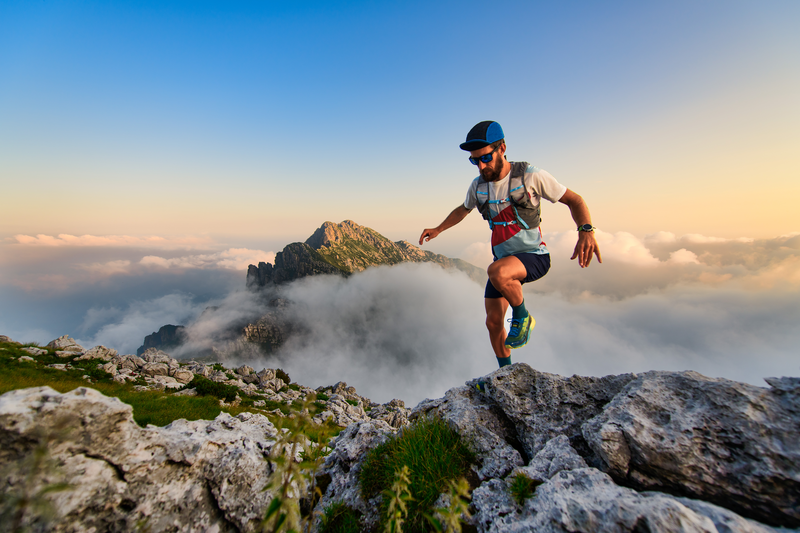
94% of researchers rate our articles as excellent or good
Learn more about the work of our research integrity team to safeguard the quality of each article we publish.
Find out more
REVIEW article
Front. Neurol. , 17 July 2019
Sec. Neuromuscular Disorders and Peripheral Neuropathies
Volume 10 - 2019 | https://doi.org/10.3389/fneur.2019.00783
This article is part of the Research Topic Strategies to Fight Exercise Intolerance in Neuromuscular Disorders View all 11 articles
Amyotrophic lateral sclerosis (ALS), a fatal neurodegenerative disease, involves the rapid deterioration of motor neurons resulting in severe muscle atrophy and respiratory insufficiency. It is considered a “multisystemic” disease with many potential mechanisms responsible for its pathology. Currently, there is no cure for ALS. Exercise training is suggested as a potential approach to reduce ALS pathology, but its beneficial role remains controversial. This review provides an overview of the effects of exercise training in ALS-affected mice and patients. It will compare the intensity, duration, and type of exercise on the health of SOD1G93A mice, a mouse model of familial ALS, and review clinical studies involving ALS patients undergoing both endurance and resistance training. In summary, mild-to-moderate swimming-based endurance training appears the most advantageous mode of exercise in SOD1G93A mice, improving animal survival, and delaying the onset and progression of disease. Furthermore, clinical studies show that both endurance and resistance training have an advantageous impact on the quality of life of ALS patients without extending life expectancy. However, small sample sizes, non-representative control populations, heterogeneous disease stage of patients, and the presence of confounders often exist in the exercise studies conducted with ALS patients. This raises concerns about the interpretation of these findings and, therefore, these results should be considered with caution. While promising, more pre-clinical and clinical studies with improved experimental design and fewer limitations are still necessary to confirm the impact of exercise training on the health of ALS patients.
Amyotrophic lateral sclerosis (ALS), a subtype of fatal motor neuron disease (MND), is a progressive and degenerative neuromuscular disorder. It is characterized by the death of the upper and lower motor neurons at the spinal or bulbar level, leading to paralysis and eventual death usually from severe muscle atrophy and respiratory insufficiency. Median survival is between 2 and 4 years from the onset of symptoms (1). The disease usually appears between 40 and 70 years of age, and affects about five in 100,000 people worldwide (2). There are two types of ALS: a familial ALS (FALS), heritable form (~5–10% of ALS), and the sporadic ALS (SALS) form, which occurs randomly (~90–95% of ALS). Mutations in the human copper/zinc (CuZn) superoxide dismutase (SOD1) gene account for ~20% of FALS cases, and the mutant SOD1G93A rodent model has been used extensively to help understand ALS pathology and to test potential therapeutic treatments for this disease. Several factors are suggested to contribute to motor neuron death in ALS. Excitotoxicity (3, 4), deficits in axonal transport (5, 6) and neurofilament aggregation (7, 8) are neuron-related mechanisms potentially involved in pathophysiology of ALS. Non-neuronal defects, including oxidative stress (9, 10), protein misfolding/aggregation (11), neuroinflammation caused by non-neuronal cells (e.g., glial cells, astrocytes) (12, 13), and skeletal muscle dysfunction (14–16) resulting from disequilibrium in mitochondria function (17, 18), satellite cell (SC) activity (19, 20) and microRNA expression (16, 21), are also considered potential contributors to ALS pathophysiology. Therefore, ALS is considered a “multisystemic” disease, where changes in different cell types may act mutually and synergistically to contribute to disease onset and severity (22). Given the complexity of the disease, it is difficult to find a specific treatment for ALS patients. Currently, there is no effective pharmacological therapy for ALS. Interestingly, non-pharmacological approaches, such as non-invasive ventilation, have been suggested to prolong the survival in ALS patients (23, 24).
Physical activity is considered as a non-pharmacological therapeutic intervention for many diseases. In the general population, exercise training induces beneficial adaptations in both cardiovascular and neuromuscular systems, including improvements in respiratory and heart function (25), capillary density increase (26), muscle hypertrophy and muscle strength/power increase (27). In addition, it promotes psychological well-being by decreasing depression and anxiety levels, and positively influencing mood state, self-esteem and self-image (28, 29). However, exercise as an approach to reduce ALS pathology is controversial. Previous epidemiological studies suggest that a lifestyle involving vigorous exercise (30–32) and reduced body fat (32) are associated with an increased incidence of ALS. Also, there are studies which suggest an increased risk for ALS among professional soccer and American football players (33, 34). This association is physiologically plausible as strenuous and prolonged exercise may induce oxidative stress, glutamate excitotoxicity and increased calcium loads, which promote selective degeneration of vulnerable motor neurons (35, 36). On the other hand, several neurotrophic factors are up-regulated by exercise in neural and muscle tissue including brain-derived neurotrophic factor (37), which promotes survival and growth of spinal cord cells (38, 39) and glial cell-derived neurotrophic factor (40), which causes synaptic remodeling and hyperinnervation of neuromuscular junctions (41). Based on these findings, the hypothesis that physical activity is a risk factor for developing ALS seems unlikely. Instead, it has been suggested that genetic profiles modified by exogenous factors, which promote physical fitness and raise ALS susceptibility, might be a more credible explanation for the association between physical activity and ALS (17). The discordant results among the epidemiological studies of ALS can be explained by the methodological limitations inherent in studying a relatively low incidence disease. Specifically, most of these studies did not entirely account for potential confounders, such as trauma, possible use of drugs, drugs of abuse, dietary supplements, exposure to environmental toxins such as pesticides or characteristics of physical activity (mode, frequency, duration, intensity) (42, 43).
Multiple studies in ALS mice and patients have been performed to assess the effect of exercise training on disease progression. In preclinical studies, data are conflicting as exercise has been suggested to be beneficial (44), null (45) or even harmful (46) in transgenic SOD1G93A mice. Similarly, in humans, there are studies supporting the benefit of exercise training on the quality of life for ALS patients (47, 48), while other studies concluded that exercise had negative outcomes on neuromuscular function of ALS patients (49). Irrespective of these findings, physical activity is still considered a potential therapeutic approach for ALS improving body function, slowing disease progression and lessening caregiver burden (50). Apart from the biological and mental improvements observed after exercise, physical activity also activates specific mechanisms disrupted during the progression of ALS. For example, exercise evokes signaling pathways modifying skeletal muscle metabolism and triggers structural myofiber remodeling through SC activation (51). Regardless of mode (endurance, resistance or concurrent training), regular exercise lowers basal oxidative stress and increases anti-oxidative capacity (52–54). Moreover, endurance exercise potently increases skeletal muscle mitochondrial content (55, 56), neurogenesis (57, 58) and has a neuroprotective effect on the brain protecting against ischemic neuronal damage in the hippocampal formation (59).
Although evidence supports the advantageous impact of exercise in ALS, it is still not clear whether physical activity should be recommended as a treatment option for ALS patients. Even if exercise training per se is not a sufficient intervention to decelerate disease progression or improve patients' survival, it could be an adjunct therapy to other pharmacological treatments of ALS. Considering the lack of treatments at this time in ALS, non-pharma therapeutics, such as physical activity, is likely to be more important than previously appreciated. This review highlights our knowledge of the role of exercise as a non-pharmacological therapeutic approach for ALS. More specifically, we have critically reviewed both pre-clinical animal and clinical studies to understand whether physical activity could improve the quality of life and life expectancy in ALS, as well as which type of exercise elicits greater compliance and efficacy in ALS patients. While we acknowledge the importance of alternative training methods, such as respiratory muscle training and range-of-motion exercises/stretching, used mainly by respiratory therapists, physiotherapists and occupational therapists to improve physical performance (e.g., respiratory function and flexibility), this review is focused on specific physical activity modes (endurance, resistance, and concurrent training) prescribed by exercise physiologists.
Pre-clinical studies in rodents have assessed the effect of exercise on ALS pathophysiology. Most of them suggest mild-to-moderate endurance exercise as a positive treatment (44, 60–63); whereas, vigorous endurance training seems to have null (45) or harmful (46, 61) effects in SOD1G93A ALS mice (Tables 1, 2). Compared to running-based training, swimming seems to be more beneficial in SOD1G93A mice (64, 65). Therefore, the exercise mode, intensity and duration are likely critical factors in eliciting potential positive effects from endurance training in ALS mice. Interestingly, none of the exercise studies in ALS mice is resistance-based, although resistance training is suggested as a major type of exercise in many disorders, including neuromuscular diseases (66).
Kirkinezos et al. (60) was the first to investigate the effect of exercise in ALS mice and concluded that 10 weeks of endurance treadmill training can significantly extend life expectancy in high-copy number SOD1G93A mice. However, when male and female mice were separately analyzed, the increase in life expectancy was only statistically significant for males, implying that testosterone levels and increased muscle mass may play a protective role in ALS (60). Sex differences in response to endurance training were also reported in ALS mice by Veldink et al. (44). However, in this case, endurance treadmill training delayed the onset of disease only in the SOD1G93A female mice with a low-copy number of transgenes, but not in male mice with a low-copy number (44), suggesting a possible neuroprotective effect of female sex hormones (e.g., estrogens) in ALS. Indeed, the neuroprotective role of estrogens (67, 68) has been suggested as the potential reason why the incidence in ALS is lower in women than in men (69, 70) and why this gender difference declines gradually among older ALS patients (70) when estrogen levels decrease. Considering that testosterone levels also decrease with age (71, 72), a postmenopausal drop in the male:female ratio in ALS may not be fully explained by sex hormones, but inherent limitations that characterize epidemiologic investigations of rare and very rapidly fatal diseases, such as ALS studies.
In any case, the inconsistency of two aforementioned pre-clinical studies (44, 60) could be explained by the different training regime (see Table 1) and transgene copy number [high (60) vs. low (44)] of the SOD1G93A transgene in the mice. Our experience in SOD1G93A mice has shown that treatment efficacy alters to a great degree when the transgene copy number is different. When Veldink et al. (44) repeated the study using only female SOD1G93A mice with a high-copy number of the transgene, they observed a significant delay in total survival time in the exercised group, compared to the sedentary group. This could be associated with the fact that the sedentary SOD1G93A mice (low-copy number) presented a period of anestrus (i.e., lack of a normal estrus cycle) related with low levels of estrogens (73) contrary to the counterpart exercised mice that all of them appear a normal estrus cycle (mice with anestrus: 3/10 vs. 0/10, p = 0.05) (44). Although estrogen levels were not presented in this study (44), as the plasma levels of estrogens were below the detection threshold of their radioimmunoassay, there are numerous studies reporting that the expression of estrogen receptors (74, 75), and serum (74) and cerebellar (76) estrogen levels increase following endurance training. High-dose treatment of 17β-estradiol, which is an estrogen steroid hormone, is suggested to delay ALS disease progression (77, 78) and rescue the lifespan (78) of ovariectomized female SOD1G93A. Surprisingly, histological analysis in the lumbar ventral horns of low-copy SOD1G93A mice showed no significant differences in motor neuron counts and muscle fiber size between exercising and sedentary mice in both male and female animals (44). More extensive histological analysis is needed to investigate whether these results could be explained by the low transgene copy number of SOD1G93A mice.
The intensity of endurance training is a parameter that potentially influences the health outcome of SOD1G93A mice. Carreras et al. found that moderate intensity endurance exercise significantly delayed the onset of motor performance deficit in male SOD1G93A mice (copy number is not reported) (61). This delay correlated with a 2-fold higher motor neuron density in the ventral horn of the lumbar spinal cord. This observation is contrary to results of Veldink et al. (44), who found no significant differences in motor neuron counts between exercising and sedentary low-copy SOD1G93A mice in both male and female after moderate-intensity endurance exercise. Although copy number is not reported by Carreras et al. (61), any mention of SOD1G93A usually refers to the more common high-copy number unless it is not specified. Therefore, the potential different copy number and the different age and, as such, disease stage that mice started exercising [30 days old (61) vs. 56 days old (44)] may explain the discrepancies observed between these two studies. Given that SOD1G93A mice become symptomatic approximately at 80–90 days old (79), experimental designs including exercise training, as a therapeutic approach, which starts before disease onset are not clinically relevant. Such pre-symptomatic studies can provide important information about the effects of exercise on ALS development rather than exercise as an ALS therapeutic on which this review focuses.
Although the study of Carreras et al. (61) was not designed to monitor the longevity of the mice, a trend for higher survival rate was detected among moderate-exercised SOD1G93A mice compared to sedentary or high intensity-exercised SOD1G93A mice (premature deaths: 5/22 moderate-exercised vs. 7/22 sedentary vs. 10/23 high intensity-exercised). In contrast, high intensity endurance exercise significantly hastened the onset of motor performance deficits in SOD1G93A mice (61). In parallel with these results, Mahoney et al. (46) concluded that regular, high intensity endurance training accelerated the deficit in motor performance and reduced survival in male, but not female, SOD1G93A mice. High intensity endurance training did not increase survival in female SOD1G93A mice (46). These sex differences could be explained by the potential neuroprotective role of estrogens, but further investigation is needed for confirming this postulation. Surprisingly, this type of exercise did not affect the onset of clinical symptoms in either male or female SOD1G93A mice (46). Based on the authors' assumptions (46), the detrimental effects of high intensity endurance training on SOD1G93A can be explained either from the intense activation of antioxidant system leading to increased oxidative stress in skeletal muscle and motor neurons or from the increased demand of adenosine triphosphate leading to excessive mitochondrial activation, which disrupts mitochondrial capacity (46). Although these physiological assumptions sound reasonable considering the potential decreased capacity of SOD1G93A mice to restore the exercise-induced neuromuscular damage, more studies are needed for supporting this notion. Similarly, Kassa et al. did not find any change in disease onset after performing high intensity, endurance exercise in SOD1G93A mice (63). Surprisingly, they suggested that this type of exercise may exert a neuroprotective effect on motoneurons acting on glial environment, reducing astrocytic activation and activating a protective microglial phenotype in SOD1G93A mice. This finding seems inconsistent, as exercise did not improve the ALS phenotype. Considering that the sample size was only 5 SOD1G93A mice, these results do not provide scientifically meaningful information.
Generally, high intensity endurance exercise is considered as a more potent stimulus for antioxidant enzyme adaptation than low intensity exercise (80). For this reason, it would be expected that higher exercise intensity may further improve the ALS phenotype and physiological responses of SOD1G93A mice. However, the existing studies suggest that SOD1G93A mice seem more vulnerable to high intensity stress-related stimuli (46, 61). Given that this type of mouse model has mutation in a major antioxidant enzyme (i.e., SOD1), the ability of these mice to deal with increased exercise-induced reactive oxygen species (ROS) levels may be restricted. Therefore, any extrapolation of the results should be interpreted with caution.
The duration of physical activity may also affect the therapeutic benefit associated with endurance exercise in SOD1G93A mice. Six and 12 h, but not 2 h, daily exposure to running wheels improves motor function in SOD1G93A mice with a high-copy number of the transgene, with the 6 h exercise exhibiting the most beneficial effect on lifespan (62). These exercise benefits were observed in both male and female SOD1G93A mice (62). In contrast, Liebetanz et al. (45) concluded that extensive endurance exercise including 10 h daily exposure to running wheels in either gender had no significant effect on the clinical onset and progression of disease or in the lifespan of SOD1G93A mice (copy number is not reported) (45). However, exercised SOD1G93A mice showed an increase in their survival by 1 week compared to the sedentary counterparts, although it was not statistically significant (45). Considering that 1 week in the age of mice corresponds to ~1 year of life for humans when correlating their entire lifespan (81) and that the median survival of ALS patients is between 2 and 4 years from the onset of disease (1), the 1 week improvement in SOD1G93A mice lifespan could be biologically significant, even if it did not reach the statistical significance (p < 0.05). In any case, the sample size (n = 12, 5 females/7 males) used in this study (45) is unlikely underpowered given previous evidence shown through power calculations that n = 8 SOD1G93A mice per group are sufficient to give a significant difference in lifespan means (p = 0.05) with 80% power (82).
Table 1 provides an overview of the effects of exercise intensity and duration, as well as the effects of gender in response to exercise in SOD1G93A mice.
Another type of endurance training investigated in SOD1G93A mice is swimming. Swimming-based training using an adjustable-flow swimming pool can significantly delay the disease onset and extend the mean survival in male SOD1G93A mice compared to sedentary male SOD1G93A mice (64). Surprisingly, no improvement was found with running-based training in the same study (64). Kirkinezos et al. who followed exactly the same training program, found a significant increase in the lifespan of male SOD1G93A mice after running-based training (60). Considering that both studies used a similar sample size of male SOD1G93A mice, the inconsistency of these results may be explained by the different time point that mice started exercising [49 day (60) vs. 70 days (64)]. In any case, findings of pre-clinical studies could have practical applications in clinical conditions only if exercise starts after disease onset. Moreover, only swimming-based training efficiently preserved the muscle phenotype including the number of myofibers, their cross-section area and distribution, in the ALS mice to the extent that it was similar in morphology to the muscles of the control group. Specifically, swimming limited the ALS-induced hypotrophy in both slow- and fast- twitch muscles, as well as maintaining the fast phenotype in fast-twitch muscles (64).
Improvements in glucose metabolism in the SOD1G93A mice after swim training have also been reported (65). Specifically, swimming-based training restored impaired glucose tolerance in late symptomatic SOD1G93A mice (65). On the other hand, running-based training had more modest effects (65). The benefits of swimming-based training were related to changes in skeletal muscle energetic metabolism of SOD1G93A mice, shifting energetic fuels to the anaerobic glycolytic pathway (65). This metabolic shift was associated with an enhanced triglyceride storage in skeletal muscle and reduced the dysregulated expression of autophagic molecules such as Bcl2, Becn1, LC3b, and Sqstm1 (P62) (65). In case of huge energetic demands, autophagy can play a crucial role in skeletal muscle metabolic balance involving the degradation of cellular intrinsic components to produce the three main energetic macromolecules, glucose, lipids and amino acids (83). Given that ALS mice are characterized by an excessive lipid catabolism (84), glucose re-use and fat deposition induced by the swimming-based training are considered beneficial adaptations in SOD1G93A mice. The beneficial role of swimming in ALS energetic metabolism is confirmed by Flis et al. who found improvements in skeletal muscle energy metabolism, oxidative stress, and mitochondrial and endoplasmic reticulum membrane modeling and function in SOD1G93A mice after training in a swimming pool with an adjustable flow (85). The swim training prolonged the lifespan of SOD1G93A mice with accompanying changes including increased caveolin-1, a key regulator of cholesterol efflux, decreased cholesterol accumulation in the crude mitochondrial function, improved bioenergetics (cytochrome c oxidase activity, respiratory capacity ratio, lactate dehydrogenase activity) and decreased oxidative stress.
Swimming also exhibited neuroprotective effects and improved motor function with delayed spinal motoneuron death and preservation of motoneurons with large soma area (64). In contrast, a dramatic motoneuron loss and an increased proportion of motoneurons with small soma area were observed after running-based training. Additionally, reduced astrogliosis and apoptosis of oligodendrocytes were evident in the spinal cord of SOD1G93A mice after swimming-based training (64). This may be explained by the fact that swimming is associated with high hindlimb movement amplitude (cm) and frequency (cycles min−1) exercise, preferentially activating a sub-population of large motoneurons innervating fast motor units (86). In contrast, running is related with low hindlimb movement amplitude and frequency exercise resulting in the activation of a sub-population of small motoneurons (86). Therefore, the combined beneficial effects of swimming on muscle, neuronal tissue and glucose metabolism suggests swimming, at least in ALS SOD1G93A mice, as a very encouraging exercise intervention. Table 2 provides an overview of the physiological adaptations to swimming in SOD1G93A mice.
Overall, mild-to-moderate endurance training seems to have positive effects in SOD1G93A mice, increasing the survival and motor performance as well as delaying the onset and progression of the disease with swimming-based endurance training perhaps the most beneficial type of exercise in ALS SOD1G93A mice. Given that resistance training is suggested as a major type of exercise in many clinical cases, future studies using resistance-based exercise intervention in ALS mice are needed. Furthermore, since ALS is a disease with complex and multiple pathologic abnormalities, successful combinatorial therapeutic approaches with different but complementary mechanism of action may have a beneficial effect in ALS treatment. Scientific evidence suggests running with ad libitum exposure in running wheels, combined with virally-induced Insulin Growth Factor-1 overexpression, has synergistic and maximal effects on survival and motor function of SOD1G93A mice, compared with either monotherapy-treated or no-treated SOD1G93A mice (62). As a result, exercise training could be an adjunct therapy to other pharmacological treatments of ALS, but further investigation is needed to confirm this notion. Finally, an additional limitation is that the SOD1G93A transgenic mouse, the most extensively used mouse model, is not representative of all ALS cases as mutations in the SOD1 gene account for only 2% of all ALS, primarily familial, cases (87). In addition, SOD1 gene is a major antioxidant enzyme and its mutation may make SOD1 mice unable to deal with any exercise-induced oxidative-stress. For these reasons, pre-clinical studies involving additional animal models based on both sporadic and familial ALS phenotype (88–90), are needed to advance our understanding of the effects of exercise in ALS.
Several clinical studies have been performed to determine whether endurance and/or resistance exercise ameliorates symptoms and improves the health of ALS patients. Regardless of its modality, exercise seems to have beneficial effects on the quality of life of ALS patients, but its impact on survival has not been confirmed. In any case, no negative outcomes have been found in ALS progression after an exercise intervention.
Even though endurance exercise promotes cardiorespiratory fitness, cellular metabolism increasing mitochondrial biogenesis and intramuscular fuel stores, and psychological well-being, little is known about its effect on ALS pathophysiology. Pinto et al. (91) investigated the possibility of reducing motor decline by exercising ALS patients to the anaerobic threshold, simultaneously ensuring their respiratory insufficiency with the assistance of a non-invasive ventilator, the Bipap STD®. ALS patients performing a ramp treadmill exercise protocol for 1 year decreased the rate of respiratory deterioration and improved their functional independent mobility score, compared to a non-exercised control group (91). Similarly, Sanjak et al. (92) demonstrated that an 8 week walking program on a weight-supported treadmill for 30 min, three times weekly, significantly improved the ALS Functional Rating Scale (ALSFRS) score as well as tolerability, gait speed, distance and stride length during 6 min walk tests for ALS patients (92). The feasibility of performing moderate endurance training with non-invasive ventilation or body weight supporting system in ALS patients was confirmed recently where improvements in functionality and cardiorespiratory factors were found (93). The same research group also suggested that tele-monitored home-based endurance exercise consisting of 15 min walking on a treadmill or outdoors, is feasible, safe, user-friendly and had excellent compliance in ALS patients (94). Despite the absence of a non-exercise ALS control group in this study, there were no differences in cardiorespiratory factors (percentage of saturation of oxygen and force vital capacity) observed over the course of the exercise program (6 months). This implies a beneficial role of a home-based endurance training in ALS patients protecting them from the degenerative effects during disease progression. On the other hand, Clawson et al. recently reported that 24 week endurance training in both lower and upper body using a minicycle was not as well-tolerated as resistance training or stretching/range of motion exercises (95). Although the endurance training was not harmful for ALS patients in this study, it did not increase their muscle strength, functionality or quality of life. The inconsistency of these results could be explained by the different type of endurance training [cycling (95) vs. walking (91–94)] and the different muscle groups [upper and lower limbs (95) vs. only lower limbs (91–94)] activated during training sessions.
While respiratory muscle training is beyond the scope of this review, it is worth highlighting its potential to improve respiratory function, one of the major factors of aerobic/endurance training, in ALS patients. Specifically, scientific evidence shows that a 12 week inspiratory muscle training programme consisted of inhaling and exhaling through a specific device (Respironics®) may slow the decline in respiratory function in ALS patients through strengthening their inspiratory muscles (96). However, more detailed clinical assessment is needed as a later study using this type of training did not find either negative or positive effects on respiratory function of ALS patients (97). Furthermore, a combinatorial training including both respiratory muscle and endurance training could potentially induce positive adaptations for ALS patients, but this requires experimental validation.
Overall, endurance training with a supplemental support such as ventilation or weight support seems to have positive effects on respiratory capacity, functionality, tolerability and physical performance in ALS patients (Table 3), but further studies with a larger number of participants and inclusion of a control group are needed to confirm these findings. Given that each type of endurance training activates particular muscle groups and creates specific mechanical loading, different adaptations are induced by walking, cycling and swimming. Although swimming is suggested as an advantageous type of exercise in many neurological disorders (98–100), its beneficial role has not yet been experimentally validated in ALS patients. For this reason, further investigation is required to optimize an endurance training protocol for ALS patients.
Resistance exercise improves muscle force/power, induces muscle hypertrophy, maintains skeletal muscle function and prevents disability (101). In ALS patients, resistance training imparts protective benefits despite not reducing disease progression (Table 4). One of the first published reports of resistance training in ALS patients concluded that resistance exercise to the upper extremities can increase muscle static strength of the upper body (102). A subsequent study observed that moderate regular resistance training may have a mild, temporary positive effect on motor deficit and disability in ALS patients (103). Specifically, modest-intensity resistance exercises involving most muscle groups of the four limbs and trunk, induced significant improvements on functional and spasticity scores, but not on muscle strength, fatigue, pain and quality-of-life scores after 3 months of intervention (103). However, after 6, 9, or 12 months of resistance training, no further change was observed in these parameters. Similarly, Kitano et al. (104) recently suggested a 6 month home-based, whole-body strength and stretching exercise program as a safe mode of exercise training without adverse effects in ALS patients. This exercise program alleviated the functional deterioration in patients with early- stage ALS, but did not improve their upper and lower body muscle strength. In addition to these results, a new study showed that although 24 week resistance training using both lower and upper body is well-tolerated and safe for ALS patients, it is not sufficient to improve their muscle strength, functionality or quality of life (95).
Furthermore, Lunetta et al. found that a 6 month strength training program, based on either active exercises against gravity in upper and lower limbs or passive exercises consisting of flexion-extension movements in the upper and lower limbs, cannot improve the functionality (ALSFRS total score), survival or quality of life in ALS patients, even though they were reporting subjectively an improvement in their sense of well-being at the end of every exercise session (48). On the other hand, a randomized controlled trial showed that a 6 month resistance training program with moderate intensity and moderate load involving both lower- and upper-body muscles, can induce significantly better function, less decline in leg strength, and higher quality of life in ALS patients without any adverse effects (47).
In contrast, negative outcomes in neuromuscular function of ALS patients have been found after 12 weeks of resistance training (49). The whole-body resistance training did not significantly affect functionality and average cross-section area of type I and II muscle fibers, but loss of muscle strength and power in both lower and upper body muscle groups increased following the training period (49). Also, an increase in the percentage of both atrophied and greatly (abnormal) hypertrophied type II fibers combined with a decrease in normal-sized type II fibers were observed after the training period (49). Taking into account of the small number of patients (only 5) recruited in this study (49), their heterogeneous disease progression and the study design (12 week “lead-in” control period without exercising), the findings should be interpreted with caution. In ALS studies, the use of a lead-in control period (i.e., a period with no exercise), before receiving an intervention instead of using a control, non-exercised ALS group may confound the final results. Considering the potential rapid decline in health and the immediacy of death in ALS patients, the muscle fiber atrophy and the deteriorated muscle strength after resistance training may be partly explained by the disease progression occurring during the 12 week lead-in period rather than by any detrimental effects caused by exercise.
In summary, most studies suggest that resistance training can improve the quality of life of ALS patients increasing their functionality and, in some cases, their muscle strength. However, resistance training seems to have no effect on the lifespan of ALS patients. Future attempts to restrict, as much as possible, the inherent limitations of ALS clinical studies such as the small sample size and the heterogeneity of patients are needed for confirming the existing findings. Ideally, further studies with a stronger experimental design including a greater number of participants with homogeneous disease progression would provide more valid information about the effects of resistance training in ALS patients. Unfortunately, the reality is different as there are too few patients and only a small proportion of them eventually participates in exercise clinical trials.
Concurrent training is a type of exercise that combines endurance and resistance training models and can stimulate both aerobic and anaerobic metabolic pathways, inducing a variety of beneficial adaptations. A recent randomized controlled trial suggested that a 6 month exercise program involving a combination of resistance and endurance training can reduce the motor deterioration of ALS patients (48). Specifically, the exercise training consisting of active exercises against gravity in the upper and lower limbs combined with cycle ergometer activity for 20 min increased the functionality (ALSFRS total score) of ALS patients. Furthermore, all exercised ALS patients reported subjectively an improvement in their sense of well-being at the end of every exercise session, although no improvements in their survival, respiratory function and quality of life were observed (48). However, the limited number of patients (n = 10, sex ratio is not reported) (48) raises concerns about the interpretation of these findings and, therefore, these results should be considered with caution. Additionally, a recent study suggested concurrent training as a feasible and beneficial intervention for ALS patients (105). Specifically, Merico et al. found that 5 week moderate, submaximal resistance and endurance training improved the functional independence, oxygen consumption, fatigue and muscle strength in ALS patients (105). The positive effects of combined endurance and resistance training in ALS patients were also confirmed in a retrospective, non-consecutive case series study (106). In this study, 2 week endurance training on a cycle ergometer combined with lower-body resistance training appeared feasible and beneficial for ALS patients improving their muscle strength at the early stage of disease but not at the late stage of disease (106). Given that initial improvements in muscle strength, especially among untrained people, are explained mainly by the increased voluntary neural activation of the trained muscles and not by muscle hypertrophy which has a gradually increasing role in strength development as the training proceeds (after the first 3 to 5 weeks) (107, 108), a longer exercise intervention (>5 weeks) could induce further and greater skeletal muscle adaptations in ALS patients at either early or late stage of disease. In addition, while encouraging, these findings should be interpreted carefully considering the lack of statistical analysis due to the very small sample size (n = 2) used in this study (106). An overview of the effects of concurrent endurance and resistance training in ALS patients is summarized in Table 5.
Given the inherent methodological limitations involving clinical studies of rare and rapidly fatal diseases, such as ALS studies, further investigation with sufficient cohort size, limited variability of ALS causality, less heterogeneity in the disease stage of patients and adequate control of confounders is needed for confirming the existing findings of endurance, resistance, and concurrent training clinical studies in ALS patients. In addition, experimental designs including control group and proper statistical analysis are important for concluding to valid results. Also, future studies involving alternative training modes with less risk of muscle damage and injuries, such as swimming, could provide useful information to conclude to an optimal exercise program for ALS patients. Recent pre-clinical studies suggest swimming (64, 65, 85) as the most beneficial type of exercise in ALS, but clinical studies are needed to translate these mouse exercise results to humans.
Although previous evidence suggests a potential relationship between heavily active lifestyles and an increased incidence of ALS (30, 31, 109), recent studies support that physical activity is not necessarily a risk factor for ALS (42, 43, 110). There is now a large body of evidence suggesting physical activity as a potential therapeutic or even holistic approach for ALS.
Pre-clinical mouse studies conclude that the intensity, duration and mode of exercise, as well as gender, can influence health outcomes in ALS progression as identified in SOD1G93A mice in response to regular long-term endurance training (44, 61, 62, 64). Findings from this review suggest that mild-to-moderate endurance training is a potential beneficial exercise intervention for ALS SOD1G93A mice (44, 60, 61), in particular, swimming (64, 65, 85). However, many of these pre-clinical studies are characterized by limitations related to variable sample sizes, heterogeneous transgene copy number or a non-clinically-relevant timing of intervention. An additional limitation is the lack of pre-clinical studies focused on the effect of resistance-based exercise, a major type of exercise, in ALS. A current in vivo study shows that repeated sessions of isometric tetanic contractions, which represent a resistance-based exercise protocol for experimental animals, can improve contractile function and ameliorate distinct histopathological features of skeletal muscle in Duchenne muscular dystrophy circumventing the concern of potentially injurious eccentric contractions (111). A similar resistance-based exercise program including non-injurious isometric contractions could also have positive effects in skeletal muscle of ALS mice. Further pre-clinical studies are needed to investigate the effects of exercise not only per se but also as an adjunct therapy to other pharmacological treatments of ALS. Finally, except for the SOD1G93A transgenic mouse, which represents only the 2% of all, primarily familial, cases, additional animal models based on both sporadic and familial ALS phenotype (88–90) are needed to advance our understanding of the effects of exercise in ALS.
Despite mild-to-moderate exercise training improving the survival of ALS mice, currently, there is no clinical evidence supporting this notion in ALS patients (48). Regardless, most clinical studies suggest that every type of exercise training including stretching, resistance, endurance or concurrent training, has an advantageous impact on the quality of life of ALS patients increasing mainly their functionality (47, 48, 91–93, 103, 105) and sometimes their muscle strength (105, 106) and/or their cardiorespiratory function (91, 93, 105). The discrepancy between preclinical and clinical findings could be partly explained by the limitations observed in most of clinical studies in ALS, such as a small sample size, non-representative control populations, inadequate control of confounders and heterogeneous disease stage of patients.
Importantly, the effect of swimming has not yet been experimentally validated in ALS patients, although exercising in water is commonly recommended for patients with other neurological disorders such as Parkinson's disease and multiple sclerosis (98–100). Given that swimming movements mainly incorporate concentric muscle contraction, a swim-based training may protect skeletal muscles of ALS patients from the high muscle stress induced by eccentric muscle contraction experienced with other types of exercise, such as running or resistance training. Furthermore, the water minimizes biomechanical stress on muscles and joints decreasing the risk of muscle damages and injuries. As water itself creates resistance to movement, performing exercises in water has been suggested as an alternative training mode to improve neuromuscular conditioning in healthy population (112, 113). Considering all these aspects, swimming with supplemental support or aquatic exercise training could be a potential exercise treatment for ALS patients.
While the benefits of exercise in ALS patients are still not clear, nor have the effects of swimming yet been determined, there appears promise ahead for further studies investigating the therapeutic benefits of exercise, which should be supported by well-designed and statistically powered, pre-clinical studies in multiple rodent models of ALS. In addition to the physical impact, the holistic value of exercise may also be key to the improved well-being of ALS patients.
All authors listed have made a substantial, direct and intellectual contribution to the work, and approved it for publication.
Deakin University (Victoria, Australia) provided funding support for publishing this work.
The authors declare that the research was conducted in the absence of any commercial or financial relationships that could be construed as a potential conflict of interest.
ST was supported by an International Postgraduate Research Scholarship, awarded by the Deakin University (Victoria, Australia) and a Greek Scholarship for doctoral studies abroad, awarded by the Onassis Foundation (Greece).
1. Chio A, Logroscino G, Hardiman O, Swingler R, Mitchell D, Beghi E, et al. Prognostic factors in ALS: a critical review. Amyotroph Lateral Scler. (2009) 10:310–23. doi: 10.3109/17482960802566824
2. Chio A, Logroscino G, Traynor BJ, Collins J, Simeone JC, Goldstein LA, et al. Global epidemiology of amyotrophic lateral sclerosis: a systematic review of the published literature. Neuroepidemiology. (2013) 41:118–30. doi: 10.1159/000351153
3. Spreux-Varoquaux O, Bensimon G, Lacomblez L, Salachas F, Pradat PF, Le Forestier N, et al. Glutamate levels in cerebrospinal fluid in amyotrophic lateral sclerosis: a reappraisal using a new HPLC method with coulometric detection in a large cohort of patients. J Neurol Sci. (2002) 193:73–8. doi: 10.1016/S0022-510X(01)00661-X
4. Roy J, Minotti S, Dong L, Figlewicz DA, Durham HD. Glutamate potentiates the toxicity of mutant Cu/Zn-superoxide dismutase in motor neurons by postsynaptic calcium-dependent mechanisms. J Neurosci. (1998) 18:9673–84. doi: 10.1523/JNEUROSCI.18-23-09673.1998
5. De Vos KJ, Grierson AJ, Ackerley S, Miller CC. Role of axonal transport in neurodegenerative diseases. Annu Rev Neurosci. (2008) 31:151–73. doi: 10.1146/annurev.neuro.31.061307.090711
6. Morfini GA, Burns M, Binder LI, Kanaan NM, LaPointe N, Bosco DA, et al. Axonal transport defects in neurodegenerative diseases. J Neurosci. (2009) 29:12776–86. doi: 10.1523/JNEUROSCI.3463-09.2009
7. Hirano A, Donnenfeld H, Sasaki S, Nakano I. Fine structural observations of neurofilamentous changes in amyotrophic lateral sclerosis. J Neuropathol Exp Neurol. (1984) 43:461–70. doi: 10.1097/00005072-198409000-00001
8. Williamson TL, Bruijn LI, Zhu Q, Anderson KL, Anderson SD, Julien JP, et al. Absence of neurofilaments reduces the selective vulnerability of motor neurons and slows disease caused by a familial amyotrophic lateral sclerosis-linked superoxide dismutase 1 mutant. Proc Natl Acad Sci USA. (1998) 95:9631–6. doi: 10.1073/pnas.95.16.9631
9. Halter B, Gonzalez de Aguilar JL, Rene F, Petri S, Fricker B, Echaniz-Laguna A, et al. Oxidative stress in skeletal muscle stimulates early expression of Rad in a mouse model of amyotrophic lateral sclerosis. Free Radic Biol Med. (2010) 48:915–23. doi: 10.1016/j.freeradbiomed.2010.01.014
10. Beal MF, Ferrante RJ, Browne SE, Matthews RT, Kowall NW, Brown RH Jr. Increased 3-nitrotyrosine in both sporadic and familial amyotrophic lateral sclerosis. Ann Neurol. (1997) 42:644–54. doi: 10.1002/ana.410420416
11. Blokhuis AM, Groen EJ, Koppers M, van den Berg LH, Pasterkamp RJ. Protein aggregation in amyotrophic lateral sclerosis. Acta Neuropathol. (2013) 125:777–94. doi: 10.1007/s00401-013-1125-6
12. Elliott JL. Cytokine upregulation in a murine model of familial amyotrophic lateral sclerosis. Brain Res Mol Brain Res. (2001) 95:172–8. doi: 10.1016/S0169-328X(01)00242-X
13. Henkel JS, Engelhardt JI, Siklos L, Simpson EP, Kim SH, Pan T, et al. Presence of dendritic cells, MCP-1, and activated microglia/macrophages in amyotrophic lateral sclerosis spinal cord tissue. Ann Neurol. (2004) 55:221–35. doi: 10.1002/ana.10805
14. Tsitkanou S, Della Gatta PA, Russell AP. Skeletal muscle satellite cells, mitochondria, and microRNAs: their involvement in the pathogenesis of ALS. Front Physiol. (2016) 7:403. doi: 10.3389/fphys.2016.00403
15. Boyer JG, Ferrier A, Kothary R. More than a bystander: the contributions of intrinsic skeletal muscle defects in motor neuron diseases. Front Physiol. (2013) 4:356. doi: 10.3389/fphys.2013.00356
16. Russell AP, Wada S, Vergani L, Hock MB, Lamon S, Leger B, et al. Disruption of skeletal muscle mitochondrial network genes and miRNAs in amyotrophic lateral sclerosis. Neurobiol Dis. (2013) 49:107–17. doi: 10.1016/j.nbd.2012.08.015
17. Menzies FM, Ince PG, Shaw PJ. Mitochondrial involvement in amyotrophic lateral sclerosis. Neurochem Int. (2002) 40:543–51. doi: 10.1016/S0197-0186(01)00125-5
18. Faes L, Callewaert G. Mitochondrial dysfunction in familial amyotrophic lateral sclerosis. J Bioenerg Biomembr. (2011) 43:587–92. doi: 10.1007/s10863-011-9393-0
19. Scaramozza A, Marchese V, Papa V, Salaroli R, Soraru G, Angelini C, et al. Skeletal muscle satellite cells in amyotrophic lateral sclerosis. Ultrastruct Pathol. (2014) 38:295–302. doi: 10.3109/01913123.2014.937842
20. Manzano R, Toivonen JM, Calvo AC, Olivan S, Zaragoza P, Munoz MJ, et al. Quantity and activation of myofiber-associated satellite cells in a mouse model of amyotrophic lateral sclerosis. Stem Cell Rev. (2012) 8:279–87. doi: 10.1007/s12015-011-9268-0
21. Williams AH, Valdez G, Moresi V, Qi X, McAnally J, Elliott JL, et al. MicroRNA-206 delays ALS progression and promotes regeneration of neuromuscular synapses in mice. Science. (2009) 326:1549–54. doi: 10.1126/science.1181046
22. Cozzolino M, Ferri A, Carri MT. Amyotrophic lateral sclerosis: from current developments in the laboratory to clinical implications. Antioxid Redox Signal. (2008) 10:405–43. doi: 10.1089/ars.2007.1760
23. Aboussouan LS, Khan SU, Meeker DP, Stelmach K, Mitsumoto H. Effect of noninvasive positive-pressure ventilation on survival in amyotrophic lateral sclerosis. Ann Intern Med. (1997) 127:450–3. doi: 10.7326/0003-4819-127-6-199709150-00006
24. Sanjuan-Lopez P, Valino-Lopez P, Ricoy-Gabaldon J, Verea-Hernando H. Amyotrophic lateral sclerosis: impact of pulmonary follow-up and mechanical ventilation on survival. A study of 114 cases. Arch Bronconeumol. (2014) 50:509–13. doi: 10.1016/j.arbr.2014.10.008
25. Lin X, Zhang X, Guo J, Roberts CK, McKenzie S, Wu WC, et al. Effects of exercise training on cardiorespiratory fitness and biomarkers of cardiometabolic health: a systematic review and meta-analysis of randomized controlled trials. J Am Heart Assoc. (2015) 4:e002014. doi: 10.1161/JAHA.115.002014
26. Laughlin MH, Roseguini B. Mechanisms for exercise training-induced increases in skeletal muscle blood flow capacity: differences with interval sprint training versus aerobic endurance training. J Physiol Pharmacol. (2008) 59(Suppl 7):71–88.
27. Ahtiainen JP, Pakarinen A, Alen M, Kraemer WJ, Hakkinen K. Muscle hypertrophy, hormonal adaptations and strength development during strength training in strength-trained and untrained men. Eur J Appl Physiol. (2003) 89:555–63. doi: 10.1007/s00421-003-0833-3
28. Bauman AE. Updating the evidence that physical activity is good for health: an epidemiological review 2000-2003. J Sci Med Sport. (2004) 7(Suppl. 1):6–19. doi: 10.1016/S1440-2440(04)80273-1
29. Scully D, Kremer J, Meade MM, Graham R, Dudgeon K. Physical exercise and psychological well being: a critical review. Br J Sports Med. (1998) 32:111–20. doi: 10.1136/bjsm.32.2.111
30. Strickland D, Smith SA, Dolliff G, Goldman L, Roelofs RI. Physical activity, trauma, and ALS: a case-control study. Acta Neurol Scand. (1996) 94:45–50. doi: 10.1111/j.1600-0404.1996.tb00038.x
31. Gotkine M, Friedlander Y, Hochner H. Triathletes are over-represented in a population of patients with ALS. Amyotroph Lateral Scler Frontotemporal Degener. (2014) 15:534–6. doi: 10.3109/21678421.2014.932383
32. Scarmeas N, Shih T, Stern Y, Ottman R, Rowland LP. Premorbid weight, body mass, and varsity athletics in ALS. Neurology. (2002) 59:773–5. doi: 10.1212/WNL.59.5.773
33. Chio A, Benzi G, Dossena M, Mutani R, Mora G. Severely increased risk of amyotrophic lateral sclerosis among Italian professional football players. Brain. (2005) 128(Pt 3):472–6. doi: 10.1093/brain/awh373
34. Lehman EJ, Hein MJ, Baron SL, Gersic CM. Neurodegenerative causes of death among retired National Football League players. Neurology. (2012) 79:1970–4. doi: 10.1212/WNL.0b013e31826daf50
35. Harwood CA, McDermott CJ, Shaw PJ. Physical activity as an exogenous risk factor in motor neuron disease (MND): a review of the evidence. Amyotroph Lateral Scler. (2009) 10:191–204. doi: 10.1080/17482960802549739
36. Harwood CA, Westgate K, Gunstone S, Brage S, Wareham NJ, McDermott CJ, et al. Long-term physical activity: an exogenous risk factor for sporadic amyotrophic lateral sclerosis? Amyotroph Lateral Scler Frontotemporal Degener. (2016) 17:377–84. doi: 10.3109/21678421.2016.1154575
37. Gomez-Pinilla F, Ying Z, Opazo P, Roy RR, Edgerton VR. Differential regulation by exercise of BDNF and NT-3 in rat spinal cord and skeletal muscle. Eur J Neurosci. (2001) 13:1078–84. doi: 10.1046/j.0953-816x.2001.01484.x
38. Koliatsos VE, Clatterbuck RE, Winslow JW, Cayouette MH, Price DL. Evidence that brain-derived neurotrophic factor is a trophic factor for motor neurons in vivo. Neuron. (1993) 10:359–67. doi: 10.1016/0896-6273(93)90326-M
39. Friedman B, Kleinfeld D, Ip NY, Verge VM, Moulton R, Boland P, et al. BDNF and NT-4/5 exert neurotrophic influences on injured adult spinal motor neurons. J Neurosci. (1995) 15:1044–56. doi: 10.1523/JNEUROSCI.15-02-01044.1995
40. Wehrwein EA, Roskelley EM, Spitsbergen JM. GDNF is regulated in an activity-dependent manner in rat skeletal muscle. Muscle Nerve. (2002) 26:206–11. doi: 10.1002/mus.10179
41. Nguyen QT, Parsadanian AS, Snider WD, Lichtman JW. Hyperinnervation of neuromuscular junctions caused by GDNF overexpression in muscle. Science. (1998) 279:1725–9. doi: 10.1126/science.279.5357.1725
42. Luna J, Logroscino G, Couratier P, Marin B. Current issues in ALS epidemiology: variation of ALS occurrence between populations and physical activity as a risk factor. Rev Neurol. (2017) 173:244–53. doi: 10.1016/j.neurol.2017.03.035
43. Huisman MH, Seelen M, de Jong SW, Dorresteijn KR, van Doormaal PT, van der Kooi AJ, et al. Lifetime physical activity and the risk of amyotrophic lateral sclerosis. J Neurol Neurosurg Psychiatry. (2013) 84:976–81. doi: 10.1136/jnnp-2012-304724
44. Veldink JH, Bar PR, Joosten EA, Otten M, Wokke JH, van den Berg LH. Sexual differences in onset of disease and response to exercise in a transgenic model of ALS. Neuromuscul Disord. (2003) 13:737–43. doi: 10.1016/S0960-8966(03)00104-4
45. Liebetanz D, Hagemann K, von Lewinski F, Kahler E, Paulus W. Extensive exercise is not harmful in amyotrophic lateral sclerosis. Eur J Neurosci. (2004) 20:3115–20. doi: 10.1111/j.1460-9568.2004.03769.x
46. Mahoney DJ, Rodriguez C, Devries M, Yasuda N, Tarnopolsky MA. Effects of high-intensity endurance exercise training in the G93A mouse model of amyotrophic lateral sclerosis. Muscle Nerve. (2004) 29:656–62. doi: 10.1002/mus.20004
47. Bello-Haas VD, Florence JM, Kloos AD, Scheirbecker J, Lopate G, Hayes SM, et al. A randomized controlled trial of resistance exercise in individuals with ALS. Neurology. (2007) 68:2003–7. doi: 10.1212/01.wnl.0000264418.92308.a4
48. Lunetta C, Lizio A, Sansone VA, Cellotto NM, Maestri E, Bettinelli M, et al. Strictly monitored exercise programs reduce motor deterioration in ALS: preliminary results of a randomized controlled trial. J Neurol. (2016) 263:52–60. doi: 10.1007/s00415-015-7924-z
49. Jensen L, Djurtoft JB, Bech RD, Nielsen JL, Jorgensen LH, Schroder HD, et al. Influence of resistance training on neuromuscular function and physical capacity in ALS patients. J Neurodegener Dis. (2017) 2017:1436519. doi: 10.1155/2017/1436519
50. Chen A, Montes J, Mitsumoto H. The role of exercise in amyotrophic lateral sclerosis. Phys Med Rehabil Clin N Am. (2008) 19:545–57. doi: 10.1016/j.pmr.2008.02.003
51. Ferraro E, Giammarioli AM, Chiandotto S, Spoletini I, Rosano G. Exercise-induced skeletal muscle remodeling and metabolic adaptation: redox signaling and role of autophagy. Antioxid Redox Signal. (2014) 21:154–76. doi: 10.1089/ars.2013.5773
52. Azizbeigi K, Stannard SR, Atashak S, Haghighi MM. Antioxidant enzymes and oxidative stress adaptation to exercise training: comparison of endurance, resistance, and concurrent training in untrained males. J Exerc Sci Fit. (2014) 12:1–6. doi: 10.1016/j.jesf.2013.12.001
53. Ji LL. Exercise-induced modulation of antioxidant defense. Ann N Y Acad Sci. (2002) 959:82–92. doi: 10.1111/j.1749-6632.2002.tb02085.x
54. Balakrishnan SD, Anuradha CV. Exercise, depletion of antioxidants and antioxidant manipulation. Cell Biochem Funct. (1998) 16:269–75.
55. Terada S, Goto M, Kato M, Kawanaka K, Shimokawa T, Tabata I. Effects of low-intensity prolonged exercise on PGC-1 mRNA expression in rat epitrochlearis muscle. Biochem Biophys Res Commun. (2002) 296:350–4. doi: 10.1016/S0006-291X(02)00881-1
56. Wright DC, Han DH, Garcia-Roves PM, Geiger PC, Jones TE, Holloszy JO. Exercise-induced mitochondrial biogenesis begins before the increase in muscle PGC-1alpha expression. J Biol Chem. (2007) 282:194–9. doi: 10.1074/jbc.M606116200
57. Rhodes JS, van Praag H, Jeffrey S, Girard I, Mitchell GS, Garland T Jr, et al. Exercise increases hippocampal neurogenesis to high levels but does not improve spatial learning in mice bred for increased voluntary wheel running. Behav Neurosci. (2003) 117:1006–16. doi: 10.1037/0735-7044.117.5.1006
58. van Praag H, Kempermann G, Gage FH. Running increases cell proliferation and neurogenesis in the adult mouse dentate gyrus. Nat Neurosci. (1999) 2:266–70. doi: 10.1038/6368
59. Stummer W, Weber K, Tranmer B, Baethmann A, Kempski O. Reduced mortality and brain damage after locomotor activity in gerbil forebrain ischemia. Stroke. (1994) 25:1862–9. doi: 10.1161/01.STR.25.9.1862
60. Kirkinezos IG, Hernandez D, Bradley WG, Moraes CT. Regular exercise is beneficial to a mouse model of amyotrophic lateral sclerosis. Ann Neurol. (2003) 53:804–7. doi: 10.1002/ana.10597
61. Carreras I, Yuruker S, Aytan N, Hossain L, Choi JK, Jenkins BG, et al. Moderate exercise delays the motor performance decline in a transgenic model of ALS. Brain Res. (2010) 1313:192–201. doi: 10.1016/j.brainres.2009.11.051
62. Kaspar BK, Frost LM, Christian L, Umapathi P, Gage FH. Synergy of insulin-like growth factor-1 and exercise in amyotrophic lateral sclerosis. Ann Neurol. (2005) 57:649–55. doi: 10.1002/ana.20451
63. Kassa RM, Bonafede R, Boschi F, Bentivoglio M, Mariotti R. Effect of physical exercise and anabolic steroid treatment on spinal motoneurons and surrounding glia of wild-type and ALS mice. Brain Res. (2017) 1657:269–78. doi: 10.1016/j.brainres.2016.12.029
64. Deforges S, Branchu J, Biondi O, Grondard C, Pariset C, Lecolle S, et al. Motoneuron survival is promoted by specific exercise in a mouse model of amyotrophic lateral sclerosis. J Physiol. (2009) 587(Pt 14):3561–72. doi: 10.1113/jphysiol.2009.169748
65. Desseille C, Deforges S, Biondi O, Houdebine L, D'Amico D, Lamaziere A, et al. Specific physical exercise improves energetic metabolism in the skeletal muscle of amyotrophic-lateral- sclerosis mice. Front Mol Neurosci. (2017) 10:332. doi: 10.3389/fnmol.2017.00332
66. Krivickas LS. Exercise in neuromuscular disease. J Clin Neuromuscul Dis. (2003) 5:29–39. doi: 10.1097/00131402-200309000-00004
67. Bar PR, Amelink GJ. Protection against muscle damage exerted by oestrogen: hormonal or antioxidant action? Biochem Soc Trans. (1997) 25:50–4. doi: 10.1042/bst0250050
68. Green PS, Simpkins JW. Neuroprotective effects of estrogens: potential mechanisms of action. Int J Dev Neurosci. (2000) 18:347–58. doi: 10.1016/S0736-5748(00)00017-4
69. Manjaly ZR, Scott KM, Abhinav K, Wijesekera L, Ganesalingam J, Goldstein LH, et al. The sex ratio in amyotrophic lateral sclerosis: a population based study. Amyotroph Lateral Scler. (2010) 11:439–42. doi: 10.3109/17482961003610853
70. McCombe PA, Henderson RD. Effects of gender in amyotrophic lateral sclerosis. Gend Med. (2010) 7:557–70. doi: 10.1016/j.genm.2010.11.010
71. Golan R, Scovell JM, Ramasamy R. Age-related testosterone decline is due to waning of both testicular and hypothalamic-pituitary function. Aging Male. (2015) 18:201–4. doi: 10.3109/13685538.2015.1052392
72. Rajfer J. Decreased testosterone in the aging male: summary and conclusions. Rev Urol. (2003) 5(Suppl. 1):S49–50.
73. Edwards DA. Induction of estrus in female mice: estrogen-progesterone interactions. Horm Behav. (1970) 1:299–304. doi: 10.1016/0018-506X(70)90022-X
74. Hao L, Wang Y, Duan Y, Bu S. Effects of treadmill exercise training on liver fat accumulation and estrogen receptor alpha expression in intact and ovariectomized rats with or without estrogen replacement treatment. Eur J Appl Physiol. (2010) 109:879–86. doi: 10.1007/s00421-010-1426-6
75. Paquette A, Wang D, Gauthier MS, Prud'homme D, Jankowski M, Gutkowska J, et al. Specific adaptations of estrogen receptor alpha and beta transcripts in liver and heart after endurance training in rats. Mol Cell Biochem. (2007) 306:179–87. doi: 10.1007/s11010-007-9568-5
76. Rauf S, Soejono SK, Partadiredja G. Effects of treadmill exercise training on cerebellar estrogen and estrogen receptors, serum estrogen, and motor coordination performance of ovariectomized rats. Iran J Basic Med Sci. (2015) 18:587–92.
77. Groeneveld GJ, Van Muiswinkel FL, Sturkenboom JM, Wokke JH, Bar PR, Van den Berg LH. Ovariectomy and 17beta-estradiol modulate disease progression of a mouse model of ALS. Brain Res. (2004) 1021:128–31. doi: 10.1016/j.brainres.2004.06.024
78. Choi CI, Lee YD, Gwag BJ, Cho SI, Kim SS, Suh-Kim H. Effects of estrogen on lifespan and motor functions in female hSOD1 G93A transgenic mice. J Neurol Sci. (2008) 268:40–7. doi: 10.1016/j.jns.2007.10.024
79. Weydt P, Hong SY, Kliot M, Moller T. Assessing disease onset and progression in the SOD1 mouse model of ALS. Neuroreport. (2003) 14:1051–4. doi: 10.1097/01.wnr.0000073685.00308.89
80. Powers SK, Hamilton K. Antioxidants and exercise. Clin Sports Med. (1999) 18:525–36. doi: 10.1016/S0278-5919(05)70166-6
81. Dutta S, Sengupta P. Men and mice: relating their ages. Life Sci. (2016) 152:244–8. doi: 10.1016/j.lfs.2015.10.025
82. Hogg MC, Halang L, Woods I, Coughlan KS, Prehn JHM. Riluzole does not improve lifespan or motor function in three ALS mouse models. Amyotroph Lateral Scler Frontotemporal Degener. (2018) 19:438–45. doi: 10.1080/21678421.2017.1407796
83. Singh R, Cuervo AM. Autophagy in the cellular energetic balance. Cell Metab. (2011) 13:495–504. doi: 10.1016/j.cmet.2011.04.004
84. Dupuis L, Oudart H, Rene F, Gonzalez de Aguilar JL, Loeffler JP. Evidence for defective energy homeostasis in amyotrophic lateral sclerosis: benefit of a high-energy diet in a transgenic mouse model. Proc Natl Acad Sci USA. (2004) 101:11159–64. doi: 10.1073/pnas.0402026101
85. Flis DJ, Dzik K, Kaczor JJ, Halon-Golabek M, Antosiewicz J, Wieckowski MR, et al. Swim training modulates skeletal muscle energy metabolism, oxidative stress, and mitochondrial cholesterol content in amyotrophic lateral sclerosis mice. Oxid Med Cell Longev. (2018) 2018:5940748. doi: 10.1155/2018/5940748
86. Grondard C, Biondi O, Pariset C, Lopes P, Deforges S, Lecolle S, et al. Exercise-induced modulation of calcineurin activity parallels the time course of myofibre transitions. J Cell Physiol. (2008) 214:126–35. doi: 10.1002/jcp.21168
87. Rosen DR, Siddique T, Patterson D, Figlewicz DA, Sapp P, Hentati A, et al. Mutations in Cu/Zn superoxide dismutase gene are associated with familial amyotrophic lateral sclerosis. Nature. (1993) 362:59–62. doi: 10.1038/364362c0
88. Spiller KJ, Cheung CJ, Restrepo CR, Kwong LK, Stieber AM, Trojanowski JQ, et al. Selective motor neuron resistance and recovery in a new inducible mouse model of TDP-43 proteinopathy. J Neurosci. (2016) 36:7707–17. doi: 10.1523/JNEUROSCI.1457-16.2016
89. Spiller KJ, Restrepo CR, Khan T, Stieber AM, Kwong LK, Trojanowski JQ, et al. Progression of motor neuron disease is accelerated and the ability to recover is compromised with advanced age in rNLS8 mice. Acta Neuropathol Commun. (2016) 4:105. doi: 10.1186/s40478-016-0377-5
90. Walker AK, Spiller KJ, Ge G, Zheng A, Xu Y, Zhou M, et al. Functional recovery in new mouse models of ALS/FTLD after clearance of pathological cytoplasmic TDP-43. Acta Neuropathol. (2015) 130:643–60. doi: 10.1007/s00401-015-1460-x
91. Pinto AC, Alves M, Nogueira A, Evangelista T, Carvalho J, Coelho A, et al. Can amyotrophic lateral sclerosis patients with respiratory insufficiency exercise? J Neurol Sci. (1999) 169:69–75. doi: 10.1016/S0022-510X(99)00218-X
92. Sanjak M, Bravver E, Bockenek WL, Norton HJ, Brooks BR. Supported treadmill ambulation for amyotrophic lateral sclerosis: a pilot study. Arch Phys Med Rehabil. (2010) 91:1920–9. doi: 10.1016/j.apmr.2010.08.009
93. Braga ACM, Pinto A, Pinto S, de Carvalho M. The role of moderate aerobic exercise as determined by cardiopulmonary exercise testing in ALS. Neurol Res Int. (2018) 2018:8218697. doi: 10.1155/2018/8218697
94. Braga AC, Pinto A, Pinto S, de Carvalho M. Tele-monitoring of a home-based exercise program in amyotrophic lateral sclerosis: a feasibility study. Eur J Phys Rehabil Med. (2018) 54:501–3. doi: 10.23736/S1973-9087.18.05129-8
95. Clawson LL, Cudkowicz M, Krivickas L, Brooks BR, Sanjak M, Allred P, et al. A randomized controlled trial of resistance and endurance exercise in amyotrophic lateral sclerosis. Amyotroph Lateral Scler Frontotemporal Degener. (2018) 19:250–8. doi: 10.1080/21678421.2017.1404108
96. Cheah BC, Boland RA, Brodaty NE, Zoing MC, Jeffery SE, McKenzie DK, et al. INSPIRATIonAL–INSPIRAtory muscle training in amyotrophic lateral sclerosis. Amyotroph Lateral Scler. (2009) 10:384–92. doi: 10.3109/17482960903082218
97. Pinto S, Swash M, de Carvalho M. Respiratory exercise in amyotrophic lateral sclerosis. Amyotroph Lateral Scler. (2012) 13:33–43. doi: 10.3109/17482968.2011.626052
98. Ayan C, Cancela J. Feasibility of 2 different water-based exercise training programs in patients with Parkinson's disease: a pilot study. Arch Phys Med Rehabil. (2012) 93:1709–14. doi: 10.1016/j.apmr.2012.03.029
99. Perez-de la Cruz S. A bicentric controlled study on the effects of aquatic Ai Chi in Parkinson disease. Complement Ther Med. (2018) 36:147–53. doi: 10.1016/j.ctim.2017.12.001
100. Kargarfard M, Shariat A, Ingle L, Cleland JA, Kargarfard M. Randomized controlled trial to examine the impact of aquatic exercise training on functional capacity, balance, and perceptions of fatigue in female patients with multiple sclerosis. Arch Phys Med Rehabil. (2018) 99:234–41. doi: 10.1016/j.apmr.2017.06.015
101. American college of sports medicine position stand. The recommended quantity and quality of exercise for developing and maintaining cardiorespiratory and muscular fitness, and flexibility in healthy adults. Med Sci Sports Exerc. (1998) 30:975–91. doi: 10.1249/00005768-199806000-00032
102. Bohannon RW. Results of resistance exercise on a patient with amyotrophic lateral sclerosis. A case report. Phys Ther. (1983) 63:965–8. doi: 10.1093/ptj/63.6.965
103. Drory VE, Goltsman E, Reznik JG, Mosek A, Korczyn AD. The value of muscle exercise in patients with amyotrophic lateral sclerosis. J Neurol Sci. (2001) 191:133–7. doi: 10.1016/S0022-510X(01)00610-4
104. Kitano K, Asakawa T, Kamide N, Yorimoto K, Yoneda M, Kikuchi Y, et al. Effectiveness of home-based exercises without supervision by physical therapists for patients with early-stage amyotrophic lateral sclerosis: a pilot study. Arch Phys Med Rehabil. (2018) 99:2114–7. doi: 10.1016/j.apmr.2018.02.015
105. Merico A, Cavinato M, Gregorio C, Lacatena A, Gioia E, Piccione F, et al. Effects of combined endurance and resistance training in Amyotrophic Lateral Sclerosis: a pilot, randomized, controlled study. Eur J Transl Myol. (2018) 28:7278. doi: 10.4081/ejtm.2018.7278
106. Kato N, Hashida G, Konaka K. Effect of muscle strengthening exercise and time since onset in patients with amyotrophic lateral sclerosis: a 2-patient case series study. Medicine (Baltimore). (2018) 97:e11145. doi: 10.1097/MD.0000000000011145
107. Hakkinen K. Neuromuscular and hormonal adaptations during strength and power training. A review. J Sports Med Phys Fitness. (1989) 29:9–26.
108. Moritani T, deVries HA. Neural factors versus hypertrophy in the time course of muscle strength gain. Am J Phys Med. (1979) 58:115–30.
109. Beghi E, Logroscino G, Chio A, Hardiman O, Millul A, Mitchell D, et al. Amyotrophic lateral sclerosis, physical exercise, trauma and sports: results of a population-based pilot case-control study. Amyotroph Lateral Scler. (2010) 11:289–92. doi: 10.3109/17482960903384283
110. Pupillo E, Messina P, Giussani G, Logroscino G, Zoccolella S, Chio A, et al. Physical activity and amyotrophic lateral sclerosis: a European population-based case-control study. Ann Neurol. (2014) 75:708–16. doi: 10.1002/ana.24150
111. Lindsay A, Larson AA, Verma M, Ervasti JM, Lowe DA. Isometric resistance training increases strength and alters histopathology of dystrophin-deficient mouse skeletal muscle. J Appl Physiol. (2018) 26:363–75. doi: 10.1152/japplphysiol.00948.2018
112. Poyhonen T, Sipila S, Keskinen KL, Hautala A, Savolainen J, Malkia E. Effects of aquatic resistance training on neuromuscular performance in healthy women. Med Sci Sports Exerc. (2002) 34:2103–9. doi: 10.1097/00005768-200212000-00036
Keywords: amyotrophic lateral sclerosis/ALS, motor neuron disease, exercise, endurance training, resistance training, SOD1G93A mice, ALS patients
Citation: Tsitkanou S, Della Gatta P, Foletta V and Russell A (2019) The Role of Exercise as a Non-pharmacological Therapeutic Approach for Amyotrophic Lateral Sclerosis: Beneficial or Detrimental? Front. Neurol. 10:783. doi: 10.3389/fneur.2019.00783
Received: 23 May 2019; Accepted: 04 July 2019;
Published: 17 July 2019.
Edited by:
Lucio Tremolizzo, University of Milano-Bicocca, ItalyReviewed by:
Christian Lunetta, Centro Clinico Nemo, ItalyCopyright © 2019 Tsitkanou, Della Gatta, Foletta and Russell. This is an open-access article distributed under the terms of the Creative Commons Attribution License (CC BY). The use, distribution or reproduction in other forums is permitted, provided the original author(s) and the copyright owner(s) are credited and that the original publication in this journal is cited, in accordance with accepted academic practice. No use, distribution or reproduction is permitted which does not comply with these terms.
*Correspondence: Stavroula Tsitkanou, c3RzaXRrYW5AZGVha2luLmVkdS5hdQ==
Disclaimer: All claims expressed in this article are solely those of the authors and do not necessarily represent those of their affiliated organizations, or those of the publisher, the editors and the reviewers. Any product that may be evaluated in this article or claim that may be made by its manufacturer is not guaranteed or endorsed by the publisher.
Research integrity at Frontiers
Learn more about the work of our research integrity team to safeguard the quality of each article we publish.