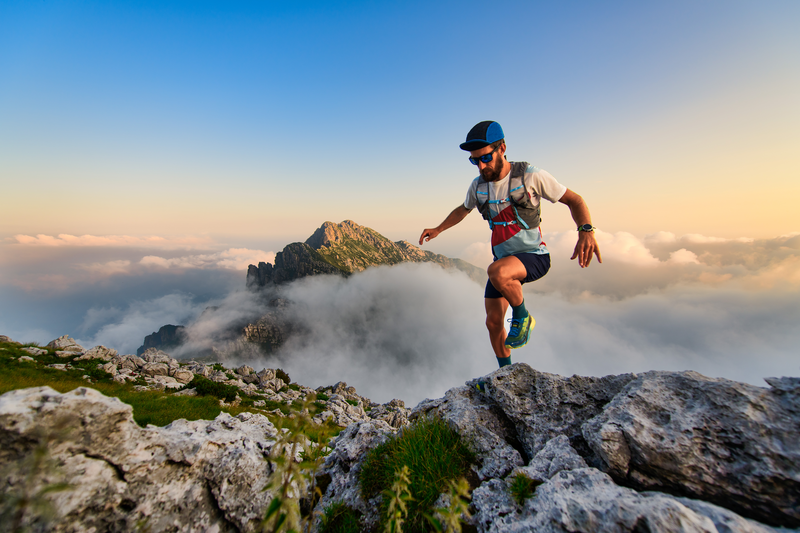
94% of researchers rate our articles as excellent or good
Learn more about the work of our research integrity team to safeguard the quality of each article we publish.
Find out more
ORIGINAL RESEARCH article
Front. Neurol. , 25 July 2018
Sec. Applied Neuroimaging
Volume 9 - 2018 | https://doi.org/10.3389/fneur.2018.00550
Background: Changes of cerebral diffusivity detected by magnetic resonance imaging (MRI) have been reported in epilepsy. Diffusion weighted imaging (DWI) detects changes in the distribution of water molecules by measuring the apparent diffusion coefficient (ADC) and is mainly used in the diagnosis of ischemic stroke. DWI changes in epilepsy were reported in status epilepticus (SE) or series of seizures. It remains unclear whether this phenomenon also occurs after single seizures. Accordingly, possible pathomechanisms have only been discussed on the presumed basis of ongoing epileptic brain activity.
Methods: In this retrospective study, we systematically analyzed DWI alterations related to epileptic seizures in 454 patients who received MRI scanning within the first 24 h after seizure onset.
Results: DWI restrictions not classified as ischemic stroke were observed in 18 patients (4%). We found DWI restrictions in 19% of patients with SE/seizure series and in 3% of patients after single focal and 2.5% after single generalized seizures. 17 patients with DWI alterations were diagnosed with a structural epilepsy. DWI signal decreased in the majority of patients within the first days and could not be detected in follow-up imaging >3 months. In all patients except one, DWI alterations were detected in the same hemisphere as the lesion. In the case of seizure series or SE, DWI restrictions mostly presented with a typical “garland-like” pattern alongside the cortical band or on the border of a defined lesion, while in isolated seizures, the restrictions were often rather subtle and small.
Discussion: We show that DWI restrictions can be observed in patients after single epileptic seizures. As the vast majority of these patients was diagnosed with an epilepsy due to structural cerebral pathology, DWI restriction may reflect a higher vulnerability in these regions. This might also explain the fact that diffusivity changes were observed after single focal seizures as well as after multiple seizures or SE. The occurence itself on one side as well as the spatial pattern of this phenomenon on the other may thus not only be related to the duration of ictal activity, but to structural pathology.
Epilepsy is a clinical condition characterized by recurrent transient seizures due to abnormal synchronous and/or excessive neuronal activity of cortical neurons. Patients experiencing a seizure may present with motoric, sensoric or cognitive symptoms.
Since it was first described in 1929 by Hans Berger, the electroencephalogram (EEG) has been used as the main diagnostic tool to detect and localize epileptic neuronal activity. In recent years, magnetic resonance imaging (MRI) has become more and more important in the diagnostic workup of epilepsy patients. In clinical routine, imaging techniques are mainly used in order to search for structural cortical abnormalities or lesions representing possible epileptogenic foci. Yet, not only structural, but also functional changes associated with epileptic brain activity can be visualized by neuroimaging. In 1933, Penfield described for the first time hemodynamic changes associated with seizures in an angiographic study (1). Since, hemodynamic alterations in epilepsy patients have been reported in several studies using different techniques such as perfusion MRI (2), blood oxygenation level-dependent (BOLD) MRI (3) and single photon emission tomography (SPECT) (4). As MRI techniques became more advanced in the course of the last few years, apart from hemodynamic changes, diffusion-weighted MRI (DWI) became a new focus of scientific interest. DWI detects changes in the distribution of water molecules in the brain by measuring the apparent diffusion coefficient (ADC). It is a sensitive technique to study the molecular motion of water, i.e. water diffusivity, which represents an intrinsic characteristic of tissues (5). In clinical routine, DWI is mainly used in the diagnosis of acute ischemic stroke, but also brain tumors, cerebral abscesses etc. As for its application in epilepsy, some older and more recent case reports (6–10) and smaller studies report changes in DWI associated with epileptic brain activity. In 2011, Chatzikonstantinou et al. (11) published a prospective series of 54 selected patients with DWI alterations related to status epilepticus. They describe hippocampal DWI abnormalities in the majority of patients and EEG changes that correlated with lateralization of DWI abnormalities. No correlation between DWI changes and clinical features could be observed. In contrast, Rennebaum et al. (12) reported on a series of 19 out of 69 cases with cortical and thalamic DWI restrictions in patients with status epilepticus (SE) who presented more often with quantitative disorder of consciousness compared to patients without DWI abnormalities. The first prospecitve study on patients with SE or seizure series was published by Jabeen et al. in 2017 (13), who observed DWI abnormalities in 20 out of 30 patients. To the best of our knowledge, apart from these studies, no larger series of patients with DWI alterations associated with epileptic brain acitivity have been published so far. Thus, many questions remain unanswered. In particular, DWI alterations have almost exclusively been observed in patients with series of seizures or SE, whereas it remains unclear whether this phenomenon also occurs in patients after single focal or generalized seizures. Accordingly, possible pathomechanisms have only been discussed on the presumed basis of an ongoing epileptic brain activity.
Another question is whether specific patient groups like patients with existing brain lesions as a cause of their epilepsy are more prone to diffusivity changes then patients with other forms of epilepsy.
To adress these questions, we performed a retrospective study on a large cohort of 454 patients with acute epileptic seizures or SE who received an MRI within the first 24 h after seizure onset.
We studied a collective of 454 patients who presented at the Department of Neurology, Ulm University Hospital, Germany, between June 2009 and March 2013 with an acute (i.e., within the last 24 h prior to admission) epileptic seizure (focal, generalized or secondary generalized), a series of seizures (i.e., ≥ 2 seizures within the last 24 h) or SE and who received an MRI scan within the first 24 h after or during epileptic symptoms. The study was approved by the ethics committee of the University of Ulm (Application No. 237/15).
We recorded seizure semiology and type of epilepsy syndrome according to the revised terminology of the International League Against Epilepsy (14). Diagnosis in the individual patient was based on clinical findings, anamnestic information and EEG findings. SE was defined as >5 min of ongoing seizure activity for generalized tonic-clonic seizures (GTCS, GSE) (15–17) and >20 min for focal seizures without impairment of consciousness or awareness (FACS, FACSE) or dyscognitive focal seizures (FICS, FICSE) or as a series with two or more seizures without full clinical recovery in between (17, 18). A series of seizures was defined as two or more seizures in the last 24 h prior to admission with full recovery in between.
MRI was performed on a 1.5 Tesla MRI system (TIM Symphony, Siemens, Germany). With a 5 mm slice thickness, standardized T2 (repetition time (TR) 4,000–6,000 ms, time to echo (TE) 102 ms), T1 (TR 573 ms, TE 12 ms), fluid attenuated inversion recovery (FLAIR, TR 6180 ms, TE 112), T2* (TR 800–1,000 ms, TE 24 ms) and DWI (TR 4,000–6,000 ms, TE 100 ms) were acquired. ADC maps were obtained from DWI. All MRI data sets were obtained in the same orientation and slice positions. We recorded all findings in the first MRI performed after seizure activity, as well as on subsequent MRIs at our department and the time between seizure onset and first MRI. DWI abnormalities were only accepted if a high contrast to background was detectable, or a corresponding reduction of ADC was present and artifacts could be excluded. Furthermore, DWI alterations were only classified as seizure-related when they were cortical or localized in adjacent subcortical areas and not respecting vascular territories or when they were restricted to the hippocampal area or pulvinar thalami.
EEG was performed with a standard 10–20 system (21 electrodes) over 15 to 30 min using a digital EEG-acquisition and analysing system (Natus, Germany) and evaluated by a board-certified neurologist. Frontal, temporal, parietal and occipital regions were studied in each patient. Artifacts were identified by visual inspection. Localization of abnormal EEG patterns was identified by the electrodes over which the patterns were maximally visible, i.e., highest amplitudes in referential derivations or phase inversion in bipolar derivations (19).
Statistical analyses were carried out using IBM SPSS Statistics21.0 (IBM Corporation, Armonk, New York, USA). We compared the total group of patients with peri-ictal DWI restriction (n = 18) with the total group without DWI restriction (n = 436) using the Chi-square test (or Fisher's exact test). A statistical significance was assumed if p < 0.05.
In total, we identified 18 out of 454 patients (4.0%) with DWI abnormalities associated with epileptic symptoms (4 males, 14 females, mean age 71.1 years). Six patients in this group (33.3%) presented with a SE (five focal, one generalized) and one patient (5.6%) with a series of focal seizures leading to admission. Five patients were diagnosed with a single GTCS (27.8%), six with single focal seizures (33.3%). As for etiology, 17 patients (94.4%) were diagnosed with structural epilepsy, one patient with epilepsy of unknown etiology. Clinical, EEG and MRI data of the patients with DWI alterations are summarized in Table 1. Twelve patients in this group received a follow-up MRI, eight within the first 7 days after seizure or SE and four within 3–31 months (see Table 1). In the majority of patients, DWI restrictions decreased significantly or dissolved completely during the first days after epileptic activity. None of the patients who received a follow-up after 3 months or later exhibited DWI changes any more.
Table 1. Clinical, EEG, and MRI characteristics of patients with DWI reductions after seizure or SE.
From the 436 patients without DWI restriction, the majority was diagnosed with a structural epilepsy (336 patients in total, 77.1%). Yet, this percentage was smaller then in the patient group with DWI restriction, although this difference did not reach statistical significance (p = 0.19). Only 25 patients in total were diagnosed with a genetic epilepsy (5.7%), and 44 patients with an epilepsy of unknown origin (10.1%). 20 patients presented with a situation-related seizure due to alcohol withdrawal and seven with a situation-related seizure due to other causes (hypoglycaemia, substance abuse, electrolyte imbalance). Four patients presented with a single occasional convulsion. The percentage of women was higher in the DWI-restriction-group with 14 female vs. only four male patients here, whereas in the non-DWI-restriction group, sex distribution was even (218 male vs. 218 female). Patients with DWI restriction were slightly older (mean age, 64.5 ± 19.9 years in the group without DWI restriction vs. 71.1 ± 14.1 years in the group with DWI restriction).
Two hundred and fifteen patients in total (47.4%) were diagnosed with a single focal seizure, six of which (2.8%) showed a DWI restriction on MRI. From 201 patients who presented with a single generalized seizure, five patients (2.5%) showed DWI abnormalities and from 23 patients with a series of GTCS or GSE, DWI restriction was observed in one patient (4.3%). Nine patients presented with a focal SE, five of them (55.6%) showing alterations in the DWI sequence. That is, from 32 patients with a series of seizures or focal or generalized SE, 18.8% (six patients) showed a DWI restriction.
In six patients without DWI restriction, exact seizure semiology could not be determined retrospectively.
In three patients, a localized post-ischemic gliosis was identified as the presumed cause of epileptic activity. In these patients, DWI restriction was observed in the same area as the structural pathology. The same was the case for two patients with brain tumors and one patient with limbic encephalitis, who showed DWI alteration in the left temporal lobe and hippocampus. One patient showed post-ischemic gliosis in both hemispheres, but DWI restriction was localized on the left hemisphere (temporal hippocampal, parietal and thalamic) only. In patients with generalized bi-hemispheric pathology, like subcortical vascular encephalopathy (SVE) or cerebral amyloid angiopathy (CAA), DWI restriction was usually localized in one hemisphere in a single subject, but varied in-between subjects without preponderance of the left or right hemisphere. One patient with bilateral cysticercosis showed DWI changes only in the right thalamus. Patients with SE or seizure series mostly showed a typical “garland-like” pattern alongside the cortical band or on the border of a defined lesion, sometimes also thalamic (Figure 1). By contrast, patients with single focal seizures showed rather subtle and smaller DWI restriction patterns (Figure 2).
Figure 1. Examples for DWI restrictions in two patients with series of focal seizures. T2w–T2 weighted imaging.
Figure 2. Examples for DWI restrictions in three patients with single focal seizures including follow-up MRI in patients (b) and (c). T2w – T2 weighted imaging.
In this large retrospective study, we systematically analyzed DWI alterations related to epileptic seizures in a cohort of 454 patients who presented at a single center with epileptic seizures or SE and received MRI scanning within the first 24 h after seizure onset. DWI restrictions were observed in 4% of patients. In previous studies, changes of cerebral diffusivity as detected by MRI have been reported in epilepsy patients (11–13, 20, 21). Yet, DWI changes in epilepsy were described to be almost exclusively related to focal or generalized SE or series of seizures. However, in our unselected patient collective, we found DWI alterations not only in 56% of patients with focal SE or series of focal seizures and in 19% of all patients with SE, but also in 3% of patients after single focal seizures and 2.5% of patients after single GTCS. While in the case of SE patients, this percentage is in accordance with two large previous series, where DWI alterations were observed in 28 and 67% of patients (12, 13), MRI alterations in patients with a single focal or generalized seizure seem to be more common than assumed until now.
Several different pathomechanisms have been discussed to explain changes in brain diffusivity related to epileptic activity: one possible reason could be an energy deficit of the Na+/K+-ATPase pump after and during seizures, resulting in increased net uptake of sodium from extracellular to intracellular compartments, which is then followed by water influx into the cell, leading to cell swelling and cytotoxic edema (22). This in turn leads to decreased ADC and DWI restriction in the region of the epileptogenic focus. A failure of the Na+/K+-ATPase pump has in a similar way been shown in ischemic stroke (23). One other possible mechanism leading to focal cell damage may be an increase of calcium influx resulting in toxic calcium levels. This influx is due to an excessive release of excitatory neurotransmitters such as glutamate (24) during ictal activity. However, there might be a common mechanism shared with ischemic pathophysiology, i.e., terminal depolarization with the difference being that it is not terminal in ictal etiology. Furthermore, clinical experience as well as studies [e.g. (25)] indicate that DWI restrictions are less pronounced under ictal than under ischemic conditions. In this regard, one limitation of our study is the fact that we did not measure the amount of ADC reduction in quantitative values. In the chronic phase (1–14 days after seizure activity) gliosis and neural cell death could lead to increased interstitial water resulting in ADC-/DWI-alterations (26). In all cases, cellular alterations leading to imaging changes remain mainly focal, and thus, DWI alterations have even been proposed as a tool to localize the center of ictal activity (20, 21, 27). In previous studies, DWI restrictions were often observed in the temporal lobe (12), especially in the hippocampus (11). Some patients, however, showed DWI alterations in the thalamus (12, 13). In our group, we saw a widespread pattern of cerebral diffusivity changes with no clear preponderance of e.g., the temporal lobe or hippocampal structures. In addition, DWI restrictions after single seizures did not show the classical “garland-like” pattern alongside the cortical band, as it has been described before in patients with SE, but were usually much smaller and often more difficult to detect.
The observed frequency of approximately 3% postictal DWI findings after single seizures gives rise to the advice that other relevant etiologies have to be ruled out before marking them as postictal in order not to delay specific therapies of other etiologies, especially ischemic stroke.
In over 90% of patients who showed a DWI restriction, a structural brain pathology was identified as the cause of the ictal activity. This percentage was significantly higher than in the group of patients without DWI abnormalities. The lateralization pattern of DWI restriction correlated well with the pattern of structural cerebral pathology. This finding has been described before in patients with SE (8, 11, 21). The fact that these structural lesions are not necessarily located in the hippocampus or temporal lobe only could explain the fact that we saw a widespread pattern of DWI changes in our patient group, affecting cortical regions of all lobes in a similar proportion.
In conclusion, our study shows that a phenomenon which has been well-known in patients with prolonged seizure activity can also be observed after single seizures, and should not be mistaken for cerebral stroke, as it does not show the same temporal and spatial characteristics.
AH, MS, and EP reviewed MRI data. KT collected clinical data. AH wrote the manuscript. MS, JK, SF, EP, and AL reviewed the manuscript.
The authors declare that the research was conducted in the absence of any commercial or financial relationships that could be construed as a potential conflict of interest.
1. Penfield W. The evidence for a cerebral vascular mechanism in epilepsy. Ann Intern Med. (1933) 7:303–10.
2. Warach S, Levin JM, Schomer DL, Holman BL, Edelman RR. Hyperperfusion of ictal seizure focus demonstrated by MR perfusion imaging. AJNR Am J Neuroradiol. (1994) 15:965–8.
3. Detre JA, Sirven JI, Alsop DC, O'Connor MJ, French JA. Localization of subclinical ictal activity by functional magnetic resonance imaging: correlation with invasive monitoring. Ann Neurol. (1995) 38:618–24.
4. Markand ON, Salanova V, Worth R, Park HM, Wellman HN. Comparative study of interictal PET and ictal SPECT in complex partial seizures. Acta Neurol Scand. (1997) 95:129–36.
5. Grant PE, He J, Halpern EF, Wu O, Schaefer PW, Schwamm LH, et al. Frequency and clinical context of decreased apparent diffusion coefficient reversal in the human brain. Radiology (2001) 221:43–50. doi: 10.1148/radiol.2211001523
6. Wieshmann UC, Symms MR, Shorvon SD. Diffusion changes in status epilepticus. Lancet (1997) 350:493–4.
7. Diehl B, Najm I, Ruggieri P, Foldvary N, Mohamed A, Tkach J, et al. Periictal diffusion-weighted imaging in a case of lesional epilepsy. Epilepsia (1999) 40:1667–71.
8. Lansberg MG, O'Brien MW, Norbash AM, Moseley ME, Morrell M, Albers GW. MRI abnormalities associated with partial status epilepticus. Neurology (1999) 52:1021–7.
9. Kim JA, Chung JI, Yoon PH, Kim DI, Chung TS, Kim EJ, et al. Transient MR signal changes in patients with generalized tonicoclonic seizure or status epilepticus: periictal diffusion-weighted imaging. AJNR Am J Neuroradiol. (2001) 22:1149–60.
10. Matsuda T, Shimakawa S, Toshikawa H, Hatanaka M, Fukui M, Mori A, et al. Hippocampal signal abnormality on the first day of illness in acute encephalopathy with biphasic seizures and late reduced diffusion caused by HHV-6 infection. Brain Dev. (2016) 38:943–6. doi: 10.1016/j.braindev.2016.05.001
11. Chatzikonstantinou A, Gass A, Forster A, Hennerici MG, Szabo K. Features of acute DWI abnormalities related to status epilepticus. Epilepsy Res. (2011) 97:45–51. doi: 10.1016/j.eplepsyres.2011.07.002
12. Rennebaum F, Kassubek J, Pinkhardt E, Hubers A, Ludolph AC, Schocke M, et al. Status epilepticus: clinical characteristics and EEG patterns associated with and without MRI diffusion restriction in 69 patients. Epilepsy Res. (2016) 120:55–64. doi: 10.1016/j.eplepsyres.2015.12.004
13. Jabeen SA, Cherukuri P, Mridula R, Harshavardhana KR, Gaddamanugu P, Sarva S, et al. A prospective study of diffusion weighted magnetic resonance imaging abnormalities in patients with cluster of seizures and status epilepticus. Clinical Neurol Neurosurg. (2017) 155:70–4. doi: 10.1016/j.clineuro.2017.02.013
14. Berg AT, Berkovic SF, Brodie MJ, Buchhalter J, Cross JH, van Emde Boas W. Revised terminology and concepts for organization of seizures and epilepsies: report of the ILAE Commission on Classification and Terminology, 2005–2009. Epilepsia (2010) 51:676–85. doi: 10.1111/j.1528-1167.2010.02522.x
15. Wasterlain CG, Chen JW. Mechanistic and pharmacologic aspects of status epilepticus and its treatment with new antiepileptic drugs. Epilepsia (2008) 49(Suppl. 9):63–73. doi: 10.1111/j.1528-1167.2008.01928.x
16. Chen JW, Wasterlain CG. Status epilepticus: pathophysiology and management in adults. Lancet Neurol. (2006) 5:246–56. doi: 10.1016/S1474-4422(06)70374-X
17. Dupont S, Crespel A. Satus epilepticus: epidemiology, definitions and classifications. Rev Neurol. (2009) 165:307–14. doi: 10.1016/j.neurol.2008.11.010
18. Meierkord H, Holtkamp M. Non-convulsive status epilepticus in adults: clinical forms and treatment. Lancet Neurol. (2007) 6:329–39. doi: 10.1016/S1474-4422(07)70074-1
19. Ebersole JS, Husain AM, Nordli DRE. Current Practice of Clinical Electroencephalograhy. 4th Edn. Philadelphia, PA: Wolters Kluewer. (2014).
20. Szabo K, Poepel A, Pohlmann-Eden B, Hirsch J, Back T, Sedlaczek O, et al. Diffusion-weighted and perfusion MRI demonstrates parenchymal changes in complex partial status epilepticus. Brain J Neurol. (2005) 128(Pt 6):1369–76. doi: 10.1093/brain/awh454
21. Di Bonaventura C, Bonini F, Fattouch J, Mari F, Petrucci S, Carni M, et al. Diffusion-weighted magnetic resonance imaging in patients with partial status epilepticus. Epilepsia (2009) 50(Suppl. 1):45–52. doi: 10.1111/j.1528-1167.2008.01970.x
22. Wang Y, Majors A, Najm I, Xue M, Comair Y, Modic M, et al. Postictal alteration of sodium content and apparent diffusion coefficient in epileptic rat brain induced by kainic acid. Epilepsia (1996) 37:1000–6.
23. Mintorovitch J, Yang GY, Shimizu H, Kucharczyk J, Chan PH, Weinstein PR. Diffusion-weighted magnetic resonance imaging of acute focal cerebral ischemia: comparison of signal intensity with changes in brain water and Na+,K+-ATPase activity. J Cereb Blood Flow Metab. (1994) 14:332–6.
24. Verheul HB, Balazs R, Berkelbach van der Sprenkel JW, Tulleken CA, Nicolay K, van Lookeren Campagne M. Temporal evolution of NMDA-induced excitoxicity in the neonatal rat brain measured with 1H nuclear magnetic resonance imaging. Brain Res. (1993) 618:203–12.
25. Bartsch T, Dohring J, Reuter S, Finke C, Rohr A, Brauer H, et al. Selective neuronal vulnerability of human hippocampal CA1 neurons: lesion evolution, temporal course, and pattern of hippocampal damage in diffusion-weighted MR imaging. J Cereb Blood Flow Metab. (2015) 35:1836–45. doi: 10.1038/jcbfm.2015.137
26. Yu JT, Tan L. Diffusion-weighted magnetic resonance imaging demonstrates parenchymal pathophysiological changes in epilepsy. Brain Res Rev. (2008) 59:34–41. doi: 10.1016/j.brainresrev.2008.04.008
Keywords: magnetic resonance imaging, diffusion weighted imaging, epilepsy, seizure, status epilepticus
Citation: Hübers A, Thoma K, Schocke M, Fauser S, Ludolph AC, Kassubek J and Pinkhardt EH (2018) Acute DWI Reductions In Patients After Single Epileptic Seizures - More Common Than Assumed. Front. Neurol. 9:550. doi: 10.3389/fneur.2018.00550
Received: 26 March 2018; Accepted: 19 June 2018;
Published: 25 July 2018.
Edited by:
Achim Gass, Universitätsklinikum Mannheim, GermanyReviewed by:
Christian Herweh, Universitätsklinikum Heidelberg, GermanyCopyright © 2018 Hübers, Thoma, Schocke, Fauser, Ludolph, Kassubek and Pinkhardt. This is an open-access article distributed under the terms of the Creative Commons Attribution License (CC BY). The use, distribution or reproduction in other forums is permitted, provided the original author(s) and the copyright owner(s) are credited and that the original publication in this journal is cited, in accordance with accepted academic practice. No use, distribution or reproduction is permitted which does not comply with these terms.
*Correspondence: Annemarie Hübers, YW5uZW1hcmllLmh1ZWJlcnNAZ214Lm5ldA==
Disclaimer: All claims expressed in this article are solely those of the authors and do not necessarily represent those of their affiliated organizations, or those of the publisher, the editors and the reviewers. Any product that may be evaluated in this article or claim that may be made by its manufacturer is not guaranteed or endorsed by the publisher.
Research integrity at Frontiers
Learn more about the work of our research integrity team to safeguard the quality of each article we publish.