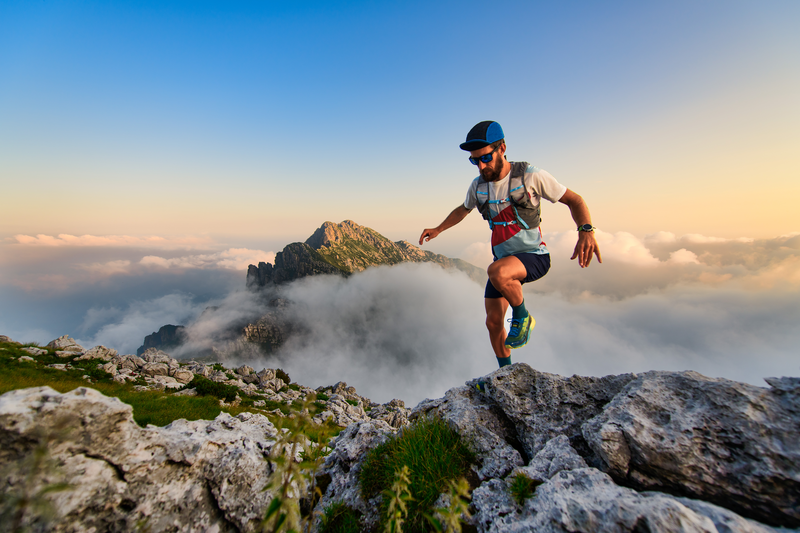
94% of researchers rate our articles as excellent or good
Learn more about the work of our research integrity team to safeguard the quality of each article we publish.
Find out more
METHODS article
Front. Neurol. , 05 July 2018
Sec. Neuro-Otology
Volume 9 - 2018 | https://doi.org/10.3389/fneur.2018.00543
This article is part of the Research Topic Vestibular Evoked Myogenic Potential - Beyond a Simple Test of Otolith Function View all 5 articles
Background: Cervical VEMPs and ocular VEMPs are tests for evaluating otolith function in clinical practice. We developed a simple, portable and affordable device to record VEMP responses on patients, named μVEMP. Our aim was to validate and field test the new μVEMP device.
Methods: We recorded cervical VEMPs and ocular VEMPs in response to bone conducted vibration using taps tendon hammer to the forehead (Fz) and to air conducted sounds using clicks. We simultaneously recorded VEMP responses (same subject, same electrode, same stimuli) in three healthy volunteers (2 females, age range: 29–57 years) with the μVEMP device and with a standard research grade commercial (CED) system used in clinics. We also used the μVEMP device to record VEMP responses from six patients (6 females, age mean±SD: 50.3 ± 20.8 years) with classical peripheral audio-vestibular diseases (unilateral vestibular neuritis, unilateral neurectomy, bilateral vestibular loss, unilateral superior canal dehiscence, unilateral otosclerosis).
Results: The first part of this paper compared the devices using simultaneous recordings. The average of the concordance correlation coefficient was rc = 0.997 ± 0.003 showing a strong similarity between the measures. VEMP responses recorded with the μVEMP device on patients with audio-vestibular diseases were similar to those typically found in the literature.
Conclusions: We developed, validated and field tested a new device to record ocular and cervical VEMPs in response to sound and vibration.This new device is portable (powered by a phone or tablet) with pocket-size dimensions (105 × 66 × 27 mm) and light weight (150 g). Although further studies and normative data are required, our μVEMP device is simpler (easier to use) and potentially more accessible than standard, commercially available equipment.
Vestibular-evoked myogenic potentials (VEMPs) are commonly used for clinical neurophysiological assessment of the vestibular system for patients with complaints of dizziness, vertigo, oscillopsia, imbalance, and falls. Cervical VEMPs (cVEMPs) and ocular VEMPs (oVEMPs) are tests for evaluating otolith function for research and in clinical practice (1, 2).
VEMPs are short latency reflexes elicited in response to vestibular stimulation: air-conducted sound (ACS) delivered via the external auditory canal, or bone-conducted vibration (BCV) using tendon hammer taps or a clinical vibrator on the head (3). In clinics, VEMPs are most commonly recorded using surface electromyography (EMG). Vestibular stimulation causes activation of afferent vestibular neurons. In oVEMPs, this neuronal activation induces a short latency crossed-reflex activation of the extra-ocular inferior oblique muscle. These myogenic potentials can be measured by surface electrodes positioned beneath the contralateral eye (4–7). In cVEMPs, myogenic potentials are measured from the ipsilateral sternocleidomastoid (SCM) muscle (8).
VEMPs have enabled the evaluation of the function of the otolithic organs. cVEMPs have been utilized for assessing the sacculo-collic reflex pathway and reflect the uncrossed inhibitory potentials originating from the saccule and the inferior vestibular nerve (8–10). oVEMPs evaluate the vestibulo-ocular reflex pathway and have been demonstrated to reflect the crossed excitatory potentials originating primarily from the utricle and the superior vestibular nerve (7).
Since the early VEMP paper published in 1994 (8), literature on VEMPs has increased and hundreds of papers have now been published. The use of VEMPs as a vestibular test providing information about otolithic function has gained a place in the standard vestibular test battery. VEMPs provide rapid and clinically useful information about the vestibular apparatus [for review see (11, 12)].
In this project, we developed, validated and field tested a new device to record VEMP responses on patients. This new device is mobile and portable because of its low weight and small dimensions. The usual laptop or desktop computer has been replaced by a smart phone or inexpensive tablet. The bio-amplifiers and data acquisition interface have been miniaturized to a pocket size device (105 × 66 × 27 mm, 150 g). We call this device μVEMP (“micro-VEMP” or “you-VEMP”) in reference to its small size, the typical response units (microvolts), and the focus on easy operation by “you.”
Our aim was to validate the data from the new μVEMP device and to evaluate its usability in the clinical environment on patients. To this end, we simultaneously recorded VEMPs on healthy subjects with the μVEMP device and with a commercially available standard system used at the Royal Prince Alfred teaching and research hospital. The first part of this paper shows the comparison between both devices. We also recorded VEMP responses from patients with classical peripheral audio-vestibular diseases.
The study was approved by the local ethics committee (Adventist HealthCare HREC 2017-021) and participants gave informed written consent according to the Declaration of Helsinki.
A picture of the μVEMP device is given in Figure 1 and a schematic block diagram is given in Supplementary Material 1 (Figure S1).
Figure 1. Picture of the new μVEMP device which consists of: (1) a smart phone (tablet, laptop or desktop computer); (2) a biopotential measurement device with five leads; (3) coaxial shielded cables, and “alligator” clips (for ECG electrodes); (4) a “smart” tendon hammer for bone-conducted vibration stimuli; (5) connection to generic peripheral ports; (6) audiometric headphones for air-conducted sound stimuli, and (7) a “smart” peripheral interface for auditory clicks.
Figure 1-1: A smart phone (Android OS), tablet (Windows 8 or 10), laptop or desktop computer is used for: user-interface, display, file storage, report generation, and networking. This connects to the μVEMP device using USB 2.0, USB OTG, Serial UART, Bluetooth or WIFI. In this study, VEMP responses were recorded with a Levono tablet running on Windows 8.
Figure 1-2: The μVEMP biopotential measurement device contains 4–8 individual, differential input, 24-bit simultaneous-sampling analog-to-digital converters (ADCs) with programmable gain and low noise (< 1 μV), an ARM Cortex-M4 core Microcontroller with DSP and FPU running at 96 MHz, and medical grade electrical isolation rated at 5 kV.
Figure 1-3: Standard ECG electrodes (not shown) are connected to the μVEMP device using coaxial shielded cables and “alligator” clips.
Figure 1-4: BCV stimulus is delivered using a “smart” tendon hammer with integrated 3-axis accelerometer. An ARM Cortex-M0 Microcontroller calculates resultant accelerations (omnidirectional), provides TTL triggering with programmable thresholds and provides operator feedback by driving a RGB led to indicate low (blue; 4–10 g) medium (green; 10–16 g) or high (red; over 16 g) nominal tap intensities.
Figure 1-5: The hammer is connected to one of the μVEMP peripheral ports for power, serial communications, and TTL trigger/ready signals.
Figure 1-6: ACS stimulus is delivered by audiometric headphones (DD52, 10 Ω ± 1.0 Ω, Rated Power: 1,000 mW, Frequency response: 100 Hz−8 kHz, Sensitivity: 108 ± 3 dB SPL@1000 Hz @ 1 mW, Harmonic distortion:Below 1% @120 dB SPL, f = 1 kHz).
Figure 1-7: A “smart” peripheral interface generates auditory click signals (square wave pulses) for 105.0 dB LAeq. The interface contains an ARM Cortex-M0 Microcontroller which is programmed with variables such as pulse duration and stimulus repetition rate, nominally 0.1 ms and 5.1 Hz respectively.
The μVEMP device is modular with multiple generic communication ports to allow connection to various combinations of “smart” (containing microcontrollers) peripheral devices such as: the BCV Fz taps, the ACS click generator, a loop back tester, an isolated external triggering interface (TTL in and out), remote controllers, wireless transceivers, human interface devices, displays, and other equipment.
These 6-pin ports carry power for the “smart” peripheral device, bidirectional communications (serial UART), and TTL signals to indicate states such as “trigger” and “busy.” These generic communication ports provide for flexible system configuration and additional functionality in the future but also simplify operation for the user because the μVEMP device can recognize connected peripherals (for example to switch desired test type) and control them automatically.
Circuit designs, PCB layouts, CAD modeling, 3D printing, firmware and software development were all conducted in our laboratory. The intensity of the air conducted stimuli was calibrated using a class 1 sound level meter (Type 2250), a condenser microphone (Type 4144), and an artificial ear (Type 4152) all from Brüel & Kjær (DK-2850 Nærum Denmark).
Simultaneous cVEMP and oVEMP recordings were performed on three healthy volunteers (2 females, age range: 29–57 years). The comparison device was a laboratory interface (Micro1401) and a bank of 1902 amplifiers coupled with Signal software (all from Cambridge Electronic Design Ltd (CED), Cambridge, United Kingdom).
The stimuli were ACS (clicks 0.1 ms, 105.0 dB LAeq, 5.1 Hz, ~100 repetitions) delivered to the right ear and BCV (taps, ~50 repetitions) applied to the forehead (Fz position). The stimuli were generated by the μVEMP device (for ACS clicks) and by the modified tendon hammer described above (for BCV Fz taps). The μVEMP device provided a TTL signal used to trigger the CED micro1401. In this way, the two machines could be synchronized: each tap with the hammer or each click with the headphone triggered data recording simultaneously on both devices.
cVEMPs were recorded from ECG surface electrodes (Cleartrace, Conmed Corp., Utica New York, USA) placed on the right, ipsilateral, SCM muscle. An active electrode was placed on the upper third part of the SCM muscle; a reference electrode was placed on the end of the scapula; and the “ground” was at the top of the sternum. oVEMPs were recorded from a pair of electrodes placed beneath the left, contralateral, eye. An active electrode was placed below the inferior eyeline; a reference electrode was placed below the active electrode; and the “ground” was at the top of the sternum. Negative potentials at the active electrodes were graphed as upward deflections.
Three cables were attached with alligator clips to each active and reference electrode. One cable provided data to the μVEMP device, and the other two provided data to two adjacent CED 1902 EMG amplifier channels with different filter settings. Two cables were attached to the ground electrode, one for each recording system. The main comparison was between the μVEMP data and the first CED channel (gain 1000), which were both recorded with filters switched off. The second CED channel had the usual clinical filters of 5 Hz−2 kHz and a gain of 3000. Data from this channel were used for development purposes and are not presented here. All channels recorded EMG with a sample rate of 4 kHz from 20 ms before to 80 ms post-stimulus. Data were exported from both systems as text files and analyzed offline. The unfiltered raw data for each frame (stimulus) were centered to zero by dividing each data point by the mean amplitude over the full 100 ms frame length, i.e., to remove the DC offset produced by the open filters. Frames (typically 100 for ACS clicks and 50 for BCV Fz taps) were then averaged to enable measurement of the response.
cVEMP responses consisted of two early waves: a first positive p13 wave and a second negative n23. oVEMP responses consisted of two early waves: a first negative n1 wave and a second positive p1. The latencies of these peaks were measured in ms from the stimulus onset. The peak to peak amplitude was calculated in microvolts (μV) from the unrectified EMG. For the cVEMPs only, amplitude was also expressed as a ratio of the background activity of the SCM muscle. EMG activity was full-wave rectified and averaged offline and measured during the 20 ms pre-stimulus period. Corrected amplitude was calculated as the peak to peak amplitude divided by the measure of muscle contraction. The evoked potential ratio (EPr) was calculated as a percentage by: (amplitude left–amplitude right)/(amplitude left + amplitude right)*100. An absolute value of the EP ratio greater than 30% was considered as abnormal (13, 14). A negative EP ratio indicated an amplitude smaller from the right ear. A positive EP ratio indicated an amplitude smaller from the left ear. For cVEMPs, the EP ratio was calculated with the corrected amplitude. For oVEMPs, the EP ratio was calculated with the peak to peak amplitude.
VEMPs from both devices were superimposed and the RMS error was calculated for both reflexes in response to ACS and BCV stimuli for the three volunteers (12 comparisons in total). The concordance correlation coefficient (rc) was also calculated.
cVEMPs and oVEMPs were recorded with the μVEMP device in six patients (6 females, age mean ± SD: 50.3 ± 2 0.8 years) with classical ear diseases. The age range and diagnosis of these patients are given in Table 1. VEMP stimuli used were the same as described above (ACS clicks and BCV Fz taps). The electrode montage was different than described above: cVEMPs and oVEMPs were recorded from the right and left ears. For cVEMPs, the active electrode was placed on the upper third part of both left and right SCM muscles; a reference electrode was placed on the top of the sternum; and the ground was on the forehead. For oVEMPs, the active electrode was placed below the inferior eyeline of both eyes; reference electrode was placed below the active electrode; and the ground was on the forehead.
All three subjects had present cVEMPs and oVEMPs in response to ACS clicks and BCV Fz taps. Separated (and superimposed) curves recorded with the μVEMP device and the CED system are given in Figure 2 (and Figure S2) for cVEMPs and Figure 3 (and Figure S3) for oVEMPs for the three subjects. The traces from both systems were strikingly similar. The average across all six instances (two stimulations, three subjects) of the RMS error of the simultaneous records was 1.86 ± 0.54 (min-max: 1.25–2.76) for cVEMPs and 0.40 ± 0.33 (min-max: 0.20–1.06) for oVEMPs. The average of the concordance correlation coefficient was rc = 0.997 ± 0.003 showing a strong similarity between VEMPs recorded with both systems.
Figure 2. Cervical VEMP responses from the right ear recorded simultaneously with the μVEMP device (red curves) and the standard CED device (blue curves) from the three healthy subjects in response to ACS clicks and BCV Fz taps. Notice the two curves have been offset by 100 μV for clarity and the difference between the two curves is shown (gray curves).
Figure 3. Ocular VEMP responses from the right ear recorded simultaneously with the μVEMP device (red curves) and the standard CED device (blue curves) from the three healthy subjects in response to ACS clicks and BCV Fz taps. Notice the two curves have been offset by 10 μV for clarity and the difference between the two curves is shown (gray curves).
Peak to peak amplitudes (raw and corrected), latencies, and EP ratio for the six patients are given in Table 2 for cVEMPs and Table 3 for oVEMPs. The healthy subject had similar, normal cVEMPs, and oVEMPs from both ears. The patient with vestibular neuritis showed normal cVEMPs but absent oVEMPs from the affected ear. The neurectomy for schwannoma removal patient showed no responses in cVEMPs and oVEMPs to both stimulations from the affected ear. The bilateral vestibular loss (BVL) patient had absent cVEMPs or oVEMPs to both stimulations from both ears. The superior canal dehiscence (SCD) patient showed normal cVEMP amplitudes and increased oVEMP amplitudes from the affected ear to both stimulations. The otosclerosis patient showed no responses to ACS stimulation from the affected ear to both cVEMPs and oVEMPs and normal responses to BCV Fz taps stimulation.
Since 2008 and the development of the first useable video head impulse test (vHIT) goggles, it is possible to assess the function of the six semicircular canals (15). Testing the remaining four otoliths end-organs still requires complicated and expensive equipment. In this project we developed a simple, portable and affordable VEMP device which we called the μVEMP (“micro-VEMP”). Similar to the strategy with the vHIT goggles, we employed recent advances in electronic component performance to miniaturize the VEMP device and simplified it by implementing only the minimum necessary features for effective testing. We recorded the traditional cVEMPs and oVEMPs in response to the simplest bone conducted vibration using taps and to air conducted sounds using clicks. Finally, the small size of the device makes it mobile and portable and so it could be used in new environments such as in emergency departments or outside the hospital.
To validate our new device, we simultaneously recorded VEMP responses in the same subjects, using the same electrodes and the same stimulation/recording parameters with the μVEMP and a standard commercially available device. Comparison showed very similar VEMP responses from both devices, validating our μVEMP device to record oVEMPs and cVEMPs. Responses recorded with the μVEMP device on patients with audio-vestibular diseases were the same as those typically reported in the literature for patients with unilateral vestibular neuritis (16, 17), unilateral neurectomy (14, 18), bilateral vestibular loss (19) and conductive hearing loss (20, 21). In a patient with superior canal dehiscence cVEMP and oVEMP amplitudes were enhanced, as expected (22–24), but we did not establish the threshold of responses. In order to interpret the results obtained on these patients, we used normative data for the EP ratio provided in the literature. Further studies are required to obtain normative data for our new μVEMP device.
One limitation of this intentionally simplified device is the range of stimuli available: VEMPs are induced with ACS clicks or BCV Fz taps only. Several other stimuli can be used to induce VEMP responses: ACS short tone bursts or BCV short tone bursts or square waves delivered with a mini-shaker (3). These other stimuli enable the vestibular system to be activated by stimuli with adjustable frequency content. It has been shown in the literature that different frequencies of the tone bursts may induce different responses in healthy subjects (25, 26) and in patients with SCD (27) or Meniere's Disease (28, 29). However, while the best stimulus frequency for inducing VEMP responses in healthy subjects may be around 500 Hz on average, many subjects or patients would better respond to an adjacent frequency [e.g., 250 or 1,000 Hz, (30)]. The optimal frequency is higher for older adults compared to young adults and for oVEMPs compared to cVEMPs (31, 30). As clicks have a broad frequency content with maximum energy between about 1 and 4 kHz (32, 33) they are suitable as a general VEMP stimulus. Another limitation of the μVEMP device is that ACS clicks stimulus amplitude cannot be adjusted by the operator (an intentional safety feature). In consequence, a threshold of response, often assessed in SCD or Meniere's patients (34, 35), cannot be evaluated with this device. These limitations have been intentionally imposed for hearing safety reasons, to minimize stimulus generation hardware and for our goal to produce a device that is as easy to use as possible (no frequencies or amplitudes to set).
In conclusion, we have developed and validated a new device to record oVEMPs and cVEMPs in response to sound and vibration. Although further studies and normative data are required for this new device, it is now possible and convenient to assess the function of all ten vestibular end-organs in about 20 min using simple and portable equipment including μVEMP.
HM developed the device and edited the manuscript. JH developed the device and edited the manuscript. SR recorded comparison data and edited the manuscript. EC recorded and analyzed the data and edited the manuscript.
HM is currently receiving a project grant from NHMRC of Australia and from the Garnett Passe and Rodney Williams Memorial Foundation. SR is funded by the NHMRC of Australia (GNT1104772).
HM is unpaid consultant to GN Otometrics. The remaining authors declare that the research was conducted in the absence of any commercial or financial relationships that could be construed as a potential conflict of interest. The handling Editor declared a shared affiliation, though no other collaboration, with the authors.
We gratefully acknowledge grant support from the NHMRC of Australia and from the Garnett Passe and Rodney Williams Memorial Foundation. We thank Mr. Leigh McGarvie for his help in simultaneous data recording and Dr. Payal Mukherjee for providing access to patients during her consultation.
The Supplementary Material for this article can be found online at: http://journal.frontiersin.org/article/10.3389/fneur.2018.00543/full#supplementary-material
Figure S1. Schematic block diagram of the μVEMP device.
Figure S2. Cervical VEMP responses from the right ear recorded simultaneously with the μVEMP device (red curves) and the standard CED device (blue curves) from the three healthy subjects in response to ACS clicks and BCV Fz taps. Notice the red curve (μVEMP) is nearly perfectly superimposed with the blue curve (CED).
Figure S3. Ocular VEMP responses from the right ear recorded simultaneously with the μVEMP device (red curves) and the standard CED device (blue curves) from the three healthy subjects in response to ACS clicks and BCV Fz taps. Notice the red curve (μVEMP) is nearly perfectly superimposed with the blue curve (CED).
ACS, air-conducted sound; ADCs, Analog to Digital Converters; Amp, Amplitude; BCV, bone-conducted vibration; BVL, bilateral vestibular loss; CED, Cambridge Electronic Design; cVEMPs, Cervical Vestibular Evoked Myogenic Potentials; dB, Decibels; ECG, Electro-Encephalogram; EMG, electromyography; EPr or EP ratio, Evoked Potential ratio; G, gravity; Hz, Hertz; Mm, millimeters; Msec or ms, milliseconds; oVEMPs, Ocular Vestibular Evoked Myogenic Potentials; PP, peak-to-peak; Rc, concordance correlation coefficient; RMS, Root Mean Square; SCD, Superior Canal Dehiscence; SCM, sternocleidomastoid; VEMPs, Vestibular Evoked Myogenic Potentials; vHIT, video-Head Impulse Test; VL, Vestibular loss; μV, microvolt; μVEMP, micro-Vestibular Evoked Myogenic Potentials.
1. Curthoys IS, Vulovic V, Burgess AM, Manzari L, Sokolic L, Pogson J, et al. Neural basis of new clinical vestibular tests: otolithic neural responses to sound and vibration. Clin Exp Pharmacol Physiol. (2014) 41:371–80. doi: 10.1111/1440-1681.12222
2. Colebatch JG, Rosengren SM, Welgampola MS. Vestibular-Evoked Myogenic Potentials. Handb Clin Neurol (2016) 137:133–55. doi: 10.1016/B978-0-444-63437-5.00010-8
3. Curthoys IS, Vulovic V, Burgess AM, Cornell ED, Mezey LE, Macdougall HG, et al. The basis for using bone-conducted vibration or air-conducted sound to test otolithic function. Ann N Y Acad Sci. (2011) 1233:231–41. doi: 10.1111/j.1749-6632.2011.06147.x
4. Kantner C, Gürkov R. Characteristics and clinical applications of ocular vestibular evoked myogenic potentials. Hear Res. (2012) 294:55–63. doi: 10.1016/j.heares.2012.10.008
5. Rosengren SM, McAngus, Todd NP, Colebatch JG. Vestibular-evoked extraocular potentials produced by stimulation with bone-conducted sound. Clin Neurophysiol. (2005) 116:1938–48. doi: 10.1016/j.clinph.2005.03.019
6. Todd NP, Rosengren SM, Aw ST, Colebatch JG. Ocular vestibular evoked myogenic potentials (ovemps) produced by air- and bone-conducted sound. Clin Neurophysiol. (2007) 118:381–90. doi: 10.1016/j.clinph.2006.09.025
7. Iwasaki S, McGarvie LA, Halmagyi GM, Burgess AM, Kim J, Curthoys IS et al. Head taps evoke a crossed vestibulo-ocular reflex. Neurology (2007) 68:1227–9. doi: 10.1212/01.wnl.0000259064.80564.21
8. Colebatch JG, Halmagyi GM, Skuse NF. Myogenic potentials generated by a click-evoked vestibulocollic reflex. J Neurol Neurosurg Psychiatry (1994) 57:190–7.
9. Uchino Y, Sato H, Sasaki M, Imagawa M, Ikegami H, Isu N, et al. Sacculocollic reflex arcs in cats. J Neurophysiol. (1997) 77:3003–12.
10. Uchino Y, Kushiro K. Differences between otolith- and semicircular canal-activated neural circuitry in the vestibular system. Neurosci Res. (2011) 71:315–27. doi: 10.1016/j.neures.2011.09.001
11. Rosengren SM, Welgampola MS, Colebatch JG. Vestibular evoked myogenic potentials: past, present and future. Clin Neurophysiol. (2010) 121:636–51. doi: 10.1016/j.clinph.2009.10.016
12. Rosengren SM, Kingma H. New perspectives on vestibular evoked myogenic potentials. Curr Opin Neurol. (2013) 26:74–80. doi: 10.1097/WCO.0b013e32835c5ef3
13. Welgampola MS, Colebatch JG. Vestibulocollic reflexes: normal values and the effect of age. Clin Neurophysiol. (2001) 112:1971–9. doi: 10.1016/S1388-2457(01)00645-9
14. Chiarovano E, Darlington C, Vidal PP, Lamas G, de Waele C. The Role of Cervical and Ocular Vestibular Evoked Myogenic Potentials in the Assessment of Patients with Vestibular Schwannomas. Edited by Matthew Thurtell. PLoS ONE (2014) 9:e105026. doi: 10.1371/journal.pone.0105026
15. MacDougall HG, Weber KP, McGarvie LA, Halmagyi GM, Curthoys IS. The video head impulse test: diagnostic accuracy in peripheral vestibulopathy. Neurology (2009) 73:1134–41. doi: 10.1212/WNL.0b013e3181bacf85
16. Govender S, Rosengren SM, Colebatch JG. Vestibular neuritis has selective effects on air- and bone-conducted cervical and ocular vestibular evoked myogenic potentials. Clin Neurophysiol. (2011) 122:1246–55. doi: 10.1016/j.clinph.2010.12.040
17. Shin BS, Oh SY, Kim JS, Kim TW, Seo MW, Lee H, et al. Cervical and ocular vestibular-evoked myogenic potentials in acute vestibular neuritis. Clin Neurophysiol. (2011) 123:369–75. doi: 10.1016/j.clinph.2011.05.029
18. Manzari L, Burgess AM, Curthoys IS. Effect of bone-conducted vibration of the midline forehead (Fz) in unilateral vestibular loss (uVL). Evidence for a new indicator of unilateral otolithic function. Acta Otorhinolaryngol Ital. (2010) 30:175. doi: 10.1016/S1388-2457(11)60029-1
19. Strupp M, Kim JS, Murofushi T, Straumann D, Jen JC, Rosengren SM. Bilateral vestibulopathy: diagnostic criteria consensus document of the classification committee of the bárány society. J Vestib Res. (2017) 27:177–89. doi: 10.3233/VES-170619
20. Tramontani O, Gkoritsa E, Ferekidis E, Korres SG. Contribution of vestibular-evoked myogenic potential (VEMP) testing in the assessment and the differential diagnosis of otosclerosis. Med Sci Monit. (2014) 20:205–13. doi: 10.12659/MSM.889753
21. Yang TL, Young YH. Vestibular-evoked myogenic potentials in patients with otosclerosis using air- and bone-conducted tone-burst stimulation. Otol Neurotol. (2007) 28:1–6. doi: 10.1097/01.mao.0000244367.62567.0d
22. Rosengren SM, Aw ST, Halmagyi GM, Todd NP, Colebatch JG. Ocular vestibular evoked myogenic potentials in superior canal dehiscence. J Neurol Neurosurg Psychiatry (2008) 79:559–68. doi: 10.1136/jnnp.2007.126730
23. Rosengren SM, Halmagyi GM, Colebatch JG. Vestibular hypersensitivity to sound in superior canal dehiscence: large evoked responses in the legs produce little postural sway. Clin Neurophysiol. (2008) 119:1674–82. doi: 10.1016/j.clinph.2008.03.021
24. Manzari L, Burgess AM, MacDougall HG, Curthoys IS. Enhanced otolithic function in semicircular canal dehiscence. Acta Oto Laryngol. (2011) 131:107–12. doi: 10.3109/00016489.2010.507780
25. Rosengren SM, Govender S, Colebatch JG. The relative effectiveness of different stimulus waveforms in evoking vemps: significance of stimulus energy and frequency. J Vestib Res. (2009) 19:33–40. doi: 10.3233/VES-2009-0345
26. Chihara Y, Iwasaki S, Fujimoto C, Ushio M, Yamasoba T, Murofushi T. Frequency tuning properties of ocular vestibular evoked myogenic potentials. Neuroreport (2009) 20:1491–5. doi: 10.1097/WNR.0b013e3283329b4a
27. Manzari L, Burgess AM, McGarvie LA, Curthoys IS. An indicator of probable semicircular canal dehiscence: ocular vestibular evoked myogenic potentials to high frequencies. Otolaryngol Head Neck Surg. (2013) 149:142–5. doi: 10.1177/0194599813489494
28. Kim-Lee Y, Ahn JH, Kim YK, Yoon TH. Tone burst vestibular evoked myogenic potentials: diagnostic criteria in patients with Ménière's Disease. Acta Oto Laryngol. (2009) 129:924–8. doi: 10.1080/00016480802495412
29. Winters SM, Berg IT, Grolman W, Klis SF. Ocular vestibular evoked myogenic potentials: frequency tuning to air-conducted acoustic stimuli in healthy subjects and Ménière's Disease. Audiol Neuro Otol. (2011) 17:12–9. doi: 10.1159/000324858
30. Taylor RL, Bradshaw AP, Halmagyi GM, Welgampola MS. Tuning characteristics of ocular and cervical vestibular evoked myogenic potentials in intact and dehiscent ears. Audiol Neuro-Otol. (2012) 17:207–18. doi: 10.1159/000336959
31. Piker EG, Jacobson GP, Burkard RF, McCaslin DL, Hood LJ. Effects of age on the tuning of the cVEMP and Ovemp. Ear Hear. (2013) 34:e65–73. doi: 10.1097/AUD.0b013e31828fc9f2
32. Hood LJ. Auditory brainstem response: estimation of hearing sensitivity. In: Katz editor. Handbook of Clinical Audiology, 7th Edn. Lippincott Williams & Wilkins (2015) p.249–66.
33. Burkard R. Sound pressure level measurement and spectral analysis of brief acoustic transients. Electroencephalogr Clin Neurophysiol. (1984) 57:83–91. doi: 10.1016/0013-4694(84)90010-5
34. Zhu Y, McPherson J, Beatty C, Driscoll C, Neff B, Eggers S, et al. Cervical VEMP Threshold Response Curve in the Identification of Ménière's Disease. J Am Acad Audiol. (2014) 25:278–88. doi: 10.3766/jaaa.25.3.7
Keywords: ocular VEMPs, cervical VEMPs, oVEMPs, cVEMPs, utricle, saccule, otoliths, vHIT
Citation: MacDougall HG, Holden J, Rosengren SM and Chiarovano E (2018) μVEMP: A Portable Interface to Record Vestibular Evoked Myogenic Potentials (VEMPs) With a Smart Phone or Tablet. Front. Neurol. 9:543. doi: 10.3389/fneur.2018.00543
Received: 30 March 2018; Accepted: 18 June 2018;
Published: 05 July 2018.
Edited by:
Aaron Camp, University of Sydney, AustraliaReviewed by:
Toru Seo, Kindai University, JapanCopyright © 2018 MacDougall, Holden, Rosengren and Chiarovano. This is an open-access article distributed under the terms of the Creative Commons Attribution License (CC BY). The use, distribution or reproduction in other forums is permitted, provided the original author(s) and the copyright owner(s) are credited and that the original publication in this journal is cited, in accordance with accepted academic practice. No use, distribution or reproduction is permitted which does not comply with these terms.
*Correspondence: Elodie Chiarovano, ZWxvZGllLmNoaWFyb3Zhbm9Ac3lkbmV5LmVkdS5hdQ==
Disclaimer: All claims expressed in this article are solely those of the authors and do not necessarily represent those of their affiliated organizations, or those of the publisher, the editors and the reviewers. Any product that may be evaluated in this article or claim that may be made by its manufacturer is not guaranteed or endorsed by the publisher.
Research integrity at Frontiers
Learn more about the work of our research integrity team to safeguard the quality of each article we publish.