- 1Molecular Biology Center, State Key Laboratory of Trauma, Burn, and Combined Injury, Research Institute of Surgery and Daping Hospital, Third Military Medical University, Chongqing, China
- 2Department of Neurology, Research Institute of Surgery and Daping Hospital, Third Military Medical University, Chongqing, China
- 3Department of ICU, Research Institute of Surgery and Daping Hospital, Third Military Medical University, Chongqing, China
Background: Acute lung injury (ALI) is a serious complication of stroke that occurs with a high incidence. Our preclinical results indicated that ALI might be related to blood glutamate levels after brain injury. The purpose of this study was to assess dynamic changes in blood glutamate levels in patients with stroke and to determine the correlation between blood glutamate levels, ALI, and long-term prognosis after stroke.
Methods: Venous blood samples were collected from controls and patients with stroke at admission and on the third and seventh day after the onset of stroke. Patients were followed for 3 months. The correlations among blood glutamate levels, severities of stroke and ALI, and long-term outcomes were analyzed, and the predictive values of blood glutamate levels and severity scores for ALI were assessed.
Results: In this study, a total of 384 patients with stroke were enrolled, with a median age of 59 years. Patients showed significantly increased blood glutamate levels within 7 days of stroke onset (p < 0.05), and patients with more severe injuries showed higher blood glutamate levels. Moreover, blood glutamate levels were closely related to the occurrence (adjusted odds ratio, 3.022, p = 0.003) and severity (p < 0.001) of ALI and the long-term prognosis after stroke (p < 0.05), and they were a more accurate predictor of ALI than the more commonly used severity scores (p < 0.01).
Conclusion: These results indicated that an increased blood glutamate level was closely related to the development of ALI and a poor prognosis after stroke.
Clinical Trial Registration: http://www.chictr.org.cn, identifier ChiCTR-RPC-15006770.
Introduction
With a reported incidence of 20–25% and a high rate of mortality or development of a persistent vegetative state, neurogenic acute lung injury (ALI) is one of the most serious systematic complications following brain injury (1, 2). ALI is primarily characterized by rapidly progressing hypoxemia and pulmonary edema early after brain injury (3, 4). To date, several risk factors for developing ALI after brain injury have been identified, including intracranial (e.g., midline shift and increased intracranial pressure) and extracranial (e.g., administration of vasoactive drugs and a history of drug abuse) factors; however, these factors have either been non-specific or difficult to measure, and an accurate, simple, and stable marker for ALI following brain injury is urgently needed (2, 3, 5).
Stroke, including primarily acute ischemic stroke (AIS), intracerebral hemorrhage (ICH), and spontaneous subarachnoid hemorrhage (SAH), is a serious threat to human health and life (6). After the occurrence of a stroke, elevated glutamate-induced excitotoxicity in the brain is known to severely affect patient prognosis (7, 8). Nevertheless, the role of glutamate levels in the blood has not been well characterized. Glutamate is a non-essential amino acid that participates in a series of basic metabolic reactions, such as deamination and gluconeogenesis (9). In addition, under physiological conditions, the blood levels of glutamate remain relatively stable, and a normal diet prevents significant fluctuations in blood glutamate levels (10); however, certain diets [e.g., rich in monosodium glutamate (MSG)] will cause a significant increase in blood glutamate levels (11). Moreover, researchers have recently found that blood glutamate levels are greatly increased in many patients who experience stroke (12, 13). Our previous animal experiments have indicated that elevated blood glutamate levels might be related to the development of ALI after brain injury (14), although there was no evidence of a close relationship in a large scale clinical study in patients with stroke. Furthermore, since these subtypes of stroke were very different in terms of their pathophysiology, evaluation of whether this is a shared phenomenon between ischemic and hemorrhagic stroke is necessary.
The aim of this study was to evaluate the relationship between blood glutamate levels and the incidence of ALI in patients with stroke, the ability of blood glutamate levels to predict the occurrence of ALI and the relationship between glutamate levels and the long-term prognosis following stroke. The results of this study increase our understanding of this common complication following brain insult.
Patients and Methods
Patients
Hospitalized patients were consecutively enrolled from Daping Hospital (Chongqing, China), which has over 2,500 beds and is ranked among the top 100 hospitals in China. It is also a teaching hospital affiliated with the Third Military Medical University and serves as a base for stroke screening and prevention in China. We enrolled patients admitted to the hospital within 6 h of presenting with clinical stroke symptoms and who received a diagnosis of ischemic or hemorrhagic stroke. A control group included 120 subjects who received a routine physical examination, were not diagnosed with stroke, and had no history of stroke/transient ischemic attack (TIA), hypertension, hyperlipidemia, hypercholesterolemia, diabetes mellitus, myocardial infarction, or atrial fibrillation. All participants were Han Chinese living in Chongqing and the surrounding provinces with an age ≥18 years. The diagnosis of stroke was confirmed in all patients by computed tomography (CT) or magnetic resonance imaging. The key exclusion criteria were as follows: transferred from other hospitals or departments; the presence of severe hepatic disease [e.g., aspirate transaminase (AST) levels >40 U/L or alanine aminotransferase (ALT) levels >40 U/L], current or previous lung disease (e.g., tuberculosis, pneumonia, or chronic obstructive pulmonary disease), or abnormal clinical manifestations (e.g., dyspnea, shortness of breath, or cyanosis), and failure of one or more organs. Stroke severity was quantified by a neurologist according to the National Institutes of Health Stroke Scale (NIHSS) score, ICH score, or World Federation of Neurosurgical Surgeons (WFNS) scale at admission and after treatment for patients with AIS, ICH, or SAH, respectively; based on the scores at admission, the patients were divided into mild (NIHSS score: ≤8; ICH score: ≤2; and WFNS scale: I), moderate (NIHSS score: 9–15; ICH score: 3–4; and WFNS scale: II–III), and severe (NIHSS score: ≥16; ICH score: 5–6; and WFNS scale: IV–V) groups (15–18). The detailed sociodemographic and baseline characteristics of patients and controls are listed in Table 1.
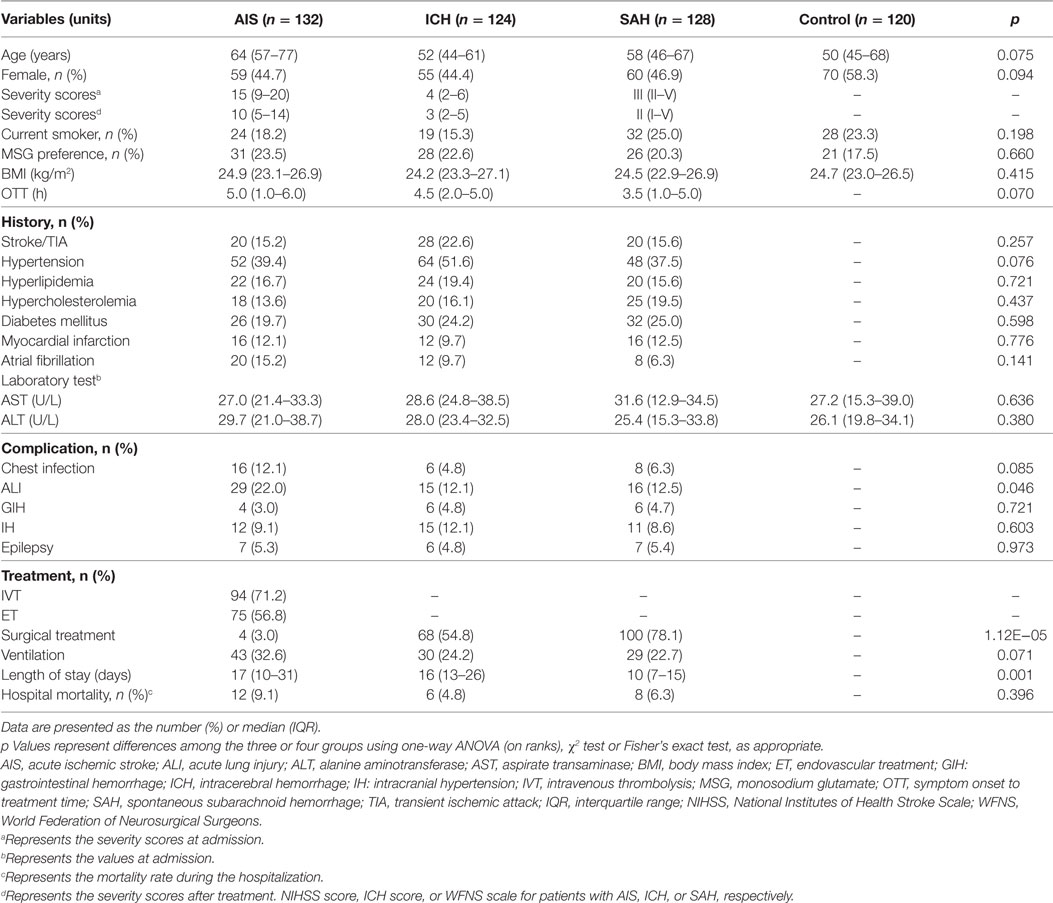
Table 1. The sociodemographic and baseline characteristics of stroke patients (n = 384) and the controls (n = 120).
This study was performed in accordance with the recommendations of international ethical guidelines for biomedical research involving human subjects, and the ethics committee of the Research Institute of Surgery and Daping Hospital approved this study (No. 2015-12). All participants (or legal guardians) in this study provided written informed consent in accordance with the Declaration of Helsinki and its later amendments. This clinical trial was registered at the Chinese Clinical Trial Registry (www.chictr.org.cn; unique identifier: ChiCTR-RPC-15006770).
Diagnosis and Classification of ALI
According to the Berlin Definition for ALI/ARDS (19), after admission, stroke patients with ALI would quickly present (usually within 72 h) with new or worsening respiratory symptoms (e.g., shortness of breath and dyspnea), an acute onset of hypoxemia (PaO2/FiO2 ≤ 300 mm Hg), and a radiographic examination (representative images, e.g., chest X-ray or CT, are shown in Figure S1 in Supplementary Material) indicating high-density patchy shadows or edema not fully explained by effusion, lobar/lung collapse, or nodules; the respiratory failure symptoms could not be fully explained by cardiac failure, which was excluded by echocardiographic measurements (20, 21). In addition, based on the degree of hypoxemia, the severity of ALI could be classified as mild (200 mm Hg < PaO2/FiO2 ≤ 300 mm Hg), moderate (100 mm Hg < PaO2/FiO2 ≤ 200 mm Hg), or severe (PaO2/FiO2 ≤ 100 mm Hg) (19, 20).
Clinical Management of Patients with Stroke
Treatment protocols were implemented by a single attending neurologist. For patients with AIS, intravenous thrombolysis (IVT) or endovascular treatment (ET) was performed after rigorous assessment, while for patients with ICH or SAH, surgical (decompressive craniectomy) or conservative treatment (e.g., reduction of intracranial pressure via mannitol) was used accordingly. In addition, at the moment the diagnosis of ALI was made, the patients were transferred to the ICU, and treatment was based primarily on ventilation with positive end-expiratory pressure (PEEP), following the ARDSnet protocol (22, 23).
Blood Collection, Processing, and Glutamate Assay
Venous blood samples were collected from the patients at the time of admission (without any treatment and within 6 h from stroke onset) and on the mornings of the third day and seventh day. Blood was obtained from controls during routine physical examination. All samples were anticoagulated with heparin; then, using standard clinical laboratory methods, the samples were evaluated for inflammatory markers, such as IL-6, C-reactive protein, and procalcitonin, and routine markers, including hepatic function markers, such as ALT and AST, and nerve injury markers, such as neuron-specific enolase (NSE) and S-100B. Blood glutamate levels were assayed using high-performance liquid chromatography with spectrofluorometric detection (Beckman Coulter, CA, USA) at a fixed excitation wavelength of 330 nm and a fixed emission wavelength of 450 nm, according to our previously published methodology (24).
Outcome Measurement
Mortality data were collected over a follow-up period of 3 months. The long-term outcomes were evaluated by modified Rankin scale (mRS) at 90 days by phone interview by an experienced neurologist. Favorable outcomes and functional independence were defined as an mRS score of 0–2 (25).
Statistical Analysis
The justification of sample sizes in each group was performed as previously described (26), in which α = 0.05, 1 − β (power analysis) = 0.90, and σ = 10.0. All results are expressed as percentages for categorical variables and as the means (SE or SD) or medians [interquartile range (IQR)] for all variables. Proportions were compared using the χ2 test or Fisher’s exact test, and ANOVA followed by Bonferroni post hoc tests or Kruskal–Wallis H tests, two-tailed t-tests, or non-parametric Mann–Whitney U tests were used to compare continuous or discrete variables among groups, as appropriate. Spearman correlation or uni-/multivariate logistic regression was used to analyze relationships between blood glutamate levels and other variables [including the occurrence and severity of ALI and clinical characteristics, e.g., age, sex, body mass index (BMI), symptom onset to treatment time (OTT), smoking status, MSG dietary preference, medical history, treatment, and levels of inflammatory markers]. The results are expressed as r values or as unadjusted/adjusted odds ratios (ORs) with corresponding 95% confidence intervals (CIs), with post hoc power analyses >0.90 (27). Areas under receiver-operating characteristic curves were utilized to evaluate and compare the accuracy of blood glutamate levels and severity scores (NIHSS score, ICH score, and WFNS scale) for predicting the occurrence of ALI (28). In addition, the possibility of defining a “cutoff value” of blood glutamate level associated with survival and poor functional outcome was explored using Kaplan–Meier survival curve analysis, followed by Log Rank testing and multivariate logistic regression, respectively. Two-tailed p values <0.05 represent statistically significant differences. All statistical analyses were performed using Sigma Plot (version 12.5; Systat Software Inc., San Jose, CA, USA).
Results
Between August 2015 and July 2016, a total of 450 patients were consecutively screened in our institution’s Department of Neurology and Department of ICU. Of these, 49 patients were excluded, and 17 patients discharged or died within 7 days. Ultimately, 384 patients were included and analyzed in this study, including 132 patients with AIS, 124 patients with ICH, and 128 patients with SAH (Figure 1). The median age of the study participants was 59 years (range, 18–89), the median NIHSS score, ICH score, or WFNS scale for patients with AIS, ICH, or SAH at admission was 15, 4, and III, respectively; after treatment, the median NIHSS score decreased markedly (p = 0.032). The median time of OTT for all patients was 5 h. There was no significant difference among these three subgroups of patients concerning the number of current smokers and MSG dietary preferences, medical history, and laboratory tests (p > 0.05). Thirty patients (7.8%) suffered a chest infection (pneumonia or bronchitis), 60 (15.6%) were diagnosed with ALI, and 16 (4.2%), 38 (9.9%), and 20 (5.2%) patients developed gastrointestinal hemorrhage, intracranial hypertension, and epilepsy, respectively. Ninety-four patients (71.2%) with AIS were treated with IVT, and 75 patients (56.8%) were treated with ET. Surgery was performed on most patients with ICH (54.8%) or SAH (78.1%), and 102 (26.6%) received ventilation therapy (Table 1). Most of the ALI cases (91.6%) developed within 24 h after brain injury, and the prevalence of severe ALI was 31.7%. All patients diagnosed with ALI were managed in the ICU with a median PEEP value of 8 cm H2O (Table 2). The total mortality was 6.8% (26 patients, including 12 with ALI) after admission during the hospitalization.
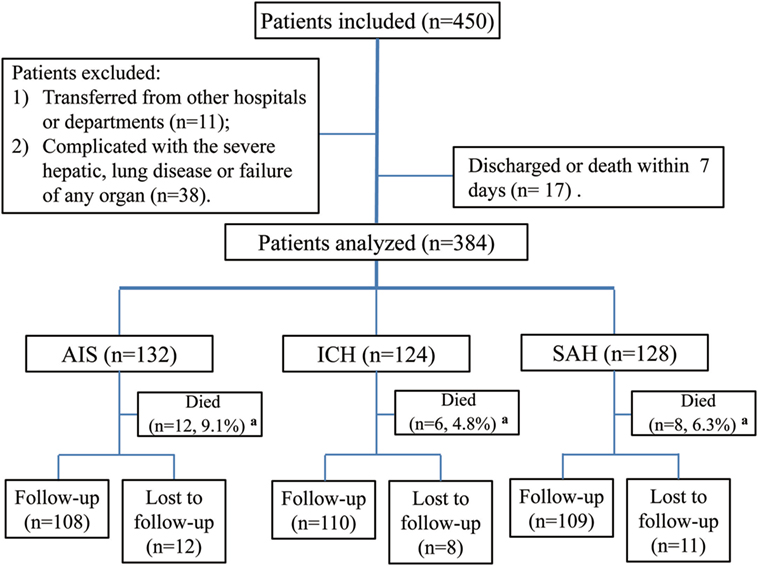
Figure 1. Study flowchart. Abbreviations: AIS, acute ischemic stroke; ICH, intracerebral hemorrhage; SAH, spontaneous subarachnoid hemorrhage. aRepresents death during hospitalization.
Alterations in Blood Glutamate Levels in Patients with Different Causes and Severities of Stroke
The observed alterations in blood glutamate levels varied among patients with different causes of stroke; glutamate levels were highest and most sustained in patients with AIS, followed by patients with ICH and those with SAH. However, for each subgroup of stroke, there were relatively few changes in glutamate levels within 7 days of admission (Figure 2A). We further found that patients with severe or moderate stroke exhibited significantly increased blood glutamate levels; these levels were higher with increased stroke severity (Figures 2B–D).
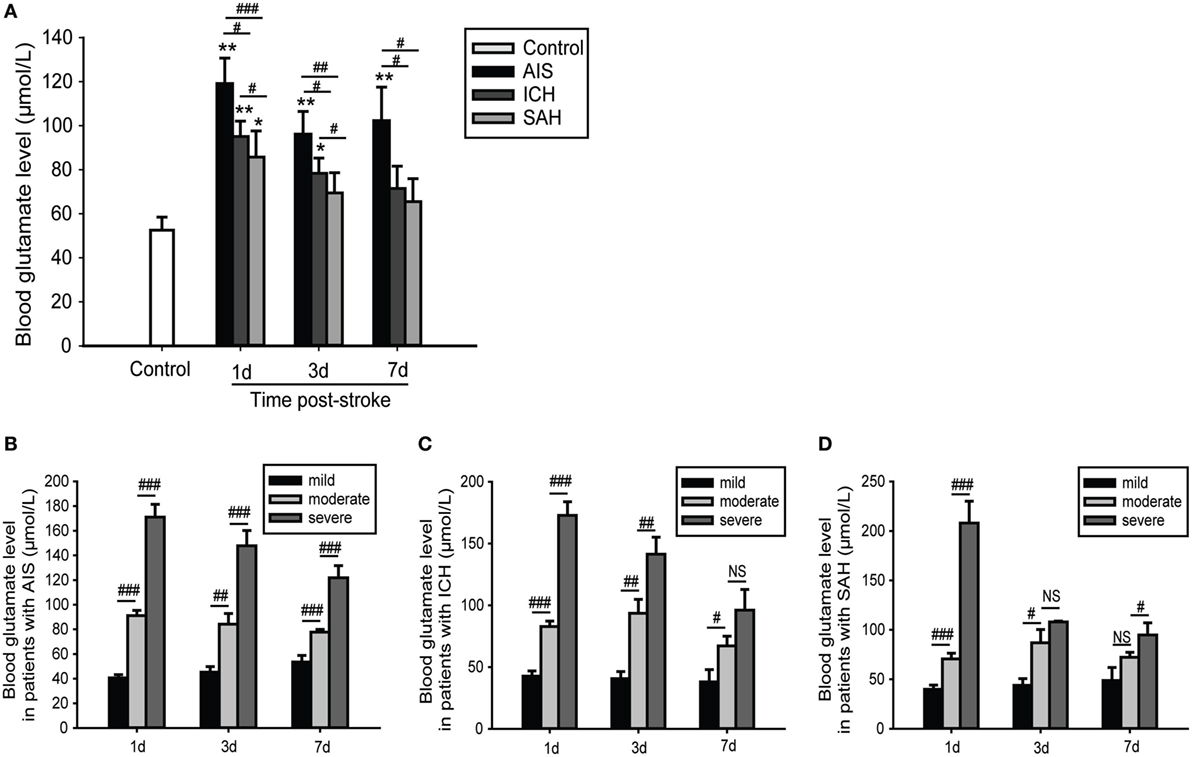
Figure 2. Time- and severity-dependent changes in blood glutamate levels in patients with stroke. (A) Comparison of blood glutamate levels with different causes of stroke. (B–D) Comparison of blood glutamate levels with different severities of stroke. *p < 0.05, **p < 0.01, and ***p < 0.001 compared with the control group; #p < 0.05, ##p < 0.01, and ###p < 0.001 compared between the two groups. Data are expressed as the means (SE), and the significance was determined by ANOVA followed by Bonferroni post hoc tests or Kruskal–Wallis H tests. Abbreviations: AIS, acute ischemic stroke; ICH, intracerebral hemorrhage; NIHSS, National Institutes of Health Stroke Scale; SAH, spontaneous subarachnoid hemorrhage.
Blood Glutamate Levels but Not Severity Scores Were Closely Related to the Incidence and Severity of ALI
For all of the stroke patients, the median severity scores of patients who developed ALI were much higher than those without ALI (Figures 3A–C). However, logistic regression analysis showed that these were not closely related with the occurrence of ALI when adjusted for confounding factors (adjusted OR: 1.060, 95% CI: 0.852–1.320, p = 0.600 for NIHSS score; adjusted OR: 1.083, 95% CI: 0.890–1.161, p = 0.060 for ICH score; and adjusted OR: 1.036, 95% CI: 0.974–1.390, p = 0.153 for WFNS scale) (Table 3). However, blood glutamate levels were much higher in patients with ALI with different stroke severities (Figures 3D–F), and the increased blood glutamate levels were associated with a higher risk of ALI (adjusted OR: 3.022, 95% CI: 2.001–4.043, p = 0.003) (Table 3). Moreover, the blood glutamate levels were closely related to the severity of ALI in patients with AIS (r = 0.591, p = 1.198E−04), ICH (r = 0.564, p = 2.627E−05), and SAH (r = 0.842, p = 2.922E−04).
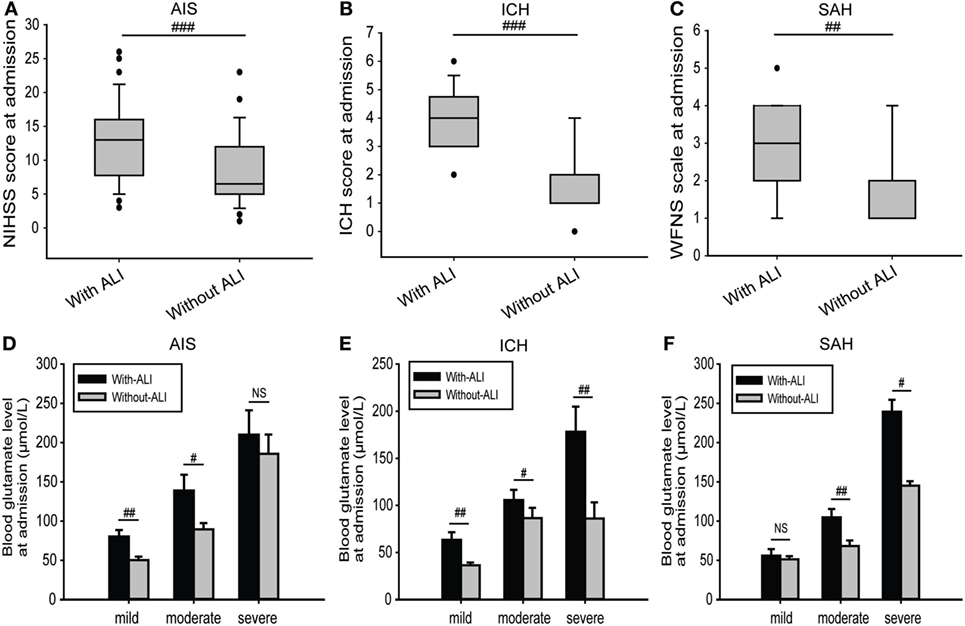
Figure 3. Comparisons of severity scores and blood glutamate levels at admission between patients with and those without ALI. (A–C) Comparison of severity scores at admission for patients in different subgroups and with or without ALI. (D–F) Comparison of blood glutamate levels at admission between different severities in patients with AIS, ICH, or SAH. Data are expressed as the median (IQR) (A–C) and means (SE) (D–F). #p < 0.05, ##p < 0.01, and ###p < 0.001 by two-tailed Student’s t-tests or non-parametric Mann–Whitney U tests; NS, no significance. Abbreviations: AIS, acute ischemic stroke; ICH, intracerebral hemorrhage; NIHSS, National Institutes of Health Stroke Scale; SAH, spontaneous subarachnoid hemorrhage; WFNS, World Federation of Neurosurgical Surgeons; ALI, acute lung injury; IQR, interquartile range.
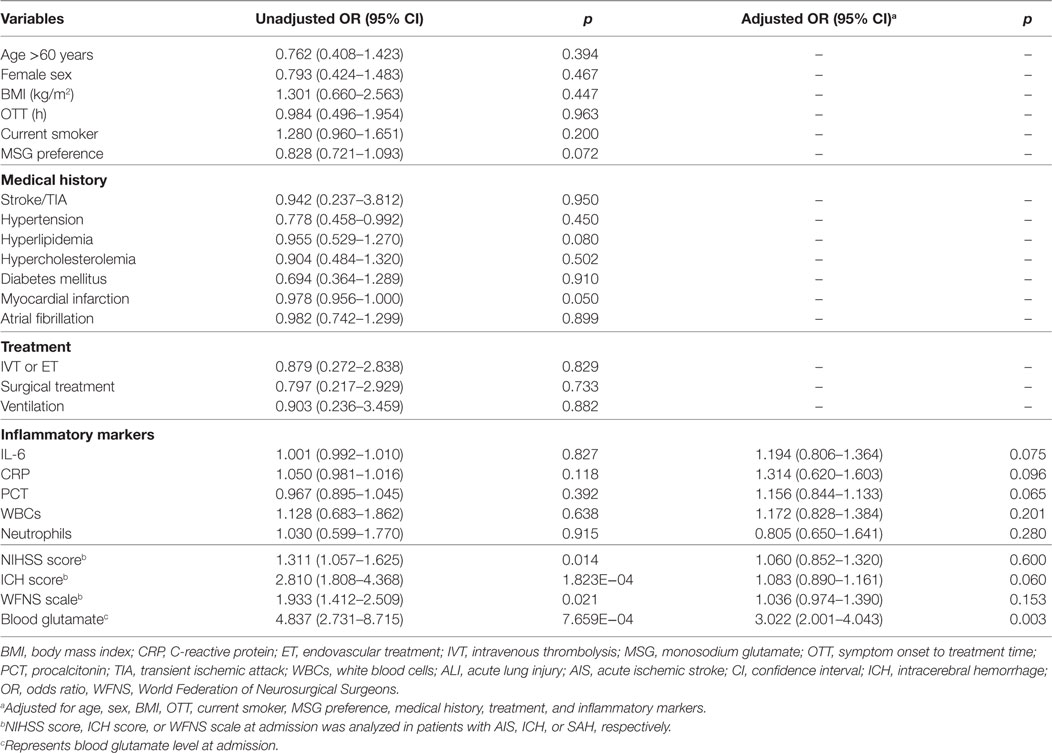
Table 3. ALI outcomes in relation to baseline and treatment characteristics of patients with stroke.
Blood Glutamate Level Analysis As a Sensitive and Specific Method for Predicting ALI
To obtain an early and accurate diagnosis of ALI, we next generated receiver-operating characteristic curves to assess the predictive accuracy of both blood glutamate levels and severity scores at admission. For all the patients with stroke, the optimal cutoff value for blood glutamate as an indicator of ALI was projected to be 87.6 µmol/L, which yielded an area under the curve (AUC) of 0.931 (95% CI: 0.905–0.957), with a sensitivity of 0.878 and a specificity of 0.892 (Figure 4A). The likelihood ratio test showed a significant increase in the predictive value of blood glutamate levels over severity scores (p = 1.665E−04, p = 0.002, and p = 0.009 for patients with AIS, ICH, and SAH, respectively). Moreover, the combination of glutamate levels and severity scores resulted in AUCs that were superior to severity scores but not superior to blood glutamate levels alone for predicting ALI (Figures 4B–D).
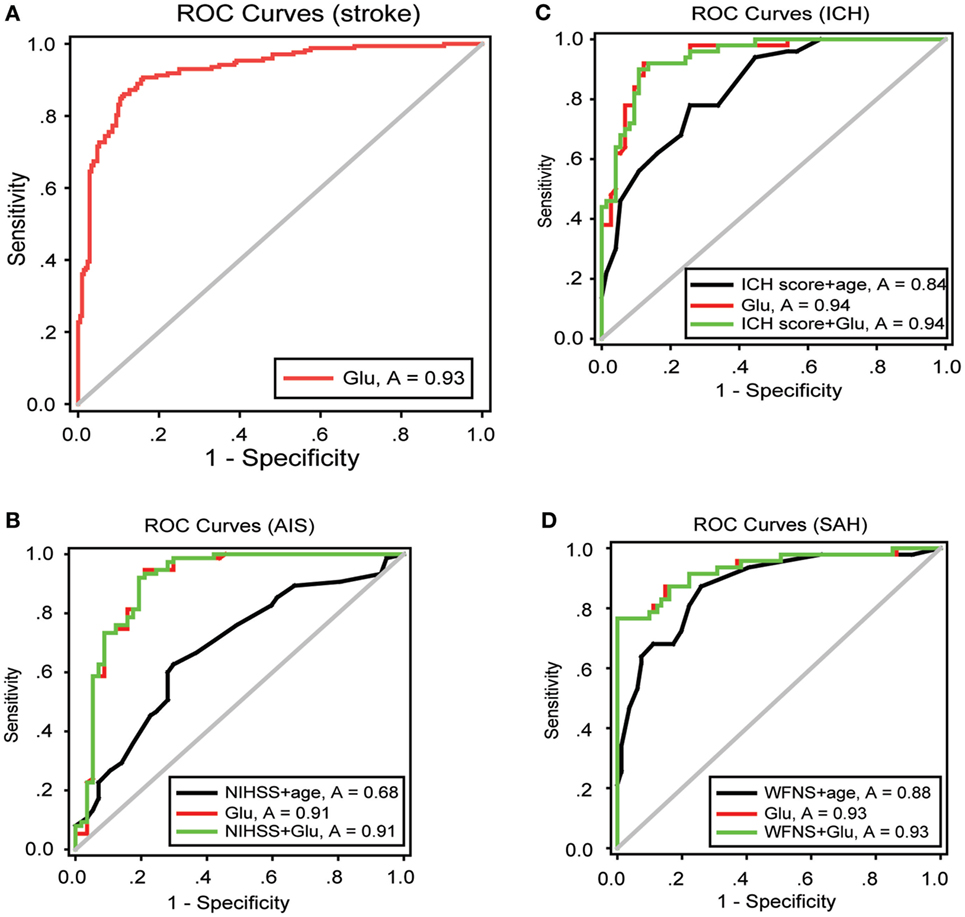
Figure 4. ROC curves showing the sensitivity versus specificity of different models for predicting ALI. (A) For all patients with stroke, the area under the curve was 0.931 (95% CI: 0.905–0.957) for glutamate levels at admission. (B–D) ROC curves for severity scores combined with age, glutamate levels at admission, and severity scores combined with glutamate levels in patients with AIS, ICH, and SAH, respectively. Abbreviations: AIS, acute ischemic stroke; ICH, intracerebral hemorrhage; NIHSS, National Institutes of Health Stroke Scale; SAH, spontaneous subarachnoid hemorrhage; WFNS, World Federation of Neurosurgical Surgeons; CI, confidence interval; ROC, receiver-operating characteristic.
Association between Blood Glutamate Levels and Long-term Clinical Outcomes
Thirty-one patients (8.7%) were lost to follow-up during the 90 days following discharge from the hospital. The analysis of blood glutamate level cutoff suggested that the survival rate was significantly lower for patients with high blood glutamate levels (AIS, p = 0.039; ICH, p = 1.325E−05; and SAH, p = 0.008) (Figures 5B–D). The distributions of mRS scores at 90 days in patients with the three stroke subtypes are shown in Figure 5A. For all three subtypes, after adjustment for potential confounders, patients with high blood glutamate levels were less likely to report functional independence [AIS (43.9 versus 60.0%; adjusted OR: 1.041, 95% CI: 1.039–1.043, p = 0.013), ICH (33.4 versus 55.7%; adjusted OR: 1.024, 95% CI: 1.023–1.025, p = 2.047E−04), and SAH (34.3 versus 52.7%; adjusted OR: 1.019, 95% CI: 1.018–1.020, p = 1.684E−04)]. In addition, in patients who died within 90 days of follow-up, cases with ALI who were diagnosed in the hospital were more likely to die than those without ALI (p = 0.004).
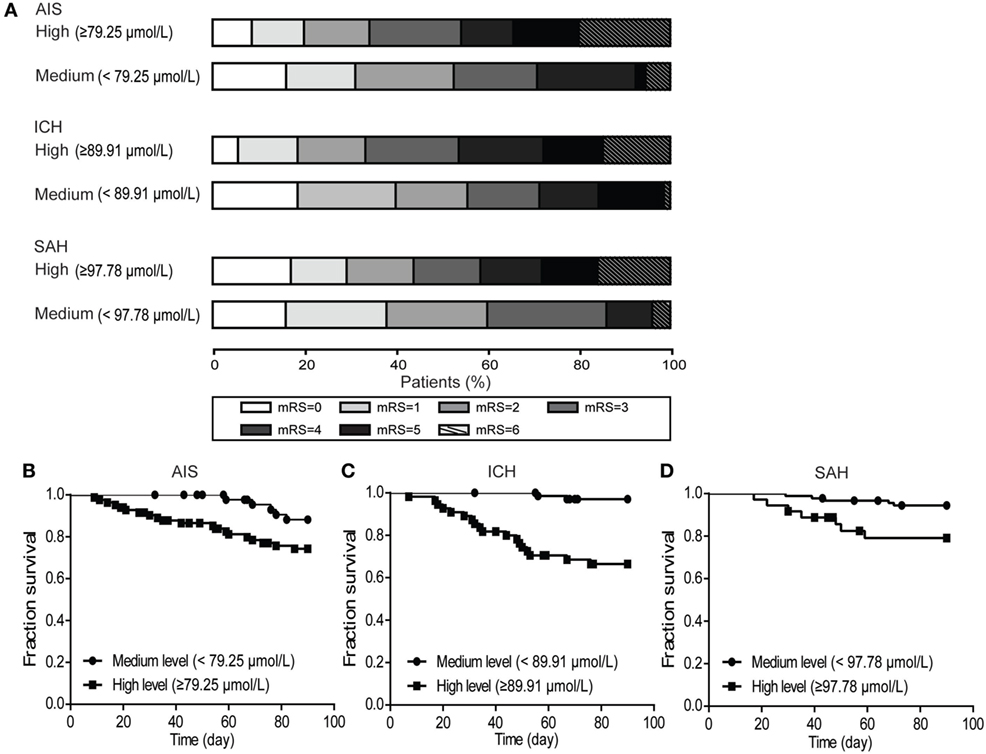
Figure 5. The distribution of modified Rankin scale (mRS) scores at 90 days and the poststroke survival curves. (A) Multivariate logistic regression analysis showed that patients with high blood glutamate levels were less likely to develop functional independence in patients with acute ischemic stroke (AIS) (p = 0.013), intracerebral hemorrhage (ICH) (p = 2.047E−04), and SAH (p = 1.684E−04). (B–D) Kaplan–Meier survival curve analysis showed that the survival rate was significantly lower in patients with high blood glutamate levels (AIS, p = 0.039; ICH, p = 1.325E−05; and SAH, p = 0.008).
Discussion
In this preliminary clinical study, we found that patients who experienced stroke (both hemorrhagic and ischemic) exhibited significantly increased blood glutamate levels; these levels could rise for approximately 7 days or more, according to previous work (12). In addition, we found that during hospitalization after a stroke (within 7 days), increased disease severity was associated with higher levels of blood glutamate. Patients with AIS exhibited higher levels of blood glutamate than patients with ICH or SAH; the proportion of patients in the AIS group who were diagnosed with ALI was also much higher than that in the ICH group and the SAH group. We speculate that this was partly because the severity of patients with AIS that we enrolled in this study was higher than that previously reported (12). Patients with ALI showed higher levels of brain injury markers (NSE and S-100B) (Table S1 in Supplementary Material) and severity scores than patients without ALI. Nevertheless, we found that blood glutamate levels but not the severity scores of stroke patients at admission could serve as a risk factor for the development of ALI, which was demonstrated by regression analysis after controlling for many potential confounding factors (including age, sex, BMI, and OTT, current smoking status, MSG dietary preference, medical history, treatment, and inflammatory markers). It was noteworthy that patients with a history of myocardial infarction showed a marginal association (p = 0.050) with ALI, partly because these patients always showed other risk factors for stroke (29). The severity scores were always influenced by these confounding factors, especially the OTT (30, 31), which may partly account for these discrepancies.
As the clinical manifestations of ALI lack specificity and are often overshadowed by primary disease, and because ALI typically develops rapidly (32), there is an urgent need to identify an objective and easily measurable parameter that can predict the occurrence of ALI. Several scores at admission have been commonly used to evaluate the severity and predict prognosis after brain injury (33–35); however, we found that blood glutamate levels were more accurate and reliable for predicting the occurrence of ALI and were closely related to the long-term prognosis. In addition, we found blood glutamate levels were always much higher in patients with ALI than in patients without ALI; in patients with ALI, blood glutamate levels gradually decreased over the length of the hospital stay, whereas few changes in blood glutamate levels were observed in patients without ALI (Figure S2B in Supplementary Material). These results collectively demonstrate the potential of using blood glutamate levels in predicting ALI after stroke and indicate that although the pathogenesis is different, the predictive value of blood glutamate levels in different types of stroke is worthy of further investigation.
The inflammatory reaction is an important facet of the occurrence and development of ALI (21). In this study, we also measured the levels of inflammatory markers in blood; we found that patients with ALI had much higher levels of inflammatory markers than patients without ALI (Table S1 in Supplementary Material), and additional analysis showed a positive correlation between blood glutamate levels and IL-6 levels during hospitalization (Figure S3 in Supplementary Material). After brain injury, the activation of the sympathetic nervous system and the hypothalamic–pituitary–adrenal axis has been shown to be associated with the development of systemic inflammatory complications (36, 37); however, it is noteworthy that in patients without ALI but who were diagnosed with a complicated chest infection, even though inflammatory mediator levels in these patients were similar to those in patients with ALI, the mean glutamate levels in these patients were much lower (Figure S2A in Supplementary Material). In addition, our previous animal experiments demonstrated that high concentrations of blood glutamate mediate interactions between the adenosine A2A receptor and the metabotropic glutamate receptor 5 on neutrophils, thereby inducing the release of inflammatory mediators (14). These results indicate not only that the elevated blood glutamate and inflammatory marker levels were not simply a concomitant phenomenon but also that blood glutamate might play a precipitating role in the occurrence of ALI, and this role may be related to the pro-inflammatory effects of glutamate. However, inflammatory cells, including neutrophils (38) and platelets (12, 39), also release glutamate into the blood after brain injury, thereby forming a mutually amplifying system that strengthens the effects of blood glutamate. These hypotheses and the specific mechanism underlying this phenomenon require further validation in animal studies. Nevertheless, as several studies have reported that hemodialysis and peritoneal dialysis are effective ways of lowering blood glutamate levels (40, 41), these might serve as a potential and effective measure for reducing blood glutamate levels to prevent or treat ALI after stroke.
This study has several limitations. First, this was a single-center observational study with a relatively small sample size. Although the interference of several known factors was controlled for statistically based on logistic regression, such as age, sex, BMI, OTT, current smoking status, MSG dietary preference, medical history, treatment, and inflammatory markers, which have been previously shown to be a risk factor for stroke (29), a potential modulating factor for blood glutamate levels (10), or disease progression (42), it is possible that hidden confounding factors exist, and our results should be interpreted carefully. Therefore, additional larger prospective studies would strengthen our conclusions. Moreover, patients were recruited over a relatively long period of time, which may have introduced a diagnostic bias for ALI based on the individual experiences of different clinicians. Finally, on the first day, only one blood sample but multiple severity scores were often obtained; therefore, we analyzed the relationship between these variables only at admission. Besides, in this study, we found acute treatment could obviously alleviate the severity of brain injury (especially in patients with AIS), which indicated the possible effects of acute treatment in the levels of blood glutamate and the occurrence of ALI. However, the analysis of additional blood samples taken at different time points through one-to-one correlation analyses and a strict randomized control trial in a future study was needed to test and confirm these effects.
Conclusion
This study further confirms the results in our previous animal studies and demonstrates that blood glutamate levels are closely related to the incidence of ALI in three subtypes of stroke patients. Moreover, our findings suggest that high blood glutamate levels are a potential effective predictor for ALI and a risk factor for the development of ALI and poor prognosis after stroke.
Ethics Statement
This study was performed in accordance with the recommendations of international ethical guidelines for biomedical research involving human subjects, and the ethics committee of the Research Institute of Surgery and Daping Hospital approved this study (No. 2015-12). All participants (or legal guardians) in this study provided written informed consent in accordance with the Declaration of Helsinki and its later amendments.
Author Contributions
WB, WL, Y-LN, and Y-GZ designed the research and drafted the manuscript. PL and YZ analyzed the data. WB, NY, and Y-LJ performed the experiments. Z-PL, D-PJ, YW, and MZ helped perform the experiments. Y-GZ revised the manuscript and contributed reagents, materials, and analysis tools. All the authors read and approved the final manuscript.
Conflict of Interest Statement
The authors declare that the research was conducted in the absence of any commercial or financial relationships that could be construed as a potential conflict of interest.
Acknowledgments
The authors thank the doctors, nurses, patients, and control subjects from the Departments of Neurology, the ICU, Respiratory Medicine and the Health Examination Center of their institution for their enthusiastic cooperation. They also thank all the statisticians and laboratory technicians.
Funding
This study was partially supported by the National Natural Science Foundation of China (31171022).
Supplementary Material
The Supplementary Material for this article can be found online at http://www.frontiersin.org/articles/10.3389/fneur.2017.00755/full#supplementary-material.
Abbreviations
AIS, acute ischemic stroke; ALI, acute lung injury; AUC-ROCs, areas under receiver-operating characteristic curves; BMI, body mass index; GIH, gastrointestinal hemorrhage; ICH, intracerebral hemorrhage; IH, intracranial hypertension; MSG, monosodium glutamate; NIHSS, National Institutes of Health Stroke Scale; OTT, symptom onset to treatment time; PEEP, positive end-expiratory pressure; SAH, spontaneous subarachnoid hemorrhage; TIA, transient ischemic attack; WFNS, World Federation of Neurosurgical Surgeons.
References
1. Rincon F, Ghosh S, Dey S, Maltenfort M, Vibbert M, Urtecho J, et al. Impact of acute lung injury and acute respiratory distress syndrome after traumatic brain injury in the United States. Neurosurgery (2012) 71(4):795–803. doi:10.1227/NEU.0b013e3182672ae5
2. Contant CF, Valadka AB, Gopinath SP, Hannay HJ, Robertson CS. Adult respiratory distress syndrome: a complication of induced hypertension after severe head injury. J Neurosurg (2001) 95(4):560–8. doi:10.3171/jns.2001.95.4.0560
3. Lou M, Chen X, Wang K, Xue Y, Cui D, Xue F. Increased intracranial pressure is associated with the development of acute lung injury following severe traumatic brain injury. Clin Neurol Neurosurg (2013) 115(7):904–8. doi:10.1016/j.clineuro.2012.09.001
4. Holland MC, Mackersie RC, Morabito D, Campbell AR, Kivett VA, Patel R, et al. The development of acute lung injury is associated with worse neurologic outcome in patients with severe traumatic brain injury. J Trauma (2003) 55(1):106–11. doi:10.1097/01.TA.0000071620.27375.BE
5. Mascia L. Acute lung injury in patients with severe brain injury: a double hit model. Neurocrit Care (2009) 11(3):417–26. doi:10.1007/s12028-009-9242-8
7. Chamoun R, Suki D, Gopinath SP, Goodman JC, Robertson C. Role of extracellular glutamate measured by cerebral microdialysis in severe traumatic brain injury. J Neurosurg (2010) 113(3):564–70. doi:10.3171/2009.12.JNS09689
8. Chamorro A, Dirnagl U, Urra X, Planas AM. Neuroprotection in acute stroke: targeting excitotoxicity, oxidative and nitrosative stress, and inflammation. Lancet Neurol (2016) 15(8):869–81. doi:10.1016/S1474-4422(16)00114-9
9. Brosnan JT. Glutamate, at the interface between amino acid and carbohydrate metabolism. J Nutr (2000) 130(4S Suppl):988S–90S.
10. Zlotnik A, Ohayon S, Gruenbaum BF, Gruenbaum SE, Mohar B, Boyko M, et al. Determination of factors affecting glutamate concentrations in the whole blood of healthy human volunteers. J Neurosurg Anesthesiol (2011) 23(1):45–9. doi:10.1097/ANA.0b013e3181f82a8f
11. Marrs TC, Salmona M, Garattini S, Burston D, Matthews DM. The absorption by human volunteers of glutamic acid from monosodium glutamate and from a partial enzymic hydrolysate of casein. Toxicology (1978) 11(2):101–7. doi:10.1016/S0300-483X(78)90739-4
12. Aliprandi A, Longoni M, Stanzani L, Tremolizzo L, Vaccaro M, Begni B, et al. Increased plasma glutamate in stroke patients might be linked to altered platelet release and uptake. J Cereb Blood Flow Metab (2005) 25(4):513–9. doi:10.1038/sj.jcbfm.9600039
13. Campos F, Sobrino T, Ramos-Cabrer P, Castellanos M, Blanco M, Rodriguez-Yanez M, et al. High blood glutamate oxaloacetate transaminase levels are associated with good functional outcome in acute ischemic stroke. J Cereb Blood Flow Metab (2011) 31(6):1387–93. doi:10.1038/jcbfm.2011.4
14. Dai SS, Wang H, Yang N, An JH, Li W, Ning YL, et al. Plasma glutamate-modulated interaction of A2AR and mGluR5 on BMDCs aggravates traumatic brain injury-induced acute lung injury. J Exp Med (2013) 210(4):839–51. doi:10.1084/jem.20122196
15. Muchada M, Rubiera M, Rodriguez-Luna D, Pagola J, Flores A, Kallas J, et al. Baseline National Institutes of Health stroke scale-adjusted time window for intravenous tissue-type plasminogen activator in acute ischemic stroke. Stroke (2014) 45(4):1059–63. doi:10.1161/STROKEAHA.113.004307
16. Oda S, Shimoda M, Sato O. Early aneurysm surgery and dehydration therapy in patients with severe subarachnoid haemorrhage without ICH. Acta Neurochir (Wien) (1996) 138(9):1050–6. doi:10.1007/BF01412307
17. Rosen DS, Macdonald RL. Subarachnoid hemorrhage grading scales: a systematic review. Neurocrit Care (2005) 2(2):110–8. doi:10.1385/NCC:2:2:110
18. Hemphill JC III, Bonovich DC, Besmertis L, Manley GT, Johnston SC. The ICH score: a simple, reliable grading scale for intracerebral hemorrhage. Stroke (2001) 32(4):891–7. doi:10.1161/01.STR.32.4.891
19. Force ADT, Ranieri VM, Rubenfeld GD, Thompson BT, Ferguson ND, Caldwell E, et al. Acute respiratory distress syndrome: the Berlin Definition. JAMA (2012) 307(23):2526–33. doi:10.1001/jama.2012.5669
20. Ferguson ND, Fan E, Camporota L, Antonelli M, Anzueto A, Beale R, et al. The Berlin Definition of ARDS: an expanded rationale, justification, and supplementary material. Intensive Care Med (2012) 38(10):1573–82. doi:10.1007/s00134-012-2682-1
21. Mascia L, Zavala E, Bosma K, Pasero D, Decaroli D, Andrews P, et al. High tidal volume is associated with the development of acute lung injury after severe brain injury: an international observational study. Crit Care Med (2007) 35(8):1815–20. doi:10.1097/01.CCM.0000275269.77467.DF
22. Sahetya SK, Goligher EC, Brower RG. Fifty years of research in ARDS. Setting positive end-expiratory pressure in the acute respiratory distress syndrome. Am J Respir Crit Care Med (2017) 195(11):1429–38. doi:10.1164/rccm.201610-2035CI
23. Grasso S, Stripoli T, De Michele M, Bruno F, Moschetta M, Angelelli G, et al. ARDSnet ventilatory protocol and alveolar hyperinflation: role of positive end-expiratory pressure. Am J Respir Crit Care Med (2007) 176(8):761–7. doi:10.1164/rccm.200702-193OC
24. Dai SS, Zhou YG, Li W, An JH, Li P, Yang N, et al. Local glutamate level dictates adenosine A2A receptor regulation of neuroinflammation and traumatic brain injury. J Neurosci (2010) 30(16):5802–10. doi:10.1523/JNEUROSCI.0268-10.2010
25. Wahlgren N, Ahmed N, Davalos A, Ford GA, Grond M, Hacke W, et al. Thrombolysis with alteplase for acute ischaemic stroke in the Safe Implementation of Thrombolysis in Stroke-Monitoring Study (SITS-MOST): an observational study. Lancet (2007) 369(9558):275–82. doi:10.1016/S0140-6736(07)60149-4
26. Chow SC, Wang H, Shao J. Sample Size Calculations in Clinical Research. 2nd ed. Boca Raton, FL: Taylor & Francis (2007).
27. Dupont WD, Plummer WD Jr. Power and sample size calculations for studies involving linear regression. Control Clin Trials (1998) 19(6):589–601. doi:10.1016/S0197-2456(98)00037-3
28. DeLong ER, DeLong DM, Clarke-Pearson DL. Comparing the areas under two or more correlated receiver operating characteristic curves: a nonparametric approach. Biometrics (1988) 44(3):837–45. doi:10.2307/2531595
29. Boehme AK, Esenwa C, Elkind MS. Stroke risk factors, genetics, and prevention. Circ Res (2017) 120(3):472–95. doi:10.1161/CIRCRESAHA.116.308398
30. Motyer R, Kok HK, Asadi H, O’Hare A, Brennan P, Power S, et al. Outcomes of endovascular treatment for acute large-vessel ischaemic stroke more than 6 h after symptom onset. J Intern Med (2017) 282(6):537–45. doi:10.1111/joim.12680
31. Wouters A, Lemmens R, Christensen S, Wilms G, Dupont P, Mlynash M, et al. Magnetic resonance imaging-based endovascular versus medical stroke treatment for symptom onset up to 12 h. Int J Stroke (2016) 11(1):127–33. doi:10.1177/1747493015607503
32. Young NH, Andrews PJ. High-frequency oscillation as a rescue strategy for brain-injured adult patients with acute lung injury and acute respiratory distress syndrome. Neurocrit Care (2011) 15(3):623–33. doi:10.1007/s12028-011-9550-7
33. Schaefer PW, Pulli B, Copen WA, Hirsch JA, Leslie-Mazwi T, Schwamm LH, et al. Combining MRI with NIHSS thresholds to predict outcome in acute ischemic stroke: value for patient selection. AJNR Am J Neuroradiol (2015) 36(2):259–64. doi:10.3174/ajnr.A4103
34. Aggarwal A, Dhandapani S, Praneeth K, Sodhi HB, Pal SS, Gaudihalli S, et al. Comparative evaluation of H&H and WFNS grading scales with modified H&H (sans systemic disease): a study on 1000 patients with subarachnoid hemorrhage. Neurosurg Rev (2017) 41(1):241–7. doi:10.1007/s10143-017-0843-y
35. Meyer DM, Begtrup K, Grotta JC; Recombinant Activated Factor VII Intracerebral Hemorrhage Trial Investigators. Is the ICH score a valid predictor of mortality in intracerebral hemorrhage? J Am Assoc Nurse Pract (2015) 27(7):351–5. doi:10.1002/2327-6924.12198
36. Wang H, Yan FL, Cunningham M, Deng QW, Zuo L, Xing FL, et al. Potential specific immunological indicators for stroke associated infection are partly modulated by sympathetic pathway activation. Oncotarget (2016) 7(32):52404–15. doi:10.18632/oncotarget.10497
37. Wang YY, Lin SY, Chuang YH, Chen CJ, Tung KC, Sheu WH. Adipose proinflammatory cytokine expression through sympathetic system is associated with hyperglycemia and insulin resistance in a rat ischemic stroke model. Am J Physiol Endocrinol Metab (2011) 300(1):E155–63. doi:10.1152/ajpendo.00301.2010
38. Collard CD, Park KA, Montalto MC, Alapati S, Buras JA, Stahl GL, et al. Neutrophil-derived glutamate regulates vascular endothelial barrier function. J Biol Chem (2002) 277(17):14801–11. doi:10.1074/jbc.M110557200
39. Morrell CN, Sun H, Ikeda M, Beique JC, Swaim AM, Mason E, et al. Glutamate mediates platelet activation through the AMPA receptor. J Exp Med (2008) 205(3):575–84. doi:10.1084/jem.20071474
40. Rogachev B, Ohayon S, Saad A, Vorobiovsky V, Gruenbaum BF, Leibowitz A, et al. The effects of hemodialysis on blood glutamate levels in chronic renal failure: implementation for neuroprotection. J Crit Care (2012) 27(6):743.e1–7. doi:10.1016/j.jcrc.2012.07.002
41. Rogachev B, Tsesis S, Gruenbaum BF, Gruenbaum SE, Boyko M, Klein M, et al. The effects of peritoneal dialysis on blood glutamate levels: implementation for neuroprotection. J Neurosurg Anesthesiol (2013) 25(3):262–6. doi:10.1097/ANA.0b013e318283f86a
Keywords: stroke, acute lung injury, blood glutamate, predictor, prognosis
Citation: Bai W, Li W, Ning Y-L, Li P, Zhao Y, Yang N, Jiang Y-L, Liang Z-P, Jiang D-P, Wang Y, Zhang M and Zhou Y-G (2018) Blood Glutamate Levels Are Closely Related to Acute Lung Injury and Prognosis after Stroke. Front. Neurol. 8:755. doi: 10.3389/fneur.2017.00755
Received: 13 October 2017; Accepted: 29 December 2017;
Published: 19 January 2018
Edited by:
Laszlo Csiba, University of Debrecen, HungaryReviewed by:
Adam Denes, University of Manchester, United KingdomSilvia Koton, Tel Aviv University, Israel
Copyright: © 2018 Bai, Li, Ning, Li, Zhao, Yang, Jiang, Liang, Jiang, Wang, Zhang and Zhou. This is an open-access article distributed under the terms of the Creative Commons Attribution License (CC BY). The use, distribution or reproduction in other forums is permitted, provided the original author(s) or licensor are credited and that the original publication in this journal is cited, in accordance with accepted academic practice. No use, distribution or reproduction is permitted which does not comply with these terms.
*Correspondence: Meng Zhang, zhangmeng861@gmail.com;
Yuan-Guo Zhou, yuanguo.zhou@gmail.com, ygzhou@tmmu.edu.cn
†These authors have contributed equally to this work.