- 1Division of Cardiovascular Sciences, University of Manchester, Manchester, United Kingdom
- 2Department of Neurology, Greater Manchester Neuroscience Centre, Salford Royal NHS Foundation Trust, Salford, United Kingdom
- 3Division of Neuroscience and Experimental Psychology, Manchester Academic Health Science Centre, University of Manchester, Manchester, United Kingdom
- 4Department of Clinical Neurophysiology, Central Manchester NHS Foundation Trust, Manchester, United Kingdom
- 5Weill Cornell Medicine-Qatar, Doha, Qatar
Background: Previous studies have shown cutaneous small fiber pathology in patients with Parkinson's disease (PD). These studies have focused on nerve degeneration, but recent reports suggest that nerve regeneration may also be important in PD pathology.
Objective: To establish the extent of intraepidermal nerve fiber (IENF) degeneration and regeneration and its relationship to clinical and neurological deficits in Parkinson's disease (PD).
Methods: Twenty-three PD patients and 10 age-matched controls underwent skin biopsy and assessment of somatic and autonomic symptoms and deficits. We have assessed Intraepidermal Nerve Fiber Density (IENFD) using standard PGP9.5 staining and GAP-43 to assess Mean Axonal Length (MAL) and Intraepidermal Total Nerve Fiber Length (IETNFL).
Results: IENFD (p < 0.0001), MAL (p < 0.0001), IETNFL/Area (p = 0.009), and IETNFL/Length (p = 0.04) were significantly reduced in patients with PD compared to controls. IENFD correlated significantly with disease duration (p = 0.03), cumulative levodopa dose (p = 0.02), Unified Parkinson's Disease Rating Scale, Part III (UPDRS-III) (p = 0.01), Schwab and England Activities of Daily Living (ADL) (p = 0.03), NSP (p = 0.03), and 30:15 ratio (p = 0.03). IETNFL/Area correlated with the Autonomic Scale for Outcomes in Parkinson's Disease (SCOPA-AUT) (p = 0.03) and Diabetic Neuropathy Symptom score (DNS) (p = 0.04) and IETNFL/Length correlated with DNS (p = 0.03). MAL correlated with SCOPA-AUT (p = 0.01), DNS (p = 0.02), and DB-HRV (p = 0.02).
Conclusion: Increased IENF degeneration and impaired regeneration correlates with somatic and autonomic symptoms and deficits in patients with PD.
Introduction
Parkinson's disease (PD) affects not only the central, but also the peripheral nervous system (1). Indeed, peripheral neuropathy (PN) has been reported in 55% of patients with idiopathic PD (2) and a recent systematic review has shown that 56.9% have biopsy proven small fiber neuropathy (3, 4). Pilomotor, sudomotor, and vasomotor dysfunction occur in patients with early PD (5). A recent study has shown a reduction in intraepidermal nerve fiber density (IENFD) and a loss of autonomic nerves to blood vessels, sweat glands, and arrector pili muscles and Meissner corpuscles and their myelinated endings in patients with PD compared to controls. Furthermore, the study also showed reduced IENFD on the predominantly affected and unaffected sides (6).
Over the last 4 years several studies have co-localized α-synuclein to nerves in skin biopsies (7, 8). However, deposition of phosphorylated and unphosphorylated α-synuclein was not associated with dermal autonomic denervation (3, 9) duration or severity of PD (10) or the side with the greatest motor deficits (11). Wang et al. have reported that α-synuclein deposition is increased in cutaneous sympathetic but not in sensory nerve fibers (3). Indeed, the utility of assessing peripheral α-synuclein deposition has been questioned, with a call for large multicentre trials using stringent detection and assessment methodology (12).
IENFD is the gold standard measure for small fiber neuropathy (SFN) (13) and has been advocated to study patients with PD (1, 7). Indeed, a recent study has shown that 82.1% of patients had reduced IENFD, whilst only 64.3% had reduced contact heat-evoked potentials and 32.1% had abnormal thermal thresholds (14). In our recent studies in patients with PD we have demonstrated a reduction in IENFD and corneal innervation, which was related to autonomic dysfunction (15) and perception of affective touch (16).
Traditionally, the morphological quantification of IENF has relied on immunostaining nerves with the pan-axonal marker, Protein Gene Product 9.5 (PGP 9.5) to derive a density of nerve fibers crossing the basement membrane into the epidermis as a marker of nerve degeneration. No previous studies have quantified detailed intraepidermal nerve fiber morphology, such as mean axonal length (MAL) or Intraepidermal Total Nerve Fiber Length (IETNFL) in PD.
Furthermore, recent studies have suggested that in early PD nerve regeneration may compensate for nerve degeneration and this regenerative capacity may decline over time (15, 17). No previous studies have directly assessed nerve regeneration in PD. Growth-associated protein-43 (GAP-43) was first described in growing nerve cones and has been shown to play a crucial role in neuromodulation and axonal regeneration in both animal and human studies (18, 19). GAP-43 staining has been applied to show altered cutaneous nerve fiber regeneration in patients with painful diabetic neuropathy (20). We have recently immunostained with GAP-43 and quantified Intraepidermal Total Nerve Fiber Length (IETNFL) and demonstrated increased epidermal nerve regeneration in patients with sarcoid neuropathy after treatment with Cibinetide (21).
In the present study we have undertaken detailed quantification of both intraepidermal nerve fiber degeneration and regeneration after immunostaining with PGP 9.5 (IENFD) and GAP-43 (MAL, IETNF). We have also related these markers of degeneration and regeneration to clinical and neurological deficits in patients with PD.
Methods
Standard Protocol Approvals, Registrations, and Patient Consents
The study was approved by NRES committee/North West (Ref. No 12/NW/0086). All participants gave their written informed consent.
Study Population
Twenty-three patients (13 males, 10 females) fulfilling the UK Brain Bank criteria for the diagnosis of Parkinson's disease were recruited from neurology clinics after screening for other causes of peripheral neuropathy (cancer, chemotherapy, diabetes, impaired glucose tolerance, alcoholism, vitamin B6 and B12 deficiencies and autoimmune conditions). Ten age-matched healthy volunteers, non-related to the PD patients, served as controls.
Clinical and Neurological Evaluation
PD duration was calculated in years from the onset of symptoms to the date of assessment. Patients were assessed in the “ON” state. The Unified Parkinson's Disease Rating Scale, Part III (UPDRS-III) (22) was used to rate motor symptom severity. The levodopa dose was expressed as total dose from the date first prescribed till the date of assessment.
Non-motor symptoms and pain were assessed using the non-motor symptoms scale (NMSS) (23), King's Parkinson's Pain Scale (KPPS) (24), Neuropathy Symptom Profile (NSP) (25), Diabetic Neuropathy Symptom score (DNS) (26), Schwab and England activities of daily living (ADL) scale and short form of McGill Pain Questionnaire (SFMPQ) (27). The Autonomic Scale for Outcomes in Parkinson's Disease (SCOPA-AUT) (28) was used to assess autonomic symptoms. Parasympathetic dysfunction was assessed by measurement of deep breathing heart variability (DB-HRV) and the heart rate ratio of the R-R interval at the 30th and 15th beat response to standing (30:15 ratio), using an ANX 3.0 autonomic nervous system device (ANSAR Medical Technologies Inc., Philadelphia). Blood pressure and heart rate in the supine position and after standing for 5 min were measured to evaluate for postural hypotension.
All patients underwent assessment of the neuropathy disability score (NDS) (29), vibration perception threshold (VPT) using a Neurothesiometer (Howell, Scientific Laboratory Supplies, Nottingham, U.K.) on both feet; thermal perception thresholds (warm [WT] and cold [CT]), heat-induced pain (WIP) and cold-induced pain (CIP) on the dorsum of the foot using a MEDOC TSA II (Medoc Ltd., Ramat-Yishai 20095, Israel) and nerve conduction studies were performed in the lower limbs.
Skin Biopsy
Patients underwent 3 mm skin punch biopsies from the dorsum of each foot 3 cm above the third metatarsal. The samples were immediately fixed in 4% paraformaldehyde, cryo-protected in graded solutions of sucrose, frozen and stored at −80°C. The biopsies were sectioned perpendicular to the epidermis at 50 μm thickness on a cryo-microtome. Six non-consecutive sections were immunostained with the pan-axonal marker PGP9.5 (Abcam, Cambridge, U.K.) and used for IENFD quantification according to international guidelines (13). Six non-consecutive sections were immunostained with GAP-43 (Novus Biologicals, Oxon, U.K.), a specific marker for new or recently regenerated nerve fibers (18). The nerve fibers were visualized using SG chromogen (Vector Laboratories, Peterborough, U.K.).
A pathologist blinded to patients' details performed analysis of the sections. When assessing the GAP-43 staining, special care was taken to orientate skin sections such that the surface of the epidermis was parallel to the upper limit of the photographed field. Sections were imaged using a Zeiss AxioImager M2 microscope (Carl Zeiss, Jena, Germany) equipped with a motorized stage and digital camera. Each image for analysis was formed from Z-stacked optical layers, so that each final image contained all nerve fibers present in the full depth of the 50 μm section (post-sample processing), and an average of 20 images was obtained from each biopsy. All image measurements were performed using the Zen 2 image analysis programme (Zeiss Microimaging, Jena, Germany). The total nerve fiber length (TNFL) in the epidermis was normalized per millimeter length (TNFL/Length) and mm2 area (TNFL/Area) of epidermis, according to our recently described method (21). The mean length of nerve fibers crossing the basement membrane (BM) into the epidermis (MAL) was measured on GAP-43 immunostained sections. All nerve fiber parameters were obtained as an average of measurements in skin biopsies for the left and right foot.
Statistical Analysis
GraphPad Prism v. 7.0 (GraphPad Software, Inc., USA) was used to perform all statistical analyses. The Shapiro-Wilk Test was used to assess normality. Two-tailed unpaired t-test or Mann-Whitney rank test were used to compare control and PD nerve fiber measurements. P<0.05 was considered to be significant. Cohen d was calculated to measure effect size. Two-tailed Spearman correlation was used to assess the relationship between nerve fiber measurements and clinical measurements. The two-stage step-up method of Benjamini, Krieger and Yekutieli for False Discovery Rate was used to correct for multiple correlation calculations. q ≤ 0.2 was considered to be significant.
Results
Patients with PD had a median Hoehn and Yahr score of II (range I–III) and a mean UPDRS motor score of 25.96 ± 12.7, consistent with moderate disease severity (Table 1).
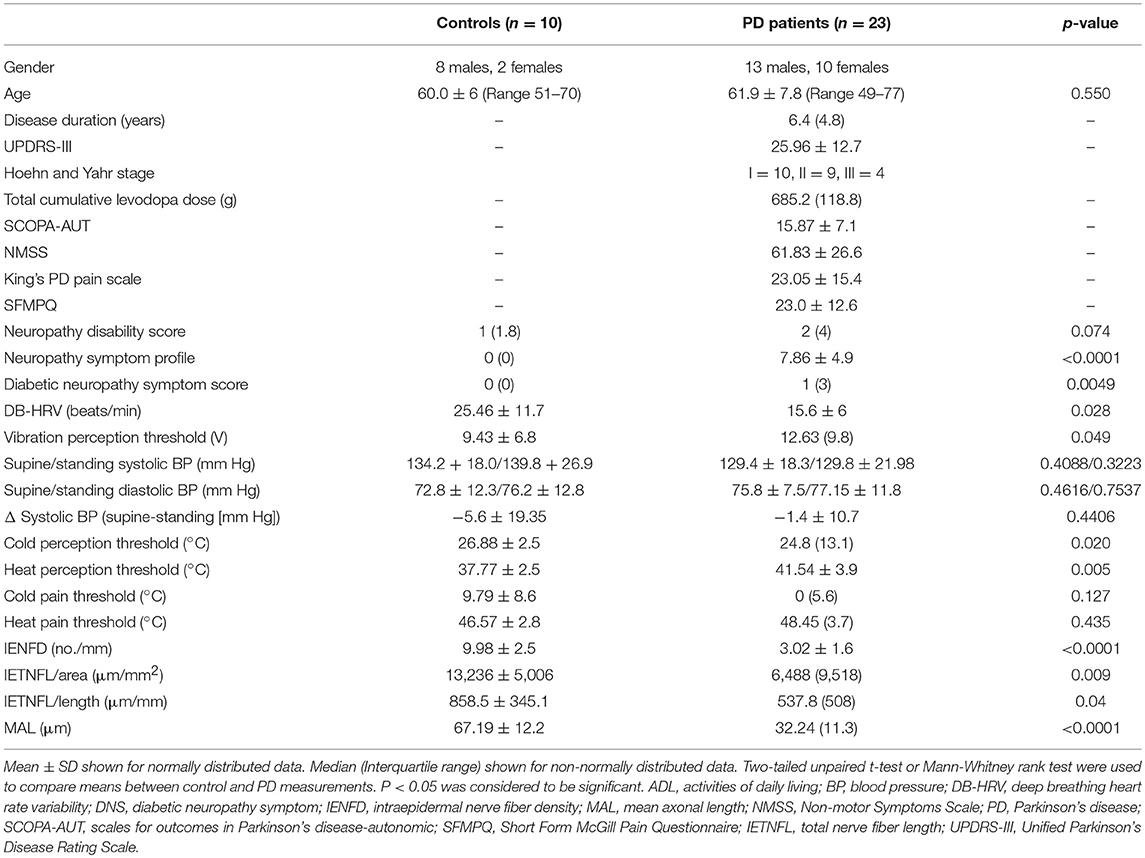
Table 1. Demographics, clinical characteristics and quantitative measures of neuropathy in patients with PD and control subjects.
Neurological Symptoms, Deficits, Quantitative Sensory, and Autonomic Testing
The NMSS, King's PD pain scale; SFMPQ and NSP indicated painful symptoms. VPT (p = 0.05), CT (p = 0.02), and WT (p = 0.005) were significantly elevated in patients with PD compared to controls (Table 1). There was no significant difference in the systolic or diastolic blood pressure on standing or 30:15 ratio, but DB-HRV was significantly reduced (p = 0.03) in patients with PD compared to controls.
Intraepidermal Nerve Fiber Morphology
There was a significant reduction in IENFD (p < 0.0001), IETNFL/Area (p = 0.009), IETNFL/Length (p = 0.04), MAL (p < 0.0001) in patients with PD compared to control subjects (Table 1; Figures 1, 2).
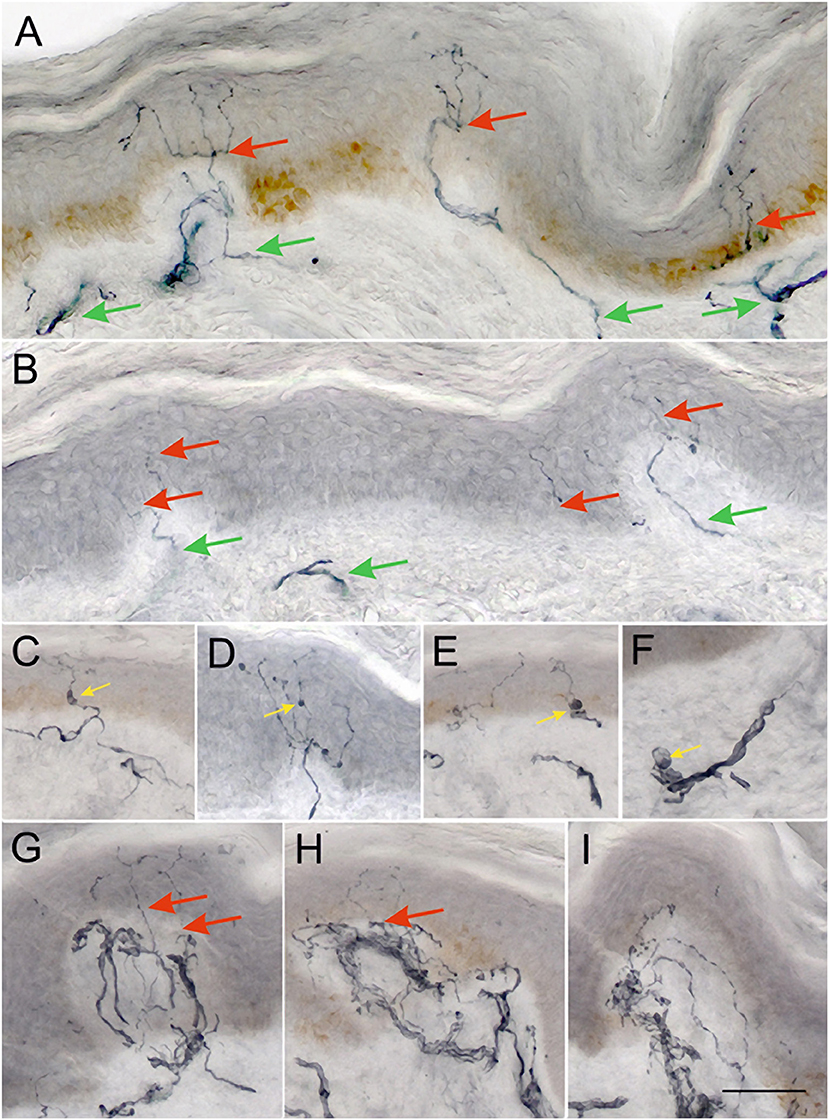
Figure 1. Representative examples of 50 μm sections from skin biopsies immunostained for GAP-43. Healthy control (A) shows numerous long branching intraepidermal nerve fibers (red arrows) reaching upper layers of epidermis and well-developed sub-epidermal nerve plexus (green arrows). Biopsy from a PD patient (B) showing scant, faintly staining intraepidermal nerve fibers (red arrows), and scant subepidermal plexus (green arrows). Images (C–E) show examples of axonal swellings along the IENFs and sub-epidermal nerve fibers (F) (yellow arrows). Images (G–I) show focal accumulations of nerve fibers in subepidermal area, which give rise only to occasional NFs crossing the BM into the epidermis (red arrows). Such areas are often separated by stretches of papillary dermis with very scant subepidermal plexus as in (B). (A–I) were taken at the same magnification ×200, scale bar = 100 μm.
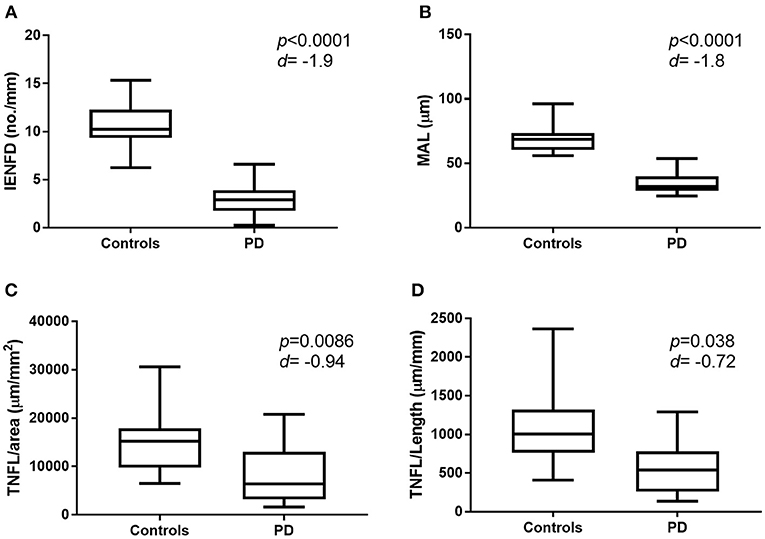
Figure 2. Graphic representation of nerve fiber measurement in healthy control subjects and patients with PD. Box and whisker plot showing mean, min and max values for IENFD (A), MAL (B), IETNFL/Area (C), and IETNFL/Length (D). Two-tailed unpaired Mann-Whitney rank test was used to compare means between control and PD nerve fiber measurements. P-value and effect size (d) shown (d > 0.5 = medium effect; over 1.0 = large effect). IENFD, intraepidermal nerve fiber density; MAL, mean axonal length; IETNFL, intraepidermal total nerve fiber length.
Correlation of IENF Morphology With Clinical Data
IENFD correlated significantly with disease duration (p = 0.03), cumulative levodopa dose (p = 0.02), UPDRS-III (p = 0.01), Schwab and England ADL (p = 0.03), NSP (p = 0.03), and 30:15 ratio (p = 0.03). IETNFL/Area correlated with SCOPA-AUT (p = 0.03) and DNS (p = 0.04) and IETNFL/Length correlated with DNS (p = 0.03). MAL correlated with SCOPA-AUT (p = 0.01), DNS (p = 0.02) and DB-HRV (p = 0.02) (Table 2).
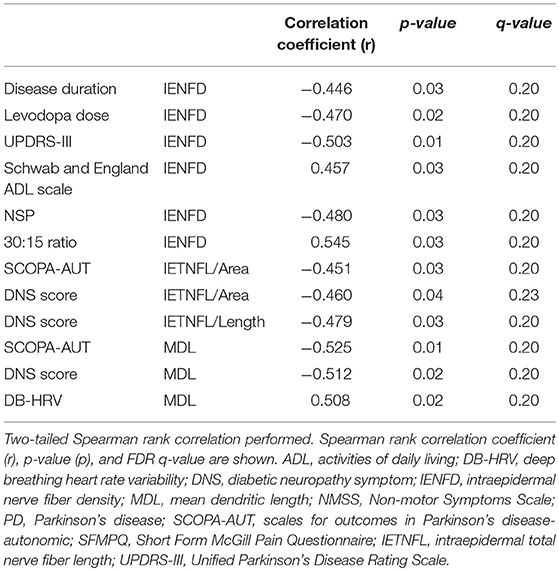
Table 2. Statistically significant Spearman's rank correlation between intraepidermal nerve fiber pathology, clinical and neurological deficits, quantitative sensory testing and autonomic deficits in PD patients.
Discussion
This study demonstrates evidence of increased degeneration and impaired regeneration of IENF in patients with PD (4). The quantification of IENF was originally pioneered by Kennedy, who used PGP 9.5 staining to demonstrate loss of nociceptive and autonomic nerve fibers in the epidermal and subepidermal plexus (30, 31). Subsequently, IENF loss was standardized by manually counting IENF crossing the epidermo-dermal junction per length of epidermis (13) and global spatial sampling has been applied to estimate nerve length (32). In addition to demonstrating a reduction in IENFD in patients with PD, we now show a reduction in MAL, providing further evidence of IENF degeneration. MAL was originally introduced by Pittenger et al. (33) and termed mean dendritic length (MDL) and we have previously shown a significantly lower MDL in subjects with impaired glucose tolerance (34).
There is considerable debate regarding the utility of neuronal growth-associated protein, GAP-43 as a marker for nerve regeneration (18, 20, 32). However, it appears to be essential in regrowth of nerve fibers, be it post-traumatic or disease-related and also plays an important role in coordinating axonal outgrowth (18, 35). We have recently used GAP-43 staining in skin biopsies in patients with sarcoidosis related neuropathy and showed that GAP-43+ nerves increased in a dose dependent manner after a relatively short period of pharmacological intervention to stimulate nerve regeneration (21). Furthermore, Bonhof et al. have recently found a higher proportion of GAP-43+ compared to PGP9.5+ nerves in the skin of patients with diabetic neuropathy (20) and attributed this to differences in their respective rates of axonal transport.
In the present study, we show a reduction in both IETNFL/length and IETNFL/area, indicating impaired IENF regeneration in patients with moderate PD. Anand et al. have also used GAP-43 staining to show increased sensory or regenerating subepidermal nerve fibers in non-freezing cold injury (36).
The current data support our recent findings demonstrating an association between reduced IENFD and corneal innervation with autonomic dysfunction (15) and altered perception of affective touch (16) in patients with PD. We now extend these observations by showing that IETNFL and MAL are also related to autonomic deficits in PD patients. Small nerve fiber degeneration has been attributed to disruption in multiple metabolic and signaling pathways in the skin of patients with PD (37). Recent studies also show widespread small fiber pathology with a reduction in corneal nerve fiber density in patients with PD (38, 39). In a previous study we showed increased corneal nerve branch density and length, indicating increased small fiber regeneration, however, we could only show small fiber degeneration in the skin as we only evaluated IENFD (15). In the present study, we have used GAP-43 staining to assess small fiber regeneration in the skin and show a reduction in skin IETNFL, which indicates reduced intraepidermal nerve fiber regeneration.
We also demonstrate increased painful neuropathic symptoms, altered thermal thresholds and heart rate variability in addition to the typical motor deficits of PD and relate them to measures of IENF degeneration and regeneration. Surprisingly, IENFD in particular correlated with disease duration, cumulative levodopa dose and severity of motor disability as well as autonomic dysfunction, but not vibration or thermal thresholds. We have previously demonstrated significant associations between IENFD and motor deficits as well as severity of neuropathy and between corneal nerve loss with motor deficits and autonomic symptoms and deficits (15). However, we did not demonstrate any correlation between disease duration/motor severity and markers of small fiber regeneration. A longitudinal study is required to investigate changes in regeneration over time in PD.
A limitation of our study is the small number of PD patients who underwent skin biopsy, however this was comparable to previous studies. Nevertheless, we have expanded the morphological assessment of IENF pathology to demonstrate a reduction not only in IENFD, but also MAL, which represents a marker of more distal small fiber degeneration in patients with PD. In addition we have utilized GAP-43 to assess altered nerve fiber regeneration in patients with PD. These morphological measures of increased degeneration and impaired nerve regeneration correlate with clinical symptoms and deficits in PD.
Conclusion
This is a small but detailed study, which shows that increased intraepidermal nerve fiber degeneration and reduced regeneration is associated with neurological deficits in patients with PD.
Author Contributions
MJ analyzed skin biopsies. CK, DG, RM, and MS organized patient referrals and coordination of assessments. LK-I performed clinical assessments. SJ performed QST and AFT. AM performed the nerve conduction studies. AA performed statistical assessments. All authors contributed to drafting the manuscript and approved the final manuscript.
Funding
This study was funded by Parkinson's UK (Ref.K-1301).
Conflict of Interest Statement
MJ, AA, LK-I, DG, SJ, and AM declared that the research was conducted in the absence of any commercial or financial relationships that could be construed as a potential conflict of interest. CK received honoraria for educational meetings from UCB, Ipsen and Allergen; travel funding and advisory board from Britannia Pharmaceuticals. MS received honoraria for educational meetings from Medtronic and Bial. RM received honoraria for educational meetings from Pfizer and Novo Nordisk.
Acknowledgments
We would like to thank the Manchester Wellcome Trust Clinical Research Facility for providing the facilities to conduct this research. We would also like to thank Amanda Woodall for her help with data collection and Wendy Jones for skillful technical assistance.
References
1. Nolano M, Provitera V, Estraneo A, Selim MM, Caporaso G, Stancanelli A, et al. Sensory deficit in Parkinson's disease: evidence of a cutaneous denervation. Brain (2008) 131(Pt 7):1903–11. doi: 10.1093/brain/awn102
2. Toth C, Breithaupt K, Ge S, Duan Y, Terris JM, Thiessen A, et al. Levodopa, methylmalonic acid, and neuropathy in idiopathic Parkinson disease. Ann Neurol. (2010) 68:28–36. doi: 10.1002/ana.22021
3. Wang N, Gibbons CH, Lafo J, Freeman R. alpha-Synuclein in cutaneous autonomic nerves. Neurology (2013) 81:1604–10. doi: 10.1212/WNL.0b013e3182a9f449
4. Zis P, Grunewald RA, Chaudhuri RK, Hadjivassiliou M. Peripheral neuropathy in idiopathic Parkinson's disease: a systematic review. J Neurol Sci. (2017) 378:204–9. doi: 10.1016/j.jns.2017.05.023
5. Siepmann T, Frenz E, Penzlin AI, Goelz S, Zago W, Friehs I, et al. Pilomotor function is impaired in patients with Parkinson's disease: a study of the adrenergic axon-reflex response and autonomic functions. Parkinsonism Relat Disord. (2016) 31:129–34. doi: 10.1016/j.parkreldis.2016.08.001
6. Nolano M, Provitera V, Manganelli F, Iodice R, Stancanelli A, Caporaso G, et al. Loss of cutaneous large and small fibers in naive and l-dopa-treated PD patients. Neurology (2017) 89:776–84. doi: 10.1212/WNL.0000000000004274
7. Schneider SA, Boettner M, Alexoudi A, Zorenkov D, Deuschl G, Wedel T. Can we use peripheral tissue biopsies to diagnose Parkinson's disease? A review of the literature. Eur J Neurol. (2016) 23:247–61. doi: 10.1111/ene.12753
8. Zange L, Noack C, Hahn K, Stenzel W, Lipp A. Phosphorylated alpha-synuclein in skin nerve fibres differentiates Parkinson's disease from multiple system atrophy. Brain (2015) 138(Pt 8):2310–21. doi: 10.1093/brain/awv138
9. Navarro-Otano J, Casanova-Molla J, Morales M, Valls-Sole J, Tolosa E. Cutaneous autonomic denervation in Parkinson's disease. J Neural Transm (Vienna) (2015) 122:1149–55. doi: 10.1007/s00702-014-1355-3
10. Gibbons CH, Wang N, Freeman R. Cutaneous alpha-synuclein from paraffin embedded autopsy specimens in Parkinson's disease. J Parkinsons Dis. (2017). doi: 10.3233/JPD-171088
11. Donadio V, Incensi A, Rizzo G, Scaglione C, Capellari S, Fileccia E, et al. Spine topographical distribution of skin alpha-synuclein deposits in idiopathic Parkinson disease. J Neuropathol Exp Neurol. (2017) 76:384–9. doi: 10.1093/jnen/nlx021
12. Lee JM, Derkinderen P, Kordower JH, Freeman R, Munoz DG, Kremer T, et al. The search for a peripheral biopsy indicator of alpha-synuclein pathology for Parkinson disease. J Neuropathol Exp Neurol. (2017) 76:2–15. doi: 10.1093/jnen/nlw103
13. Lauria G, Hsieh ST, Johansson O, Kennedy WR, Leger JM, Mellgren SI, et al. European Federation of Neurological Societies/Peripheral Nerve Society Guideline on the use of skin biopsy in the diagnosis of small fiber neuropathy. Report of a joint task force of the European Federation of Neurological Societies and the Peripheral Nerve Society. Eur J Neurol. (2010) 17:903–12, e44–9. doi: 10.1111/j.1468-1331.2010.03023.x
14. Lin CH, Chao CC, Wu SW, Hsieh PC, Feng FP, Lin YH, et al. Pathophysiology of small-fiber sensory system in Parkinson's disease: skin innervation and contact heat evoked potential. Medicine (Baltimore) (2016) 95:e3058. doi: 10.1097/MD.0000000000003058
15. Kass-Iliyya L, Javed S, Gosal D, Kobylecki C, Marshall A, Petropoulos IN, et al. Small fiber neuropathy in Parkinson's disease: a clinical, pathological and corneal confocal microscopy study. Parkinsonism Relat Disord. (2015) 21:1454–60. doi: 10.1016/j.parkreldis.2015.10.019
16. Kass-Iliyya L, Leung M, Marshall A, Trotter P, Kobylecki C, Walker S, et al. The perception of affective touch in Parkinson's disease and its relation to small fibre neuropathy. Eur J Neurosci. (2017) 45:232–7. doi: 10.1111/ejn.13481
17. Nolano M, Provitera V, Stancanelli A, Saltalamacchia AM, Caporaso G, Lullo F, et al. Small fiber pathology parallels disease progression in Parkinson disease: a longitudinal study. Acta Neuropathol. (2018) 136:501–03. doi: 10.1007/s00401-018-1876-1
18. Denny JB. Molecular mechanisms, biological actions, and neuropharmacology of the growth-associated protein GAP-43. Curr Neuropharmacol. (2006) 4:293–304. doi: 10.2174/157015906778520782
19. Holahan M, Routtenberg A. The protein kinase C phosphorylation site on GAP-43 differentially regulates information storage. Hippocampus (2008) 18:1099–102. doi: 10.1002/hipo.20486
20. Bonhof GJ, Strom A, Puttgen S, Ringel B, Bruggemann J, Bodis K, et al. Patterns of cutaneous nerve fibre loss and regeneration in type 2 diabetes with painful and painless polyneuropathy. Diabetologia (2017) 60:2495–503. doi: 10.1007/s00125-017-4438-5
21. Culver DA, Dahan A, Bajorunas D, Jeziorska M, van Velzen M, Aarts L, et al. Cibinetide improves corneal nerve fiber abundance in patients with sarcoidosis-associated small nerve fiber loss and neuropathic pain. Invest Ophthalmol Vis Sci. (2017) 58:BIO52–60. doi: 10.1167/iovs.16-21291
22. Fahn S, ELton RL. Unified Parkinson's disease rating scale. In: Fahn SMC, Goldstein M, Calne DB editors. Recent Developments in Parkinson's Disease. Florham Park, NJ: Macmillan Healthcare Information (1987). p. 153–63.
23. Chaudhuri KR, Martinez-Martin P, Brown RG, Sethi K, Stocchi F, Odin P, et al. The metric properties of a novel non-motor symptoms scale for Parkinson's disease: results from an international pilot study. Mov Disord. (2007) 22:1901–11. doi: 10.1002/mds.21596
24. Chaudhuri KR, Rizos A, Trenkwalder C, Rascol O, Pal S, Martino D, et al. King's Parkinson's disease pain scale, the first scale for pain in PD: an international validation. Mov Disord. (2015) 30:1623–31. doi: 10.1002/mds.26270
25. Dyck PJ, Karnes J, O'Brien PC, Swanson CJ. Neuropathy symptom profile in health, motor neuron disease, diabetic neuropathy, and amyloidosis. Neurology (1986) 36:1300–8.
26. Meijer JW, Smit AJ, Sonderen EV, Groothoff JW, Eisma WH, Links TP. Symptom scoring systems to diagnose distal polyneuropathy in diabetes: the diabetic neuropathy symptom score. Diabet Med. (2002) 19:962–5. doi: 10.1046/j.1464-5491.2002.00819.x
28. Visser M, van Rooden SM, Verbaan D, Marinus J, Stiggelbout AM, van Hilten JJ. A comprehensive model of health-related quality of life in Parkinson's disease. J Neurol. (2008) 255:1580–7. doi: 10.1007/s00415-008-0994-4
29. Young MJ, Boulton AJ, MacLeod AF, Williams DR, Sonksen PH. A multicentre study of the prevalence of diabetic peripheral neuropathy in the United Kingdom hospital clinic population. Diabetologia (1993) 36:150–4.
30. Kennedy WR, Wendelschafer-Crabb G. Utility of skin biopsy in diabetic neuropathy. Semin Neurol. (1996) 16:163–71. doi: 10.1055/s-2008-1040972
31. Kennedy WR, Wendelschafer-Crabb G, Brelje TC. Innervation and vasculature of human sweat glands: an immunohistochemistry-laser scanning confocal fluorescence microscopy study. J Neurosci. (1994) 14(11 Pt 2):6825–33.
32. Karlsson P, Moller AT, Jensen TS, Nyengaard JR. Epidermal nerve fiber length density estimation using global spatial sampling in healthy subjects and neuropathy patients. J Neuropathol Exp Neurol. (2013) 72:186–93. doi: 10.1097/NEN.0b013e318284e849
33. Pittenger GL, Ray M, Burcus NI, McNulty P, Basta B, Vinik AI. Intraepidermal nerve fibers are indicators of small-fiber neuropathy in both diabetic and nondiabetic patients. Diabetes Care (2004) 27:1974–9. doi: 10.2337/diacare.27.8.1974
34. Azmi S, Ferdousi M, Petropoulos IN, Ponirakis G, Alam U, Fadavi H, et al. Corneal Confocal Microscopy Identifies Small-Fiber Neuropathy in Subjects With Impaired Glucose Tolerance Who Develop Type 2 Diabetes. Diabetes Care (2015) 38(8):1502-8. Epub 2015/04/17. doi: 10.2337/dc14-2733
35. Holahan MR. A shift from a pivotal to supporting role for the growth-associated protein (GAP-43) in the coordination of axonal structural and functional plasticity. Front Cell Neurosci. (2017) 11:266. doi: 10.3389/fncel.2017.00266
36. Anand P, Privitera R, Yiangou Y, Donatien P, Birch R, Misra P. Trench foot or non-freezing cold injury as a painful vaso-neuropathy: clinical and skin biopsy assessments. Front Neurol. (2017) 8:514. doi: 10.3389/fneur.2017.00514
37. Planken A, Kurvits L, Reimann E, Kadastik-Eerme L, Kingo K, Koks S, et al. Looking beyond the brain to improve the pathogenic understanding of Parkinson's disease: implications of whole transcriptome profiling of Patients' skin. BMC Neurol. (2017) 17:6. doi: 10.1186/s12883-016-0784-z
38. Misra SL, Kersten HM, Roxburgh RH, Danesh-Meyer HV, McGhee CN. Corneal nerve microstructure in Parkinson's disease. J Clin Neurosci. (2017) 39:53–8. doi: 10.1016/j.jocn.2017.02.033
Keywords: Parkinson's disease, skin biopsy, small fiber neuropathy, intraepidermal nerve, motor, autonomic
Citation: Jeziorska M, Atkinson A, Kass-Iliyya L, Javed S, Kobylecki C, Gosal D, Marshall A, Silverdale M and Malik RA (2019) Increased Intraepidermal Nerve Fiber Degeneration and Impaired Regeneration Relate to Symptoms and Deficits in Parkinson's Disease. Front. Neurol. 10:111. doi: 10.3389/fneur.2019.00111
Received: 23 August 2018; Accepted: 28 January 2019;
Published: 14 February 2019.
Edited by:
Alain Kaelin-Lang, Neurocenter of Southern Switzerland (NSI), SwitzerlandReviewed by:
Giorgia Melli, Neurocenter of Southern Switzerland (NSI), SwitzerlandAntonella Conte, Sapienza University of Rome, Italy
Copyright © 2019 Jeziorska, Atkinson, Kass-Iliyya, Javed, Kobylecki, Gosal, Marshall, Silverdale and Malik. This is an open-access article distributed under the terms of the Creative Commons Attribution License (CC BY). The use, distribution or reproduction in other forums is permitted, provided the original author(s) and the copyright owner(s) are credited and that the original publication in this journal is cited, in accordance with accepted academic practice. No use, distribution or reproduction is permitted which does not comply with these terms.
*Correspondence: Rayaz A. Malik, cmFtMjQ1QHFhdGFyLW1lZC5jb3JuZWxsLmVkdQ==;cmF5YXouYS5tYWxpa0BtYW5jaGVzdGVyLmFjLnVr