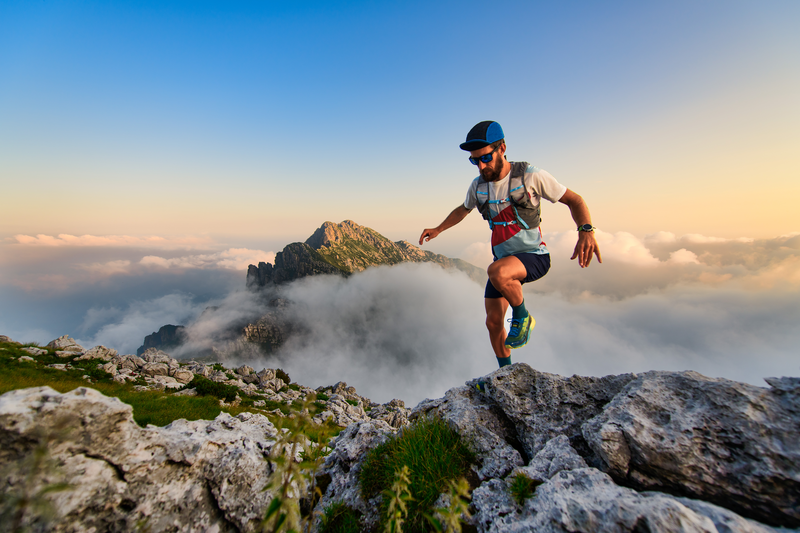
95% of researchers rate our articles as excellent or good
Learn more about the work of our research integrity team to safeguard the quality of each article we publish.
Find out more
REVIEW article
Front. Neurol. , 03 December 2018
Sec. Multiple Sclerosis and Neuroimmunology
Volume 9 - 2018 | https://doi.org/10.3389/fneur.2018.01048
This article is part of the Research Topic Role of Coagulation Pathways in Neurological Diseases View all 13 articles
Lyme borreliosis (LB) is the most common tick-borne disease caused by the spirochete Borrelia burgdorferi in North America and Borrelia afzelii or Borrelia garinii in Europe and Asia, respectively. The infection affects multiple organ systems, including the skin, joints, and the nervous system. Lyme neuroborreliosis (LNB) is the most dangerous manifestation of Lyme disease, occurring in 10–15% of infected individuals. During the course of the infection, bacteria migrate through the host tissues altering the coagulation and fibrinolysis pathways and the immune response, reaching the central nervous system (CNS) within 2 weeks after the bite of an infected tick. The early treatment with oral antimicrobials is effective in the majority of patients with LNB. Nevertheless, persistent forms of LNB are relatively common, despite targeted antibiotic therapy. It has been observed that the antibiotic resistance and the reoccurrence of Lyme disease are associated with biofilm-like aggregates in B. burgdorferi, B. afzelii, and B. garinii, both in vitro and in vivo, allowing Borrelia spp. to resist to adverse environmental conditions. Indeed, the increased tolerance to antibiotics described in the persisting forms of Borrelia spp., is strongly reminiscent of biofilm growing bacteria, suggesting a possible role of biofilm aggregates in the development of the different manifestations of Lyme disease including LNB.
Lyme borreliosis (LB) is the most prevalent vector-borne disease (1) caused by the spirochete Borrelia burgdorferi. This Gram-negative bacterium is an obligate pathogen, transmitted to different hosts by ticks in the genus Ixodes. LB is frequently reported in North America, Europe, in different parts of Asia, including Mongolia and China as well as in Australia and in Africa (2–4).
LB affects multiple organ systems, including the skin, eyes, joints, muscles, cardiac, and nervous system, presenting, at different stages, with a variety of clinical manifestations (5). Incubation varies from 3 to 32 days, after which a characteristic skin rash, known as erythema migrans, appears in association with fever, headache, malaise, and myalgias (stage 1) (6). After several weeks to months, in 8–15% of patients can be reported the presence of neurologic and cardiac abnormalities (stage 2). Within few weeks, in untreated patients or in case of delayed antibiotic treatment, the infection can disseminate leading to systemic inflammation (7, 8). In the last phase of LB (stage 3), patients may experience chronic monoarticular or oligoarticular arthritis, involving large joints, particularly the knee (9–11).
The most severe manifestations of LB, is Lyme neuroborreliosis (LNB), reported in 10–15% of individuals with localized erythema migrans (12, 13). The activation of the inflammatory response in LNB contributes to the pathogenesis of a broad spectrum of neurologic disorders. Different geographical distribution of B. burgdorferi species correlates with specific manifestation of LNB, which is more frequent in Europe than in the North America (6). The most common symptoms of LNB in Europe are painful meningoradiculitis known as Bannwarth syndrome and facial nerve palsy (14). Symptoms involving the central nervous system (CNS) are less common and their exact incidence is not known. B. burgdorferi infection of the CNS cause mainly encephalitis, segmental myelitis, cranial neuritis, radiculoneuritis, vasculitis, and intracranial hypertension (13, 15, 16). The clinical manifestation of the LNB may include ataxia, paraparesis, sphincter dysfunction, Parkinson-like symptoms, confusion and cognitive impairment (17, 18). Ischemic stroke is the most frequent cerebrovascular manifestation of LNB presenting in 76% of cases, followed by transient ischemic attack (11%) (19).
LNB is, in many cases, responsive to appropriate antimicrobial therapy and the clinical improvement sustained by the antibiotic treatment provide further evidence for the direct contribution of B. burgdorferi in disease pathogenesis. However, the chronic persistence, the frequent reoccurrence of LNB and the ability of B. burgdorferi to tolerate multiple cycles of antibiotic treatment is strongly suggestive for the formation of biofilm or biofilm-like protective structure (20–23). Indeed, different studies have shown that B. burgdorferi can switch from a motile to a stationary status, in which the cells are embedded within a biofilm matrix (22). B. burgdorferi biofilms have been observed both in vitro and in human infected skin tissues (22, 23). These structures express different mucopolysaccharides, particularly alginate, extracellular DNA and calcium, which are all typical markers of biofilm (22). The presence of biofilm may explain the low rate of Borrelia detection in the blood of infected patients as well as the ability of the spirochetes to evade the host immune system and resist the antibiotic therapy (21, 24–27).
This review investigates the differences in the epidemiology and clinical manifestations of LNB with particular emphasis on the pathogenetic role of B. burgdorferi biofilm in tissue adhesion, colonization and survival.
The present review focuses on a systematic review of the literature to identify all published articles of LNB using online databases (PubMed, Web of Science, and Google Scholar). The reference list was updated in September 2018. There were no language restrictions; The search terms were “Borrelia,” “B. burgdorferi,” “Borrelia biofilm,” “Lyme disease,” “neuroborreliosis,” “LNB,” “borreliosis.” We reviewed titles, abstracts, case reports, and full articles to assess their relationship with the research criteria. References reported in each article were also reviewed to identify additional study not found by initial search terms.
LB is increasing worldwide with ~300,000 new cases annually in the United States and 85,000 cases in Europe each year (28–30). Incidence of human LB in endemic areas of the United States ranges from 10 to 100 per 100,000 population with a peak of 134 per 100,000, reported in Connecticut in 2002 (4, 31, 32). The number of documented LB cases and the geographic distribution has expanded during the last two decades, from the Northeastern and North Central United States (4). LB is widespread also in Europe and the incidence for LB ranges from 20 to 80 per 100,000 in the Czech Republic, Germany, Latvia, the Netherlands, Poland, Switzerland, and Sweden, peaking to more than 100 per 100,000 in Austria, Estonia, Lithuania, and Slovenia (4, 29, 33, 34). Incidence of LB decreases southward, in Spain, France, Italy, and Greece with approximately 1 case per 100,000 (4, 29).
B. burgdorferi sensu stricto, B. garinii, and B. afzelii, are primarily responsible for human LB in different geographical regions presenting specific symptoms (34–38).
The genomes of Borrelia species consist of a set of circular and linear plasmids and a linear chromosome of ~900 kb ending with DNA sequences regulated by breakage and reunion reactions (39, 40). Different isolates show a variable number of plasmids depending on the species and affected by frequent reorganization (41–47). B. burgdorferi B31 strain harbors 10 circulars and 12 linear plasmids while B. afzelii B023 and B. garinii CIP 103362 have 6 linear and 2 circular plasmids and 4 linear and 1 circular plasmids in Fraser et al. (41), Casjens et al. (42), and Bontemps-Gallo et al. (47).
Most of the essential genes involved in metabolism or regulation are located in the linear chromosome while only a subset of genes encoding proteins required for growth and specific virulence factors are located on plasmids (41, 48–50).
B. burgdorferi sensu stricto is the predominant causative agent of LB, Lyme arthritis, and also LNB in the United States (51). Nevertheless, consistent differences in the ability to induce LB exist between B. burgdorferi sensu stricto subtypes suggesting that, neurotropism is an ability present only in a restricted subtype of Borrelia (52, 53). Different genotypes of B. burgdorferi sensu stricto diverge ecologically and epidemiologically, suggesting that genotype classification is relevant to understanding the basic biology of the spirochete (54–56). Studies conducted in endemic areas of the United States revealed that patients with disseminated infection were more likely infected by the RST1 strains of B. burgdorferi than with RST3 strains (57, 58). Moreover, dissemination of B. burgdorferi to blood or cerebrospinal fluid (CSF) was mostly related to ospC genotypes A, B, I, or K (58–62).
The distribution and relative frequency of infection by the different genospecies of Borrelia sensu latu vary across European regions. B. burgdorferi sensu lato comprises 20 different genospecies and this diversity correlates with the large variability in the clinical manifestations observed in LB (4, 13, 63). In the northern and eastern Europe B. afzelii is the most prevalent species, whereas in Western European countries B. garinii is the most common pathogen (4, 29). B. afzelii, B. garini, and the recently identified species B. bavariensis are major cause of LB and LNB in Europe (52, 64–68). The heterogeneity among B. burgdorferi sensu lato genospecies is linked to different geographical areas, which, in turn, correlates with the different clinical expression of human LB (69). For instance, B. afzelii induces prevalently skin infections, whereas B. garinii is in most cases neurotropic (5, 69). Other species, such as B. lusitaniae or B. valaisiana, have only occasionally been associated with human disease (70–72). In endemic areas of Europe was proposed that the variety of symptoms observed in children and adults with LNB correlated with the B. burgdorferi sensu lato genotype (73–75). Individuals with erythema migrans caused by B. afzelii and B. garinii showed distinct epidemiological and clinical characteristics. Indeed, erythema migrans caused by B. garinii were located prevalently on the trunk and less often on extremities, had shorter incubation and faster evolution, leading to frequent systemic symptoms, abnormal liver function test results than individuals with erythema migrans caused by B. afzelii (76, 77).
The genetic diversity observed in B. burgdorferi, at both inter- and intra-species level, is probably the reason for the multiple epidemiological and clinical presentation of these bacteria in humans (62, 78–80). A major role in maintaining the intraspecific genetic diversity of B. burgdorferi is the adaptation to multiple vertebrate hosts, which act as ecological niches for different genotypes (81, 82). Consequently, variations in the vertebrate host fitness may result in changes in the abundance of the more pathogenic species (83–85).
Colonization, dissemination and invasion of the tick vector and mammalian host by B. burgdorferi requires a complex temporal and spatial regulation of borrelial genes to adapt to environmental challenges. Transition of B. burgdorferi from the tick midgut to the hemolymph during a blood meal is an important step for bacterial diffusion through the salivary glands to a mammalian host (86). Borrelia possesses a sophisticated mechanism of gene regulation based on the two-component pathways HK1/Rrp1 and Rrp2-RpoN-RpoS, which regulate metabolism, antigenic variation, chemotaxis, and adhesion in a tissue- and temporal-specific manner in both the tick vector and mammalian host (48, 87). During the blood uptake B. burgdorferi expresses the outer surface proteins (Osp) A and B. These proteins mediate the adherence to the tick's gut by the binding to the tick receptor of OspA (TROSPA), thus facilitating the subsequent transmission into the mammalian host (88–90). Infection of the mammalian host requires the migration of the spirochetes from the midgut to the salivary glands of the tick. After the blood uptake into the midgut of the tick, the production of OspA and OspB decrease while ospC is expressed in conjunction with many other genes controlled by the RpoN, RpoS, and Rrp2 system (90–96).
OspC is required to establish the early phase of B. burgdorferi infection in mammalian host and to promote evasion from the innate immune defenses (96–98). Different studies revealed that the OspC mutant strains are unable to establish infection in mice, suggesting a protective role of this protein against host innate defenses (96, 99–104). Nevertheless, to escape from the host immune system, the expression of ospC decreases within 2–3 weeks after infection in response to anti-OspC antibodies in mice (105, 106). In addition to OspC, B. burgdorferi hides other important immunogenic surface proteins (107). In particular, OspA, which stimulates neutrophils and a strong inflammatory response mediated by interleukin (IL)-1β, tumor necrosis factor (TNF)-α, and IL-6, is highly expressed in the tick gut but it is rapidly downregulated in the host (108–112). OspA-positive strains of B. burgdorferi penetrate the host, but are unable to establish an infection (113). Similarly, Borrelia strains isolated from mice 4 days after infection, were found to be OspA negative suggesting that this protein is not expressed in the early phase of the host infection (114, 115).
The expression of different proteins, including OspC, ErpP, ErpA, ErpC, and enolase is required to readily immobilize host plasminogen on spirochetal surface, facilitating efficient dissemination (116–118). Plasminogen is a glycoprotein produced by the liver and abundant in the plasma and in certain tissues (119). Conversion of plasminogen to active plasmin is promoted by proteolytic activation induced by either tissue-type plasminogen activator (tPA) and/or urokinase-type plasminogen activator urokinase (uPA). Plasmin is responsible for intravascular fibrinolysis and contributes to numerous physiological and pathological processes, including tissue remodeling, cell migration, thrombolysis, wound healing, and cancer progression (120, 121). Invasive forms of B. burgdorferi are known to expresses multiple plasminogen-binding surface proteins that likely assist pathogen dissemination through host tissues (120, 122). Enolase is an integral enzyme of the glycolysis and gluconeogenesis pathways, and a multifunctional protein found in both prokaryote and eukaryotes (123). In eukaryotic cells, surface enolase acts as a plasminogen receptor in certain tumor cells (123, 124). Similarly, this enzyme is also localized on the cell surface of different microorganisms including B. burgdorferi (118, 125–127). The surface-localized enolase acts as a plasminogen receptor contributing to spirochetal survival in feeding ticks (118). Although dispensable for infection, plasminogen is required for dissemination in ticks, and its absence is associated with a decreased spirochetemia in plasminogen-deficient mice (128). Surface-associated plasmin on B. burgdorferi degrade fibronectin, which is an important component of the ECM, laminin and vitronectin (129, 130). B. burgdorferi also induces the release of host matrix metalloproteases 9 (MMP-9) and MMP-1, and plasmin-coated B. burgdorferi activates pro-MMP-9, leading to degradation of basement membranes (131).
B. burgdorferi exhibits a specific affinity for the CNS as demonstrated by the presence of spirochetes in the human CSF within 14–18 days after the tick bite (18, 132, 133). From the initial site of entry in the skin the spirochetes can reach the CNS either through the bloodstream or, alternatively, by the peripheral nerves (114).
Hematogenous dissemination from the tick bite on the skin to the CNS is a key pathogenetic event in LNB (114). However, it has been proposed that, at least for B. garinii which is mostly responsible for LNB in Europe, spirochetes can pass along the peripheral nerves (114). To penetrate the brain, spirochetes must first cross the blood-brain barrier reaching the brain microvascular endothelium and astrocytes (134). This barrier is composed by the brain microvascular endothelial cells (BMEC), astrocytes, basement membrane, pericytes, and neurons. The BMEC are firmly held together by tight junctions, presenting with a reduced transcytotic vesicles and an absence of fenestrae. All of these elements contribute to reduce the transport of solutes defending the brain from most pathogens or toxic agents (135, 136). Invasion of the blood-brain barrier by B. burgdorferi is still a matter of debate. Some studies suggest that Borrelia uses a paracellular route of translocation (134, 137), although other evidences suggest a possible transcellular passage of the spirochetes (138). Neurotropic B. burgdorferi strains showed the activation of the host plasminogen system, MMPs, and calcium signaling pathway to facilitate an efficient translocation through the blood-brain barrier (120, 134, 139). Compelling evidence suggest that spirochetes can adhere to murine neural and glial cell lines, primary neural cells, and primary rat brain cultures (140). In addition, in vitro studies show that B. burgdorferi can promote an intracellular invasion of human fibroblast, umbilical vein endothelial, synovial, neuronal, and glial cells without affecting the cell viability. This suggests that spirochetal cellular invasion may provide a mechanism for immune evasion and disease pathogenesis (140–142).
A common clinical and pathological manifestations of LNB in Europe is painful lymphocytic meningoradiculitis also known as Bannwarth syndrome, frequently accompanied by CSF signs of inflammation (13, 14, 143). The early manifestation of LNB generally appears within 2–18 weeks after infection (13, 143). The clinical description of painful meningoradiculitis was first reported in 1922, but the etiology remained unknown till the isolation of spirochetes by Burgdorfer in 1982 and the isolation in 1984 of spirochetes from the CSF of a patient with Bannwarth syndrome (144–146).
In addition to Bannwarth syndrome, other important neurological symptoms of the early stages of LNB include meningitis, meningeal perivascular, and vasculitic lymphoplasmocytic infiltrates, neuritis, and in rare acute LNB cases encephalitis and myelitis (143, 147). CNS vasculitis are rare in LNB, affecting mainly the large/medium-sized vessels and are associated with ischemia and stroke (19, 148). However, in European patients with LNB the mortality rate is comparable to that of the general population. Nevertheless, LNB is associated with increased risk of hematological and non-melanoma skin cancers (149).
Treatment with conventional intravenous antibiotic therapy, leads, in most cases, to a gradual improvement of the symptoms after several weeks or months, accompanied frequently by a normalization of CSF findings (150, 151). However, <2% of patients treated for LNB experience late neurological manifestations that persist months or years after B. burgdorferi infection (14, 143). The clinical symptoms of late LNB include several neurological and psychiatric symptoms such as meningoradiculitis, encephalomyelitis, chronic meningitis, and cerebral vasculitis (152–154). The presence of depressive states was described in 26–66% of patients with late LNB together with psychosis, schizophrenia, hallucinations, paranoia, anorexia nervosa, obsessive-compulsive disorder, and dementia (155–163). A frequent manifestation appearing in the late stage of LNB is the chronic vascular damage, clinically characterized by recurrent stroke or transient ischemic attacks (153, 154). Other distinctive findings in patients with late LNB are inflammatory CSF changes (CSF pleocytosis and elevated total protein content) and the presence of specific B. burgdorferi intrathecal antibody (150, 151).
The clinical outcome of antibiotic treatment of either early or late manifestations of LNB may include progression to a chronic form characterized by nonspecific and persistent fatigue, arthralgia, myalgia, musculoskeletal, and cognitive symptoms. This condition, frequently defined as posttreatment Lyme disease syndrome (PTLDS), can be intermittent or persistent, lasting for at least six or more months after completion of antibiotic treatment (143, 164).
Specific diagnostic criteria for PTLDS proposed by the Infectious Disease Society of America relies on the objective proof of previous LB, the presence of subjective symptoms that compromise function in daily life, and the absence of clinical evidences for another underlying illness (7). However, those criteria have rarely used in clinical studies, contributing to confusion and controversy about the clinical significance of PTLDS syndrome (7). The frequency of PTLDS among patients with LB varies largely, ranging from 0 to 50%, depending upon differences in study design and enrollment criteria (165, 166). A long-term follow-up study of patients with early presentation of erythema migrans and treated with antibiotics at the time of diagnosis showed an excellent rate of remission, with only 4% of patients remaining symptomatic during follow-up evaluation (167). Conversely, other trials reported rates of PTLDS ranging from approximatively 10–20% (168). Nevertheless, in the community medical practice, where prompt LB diagnosis and treatment are not common, PTLDS rates may reach 50% (169, 170). Notably, xenodiagnoses demonstrated the presence of B. burgdorferi DNA in a patient with PTLDS, despite repeated cycles of antibiotic treatments (171). B. burgdorferi DNA was detected in mice after prolonged (up to 12 months) treatment with antibiotics despite the persistence of non-cultivable bacteria. Moreover, the study revealed B. burgdorferi DNA and the presence of RNA transcripts of multiple spirochetal genes in host tissues (172). These findings suggest that B. burgdorferi persist within the host indicating that the immune system and antimicrobial treatment may not be effective at eradicating B. burgdorferi. This may contribute to antibiotic-refractory arthritis, as observed in a murine model in which spirochetal antigens, but not infectious spirochetes, were recovered near cartilage for extended periods after LB therapy (173).
According to the guidelines of the European Federation of Neurological Sciences and the Infectious Diseases Society of America, treatment with beta-lactams antibiotics, like ceftriaxone, penicillin, or cefotaxime, or oral doxycycline for 14–21 days is recommended for the treatment of LNB (7, 25, 174). Intravenous administration of ceftriaxone is often recommended for the treatment of Lyme meningitis. Oral treatment with doxycycline demonstrated to be as effective as ceftriaxone for Lyme meningitis in adults in Europe, although not recommended as first-line therapy in the United States (175). Nevertheless, four NIH-sponsored trials aimed at assessing the administration of antibiotic treatment in patients with persistent unexplained symptoms despite previous antimicrobial treatment of LB indicated that the new treatment cycle provides little if any clinical benefit (176). A randomized, double-blinded, placebo-controlled trial conducted in Europe, in patients with persistent symptoms attributed to Lyme disease showed that longer-term antibiotic treatment did not have a better outcome as compared with shorter-term treatment (177).
B. burgdorferi can switch from motile cellular forms into several defensive morphological forms such as round bodies, stationary phase, persister cells, and biofilm (23, 24, 178–182). Transition between different morphologies represents an adaptation strategy to survive in unfavorable environmental conditions, including pH variations, nutrient starvation, host immune system attacks, or the presence of antimicrobial agents (21, 23, 24, 172, 179, 181).
Notably, within the biofilm, bacteria are physically joined together producing a matrix, characterized by the presence of an extracellular polymeric substance (EPS) composed by polysaccharides, proteins, and extracellular DNA (183). Bacterial biofilms are intrinsically more resistant to environmental agents and antimicrobials than the corresponding planktonic counterpart and this can lead to chronic and recurrent infections (184–186). In vitro and in vivo studies revealed that both B. burgdorferi sensu stricto and sensu lato (B. afzelii and B. garinii) aggregates, but not free-floating spirochetes, present typical markers found in the EPS of other pathogenic bacteria such as sulfated mucins, non-sulfated mucins (mainly alginate), extracellular DNA and calcium (22, 23, 187). B. burgdorferi biofilm is also characterized by the presence of a distinctive architecture with channel-like elements that in mature biofilm are required for oxygen and nutrient diffusion and waste removal (22, 23, 187). Biofilm formation by B. burgdorferi follows the same evolution described for other bacteria. Initially, individual spirochetes adhere to biotic or abiotic surfaces forming microcolonies, coated by the EPS. From this point, Borrelia aggregates expand undergoing changes in the growth rate, gene expression and structural rearrangements in the EPS components (22, 23). The rapid rearrangements occurring within the biofilm matrix, culminate in a complex three-dimensional structure with common traits observed among Borrelia genera (Figure 1) (23, 189). The existence of biofilm-like structures was further found in human skin biopsies obtained from patients with borrelial lymphocytoma, a common manifestation of LB in Europe, revealing the presence of Borrelia-positive aggregates characterized by mucopolysaccharides, especially alginate (22). Other reports demonstrated that Borrelia DNA is deposited in the Alzheimer brain showing structural similarities between spirochetal aggregates and the profiles of amyloid plaques in patients with Alzheimer disease (188, 190). Nevertheless, the specific contribution of biofilm to borrelial persistent infections remain unclear. Besides, additional in vivo studies showed the presence of Borrelia aggregates in the midguts of naturally-infected nymphs during their blood meal (191). These results strongly suggest that biofilm may contribute to the spirochetal successful transmission to the mammalian host and to the ensuing disease manifestations (191).
Figure 1. (A) Confocal microscopy images of B. burgdorferi B31 strain (American Type Tissue Collection 35210) biofilms. Upper panel show the X-Y planes (top view), while the lower panel show the Z section (side view). The sample was stained with BacLight Live/Dead (Invitrogen Life Technologies, Carlsbad, CA, USA) (188). Representative images of biofilms developed on polystyrene pegs following 72 h incubation at 37°C. Spirochetes were grown in a μ-Slide 8-well system (ibidi, Germany) and cultured in BSK-H medium containing 6% rabbit serum (Sigma-Aldrich). (B) Schematic representation of a B. burgdorferi biofilm. The biofilm matrix produced by spirochetes (green) provides shelter from host defenses, and reduces the diffusion of antibiotics. Persister forms of B. burgdorferi (in blue), exhibit multidrug tolerance and are likely responsible for the recalcitrance of chronic LB. The illustration is adapted from Mind the Graph (https://mindthegraph.com) under the Creative Commons License.
Biofilm production in Borrelia requires the modulation of a complex array of signaling processes which allows spirochetes to communicate with the surrounding environment. The RpoN–RpoS alternative sigma factor and the LuxS quorum-sensing pathways, which are involved in several cellular functions in response to environmental stresses (pH and temperature variations, high osmolarity, oxidative stress, high cell density, nutrient starvation, host infection), participate in biofilm production in B. burgdorferi (22, 192). The RpoN–RpoS pathway, also known as the σ54-σS cascade, regulates adaptive changes in B. burgdorferi during the transition between the tick vector and mammalian host (91, 95). The RpoN–RpoS pathway relies on the activity of RpoN (σ54), which controls the transcription of RpoS (σS) through the binding to a canonical −24/−12 RpoN-type promoter sequence (95, 193). The activation of the σ54-σS cascade, in turn, is modulated by a bacterial enhancer-binding protein (bEBP)/σ54-dependent activator (Rrp2) in concert with BosR (91, 95, 193–198). After the activation, RpoS acts as a global gene regulator controlling the expression of over 100 different genes involved in stress responses, host infection and survival, including biofilm formation (87, 95, 182).
Mutant strains of B. burgdorferi lacking RpoN, RpoS, presented a less compact biofilm with loose and dispersed small aggregates compared to wild-type strains (182). Notably, all mutants expressed Borrelia biofilm markers such as alginate, extracellular DNA, and calcium, although they showed significantly higher sensitivity to low MIC dose of doxycyline (0.1 μg/ml) than the wild-type strain (182). In addition, the quorum sensing (QS) molecules LuxS also contributes to B. burgdorferi biofilm. The QS signaling system is a cell-to-cell communication mechanism, shared by different bacteria, which is based on the release of small molecules called autoinducers (AI) in environment (199). LuxS pathways regulate biofilm formation in various ways according to bacterial species and environmental conditions (183). Specifically, luxS mutant strains of Streptococcus gordonii and Porphyromonas gingivalis, which are two important components of dental plaque, are unable to produce a mixed-species biofilm (200). Besides, in Helicobacter pylori the presence of luxS mutation leads to a more efficient biofilm formation than the wild type whereas a luxS mutant of Streptococcus mutans shows an altered biofilm structure (183, 201–203). B. burgdorferi significantly increases transcription of luxS during transition from ticks to mammalian hosts where it is involved in the regulation of several genes such as vlsE, erpA, and ipLA7 (204–207). luxS mutant strains in stationary cultures of B. burgdorferi showed a higher tendency to form smaller and looser aggregates and a greater sensitivity to antibiotics than the wild-type counterpart (182).
Although antibiotic treatment resolves most of clinical manifestations of LB, persistent forms occur in ~10% of patients after treatment for erythema migrans disease (208, 209). The long-term persistence of symptoms and failure of the antibiotic therapy are reminiscent of chronic biofilm-associated infections. Biofilm aggregates display an enhanced tolerance to various antibiotics, which, conversely, are effective against the planktonic spirochetes and round body forms of B. burgdorferi (210). In particular, doxycycline and amoxicillin were found to effectively kill the motile spirochete forms in vitro, but failed to completely remove B. burgdorferi in biofilms (20, 21, 24, 26, 210–214). High throughput screens of B. burgdorferi identified several promising Food and Drug Administration (FDA)-approved drugs that have excellent anti-persister activity (24, 181, 213, 215). Among them, daptomycin, which is a lipopeptide targeting bacterial cell membranes, clofazimine, carbomycin, sulfa drugs such as sulfamethoxazole, and certain cephalosporins such as cefoperazone, showed higher activity against B. burgdorferi persister cells resulting more effective than doxycycline or amoxicillin (213). Although the combination of these drugs was found to be active against B. burgdorferi persisters, they showed poor activity when used individually (24). Daptomycin was found to be the most active antibiotic when combined with doxycycline plus either beta-lactams like cefoperazone or carbenicillin or alternatively with clofazimine (212). Daptomycin in combination with doxycycline and cefoperazone was found to be able to completely eradicate B. burgdorferi persisters, revealing a durable killing activity that was not achieved by any other drug combinations (212). These results where further supported by prospective randomized clinical studies which failed to demonstrate significant beneficial effect of additional prolonged therapy with doxycycline, amoxicillin or ceftriaxone in monotherapy, in patients with Lyme encephalopathy and post-treatment symptoms of Lyme disease (176, 215).
In addition to biofilm formation, the ability of Borrelia to localize intracellularly in the host has been proposed as a mechanism which might favor chronic or persistent infection and may contribute in reducing the efficacy of antibiotics. However, Borrelia predominantly occupies the extracellular matrix, and the antibiotics recommended for the treatment of LB are first-line drugs in several intracellular infections (216, 217). Doxycycline and azithromycin are commonly used for the treatment of Mycoplasma, Chlamydia, and Legionella, while ceftriaxone is effective against Salmonella and Neisseria, and amoxicillin is used to treat Listeria infections (217, 218). Nevertheless, biofilm production by extracellular bacteria and intracellular localization of Borrelia are not mutually exclusive and may both participate in supporting chronic bacterial persistence in the host.
On the other hand, a polymicrobial infection is a frequent occurrence in ticks (219, 220). Chronic and persistent forms of Lyme have been also associated to infections caused by Babesia spp. and Anaplasma phagocytophilum, Bartonella henselae, or other minor pathogens (217, 219, 221). This condition may add a further level of complexity to the clinical and therapeutic management of LB since it may lead to inappropriate diagnoses and apparent failure of the antibiotic treatment targeted exclusively against Borrelia. However, the real clinical relevance of these coinfections is unclear and requires further, more in depth evaluation.
LNB is the most dangerous manifestation of Lyme disease. Although the early antimicrobial treatment is effective in the majority of patients, persistent forms are relatively common. The mechanisms underlying chronic LNB and other persistent forms of Lyme are unknown. Patients who have late manifestations of LB generally show a slower response to therapy with incomplete resolution. Persistent Borrelia infection requires prolonged antimicrobial treatment, with limited and controversial clinical efficacy. Recent evidences suggest that the antibiotic resistance and the reoccurrence of LB are associated with biofilm-like aggregates, which allow Borrelia spp. to resist to adverse environmental conditions. Several promising FDA-approved drugs have been shown to have excellent anti-persister activity when used in combination while their use in monotherapy regimens showed a poor effectiveness. This notion should be taken into careful consideration for the clinical management of Lyme Disease in order to prevent long-term complications.
In preliminary studies by the clinical Biofilm Ring Test® (cBRT), we found that Borrelia is able to readily produce biofilm within 24–48 h. Diagnostic procedures such as the cBRT, which allow for a rapid biofilm measurement may represent very useful tools for clinical applications (222, 223), since the rapid identification of biofilm-producing Borrelia strains, may help identify forms of LB which are at risk of chronicity (224). Further, characterization of Borrelia biofilm as well as the ensuing inflammatory process will likely provide novel insight to better understand the mechanism(s) concurring to LNB pathogenesis and may offer new therapeutic targets for intervention.
Conceived and designed the study: ED, IC, LT, FP, and FE. Performed the confocal microscopy analysis: ED, IC, MP, GP, and MG. All authors analyzed data. Wrote the paper: ED, IC, LT, VB, GD, ET, and FE. All the authors read and approved the final version of the manuscript.
The authors declare that the research was conducted in the absence of any commercial or financial relationships that could be construed as a potential conflict of interest.
This work was partially supported by L'Associazione Nazionale Contro le Infezioni Ospedaliere (L'ANCIO).
1. Steere AC, Strle F, Wormser GP, Hu LT, Branda JA, Hovius JW, et al. Lyme borreliosis. Nat Rev Dis Primers (2016) 2:16090. doi: 10.1038/nrdp.2016.90
2. Ai CX, Zhang WF, Zhao JH. Sero-epidemiology of Lyme disease in an endemic area in China. Microbiol Immunol. (1994) 38:505–9.
3. Rudenko N, Golovchenko M, Grubhoffer L, Oiver JH Jr. Updates on Borrelia burgdorferi sensu lato complex with respect to public health. Ticks Tick Borne Dis. (2011) 2:123–8. doi: 10.1016/j.ttbdis.2011.04.002
4. Mead PS. Epidemiology of lyme disease. Infect Dis Clin North Am. (2015) 29:187–210. doi: 10.1016/j.idc.2015.02.010
5. Steere AC, Strle F, Wormser GP, Hu LT, Branda JA, Hovius JW, et al. Correction: lyme borreliosis. Mead Nat Rev Dis Primers (2017) 3:17062. doi: 10.1038/nrdp.2017.62
6. Hengge UR, Tannapfel A, Tyring SK, Erbel R, Arendt G, Ruzicka T. Lyme borreliosis. Lancet Infect Dis. (2003) 3:489–500. doi: 10.1016/S1473-3099(03)00722-9
7. Wormser GP, Dattwyler RJ, Shapiro ED, Halperin JJ, Steere AC, Klempner MS, et al. The clinical assessment, treatment, and prevention of lyme disease, human granulocytic anaplasmosis, and babesiosis: clinical practice guidelines by the Infectious Diseases Society of America. Clin Infect Dis. (2006) 43:1089–134. doi: 10.1086/508667
8. Halperin JJ. Nervous system lyme disease. Curr Infect Dis Rep. (2015) 17:445. doi: 10.1007/s11908-014-0445-6
10. Vanzieleghem B, Lemmerling M, Carton D, Achten E, Vanlangenhove P, Matthys E, et al. Lyme disease in a child presenting with bilateral facial nerve palsy: MRI findings and review of the literature. Neuroradiology (1998) 40:739–42.
11. Stonehouse A, Studdiford JS, Henry CA. An update on the diagnosis and treatment of early Lyme disease: “focusing on the bull's eye, you may miss the mark”. J Emerg Med. (2010) 39:e147–51. doi: 10.1016/j.jemermed.2007.06.007
12. Reik L, Steere AC, Bartenhagen NH, Shope RE, Malawista SE. Neurologic abnormalities of Lyme disease. Medicine (1979) 58:281–94.
13. Koedel U, Fingerle V, Pfister HW. Lyme neuroborreliosis-epidemiology, diagnosis and management. Nat Rev Neurol. (2015) 11:446–56. doi: 10.1038/nrneurol.2015.121
14. Ogrinc K, Lusa L, Lotric-Furlan S, Bogovič P, Stupica D, Cerar T, et al. Course and outcome of early European Lyme neuroborreliosis (Bannwarth syndrome): clinical and laboratory findings. Clin Infect Dis. (2016) 63:346–53. doi: 10.1093/cid/ciw299
15. Uldry PA, Regli F, Bogousslavsky J. Cerebral angiopathy and recurrent strokes following Borrelia burgdorferi infection. J Neurol Neurosurg Psychiatry (1987) 50:1703–4. doi: 10.1136/jnnp.50.12.1703
16. Stanek G, Fingerle V, Hunfeld KP, Jaulhac B, Kaiser R, Krause A, et al. Lyme borreliosis: clinical case definitions for diagnosis and management in Europe. Clin Microbiol Infect. (2011) 17:69–79. doi: 10.1111/j.1469-0691.2010.03175.x
17. Oschmann P, Dorndorf W, Hornig C, Schafer C, Wellensiek HJ, Pflughaupt KW. Stages and syndromes of neuroborreliosis. J Neurol. (1998) 245:262–72.
18. Fallon BA, Levin ES, Schweitzer PJ, Hardesty D. Inflammation and central nervous system Lyme disease. Neurobiol Dis. (2010) 37:534–41. doi: 10.1016/j.nbd.2009.11.016
19. Garkowski A, Zajkowska J, Zajkowska A, Kułakowska A, Zajkowska O, Kubas B, Jurgilewicz D, et al. Cerebrovascular manifestations of lyme neuroborreliosis-a systematic review of published cases. Front Neurol. (2017) 8:146. doi: 10.3389/fneur.2017.00146
20. Steere AC, Angelis SM. Therapy for Lyme arthritis: strategies for the treatment of antibiotic-refractory arthritis. Arthritis Rheum. (2006) 54:3079–86. doi: 10.1002/art.22131
21. Berndtson K. Review of evidence for immune evasion and persistent infection in Lyme disease. Int J Gen Med. (2013) 6:291–306 doi: 10.2147/IJGM.S44114
22. Sapi E, Bastian SL, Mpoy CM, Scott S, Rattelle A, Pabbati N, et al. Characterization of biofilm formation by Borrelia burgdorferi in vitro. PLoS ONE (2012) 7:e48277. doi: 10.1371/journal.pone.0048277
23. Sapi E, Balasubramanian K, Poruri A, Maghsoudlou JS, Socarras KM, Timmaraju AV, et al. Evidence of in vivo existence of Borrelia Biofilm in Borrelial Lymphocytomas. Eur J Microbiol Immunol. (2016) 6:9–24. doi: 10.1556/1886.2015.00049
24. Feng J, Wang T, Shi W, Zhang S, Sullivan D, Auwaerter PG, et al. Identification of novel activity against Borrelia burgdorferi persisters using an FDA approved drug library. Emerg Microbes Infect. (2014) 3:e49. doi: 10.1038/emi.2014.53
25. Bremell D, Dotevall L. Oral doxycycline for Lyme neuroborreliosis with symptoms of encephalitis, myelitis, vasculitis or intracranial hypertension. Eur J Neurol. (2014) 21:1162–7. doi: 10.1111/ene.12420
26. Feng J, Shi W, Zhang S, Zhang Y. Identification of new compounds with high activity against stationary phase Borrelia burgdorferi from the NCI compound collection. Emerg Microbes Infect. (2015) 4:e31. doi: 10.1038/emi.2015.31
27. Middelveen MJ, Sapi E, Burke J, Filush KR, Franco A, Fesler MC, et al. Persistent borrelia infection in patients with ongoing symptoms of lyme disease. Healthcare (2018) 6:E33. doi: 10.3390/healthcare6020033
28. Hinckley AF, Connally NP, Meek JI, Johnson BJ, Kemperman MM, Feldman KA, et al. Lyme disease testing by large commercial laboratories in the United States. Clin Infect Dis. (2014) 59:676–81. doi: 10.1093/cid/ciu397
29. Sykes RA, Makiello P. An estimate of lyme borreliosis incidence in western europe. J Public Health (2017) 39:74–81. doi: 10.1093/pubmed/fdw017
30. van den Wijngaard CC, Hofhuis A, Simões M, Rood E, van Pelt W, Zeller H, et al. Surveillance perspective on Lyme borreliosis across the European Union and European Economic Area. Euro Surveill. (2017) 22:27. doi: 10.2807/1560-7917.ES.2017.22.27.30569
31. Bacon RM, Kugeler KJ, Mead PS. Centers for Disease Control and Prevention (CDC). Surveillance for lyme disease—United States, 1992–2006. MMWR Surveill Summ. (2008) 57:1–9.
32. Connecticut Department of Public Health. Lyme disease – Connecticut, 2008. Connecticut Epidemiol. (2009) 29:14–16.
33. Lindgren E, Jaenson T. Lyme Borreliosis in Europe: Influences of Climate and Climate Change, Epidemiology, Ecology and Adaptation Measures. (2006). Available online at: http://www.euro.who.int/__data/assets/pdf_file/0006/96819/E89522.pdf (Accessed October 1, 2010).
34. EUCALB. European Union Concerted Action on Lyme Borreliosis. (2014) Available online at: http://www.eucalb.com (Accessed October 1, 2014).
35. van Dam AP, Kuiper H, Vos K, Widjojokusumo A, de Jongh BM, Spanjaard L, et al. Different genospecies of Borrelia burgdorferi are associated with distinct clinical manifestations of Lyme borreliosis. Clin Infect Dis. (1993) 17:708–17.
36. Wilske B. Epidemiology and diagnosis of Lyme borreliosis. Ann Med. (2005) 37:568–79. doi: 10.1080/07853890500431934
37. Brisson D, Drecktrah D, Eggers CH, Samuels DS. Genetics of Borrelia burgdorferi. Annu Rev Genet. (2012) 46:515–36. doi: 10.1146/annurev-genet-011112-112140
38. Seifert SN, Khatchikian CE, Zhou W, Brisson D. Evolution and population genomics of the Lyme borreliosis pathogen, Borrelia burgdorferi. Trends Genet. (2015) 31:201–7. doi: 10.1016/j.tig.2015.02.006
39. Di Domenico EG, Auriche C, Viscardi V, Longhese MP, Gilson E, Ascenzioni F. The Mec1p and Tel1p checkpoint kinases allow humanized yeast to tolerate chronic telomere dysfunctions by suppressing telomere fusions. DNA Repair (2009) 8: 209–18. doi: 10.1016/j.dnarep.2008.10.005
40. Kobryn K, Chaconas G. Hairpin telomere resolvases. Microbiol Spectr. (2014) 2:6. doi: 10.1128/microbiolspec.MDNA3-0023-2014
41. Fraser CM, Casjens S, Huang WM, Sutton GG, Clayton R, Lathigra R, et al. Genomic sequence of a Lyme disease spirochaete, Borrelia burgdorferi. Nature (1997) 390:580–586.
42. Casjens S, Palmer N, van Vugt R, Huang WM, Stevenson B, Rosa P, et al. A bacterial genome in flux: the twelve linear and nine circular extrachromosomal DNAs in an infectious isolate of the Lyme disease spirochete Borrelia burgdorferi. Mol Microbiol. (2000) 35:490–516.
43. Casjens SR, Mongodin EF, Qiu WG, Dunn JJ, Luft BJ, Fraser-Liggett CM, et al. Whole-genome sequences of two Borrelia afzelii and two Borrelia garinii Lyme disease agent isolates. J Bacteriol. (2011) 193:6995–6. doi: 10.1128/JB.05951-11
44. Schutzer SE, Fraser-Liggett CM, Casjens SR, Qiu WG, Dunn JJ, Mongodin EF, et al. Whole-genome sequences of thirteen isolates of Borrelia burgdorferi. J Bacteriol. (2011) 193:1018–20. doi: 10.1128/JB.01158-10
45. Casjens SR, Mongodin EF, Qiu WG, Luft BJ, Schutzer SE, Gilcrease EB, et al. Genome stability of Lyme disease spirochetes: comparative genomics of Borrelia burgdorferi plasmids. PLoS ONE (2012) 7: e33280. doi: 10.1371/journal.pone.0033280
46. Schutzer SE, Fraser-Liggett CM, Qiu WG, Kraiczy P, Mongodin EF, Dunn JJ, et al. Whole-genome sequences of Borrelia bissettii, Borrelia valaisiana, and Borrelia spielmanii. J Bacteriol. (2012) 194:545–6. doi: 10.1128/JB.06263-11
47. Bontemps-Gallo S, Lawrence KA, Richards CL, Gherardini FC. Genomic and phenotypic characterization of Borrelia afzelii BO23 and Borrelia garinii CIP 103362. PLoS ONE (2018) 13:e0199641. doi: 10.1371/journal.pone.0199641
48. Radolf JD, Caimano MJ, Stevenson B, Hu LT. Of ticks, mice and men: understanding the dual-host lifestyle of Lyme disease spirochaetes. Nat Rev Microbiol. (2012) 10:87–99. doi: 10.1038/nrmicro2714
49. Verhaegh D, Joosten LAB, Oosting M. The role of host immune cells and Borrelia burgdorferi antigens in the etiology of Lyme disease. Eur Cytokine Netw. (2017) 28: 70–84. doi: 10.1684/ecn.2017.0396
50. Stewart PE, Rosa PA. Physiologic and genetic factors influencing the zoonotic cycle of Borrelia burgdorferi. Curr Top Microbiol Immunol. (2017) 415:63–82. doi: 10.1007/82_2017_43
51. Tijsse-Klasen E, Pandak N, Hengeveld P, Takumi K, Koopmans MP, Sprong H. Ability to cause erythema migrans differs between Borrelia burgdorferi sensu lato isolates. Parasit Vectors (2013) 6:23. doi: 10.1186/1756-3305-6-23
52. Brisson D, Baxamusa N, Schwartz I, Wormser GP. Biodiversity of Borrelia burgdorferi strains in tissues of Lyme disease patients. PLoS ONE (2011) 6:e22926. doi: 10.1371/journal.pone.0022926
53. Wormser GP, Halperin JJ. Toward a better understanding of European lyme neuroborreliosis. Clin Infect Dis. (2013) 57:510–2. doi: 10.1093/cid/cit322
54. Hanincová K, Kurtenbach K, Diuk-Wasser M, Brei B, Fish D. Epidemic spread of Lyme borreliosis, northeastern United States. Emerg Infect Dis. (2006) 12:604–11. doi: 10.3201/eid1204.051016
55. Hanincová K, Ogden NH, Diuk-Wasser M, Pappas CJ, Iyer R, Fish D, et al. Fitness variation of Borrelia burgdorferi sensu stricto strains in mice. Appl Environ Microbiol. (2007) 74:153–7. doi: 10.1128/AEM.01567-07
56. Brisson D, Dykhuizen DE, Ostfeld RS. Conspicuous impacts of inconspicuous hosts on the Lyme disease epidemic. Proc Biol Sci. (2008) 275:227–35. doi: 10.1098/rspb.2007.1208
57. Wormser GP, Liveris D, Nowakowski J, Nadelman RB, Cavaliere LF, McKenna D, et al. Association of specific subtypes of Borrelia burgdorferi with hematogenous dissemination in early Lyme disease. J Infect Dis. (1999) 180:720–5.
58. Jones KL, Glickstein LJ, Damle N, Sikand VK, McHugh G, Steere AC. Borrelia burgdorferi genetic markers and disseminated disease in patients with early Lyme disease. J Clin Microbiol. (2006) 44:4407–13. doi: 10.1128/JCM.01077-06
59. Seinost G, Dykhuizen DE, Dattwyler RJ, Golde WT, Dunn JJ, Wang IN, et al. Four clones of Borrelia burgdorferi sensu stricto cause invasive infection in humans. Infect Immun. (1999) 67:3518–24.
60. Earnhart CG, Buckles EL, Dumler JS, Marconi RT. Demonstration of OspC type diversity in invasive human Lyme disease isolates and identification of previously uncharacterized epitopes that define the specificity of the OspC murine antibody response. Infect Immun (2005) 73:7869–77. doi: 10.1128/IAI.73.12.7869-7877.2005
61. Alghaferi MY, Anderson JM, Park J, Auwaerter PG, Aucott JN, Norris DE, et al. Borrelia burgdorferi ospC heterogeneity among human and murine isolates from a defined region of northern Maryland and southern Pennsylvania: lack of correlation with invasive and noninvasive genotypes. J Clin Microbiol. (2005) 43:1879–84. doi: 10.1128/JCM.43.4.1879-1884.2005
62. Wormser GP, Brisson D, Liveris D, Hanincova K, Sandigursky S, Nowakowski J, et al. Borrelia burgdorferi genotype predicts the capacity for hematogenous dissemination during early Lyme disease. J Infect Dis. (2008) 198:1358–64. doi: 10.1086/592279
63. Lin YP, Benoit V, Yang X, Martínez-Herranz R, Pal U, Leong JM. Strain-specific variation of the decorin-binding adhesin DbpA influences the tissue tropism of the Lyme disease spirochete. PLoS Pathog. (2014) 10:e1004238. doi: 10.1371/journal.ppat.1004238
64. Ornstein K, Berglund J, Bergstrom S, Norrby R, Barbour AG. Three major Lyme Borrelia genospecies (Borrelia burgdorferi sensu stricto, B. afzelii and B. garinii) identified by PCR in cerebrospinal fluid from patients with neuroborreliosis in Sweden. Scand J Infect Dis. (2002) 34:341–6. doi: 10.1080/00365540110080313
65. Fingerle V, Schulte-Spechtel UC, Ruzic-Sabljic E, Leonhard S, Hofmann H, Weber K, et al. Epidemiological aspects and molecular characterization of Borrelia burgdorferi s.l. from southern Germany with special respect to the new species Borrelia spielmanii sp. nov. Int J Med Microbiol. (2008) 298:279–90. doi: 10.1016/j.ijmm.2007.05.002
66. Bazovska S, Durovska J, Derdakova M, Taragelova V, Pancak J, Zaborska M, et al. The genospecies B. burgdorferi s.l., isolated from ticks and from neurological patients with suspected Lyme borreliosis. Neuro Endocrinol Lett. (2011) 32:491–495.
67. Ogrinc K, Lotrič-Furlan S, Maraspin V, Lusa L, Cerar T, RuŽič-Sabljič E, et al. Suspected early Lyme neuroborreliosis in patients with erythema migrans. Clin Infect Dis. (2013) 57:501–9. doi: 10.1093/cid/cit317
68. Margos G, Wilske B, Sing A, Hizo-Teufel C, Cao WC, Chu C, et al. Borrelia bavariensis sp. nov. is widely distributed in Europe and Asia. Int J Syst Evol Microbiol. (2013) 63:4284–8. doi: 10.1099/ijs.0.052001-0
69. Margos G, Vollmer SA, Ogden NH, Fish D. Population genetics, taxonomy, phylogeny and evolution of Borrelia burgdorferi sensu lato. Infect Genet Evol. (2011) 11:1545–63. doi: 10.1016/j.meegid.2011.07.022
70. Collares-Pereira M, Couceiro S, Franca I, Kurtenbach K, Schafer SM, Vitorino L, et al. First isolation of Borrelia lusitaniae from a human patient. J Clin Microbiol. (2004) 42:1316–8. doi: 10.1128/JCM.42.3.1316-1318.2004
71. Diza E, Papa A, Vezyri E, Tsounis S, Milonas I, Antoniadis A. Borrelia valaisiana in cerebrospinal fluid. Emerg Infect Dis. (2004) 10:1692–3. doi: 10.3201/eid1009.030349
72. Derdáková M, Lenčáková D. Association of genetic variability within the Borrelia burgdorferi sensu lato with the ecology, epidemiology of Lyme borreliosis in Europe. Ann Agric Environ Med. (2005) 12:165–72.
73. Strle F, RuŽic'-Sabljic' E, Cimperman J, Lotric-Furlan S, Maraspin V. Comparison of findings for patients with Borrelia garinii and Borrelia afzelii isolated from cerebrospinal fluid. Clin Infect Dis. (2006) 43:704–10. doi: 10.1086/506936
74. Øymar K, Tveitnes D. Clinical characteristics of childhood Lyme neuroborreliosis in an endemic area of northern Europe. Scand J Infect Dis. (2009) 41:88–94. doi: 10.1080/00365540802593453
75. Barstad B, Quarsten H, Tveitnes D, Noraas S, Ask IS, Saeed M, et al. Direct molecular detection and genotyping of Borrelia burgdorferi Sensu Lato in cerebrospinal fluid of children with lyme neuroborreliosis. J Clin Microbiol. (2018) 56:e01868–17. doi: 10.1128/JCM.01868-17
76. Carlsson SA, Granlund H, Jansson C, Nyman D, Wahlberg P. Characteristics of erythema migrans in Borrelia afzelii and Borrelia garinii infections. Scand J Infect Dis. (2003) 35:31–3.
77. Logar M, Ruzic-Sabljic E, Maraspin V, Lotric-Furlan S, Cimperman J, Jurca T, et al. Comparison of erythema migrans caused by Borrelia afzelii and Borrelia garinii. Infection (2004) 32:15–9. doi: 10.1007/s15010-004-3042-z
78. Dykhuizen DE, Brisson D, Sandigursky S, Wormser GP, Nowakowski J, Nadelman RB, et al. The propensity of different Borrelia burgdorferi sensu stricto genotypes to cause disseminated infections in humans. Am J Trop Med Hyg. (2008) 78:806–10.
79. Strle K, Jones KL, Drouin EE, Li X, Steere AC. Borrelia burgdorferi RST1 (OspC type A) genotype is associated with greater inflammation and more severe Lyme disease. Am J Pathol. (2011) 178:2726–39. doi: 10.1016/j.ajpath.2011.02.018
80. Coipan EC, Jahfari S, Fonville M, Oei GA, Spanjaard L, Takumi K, et al. Imbalanced presence of Borrelia burgdorferi s.l. multilocus sequence types in clinical manifestations of Lyme borreliosis. Infect Genet Evol. (2016) 42: 66–76. doi: 10.1016/j.meegid.2016.04.019
81. Levene H. Genetic equilibrium when more than one ecological niche is available. Am Nat. (1953) 87:331–3.
82. Gliddon C, Strobeck C. Necessary and sufficient conditions for multipleniche polymorphism in haploids. Am Nat. (1975) 109:233–5.
83. Norman R, Bowers RG, Begon M, Hudson PJ. Persistence of tick-borne virus in the presence of multiple host species: tick reservoirs and parasitemediated competition. J Theor Biol. (1999) 200:111–8.
84. Schmidt KA, Ostfeld RS. Biodiversity and the dilution effect in disease ecology. Ecology (2001) 82:609–19. doi: 10.1890/0012-9658(2001)082[0609:BATDEI]2.0.CO;2
85. States SL, Brinkerhoff RJ, Carpi G, Steeves TK, Folsom-O'Keefe C, DeVeaux M, et al. Lyme disease risk not amplified in a species-poor vertebrate community: similar Borrelia burgdorferi tick infection prevalence and OspC genotype frequencies. Infect Gen Evol. (2014) 27:566–75. doi: 10.1016/j.meegid.2014.04.014
86. Hyde JA. Borrelia burgdorferi keeps moving and carries on: a review of borrelial dissemination and invasion. Front Immunol. (2017) 8:114. doi: 10.3389/fimmu.2017.00114
87. Caimano MJ, Drecktrah D, Kung F, Samuels DS. Interaction of the Lyme disease spirochete with its tick vector. Cell Microbiol. (2016) 18:919–27. doi: 10.1111/cmi.12609
88. Yang XF, Pal U, Alani SM, Fikrig E, Norgard MV. Essential role for OspA/B in the life cycle of the Lyme disease spirochete. J Exp Med. (2004) 199:641–8. doi: 10.1084/jem.20031960
89. Fikrig E, Pal U, Chen M, Anderson JF, Flavell RA. OspB antibody prevents Borrelia burgdorferi colonization of Ixodes scapularis. Infect Immun. (2004) 72:1755–9.
90. Pal U, Li X, Wang T, Montgomery RR, Ramamoorthi N, Desilva AM, et al. TROSPA, an Ixodes scapularis receptor for Borrelia burgdorferi. Cell (2004) 119:457–68. doi: 10.1016/j.cell.2004.10.027
91. Hubner A, Yang X, Nolen DM, Popova TG, Cabello FC, Norgard MV. Expression of Borrelia burgdorferi OspC and DbpA is controlled by a RpoN-RpoS regulatory pathway. Proc Natl Acad Sci USA. (2001) 98:12724–9. doi: 10.1073/pnas.231442498
92. Anguita J, Hedrick MN, Fikrig E. Adaptation of Borrelia burgdorferi in the tick and the mammalian host. FEMS Microbiol Rev. (2003) 27:493–504. doi: 10.1016/S0168-6445(03)00036-6
93. Srivastava SY, de Silva AM. Reciprocal expression of ospA and ospC in single cells of Borrelia burgdorferi. J Bacteriol. (2008) 190:3429–33. doi: 10.1128/JB.00085-08
94. Shi Y, Dadhwal P, Li X, Liang FT. BosR functions as a repressor of the ospAB operon in Borrelia burgdorferi. PLoS ONE (2014) 9:e109307. doi: 10.1371/journal.pone.0109307
95. Ouyang Z, Zhou J, Norgard MV. Synthesis of RpoS is dependent on a putative enhancer binding protein Rrp2 in Borrelia burgdorferi. PLoS ONE (2014) 9:e96917. doi: 10.1371/journal.pone.0096917
96. Carrasco SE, Troxell B, Yang Y, Brandt SL, Li H, Sandusky GE, et al. Outer surface protein OspC is an antiphagocytic factor that protects Borrelia burgdorferi from phagocytosis by macrophages. Infect Immun. (2015) 83:4848–60. doi: 10.1128/IAI.01215-15
97. Gilmore RD Jr, Kappel KJ, Dolan MC, Burkot TR, Johnson BJ. Outer surface protein C (OspC), but not P39, is a protective immunogen against a tick-transmitted Borrelia burgdorferi challenge: evidence for a conformational protective epitope in OspC. Infect Immun. (1996) 64:2234–9.
98. Bockenstedt LK, Hodzic E, Feng S, Bourrel KW, de Silva A, Montgomery RR, et al. Borrelia burgdorferi strain-specific OspC-mediated immunity in mice. Infect Immun. (1997) 65:4661–7.
99. Grimm D, Tilly K, Byram R, Stewart PE, Krum JG, Bueschel DM, et al. Outer-surface protein C of the Lyme disease spirochete: a protein induced in ticks for infection of mammals. Proc Natl Acad Sci USA. (2004) 101:3142–7. doi: 10.1073/pnas.0306845101
100. Tilly K, Krum JG, Bestor A, Jewett MW, Grimm D, Bueschel D, et al. Borrelia burgdorferi OspC protein required exclusively in a crucial early stage of mammalian infection. Infect Immun. (2006) 74:3554–64. doi: 10.1128/IAI.01950-05
101. Xu Q, McShan K, Liang FT. Identification of an ospC operator critical for immune evasion of Borrelia burgdorferi. Mol Microbiol. (2007) 64:220–31. doi: 10.1111/j.1365-2958.2007.05636.x
102. Gilbert MA, Morton EA, Bundle SF, Samuels DS. Artificial regulation of ospC expression in Borrelia burgdorferi. Mol Microbiol. (2007) 63:1259–73. doi: 10.1111/j.1365-2958.2007.05593.x
103. Tilly K, Bestor A, Jewett MW, Rosa P. Rapid clearance of Lyme disease spirochetes lacking OspC from skin. Infect Immun. (2007) 75:1517–9. doi: 10.1128/IAI.01725-06
104. Xu Q, McShan K, Liang FT. Essential protective role attributed to the surface lipoproteins of Borrelia burgdorferi against innate defences. Mol Microbiol. (2008) 69:15–29. doi: 10.1111/j.1365-2958.2008.06264.x
105. Liang FT, Jacobs MB, Bowers LC, Philipp MT. An immune evasion mechanism for spirochetal persistence in Lyme borreliosis. J Exp Med. (2002) 195:415–22. doi: 10.1084/jem.20011870
106. Liang FT, Yan J, Mbow ML, Sviat SL, Gilmore RD, Mamula M, et al. Borrelia burgdorferi changes its surface antigenic expression in response to host immune responses. Infect Immun. (2004) 72:5759–67. doi: 10.1128/IAI.72.10.5759-5767.2004
107. Norris SJ. Antigenic variation with a twist: the Borrelia story. Mol Microbiol. (2006) 60:1319–22. doi: 10.1111/j.1365-2958.2006.05204.x
109. Morrison TB, Weis JH, Weis JJ. Borrelia burgdorferi outer surface protein A (OspA) activates and primes human neutrophils. J Immunol. (1997) 158:4838–45.
110. Häupl T, Landgraf S, Netusil P, Biller N, Capiau C, Desmons P, et al. Activation of monocytes by three OspA vaccine candidates: lipoprotein OspA is a potent stimulator of monokines. FEMS Immunol Med Microbiol. (1997) 19:15–23.
111. Pal U, de Silva AM, Montgomery RR, Fish D, Anguita J, Anderson JF, et al. Attachment of Borrelia burgdorferi within Ixodes scapularis mediated by outer surface protein A. J Clin Invest. (2000) 106:561–9. doi: 10.1172/JCI9427
112. Schwan TG, Piesman J. Temporal changes in outer surface proteins A and C of the Lyme disease-associated spirochete, Borrelia burgdorferi, during the chain of infection in ticks and mice. J Clin Microbiol. (2000) 38:382–8.
113. Ohnishi J, Piesman J, de Silva AM. Antigenic and genetic heterogeneity of Borrelia burgdorferi populations transmitted by ticks. Proc Natl Acad Sci USA. (2001) 98:670–5. doi: 10.1073/pnas.98.2.670
114. Rupprecht TA, Koedel U, Fingerle V, Pfister HW. The pathogenesis of Lyme neuroborreliosis: from infection to inflammation. Mol Med. (2008) 14:205–12. doi: 10.1016/j.ajpath.2015.01.024
115. Cassatt DR, Patel NK, Ulbrandt ND, Hanson MS. DbpA, but not OspA, is expressed by Borrelia burgdorferi during spirochetemia and is a target for protective antibodies. Infect Immun. (1998) 66:5379–87.
116. Brissette CA, Verma A, Bowman A, Cooley AE, Stevenson B. The Borrelia burgdorferi outer-surface protein ErpX binds mammalian laminin. Microbiology (2009) 155:863–72. doi: 10.1099/mic.0.024604-0
117. Önder Ö, Humphrey PT, McOmber B, Korobova F, Francella N, Greenbaum DC, et al. OspC is potent plasminogen receptor on surface of Borrelia burgdorferi. J Biol Chem. (2012) 287:16860–8. doi: 10.1074/jbc.M111.290775
118. Nogueira SV, Smith AA, Qin JH, Pal U. A surface enolase participates in Borrelia burgdorferi-plasminogen interaction and contributes to pathogen survival within feeding ticks. Infect Immun. (2012) 80:82–90. doi: 10.1128/IAI.05671-11
119. Sun H. The interaction between pathogens and the host coagulation system. Physiology (2006) 21:281–8. doi: 10.1152/physiol.00059.2005
120. Vieira ML, Nascimento AL. Interaction of spirochetes with the host fibrinolytic system and potential roles in pathogenesis. Crit Rev Microbiol. (2016) 42:573–87. doi: 10.3109/1040841X.2014.972336
121. Plow EF, Herren T, Redlitz A, Miles LA, Hoover-Plow JL. The cell biology of the plasminogen system. FASEB J. (1995) 9:939–45.
122. Lagal V, Portnoï D, Faure G, Postic D, Baranton G. Borrelia burgdorferi sensu stricto invasiveness is correlated with OspC-plasminogen affinity. Microbes Infect. (2006) 8:645–52. doi: 10.1016/j.micinf.2005.08.017
123. Pancholi V. Multifunctional alpha-enolase: its role in diseases. Cell Mol Life Sci. (2001) 58:902–20.
124. Pancholi V, Fischetti VA. Alpha-enolase, a novel strong plasmin-(ogen) binding protein on the surface of pathogenic streptococci. J Biol Chem. (1998) 273:14503–15.
125. Bercic RL, Slavec B, Lavric M, Narat M, Bidovec A, Dovc P, et al. Identification of major immunogenic proteins of Mycoplasma synoviae isolates. Vet Microbiol. (2008) 127:147–54. doi: 10.1016/j.vetmic.2007.07.020
126. Mundodi V, Kucknoor AS, Alderete JF. Immunogenic and plasminogen-binding surface-associated alpha-enolase of Trichomonas vaginalis. Infect Immun. (2008) 76:523–31. doi: 10.1128/IAI.01352-07
127. Pitarch A, Jimenez A, Nombela C, Gil C. Decoding serological response to Candida cell wall immunome into novel diagnostic, prognostic, and therapeutic candidates for systemic candidiasis by proteomic and bioinformatic analyses. Mol Cell Proteom. (2006) 5:79–96. doi: 10.1074/mcp.M500243-MCP200
128. Coleman JL, Gebbia JA, Piesman J, Degen JL, Bugge TH, Benach JL. Plasminogen is required for efficient dissemination of B. burgdorferi in ticks and for enhancement of spirochetemia in mice. Cell (1997) 89:1111–9.
129. Fuchs H, Wallich R, Simon MM, Kramer MD. The outer surface protein A of the spirochete Borrelia burgdorferi is a plasmin(ogen) receptor. Proc Natl Acad Sci USA. (1994) 91:12594–8.
130. Coleman JL, Roemer EJ, Benach JL. Plasmin-coated Borrelia burgdorferi degrades soluble and insoluble components of the mammalian extracellular matrix. Infect Immun. (1999) 67:3929–36.
131. Gebbia JA, Coleman JL, Benach JL. Borrelia spirochetes upregulate release and activation of matrix metalloproteinase gelatinase B (MMP-9) and collagenase 1 (MMP-1) in human cells. Infect Immun. (2001) 69:456–62. doi: 10.1128/IAI.69.1.456-462.2001
132. Allal J, Thomas P, Mazzonelli J. Borrelia isolated from cerebrospinal fluid in a French case of Lyme disease. Ann Rheum Dis. (1986) 45:789–90.
133. Luft BJ, Steinman CR, Neimark HC, Muralidhar B, Rush T, Finkel MF, et al. Invasion of the central nervous system by Borrelia burgdorferi in acute disseminated infection. JAMA (1992) 267:1364–7.
134. Grab DJ, Perides G, Dumler JS, Kim KJ, Park J, Kim YV, et al. Borrelia burgdorferi, host-derived proteases, and the blood-brain barrier. Infect Immun. (2005) 73:1014–22. doi: 10.1128/IAI.73.2.1014-1022.2005
135. Biegel D, Spencer DD, Pachter JS. Isolation and culture of human brain microvessel endothelial cells for the study of blood-brain barrier properties in vitro. Brain Res. (1995) 692:183–9.
136. Coureuil M, Lécuyer H, Bourdoulous S, Nassif X. A journey into the brain: insight into how bacterial pathogens cross blood-brain barriers. Nat Rev Microbiol. (2017) 15:149–59. doi: 10.1038/nrmicro.2016.178
137. Szczepanski A, Furie MB, Benach JL, Lane BP, Fleit HB. Interaction between Borrelia burgdorferi and endothelium in vitro. J Clin Invest. (1990) 85:1637–47. doi: 10.1172/JCI114615
138. Comstock LE, Thomas DD. Penetration of endothelial cell monolayers by Borrelia burgdorferi. Infect Immun. (1989) 57:1626–8.
139. Grab DJ, Nyarko E, Nikolskaia OV, Kim YV, Dumler JS. Human brain microvascular endothelial cell traversal by Borrelia burgdorferi requires calcium signaling. Clin Microbiol Infect. (2009) 15:422–6. doi: 10.1111/j.1469-0691.2009.02869.x
140. Livengood JA, Gilmore J. Invasion of human neuronal and glial cells by an infectious strain of Borrelia burgdorferi. Microbes Infect. (2006) 8:2832–40. doi: 10.1016/j.micinf.2006.08.014
141. Ma Y, Sturrock A, Weis JJ. Intracellular localization of Borrelia burgdorferi within human endothelial cells. Infect Immun. (1991) 59:671–8.
142. Girschick HJ, Huppertz HI, Russmann H, Krenn V, Karch H. Intracellular persistence of Borrelia burgdorferi in human synovial cells. Rheumatol Int. (1996) 16:125–32. doi: 10.1007/BF01409985
143. Koedel U, Pfister HW. Lyme neuroborreliosis. Curr Opin Infect Dis. (2017) 30:101–7. doi: 10.1097/QCO.0000000000000332
145. Burgdorfer W, Barbour AG, Hayes SF, Benach JL, Grunwaldt E, Davis JP. Lyme disease: a tick-borne spirochetosis? Science (1982) 216:1317–9.
146. Pfister HW, Einhaupl K, Preac-Mursic V, Wilske B, Schierz G. The spirochetal etiology of lymphocytic meningoradiculitis of Bannwarth (Bannwarth's syndrome). J Neurol. (1984) 231:141–4.
147. Miklossy J. Chronic or late lyme neuroborreliosis: analysis of evidence compared to chronic or late neurosyphilis. Open Neurol J. (2012) 6:146–57. doi: 10.2174/1874205X01206010146
148. Alba MA, Espígol-Frigolé G, Prieto-González S, Tavera-Bahillo I, García-Martínez A, Butjosa M, et al. Central nervous system vasculitis: still more questions than answers. Curr Neuropharmacol. (2011) 9:437–48. doi: 10.2174/157015911796557920
149. Obel N, Dessau RB, Krogfelt KA, Bodilsen J, Andersen NS, Møller JK, et al. Long term survival, health, social functioning, and education in patients with European Lyme neuroborreliosis: nationwide population based cohort study. BMJ (2018) 361:k1998. doi: 10.1136/bmj.k1998
150. Kruger H, Heim E, Schuknecht B, Scholz S. Acute and chronic neuroborreliosis with and without CNS involvement: a clinical, MRI, and HLA study of 27 cases. J Neurol. (1991) 238:271–80.
151. Hansen K, Lebech AM. The clinical and epidemiological profile of Lyme neuroborreliosis in Denmark 1985–1990. A prospective study of 187 patients with Borrelia burgdorferi specific intrathecal antibody production. Brain (1992) 115:399–423.
152. Kruger H, Reuss K, Pulz M, Rohrbach E, Pflughaupt KW, Martin R, et al. Meningoradiculitis and encephalomyelitis due to Borrelia burgdorferi: a follow-up study of 72 patients over 27 years. J Neurol. (1989) 236:322–8.
153. Back T, Grunig S, Winter Y, Bodechtel U, Guthke K, Khati D, et al. Neuroborreliosis-associated cerebral vasculitis: long-term outcome and health-related quality of life. J Neurol. (2013) 260:1569–75. doi: 10.1007/s00415-013-6831-4
154. Pearson S. On the radar: Lyme neuroborreliosis, an infectious cause of cerebral vasculitis. QJM (2015) 108:755. doi: 10.1093/qjmed/hcv043
155. Reik L Jr, Burgdorfer W, Donaldson JO. Neurologic abnormalities in Lyme disease without erythema chronicum migrans. Am J Med. (1986) 81:73–8.
156. Dupuis MJ. Multiple neurologic manifestations of Borrelia burgdorferi infection. Rev Neurol. (1988) 144:765–75.
157. Roelcke U, Barnett W, Wilder-Smith E, Sigmund D, Hacke W. Untreated neuroborreliosis: Bannwarth's syndrome evolving into acute schizophrenia-like psychosis. A case report. J Neurol. (1992) 239:129–31.
158. van den Bergen HA, Smith JP, van der Zwan A. Lyme psychosis. Ned Tijdschr Geneeskd (1993) 137:2098–100.
159. Stricker RB, Winger EE. Musical hallucinations in patients with Lyme disease. South Med J. (2003) 96:711–5. doi: 10.1097/01.SMJ.0000053458.21691.2E
160. Almeida OP, Lautenschlager NT. Dementia associated with infectious diseases. Int Psychogeriatr. (2005) 17:S65–77.
161. Hassett AL, Radvanski DC, Buyske S, Savage SV, Gara M, Escobar JI, et al. Role of psychiatric comorbidity in chronic Lyme disease. Arthritis Rheum. (2008) 59:1742–9. doi: 10.1002/art.24314
162. Cameron D. Severity of Lyme disease with persistent symptoms. Insights from a double-blind placebo-controlled clinical trial. Minerva Med. (2008) 99:489–496.
163. Miklossy J. Historic evidence to support a causal relationship between spirochetal infections and Alzheimer's disease. Front Aging Neurosci. (2015) 7:46. doi: 10.3389/fnagi.2015.00046
164. Aucott JN. Posttreatment Lyme disease syndrome. Infect Dis Clin North Am. (2015) 29:309–23. doi: 10.1016/j.idc.2015.02.012
165. Steere AC, Hutchinson GJ, Rahn DW, Sigal LH, Craft JE, DeSanna ET, et al. Treatment of the early manifestations of Lyme disease. Ann Intern Med. (1983) 99:22–26.
166. Cerar D, Cerar T, Ruzic-Sabljic E, Wormser GP, Strle F. Subjective symptoms after treatment of early Lyme disease. Am J Med. (2010) 123:79–86. doi: 10.1016/j.amjmed.2009.05.011
167. Nowakowski J, Nadelman RB, Sell R, McKenna D, Cavaliere LF, Holmgren D, et al. Long-term follow-up of patients with culture-confirmed Lyme disease. Am J Med. (2003) 115:91–6. doi: 10.1016/S0002-9343(03)00308-5
168. Marques A. Chronic lyme disease: a review. Infect Dis Clin North Am. (2008) 22:341–60. doi: 10.1016/j.idc.2007.12.011
169. Asch ES, Bujak DI, Weiss M, Peterson MG, Weinstein A. Lyme disease: an infectious and postinfectious syndrome. J Rheumatol. (1994) 21:454–61.
170. Aucott J, Morrison C, Munoz B, Rowe PC, Schwarzwalder A, West SK. Diagnostic challenges of early Lyme disease: lessons from a community case series. BMC Infect Dis. (2009) 9:79. doi: 10.1186/1471-2334-9-79
171. Marques A, Telford SR III, Turk SP, Chung E, Williams C, Dardick K, et al. Xenodiagnosis to detect Borrelia burgdorferi infection: a first-in-human study. Clin Infect Dis. (2014) 58:937–45. doi: 10.1093/cid/cit939
172. Hodzic E, Imai D, Feng S, Barthold SW. Resurgence of persisting non-cultivable Borrelia burgdorferi following antibiotic treatment in mice. PLoS ONE (2014) 9:e86907. doi: 10.1371/journal.pone.0086907
173. Bockenstedt LK, Gonzalez DG, Haberman AM, Belperron AA. Spirochete antigens persist near cartilage after murine Lyme borreliosis therapy. J Clin Invest. (2012) 122:2652–60. doi: 10.1172/JCI58813
174. Mygland A, Ljostad U, Fingerle V, Rupprecht T, Schmutzhard E, Steiner I, European Federation of Neurological S. EFNS guidelines on the diagnosis and management of European Lyme neuroborreliosis. Eur J Neurol Off J Eur Fed Neurol Soc. (2010) 17:e11–4. doi: 10.1111/j.1468-1331.2009.02862.x
175. Shapiro ED. Borrelia burgdorferi (Lyme disease). Pediatr Rev. (2014) 35:500–9. doi: 10.1542/pir.35-12-500
176. Klempner MS, Baker PJ, Shapiro ED, Marques A, Dattwyler RJ, Halperin JJ, et al. Treatment trials for post-Lyme disease symptoms revisited. Am J Med. (2013) 126:665–9. doi: 10.1016/j.amjmed.2013.02.014
177. Berende A, ter Hofstede HJ, Vos FJ, van Middendorp H, Vogelaar ML, Tromp M, et al. Randomized trial of longer-term therapy for symptoms attributed to Lyme disease. N Engl J Med. (2016) 374:1209–20. doi: 10.1056/NEJMoa1505425
178. Kersten A, Poitschek C, Rauch S, Aberer E. Effects of penicillin, ceftriaxone, and doxycycline on morphology of Borrelia burgdorferi. Antimicrob Agents Chemother. (1995) 39:1127–33.
179. Miklossy J, Kasas S, Zurn AD, McCall S, Yu S, McGeer PL. Persisting atypical and cystic forms of Borrelia burgdorferi and local inflammation in Lyme neuroborreliosis. J Neuroinflammation (2008) 5:1–18. doi: 10.1186/1742-2094-5-40
180. Brorson Ø, Brorson S-H, Scythes J, MacAllister J, Wier A, Margulis L. Destruction of spirochete Borrelia burgdorferi round-body propagules (RBs) by the antibiotic tigecycline. Proc Natl Acad Sci USA. (2009) 106:18656–61. doi: 10.1073/pnas.0908236106
181. Feng J, Shi W, Zhang S, Zhang Y. Persister mechanisms in Borrelia burgdorferi: implications for improved intervention. Emerg Microbes Infect. (2015) 4:e51. doi: 10.1038/emi.2015.51
182. Sapi E, Theophilus PA, Pham TV, Burugu D, Luecke DF. Effect of RpoN, RpoS and LuxS pathways on the biofilm formation and antibiotic sensitivity of Borrelia Burgdorferi. Eur J Microbiol Immunol. (2016) 6:272–86. doi: 10.1556/1886.2016.00026
183. Koo H, Allan RN, Howlin RP, Stoodley P, Hall-Stoodley L. Targeting microbial biofilms: current and prospective therapeutic strategies. Nat Rev Microbiol. (2017) 15:740–55. doi: 10.1038/nrmicro.2017.99
184. Hall-Stoodley L, Stoodley P. Evolving concepts in biofilm infections. Cell Microbiol. (2009) 11:1034–43. doi: 10.1111/j.1462-5822.2009.01323.x
185. Di Domenico EG, Toma L, Provot C, Ascenzioni F, Sperduti I, Prignano G, et al. Development of an in vitro Assay, Based on the BioFilm Ring Test®, for rapid profiling of biofilm-growing bacteria. Front Microbiol. (2016) 7:1429. doi: 10.3389/fmicb.2016.01429
186. Di Domenico EG, Farulla I, Prignano G, Gallo MT, Vespaziani M, Cavallo I, et al. Biofilm is a major virulence determinant in bacterial colonization of chronic skin ulcers independently from the multidrug resistant phenotype. Int J Mol Sci. (2017) 18:E1077. doi: 10.3390/ijms18051077
187. Timmaraju VA, Theophilus PA, Balasubramanian K, Shakih S, Luecke DF, Sapi E. Biofilm formation by Borrelia burgdorferi sensu lato. FEMS Microbiol Lett. (2015) 362:fnv120. doi: 10.1093/femsle/fnv120
188. Allen HB. Alzheimer's Disease: assessing the role of spirochetes, biofilms, the immune system, and amyloid-β with regard to potential treatment and prevention. J Alzheimers Dis. (2016) 53:1271–6. doi: 10.3233/JAD-160388
189. Donlan RM, Costerton WJ. Biofilms: survival mechanisms of clinically relevant microorganisms. Clin Microbiol Rev. (2002) 15:167–93. doi: 10.1128/CMR.15.2.167-193.2002
190. MacDonald AB. Plaques of Alzheimer's disease originate from cysts of Borrelia burgdorferi, the Lyme disease spirochete. Med Hypotheses (2006) 67:592–600. doi: 10.1016/j.mehy.2006.02.035
191. Dunham-Ems SM, Caimano MJ, Pal U, Wolgemuth CW, Eggers CH, Balic A, et al. Live imaging reveals a biphasic mode of dissemination of Borrelia burgdorferi within ticks. J Clin Invest. (2009) 119:3652–65. doi: 10.1172/JCI39401
192. Kazmierczak MJ, Wiedmann M, Boor KJ. Alternative sigma factors and their roles in bacterial virulence. Microbiol Mol Biol Rev. (2005) 69:527–43. doi: 10.1128/MMBR.69.4.527-543.2005
193. Caimano M, Iyer R, Eggers C, Gonzalez C, Morton E, Gilbert M, et al. Analysis of the RpoS regulon in Borrelia burgdorferi in response to mammalian host signals provides insight into RpoS function during the enzootic cycle. Mol Microbiol. (2007) 65:1193–217. doi: 10.1111/j.1365-2958.2007.05860.x
194. Yang XF, Alani SM, Norgard MV. The response regulator Rrp2 is essential for the expression of major membrane lipoproteins in Borrelia burgdorferi. Proc Natl Acad Sci USA. (2003) 100:11001–6. doi: 10.1073/pnas.1834315100
195. Fisher MA, Grimm D, Henion AK, Elias AF, Stewart PE, Rosa PA, et al. Borrelia burgdorferi sigma54 is required for mammalian infection and vector transmission but not for tick colonization. Proc Natl Acad Sci USA. (2005) 102:5162–7. doi: 10.1073/pnas.0408536102
196. Boardman BK, He M, Ouyang Z, Xu H, Pang X, Yang XF. Essential role of the response regulator Rrp2 in the infectious cycle of Borrelia burgdorferi. Infect Immun. (2008) 76:3844–53. doi: 10.1128/IAI.00467-08
197. Yin Y, Yang Y, Xiang X, Wang Q, Yang ZN, Blevins J, et al. Insight into the Dual functions of bacterial enhancer-binding protein Rrp2 of Borrelia burgdorferi. J Bacteriol. (2016) 198:1543–52. doi: 10.1128/JB.01010-15
198. Ouyang Z, Zhou J. The putative Walker A and Walker B motifs of Rrp2 are required for the growth of Borrelia burgdorferi. Mol Microbiol. (2017) 103:86–98. doi: 10.1111/mmi.13545
199. Favre-Bonté S, Chamot E, Köhler T, Romand JA, Van Delden C. Auto inducer production and quorum-sensing dependent phenotypes of Pseudomonas aeruginosa vary according to isolation site during colonization of incubated patients. J Sci BMC Microbiol. (2010) 8:623e33. doi: 10.1186/1471-2180-7-33
200. McNab R, Ford SK, El-Sabaeny A, Barbieri B, Cook GS, Lamont RJ. LuxS-based signaling in Streptococcus gordonii: autoinducer 2 controls carbohydrate metabolism and biofilm formation with Porphyromonas gingivalis. J Bacteriol. (2003) 185:274–84. doi: 10.1128/JB.185.1.274-284.2003
201. Merritt J, Qi F, Goodman SD, Anderson MH, Shi W. Mutation of luxS affects biofilm formation in Streptococcus mutans. Infect Immun. (2003) 71:1972–1979. doi: 10.1128/IAI.71.4.1972-1979.2003
202. Cole SP, Harwood J, Lee R, She R, Guiney DG. Characterization of monospecies biofilm formation by Helicobacter pylori. J Bacteriol. (2004) 186:3124–32. doi: 10.1128/JB.186.10.3124-3132.2004
203. Wen ZT, Nguyen AH, Bitoun JP, Abranches J, Baker HV, Burne RA. Transcriptome analysis of LuxS-deficient Streptococcus mutans grown in biofilms. Mol Oral Microbiol. (2011) 26:2–18. doi: 10.1111/j.2041-1014.2010.00581.x
204. Narasimhan S, Santiago F, Koski RA, Brei B, Anderson JF, Fish D, et al. Examination of the Borrelia burgdorferi transcriptome in Ixodes scapularis during feeding. J Bacteriol. (2002) 184:3122–5. doi: 10.1128/JB.184.11.3122-3125.2002
205. Stevenson B, Babb K. LuxS-mediated quorum sensing in Borrelia burgdorferi, the Lyme disease spirochete. Infect Immun. (2002) 70:4099–105. doi: 10.1128/IAI.70.8.4099-4105.2002
206. Babb K, von Lackum K, Wattier RL, Riley SP, Stevenson B. Synthesis of autoinducer 2 by the Lyme disease spirochete, Borrelia burgdorferi. J Bacteriol. (2005) 187:3079–87. doi: 10.1128/JB.187.9.3079-3087.2005
207. Arnold WK, Savage CR, Antonicello AD, Stevenson B. Apparent role for Borrelia burgdorferi LuxS during mammalian infection. Infect Immun. (2015) 83:1347–53. doi: 10.1128/IAI.00032-15
208. Steere AC, Malawista SE, Snydman DR, Shope RE, Andiman WA, Ross MR, et al. Lyme arthritis: an epidemic of oligoarticular arthritis in children and adults in three Connecticut communities. Arthritis Rheum. (1997) 20:7–17.
209. Rudenko N, Golovchenko M, Vancova M, Clark K, Grubhoffer L, Oliver JH Jr. Isolation of live Borrelia burgdorferi sensu lato spirochaetes from patients with undefined disorders and symptoms not typical for Lyme borreliosis. Clin Microbiol Infect. (2016) 22:267.e9–15. doi: 10.1016/j.cmi.2015.11.009
210. Barthold SW, Hodzic E, Imai DM, Feng S, Yang S, Luft BJ. Ineffectiveness of tigecycline against persistent Borrelia burgdorferi. Antimicrob Agents Chemother. (2010) 54:643–51. doi: 10.1128/AAC.00788-09
211. Feng J, Weitner M, Shi W, Zhang S, Zhang Y. Eradication of biofilm-like microcolony structures of Borrelia burgdorferi by daunomycin and daptomycin but not mitomycin C in combination with doxycycline and cefuroxime. Front Microbiol. (2016) 7:62. doi: 10.3389/fmicb.2016.00062
212. Feng J, Auwaerter PG, Zhang Y. Drug combinations against Borrelia burgdorferi persisters in vitro: eradication achieved by using daptomycin, cefoperazone and doxycycline. PLoS ONE (2015) 10:e0117207. doi: 10.1371/journal.pone.0117207
213. Caskey JR, Embers ME. Persister development by Borrelia burgdorferi populations in vitro. Antimicrob Agents Chemother. (2015) 59:6288–95. doi: 10.1128/AAC.00883-15
214. Feng J, Zhang S, Shi W, Zhang Y. Activity of sulfa drugs and their combinations against stationary phase B. burgdorferi in vitro. Antibiotics (2017) 6:E10. doi: 10.3390/antibiotics6010010
215. Fallon BA, Keilp JG, Corbera KM, Petkova E, Britton CB, Dwyer E, et al. A randomized, placebo-controlled trial of repeated IV antibiotic therapy for Lyme encephalopathy. Neurology (2008) 70:992–1003. doi: 10.1212/01.WNL.0000284604.61160.2d
216. Cabello FC, Godfrey HP, Newman SA. Hidden in plain sight: Borrelia burgdorferi and the extracellular matrix. Trends Microbiol. (2007) 15:350–4. doi: 10.1016/j.tim.2007.06.003
217. Lantos PM. Chronic lyme disease. Infect Dis Clin N Am. (2015) 325–40. doi: 10.1016/j.idc.2015.02.006
218. Lantos PM, Auwaerter PG, Wormser GP. A systematic review of Borrelia burgdorferi morphologic variants does not support a role in chronic Lyme disease. Clin Infect Dis. (2014) 58:663–71. doi: 10.1093/cid/cit810
219. Stricker RB, Gaito A, Harris NS, et al. Coinfection in patients with Lyme disease: how big a risk? Clin Infect Dis. (2003) 37:1277–8. doi: 10.1086/378893
220. Tokarz R, Jain K, Bennett A, Briese TW. Ian Lipkin. Assessment of polymicrobial infections in ticks in New York State. Vector Borne Zoo Dis. (2010) 10:217–21. doi: 10.1089/vbz.2009.0036
221. Owen DC. Is Lyme disease always poly microbial? – The jigsaw hypothesis. Med Hypotheses (2006) 67:860–4. doi: 10.1016/j.mehy.2006.03.046
222. Di Domenico EG, Cavallo I, Bordignon V, Prignano G, Sperduti I, Gurtner A, et al. Inflammatory cytokines and biofilm production sustain Staphylococcus aureus outgrowth and persistence: a pivotal interplay in the pathogenesis of Atopic Dermatitis. Sci Rep. (2018) 8:9573. doi: 10.1038/s41598-018-27421-1
223. Cavallo I, Di Domenico EG. Rapid profiling of Borrelia burgdorferi biofilm by the clinical Biofilm Ring Test®. In: Italian National Congress, Gruppo italiano per lo studio della Malattia di Lyme. (2018) Milan (Accessed June 9, 2018).
Keywords: Borrelia, lyme, neuroborreliosis, biofilm, skin, erythema migrans
Citation: Di Domenico EG, Cavallo I, Bordignon V, D'Agosto G, Pontone M, Trento E, Gallo MT, Prignano G, Pimpinelli F, Toma L and Ensoli F (2018) The Emerging Role of Microbial Biofilm in Lyme Neuroborreliosis. Front. Neurol. 9:1048. doi: 10.3389/fneur.2018.01048
Received: 29 September 2018; Accepted: 19 November 2018;
Published: 03 December 2018.
Edited by:
Matilde Inglese, Icahn School of Medicine at Mount Sinai, United StatesReviewed by:
Manola Comar, University of Trieste, ItalyCopyright © 2018 Di Domenico, Cavallo, Bordignon, D'Agosto, Pontone, Trento, Gallo, Prignano, Pimpinelli, Toma and Ensoli. This is an open-access article distributed under the terms of the Creative Commons Attribution License (CC BY). The use, distribution or reproduction in other forums is permitted, provided the original author(s) and the copyright owner(s) are credited and that the original publication in this journal is cited, in accordance with accepted academic practice. No use, distribution or reproduction is permitted which does not comply with these terms.
*Correspondence: Enea Gino Di Domenico, ZW5lYS5kaWRvbWVuaWNvQGlmby5nb3YuaXQ=
Disclaimer: All claims expressed in this article are solely those of the authors and do not necessarily represent those of their affiliated organizations, or those of the publisher, the editors and the reviewers. Any product that may be evaluated in this article or claim that may be made by its manufacturer is not guaranteed or endorsed by the publisher.
Research integrity at Frontiers
Learn more about the work of our research integrity team to safeguard the quality of each article we publish.