- 1Division of Physical Medicine and Rehabilitation, ICS Maugeri SPA SB, Pavia, Italy
- 2Department of Exercise and Sport Science, International University of Health, Exercise and Sports, LUNEX University, Differdange, Luxembourg
In this review, we briefly recall the fundamental processes allowing us to change locomotion trajectory and keep walking along a curved path and provide a review of contemporary literature on turning in older adults and people with Parkinson's Disease (PD). The first part briefly summarizes the way the body exploits the physical laws to produce a curved walking trajectory. Then, the changes in muscle and brain activation underpinning this task, and the promoting role of proprioception, are briefly considered. Another section is devoted to the gait changes occurring in curved walking and steering with aging. Further, freezing during turning and rehabilitation of curved walking in patients with PD is mentioned in the last part. Obviously, as the research on body steering while walking or turning has boomed in the last 10 years, the relevant critical issues have been tackled and ways to improve this locomotor task proposed. Rationale and evidences for successful training procedures are available, to potentially reduce the risk of falling in both older adults and patients with PD. A better understanding of the pathophysiology of steering, of the subtle but vital interaction between posture, balance, and progression along non-linear trajectories, and of the residual motor learning capacities in these cohorts may provide solid bases for new rehabilitative approaches.
Introduction
Every day we frequently follow circular courses and turns when we move (1). Our inherently unstable bipedal gait (2) requires adaptive control during curved walking (3). Stride length and duration of the stance phase differ between the inner and outer leg (4–6), and the center of mass moves toward the interior of the trajectory (7). This is made possible by appropriate placement of the feet to create a gravity-dependent torque that counteracts the centrifugal force. Central commands would take over the control of locomotion at the expense of the spinal automatisms, very much as it happens for crayfish (8) and giant stick insects, where rotation of the body is accomplished by modulation of ground reaction forces among legs (9). Obviously, the brain generates ad-hoc activity that follows the basic laws of physics when moving along circular trajectories, regardless of the number of legs (10).
A thoughtful study of walking and turning in humans had been done more than 140 years ago by Eadweard Muybridge [see (11)]. Foot yaw orientation, body tilt in the frontal plane and pelvis rotation over the stance leg could already be seen in his remarkable photographic freeze frames. Then, non-linear walking trajectories had been almost ignored by scholars and scientist, until Takei et al. published a note (12) about the walking trajectory of young and older subjects on a circular path in darkness.
The interaction between the spinal circuits with the descending command and the adaptation of the body medio-lateral inclination and step length asymmetry to the progression velocity would represent a challenge for the control system. This task requires anticipatory adjustments (13–19) in advance of taking the curve (5, 20–22). It is not implausible that basal ganglia are subjected to an extra load during curved walking, since they operate through two parallel distinct pathways having opposing effects, allowing the execution of movement while gating the antigravity activity of the postural muscles (14, 23). This short review aims to summarize the characteristics of curved walking in young and older adults, and to address gait differences between people with Parkinson's Disease (PD) and healthy subjects. Proposals for an integrated rehabilitation approach in PD are put forward.
Steering the Body Along Curved Paths
Successful locomotion along curved trajectories requires fine coordination of body segments' movements. Any minor change in the adjustment of the asymmetric step length of the two legs produces dramatic effects in the kinematics, very much as it occurs with minimal changes in the localization of ground reaction forces underneath the feet during the stance phases (6). Therefore, accurate brain control is required for correct rotation of the lower limbs and inversion or eversion of the ankle for successful placement of the foot on the ground (4, 24–31).
During linear walking, the feet create mediolateral impulses at heel strike that symmetrically move the body toward the contralateral limb. In turning, the body is moved toward the interior of the trajectory by both the internal and the external foot (32). In order to exploit gravity, the center of foot pressure during heel strike and toe off is being slightly displaced with respect to its position under linear walking. This creates a mediolateral torque that produces and controls trunk roll tilt and progression along the circular trajectory (6, 33–35), and generates the proper centripetal force to avoid going off on the tangent (4, 5, 27). Appropriate braking of the body fall toward the interior of the trajectory is exerted by the feet at foot-off (6, 36), such as to counterbalance the reaction forces produced at heel strike (37).
In addition to studying walking along circular trajectories, investigators have focused also on the strategies used to navigate sharp turns (26, 30, 38–40). Others have exploited figures of eight trajectories that include both clockwise and counter-clockwise segments (3, 41–43). Consistently, turns imply reduction of progression velocity, placement of the foot in the direction of the new trajectory (25) and lateral translation of the body in addition to body reorientation to align with the new travel direction (15). Foot placement and trunk inclination move the center of mass toward the new direction in the transition stride. The top-down temporal sequence in body segments reorientation slightly changes as turn proceeds (26, 44). This pattern is robust to turning velocity, therefore inherent in the command to turn (45). Gaze redirection accompanies steering, so that visual or oculomotor deficits should be considered when assessing turning behavior (24, 46).
What Do we Know of the Neural Command for Steering?
Motor tasks employ muscle synergies, i.e., one or more sets of muscles synchronously activated and specific to the task (47). Synergy studies concluded that rectilinear and curvilinear walking share the same motor command; however, fine-tuning in muscle synergies is necessary for circular trajectories, where the kinematic strategy conforms to the physical laws that underpin curved walking while keeping balance (14, 27, 48–50). Courtine and Schieppati (28), using the principal component analysis, found that both straight-ahead and curved walking were low dimensional, and two components accounted for more than 70% of the movement variability. Fine modulation of the muscle synergies underlying the straight-ahead locomotion is sufficient for generating the adequate propulsive forces to steer walking and maintain balance (48).
Bejarano et al. (49) found four muscle synergies for both walking conditions. Muscle activation profiles lasted longer and were larger during curvilinear than straight walking, and more so for the muscles of the limb inside than outside the trajectory. However, several deep muscles responsible for intra- and extra-rotation of pelvis on thigh had not been recorded. The asymmetric activation of these muscles and the amplitude and time-course of the modulation of their activity might configure an additional synergy peculiar to turning. The contribution of the gluteus medius to the trunk orientation during turning should be also considered (51).
The origin of the adaptation of the motor command to the curved path is a matter of speculation. Jahn et al. (52) have described brain activation for imagined straight walking and for imagined walking along a curved path (53). They observed asymmetric basal ganglia activation at turn initiation, enhanced activity in cortical areas associated with navigation, and decreased activity in areas supposed to process vestibular input. These findings point to the complexity of the organization of the command for producing the curved walking trajectory, while the deactivation of certain brain regions may explain why the vestibular input seems to be down regulated during continuous turning while stepping in place (54), similarly to what occurs at the transition between stance and gait (55).
Sensory Feedback During Walking and Turning
Asymmetric proprioceptive input elicited by vibration of axial (neck and trunk) muscles produces steering and turning (29, 56–59), whereas proprioceptive input from the leg contributes to fine adjustment of the spinal pattern generators for walking (60, 61). Input from axial muscles would play the role of a servo-mechanism, whereby minor asymmetries initiated by asymmetric foot placement (1, 62) would affect the spinal generators to produce the necessary fine changes in leg and foot kinematics accompanying heading changes.
Whether or not continuous walking along a circular trajectory is also favored by a shift in our straight-ahead goes beyond the scope of this short review, but we would note that a shift in subjective straight-ahead occurs after a period of stepping in place on a rotating treadmill (63). In turn, it is not unlikely that a shift in the straight-ahead is produced by the feedback from the muscles producing the rotation of the pelvis and trunk over the standing leg when walking along a curved trajectory or when stepping in place and turning (54, 59). Vision is obviously not necessary for implementing a curved trajectory (5), but the continuous visual field motion would nonetheless favor the fine tuning of the gait synergies underpinning the production of the circular trajectory (24, 64).
These findings suggest that asymmetric proprioceptive input, either produced by the asymmetric kinematics initially produced by the central command to turn or by the artificial activation through muscle vibration, would favor and sustain the steering synergy.
Aging Affects Steering
Locomotor impairments are an inevitable consequence of aging, and worsen with the associated cognitive decline (65, 66). However, we did not expand here on the issue of the effects of cognitive decline on locomotion. This was a deliberate choice, because this would require an ad-hoc article, given the growing number of papers on this complex topic [see the reviews by (67–69)]. Furthermore, the effect of cognitive decline on turning has not received the attention it would deserve, yet.
Walking speed is definitely lower than in the young (70–72), and older adults adopt a more cautious attitude when steering (73–75). Normally, during the swing period, young subjects reverse the fall of the center of mass before foot-contact by active braking via activation of the triceps of the stance leg (76, 77). The control of this braking phase is impaired in older adults, and the braking phase is compensated for by reducing the step length (78). Neurological (PD, cerebellar syndromes, neuropathies, hemiparesis, dementia) and non-neurological conditions (cardiovascular and respiratory) contribute to gait problems (79, 80).
In young adults, curved walking significantly decreases walking speed (by about 15%) and stride-length (more so in the leg inner to curvature), whilst cadence is barely diminished (5, 27). In older adults whose linear gait speed is within the limits of normality (81), curved gait speed diminishes by about 20%, with minor differences across the studied cohorts (42, 82–86) (Figure 1A). In older adults with poor mobility and linear walking speed below the 0.90 m/s, the reduction in gait speed between linear and curved walking is of about 15% (41) and 5% (87). Likely, frailty and balance-related anxiety (93) reduces speed during linear walking to such low values that the time necessary for the added coordination of posture and progression during curved walking becomes proportionally negligible.
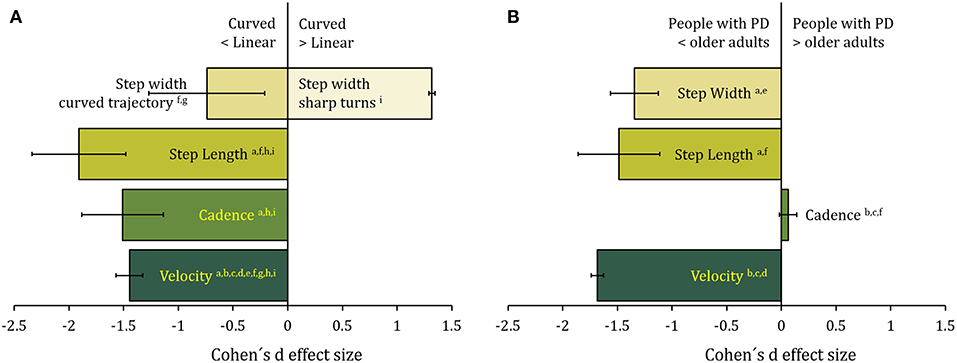
Figure 1. (A) The effect of the trajectory (curved with respect to linear) on spatiotemporal gait variables in older adults. Data are the sample-size-weighted mean of Cohen's d effect-size (ES) of the illustrated variables [calculated from a(82); b(41); c(83); d(42); e(87); f(86); g(88); h(85); i(84)]. Negative values in the x-axis represent a decrease in the variables in curved compared to linear walking. Error bars represent 95% confidence intervals. There is an overall decrease in step length, cadence, and step width during protracted curved path. On the contrary, during sharp turns, step width is increased in the older adults. (B) The effect of Parkinson's disease on walking along curved trajectories. Data are the sample-size-weighted mean of Cohen's d effect-size of the illustrated spatiotemporal gait variables [calculated from a(89); b(82, 90) d(91); e(92); f(85)]. Negative values in the x axis represent a decrease in the variable values in patients with PD compared to age-matched controls. Error bars represent 95% confidence intervals. Step length and width decrease, while cadence is unaffected. Velocity is diminished mainly because of reduction in step length.
Cadence diminishes by <10% (84–86) for older adults during curved when compared to linear walking. Step length is also reduced by <20% (82, 84). While velocity, cadence, and step length changes are common to different types of turning, step width behaves differently between curved walking and sharp turning. Older adults increase their step width during the transition phase from linear to curved path (88). On the contrary, for protracted curved path, step width is narrow (89, 94) and it decreases until 30% (84). Slow anticipatory adjustments may play a role, as shown by abnormal turning pattern in older adults with balance deficits when the command to turn is unexpected (95).
The variability of step length, cadence, and step width increases during curved walking (84, 89, 91, 96). However, in older people who have not fallen, a moderate amount of step variability is required to adapt to situations that challenge postural control (97, 98), while too little variability is associated with fall history in older adults (99). This could explain why increased spatial variability during curved path identified subjects with better motor skills of walking (86).
Additionally, older adults who frequently fall display a reduced turning angle variability compared with non-fallers (100), denoting a lack of dynamic balance skills necessary to seamlessly modulate turning angles while maintaining balance (101). It seems that the ability to vary step length and step width enables both smooth continuity of the center of pressure path and energy-efficient navigation of curves (86).
The multisegmental control has been studied during planned turning on the spot (102–104). Older adults tend to reorient their head, shoulder and pelvis simultaneously, followed by foot displacement, regardless of visual condition. The command to implement curved walking implies modification of all the fundamental spatio-temporal variables of gait (105), more so in older adults who frequently fall compared with non-fallers (100). Interestingly, multiple fallers show a simplified turning pattern to assist balance control (106). Moreover, changes in timing and sequencing of segment reorientation produce earlier anticipation of turns (102, 107). Activity in prefrontal cortex is increased in older compared to young adults (108) suggesting a higher cognitive cost for gait control even during linear walking. In complex walking, known to depend on appropriate executive function and balance control (109), obstacle negotiation further increases prefrontal activity (110).
These studies point to definite changes in the spatio-temporal gait variables between linear and curved walking. The increased variability in the curved gait kinematics can be the result of a coordination disorder but represents kind of a safety factor for reducing the risk of falling. Adaptive strategies for turning might be driven by the effort to diminish the cognitive cost associated with turning.
Parkinson's Disease Problems During Steering
In patients with neurological disorders, impaired locomotion is a frequent and serious symptom (111). Gait and balance impairments are typical of patients with PD (112, 113). Impaired locomotion is often associated with reduced ability to brake the fall of the body in late stance compared to matched controls (114). This may be a sign of poor coordination between trunk and lower limb, and forces patients to take short steps. A study of muscle synergies has identified a reduced number of synergies during straight walking (115), providing evidence that control of gait is less complex or flexible in patients with PD. Whether this can affect the coordination between trunk and lower limb muscle, and whether synergies are further affected by turning, remains to be established (115).
In neurological patients, additional problems emerge during curved compared to linear trajectories (116–118). Patients with PD are no exception, and all of them are indeed critically challenged by curved walking (119–123), in spite of their diverse phenotype and motor symptomatology. There is a strong indication, based on functional MRI, that patients with PD have reduced activity in the globus pallidus and enhanced activity in the supplementary motor area during imagined walking and turning (124). Functional near infrared spectroscopy shows higher prefrontal cortex activity in the patients compared to healthy subjects (125). Interestingly, prefrontal activation during obstacle negotiation was increased more than during dual task walking (126).
The changes observed in curved compared to linear walking are usually non-negligible. Kinematic analysis demonstrated en-bloc rotation of axial segments in patients with PD (127–131). Coordinated axial muscle activation seems to be particularly affected (132). This has been repeatedly shown and assessed quantitatively (133–138). The en-bloc rotation of axial segments in patients with PD contrasts with the lower extremity muscle activation pattern that appears to be overall normal (131). Huxham et al. (139) have shown that stride length reduction appears to contribute more than downscaled rotation amplitude to inefficient turning in patients with PD, possibly because of reduced axial mobility. Compared to straight walking, during curved walking speed diminishes by about 20% (90) and by about 35% (82, 91) in less and more affected patients, respectively. Cadence also diminishes by more than 5% and step length diminishes by about 20% (82, 89) (Figure 1B). In contrast to age-matched controls, patients with PD turn with narrower steps (89, 92, 122).
Greater variability of the temporal gait parameters is detectable during curved but not linear walking, over and above the increased variability exhibited by healthy subjects (6). Problems in turning and curved walking are detectable even at an early stage of the disease, and they persist while on-phase (92, 140). Most interestingly, spatio-temporal gait pattern and variability during curved walking are abnormal even in well-treated, well-functioning patients with PD, exhibiting no change in speed in straight-line walking compared to age-matched healthy subjects (85). Anxiety should be considered when evaluating walking performances, since well-treated patients with fear of falling spend more time turning, both in the laboratory and at home (141). This would be connected to the effect of temporal pressure on the control of medio-lateral stability, a critical issue when changing direction (142, 143).
All in all, it seems safe to posit that patients with PD suffer from specific disorders of curved walking and turning, which may not be obvious during straight walking. Again, it may not be immediately clear whether some abnormalities are direct consequences of the neuronal damage or, at least in part, the outcome of a long-duration adaptive process.
Freezing in People with Parkinson's Disease
Freezing can occur during all types of gait and is related to the increased stride-to-stride variability (144). However, it is most common during turning (22, 145–153) and is a major cause of falling in these patients (154). Turning during daily activities is more compromised in patients with than without freezing (155).
The increase in freezing events during turning may be due in part to the asymmetric nature of the task and the necessary anticipatory adjustment for ensuring postural stability along the medio-lateral direction. The temporal and spatial asymmetry of steps during turning represents a more complex control problem than forward walking (30, 121, 149, 156, 157), as suggested by increased activation in prefrontal areas accompanying freezing before anticipated turns (125). Perhaps, these changes accompany and compensate for the structural and functional alterations in the brain stem centers for locomotion (158).
Neck and axial rigidity during turning may reduce forward progression (159, 160). Patients take shorter turns with smaller turn angles and more steps and exhibit larger variability with respect to controls (161). Freezers, irrespective of freezing episodes, adopt a narrower step width compared to controls and non-freezers during turning (89, 91).
Problems in fast axial turning appear when stepping is performed on a narrow base (160, 162, 163). Freezing episodes are more frequent at sharp turns (91) and turning in place (164), indicating that problems exist both in adjusting the anticipation to the intended trajectory and in controlling body segment coordination and balance during rotation on the spot, regardless of the speed of turning and the severity of the disease (22, 94). Again, this speaks in favor of a delayed preparation for the change in walking direction.
Anticipatory adjustments are indeed abnormal in patients with PD (165–167) including eye and head anticipatory movements for exploration (53) and movements to correct a lateral disequilibrium (168, 169). Plate et al. (170) have confirmed that anticipatory adjustments are slower and of smaller amplitude, in keeping with the overall bradykinesia of the patients. Interestingly, anticipatory postural adjustments are not followed by coordinated steps (171). Anticipatory adjustments associated with gait initiation may not be strictly abnormal per se in patients with respect to age-matched subjects (169, 172), but may be impaired in people with freezing of gait compared to those without freezing (173). All in all, these findings are in keeping with the hypothesis that freezing would depend on the sheer control of the trunk rotation over the standing leg (54, 85).
Freezing is elusive. However, any voluntary human movement requires a complex array of in-series and in-parallel processes, from anticipatory postural adjustment to ongoing feedback-related correction. No wonder some peculiar interaction of aberrant events in patients with PD, from cognitive to reflex nature, can finally produce what we call freezing, in our case of gait, but not necessarily limited to gait [see e.g., (174)].
Rehabilitation of Curved Walking
The ability to turn, above all in restricted spaces, is very important in autonomy maintenance in everyday life. Furthermore, falls during turning result in more hip fractures than falls during linear gait (175). Therefore, rehabilitation of curved walking and turning may be meaningful since turning impairments are not improved by dopaminergic medication (92). Exercise has potential to improve many clinical issues in patients with PD such as strength, balance, walking, and quality of life (176–179). A recent review, not centered on curved walking (180), confirmed the benefits of physiotherapy in most outcomes over the short term. However, most of the observed differences between treatments were considered of minimal clinical importance.
Rehabilitation should address the critical steps in producing successful steering, the anticipatory adjustments in preparation to turn and direct steps along the curved trajectory, the rhythmic and regular production of steps, axial mobility and head-trunk coordination, and the coordinated activity of the pelvic muscles producing intra- and extra-rotation of the lower limbs. Anticipatory postural adjustments can be possibly enhanced by focused appropriate rehabilitation in older adults (181). This is significant in consideration of the relevance of the delayed release of anticipatory adjustments in these patients (92, 167).
The use of linear treadmill for the rehabilitation of gait in patients with PD has produced modest but significant effects on gait speed and stride length (182–185), indicating that these patients can be trained on treadmill and show some improvement. An earlier study with the use of the rotating treadmill in two patients showed that a short period of walking on the rotating treadmill reduces freezing episodes (186, 187). However, training curved walking by a rotating treadmill produced no improvements in gait or turning after 5 days of training (90). A later study, administering a progressive training with augmenting rotation velocity and trial duration through 10 daily sessions, showed instead a significant benefit on walking velocity along a circular path (188). In the latter study, before and at the end of the treatment, all patients walked over ground along linear and circular trajectories, and the velocity of walking bouts increased post-treatment, more so for the circular than the linear trajectory. Therefore, in spite of their known problems in attentional resources and cognitive strategies (189), patients can learn to produce turning while stepping on the rotating treadmill and this capacity translates into improved over ground curved walking. These findings strengthen the conclusions of independent reports by Cheng et al. (190, 191), employing a complex walking program (S-shaped, figure-of-8, square- and oval-shaped paths) or a rotating platform.
Notably, the synergies responsible for maintaining a fixed body orientation in space while stepping on the rotating treadmill (45) are the same that are put in place when stepping in place while voluntarily turning (54). Prolonged stepping in place and turning produces in normal subjects an after-effect consisting in a long lasting spontaneous turning on the spot (eyes closed), likely created by adaptation to the continuously activated somatosensory channel (54, 59, 192). Time decay of angular velocity, stepping cadence, and head acceleration were remarkably similar after both conditioning procedures [voluntarily stepping-and-turning and the so-called podokinetic stimulation by the rotating treadmill (193)]. Therefore, stepping in place while voluntarily turning could be administered in lieu of the rotating platform for training the turning task in patients with PD. This treatment could be as successful as the rotating platform for the purpose of exercising the coordinated movements underpinning turning and of training curved walking. In this connection, it is worthwhile to mention that Aman et al. (194) have shown the effectiveness of proprioceptive training in improving sensorimotor function, and Elangovan et al. (195) proved that proprioceptive training is indeed effective in patients with PD.
The responses of patients with PD to vibration of postural muscles are largely normal, even if they show abnormal transient postural responses to vibration-off, a sign of impaired sensory reweighting in balance control (196). This is reminiscent of their inappropriate response to light-off when balancing on a movable platform (197). Similarly, alternate trains of postural muscle vibration promote cyclic body displacement in standing patients much as in age-matched subjects (198), showing that these patients can integrate and exploit the vibratory proprioceptive input to produce postural oscillations comparable to those occurring during walking. Moreover, vibratory stimulation of trunk muscles significantly increases stride length, cadence and velocity in both patients and healthy subjects (199). As far as curved walking is concerned, it is notable that, in healthy subjects, vibration of trunk muscles interferes with the above mentioned podokinetic aftereffect by enhancing—or reducing—body rotation velocity depending on the vibrated side (59). The summation of vibration and podokinetic effect speaks for the capacity of the proprioceptive input from the trunk and from the pelvis muscles to affect steering by modulating the activity of the responsible brain centers through a common mechanism. These interventions (unilateral axial muscle vibration, stepping on the rotating treadmill, voluntarily stepping-and-turning) might be considered when planning a training protocol aimed at rehabilitating gait with emphasis on curved walking.
Overall, it seems that interventions based on the new evidence about planning, organization, and execution of curved walking and associated postural control represent a promising rehabilitation approach. We are now ready for undertaking large-scale studies that address the effects of sensory feedback and of stepping-and-turning task repetition on steering and turning capacities in older subjects and patients with PD, also considering patients with typical PD and atypical parkinsonism [see (200)].
Conclusions
The findings summarized here suggest that the turn-related command operates by fine modulation of the phase relationships between the tightly coupled neuronal assemblies that drive motor neuron activity during walking. Aging and Parkinson's disease seem to affect steering by slowing the expression of this modulation and simplifying the neural command by coupling subroutines. Older adults and more so patients with PD are compelled to modify the gait pattern, reduce some spatiotemporal variables when facing curvilinear trajectories, and adopt en-bloc rotation of axial segments. This strategy allows the CNS to manage a less complex task, even if it does not fully protect these subjects from the risk of falling, likely because of persisting poor posturo-kinetic coordination. The cost of the new control mode, implying a newly designed integration of vestibular, proprioceptive, and visual information for equilibrium control during curved walking, turns out to be substantial in patients with PD, and would lead to freezing and frequent falls. More research is needed on rehabilitative interventions to train voluntary or treadmill-induced body rotation and on their potential of improving walking performance during curvilinear paths.
Author Contributions
All authors listed have made a substantial, direct and intellectual contribution to the work, and approved it for publication.
Funding
This work was partially supported by the Ricerca Corrente, funding scheme of the Ministry of Health, Italy.
Conflict of Interest Statement
The authors declare that the research was conducted in the absence of any commercial or financial relationships that could be construed as a potential conflict of interest.
References
1. Glaister BC, Bernatz GC, Klute GK, Orendurff MS. Video task analysis of turning during activities of daily living. Gait Posture. (2007) 25:289–94. doi: 10.1016/j.gaitpost.2006.04.003
2. Ivanenko YP, Dominici N, Lacquaniti F. Development of independent walking in toddlers. Exerc Sport Sci Rev. (2007) 35:67–73. doi: 10.1249/JES.0b013e31803eafa8
3. Welch SA, Ward RE, Kurlinski LA, Kiely DK, Goldstein R, VanSwearingen J, et al. Straight and curved path walking among older adults in primary care: associations with fall-related outcomes. P Mand R. (2016) 8:754–60. doi: 10.1016/j.pmrj.2015.12.004
4. Imai T, Moore ST, Raphan T, Cohen B. Interaction of the body, head, and eyes during walking and turning. Exp. Brain Res. (2001) 136:1–18. doi: 10.1007/s002210000533
5. Courtine G, Schieppati M. Human walking along a curved path. I. Body trajectory, segment orientation and the effect of vision. Eur J Neurosci. (2003) 18:177–90. doi: 10.1046/j.1460-9568.2003.02736.x
6. Turcato AM, Godi M, Giordano A, Schieppati M, Nardone A. The generation of centripetal force when walking in a circle: insight from the distribution of ground reaction forces recorded by plantar insoles. J Neuroeng Rehabil. (2015) 12:4. doi: 10.1186/1743-0003-12-4
7. Schmid M, De Nunzio AM, Beretta MV, Schieppati M. Walking along a curved trajectory. Insight into the generation of the centripetal force. Gait Posture. (2004) 20:S116.
8. Domenici P, Jamon M, Clarac F. Curve walking in freely moving crayfish (Procambarus clarkii). J Exp Biol. (1998) 201:1315–29.
9. Dürr V, Ebeling W. The behavioural transition from straight to curve walking: kinetics of leg movement parameters and the initiation of turning. J Exp Biol. (2005) 208:2237–52. doi: 10.1242/jeb.01637
10. Clayton HM, Sha DH. Head and body centre of mass movement in horses trotting on a circular path. Equine Vet J Suppl. (2006) 38:462–7. doi: 10.1111/j.2042-3306.2006.tb05588.x
12. Takei Y, Grasso R, Berthoz A. Quantitative analysis of human walking trajectory on a circular path in darkness. Brain Res. Bull. (1996) 40:491–5.
13. Grasso R, Glasauer S, Takei Y, Berthoz A. The predictive brain: anticipatory control of head direction for the steering of locomotion. Neuroreport. (1996) 7:1170–4.
14. Grasso R, Prévost P, Ivanenko YP, Berthoz A. Eye-head coordination for the steering of locomotion in humans: an anticipatory synergy. Neurosci Lett. (1998) 253:115–8.
15. Hollands M, Sorensen K, Patla A. Effects of head immobilization on the coordination and control of head and body reorientation and translation during steering. Exp Brain Res. (2001) 140:223–33. doi: 10.1007/s002210100811
16. Prévost P, Ivanenko Y, Grasso R, Berthoz A. Spatial invariance in anticipatory orienting behaviour during human navigation. Neurosci Lett. (2003) 339:243–7. doi: 10.1016/S0304-3940(02)01390-3
17. Massion J, Alexandrov A, Frolov A. Why and how are posture and movement coordinated? Prog Brain Res. (2004) 143:13–27. doi: 10.1016/S0079-6123(03)43002-1
18. Hicheur H, Vieilledent S, Berthoz A. Head motion in humans alternating between straight and curved walking path: combination of stabilizing and anticipatory orienting mechanisms. Neurosci Lett. (2005) 383:87–92. doi: 10.1016/j.neulet.2005.03.046
19. Yiou E, Hamaoui A, Allali G. The contribution of postural adjustments to body balance and motor performance. Front Hum Neurosci. (2018) 12:487. doi: 10.3389/fnhum.2018.00487
20. Xu D, Carlton LG, Rosengren KS. Anticipatory postural adjustments for altering direction during walking. J Mot Behav. (2004) 36:316–26. doi: 10.3200/JMBR.36.3.316-326
21. Galna B, Lord S, Daud D, Archibald N, Burn D, Rochester L. Visual sampling during walking in people with Parkinson's disease and the influence of environment and dual-task. Brain Res. (2012) 1473:35–43. doi: 10.1016/j.brainres.2012.07.017
22. Spildooren J, Vercruysse S, Heremans E, Galna B, Vandenbossche J, Desloovere K, et al. Head-pelvis coupling is increased during turning in patients with Parkinson's disease and freezing of gait. Mov Disord. (2013) 28:619–25. doi: 10.1002/mds.25285
23. MacKinnon CD. Sensorimotor anatomy of gait, balance, and falls. Handb Clin Neurol. (2018) 159:3–26. doi: 10.1016/B978-0-444-63916-5.00001-X
24. Reed-Jones RJ, Hollands MA, Reed-Jones JG, Vallis LA. Visually evoked whole-body turning responses during stepping in place in a virtual environment. Gait Posture. (2009) 30:317–21. doi: 10.1016/j.gaitpost.2009.06.001
25. Hase K, Stein RB. Turning strategies during human walking. J Neurophysiol. (1999) 81:2914–22. doi: 10.1152/jn.1999.81.6.2914
26. Patla AE, Adkin A, Ballard T. Online steering: coordination and control of body center of mass, head and body reorientation. Exp Brain Res. (1999) 129:629–34.
27. Courtine G, Schieppati M. Human walking along a curved path. II. Gait features and EMG patterns. Eur J Neurosci. (2003) 18:191–205. doi: 10.1046/j.1460-9568.2003.02737.x
28. Courtine G, Schieppati M. Tuning of a basic coordination pattern constructs straight-ahead and curved walking in humans. J Neurophysiol. (2004) 91:1524–35. doi: 10.1152/jn.00817.2003
29. Schmid M, De Nunzio AM, Schieppati M. Trunk muscle proprioceptive input assists steering of locomotion. Neurosci Lett. (2005) 384:127–32. doi: 10.1016/j.neulet.2005.04.059
30. Huxham F, Gong J, Baker R, Morris M, Iansek R. Defining spatial parameters for non-linear walking. Gait Posture. (2006) 23:159–63. doi: 10.1016/j.gaitpost.2005.01.001
31. Bancroft MJ, Day BL. The throw-and-catch model of human gait: evidence from coupling of pre-step postural activity and step location. Front Hum Neurosci. (2016) 10:635. doi: 10.3389/fnhum.2016.00635
32. Orendurff MS, Segal AD, Berge JS, Flick KC, Spanier D, Klute GK. The kinematics and kinetics of turning: limb asymmetries associated with walking a circular path. Gait Posture. (2006) 23:106–11. doi: 10.1016/j.gaitpost.2004.12.008
33. Godi M, Turcato AM, Schieppati M, Nardone A. Test-retest reliability of an insole plantar pressure system to assess gait along linear and curved trajectories. J Neuroeng Rehabil. (2014) 11:95. doi: 10.1186/1743-0003-11-95
34. Peyer KE, Brassey CA, Rose KA, Sellers WI. Locomotion pattern and foot pressure adjustments during gentle turns in healthy subjects. J Biomech. (2017) 60:65–71. doi: 10.1016/j.jbiomech.2017.06.025
35. Xu R, Wang X, Yang J, He F, Zhao X, Qi H, et al. Comparison of the COM-FCP inclination angle and other mediolateral stability indicators for turning. Biomed Eng Online. (2017) 16:37. doi: 10.1186/s12938-017-0325-z
36. Schieppati M. Coordination entre la posture et le movement. Le cas de la marche en courbe. In: Paillard T, editor. Posture et Équilibration Humaines. Paris: De Boeck Superieur (2016). p. 131–40.
37. Memari S, Do MC, Le Bozec S, Bouisset S. The consecutive postural adjustments (CPAs) that follow foot placement in single stepping. Neurosci Lett. (2013) 543:32–6. doi: 10.1016/j.neulet.2013.02.061
38. Taylor MJD, Dabnichki P, Strike SC. A three-dimensional biomechanical comparison between turning strategies during the stance phase of walking. Hum Mov Sci. (2005) 24:558–73. doi: 10.1016/j.humov.2005.07.005
39. Akram SB, Frank JS, Fraser J. Coordination of segments reorientation during on-the-spot turns in healthy older adults in eyes-open and eyes-closed conditions. Gait Posture. (2010) 32:632–6. doi: 10.1016/j.gaitpost.2010.09.006
40. Dotov DG, Bardy BG, Dalla Bella S. The role of environmental constraints in walking: effects of steering and sharp turns on gait dynamics. Sci Rep. (2016) 6:28374. doi: 10.1038/srep28374
41. Hess RJ, Brach JS, Piva SR, VanSwearingen JM. Walking skill can be assessed in older adults: validity of the Figure-of-8 Walk Test. Phys Ther. (2010) 90:89–99. doi: 10.2522/ptj.20080121
42. Lowry KA, Brach JS, Nebes RD, Studenski SA, VanSwearingen JM. Contributions of cognitive function to straight-and curved-path walking in older adults. Arch Phys Med Rehabil. (2012) 93:802–7. doi: 10.1016/j.apmr.2011.12.007
43. Cheung R. Parkinson's Disease and Segmental Coordination During Modified Figure of Eight Walking Turning Task (Master's Thesis). University of Jyväskylä (2016).
44. Solomon D, Kumar V, Jenkins RA, Jewell J. Head control strategies during whole-body turns. Exp Brain Res. (2006) 173:475–86. doi: 10.1007/s00221-006-0393-y
45. Earhart GM, Hong M. Kinematics of podokinetic after-rotation: similarities to voluntary turning and potential clinical implications. Brain Res Bull. (2006) 70:15–21. doi: 10.1016/j.brainresbull.2005.11.004
46. Robins RK, Hollands MA. The effects of constraining vision and eye movements on whole-body coordination during standing turns. Exp Brain Res. (2017) 235:3593–603. doi: 10.1007/s00221-017-5079-0
47. Ivanenko YP, Wright WG, Gurfinkel VS, Horak F, Cordo P. Interaction of involuntary post-contraction activity with locomotor movements. Exp Brain Res. (2006) 169:255–60. doi: 10.1007/s00221-005-0324-3
48. Courtine G, Papaxanthis C, Schieppati M. Coordinated modulation of locomotor muscle synergies constructs straight-ahead and curvilinear walking in humans. Exp Brain Res. (2006) 170:320–35. doi: 10.1007/s00221-005-0215-7
49. Bejarano NC, Pedrocchi A, Nardone A, Schieppati M, Baccinelli W, Monticone M, et al. Tuning of muscle synergies during walking along rectilinear and curvilinear trajectories in humans. Ann Biomed Eng. (2017) 45:1204–18. doi: 10.1007/s10439-017-1802-z
50. Choi Y, Kim Y, Kim M, Yoon B. Muscle synergies for turning during human walking. J Mot Behav. (2017) 51:1–9. doi: 10.1080/00222895.2017.1408558
51. Ventura JD, Klute GK, Neptune RR. Individual muscle contributions to circular turning mechanics. J Biomech. (2015) 48:1067–74. doi: 10.1016/j.jbiomech.2015.01.026
52. Jahn K, Deutschländer A, Stephan T, Strupp M, Wiesmann M, Brandt T. Brain activation patterns during imagined stance and locomotion in functional magnetic resonance imaging. Neuroimage. (2004) 22:1722–31. doi: 10.1016/j.neuroimage.2004.05.017
53. Wagner J, Stephan T, Kalla R, Brückmann H, Strupp M, Brandt T, et al. Mind the bend: cerebral activations associated with mental imagery of walking along a curved path. Exp Brain Res. (2008) 191:247–55. doi: 10.1007/s00221-008-1520-8
54. Sozzi S, Schieppati M. Stepping in place while voluntarily turning around produces a long-lasting posteffect consisting in inadvertent turning while stepping eyes closed. Neural Plast. (2016) 2016:7123609. doi: 10.1155/2016/7123609
55. Tisserand R, Dakin CJ, Van der Loos MH, Croft EA, Inglis TJ, Blouin JS. Down regulation of vestibular balance stabilizing mechanisms to enable transition between motor states. Elife. (2018) 7:e36123. doi: 10.7554/eLife.36123
56. Bove M, Diverio M, Pozzo T, Schieppati M. Neck muscle vibration disrupts steering of locomotion. J Appl Physiol. (2001) 91:581–8. doi: 10.1152/jappl.2001.91.2.581
57. Bove M, Courtine G, Schieppati M. Neck muscle vibration and spatial orientation during stepping in place in humans. J Neurophysiol. (2002) 88:2232–41. doi: 10.1152/jn.00198.2002
58. Courtine G, De Nunzio AM, Schmid M, Beretta MV, Schieppati M. Stance-and locomotion-dependent processing of vibration-induced proprioceptive inflow from multiple muscles in humans. J Neurophysiol. (2007) 97:772–9. doi: 10.1152/jn.00764.2006
59. Sozzi S, Nardone A, Crisafulli O, Schieppati M. Podokinetic after-rotation is transiently enhanced or reversed by unilateral axial muscle proprioceptive stimulation. Neural Plast. (2019) 2019:7129279. doi: 10.1155/2019/7129279
60. Duysens J, Faist M, Kooloos JG. The role of afferent feedback in the control of hamstrings activity during human gait. Eur J Morphol. (1998) 36:293–299.
61. Hubli M, Dietz V. The physiological basis of neurorehabilitation-locomotor training after spinal cord injury. J Neuroeng Rehabil. (2013) 10:5. doi: 10.1186/1743-0003-10-5
62. Glaister BC, Schoen JA, Orendurff MS, Klute GK. Mechanical behavior of the human ankle in the transverse plane while turning. IEEE Trans Neural Syst Rehabil Eng. (2007) 15:552–9. doi: 10.1109/TNSRE.2007.908944
63. Scott JT, Lohnes CA, Horak FB, Earhart GM. Podokinetic stimulation causes shifts in perception of straight ahead. Exp Brain Res. (2011) 208:313–21. doi: 10.1007/s00221-010-2480-3
64. Mulavara AP, Richards JT, Ruttley T, Marshburn A, Nomura Y, Bloomberg JJ. Exposure to a rotating virtual environment during treadmill locomotion causes adaptation in heading direction. Exp Brain Res. (2005) 166:210–9. doi: 10.1007/s00221-005-2356-0
65. Holtzer R, Epstein N, Mahoney JR, Izzetoglu M, Blumen HM. Neuroimaging of mobility in aging: a targeted review. J Gerontol A Biol Sci Med Sci. (2014) 69:1375–88. doi: 10.1093/gerona/glu052
66. Rosso AL, Verghese J, Metti AL, Boudreau RM, Aizenstein HJ, Kritchevsky S, et al. Slowing gait and risk for cognitive impairment: the hippocampus as a shared neural substrate. Neurology. (2017) 89:336–342. doi: 10.1212/WNL.0000000000004153
67. Cohen JA, Verghese J, Zwerling JL. Cognition and gait in older people. Maturitas. (2016) 93:73–7. doi: 10.1016/j.maturitas.2016.05.005
68. Kikkert LHJ, Vuillerme N, van Campen JP, Hortobágyi T, Lamoth CJ. Walking ability to predict future cognitive decline in old adults: a scoping review. Ageing Res Rev. (2016) 27:1–14. doi: 10.1016/j.arr.2016.02.001
69. Morris R, Lord S, Bunce J, Burn D, Rochester L. Gait and cognition: mapping the global and discrete relationships in ageing and neurodegenerative disease. Neurosci Biobehav Rev. (2016) 64:326–45. doi: 10.1016/j.neubiorev.2016.02.012
70. Bohannon RW. Comfortable and maximum walking speed of adults aged 20-79 years: reference values and determinants. Age Ageing. (1997) 26:15–19.
71. Beauchet O, Allali G, Sekhon H, Verghese J, Guilain S, Steinmetz JP, et al. Guidelines for assessment of gait and reference values for spatiotemporal gait parameters in older adults: the biomathics and canadian gait consortiums initiative. Front Hum Neurosci. (2017) 11:353. doi: 10.3389/fnhum.2017.00353
72. Herssens N, Verbecque E, Hallemans A, Vereeck L, Van Rompaey V, Saeys W. Do spatiotemporal parameters and gait variability differ across the lifespan of healthy adults? A systematic review. Gait Posture. (2018) 64:181–90. doi: 10.1016/j.gaitpost.2018.06.012
73. Thigpen MT, Light KE, Creel GL, Flynn SM. Turning difficulty characteristics of adults aged 65 years or older. Phys Ther. (2000) 80:1174–87. doi: 10.1093/ptj/80.12.1174
74. Kuo FC, Hong CZ, Liau BY. Kinematics and muscle activity of the head, lumbar and knee joints during 180 turning and sitting down task in older adults. Clin Biomech. (2014) 29:14–20. doi: 10.1016/j.clinbiomech.2013.10.020
75. Leach JM, Mellone S, Palumbo P, Bandinelli S, Chiari L. Natural turn measures predict recurrent falls in community-dwelling older adults: a longitudinal cohort study. Sci Rep. (2018) 8:4316. doi: 10.1038/s41598-018-22492-6
76. Do MC, Brenière Y, Brenguier P. A biomechanical study of balance recovery during the fall forward. J Biomech. (1982) 15:933–9.
77. Honeine JL, Schieppati M, Gagey O, Do MC. The functional role of the triceps surae muscle during human locomotion. PLoS ONE. (2013) 8:e52943. doi: 10.1371/journal.pone.0052943
78. Chong RK, Chastan N, Welter ML, Do MC. Age-related changes in the center of mass velocity control during walking. Neurosci Lett. (2009) 458:23–7. doi: 10.1016/j.neulet.2009.04.022
79. Verghese J, LeValley A, Hall CB, Katz MJ, Ambrose AF, Lipton RB. Epidemiology of gait disorders in community-residing older adults. J Am Geriatr Soc. (2006) 54:255–61. doi: 10.1111/j.1532-5415.2005.00580.x
80. Allali G, Verghese J. Management of gait changes and fall risk in MCI and dementia. Curr Treat Options Neurol. (2017) 19:29. doi: 10.1007/s11940-017-0466-1
81. Bohannon RW, Andrews AW. Normal walking speed: a descriptive meta-analysis. Physiotherapy. (2011) 97:182–9. doi: 10.1016/j.physio.2010.12.004
82. Guglielmetti S, Nardone A, De Nunzio AM, Godi M, Schieppati M. Walking along circular trajectories in Parkinson's disease. Mov Disord. (2009) 24:598–604. doi: 10.1002/mds.22424
83. Brach JS, McGurl D, Wert D, VanSwearingen JM, Perera S, Cham R, et al. Validation of a measure of smoothness of walking. J Gerontol A Biol Sci Med Sci. (2011) 66:136–41. doi: 10.1093/gerona/glq170
84. Gordt K, Müller C, Gerhardy T, Schwenk M. Einfluss von dual-tasking auf das Geradeaus-und Kurvengehen älterer Menschen. Z Gerontol Geriatr. (2018) 878:1–7. doi: 10.1007/s00391-018-01482-3
85. Turcato AM, Godi M, Giardini M, Arcolin I, Nardone A, Giordano A, et al. Abnormal gait pattern emerges during curved trajectories in high-functioning Parkinsonian patients walking in line at normal speed. PLoS ONE. (2018) 13:e0197264. doi: 10.1371/journal.pone.0197264
86. Bland K, Lowry K, Krajek A, Woods T, VanSwearingen J. Spatiotemporal variability underlying skill in curved-path walking. Gait Posture. (2019) 67:137–41. doi: 10.1016/j.gaitpost.2018.10.001
87. Odonkor CA, Thomas JC, Holt N, Latham N, VanSwearingen J, Brach JS, et al. A comparison of straight-and curved-path walking tests among mobility-limited older adults. J Gerontol A Biol Sci Med Sci. (2013) 68:1532–9. doi: 10.1093/gerona/glt060
88. He C, Xu R, Zhao M, Guo Y, Jiang S, He F, et al. Dynamic stability and spatiotemporal parameters during turning in healthy young adults. Biomed Eng Online. (2018) 17:127. doi: 10.1186/s12938-018-0558-5
89. Willems AM, Nieuwboer A, Chavret F, Desloovere K, Dom R, Rochester L, et al. Turning in Parkinson's disease patients and controls: the effect of auditory cues. Mov Disord. (2007) 22:1871–8. doi: 10.1002/mds.21445
90. McNeely ME, Earhart GM. Lack of short-term effectiveness of rotating treadmill training on turning in people with mild-to-moderate Parkinson's disease and healthy older adults: a randomized, controlled study. Parkinsons Dis. (2012) 2012:623985. doi: 10.1155/2012/623985
91. Bhatt H, Pieruccini-Faria F, Almeida QJ. Dynamics of turning sharpness influences freezing of gait in Parkinson's disease. Parkinsonism Relat Disord. (2013) 19:181–5. doi: 10.1016/j.parkreldis.2012.09.006
92. Conradsson D, Paquette C, Lökk J, Franzén E. Pre-and unplanned walking turns in Parkinson's disease–Effects of dopaminergic medication. Neuroscience. (2017) 341:18–26. doi: 10.1016/j.neuroscience.2016.11.016
93. Castro P, Kaski D, Schieppati M, Furman M, Arshad Q, Bronstein A. Subjective stability perception is related to postural anxiety in older subjects. Gait Posture. (2019) 68:538–44. doi: 10.1016/j.gaitpost.2018.12.043
94. Bengevoord A, Vervoort G, Spildooren J, Heremans E, Vandenberghe W, Bloem BR, et al. Center of mass trajectories during turning in patients with Parkinson's disease with and without freezing of gait. Gait Posture. (2016) 43:54–9. doi: 10.1016/j.gaitpost.2015.10.021
95. Dixon PC, Jacobs JV, Dennerlein JT, Schiffman JM. Late-cueing of gait tasks on an uneven brick surface impacts coordination and center of mass control in older adults. Gait Posture. (2018) 65:143–8. doi: 10.1016/j.gaitpost.2018.07.168
96. Huntley AH, Zettel JL, Vallis LA. Simultaneous turn and step task for investigating control strategies in healthy young and community dwelling older adults. Motor Control. (2017) 21:265–83. doi: 10.1123/mc.2015-0069
98. Maki BE. Gait changes in older adults: predictors of falls or indicators of fear? J Am Geriatr Soc. (1997) 45:313–20.
99. Brach JS, Berlin JE, VanSwearingen JM, Newman AB, Studenski SA. Too much or too little step width variability is associated with a fall history in older persons who walk at or near normal gait speed. J Neuroeng Rehabil. (2005) 2:21. doi: 10.1186/1743-0003-2-21
100. Mancini M, Schlueter H, El-Gohary M, Mattek N, Duncan C, Kaye J, et al. Continuous monitoring of turning mobility and its association to falls and cognitive function: a pilot study. J Gerontol A Biol Sci Med Sci. (2016) 71:1102–8. doi: 10.1093/gerona/glw019
101. Earhart GM. Dynamic control of posture across locomotor tasks. Mov Disord. (2013) 28:1501–8. doi: 10.1002/mds.25592
102. Fuller JR, Adkin AL, Vallis LA. Strategies used by older adults to change travel direction. Gait Posture. (2007) 25:393–400. doi: 10.1016/j.gaitpost.2006.05.013
103. Baird JL, Van Emmerik RE. Young and older adults use different strategies to perform a standing turning task. Clin Biomech. (2009) 24:826–32. doi: 10.1016/j.clinbiomech.2009.08.006
104. Akram SB, Frank JS, Fraser J. Effect of walking velocity on segment coordination during pre-planned turns in healthy older adults. Gait Posture. (2010) 32:211–4. doi: 10.1016/j.gaitpost.2010.04.017
105. Hassani A, Kubicki A, Brost V, Mourey F, Yang F. Kinematic analysis of motor strategies in frail aged adults during the Timed Up and Go: how to spot the motor frailty? Clin Interv Aging. (2015) 10:505–13. doi: 10.2147/CIA.S74755
106. Wright RL, Peters DM, Robinson PD, Sitch AJ, Watt TN, Hollands MA. Differences in axial segment reorientation during standing turns predict multiple falls in older adults. Gait Posture. (2012) 36:541–5. doi: 10.1016/j.gaitpost.2012.05.013
107. Paquette MR, Fuller JR, Adkin AL, Vallis LA. Age-related modifications in steering behaviour: effects of base-of-support constraints at the turn point. Exp Brain Res. (2008) 190:1–9. doi: 10.1007/s00221-008-1448-z
108. Mirelman A, Maidan I, Bernad-Elazari H, Shustack S, Giladi N, Hausdorff JM. Effects of aging on prefrontal brain activation during challenging walking conditions. Brain Cogn. (2017) 115:41–6. doi: 10.1016/j.bandc.2017.04.002
109. Caetano MJD, Menant JC, Schoene D, Pelicioni PHS, Sturnieks DL, Lord SR. Sensorimotor and cognitive predictors of impaired gait adaptability in older people. J Gerontol A Biol Sci Med Sci. (2016) 72:1257–63. doi: 10.1093/gerona/glw171
110. Chen M, Pillemer S, England S, Izzetoglu M, Mahoney JR, Holtzer R. Neural correlates of obstacle negotiation in older adults: An fNIRS study. Gait Posture. (2017) 58:130–5. doi: 10.1016/j.gaitpost.2017.07.043
111. Allali G, Blumen HM, Devanne H, Pirondini E, Delval A, Van De Ville D. Brain imaging of locomotion in neurological conditions. Neurophysiol Clin. (2018) 48:337–59. doi: 10.1016/j.neucli.2018.10.004
112. Boonstra TA, Van Der Kooij H, Munneke M, Bloem BR. Gait disorders and balance disturbances in Parkinson's disease: clinical update and pathophysiology. Curr Opin Neurol. (2008) 21:461–71. doi: 10.1097/WCO.0b013e328305bdaf
113. Manckoundia P, Mourey F, Pérennou D, Pfitzenmeyer P. Backward disequilibrium in elderly subjects. Clin Interv Aging. (2008) 3:667–72. doi: 10.2147/CIA.S3811
114. Chastan N, Do MC, Bonneville F, Torny F, Bloch F, Westby GM, et al. Gait and balance disorders in Parkinson's disease: impaired active braking of the fall of centre of gravity. Mov Disord. (2009) 24:188–95. doi: 10.1002/mds.22269
115. Rodriguez KL, Roemmich RT, Cam B, Fregly BJ, Hass CJ. Persons with Parkinson's disease exhibit decreased neuromuscular complexity during gait. Clin Neurophysiol. (2013) 124:1390–7. doi: 10.1016/j.clinph.2013.02.006
116. Lamontagne A, Fung J. Gaze and postural reorientation in the control of locomotor steering after stroke. Neurorehabil Neural Repair. (2009) 23:256–66. doi: 10.1177/1545968308324549
117. Godi M, Nardone A, Schieppati M. Curved walking in hemiparetic patients. J Rehabil Med. (2010) 42:858–65. doi: 10.2340/16501977-0594
118. Denommé LT, Mandalfino P, Cinelli ME. Strategies used by individuals with multiple sclerosis and with mild disability to maintain dynamic stability during a steering task. Exp Brain Res. (2014) 232:1811–22. doi: 10.1007/s00221-014-3873-5
119. Morris ME, Huxham F, McGinley J, Dodd K, Iansek R. The biomechanics and motor control of gait in Parkinson disease. Clin Biomech. (2001) 16:459–70. doi: 10.1016/S0268-0033(01)00035-3
120. Crenna P, Carpinella I, Rabuffetti M, Calabrese E, Mazzoleni P, Nemni R, et al. The association between impaired turning and normal straight walking in Parkinson's disease. Gait Posture. (2007) 26:172–8. doi: 10.1016/j.gaitpost.2007.04.010
121. Huxham F, Baker R, Morris ME, Iansek R. Footstep adjustments used to turn during walking in Parkinson's disease. Mov Disord. (2008) 23:817–23. doi: 10.1002/mds.21932
122. Mak MK, Patla A, Hui-Chan C. Sudden turn during walking is impaired in people with Parkinson's disease. Exp Brain Res. (2008) 190:43–51. doi: 10.1007/s00221-008-1446-1
123. Paquette C, Franzén E, Jones GM, Horak FB. Walking in circles: navigation deficits from Parkinson's disease but not from cerebellar ataxia. Neuroscience. (2011) 190:177–83. doi: 10.1016/j.neuroscience.2011.06.020
124. Peterson DS, Pickett KA, Duncan RP, Perlmutter JS, Earhart GM. Brain activity during complex imagined gait tasks in Parkinson disease. Clin Neurophysiol. (2014) 125:995–1005. doi: 10.1016/j.clinph.2013.10.008
125. Maidan I, Bernad-Elazari H, Gazit E, Giladi N, Hausdorff JM, Mirelman A. Changes in oxygenated hemoglobin link freezing of gait to frontal activation in patients with Parkinson disease: an fNIRS study of transient motor-cognitive failures. J Neurol. (2015) 262:899–908. doi: 10.1007/s00415-015-7650-6
126. Maidan I, Nieuwhof F, Bernad-Elazari H, Reelick MF, Bloem BR, Giladi N, et al. The role of the frontal lobe in complex walking among patients with Parkinson's disease and healthy older adults: an fNIRS study. Neurorehabil Neural Repair. (2016) 30:963–71. doi: 10.1177/1545968316650426
127. Ferrarin M, Carpinella I, Rabuffetti M, Calabrese E, Mazzoleni P, Nemni R. Locomotor disorders in patients at early stages of Parkinson's disease: a quantitative analysis. Conf Proc IEEE Eng Med Biol Soc. (2006) 1:1224–7. doi: 10.1109/IEMBS.2006.260677
128. Carpinella I, Crenna P, Calabrese E, Rabuffetti M, Mazzoleni P, Nemni R, et al. Locomotor function in the early stage of Parkinson's disease. IEEE Trans Neural Syst Rehabil Eng. (2007) 15:543–51. doi: 10.1109/TNSRE.2007.908933
129. Visser JE, Voermans NC, Nijhuis LBO, van der Eijk M, Nijk R, Munneke M, et al. Quantification of trunk rotations during turning and walking in Parkinson's disease. Clin Neurophysiol. (2007) 118:1602–6. doi: 10.1016/j.clinph.2007.03.010
130. Moore ST, MacDougall HG, Ondo WG. Ambulatory monitoring of freezing of gait in Parkinson's disease. J Neurosci Methods. (2008) 167:340–8. doi: 10.1016/j.jneumeth.2007.08.023
131. Hong M, Perlmutter JS, Earhart GM. A kinematic and electromyographic analysis of turning in people with Parkinson disease. Neurorehabil Neural Repair. (2009) 23:166–76. doi: 10.1177/1545968308320639
132. Akram S, Frank JS, Jog M. Parkinson's disease and segmental coordination during turning: I. Standing turns. Can. J Neurol Sci. (2013) 40:512–9. doi: 10.1017/S0317167100014591
133. Salarian A, Zampieri C, Horak FB, Carlson-Kuhta P, Nutt JG, Aminian K. Analyzing 180 degrees turns using an inertial system reveals early signs of progression of Parkinson's disease. Conf Proc IEEE Eng Med Biol Soc. (2009) 2009:224–7. doi: 10.1109/IEMBS.2009.5333970
134. Zampieri C, Salarian A, Carlson-Kuhta P, Aminian K, Nutt JG, Horak FB. The instrumented timed up and go test: potential outcome measure for disease modifying therapies in Parkinson's disease. J Neurol Neurosurg Psychiatry. (2010) 81:171–6. doi: 10.1136/jnnp.2009.173740
135. Palmerini L, Mellone S, Avanzolini G, Valzania F, Chiari L. Quantification of motor impairment in Parkinson's disease using an instrumented timed up and go test. IEEE Trans Neural Syst Rehabil Eng. (2013) 21:664–73. doi: 10.1109/TNSRE.2012.2236577
136. Ashburn A, Kampshoff C, Burnett M, Stack E, Pickering RM, Verheyden G. Sequence and onset of whole-body coordination when turning in response to a visual trigger: comparing people with Parkinson's disease and healthy adults. Gait Posture. (2014) 39:278–83. doi: 10.1016/j.gaitpost.2013.07.128
137. Mancini M, El-Gohary M, Pearson S, McNames J, Schlueter H, Nutt JG, et al. Continuous monitoring of turning in Parkinson's disease: rehabilitation potential. NeuroRehabil. (2015) 37:3–10. doi: 10.3233/NRE-151236
138. Hulbert S, Ashburn A, Robert L, Verheyden G. A narrative review of turning deficits in people with Parkinson's disease. Disabil Rehabil. (2015) 37:1382–9. doi: 10.3109/09638288.2014.961661
139. Huxham F, Baker R, Morris ME, Iansek R. Head and trunk rotation during walking turns in Parkinson's disease. Mov Disord. (2008) 23:1391–7. doi: 10.1002/mds.21943
140. Song J, Sigward S, Fisher B, Salem GJ. Altered dynamic postural control during step turning in persons with early-stage Parkinson's disease. Parkinsons Dis. (2012) 2012:386962. doi: 10.1155/2012/386962
141. Haertner L, Elshehabi M, Zaunbrecher L, Pham MH, Maetzler C, van Uem JM, et al. Effect of fear of falling on turning performance in parkinson's disease in the lab and at home. Front Aging Neurosci. (2018) 10:78. doi: 10.3389/fnagi.2018.00078
142. Yiou E, Deroche T, Do MC, Woodman T. Influence of fear of falling on anticipatory postural control of medio-lateral stability during rapid leg flexion. Eur J Appl Physiol. (2011) 111:611–20. doi: 10.1007/s00421-010-1680-7
143. Yiou E, Hussein T, Larue J. Influence of temporal pressure on anticipatory postural control of medio-lateral stability during rapid leg flexion. Gait Posture. (2012) 35:494–9. doi: 10.1016/j.gaitpost.2011.11.015
144. Hausdorff JM, Schaafsma JD, Balash Y, Bartels AL, Gurevich T, Giladi N. Impaired regulation of stride variability in Parkinson's disease subjects with freezing of gait. Exp Brain Res. (2003) 149:187–94. doi: 10.1007/s00221-002-1354-8
145. Schaafsma JD, Balash Y, Gurevich T, Bartels AL, Hausdorff JM, Giladi N. Characterization of freezing of gait subtypes and the response of each to levodopa in Parkinson's disease. Eur J Neurol. (2003) 10:391–8. doi: 10.1046/j.1468-1331.2003.00611.x
146. Rahman S, Griffin HJ, Quinn NP, Jahanshahi M. The factors that induce or overcome freezing of gait in Parkinson's disease. Behav Neurol. (2008) 19:127–36. doi: 10.1155/2008/456298
147. Spildooren J, Vercruysse S, Desloovere K, Vandenberghe W, Kerckhofs E, Nieuwboer A. Freezing of gait in Parkinson's disease: the impact of dual-tasking and turning. Mov Disord. (2010) 25:2563–70. doi: 10.1002/mds.23327
148. Nutt JG, Bloem BR, Giladi N, Hallett M, Horak FB, Nieuwboer A. Freezing of gait: moving forward on a mysterious clinical phenomenon. Lancet Neurol. (2011) 10:734–44. doi: 10.1016/S1474-4422(11)70143-
149. Peterson DS, Plotnik M, Hausdorff JM, Earhart GM. Evidence for a relationship between bilateral coordination during complex gait tasks and freezing of gait in Parkinson's disease. Parkinsonism Relat Disord. (2012) 18:1022–6. doi: 10.1016/j.parkreldis.2012.05.019
150. Spildooren J, Vercruysse S, Meyns P, Vandenbossche J, Heremans E, Desloovere K, et al. Turning and unilateral cueing in Parkinson's disease patients with and without freezing of gait. Neuroscience. (2012) 207:298–306. doi: 10.1016/j.neuroscience.2012.01.024
151. Djurić-Jovičić MD, Jovičić NS, Radovanović SM, Stanković ID, Popović MB, Kostić VS. Automatic identification and classification of freezing of gait episodes in Parkinson's disease patients. IEEE Trans Neural Syst Rehabil Eng. (2014) 22:685–94. doi: 10.1109/TNSRE.2013.2287241
152. Nonnekes J, Janssen AM, Mensink SH, Nijhuis LBO, Bloem BR, Snijders AH. Short rapid steps to provoke freezing of gait in Parkinson's disease. J Neurol. (2014) 261:1763–7. doi: 10.1007/s00415-014-7422-8
153. Peterson DS, Pickett KA, Duncan R, Perlmutter J, Earhart GM. Gait-related brain activity in people with Parkinson disease with freezing of gait. PLoS ONE. (2014) 9:e90634. doi: 10.1371/journal.pone.0090634
154. Okuma Y. Freezing of gait and falls in Parkinson's disease. J Parkinsons Dis. (2014) 4:255–60. doi: 10.3233/JPD-130282
155. Mancini M, Weiss A, Herman T, Hausdorff JM. Turn around freezing: community-living turning behavior in people with Parkinson's disease. Front Neurol. (2018) 9:18. doi: 10.3389/fneur.2018.00018
156. Plotnik M, Giladi N, Hausdorff JM. Bilateral coordination of walking and freezing of gait in Parkinson's disease. Eur J Neurosci. (2008) 27:1999–2006. doi: 10.1111/j.1460-9568.2008.06167.x
157. Fasano A, Herzog J, Seifert E, Stolze H, Falk D, Reese R, et al. Modulation of gait coordination by subthalamic stimulation improves freezing of gait. Mov Disord. (2011) 26:844–51. doi: 10.1002/mds.23583
158. Snijders AH, Leunissen I, Bakker M, Overeem S, Helmich RC, Bloem BR, et al. Gait-related cerebral alterations in patients with Parkinson's disease with freezing of gait. Brain. (2010) 134:59–72. doi: 10.1093/brain/awq324
159. Franzén E, Paquette C, Gurfinkel VS, Cordo PJ, Nutt JG, Horak FB. Reduced performance in balance, walking and turning tasks is associated with increased neck tone in Parkinson's disease. Exp Neurol. (2009) 219:430–8. doi: 10.1016/j.expneurol.2009.06.013
160. Yang WC, Hsu WL, Wu RM, Lu TW, Lin KH. Motion analysis of axial rotation and gait stability during turning in people with Parkinson's disease. Gait Posture. (2016) 44:83–8. doi: 10.1016/j.gaitpost.2015.10.023
161. El-Gohary M, Pearson S, McNames J, Mancini M, Horak F, Mellone S, et al. Continuous monitoring of turning in patients with movement disability. Sensors. (2014) 14:356–69. doi: 10.3390/s140100356
162. Fietzek UM, Stuhlinger L, Plate A, Ceballos-Baumann A, Bötzel K. Spatial constraints evoke increased number of steps during turning in Parkinson's disease. Clin Neurophysiol. (2017) 128:1954–60. doi: 10.1016/j.clinph.2017.07.399
163. van Dijsseldonk K, Wang Y, van Wezel R, Bloem BR, Nonnekes J. Provoking freezing of gait in clinical practice: turning in place is more effective than stepping in place. J Parkinsons Dis. (2018) 8:363–5. doi: 10.3233/JPD-181332
164. Mancini M, Smulders K, Cohen RG, Horak FB, Giladi N, Nutt JG. The clinical significance of freezing while turning in Parkinson's disease. Neuroscience. (2017) 343:222–8. doi: 10.1016/j.neuroscience.2016.11.045
165. Traub MM, Rothwell JC, Marsden CD. Anticipatory postural reflexes in Parkinson's disease and other akinetic-rigid syndromes and in cerebellar ataxia. Brain. (1980) 103:393–412
166. Burleigh-Jacobs A, Horak FB, Nutt JG, Obeso JA. Step initiation in Parkinson's disease: influence of levodopa and external sensory triggers. Mov Disord. (1997) 12:206–15. doi: 10.1002/mds.870120211
167. Delval A, Tard C, Defebvre L. Why we should study gait initiation in Parkinson's disease. Neurophysiol Clin. (2014) 44:69–76. doi: 10.1016/j.neucli.2013.10.127
168. King LA, Horak FB. Lateral stepping for postural correction in Parkinson's disease. Arch Phys Med Rehabil. (2008) 89:492–9. doi: 10.1016/j.apmr.2007.11.017
169. Schlenstedt C, Mancini M, Horak F, Peterson D. Anticipatory postural adjustment during self-initiated, cued, and compensatory stepping in healthy older adults and patients with Parkinson disease. Arch Phys Med Rehabil. (2017) 98:1316–24. doi: 10.1016/j.apmr.2017.01.023
170. Plate A, Klein K, Pelykh O, Singh A, Bötzel K. Anticipatory postural adjustments are unaffected by age and are not absent in patients with the freezing of gait phenomenon. Exp Brain Res. (2016) 234:2609–18. doi: 10.1007/s00221-016-4665-x
171. Jacobs JV, Nutt JG, Carlson-Kuhta P, Stephens M, Horak FB. Knee trembling during freezing of gait represents multiple anticipatory postural adjustments. Exp Neurol. (2009) 215:334–41. doi: 10.1016/j.expneurol.2008.10.019
172. Peterson DS, Lohse KR, Mancini M. Anticipatory postural responses prior to protective steps are not different in people with PD who do and do not freeze. Gait Posture. (2018) 64:126–9. doi: 10.1016/j.gaitpost.2018.06.006
173. Schlenstedt C, Mancini M, Nutt J, Hiller AP, Maetzler W, Deuschl G, et al. Are hypometric anticipatory postural adjustments contributing to freezing of gait in Parkinson's disease? Front Aging Neurosci. (2018) 10:36. doi: 10.3389/fnagi.2018.00036
174. Capato TTC, Nonnekes J, Barbosa ER, Bloem BR. Internal and external compensation strategies to alleviate upper limb freezing in Parkinson's disease. Parkinsonism Relat Disord. (2019). doi: 10.1016/j.parkreldis.2019.03.008. [Epub ahead of print].
175. Cumming RG, Klineberg RJ. Fall frequency and characteristics and the risk of hip fractures. J Am Geriatr Soc. (1994) 42:774–8.
176. Morris ME. Locomotor training in people with Parkinson disease. Phys Ther. (2006) 86:1426–35. doi: 10.2522/ptj.20050277
177. Xu Q, Park Y, Huang X, Hollenbeck A, Blair A, Schatzkin A, et al. Physical activities and future risk of Parkinson disease. Neurology. (2010) 75:341–8. doi: 10.1212/WNL.0b013e3181ea1597
178. Frazzitta G, Balbi P, Maestri R, Bertotti G, Boveri N, Pezzoli G. The beneficial role of intensive exercise on Parkinson disease progression. Am J Phys Med Rehabil. (2013) 92:523–32. doi: 10.1097/PHM.0b013e31828cd254
179. Giardini M, Nardone A, Godi M, Guglielmetti S, Arcolin I, Pisano F, et al. Instrumental or physical-exercise rehabilitation of balance improves both balance and gait in Parkinson's disease. Neural Plast. (2018) 2018:5614242. doi: 10.1155/2018/5614242
180. Tomlinson CL, Patel S, Meek C, Herd CP, Clarke CE, Stowe R, et al. Physiotherapy versus placebo or no intervention in Parkinson's disease. Cochrane Database Syst Rev. (2013) 11:CD002817. doi: 10.1002/14651858.CD002817.pub4
181. Aruin AS, Kanekar N, Lee YJ, Ganesan M. Enhancement of anticipatory postural adjustments in older adults as a result of a single session of ball throwing exercise. Exp Brain Res. (2015) 233:649–55. doi: 10.1007/s00221-014-4144-1
182. Bello O, Fernandez-Del-Olmo M. How does the treadmill affect gait in Parkinson's disease? Curr Aging Sci. (2012) 5:28–34. doi: 10.2174/1874612811205010028
183. Arcolin I, Pisano F, Delconte C, Godi M, Schieppati M, Mezzani A, et al. Intensive cycle ergometer training improves gait speed and endurance in patients with Parkinson's disease: a comparison with treadmill training. Restor Neurol Neurosci. (2016) 34:125–38. doi: 10.3233/RNN-150506
184. Mehrholz J, Kugler J, Storch A, Pohl M, Hirsch K, Elsner B. Treadmill training for patients with Parkinson Disease. An abridged version of a Cochrane Review. Eur J Phys Rehabil Med. (2016) 52:704–13.
185. Warlop T, Detrembleur C, Stoquart G, Lejeune T, Jeanjean A. Gait complexity and regularity are differently modulated by treadmill walking in parkinson's disease and healthy population. Front Physiol. (2018) 9:68. doi: 10.3389/fphys.2018.00068
186. Hong M, Perlmutter JS, Earhart GM. Podokinetic after-rotation in Parkinson disease. Brain Res. (2007) 1128:99–106. doi: 10.1016/j.brainres.2006.10.053
187. Hong M, Earhart GM. Rotating treadmill training reduces freezing in Parkinson disease: preliminary observations. Parkins Relat Disord. (2008) 14:359–63. doi: 10.1016/j.parkreldis.2007.07.003
188. Godi M, Giardini M, Nardone A, Turcato AM, Caligari M, Pisano F, et al. Curved walking rehabilitation with a rotating treadmill in patients with Parkinson's disease: a proof of concept. Front Neurol. (2017) 8:53. doi: 10.3389/fneur.2017.00053
189. Marinelli L, Quartarone A, Hallett M, Frazzitta G, Ghilardi MF. The many facets of motor learning and their relevance for Parkinson's disease. Clin Neurophysiol. (2017) 128:1127–41. doi: 10.1016/j.clinph.2017.03.042
190. Cheng FY, Yang YR, Chen LM, Wu YR, Cheng SJ, Wang RY. Positive effects of specific exercise and novel turning-based treadmill training on turning performance in individuals with Parkinson's disease: a randomized controlled trial. Sci Rep. (2016) 6:33242. doi: 10.1038/srep33242
191. Cheng FY, Yang YR, Wu YR, Cheng SJ, Wang RY. Effects of curved-walking training on curved-walking performance and freezing of gait in individuals with Parkinson's disease: a randomized controlled trial. Parkinsonism Relat Disord. (2017) 43:20–6. doi: 10.1016/j.parkreldis.2017.06.021
192. Jürgens R, Boss T, Becker W. Podokinetic after-rotation does not depend on sensory conflict. Exp Brain Res. (1999) 128:563–7.
193. Weber KD, Fletcher WA, Gordon CR, Melvill Jones G, Block EW. Motor learning in the “podokinetic” system and its role in spatial orientation during locomotion. Exp Brain Res. (1998) 120:377–85.
194. Aman JE, Elangovan N, Yeh I, Konczak J. The effectiveness of proprioceptive training for improving motor function: a systematic review. Front Hum Neurosci. (2015) 8:1075. doi: 10.3389/fnhum.2014.01075
195. Elangovan N, Tuite P, Konczak J. Somatosensory training improves proprioception and untrained motor function in Parkinson's disease. Front Neurol. (2018) 9:1053. doi: 10.3389/fneur.2018.01053
196. Bzdúšková D, Valkovič P, Hirjaková Z, Kimijanová J, Hlavačka F. Parkinson's disease versus ageing: different postural responses to soleus muscle vibration. Gait Posture. (2018) 65:169–75. doi: 10.1016/j.gaitpost.2018.07.162
197. De Nunzio AM, Nardone A, Schieppati M. The control of equilibrium in Parkinson's disease patients: delayed adaptation of balancing strategy to shifts in sensory set during a dynamic task. Brain Res Bull. (2007) 74:258–70. doi: 10.1016/j.brainresbull.2007.06.020
198. De Nunzio AM, Nardone A, Picco D, Nilsson J, Schieppati M. Alternate trains of postural muscle vibration promote cyclic body displacement in standing parkinsonian patients. Mov Disord. (2008) 23:2186–93. doi: 10.1002/mds.22201
199. De Nunzio AM, Grasso M, Nardone A, Godi M, Schieppati M. Alternate rhythmic vibratory stimulation of trunk muscles affects walking cadence and velocity in Parkinson's disease. Clin Neurophysiol. (2010) 121:240–7. doi: 10.1016/j.clinph.2009.10.018
Keywords: curved walking, aging, Parkinson's disease, freezing of gait, curved walking rehabilitation
Citation: Godi M, Giardini M and Schieppati M (2019) Walking Along Curved Trajectories. Changes With Age and Parkinson's Disease. Hints to Rehabilitation. Front. Neurol. 10:532. doi: 10.3389/fneur.2019.00532
Received: 08 February 2019; Accepted: 03 May 2019;
Published: 24 May 2019.
Edited by:
Helena Blumen, Albert Einstein College of Medicine, United StatesReviewed by:
Gammon Earhart, Washington University in St. Louis, United StatesElisabetta Farina, Fondazione Don Carlo Gnocchi Onlus (IRCCS), Italy
Copyright © 2019 Godi, Giardini and Schieppati. This is an open-access article distributed under the terms of the Creative Commons Attribution License (CC BY). The use, distribution or reproduction in other forums is permitted, provided the original author(s) and the copyright owner(s) are credited and that the original publication in this journal is cited, in accordance with accepted academic practice. No use, distribution or reproduction is permitted which does not comply with these terms.
*Correspondence: Marco Godi, bWFyY28uZ29kaUBpY3NtYXVnZXJpLml0
†Present Address: Marco Schieppati, ICS Maugeri SPA SB, Pavia, Italy