 weeks after surgery. At 6 weeks after surgery, the difference in FST (Figure 2A, P < 0.01) and TPT (Figure 2B, P < 0.01) between the sham group and the surgery group was significant.
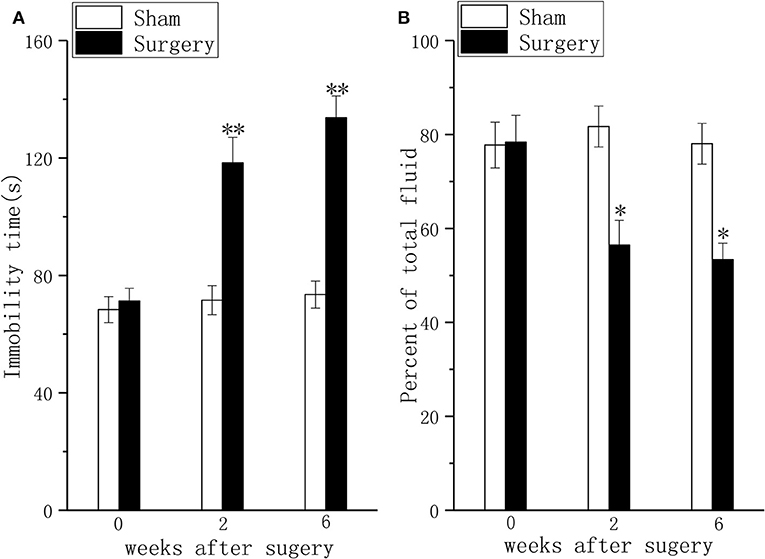
Figure 2. Behavioral responses in the forced swim test and the taste preference test in a lumbar disc herniation model. The duration of immobility during the forced swim test (A) and saccharin consumption (B) were used to evaluate depressive behavior at 2 and 6 weeks after surgery. The data are presented as the means ± SEM, n = 10; *P < 0.05; **P < 0.01.
Consistent With the Behavior Alteration, TNF-α Expression Was Increased and 5-HT Concentration Was Decreased in Rats With Coexisting Chronic Pain and Depressive Behavior
The hippocampal TNF-α (Figure 3A, P < 0.05) mRNA levels were increased and hippocampal 5-HT concentration (Figure 3B, P < 0.05) was decreased at 2 weeks after surgery in rats with coexisting chronic pain and depressive behavior compared to those in the rats in the sham group. The difference in TNF-α mRNA (Figure 3A, P < 0.05) expression and 5-HT concentration (Figure 3B, P < 0.05) in the hippocampus of rats with coexisting chronic pain and depressive behavior was maintained for 6 weeks after surgery.
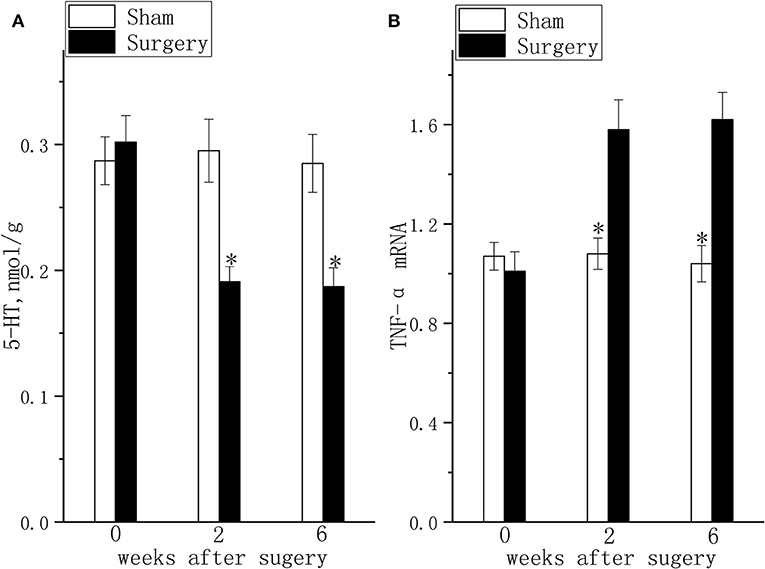
Figure 3. 5-HT levels and TNF-α mRNA expression in the lumbar disc herniation model. The 5-HT concentration (A) and TNF-α mRNA expression (B) were detected at 2 and 6 weeks after surgery. The data are presented as the means ± SEM, n = 10; *P < 0.05.
Effect of FLX on Pain Behavior and Depressive Behavior
FLX, compared with water, produced significant but mild analgesic effects in the mechanical allodynia test (Figure 4A, P < 0.05) and thermal hyperalgesia test (Figure 4B, P < 0.05) after 2 weeks of treatment; FLX, compared with water, improved depressive behavior in the FST (Figure 5A, P < 0.05), and the TPT (Figure 5B, P < 0.05) after 2 weeks of treatment.
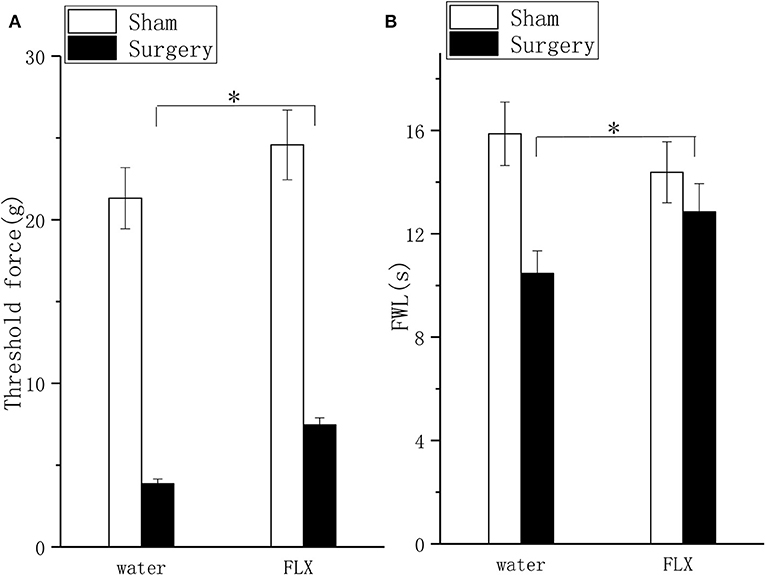
Figure 4. Effects of FLX on mechanical hyperalgesia and thermal hyperalgesia in a lumbar disc herniation model. The ipsilateral mechanical withdrawal threshold (A) and the ipsilateral thermal withdrawal latency (B) were used to evaluate pain behavior after 2 weeks of FLX and distilled water treatment (at 6 weeks after surgery). The data are presented as the means ± SEM, n = 10; *P < 0.05. FWL, foot withdrawal latency.
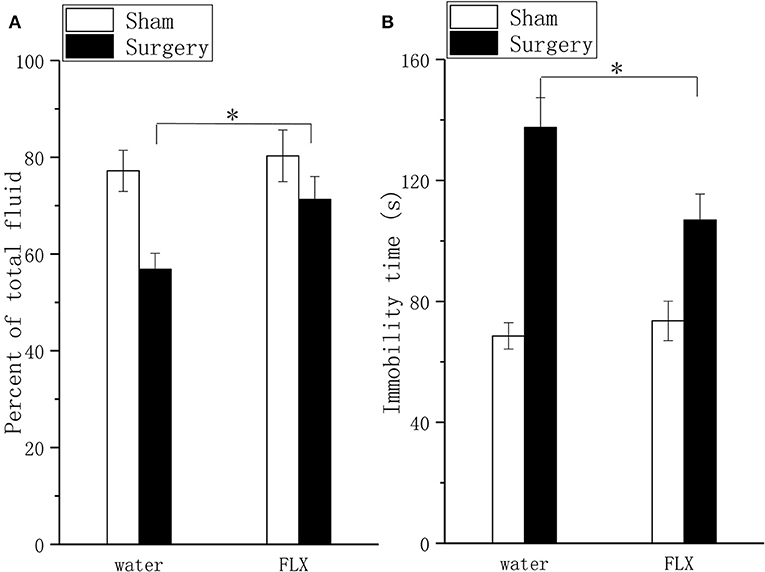
Figure 5. Effects of FLX on depressive behavior in the taste preference test and forced swim test in a lumbar disc herniation model. Saccharin consumption (A) and the duration of immobility during the forced swim test (B) were used to evaluate depressive behavior after 2 weeks of FLX and distilled water treatment (at 6 weeks after surgery). The data are presented as the means ± SEM, n = 10; *P < 0.05.
Effect of FLX on 5-HT Concentration and TNF-α mRNA Expression
FLX, compared with water, inhibited TNF-α mRNA expression (Figure 6A, P < 0.05) and elevated 5-HT (Figure 6B, P < 0.05) levels after 2 weeks of treatment.
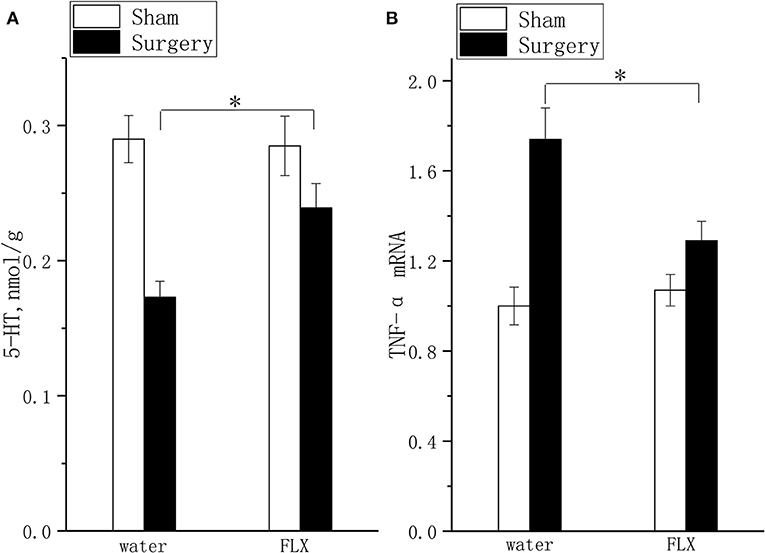
Figure 6. Effects of FLX on 5-HT concentration and TNF-α mRNA expression. The 5-HT concentration (A) and TNF-α mRNA expression (B) were detected after 2 weeks of FLX and distilled water treatment (at 6 weeks after surgery). The data are presented as the means ± SEM, n = 10; *P < 0.05.
Discussion
Here, we demonstrated that rats with LDH showed persistent pain, which induced persistent depressive behavior. Consistent with the depressive behavior induced by persistent pain, TNF-α mRNA expression was elevated, and 5-HT was decreased in the hippocampus of rats with chronic pain. FLX effectively alleviated pain and depressive behavior through the inhibition of TNF-α mRNA expression and elevated 5-HT levels. These results indicated that rats with LDH showed behavioral and biochemical alterations that were in accordance with the characteristics of the comorbidity of pain and depression and were sensitive to FLX.
LDH is one of the most frequent causes of chronic LBP, but the mechanisms of depression in LBP patients are not completely understood. The establishment of an appropriate animal model is key to exploring the mechanisms. To our knowledge, no LBP model with depressive behavior has been established thus far. The present study provides the first evidence that chronic pain caused by LDH leads to depression, and this is the first study to verify the comorbidity of pain and depression in an LDH model in which behavioral alterations were similar to those observed in LDH patients.
In our study, nucleus pulposus application in the rat contributed to chronic thermal hyperalgesia and mechanical allodynia of both feet. These results are consistent with a previous study in which mechanical allodynia and thermal hyperalgesia also developed in the contralateral hindpaw (24). In fact, contralateral hyperalgesia has also been reported in other neuropathic pain models (31) and patients (32–34) and has been hypothesized to be mediated by a hypotenusal effect (34), migrated epidural fat (32), or prominent stenotic changes of the contralateral side (35).
We also found that LDH rats showed depressive behavior in the FST and TPT, and this depressive behavior was accompanied by pain and persisted for 6 weeks after surgery. The mechanism by which neuropathic pain arising in the spinal cord transmits nociceptive input to the thalamus through ascending pathways is complex. Many relay stations related to multidimensional emotional experiences play roles in this process. The hippocampus is one such important area that is involved in the formation and storage of memories associated with emotional events, affect, arousal and attention to pain and learning (36). Importantly, the neurochemical and morphological changes in the hippocampal network contribute to the comorbidity of pain and depression (37). Therefore, we assessed neurochemical changes in our study.
Consistent with the change in nociceptive and depressive behavior, TNF-α mRNA expression was elevated and 5-HT was lowered in the hippocampus of the LDH rats. These findings were consistent with previous reports that the downregulation of 5-HT and chronic neuroinflammation play key roles in the comorbidity of pain and depression (37). In fact, the behavioral and biochemical alterations are in accordance with the stress mechanism of the comorbidity of pain and depression (37). Persistent pain is a chronic stressor that induces central procytokine upregulation, and chronic neuroinflammation is further induced by oxidative stress (38), IDO activation and the kynurenine pathway (39). These factors lead to a deficit in 5-HT, which is considered one of the main reasons for depression (40, 41). Similar to its role in depression, central chronic neuroinflammation further activates afferent autonomic nerve pathways, decreases hippocampal 5-HT levels, decreases BDNF and contributes to persistent chronic pain (37, 42). In the present study, we did not explore the relationship between 5-HT levels and TNF-α expression because we only attempted to identify the biochemical alterations of the comorbidity of pain and depression.
SSRIs have been used to treat the comorbidity of pain and depression (13). They improve depressive behavior through inhibiting 5-HT reuptake, alleviating neuroinflammation—which further upregulates the 5-HT level—and decreasing the kynurenine derivatives that cause mitochondrial dysfunctions and neurotoxic effects in the CNS (43). The present study is supportive of those findings and showed that FLX increased 5-HT levels and inhibited TNF-α mRNA expression. These findings are consistent with previous reports that FLX improved depressive behavior partly through its anti-inflammatory effects (44, 45). As with the similar effect in depression treatment, FLX alleviated pain through its serotonergic antinociceptive effects and its intrinsic anti-inflammatory effect (22). The present results that the downregulated 5-HT level and increased TNF-α expression were normalized by FLX support the previous conclusion (22). Synthesizing the behavioral and biochemical alterations produced by FLX, the present study indicated that FLX attenuated pain and improved depressive behavior through the inhibition of 5-HT reuptake and anti-inflammatory effects. A previous study verified that FLX was inactive in mice with chronic constriction injury (46), which is why the models are different. Another study also found that FLX failed to attenuate mechanical hypersensitivity and depression-like behavior due to confounding pronociceptive actions at 5-HT3 receptors (47). However, in the present study, we found that FLX alleviated pain and depressive behavior, and the effect was moderate (P < 0.05 in the mechanical withdrawal threshold test, not P < 0.01). This result is consistent with previous reports that the analgesic effects of FLX are weak (29).
In fact, the analgesic effects and antidepressant effects of FLX were mild, which was consistent with the effects of FLX on TNF-α expression and 5-HT level. FLX has an anti-inflammatory effect, but the effect was moderate (22); the inflammation still existed, TNF-α expression was still high and the persistent low-grade inflammation decreased hippocampal 5-HT levels through many ways in accordance with previous descriptions, so the 5-HT level was still low. Considering the key roles of inflammation and 5-HT in the comorbidity of pain and depression, the mechanism by which persistent low-grade inflammation and low 5-HT level lead to residual pain and depressive behavior after FLX treatment could explain the results. After all, previous reports had verified that anti-inflammatory drugs markedly augmented the effects of FLX to alleviate pain and depressive behaviors (29).
Although the mechanism of the comorbidity of pain and depression is complex, we established an LDH model that showed behavioral and biochemical alterations that are in accordance with the characteristics of this comorbidity. This model may partly reflect the mechanism by which LDH patients develop depressive behavior, and the model verified the role of FLX in this comorbidity. The biochemical alterations should be further examined in LDH patients with depressive behavior. Although we verified the effect of FLX on pain, the mechanism by which FLX is moderately effective in attenuating pain and whether other medications could enhance these effects should be further investigated.
Conclusion
The studies provide initial evidence that the LDH chronic pain model might serve as a model of the comorbidity of low back pain and depression. The finding that FLX improved depressive behavior and pain through normalized 5-HT concentrations and TNF-α mRNA expression establishes the initial mechanism of the comorbidity of pain and depression.
Data Availability Statement
The datasets generated for this study are available on request to the corresponding author.
Ethics Statement
The animals were housed and handled in strict accordance with the guidelines of the institutional and national Committees of Animal Use and Protection. The protocol was approved through the Committee on the Ethics of Animal Experiments of the Guangxi University of Chinese Medicine.
Author Contributions
LC contributed to the study design, participated in the acquisition and analysis of data, the statistical analysis, and the manuscript drafting and revision. QH contributed to the study design. YL participated in the data analysis and manuscript revision. YuyH, WC, and LW contributed to the experimental implementation. The whole experiment was completed under the guidance of YueH.
Funding
This work was supported by grants from the Guangxi Natural Science Foundation (2015GXNSFBA139172).
Conflict of Interest
The authors declare that the research was conducted in the absence of any commercial or financial relationships that could be construed as a potential conflict of interest.
References
1. Stubbs B, Koyanagi A, Thompson T, Veronese N, Carvalho AF, Solomi M, et al. The epidemiology of back pain and its relationship with depression, psychosis, anxiety, sleep disturbances, and stress sensitivity: data from 43 low- and middle-income countries. Gen Hosp Psychiatry. (2016) 43:63–70. doi: 10.1016/j.genhosppsych.2016.09.008
2. Tsuji T, Matsudaira K, Sato H, Vietri J. The impact of depression among chronic low back pain patients in Japan. BMC Musculoskelet Disord. (2016) 17:447. doi: 10.1186/s12891-016-1304-4
3. Nordstoga AL, Nilsen TIL, Vasseljen O, Unsgaard-Tondel M, Mork PJ. The influence of multisite pain and psychological comorbidity on prognosis of chronic low back pain: longitudinal data from the Norwegian HUNT Study. BMJ Open. (2017) 7:e015312. doi: 10.1136/bmjopen-2016-015312
4. Axon DR, Patel MJ, Martin JR, Slack MK. Use of multidomain management strategies by community dwelling adults with chronic pain: evidence from a systematic review. Scand J Pain. (2018) 19:9–23. doi: 10.1515/sjpain-2018-0306
5. Sheng J, Liu S, Wang Y, Cui R, Zhang X. The link between depression and chronic pain: neural mechanisms in the brain. Neural Plast. (2017) 2017:9724371. doi: 10.1155/2017/9724371
6. Humo M, Lu H, Yalcin I. The molecular neurobiology of chronic pain-induced depression. Cell Tissue Res. (2019) 377:21–43. doi: 10.1007/s00441-019-03003-z
7. Usdin TB, Dimitrov EL. The effects of extended pain on behavior: recent progress. Neuroscientist. (2016) 22:521–33. doi: 10.1177/1073858416633104
8. Arango-Davila CA, Rincon-Hoyos HG. Depressive disorder, anxiety disorder and chronic pain: multiple manifestations of a common clinical and pathophysiological core. Rev Colomb Psiquiatr. (2018) 47:46–55. doi: 10.1016/j.rcp.2016.10.007
9. Benson C, Mifflin K, Kerr B, Jesudasan SJ, Dursun S, Baker G. Biogenic amines and the amino acids GABA and glutamate: relationships with pain and depression. Mod Trends Pharmacopsychiatry. (2015) 30:67–79. doi: 10.1159/000435933
10. Obata H. Analgesic mechanisms of antidepressants for neuropathic pain. Int J Mol Sci. (2017) 18:e2483. doi: 10.3390/ijms18112483
11. Martin SL, Power A, Boyle Y, Anderson IM, Silverdale MA, Jones AKP. 5-HT modulation of pain perception in humans. Psychopharmacology. (2017) 234:2929–39. doi: 10.1007/s00213-017-4686-6
12. Kaufman J, DeLorenzo C, Choudhury S, Parsey RV. The 5-HT1A receptor in major depressive disorder. Eur Neuropsychopharmacol. (2016) 26:397–410. doi: 10.1016/j.euroneuro.2015.12.039
13. Gebhardt S, Heinzel-Gutenbrunner M, Konig U. Pain relief in depressive disorders: a meta-analysis of the effects of antidepressants. J Clin Psychopharmacol. (2016) 36:658–68. doi: 10.1097/JCP.0000000000000604
14. Wigner P, Czarny P, Galecki P, Su KP, Sliwinski T. The molecular aspects of oxidative & nitrosative stress and the tryptophan catabolites pathway (TRYCATs) as potential causes of depression. Psychiatry Res. (2018) 262:566–74. doi: 10.1016/j.psychres.2017.09.045
15. Kim H, Chen L, Lim G, Sung B, Wang S, McCabe MF, et al. Brain indoleamine 2,3-dioxygenase contributes to the comorbidity of pain and depression. J Clin Invest. (2012) 122:2940–54. doi: 10.1172/JCI61884
16. Zhao YP, Su M, Wang FY, Bian ZX, Yang JQ, Wang W, et al. [Effect of Chang'an No. I recipe on 5-hydroxytryptamine signal system and mRNA expression levels of hippocampal brain derived neurotrophic factor in visceral hypersensitivity rats with irritable bowel syndrome]. Zhongguo Zhong Xi Yi Jie He Za Zhi. (2015) 35:1228–35. doi: 10.7661/CJIM.2015.10.1228
17. Zu X, Zhang M, Li W, Xie H, Lin Z, Yang N, et al. Antidepressant-like effect of bacopaside I in mice exposed to chronic unpredictable mild stress by modulating the hypothalamic-pituitary-adrenal axis function and activating BDNF signaling pathway. Neurochem Res. (2017) 42:3233–44. doi: 10.1007/s11064-017-2360-3
18. Marcussen AB, Flagstad P, Kristjansen PE, Johansen FF, Englund U. Increase in neurogenesis and behavioural benefit after chronic fluoxetine treatment in Wistar rats. Acta Neurol Scand. (2008) 117:94–100. doi: 10.1111/j.1600-0404.2007.00910.x
19. Zhao Y, Zhang L, Wang M, Yu J, Yang J, Liu A, et al. Anxiety specific response and contribution of active hippocampal neural stem cells to chronic pain through wnt/beta-catenin signaling in mice. Front Mol Neurosci. (2018) 11:296. doi: 10.3389/fnmol.2018.00296
20. Marrocco J, Reynaert ML, Gatta E, Gabriel C, Mocaer E, Di Prisco S, et al. The effects of antidepressant treatment in prenatally stressed rats support the glutamatergic hypothesis of stress-related disorders. J Neurosci. (2014) 34:2015–24. doi: 10.1523/JNEUROSCI.4131-13.2014
21. Barygin OI, Nagaeva EI, Tikhonov DB, Belinskaya DA, Vanchakova NP, Shestakova NN. Inhibition of the NMDA and AMPA receptor channels by antidepressants and antipsychotics. Brain Res. (2017) 1660:58–66. doi: 10.1016/j.brainres.2017.01.028
22. Barakat A, Hamdy MM, Elbadr MM. Uses of fluoxetine in nociceptive pain management: a literature overview. Eur J Pharmacol. (2018) 829:12–25. doi: 10.1016/j.ejphar.2018.03.042
23. Kim EJ, Felsovalyi K, Young LM, Shmelkov SV, Grunebaum MF, Cardozo T. Molecular basis of atypicality of bupropion inferred from its receptor engagement in nervous system tissues. Psychopharmacology. (2018) 235:2643–50. doi: 10.1007/s00213-018-4958-9
24. Kim SJ, Park SM, Cho YW, Jung YJ, Lee DG, Jang SH, et al. Changes in expression of mRNA for interleukin-8 and effects of interleukin-8 receptor inhibitor in the spinal dorsal horn in a rat model of lumbar disc herniation. Spine. (2011) 36:2139–46. doi: 10.1097/BRS.0b013e31821945a3
25. Hargreaves K, Dubner R, Brown F, Flores C, Joris J. A new and sensitive method for measuring thermal nociception in cutaneous hyperalgesia. Pain. (1988) 32:77–88. doi: 10.1016/0304-3959(88)90026-7
26. Schistad EI, Espeland A, Pedersen LM, Sandvik L, Gjerstad J, Roe C. Association between baseline IL-6 and 1-year recovery in lumbar radicular pain. Euro J Pain. (2014) 18:1394–401. doi: 10.1002/j.1532-2149.2014.502.x
27. Mazarati A, Siddarth P, Baldwin RA, Shin D, Caplan R, Sankar R. Depression after status epilepticus: behavioural and biochemical deficits and effects of fluoxetine. Brain. (2008) 131:2071–83. doi: 10.1093/brain/awn117
28. Xie W, Cai L, Yu Y, Gao L, Xiao L, He Q, et al. Activation of brain indoleamine 2,3-dioxygenase contributes to epilepsy-associated depressive-like behavior in rats with chronic temporal lobe epilepsy. J Neuroinflamm. (2014) 11:41. doi: 10.1186/1742-2094-11-41
29. Hu X, Dong Y, Jin X, Zhang C, Zhang T, Zhao J, et al. The novel and potent anti-depressive action of triptolide and its influences on hippocampal neuroinflammation in a rat model of depression comorbidity of chronic pain. Brain Behav Immun. (2017) 64:180–94. doi: 10.1016/j.bbi.2017.03.005
30. Moller M, Du Preez JL, Harvey BH. Development and validation of a single analytical method for the determination of tryptophan, and its kynurenine metabolites in rat plasma. J Chromatogr B Analyt Tech Biomed Life sci. (2012) 898:121–9. doi: 10.1016/j.jchromb.2012.04.030
31. Li Y, Xi C, Niu M, Liu X, Chi Z, Wang X, et al. Contralateral neuropathology in dorsal root ganglia in a rat model of noncompressive disc herniation. Neurosci Lett. (2011) 493:49–54. doi: 10.1016/j.neulet.2011.02.018
32. Yang JS, Zhang DJ, Hao DJ. Lumbar disc herniation with contralateral radiculopathy: do we neglect the epidural fat? Pain Phys. (2015) 18:E253–6.
33. Kim P, Ju CI, Kim HS, Kim SW. Lumbar disc herniation presented with contralateral symptoms. J Korean Neurosurg Soc. (2017) 60:220–4. doi: 10.3340/jkns.2016.1010.015
34. Asan Z. Lumbar disc herniations causing contralateral radicular symptoms: can they be explained by hypotenusal theory? World Neurosurg. (2018) 114:e1297–301. doi: 10.1016/j.wneu.2018.03.201
35. Kalemci O, Kizmazoglu C, Ozer E, Arda MN. Lumbar disc herniation associated with contralateral neurological deficit: can venous congestion be the cause? Asian Spine J. (2013) 7:60–2. doi: 10.4184/asj.2013.7.1.60
36. Cohen SP, Mao J. Neuropathic pain: mechanisms and their clinical implications. BMJ. (2014) 348:f7656. doi: 10.1136/bmj.f7656
37. Fasick V, Spengler RN, Samankan S, Nader ND, Ignatowski TA. The hippocampus and TNF: common links between chronic pain and depression. Neurosci Biobehav Rev. (2015) 53:139–59. doi: 10.1016/j.neubiorev.2015.03.014
38. Czarny P, Wigner P, Galecki P, Sliwinski T. The interplay between inflammation, oxidative stress, DNA damage, DNA repair and mitochondrial dysfunction in depression. Prog Neuropsychopharmacol Biol Psychiatry. (2018) 80:309–21. doi: 10.1016/j.pnpbp.2017.06.036
39. Ramirez LA, Perez-Padilla EA, Garcia-Oscos F, Salgado H, Atzori M, Pineda JC. A new theory of depression based on the serotonin/kynurenine relationship and the hypothalamicpituitary- adrenal axis. Biomedica. (2018) 38:437–50. doi: 10.7705/biomedica.v38i3.3688
40. Lam CS, Li JJ, Tipoe GL, Youdim MBH, Fung ML. Monoamine oxidase a upregulated by chronic intermittent hypoxia activates indoleamine 2,3-dioxygenase and neurodegeneration. PLoS ONE. (2017) 12:e0177940. doi: 10.1371/journal.pone.0177940
41. Galecki P, Talarowska M. Inflammatory theory of depression. Psychiatr Pol. (2018) 52:437–47. doi: 10.12740/PP/76863
42. Vachon-Presseau E. Effects of stress on the corticolimbic system: implications for chronic pain. Prog Neuropsychopharmacol Biol Psychiatry. (2018) 87:216–23. doi: 10.1016/j.pnpbp.2017.10.014
43. Maes M, Leonard BE, Myint AM, Kubera M, Verkerk R. The new ‘5-HT' hypothesis of depression: cell-mediated immune activation induces indoleamine 2,3-dioxygenase, which leads to lower plasma tryptophan and an increased synthesis of detrimental tryptophan catabolites (TRYCATs), both of which contribute to the onset of depression. Prog Neuropsychopharmacol Biol Psychiatry. (2011) 35:702–21. doi: 10.1016/j.pnpbp.2010.12.017
44. Rodrigues FTS, de Souza MRM, Lima CNC, da Silva FER, Costa D, Dos Santos CC, et al. Major depression model induced by repeated and intermittent lipopolysaccharide administration: long-lasting behavioral, neuroimmune and neuroprogressive alterations. J Psychiatr Res. (2018) 107:57–67. doi: 10.1016/j.jpsychires.2018.10.003
45. Wang W, Liu X, Liu J, Cai E, Zhao Y, Li H, et al. Sesquiterpenoids from the root of panax ginseng attenuates lipopolysaccharide-induced depressive-like behavior through the brain-derived neurotrophic factor/tropomyosin-related B kinase and sirtuin type 1/nuclear factor-kappaB signaling pathways. J Agric Food Chem. (2018) 66:265–71. doi: 10.1021/acs.jafc.7b04835
46. Zuena AR, Maftei D, Alema GS, Dal Moro F, Lattanzi R, Casolini P, et al. Multimodal antidepressant vortioxetine causes analgesia in a mouse model of chronic neuropathic pain. Mol Pain. (2018) 14:1744806918808987. doi: 10.1177/1744806918808987
Keywords: lumbar disc herniation, mechanical allodynia, thermal hyperalgesia, depression, serotonin, TNF-α, taste preference test, forced swim test
Citation: Cai L, He Q, Lu Y, Hu Y, Chen W, Wei L and Hu Y (2019) Comorbidity of Pain and Depression in a Lumbar Disc Herniation Model: Biochemical Alterations and the Effects of Fluoxetine. Front. Neurol. 10:1022. doi: 10.3389/fneur.2019.01022
Received: 17 April 2019; Accepted: 09 September 2019;
Published: 24 September 2019.
Edited by:
Domenico De Berardis, Azienda Usl Teramo, ItalyReviewed by:
Ali Fahir Ozer, Koç University, TurkeyFederica Vellante, Università degli Studi G. d'Annunzio Chieti e Pescara, Italy
Copyright © 2019 Cai, He, Lu, Hu, Chen, Wei and Hu. This is an open-access article distributed under the terms of the Creative Commons Attribution License (CC BY). The use, distribution or reproduction in other forums is permitted, provided the original author(s) and the copyright owner(s) are credited and that the original publication in this journal is cited, in accordance with accepted academic practice. No use, distribution or reproduction is permitted which does not comply with these terms.
*Correspondence: Yueqiang Hu, MTM3NDYzMTk1QHFxLmNvbQ==
†These authors have contributed equally to this work