- 1Department of Medicine, The University of British Columbia, Vancouver, BC, Canada
- 2Department of Pediatrics, The University of British Columbia, Vancouver, BC, Canada
- 3BC Children’s Hospital Research Institute, The University of British Columbia, Vancouver, BC, Canada
- 4Department of Family Practice, The University of British Columbia, Vancouver, BC, Canada
- 5Department of Family and Community Medicine, Providence Healthcare, Vancouver, BC, Canada
- 6Department of Biomedical Physiology and Kinesiology, Simon Fraser University, Burnaby, BC, Canada
Transient ischemic attack (TIA) and minor stroke have high risks of recurrence and deterioration into severe ischemic strokes. Risk stratification of TIA and minor stroke is essential for early effective treatment. Traditional tools have only moderate predictive value, likely due to their inclusion of the limited number of stroke risk factors. Our review follows Hans Selye’s fundamental work on stress theory and the progressive shift of the autonomic nervous system (ANS) from adaptation to disease when stress becomes chronic. We will first show that traditional risk factors and acute triggers of ischemic stroke are chronic and acute stress factors or “stressors,” respectively. Our first review shows solid evidence of the relationship between chronic stress and stroke occurrence. The stress response is tightly regulated by the ANS whose function can be assessed with heart rate variability (HRV). Our second review demonstrates that stress-related risk factors of ischemic stroke are correlated with ANS dysfunction and impaired HRV. Our conclusions support the idea that HRV parameters may represent the combined effects of all body stressors that are risk factors for ischemic stroke and, thus, may be of important predictive value for the risk of subsequent ischemic events after TIA or minor stroke.
Introduction
Transient ischemic attack (TIA) and minor ischemic stroke are two types of cerebrovascular ischemic events with mild or transient symptoms and non-disabling consequences (1, 2). However, they are markers of reduced cerebral blood flow and “warning signals” for the possible occurrence of severe ischemic strokes (2). TIA and minor stroke do, therefore, offer a unique opportunity to forestall the onset of permanent brain injury by initiating early treatment (3, 4). Guidelines recommend urgent treatment to TIA and minor stroke, which may reduce the volume of brain damaged by ischemia, promote recanalization of blocked vessels, and decrease the risk of severe ischemic stroke (5, 6). However, these treatments may generate safety concerns related to minor bleedings (7) and/or fatal intracranial hemorrhage (7, 8). Therefore, an urgent and precise risk stratification for TIA or minor stroke is of the utmost importance for medical caregivers to identify high-risk patients and provide personalized treatment. Current risk stratification tools include scoring systems and imaging techniques, all of which have their own individual limitations that inevitably reduce their clinical utility. Accordingly, currently, it remains a challenge to precisely and easily identify the risk of secondary ischemic events after TIA or minor stroke.
People who live with chronic stroke risk factors and suffer an acute TIA or minor stroke episode are at high risk of developing secondary ischemic events (9, 10). Each identified chronic risk factor and acute trigger of ischemic stroke is considered a source of stress for the body. Given the critical role of the autonomic nervous system (ANS) in regulating stress responses (11), it seems possible to determine the comprehensive effect of different stressors by assessing the status of their ANS function. This article is intended to address this issue by reviewing the existing knowledge and evidence and providing an evidence-based deduction on the association between ischemic stroke risk factors, stress, and ANS function in patients with TIA or minor stroke.
We first provide a description of ischemic stroke and TIA with focus on their risk factors and current risk stratification tools. We then present concepts related to stress and the stress response regulatory system, in particular the ANS, whose function can be assessed through the analyses of heart rate variability (HRV). We demonstrate the progressive shift from “stress adaptation” to “stress-related diseases,” with emphasis on the changes to the ANS response throughout this process. Finally, we review evidence in favor of an association between stroke risk factors and ANS dysfunction indexed by impaired HRV parameters.
Risk Stratification of TIA and Minor Stroke
Beyond all chronic risk factors, TIAs and minor stroke episodes provide additional risk information for secondary ischemic events. Johnston et al. determined that 90 days after emergency diagnosis of TIA, 428 of 1,707 patients (25.1%) developed adverse events including stroke, recurrent TIAs, cardiovascular hospitalization, and death (10). Moreover, Rothwell and Warlow showed that around 17% of ischemic strokes were preceded by a warning TIA; in 43% of cases, this warning TIA occurred within 1 week of the subsequent stroke (12). Although the stroke rate in the first 90 days after an initial event has dropped to 7–13% in recent randomized control trials (13), this overwhelming risk of severe ischemic events after initial TIA or minor stroke underscores the ongoing need for urgent evaluation and treatment of at-risk patients.
The development of secondary ischemic events is generally predicted by assessing the combination of several risk factors (6, 14). AHA/ASA guidelines (6, 14) propose a list of recognized chronic risk factors for ischemic stroke and TIA that are summarized in Table 1. Apart from the traditional chronic risk factors that predispose to the occurrence of ischemic stroke, some acute triggers may precipitate this process (15, 16) (Table 1). Several scoring systems have been widely used in clinical practice to evaluate the risk of early occurrence of severe ischemic stroke after TIA or minor stroke, including ABCD2 (A for age, B for blood pressure, C for clinical feature, D for duration of the symptoms, and another D for diabetes), ABCD3 (the presence of ≥2 TIA symptoms within 7 days added to the ABCD2 score), and ABCD3-I (the presence of abnormal findings on neuroimaging further added to the ABCD3 score). The predictive ability of ABCD2 score is only moderate with an area under the curve (AUC) between 0.55 and 0.7 (17, 18). This may be attributed to the difficulty to qualify the effect of risk factors on an individual basis due to intrapersonal heterogeneity. For instance, “diabetes” has different degrees of severity; and individuals have different ways of coping with the consequences of chronic metabolic stress. Furthermore, other important risk factors, such as smoking, obesity, sedentary life, and psychological stress, as well as other factors not yet identified, are not considered in the classic clinical assessment. Incorporation of the imaging assessment (ABCD3-I) improves the predictive power for future ischemic stroke after TIA or minor stroke, with AUC > 0.8 (19, 20). However, emergency imaging is costly and technology dependent, therefore affecting the universal use with subsequent delays to the scoring process and associated risk assessment. The limitation of current tools for personal risk prediction calls for the development of new valid, precise, and convenient tools to determine the risk of a secondary ischemic event after initial TIA or minor stroke and to direct appropriate medical care for affected patients.
One approach that seems promising is to consider that most stroke risk factors listed in Table 1 are also body stressors and therefore affect the ANS response. It may then be possible to determine the overall effect of all life stressors on the body in patients after TIA or minor stroke, by assessing their ANS function. The next section will present evidence of this association, using HRV parameters as markers of ANS function.
Stress, ANS, and Health
In the central construct of Selye’s stress theory (11, 21), the generalized definition of “stress” describes a state of threatened homeostasis (refers to the stability of physiological systems that maintain life) caused by any form of internal or external disturbing forces, or “stressors.” The person’s life experiences (i.e., the accumulation of stress experiences) contributes to building an idiosyncratic “stress profile” of the individual. This “stress profile,” measured at one specific time, includes both previous and current stress experiences: physical, physiological, psychological, and environmental (11, 22). The “stress response” or “adaptive response” is a counteracting force initiated to neutralize the effects of stressors and re-establish homeostasis. The stress response is a succession of processes that occur in response to the perception of stress by the brain (23, 24). One main stress regulatory system is the ANS, which plays a particularly critical role in modulating the stress response (25, 26).
The ANS is a part of the peripheral nervous system and regulates physiological processes without conscious control. The two major divisions of ANS comprise the sympathetic nervous system (SNS) and the parasympathetic nervous system (PNS). The ANS innervates most organs and controls important physiological and behavioral processes (27). In many physiological situations, the stress response is regulated by the complementary interaction of SNS and PNS. Activation of either SNS or PNS outflow is accompanied by the relative inhibition of the other, suggesting the concept of “sympathovagal balance” (27). The ANS dynamically controls the body response to a range of external and internal stimuli/stressors, providing physiological stability to the body (28). Typically, when the source of stress is acute [i.e., lasting for a period of minutes to hours (22)], the ANS, via sympathetic and parasympathetic branches, provides an instantaneous physiological/adaptive response that provokes immediate physiological state alterations through neural innervation of the target organs (24, 28). A typical example of stress response is the physiological inflammatory response (29). The anti-inflammatory response is mostly controlled by PNS, with synergistic input from the SNS (25). As illustrated by Tracey (25, 30), the cholinergic anti-inflammatory pathway represents the afferent branch of the neuronal reflex that modulates local inflammatory responses (25, 30). The efferent branch of the vagus nerve produces acetylcholine that effectively reduces the production of pro-inflammatory cytokines. In addition, both SNS and the humoral anti-inflammatory pathway are triggered, releasing stress hormones that include cortisol and catecholamines to elicit anti-inflammatory effects (25, 30). In this situation, the PNS and SNS act synergistically to control the stress response.
This short-term, tightly controlled regulatory response serves to preserve homeostasis. However, when the source of stress persists for days to months, it is considered to be a chronic stress (22). Chronic stressful conditions represent situations in which environmental demand exceeds the natural regulatory capacity of the body (31). Long-term exposure to these chronic stressors leads to a progressive dysfunctional ANS response to stress, and in particular, to a constrained PNS capacity to control the stress response (21, 32), which may lead to an anticipatory stress response (unpredictable) and a reduced control of the neuroendocrine reaction (uncontrollable) (29, 31). This progressive deterioration of the stress response provides a neuromodulation basis to understand the progression from “stress adaptation” to “stress-related disorders” (32, 33).
The established traditional risk factors of ischemic stroke, such as aging, diet, cigarette smoking, excessive alcohol consumption, and psychological stress, are typically chronic stressors that continuously and cumulatively affect the stress systems (ANS and hypothalamic–pituitary–adrenocortical (HPA) axis) (28). The affected neural stress systems will then produce excessive stress hormones such as catecholamines and cortisol, which affect the target tissues and cause various metabolic disorders, such as hypertension, hyperglycemia, and dyslipidemia (28). These metabolic disorders, acting as “secondary” stressors, may further impair the ANS function, creating new pathological cascades, which ultimately leads to cardiovascular and cerebrovascular complications (28). Such disease progression is illustrated in Figure 1. In other words, inappropriate responses to initial stress becomes the source of new stress, leading to a sustained negative cycle of mutual reinforcement toward the development of chronic conditions (28, 33). In this deregulated cascade, it is difficult to distinguish between causes and consequences. The stress system is to a large extent “nonspecific” and meant to interact with internal or external perturbations in a similar manner.
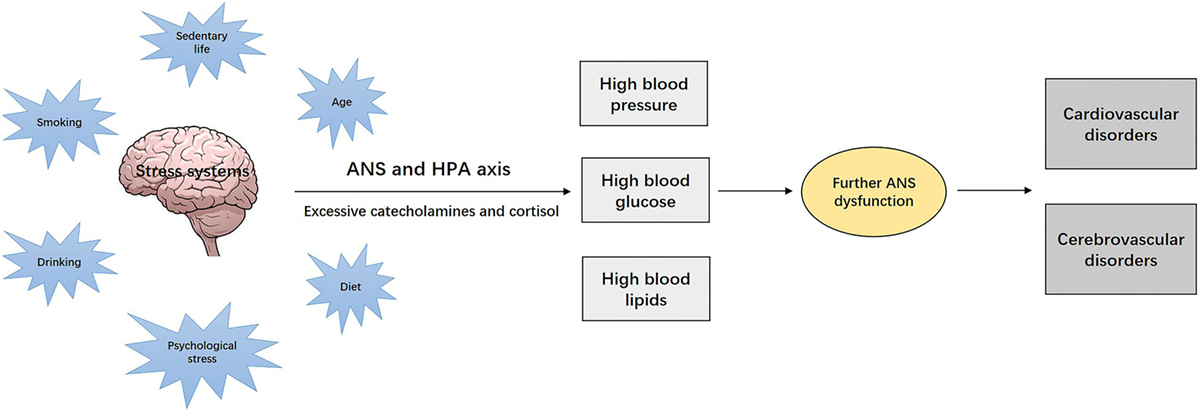
Figure 1. Chronic stress, the nervous system, and development of the stress-related disorders. Chronic stressors, such as aging, diet, cigarette smoking, alcohol consumption, and psychological stress, continuously and cumulatively affect the stress systems [autonomic nervous system (ANS) and hypothalamic–pituitary–adrenocortical (HPA) axis], which lead to excessive production of stress hormones such as catecholamines and cortisol. These stress hormones affect the target tissues and cause various metabolic disorders, such as hypertension, diabetes, and dyslipidemia, which act as “secondary” stressors, and may progressively impair ANS function and ultimately lead to cardiovascular and cerebrovascular diseases.
In this chronic stress situation, an additional acute event may extend the overall stress level beyond the range of the physiological and adaptive ANS response. In stroke research, the dynamic nature of stroke development follows such a pattern, in which the acute triggers for ischemic stroke (such as recent infections and TIA episodes) are seen as sources of acute stress to the body that superimpose their effects on the original chronic stress context; and this may increase the overall stress level to a new threshold that precipitates the occurrence of cerebrovascular ischemic events (15, 16, 34).
This framework supports the association between stress, ANS, and development of ischemic stroke as portrayed in Figure 2. Through the negative spiral described in Figure 2, progressive disease development leads to an accumulation of stress that affects the entire body, with ischemic stroke as the “final endpoint” of the overall effects of multiple stressors.
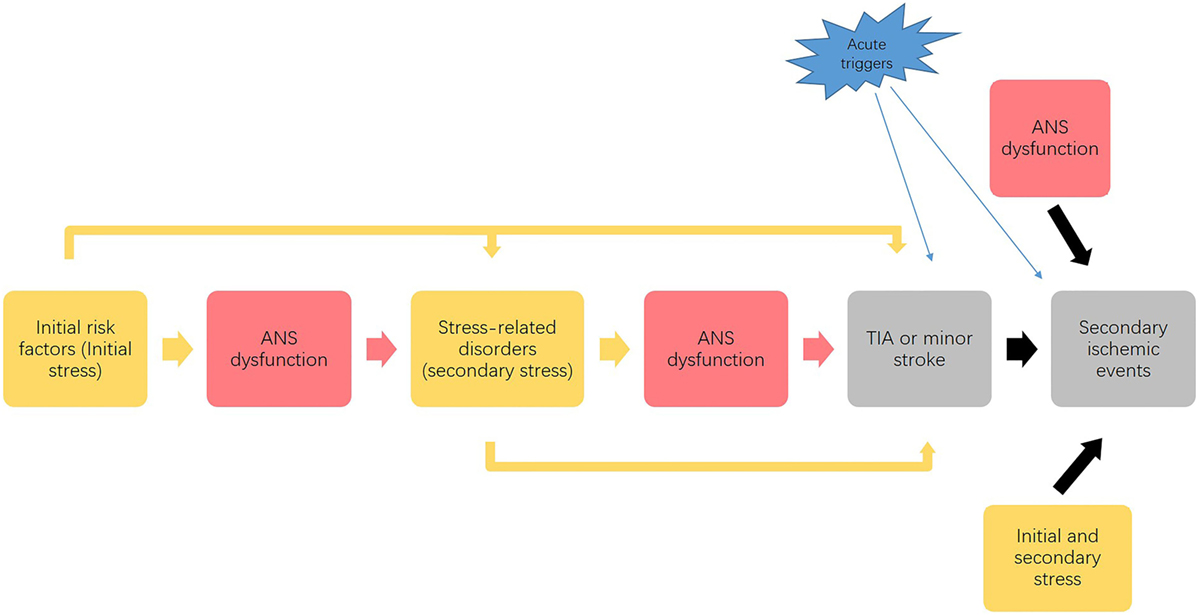
Figure 2. Possible link between stress, autonomic nervous system (ANS) and progression of ischemic stroke. This process illustrates that the initial stress (as risk factors) affects ANS function and causes a dysfunctional ANS response to stress, which combined with the initial stressors causes the development of stress-related disorders. Acting as secondary stressors, these stress-related disorders may further impair ANS function and predispose to transient ischemic attack (TIA) or minor stroke. Finally, the initial and secondary stressors, along with dysfunctional ANS responses, contribute to the development of secondary ischemic events. Acute stressors precipitate the development of both initial TIA and minor stroke events and subsequent ischemic events. This vicious cycle leads to an accumulation of stress that affects the entire body, which potentially promotes the development of initial TIAs and the secondary ischemic events.
ANS Measurement: HRV
The ANS dynamically controls the response of the body to a range of external and internal stimuli/stressors, providing physiological stability in an individual (28). Because most of ANS actions are not accessible to direct and easy physiological testing, in clinical settings the most widely used techniques entail the assessment of an end-organ response to an ANS physiological provocation (35). Some clinical tests for measuring ANS function (35–38) are summarized in Table 2. Among these tests, HRV assessment is a recognized, non-invasive, convenient, and reliable method to measure the ANS function, which will be mainly described in this article. HRV is defined as the fluctuations in the intervals between normal heartbeats (39), and it is mainly based on three analytic methods: frequency domain method, time domain method, and non-linear method (39). The time and frequency domain analyses of HRV are well-developed methods that are recognized as valid and reliable procedures for assessing autonomic function in both clinical and experimental settings (40, 41). Within each analysis, different parameters reflect different aspects of ANS function (Table 3). HRV is generated and analyzed from the ECG waves, which can be recorded from specific devices/software or using 24-h Holter (or other long-term cardiac telemetry) (39).
Due to the dynamic nature of ANS activity, HRV is constantly changing. A single HRV assessment reflects the instantaneous ANS activity at a specific time. According to the uncoupling theory (42), decreased HRV signifies diminished ANS responses; and this process is correlated with disease severity. Conversely, organ recoupling indexed by increased HRV would represent the return of ANS modulation (42). Therefore, assessing the change in HRV between periods can reflect the dynamic/trend of ANS activity over time when stress is changing (40, 43). Accordingly, HRV may serve as a proxy for the neurological mechanisms that guide flexible control of physiology and behavior in the context of stress (44). Through several decades’ progress in HRV research, today HRV measurement is not only an established tool in cardiology research (45) but also increasingly being used in a wide range of clinical and psychophysiological research (46, 47), including informing cardiovascular risk stratification and ischemic stroke prediction (48, 49). Therefore, the critical idea is that HRV may be more than just an index of cardiac function; it may act as an indicator of central modulation of global stress responses. Accordingly, HRV may serve as an easy measurement of the stress regulatory neural network and may provide useful information on the capacity of the body to effectively respond in a stressful situation.
ANS Dysfunction, Impaired HRV, and Risk Factors of Ischemic Stroke
The relationship between ANS dysfunction and ischemic stroke is complex and bidirectional. ANS dysfunction (a sign of chronic stress) may predict the occurrence of ischemic stroke and, on the other hand, ischemic stroke as a source of new stress affects ANS (50). Ischemic stroke is a source of huge stress characterized by sympathetic predominance, and the associated catecholamine surge may cause cardiac autonomic derangement (51, 52), myocardial damage, and thus possible cardiac dysfunction with increased mortality after ischemic stroke (46, 53). Impaired autonomic function is likely to be a common feature in all ischemic stroke patients (52, 53). Earlier findings showed that: (i) lower HF and/or total power was correlated with a higher risk of incident stroke in adults (49); (ii) other HRV parameters such as dichotomized coefficient of variance of NN intervals (CV%) and power law slope (SLOPE) may also stratify high-risk patients to develop stroke, with 0.68 c-statistic for combined high CV% and high SLOPE (54); (iii) patients with ischemic stroke irrespective of the side of the ischemia in the brain had dysfunctional ANS and decreased HRV [HF, LF and total power (TP)] compared to healthy controls (50, 55); (iv) certain locations of stroke, such as right insular stroke, right middle cerebral artery stroke, and parietal or frontal lobe stroke, were correlated with higher risks of cardiac dysfunction (56), atrial fibrillation (AF) (52, 56), and myocardial injury (57), compared to other sites; (v) acute large strokes, because of the huge stress and catecholamine release, were more likely to cause cardiac dysfunction compared to lacunar strokes; and (vi) poststroke decreased HRV was associated with stroke severity, incidence of early and late complications, and mortality (47, 58). The potential therapeutic effects of parasympathetic activation on ischemic stroke have also been documented (59, 60).
From the perspective of chronic stress leading to disease development (from stress adaptation to stress-related disorders), most risk factors of ischemic stroke can be considered as body stressors (some of them are also consequences of multiple other stressors), with the possible stress cascade as described in previous text. ANS dysfunction assessed by HRV parameters may then reflect the overall effects of different stressors/risk factors (both chronic and acute), including the initial TIA or minor stroke episode. A number of studies (Table 4) report the relationship between autonomic dysfunction measured by impaired HRV and main risk factors of ischemic stroke described in Table 1.
Metabolic Disorders and ANS Dysfunction
Metabolic disorders including hypertension, hyperglycemia, and dyslipidemia are sources of chronic stress to the body and well-documented modifiable risk factors for both first and recurrent ischemic stroke (6).
It has been confirmed for several decades that SNS hyperactivity and PNS underactivity are central components in the etiology of early and borderline hypertension, as well as sustained essential hypertension (61, 62). A “neuro-adrenergic” overdrive (i.e., hyperactivity of the SNS) was found in both hypertensive males and females, in young and elderly people with hypertension (63). Therefore, beta-blockers that are competitive antagonists of the beta-adrenergic receptor are widely used to control hypertension (64). Numerous early studies have demonstrated the association between hypertension and autonomic dysfunction measured by lower values of both time (SDNN, SDANN, and RMSSD) and frequency (HF, LF, VLF, and TP) domain HRV parameters (65–68).
Similarly, abundant evidence has demonstrated that an altered balance of PNS and SNS, mainly explained by attenuated parasympathetic activity and a relative elevated sympathetic activity, are causative factors that trigger a cascade of inflammatory/stress responses in the development and progression of diabetes (69, 70). The effect of stress and catecholamines on impairing glycemic control supports the involvement of SNS in the pathophysiology of diabetes (71, 72). A number of studies have shown that an attenuated PNS activity contributes to the development of insulin resistance and diabetes with significantly reduced values of all HRV parameters (HF, LF, TP, SDNN, RMSSN, and pNN50) in diabetic patients, compared to healthy controls (71–73).
Finally, dyslipidemia has also been shown to correlate with SNS activation and PNS suppression (74). High levels of low-density lipoprotein (LDL) and total cholesterol are associated with low HRV values (HF, LF, TP, SDNN, and RMSSD), suggesting an impaired ANS function in individuals with dyslipidemia (75–77).
Arterial Stiffness, Atherosclerosis, and ANS Dysfunction
Arterial stiffness is associated with a degenerative process affecting mainly the extracellular matrix of elastic arteries with aging and other risk factors, such as high blood pressure (78, 79). On the other hand, arterial stiffening may result in changes to vessel walls and activate a number of complex mechanisms involved in the process of atherosclerosis with associated development of cardiovascular events (80–82). Both arterial stiffness and atherosclerosis are sources of chronic stress to the body and independent risk factors for ischemic stroke (83–85). Atherosclerosis is responsible for the thrombosis and occlusion of large brain arteries (large-artery atherosclerosis subtype), associated with an increased risk of small-vessel stroke (lacunar subtype), and partially contributing to embolism (cardioembolic subtype) (83).
Arterial stiffness is associated with sympathovagal imbalance, particularly increased sympathetic activity (86, 87). In a normal state, the ANS and the endothelium work together to maintain the vascular tone. There is a tonic balance between the release of vasodilating factors from the endothelium and vasoconstricting factors from sympathetic nerve terminals (88). This balance acts on the vascular smooth muscle cells to maintain the appropriate vessel tone (89). Impaired ANS regulation contributes to abnormal changes in endothelial cells, resulting in endothelial dysfunction. Some mechanisms may be that the high SNS activity and increased catecholamines influence the inflammatory process, increase the uptake of LDLs, activate beta- and/or alpha-adrenergic receptors, and finally cause endothelial damage (88). Reduced total power and HF values, as well as higher LF/HF ratio, have been shown to be correlated with reduce arterial distensibility in patients with hypertension (90). Moreover, exercise with dietary restriction improves cardiac autonomic activity reflected by increased SDNN, RMSSD, TP LF, and HF and decreased LF/HF; and this enhanced cardiac autonomic modulation (assessed with decreased LF/HF) was associated with decreased arterial stiffness (91).
According to the prevailing theory proposed by Ross (92), atherosclerosis development is predominantly a cascade of inflammation/stress response-mediated events, from initiation through progression, rupture, and ultimately to the thrombotic and embolic complications. During the process, ANS dysfunction is characterized by the stimulation of SNS and downregulation of PNS, with subsequent impairment of the tight control of inflammatory responses (25). Decreased parasympathetic function (decreased HF, normalized HF, and increased LF/HF) and increased sympathetic function have been reported to correlate with the progression of coronary artery atherosclerosis (93) and coronary artery remodeling (94). In addition, the ANS also plays a crucial role in thrombogenesis (95).
Cardiovascular Diseases and ANS Dysfunction
The contribution of autonomic dysfunction to the development of cardiovascular diseases has been well illustrated (32, 48). Increased SNS promotes vasoconstriction, increases platelet aggregation and pulse and blood pressure, and decreases fibrinolysis, while decreased PNS leads to reduced arterial pressure and cardiac output. These pathophysiological changes increase the risk of thrombosis due to sluggish flow and arterial wall collapse, and the risk of consequent cardiovascular disorders (96, 97). From the 1980s to recent times, numerous studies have clearly demonstrated that reductions in both time and frequency domain parameters of HRV (SDNN, ULF, VLF, LF, and HF) were present in MI survivors and were correlated with poor prognosis (such as mortality and arrhythmia events) after acute MI (98–100). These HRV indexes are depressed at the early phase of acute MI with substantial improvement during recovery (101, 102).
Atrial Fibrillation (AF) and ANS Dysfunction
Atrial fibrillation is one of the high-risk cardiac sources for cardioembolic ischemic stroke (14, 103). All types of AF, including paroxysmal, persistent, and permanent, are associated with around fivefold increased risk of ischemic stroke (104, 105). Histological studies have shown that the pulmonary veins where the AF impulses originate are richly innervated by both sympathetic and parasympathetic nerves (106). As early as 1978, Coumel et al. reported that cardiac autonomic dysfunction might predispose patients to develop paroxysmal atrial fibrillation (PAF) (107). Later studies on HRV and AF have further determined the crucial role of the ANS, with relative increased SNS and decreased PNS, contributing to the development, progression, and maintenance of AF (108). Patients with AF are reported to have significantly lower values of HF, LF, VLF, and TP and increased LF/HF, compared to those without AF (109, 110). Interestingly, another study showed that LF/HF increased during the preceding 24 h but decreased sharply at 5 min before the onset of PAF, which may suggest a primary increase in SNS followed by short-term PNS predominance prior to the onset of PAF (111). In addition, the classic treatment for all types of AF is the administration of beta-blockers to inhibit the SNS (112, 113).
Cardiac Surgery and ANS Dysfunction
Cerebrovascular complications including ischemic stroke and TIA are common after cardiac surgery, especially heart transplantation (114). The incidence of stroke increases with the number of preoperative stroke risk factors, such as a history of hypertension, diabetes, smoking, stroke, and vascular diseases (115). Heart transplantation interrupts the parasympathetic vagal neurons and the intrinsic postganglionic sympathetic nerve fibers traveling from the stellate ganglia to the myocardium, which may cause axonal Wallerian degeneration and thus cardiac denervation (116). The cardiac denervation will cause the lack of parasympathetic connections and abnormal cardiopulmonary baroreflexes, which alters autonomic regulation on cardiovascular function, resulting in lower HRV, cardiac index, abnormal catecholamine levels, and higher heart rate (117–119). Moreover, cardiac surgical procedures often cause AF. As described in the last section, this is associated with an increased risk of embolic strokes, and the use of beta-blockers aims at preventing such strokes (120, 121).
Aging and ANS Dysfunction
Aging, as a marker of stress experience, can be seen as a chronic body stressor, which leads to ANS alteration (122, 123). Autonomic dysfunction in seniors is also attributed to several main features associated with aging, such as loss of neurons, loss of axon branches, alterations in neurotransmitters, and degenerative changes in effector organs innervated by autonomic nerves (122, 123). Many clinical symptoms associated with aging, such as increased blood pressure and decreased baroreflex function, are associated with relatively elevated SNS and diminished PNS activities that elicit inadequate autonomic responses to physiological stressors (122, 123). Previous studies have shown that elderly people have significantly lower values of HF, LF, and TP powers than young people (124, 125).
Unhealthy Lifestyle and ANS Dysfunction
Unhealthy lifestyles including cigarette smoking, heavy alcohol use, sedentary lifestyle, and others are all correlated with autonomic dysfunction. Lower HRV values (HF, normalized HF power, LF, TP, and SDNN) and higher LF/HF ratio are found in smokers than in non-smokers in both early and recent studies (126–128). Similarly, people suffering from heavy alcohol abuse had decreased RMSSD and HF and increased LF/HF ratio, compared to controls (129, 130), indicating an impaired vagal function in alcoholics. Further, sedentary lifestyle is also related to autonomic imbalance, primarily suppressed PNS activity (decreases in HF, pNN50, and RMSSD), while exercise may improve autonomic function with increases in HRV parameters (131, 132).
Psychological Stress and ANS Dysfunction
Psychological stress, caused by occupational, familial, or life events, is recognized as a potential contributor to an individual’s perceptions of stress (133) and possibly interacts with the ability to cope with specific stressors (134). Various components of psychological stress, including self-perceived stress, stressful life events, and poor coping ability, are associated with an increased risk of ischemic stroke (135). High levels of chronic psychological stress lead to continuous activation of the stress system, with prolonged secretion of stress mediators such as catecholamines and cortisol (24, 133), which eventually promotes SNS activity and suppresses PNS activity (28). Studies have shown decreased HF and normalized HF as well as increased LF/HF in perceived psychological stressful situations (136–138). These findings indicate a lower cardiac vagal activity in people who perceive a higher level of psychological stress.
Recent Infections and ANS Dysfunction
Infection possibly contributes to atherosclerotic plaque pathology via inflammation, by activating inflammatory cytokines that accelerate the maturation of plaques and promote plaque instability and rupture (139). Previous studies have shown that patients with infections have decreased HF, LF, and TP, as well as increased LF/HF ratio, compared to both their recovery states (140), and healthy controls (141). Changes to markers of HRV have also been identified in association with the stage and deterioration of infection, which supports the use of HRV as an indicator of illness severity (140, 141). In addition, a recent study shows that changes in HRV parameters could predict the onset of infection and ischemic events after subarachnoid hemorrhage (142).
HRV-Based Comprehensive Stress Model
According to the stress theory, and association between stroke risk factors, stress, and ANS/HRV, it is possible to establish a “comprehensive stress model” using HRV as a marker of ANS activity and adaptation to stress. This model would represent the overall effects of stroke risk factors and could be used to identify personalized risk of experiencing a secondary ischemic event after TIA and minor stroke.
The HRV-based comprehensive stress model refers to a model that comprises HRV parameters and multiple dimensions of stress variables, including chronic and acute, physiological, and psychological. The assessment of HRV measures the objective physiological response to stress factors. For an individual, HRV values may represent the comprehensive effect of “multiple stressors” at a given point in time. The HRV-based theoretical model is presented in Figure 3.
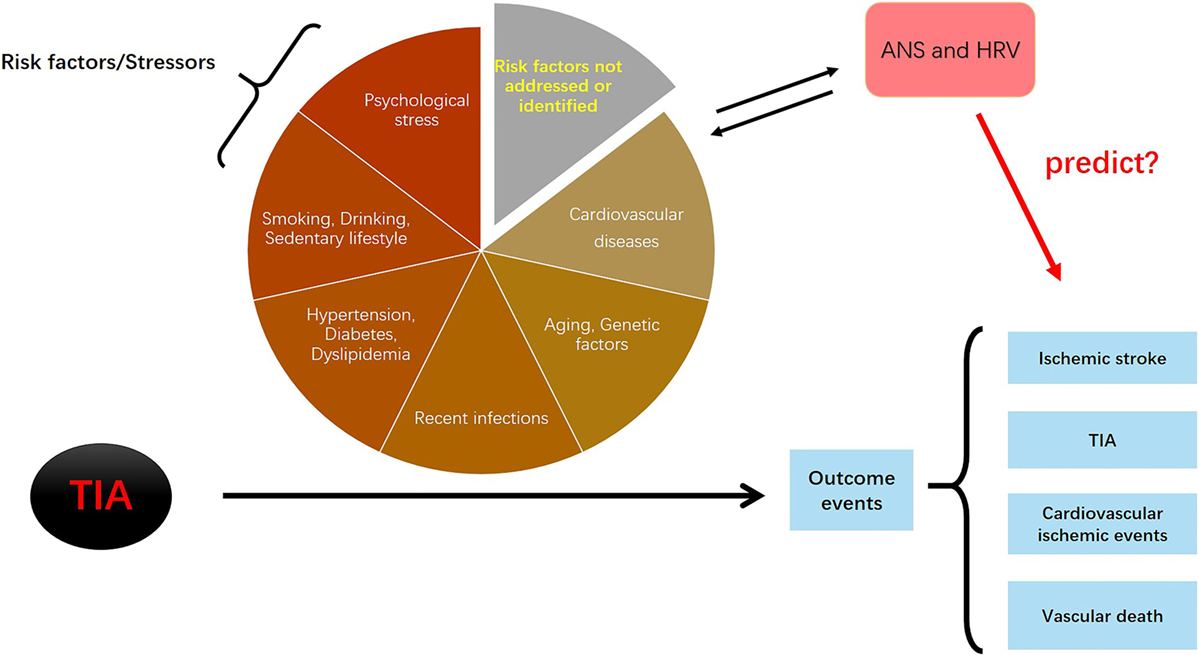
Figure 3. The logic of the heart rate variability (HRV)-based stress predictive model. All risk factors (stressors) have effects during the progression from transient ischemic attack (TIA) to the development of outcome events (ischemic stroke, TIAs, cardiovascular diseases, and vascular death). Autonomic nervous system (ANS) is directly affected by the risk factors/stressors; on the other hand, dysfunctional ANS activity conversely contributes to the development of risk factors/stressors. If correct, this model would suggest that whether HRV parameters (as markers of ANS) can predict the occurrence of secondary outcome events.
Identification and Selection of HRV Predictors in the HRV-Based Model
Early studies have shown the 24-h rhythm of HRV in both healthy individuals and those with disease (for example, diabetes, chronic stable angina, or coronary artery disease) (143–145). In general, the absolute values of all HRV parameters (HF, LF, VLF, and TP) in healthy individuals are consistently higher than those in diseased people during the entire 24 h (143, 145). In normal conditions, ANS activity has a circadian rhythm with PNS increasing during nighttime and SNS activating during daytime. This circadian rhythm leads to a 24-h HRV rhythm. However, in patients with chronic disease, HRV parameters fail to show normal diurnal changes (143, 145). Among these HRV parameters, HF provides the highest discriminative ability between patients with chronic disease and healthy individuals; more specifically, people with diabetes, chronic stable angina, or coronary artery disease show lower HF values, less day–night rhythm and less daytime rhythm in HF, compared to healthy individuals (144, 145).
Based on the expected values and changes in HRV during a 24-h period, two-dimensional HRV measurement, including HRV absolute values at a given time (as indicators of static ANS activity) and HRV changes over time (as indicators of dynamic ANS activity), can be considered for inclusion in HRV-based predictive models. Several further hypotheses regarding the identification and selection of HRV predictors are made to establish the HRV-based comprehensive stress model to predict secondary ischemic events after TIA or minor stroke.
• Regarding types of HRV parameters: HF may be the primary HRV parameter to select because it is a precise indicator of PNS activity and should be lower in patients under stress. Normalized HF that represents the balanced PNS and the proportion of PNS to ANS may also be selected. TP as a marker of overall ANS activity may be included in the assessment. HF + LF, although not a traditional parameter, may be considered as the fraction of HRV that can be totally explained by ANS modulation based on the physiology of HF and LF and thus may represent a more precise indicator of the overall ANS activity. Absolute values of HRV parameters are indicators of people’s health condition; therefore, a lower HRV value may be associated with worse health conditions and a higher level of stress, and thus, a higher risk of developing secondary ischemic events after TIA or minor stroke.
• Regarding time periods: In healthy situations, HF power that presents the PNS activity is increased during the night and relaxation period, such as napping. The morning time, especially the few hours after waking up is the period of SNS activation, which leads to the decrease of both absolute and normalized values of HF (6:00 a.m.–9:00 a.m.). HF recovers after 9:00 a.m. and remains comparatively stable with small fluctuations during daytime and early evening (around 9:00 a.m.–9:00 p.m.) (143, 145). Therefore, 9:00 a.m.–12:00 p.m. may be used to represent “morning time” to avoid the sharp decrease in PNS and increase in SNS immediately after waking up (144). 3:00 p.m.–6:00 p.m. may represent afternoon time to avoid the effect of the midday napping. 12:00 a.m. to 3:00 a.m. is to represent the night time because it is most likely period that people are sleeping.
• Use of HRV changes between day and night: HRV demonstrates greater day vs. night discrepancies among healthy populations than among people with diseases. It is therefore reasonable to postulate that decreased amplitude of HRV changes between day and night may suggest less restoration of ANS activity and less control of stress and thus a higher risk of secondary ischemic events.
• Use of HRV changes during daytime: If people are under stable conditions, HRV remains stable during daytime, i.e., from late morning (after 9:00 a.m.) to afternoon (around 6:00 p.m.) (144, 145), which support the uncoupling and recoupling theories explained in previous text. Accordingly, decreases in HRV parameters during daytime may indicate less rebound capacity of the body or deterioration of health condition (excessive stress) and, therefore, may be associated with a higher risk of development of new ischemic events.
Some Issues with Regard to the Implication of HRV-Based Model for Ischemic Event Prediction
• For patients with acute TIA: Because the risk of secondary ischemic events after TIA is high in the first several hours and days (146, 147), the optimal design is to start ECG recording just after the occurrence of the TIA episode. Ideally, it would be best to recruit patients within 24 or 48 h of the TIA event and start recording ECG as soon as possible. The use of HRV can be compared with the traditional predictive ABCD2 score (or other tools such as ABCD3 and ABCD3-I), with regard to their predictive values on ischemic events after initial TIA or minor stroke.
• Cutoffs of HRV for risk stratification: Previous studies showed different cutoffs of HRV parameters, such as SDNN less than 50, or 70 or 100 ms, or HF less than 10 ms2, for the risk stratification of cardiovascular diseases (98, 148). Our view is that an attempt to find HRV cutoffs should be sought only in the context of a specific outcome and study population. Moreover, different HRV parameters may have different predictive values, which include both the absolute values and changes during a specific time period of each time domain, frequency domain, and non-linear parameter. Finally, the cutoffs depend on the sensitivity and specificity that clinicians/investigators select. Therefore, there may be no consensus on the ideal cutoffs for different HRV measures with regard to ischemic events occurrence. To define optimal cutoffs for different parameters or the best type of HRV parameter deserves further investigation.
Summary
It is critical to estimate the risk of stroke occurrence or recurrence after initial TIA and to clearly identify those at a higher risk of developing secondary ischemic events among people with a burden of chronic risk factors/stressors. However, this remains challenging using current criteria, partly because the specific contributions of these risk factors are difficult to quantify given individual heterogeneity and also many other risk factors (unaddressed and unidentified) are not assessed when determining an individual’s risk profile. We have shown that both chronic risk factors and acute triggers of ischemic stroke are sources of stress to the body and are closely associated with ANS dysfunction that supports the neurogenic hypothesis of ischemic stroke development. The usual compensatory stress response of ANS may fail in the context of chronicity, which makes it challenged to face new acute stressors. The improper stress responses render these normally short-term responses prolonged and maladaptive, which relentlessly disrupts normal physiological pathways and progressively contributes to the development of stress-related diseases, such as TIA and ischemic stroke. Accordingly, we advocate that HRV assessment, as a measurement of ANS function, may represent the comprehensive effect of “multiple stressors” and may reflect the overall health condition at a given point in time. This review provides evidence for the use of HRV data to predict the occurrence of secondary ischemic events after initial TIA or minor stroke, as illustrated in Figure 3. This theoretical HRV-based comprehensive stress model and further hypotheses on identification of HRV predictors will initiate studies on identifying an innovative way to stratify the risk of TIA or minor stroke through assessing the effect of ANS and stress.
Author Contributions
LG and J-PC proposed the study questions, conducted the literature review, and prepared the first draft of the manuscript. GM improved the study conception and provided a detailed review and editing. VC provided insight on specific aspects of the paper and provided a detailed review and editing. None of the authors received payment for writing the article. They all approve the submission of this version of the manuscript and take full responsibility for the manuscript.
Conflict of Interest Statement
This publication is original: There are no prior publications or submissions with any overlapping information. None of the authors has disclosed any potential conflicts of interest. J-PC is supported in part by a scholarship of the BC Children’s Hospital Research Institute in Vancouver, Canada. The remaining authors have no financial relationships relevant to this article to disclose.
Funding
J-PC is supported in part by a scholarship of the BC Children’s Hospital Research Institute in Vancouver, Canada.
References
1. Sacco RL, Kasner SE, Broderick JP, Caplan LR, Connors JJ, Culebras A, et al. An updated definition of stroke for the 21st century: a statement for healthcare professionals from the American Heart Association/American Stroke Association. Stroke (2013) 44(7):2064–89. doi:10.1161/STR.0b013e318296aeca
2. Easton JD, Saver JL, Albers GW, Alberts MJ, Chaturvedi S, Feldmann E, et al. Definition and evaluation of transient ischemic attack: a scientific statement for healthcare professionals from the American Heart Association/American Stroke Association Stroke Council; Council on Cardiovascular Surgery and Anesthesia; Council on Cardiovascular Radiology and Intervention; Council on Cardiovascular Nursing; and the Interdisciplinary Council on Peripheral Vascular Disease. The American Academy of Neurology affirms the value of this statement as an educational tool for neurologists. Stroke (2009) 40(6):2276–93. doi:10.1161/STROKEAHA.108.192218
3. Lavallée PC, Meseguer E, Abboud H, Cabrejo L, Olivot J-M, Simon O, et al. A transient ischaemic attack clinic with round-the-clock access (SOS-TIA): feasibility and effects. Lancet Neurol (2007) 6(11):953–60. doi:10.1016/S1474-4422(07)70248-X
4. Rothwell P, Giles M, Chandratheva A, Marquardt L, Geraghty O, Redgrave J, et al. Effect of urgent treatment of transient ischaemic attack and minor stroke on early recurrent stroke (EXPRESS study): a prospective population-based sequential comparison. Lancet (2007) 370(9596):1432–42. doi:10.1016/S0140-6736(07)61448-2
5. Jauch EC, Saver JL, Adams HP, Bruno A, Connors JJB, Demaerschalk BM, et al. Guidelines for the early management of patients with acute ischemic stroke: a guideline for healthcare professionals from the American Heart Association/American Stroke Association. Stroke (2013) 44:870–947. doi:10.1161/STR.0b013e318284056a
6. Kernan WN, Ovbiagele B, Black HR, Bravata DM, Chimowitz MI, Ezekowitz MD, et al. Guidelines for the prevention of stroke in patients with stroke and transient ischemic attack: a guideline for healthcare professionals from the American Heart Association/American Stroke Association. Stroke (2014) 45(7):2160–236. doi:10.1161/STR.0000000000000024
7. Diener H, Bogousslavsky J, Brass L, Cimminiello C, Csiba L, Kaste M, et al. Aspirin and clopidogrel compared with clopidogrel alone after recent ischaemic stroke or transient ischaemic attack in high-risk patients (MATCH): randomised, double-blind, placebo-controlled trial. Lancet (2004) 364(9431):331–7. doi:10.1016/S0140-6736(04)16721-4
8. Miller DJ, Simpson JR, Silver B. Safety of thrombolysis in acute ischemic stroke: a review of complications, risk factors, and newer technologies. Neurohospitalist (2011) 1(3):138–47. doi:10.1177/1941875211408731
9. Prencipe M, Culasso F, Rasura M, Anzini A, Beccia M, Cao M, et al. Long-term prognosis after a minor stroke: 10-year mortality and major stroke recurrence rates in a hospital-based cohort. Stroke (1998) 29(1):126–32. doi:10.1161/01.STR.29.1.126
10. Johnston SC, Gress DR, Browner WS, Sidney S. Short-term prognosis after emergency department diagnosis of TIA. J Am Med Assoc (2000) 284(22):2901–6. doi:10.1001/jama.284.22.2901
11. Chrousos G, Gold P. The concepts of stress and stress system disorders. J Am Med Assoc (1992) 267:1244–52. doi:10.1001/jama.267.9.1244
12. Rothwell PM, Warlow CP. Timing of TIAs preceding stroke: time window for prevention is very short. Neurology (2005) 64(5):817–20. doi:10.1212/01.WNL.0000152985.32732.EE
13. Johnston SC, Amarenco P, Albers GW, Denison H, Easton JD, Evans SR, et al. Ticagrelor versus aspirin in acute stroke or transient ischemic attack. N Engl J Med (2016) 375(1):35–43. doi:10.1056/NEJMoa1603060
14. Meschia JF, Bushnell C, Boden-albala B, Braun LT, Bravata DM, Chaturvedi S, et al. Guidelines for the primary prevention of stroke: a statement for healthcare professionals from the American Heart Association/American Stroke Association. Stroke (2014) 45(12):3754–832. doi:10.1161/STR.0000000000000046
15. Elkind M. Why now? Moving from stroke risk factors to stroke triggers. Curr Opin Neurol (2007) 20:51–7. doi:10.1097/WCO.0b013e328012da75
16. Guiraud V, Amor MB, Mas J-L, Touze E. Triggers of ischemic stroke: a systematic review. Stroke (2010) 41:2669–77. doi:10.1161/STROKEAHA.110.597443
17. Chandratheva A, Geraghty OC, Rothwell PM. Poor performance of current prognostic scores for early risk of recurrence after minor stroke. Stroke (2011) 42(3):632–7. doi:10.1161/STROKEAHA.110.593301
18. Perry J, Sharma M, Sivilotti M, Sutherland J, Symington C, Worster A, et al. Prospective validation of the ABCD2 score for patients in the emergency department with transient ischemic attack. Can Med Assoc J (2011) 183(10): 1137–45. doi:10.1503/cmaj.101668
19. Merwick Á, Albers GW, Amarenco P, Arsava EM, Ay H, Calvet D, et al. Addition of brain and carotid imaging to the ABCD2 score to identify patients at early risk of stroke after transient ischaemic attack: a multicentre observational study. Lancet Neurol (2010) 9(11):1060–9. doi:10.1016/S1474-4422(10)70240-4
20. Coutts SB, Eliasziw M, Hill MD, Scott JN, Subramaniam S, Buchan AM, et al. An improved scoring system for identifying patients at high early risk of stroke and functional impairment after an acute transient ischemic attack or minor stroke. Int J Stroke (2008) 3(1):3–10. doi:10.1111/j.1747-4949.2008.00182.x
22. Johnson EO, Kamilaris TC, Chrousos GP, Gold PW. Mechanisms of stress: a dynamic overview of hormonal and behavioral homeostasis. Neurosci Biobehav Rev (1992) 16(2):115–30. doi:10.1016/S0149-7634(05)80175-7
23. McEwen BS. Stress and the individual: mechanism leading to disease. Arch Intern Med (1993) 153:2093–101. doi:10.1001/archinte.1993.00410180039004
24. Chrousos GP. Stressors, stress and neuroendocrine integration of the adaptive response. Ann N Y Acad Sci (1998) 851:311–35. doi:10.1111/j.1749-6632.1998.tb09006.x
26. Ulrich-Lai YM, Herman JP. Neural regulation of endocrine and autonomic stress responses. Nat Rev Neurosci (2009) 10(6):397–409. doi:10.1038/nrn2647
27. Goyal RK, Hirano I. The enteric nervous system. N Engl J Med (1996) 334(17):1106–15. doi:10.1056/NEJM199604253341707
28. Chrousos GP. Stress and disorders of the stress system. Nat Rev Endocrinol (2009) 5(7):374–81. doi:10.1038/nrendo.2009.106
29. Black PH. The inflammatory response is an integral part of the stress response: implications for atherosclerosis, insulin resistance, type II diabetes and metabolic syndrome X. Brain Behav Immun (2003) 17(5):350–64. doi:10.1016/S0889-1591(03)00048-5
30. Tracey KJ. Physiology and immunology of the cholinergic antiinflammatory pathway. J Clin Invest (2007) 117(2):289–96. doi:10.1172/JCI30555
31. Koolhaas JM, Bartolomucci A, Buwalda B, de Boer SF, Flugge G, Korte SM, et al. Stress revisited: a critical evaluation of the stress concept. Neurosci Biobehav Rev (2011) 35(5):1291–301. doi:10.1016/j.neubiorev.2011.02.003
32. Curtis BM, O’Keefe JH. Autonomic tone as a cardiovascular risk factor: the dangers of chronic fight or flight. Mayo Clin Proc (2002) 77(1):45–54. doi:10.4065/77.1.45
33. Kloet ER, Joëls M, Holsboer F. Stress and the brain: from adaptation to disease. Nat Rev Neurosci (2005) 6(6):463–75. doi:10.1038/nrn1683
34. Tofler GH, Muller JE. Triggering of acute cardiovascular disease and potential preventive strategies. Circulation (2006) 114(17):1863–72. doi:10.1161/CIRCULATIONAHA.105.596189
35. Freeman R. Assessment of cardiovascular autonomic function. Clin Neurophysiol (2006) 117(4):716–30. doi:10.1016/j.clinph.2005.09.027
36. Zygmunt A, Stanczyk J. Methods of evaluation of autonomic nervous system function. Arch Med Sci (2010) 6(1):11–8. doi:10.5114/aoms.2010.13500
37. Low PA. Testing the autonomic nervous system. Semin Neurol (2003) 23(4):407–22. doi:10.1055/s-2004-817725
38. Vallbo AB, Hagbarth KE, Wallin BG. Microneurography: how the technique developed and its role in the investigation of the sympathetic nervous system. J Appl Physiol (2004) 96(4):1262–9. doi:10.1152/japplphysiol.00470.2003
39. Task Force of ESC. Heart rate variability: standards of measurement, physiological interpretation, and clinical use. Circulation (1996) 93:1043–65. doi:10.1161/01.CIR.93.5.1043
40. Montano N, Porta A, Cogliati C, Costantino G, Tobaldini E, Casali KR, et al. Heart rate variability explored in the frequency domain: a tool to investigate the link between heart and behavior. Neurosci Biobehav Rev (2009) 33(2):71–80. doi:10.1016/j.neubiorev.2008.07.006
41. Malliani A, Lombardi F, Pagani M. Power spectrum analysis of heart rate variability: a tool to explore neural regulatory mechanisms. Br Heart J (1994) 71:1–2. doi:10.1136/hrt.71.1.1
42. Godin PJ, Buchman TG. Uncoupling of biological oscillators: a complementary hypothesis concerning the pathogenesis of multiple organ dysfunction syndrome. Crit Care Med (1996) 24(7):1107–16. doi:10.1097/00003246-199607000-00008
43. Pumprla J, Howorka K, Groves D, Chester M, Nolan J. Functional assessment of heart rate variability: physiological basis and practical applications. Int J Cardiol (2002) 84(1):1–14. doi:10.1016/S0167-5273(02)00057-8
44. Thayer JF, Åhs F, Fredrikson M, Sollers JJ, Wager TD. A meta-analysis of heart rate variability and neuroimaging studies: implications for heart rate variability as a marker of stress and health. Neurosci Biobehav Rev (2012) 36(2):747–56. doi:10.1016/j.neubiorev.2011.11.009
45. Thayer JF, Yamamoto SS, Brosschot JF. The relationship of autonomic imbalance, heart rate variability and cardiovascular disease risk factors. Int J Cardiol (2010) 141(2):122–31. doi:10.1016/j.ijcard.2009.09.543
46. Al-Qudah ZA, Yacoub HA, Souayah N. Disorders of the autonomic nervous system after hemispheric cerebrovascular disorders: an update. J Vasc Interv Neurol (2015) 8(4):43–52.
47. Yperzeele L, van Hooff RJ, Nagels G, De Smedt A, De Keyser J, Brouns R. Heart rate variability and baroreceptor sensitivity in acute stroke: a systematic review. Int J Stroke (2015) 10(6):796–800. doi:10.1111/ijs.12573
48. Huikuri HV, Stein PK. Heart rate variability in risk stratification of cardiac patients. Prog Cardiovasc Dis (2013) 56(2):153–9. doi:10.1016/j.pcad.2013.07.003
49. Fyfe-Johnson AL, Muller CJ, Alonso A, Folsom AR, Gottesman RF, Rosamond WD, et al. Heart rate variability and incident stroke: the atherosclerosis risk in communities study. Stroke (2016) 47(6):1452–8. doi:10.1161/STROKEAHA.116.012662
50. Dütsch M, Burger M, Dörfler C, Schwab S, Hilz MJ. Cardiovascular autonomic function in poststroke patients. Neurology (2007) 69(24):2249–55. doi:10.1212/01.wnl.0000286946.06639.a7
51. Colivicchi F, Bassi A, Santini M, Caltagirone C. Cardiac autonomic derangement and arrhythmias in right-sided stroke with insular involvement. Stroke (2004) 35(9):2094–8. doi:10.1161/01.STR.0000138452.81003.4c
52. Colivicchi F, Bassi A, Santini M, Caltagirone C. Prognostic implications of right-sided insular damage, cardiac autonomic derangement, and arrhythmias after acute ischemic stroke. Stroke (2005) 36(8):1710–5. doi:10.1161/01.STR.0000173400.19346.bd
53. Micieli G, Cavallini A. The autonomic nervous system and ischemic stroke: a reciprocal interdependence. Clin Auton Res (2008) 18(6):308–17. doi:10.1007/s10286-008-0495-7
54. Bodapati RK, Kizer JR, Kop WJ, Kamel H, Stein PK. Addition of 24-hour heart rate variability parameters to the cardiovascular health study stroke risk score and prediction of incident stroke: the cardiovascular health study. J Am Heart Assoc (2017) 6(7):1–9. doi:10.1161/JAHA.116.004305
55. Xiong L, Leung HHW, Chen XY, Han JH, Leung TWH, Soo YOY, et al. Comprehensive assessment for autonomic dysfunction in different phases after ischemic stroke. Int J Stroke (2013) 8(8):645–51. doi:10.1111/j.1747-4949.2012.00829.x
56. Scheitz JF, Erdur H, Haeusler KG, Audebert HJ, Roser M, Laufs U, et al. Insular cortex lesions, cardiac troponin, and detection of previously unknown atrial fibrillation in acute ischemic stroke. Stroke (2015) 46(5):1196–201. doi:10.1161/STROKEAHA.115.008681
57. Krause T, Werner K, Fiebach JB, Villringer K, Piper SK, Haeusler KG, et al. Stroke in right dorsal anterior insular cortex Is related to myocardial injury. Ann Neurol (2017) 81(4):502–11. doi:10.1002/ana.24906
58. Graff B, Gasecki D, Rojek A, Boutouyrie P, Nyka W, Laurent S, et al. Heart rate variability and functional outcome in ischemic stroke: a multiparameter approach. J Hypertens (2013) 31(8):1629–36. doi:10.1097/HJH.0b013e328361e48b
59. Han B, Li X, Hao J. The cholinergic anti-inflammatory pathway: an innovative treatment strategy for neurological diseases. Neurosci Biobehav Rev (2017) 77(February):358–68. doi:10.1016/j.neubiorev.2017.04.002
60. Cheyuo C, Jacob A, Wu R, Zhou M, Coppa GF, Wang P. The parasympathetic nervous system in the quest for stroke therapeutics. J Cereb Blood Flow Metab (2011) 31(5):1187–95. doi:10.1038/jcbfm.2011.24
61. Palatini P, Julius S. The role of cardiac autonomic function in hypertension and cardiovascular disease. Curr Hypertens Rep (2009) 11(3):199–205. doi:10.1007/s11906-009-0035-4
62. Grassi G, Seravalle G, Bertinieri G, Turri C, Dell’Oro R, Stella M, et al. Sympathetic and reflex alterations in systo-diastolic and systolic hypertension of the elderly. J Hypertens (2000) 18:587–93. doi:10.1097/00004872-200018050-00012
63. Grassi G. Assessment of sympathetic cardiovascular drive in human hypertension: achievements and perspectives. Hypertension (2009) 54:690–7. doi:10.1161/HYPERTENSIONAHA.108.119883
64. Wiysonge CS, Bradley HA, Volmink J, Mayosi BM, Opie LH. Beta-blockers for hypertension. Cochrane Database Syst Rev (2012) 8:1–77. doi:10.1002/14651858.CD002003.pub3
65. Liao D, Cai J, Barnes RW, Tyroler HA, Rautaharju P, Holme I, et al. Association of cardiac autonomic function and the development of hypertension: the ARIC study. Am J Hypertens (1996) 9:1147–56. doi:10.1016/S0895-7061(96)00249-X
66. Schroeder E, Liao D, Chambless L, Prineas R, Evans G, Heiss G. Hypertension, blood pressure, and heart rate variability: the Atherosclerosis Risk in Communities (ARIC) study. Hypertension (2003) 42:1106–11. doi:10.1161/01.HYP.0000100444.71069.73
67. Singh J, Larson M, Tsuji H, Evans J, O’Donnell C, Levy D. Reduced heart rate variability and new-onset hypertension: insights into pathogenesis of hypertension: the Framingham Heart Study. Hypertension (1998) 32:293–7. doi:10.1161/01.HYP.32.2.293
68. Huikuri HV, Ylitalo A, Pikkujgms SM, Iktiheimo MJ, Juhani Airaksinen KE, Rantala A, et al. Heart rate variability in systemic hypertension. Am J Cardiol (1996) 77:1073–7. doi:10.1016/S0002-9149(96)00135-X
69. Wellen KE, Hotamisligil GS. Inflammation, stress, and diabetes. J Clin Invest (2005) 115(5):1111–9. doi:10.1172/JCI200525102
70. Schlaich M, Straznicky N, Lambert E, Lambert G. Metabolic syndrome: a sympathetic disease? Lancet Diabetes Endocrinol (2015) 3(2):148–58. doi:10.1016/S2213-8587(14)70033-6
71. Carnethon MR, Golden SH, Folsom AR, Haskell W, Liao D. Prospective investigation of autonomic nervous system function and the development of type 2 diabetes: the atherosclerosis risk in communities study, 1987–1998. Circulation (2003) 107(17):2190–5. doi:10.1161/01.CIR.0000066324.74807.95
72. Tarvainen MP, Laitinen TP, Lipponen JA, Cornforth DJ, Jelinek HF. Cardiac autonomic dysfunction in type 2 diabetes – effect of hyperglycemia and disease duration. Front Endocrinol (2014) 5(AUG):1–9. doi:10.3389/fendo.2014.00130
73. Kudat H, Akkaya V, Sozen A, Salman S, Demirel S, Ozcan M, et al. Heart rate variability in diabetes patients. J Int Med Res (2006) 34(3):291–6. doi:10.1177/147323000603400308
74. Lambert E, Straznicky N, Sari C, Eikelis N, Hering D, Head G, et al. Dyslipidemia is associated with sympathetic nervous activation and impaired endothelial function in young females. Am J Hypertens (2013) 26:250–6. doi:10.1093/ajh/hps016
75. Christensen J, Toft E, Christensen M, Schmidt E. Heart rate variability and plasma lipids in men with and without ischaemic heart disease. Atherosclerosis (1999) 145:181–6. doi:10.1016/S0021-9150(99)00052-0
76. Liao D, Sloan RP, Cascio WE, Folsom AR, Liese AD, Evans GW, et al. Multiple metabolic syndrome is associated with lower heart rate variability. The Atherosclerosis Risk in Communities Study. Diabetes Care (1998) 21(12):2116–22. doi:10.2337/diacare.21.12.2116
77. Kimura T, Matsumoto T, Akiyoshi M, Owa Y, Miyasaka N, Aso T, et al. Body fat and blood lipids in postmenopausal women are related to resting autonomic nervous system activity. Eur J Appl Physiol (2006) 97(5):542–7. doi:10.1007/s00421-006-0207-8
78. AlGhatrif M, Strait JB, Morrell CH, Canepa M, Wright J, Elango P, et al. Longitudinal trajectories of arterial stiffness and the role of blood pressure: the Baltimore longitudinal study of aging. Hypertension (2013) 62(5):934–41. doi:10.1161/HYPERTENSIONAHA.113.01445
79. Cecelja M, Chowienczyk P. Dissociation of aortic pulse wave velocity with risk factors for cardiovascular disease other than hypertension: a systematic review. Hypertension (2009) 54(6):1328–36. doi:10.1161/HYPERTENSIONAHA.109.137653
80. Palombo C, Kozakova M. Arterial stiffness, atherosclerosis and cardiovascular risk: pathophysiologic mechanisms and emerging clinical indications. Vascul Pharmacol (2016) 77:1–7. doi:10.1016/j.vph.2015.11.083
81. Mitchell GF, Hwang S-J, Vasan RS, Larson MG, Pencina MJ, Hamburg NM, et al. Arterial stiffness and cardiovascular events. Circulation (2010) 121(4):505L–511. doi:10.1161/CIRCULATIONAHA.109.886655
82. Ben-Shlomo Y, Spears M, Boustred C, May M, Anderson SG, Benjamin EJ, et al. Aortic pulse wave velocity improves cardiovascular event prediction: an individual participant meta-analysis of prospective observational data from 17,635 subjects. J Am Coll Cardiol (2014) 63(7):636–46. doi:10.1016/j.jacc.2013.09.063
83. Elkind M. Inflammation, atherosclerosis, and stroke. Neurologist (2006) 12:140–8. doi:10.1097/01.nrl.0000215789.70804.b0
84. Sugioka K, Hozumi T, Sciacca RR, Miyake Y, Titova I, Gaspard G, et al. Impact of aortic stiffness on ischemic stroke in elderly patients. Stroke (2002) 33(8):2077–81. doi:10.1161/01.STR.0000021410.83049.32
85. Laurent S, Katsahian S, Fassot C, Tropeano AI, Gautier I, Laloux B, et al. Aortic stiffness is an independent predictor of fatal stroke in essential hypertension. Stroke (2003) 34(5):1203–6. doi:10.1161/01.STR.0000065428.03209.64
86. Saini S, Saxena Y, Gupta R. Arterial compliance and autonomic functions in adult male smokers. J Clin Diagn Res (2016) 10(5):CC12–6. doi:10.7860/JCDR/2016/19547.7831
87. Harvey RE, Barnes JN, Hart ECJ, Nicholson WT, Joyner MJ, Casey DP. Influence of sympathetic nerve activity on aortic hemodynamics and pulse wave velocity in women. Am J Physiol Hear Circ Physiol (2017) 312(2):H340–6. doi:10.1152/ajpheart.00447.2016
88. Harris KF, Matthews KA. Interactions between autonomic nervous system activity and endothelial function: a model for the development of cardiovascular disease. Psychosom Med (2004) 66(2):153–64. doi:10.1097/01.psy.0000116719.95524.e2
89. Burnstock G. Local mechanisms of blood flow control by perivascular nerves and endothelium. J Hypertens Suppl (1990) 8(7):S95–106.
90. Acampa M, Franchi M, Guideri F, Lamberti I, Bruni F, Pastorelli M, et al. Cardiac dysautonomia and arterial distensibility in essential hypertensives. Auton Neurosci (2009) 146(1–2):102–5. doi:10.1016/j.autneu.2008.11.009
91. Hu M, Wang S, Wang D, Lai Q, Chen X, Duan S, et al. Combined moderate and high intensity exercise with dietary restriction improves cardiac autonomic function associated with a reduction in central and systemic arterial stiffness in obese adults: a clinical trial. PeerJ (2017) 5:e3900. doi:10.7717/peerj.3900
92. Ross R. Inflammation or atherogenesis. N Engl J Med (1999) 340(2):115–26. doi:10.1056/NEJM199901143400207
93. Huikuri HV, Jokinen V, Syva M, Nieminen MS, Airaksinen KEJ, Koistinen JM, et al. Heart rate variability and progression of coronary atherosclerosis. Arterioscler Thromb Vasc Biol (1999) 19:1979–85. doi:10.1161/01.ATV.19.8.1979
94. Manfrini O, Pizzi C, Viecca M, Bugiardini R. Abnormalities of cardiac autonomic nervous activity correlate with expansive coronary artery remodeling. Atherosclerosis (2008) 197(1):183–9. doi:10.1016/j.atherosclerosis.2007.03.013
95. Shebuski RJ, Kilgore KS. Role of inflammatory mediators in thrombogenesis. J Pharmacol Exp Ther (2002) 300:729–35. doi:10.1124/jpet.300.3.729
96. Badimon L, Vilahur G. Thrombosis formation on atherosclerotic lesions and plaque rupture. J Intern Med (2014) 276(6):618–32. doi:10.1111/joim.12296
97. Steptoe A, Kivimäki M. Stress and cardiovascular disease. Nat Rev Cardiol (2012) 9(6):360–70. doi:10.1038/nrcardio.2012.45
98. Kleiger RE, Miller JP, Bigger JT, Moss AJ. Decreased heart rate variability and its association with increased mortality after acute myocardial infarction. Am J Cardiol (1987) 59:256–67. doi:10.1016/0002-9149(87)90795-8
99. Bigger JT, Fleiss JL, Steinman RC, Rolnitzky LM, Kleiger RE, Rottman JN. Frequency domain measures of heart period variability and mortality after myocardial infarction. Circulation (1992) 85(1):164–71. doi:10.1161/01.CIR.85.1.164
100. Huikuri HV, Raatikainen MJP, Moerch-Joergensen R, Hartikainen J, Virtanen V, Boland J, et al. Prediction of fatal or near-fatal cardiac arrhythmia events in patients with depressed left ventricular function after an acute myocardial infarction. Eur Heart J (2009) 30(6):689–98. doi:10.1093/eurheartj/ehn537
101. Jokinen V, Tapanainen JM, Seppänen T, Huikuri HV. Temporal changes and prognostic significance of measures of heart rate dynamics after acute myocardial infarction in the beta-blocking era. Am J Cardiol (2003) 92(8):907–12. doi:10.1016/S0002-9149(03)00968-8
102. Sandercock GRH, Grocott-Mason R, Brodie DA. Changes in short-term measures of heart rate variability after eight weeks of cardiac rehabilitation. Clin Auton Res (2007) 17(1):39–45. doi:10.1007/s10286-007-0392-5
103. Goldstein LB, Bushnell CD, Adams RJ, Appel LJ, Braun LT, Chaturvedi S, et al. Guidelines for the primary prevention of stroke: a guideline for healthcare professionals from the American Heart Association/American Stroke Association. Stroke (2011) 42(2):517–84. doi:10.1161/STR.0b013e3181fcb238
104. Wolf PA, Abbott RD, Kannel WB. Atrial fibrillation as an independent risk factor for stroke: the Framingham study. Stroke (1991) 22:983–8. doi:10.1161/01.STR.22.8.983
105. Riccio PM, Klein FR, Pagani Cassará F, Muñoz Giacomelli F, González Toledo ME, Racosta JM, et al. Newly diagnosed atrial fibrillation linked to wake-up stroke and TIA: hypothetical implications. Neurology (2013) 80(20):1834–40. doi:10.1212/WNL.0b013e318292a330
106. Patterson E, Po S, Scherlag B, Lazzara R. Triggered firing in pulmonary veins initiated by in vitro autonomic nerve stimulation. Hear Rhythm (2005) 2:624–31. doi:10.1016/j.hrthm.2005.02.012
107. Coumel P, Attuel P, Lavallée J, Flammang D, Leclercq JF, Slama R. The atrial arrhythmia syndrome of vagal origin. Arch Mal Coeur Vaiss (1978) 6:645–9.
108. Shen MJ, Choi E-K, Tan AY, Lin S-F, Fishbein MC, Chen LS, et al. Neural mechanisms of atrial arrhythmias. Nat Rev Cardiol (2012) 9(1):30–9. doi:10.1038/nrcardio.2011.139
109. Perkiömäki J, Ukkola O, Kiviniemi A, Tulppo M, Ylitalo A, Kesäniemi YA, et al. Heart rate variability findings as a predictor of atrial fibrillation in middle-aged population. J Cardiovasc Electrophysiol (2014) 25(7):719–24. doi:10.1111/jce.12402
110. Jons C, Raatikainen P, Gang UJ, Huikuri HV, Joergensen RM, Johannesen A, et al. Autonomic dysfunction and new-onset atrial fibrillation in patients with left ventricular systolic dysfunction after acute myocardial infarction: a CARISMA substudy. J Cardiovasc Electrophysiol (2010) 21(9):983–90. doi:10.1111/j.1540-8167.2010.01795.x
111. Bettoni M, Zimmermann M. Autonomic tone variations before the onset of paroxysmal atrial fibrillation. Circulation (2002) 105:2753–9. doi:10.1161/01.CIR.0000018443.44005.D8
112. Healey JS, Baranchuk A, Crystal E, Morillo CA, Garfinkle M, Yusuf S, et al. Prevention of atrial fibrillation with angiotensin-converting enzyme inhibitors and angiotensin receptor blockers: a meta-analysis. J Am Coll Cardiol (2005) 45(11):1832–9. doi:10.1016/j.jacc.2004.11.070
113. Fuster V, Rydén LE, Cannom DS, Crijns HJ, Curtis AB, Ellenbogen KA, et al. 2011 ACCF/AHA/HRS focused updates incorporated into the ACC/AHA/ESC 2006 guidelines for the management of patients with atrial fibrillation. J Am Coll Cardiol (2011) 57(11):e101–98. doi:10.1016/j.jacc.2010.09.013
114. Acampa M, Lazzerini PE, Guideri F, Tassi R, Martini G. Ischemic stroke after heart transplantation. J Stroke (2016) 18(2):157–68. doi:10.5853/jos.2015.01599
115. Pérez-Miralles F, Sánchez-Manso JC, Almenar-Bonet L, Sevilla-Mantecón T, Martínez-Dolz L, Vílchez-Padilla JJ. Incidence of and risk factors for neurologic complications after heart transplantation. Transplant Proc (2005) 37(9):4067–70. doi:10.1016/j.transproceed.2005.09.162
116. Awad M, Czer LSC, Hou M, Golshani SS, Goltche M, De Robertis M, et al. Early denervation and later reinnervation of the heart following cardiac transplantation: a review. J Am Heart Assoc (2016) 5(11):1–22. doi:10.1161/JAHA.116.004070
117. Keeley EC, Toth ZK, Goldberg AD. Long-term assessment of heart rate variability in cardiac transplant recipients. J Hear Lung Transplant (2000) 19(3):310–2. doi:10.1016/S1053-2498(99)00125-4
118. Halpert I, Goldberg AD, Levine AB, Levine TB, Kornberg R, Kelly C, et al. Reinnervation of the transplanted human heart as evidenced from heart rate variability studies. Am J Cardiol (1996) 77(2):180–3. doi:10.1016/S0002-9149(96)90592-5
119. Buendía Fuentes F, Martínez-Dolz L, Almenar Bonet L, Sánchez-Lázaro I, Navarro Manchón J, Sánchez-Gómez JM, et al. Normalization of the heart rate response to exercise 6 months after cardiac transplantation. Transplant Proc (2010) 42(8):3186–8. doi:10.1016/j.transproceed.2010.05.056
120. Yadava M, Hughey AB, Crawford TC. Postoperative atrial fibrillation: incidence, mechanisms, and clinical correlates. Heart Fail Clin (2016) 12(2):299–308. doi:10.1016/j.hfc.2015.08.023
121. Thijs V, Lemmens R, Farouque O, Donnan G, Heidbuchel H. Postoperative atrial fibrillation: target for stroke prevention? Eur Stroke J (2017) 2(3):222–8. doi:10.1177/2396987317719363
122. Marigold JRG, Arias M, Vassallo M, Allen SC, Kwan JS. Autonomic dysfunction in older people. Rev Clin Gerontol (2010) 21(1):28–44. doi:10.1017/S0959259810000286
123. Collins KJ. Aging, disease and the autonomic nervous system. Rev Clin Gerontol (1997) 7(2):119–26. doi:10.1017/S0959259897000130
124. Antelmi I, De Paula R, Shinzato A, Peres C, Mansur A, Grupi C. Influence of age, gender, body mass index, and functional capacity on heart rate variability in a cohort of subjects without heart disease. Am J Cardiol (2004) 93(3):381–5. doi:10.1016/j.amjcard.2003.09.065
125. Stein PK, Barzilay JI, Chaves PHM, Domitrovich PP, Gottdiener JS. Heart rate variability and its changes over 5 years in older adults. Age Ageing (2009) 38(2):212–8. doi:10.1093/ageing/afn292
126. Harte CB, Meston CM. Effects of smoking cessation on heart rate variability among long-term male smokers. Int J Behav Med (2014) 21(2):302–9. doi:10.1007/s12529-013-9295-0
127. Yuksel R, Yuksel RN, Sengezer T, Dane S. Autonomic cardiac activity in patients with smoking and alcohol addiction by heart rate variability analysis. Clin Invest Med (2016) 39(6):147–53. doi:10.25011/cim.v39i6.27519
128. Hayano J, Yamada M, Sakakibara Y, Fujinami T, Yokoyama K, Watanabe Y, et al. Short- and long-term effects of cigarette smoking on heart rate variability. Am J Cardiol (1990) 65:84–8. doi:10.1016/0002-9149(90)90030-5
129. Thayer JF, Hall M, Sollers JJ, Fischer JE. Alcohol use, urinary cortisol, and heart rate variability in apparently healthy men: evidence for impaired inhibitory control of the HPA axis in heavy drinkers. Int J Psychophysiol (2006) 59(3):244–50. doi:10.1016/j.ijpsycho.2005.10.013
130. Irwin MR, Valladares EM, Motivala S, Thayer JF, Ehlers CL. Association between nocturnal vagal tone and sleep depth, sleep quality, and fatigue in alcohol dependence. Psychosom Med (2006) 68(1):159–66. doi:10.1097/01.psy.0000195743.60952.00
131. Sloan RP, Shapiro PA, DeMeersman RE, Bagiella E, Brondolo EN, McKinley PS, et al. The effect of aerobic training and cardiac autonomic regulation in young adults. Am J Public Health (2009) 99(5):921–8. doi:10.2105/AJPH.2007.133165
132. Earnest CP, Blair SN, Church TS. Heart rate variability and exercise in aging women. J Womens Health (Larchmt) (2012) 21(3):334–9. doi:10.1089/jwh.2011.2932
133. Campbell J, Ehlert U. Acute psychosocial stress: does the emotional stress response correspond with physiological responses? Psychoneuroendocrinology (2012) 37(8):1111–34. doi:10.1016/j.psyneuen.2011.12.010
134. Kemeny ME. The psychobiology of stress. Curr Dir Psychol Sci (2003) 12(4):124–9. doi:10.1111/1467-8721.01246
135. Booth J, Connelly L, Lawrence M, Chalmers C, Joice S, Becker C, et al. Evidence of perceived psychosocial stress as a risk factor for stroke in adults: a meta-analysis. BMC Neurol (2015) 15:233. doi:10.1186/s12883-015-0456-4
136. Dishman RK, Nakamura Y, Garcia ME, Thompson RW, Dunn AL, Blair SN. Heart rate variability, trait anxiety, and percieved stress among physically fit men and women. Int J Psychophysiol (2000) 37:121–33. doi:10.1016/S0167-8760(00)00085-4
137. Miu AC, Heilman RM, Miclea M. Reduced heart rate variability and vagal tone in anxiety: trait versus state, and the effects of autogenic training. Auton Neurosci (2009) 145(1–2):99–103. doi:10.1016/j.autneu.2008.11.010
138. Hall M, Vasko R, Buysse D, Ombao H, Chen Q, Cashmere JD, et al. Acute stress affects heart rate variability during sleep. Psychosom Med (2004) 66(1):56–62. doi:10.1097/01.PSY.0000106884.58744.09
139. McColl BW, Allan SM, Rothwell NJ. Systemic infection, inflammation and acute ischemic stroke. Neuroscience (2009) 158(3):1049–61. doi:10.1016/j.neuroscience.2008.08.019
140. Toweill D, Sonnenthal K, Kimberly B, Lai S, Goldstein B. Linear and nonlinear analysis of hemodynamic signals during sepsis and septic shock. Crit Care Med (2000) 28(6):2051–7. doi:10.1097/00003246-200006000-00063
141. Buchman TG, Stein PK, Goldstein B. Heart rate variability in critical illness and critical care. Curr Opin Crit Care (2002) 8(4):311–5. doi:10.1097/00075198-200208000-00007
142. Schmidt JM, Sow D, Crimmins M, Albers D, Agarwal S, Claassen J, et al. Heart rate variability for preclinical detection of secondary complications after subarachnoid hemorrhage. Neurocrit Care (2014) 20(3):382–9. doi:10.1007/s12028-014-9966-y
143. Ishida S, Nakagawa M, Fujino T, Yonemochi H, Saikawa T, Ito M. Circadian variation of QT interval dispersion: correlation with heart rate variability. J Electrocardiol (1997) 30(3):205–10. doi:10.1016/S0022-0736(97)80005-2
144. Burger AJ, Charlamb M, Sherman HB. Circadian patterns of heart rate variability in normals, chronic stable angina and diabetes mellitus. Int J Cardiol (1999) 71(1):41–8. doi:10.1016/S0167-5273(99)00110-2
145. Huikuri HV, Niemela MJ, Ojala S, Rantala A, Ikaheimo MJ, Airaksinen KE. Circadian rhythms of frequency domain measures of heart rate variability in healthy subjects and patients with coronary artery disease. Effects of arousal and upright posture. Circulation (1994) 90(1):121–6. doi:10.1161/01.CIR.90.1.121
146. Chandratheva A, Metha Z, Geraghty OC, Marquardt L, Rothwell PM. Population-based study of risk of stroke in the first few hours after a TIA. Neurology (2009) 72(22):1941–7. doi:10.1212/WNL.0b013e3181a826ad
147. Coull AJ, Lovett JK, Rothwell PM; Oxford Vascular Study. Population based study of early risk of stroke after transient ischaemic attack or minor stroke: implications for public education and organisation of services. BMJ (2004) 328(7435):326. doi:10.1136/bmj.37991.635266.44
Keywords: autonomic nervous system, stress, heart rate variability, ischemic stroke, transient ischemic attack, prediction
Citation: Guan L, Collet J-P, Mazowita G and Claydon VE (2018) Autonomic Nervous System and Stress to Predict Secondary Ischemic Events after Transient Ischemic Attack or Minor Stroke: Possible Implications of Heart Rate Variability. Front. Neurol. 9:90. doi: 10.3389/fneur.2018.00090
Received: 30 September 2017; Accepted: 08 February 2018;
Published: 05 March 2018
Edited by:
Ayrton R. Massaro, Hospital Sírio-Libanês, BrazilReviewed by:
Jan F. Scheitz, Charité Universitätsmedizin Berlin, GermanyMaurizio Acampa, Azienda Ospedaliera Universitaria Senese, Italy
Copyright: © 2018 Guan, Collet, Mazowita and Claydon. This is an open-access article distributed under the terms of the Creative Commons Attribution License (CC BY). The use, distribution or reproduction in other forums is permitted, provided the original author(s) and the copyright owner are credited and that the original publication in this journal is cited, in accordance with accepted academic practice. No use, distribution or reproduction is permitted which does not comply with these terms.
*Correspondence: Jean-Paul Collet, amNvbGxldCYjeDAwMDQwO2N3LmJjLmNh