- 1Department of Medicine, University of Montreal, Montreal, Quebec, Canada
- 2Programa de Postgrado en Fisiopatología Médica, Universidade Estadual de Campinas (UNICAMP), Campinas, São Paulo, Brazil
The vegetative state (VS), also known as “unresponsive wakefulness syndrome,” is considered one of the most devastating outcomes of acquired brain injury. While diagnosis of this condition is generally well-defined clinically, patients often appear to be awake despite an absence of behavioral signs of awareness, which to the family can be confusing, leading them to believe the loved one is aware of their surroundings. This inequality of agreement can be very distressing. Currently, no cure for the VS is available; as a result, patients may remain in this condition for the rest of their lives, which in some cases amount to decades. Recent advances in stem cell approaches for the treatment of other neurological conditions may now provide an opportunity to intervene in this syndrome. This mini review will address the development of VS, its diagnosis, affected cerebral structures, and the underlying basis of how stem cells can offer therapeutic promise that would take advantage of the often long-term features associated with this maladie to effect a repair of the severely damaged circuitry. In addition, current limitations of this treatment strategy, including a lack of animal models, few long-term clinical studies that might identify benefits of stem cell treatment, and the potential for development of tumors are considered.
Introduction
The diagnosis of “vegetative state” (VS) (1) assigned to patients following a severe injury to the brain is a devastating one. Originally, this condition was meant to indicate a complete lack of cortical functioning. However, a number of studies (2, 3) suggest that some VS patients possess at least elements of intact cognitive functions, e.g., pitch discrimination, or recognition of nonsensical sentence endings (3–6), and that following brain damage, some form of consciousness persists (7). Recently, VS has been termed an “unresponsive wakefulness syndrome” (8), in which patients may appear to be awake yet still not show behavioral signs of awareness. Indeed, some of these patients may start to show non-reflexive behavior, albeit inconsistently, e.g., gaze following, orienting responses, or command following. Such patients are then diagnosed as in a minimally conscious state (9). Correctly diagnosing these patients can be difficult, reflected in high rates of misdiagnoses (10).
In many countries, including the United States and Great Britain, the current prevalence of VS is unknown (11). However, in 1994, it was determined that as many as 10,000–25,000 U.S. adults and 4,000–10,000 children were diagnosed as being “awake but not aware” (12, 13). A recent systematic review of prevalence studies on VS revealed a wide variation in outcome of 0.2–6.1 patients per 100,000 members of the general population (14). In a nationwide study in the Netherlands, a prevalence of 0.1–0.2 hospitalized and institutionalized VS/UWS patients per 100,000 members of the general population was indicated (15).
Persistent VS has been defined as the condition remaining 1 month after acute traumatic or non-traumatic brain injury (TBI) and it implies reversibility (12). However, VS which is 3 months after non-TBI or 12 months after TBI to all intents and purposes is regarded as a permanent VS, and it reflects irreversible injury of impaired consciousness. Currently, the official definition of permanent VS is based on the following seven diagnostic criteria: (1) no evidence of awareness of self or environment and an inability to interact with others; (2) no evidence of sustained, reproducible, purposeful, or voluntary behavioral responses to visual, auditory, tactile, or noxious stimuli; (3) no evidence of language comprehension or expression; (4) intermittent wakefulness manifested by the presence of sleep–wake cycles; (5) sufficiently preserved hypothalamic and brain stem autonomic functions to permit survival with medical and nursing care; (6) bowel and bladder incontinence; and (7) variably preserved cranial-nerve reflexes and spinal reflexes (12).
Development of the VS and Its Diagnosis
Typically, VS develops as a consequence of hypoxic-ischemic encephalopathy in which the entire brain is involved, with cardiac/pulmonary arrest, strangulation, head injury, or near drowning being the causative factor (Figure 1). Brain swelling secondary to TBI is also a common cause of this global ischemia. The VS is usually preceded by a coma, with about 1–14% of cases developing clinical indicators of this condition, that include the presence of decorticate posturing, ventilatory dysfunction, and extraneural trauma shortly following the insult (7). Other features suggestive of a vegetative outcome include a poor motor response, advanced age, and an abnormal pupillary reflex. On the other hand, patients in non-traumatic comas display impaired eye opening, altered motor responses, and a loss of the ability to respond adequately to commands after 2 weeks, which together are highly suggestive of VS.
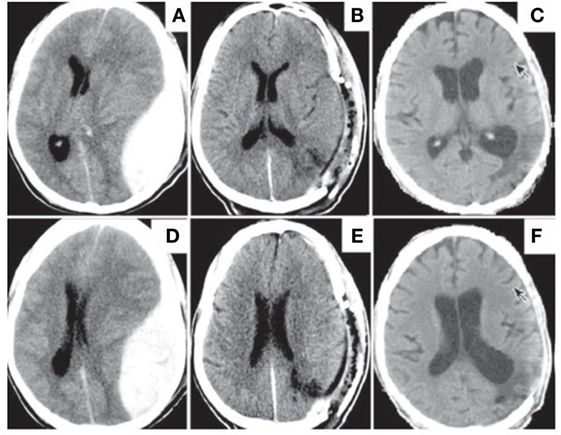
Figure 1. Traumatic head injury resulting in development of VS with subsequent bilateral cortical atrophy. Patient was admitted with extradural hematoma (A,D). Evacuation of hematoma relieved brain asymmetry (B,E). However, patient decompensated to a VS with delayed cerebral atrophy observed at 4 months post-op. Note enlarged sulci, particularly in the fronto-temporal lobes (C,F). Reproduced from Louzada et al. (65) with permission.
The diagnosis of VS is usually dependent on a number of investigations that include (i) bedside clinical evaluation, (ii) electroencephalographic (EEG) measurements, including event related potentials, a derivate of the EEG, which can be helpful in investigating cognitive processing and possibly conscious awareness in VS patients, and (iii) functional neuroimaging that can include positron emission tomography which provides information on cerebral metabolism and activation of particular brain regions, and functional magnetic resonance imaging, which can also identify brain activity in terms of increased local cerebral blood flow in response to sensory stimulation.
Cerebral Structures Associated with VS
Results of autopsy studies of patients in VS at the time of death have identified widespread neuronal death throughout the thalamus as the common finding following either anoxia or diffuse axonal injury that produces widespread disruption of white matter connections (16). The severe bilateral thalamic damage after either trauma or anoxia seen in permanent VS is not, however, invariably associated with diffuse neocortical neuronal cell death. Specific thalamic regions exhibit profound neuronal cell death as a consequence of brain trauma injuries, e.g., the central thalamic nuclei that include the intralaminar nuclei and paralaminar nuclei (17). Rostrocaudally, severe disability is reflected in the extent of neuronal cell death, in which severity of dysfunction progresses in the rostrocaudal direction, with the anterior intralaminar and surrounding areas at first exhibiting negative volume change that is linked to moderate disability, while damage to the posterior intralaminar nuclei is associated with increased disability, often seen in both VS and in minimally conscious patients (17). This large-scale neuronal loss in the thalamus is probably a consequence of severe cerebral injury mainly due to diffuse trauma, hypoxia, and other non-specific damage. Direct injury to the central thalamus leads to coma, indicating these cells are an important factor in the regulation of arousal (18). This part of the brain contains circuitry that has a key role to play in the sleep–wake cycle, involving the brain stem associated arousal system (18). It is also innervated by projections from the basal forebrain. Thus, these wiring influences can modulate the level of arousal associated with generalized alertness and variations in cognitive effort, stress, sleep deprivation, and other variables affecting the wakeful state (18, 19). In addition, studies have identified diffuse axonal injury in 70–80% of the studied brains, along with widespread damage to the white matter (20, 21).
Stem Cell-Mediated Therapeutic Intervention in Patients with VS
Endogenous NSCs
Given that patients in VS can spend months, even years in this condition, an important consideration should be whether a stem cell treatment paradigm might be beneficial under these circumstances, taking advantage of the long-term condition of these cases. In recent years, a new approach to the treatment of disease has been developing, one that holds considerable potential for large numbers of individuals in which damage to an organ is presently unrepairable with drugs, surgery, or other forms of therapy. The relatively recent discovery that in selected regions of the brain neurons can be produced during adult life has invigorated both scientists and the public, focusing interest on the possibility of manipulating the development of these endogenous neurogenic populations and utilising them for treating a variety of neurological and neurodegenerative diseases. Studies have demonstrated that neurogenesis occurs throughout life but is mainly limited to the periventricular subventricular zone (SVZ), in which neurons develop that are destined for the olfactory bulb, and the subgranular zone (SGZ) of the hippocampus, in which new neurons are established within the dentate gyrus (22, 23). Neural stem cells (NSCs) are an undifferentiated population of cells residing in the SVZ and SGZ of both the embryonic and adult mammalian brain (24–26). In the adult brain, pathological events, such as TBI (27), stroke (28), seizures (29, 30), and neuroinflammatory states (31), can stimulate neurogenesis, which can have a protective role. Evidence thus far suggests that while these cells show a positive response to the insult, the long-term beneficial effects are limited in nature. On the other hand, the response of these cells can be enhanced via exogenous means and augmentation of endogenous NSCs could therefore be a potential therapy for treating the injured brain. For example, basic fibroblast growth factor or epidermal growth factor can enhance TBI-induced cell proliferation in the hippocampus and the SVZ, and drastically improve cognitive functional recovery (32, 33). In addition, several drugs have shown the potential to enhance neurogenesis and improve cognitive function in experimental TBI. These include statins (34), progesterone (35), erythropoietin (36, 37), and the antidepressant imipramine (38). Such studies lend credence to the notion that increasing the activity of endogenous NSCs may provide an important therapeutic approach for treating patients in VS.
Stem Cell Transplantation
Due to the limited repair potential available to the damaged brain, the prospect of neural transplantation represents an important and viable choice for the treatment of injured cerebral tissue (39). In addition to providing a substrate for the regeneration of circuitry lost by the insult, transplanted cells originating from different stem cell sources may also stimulate surviving circuitry to establish new synaptic connections by way of providing trophic support. Potential sources of these cells include adult NSCs, bone marrow cells, umbilical cord cells, and induced pluripotent stem cells (iPSCs).
Adult NSCs
In humans, multipotent stem/progenitor cells have been identified and successfully isolated from various regions of adult human brain, including the hippocampus, SVZ, neocortex, and subcortical white matter from neurosurgical resection tissue (40–42). These cells could potentially be used as an autologous cell source for transplantation therapy. Thus far, very few studies have examined the behavior of adult-derived human NSCs in the injured adult CNS. Previous studies have reported that 4% of adult human NSCs survive for 4 months following transplantation in rats with hippocampal damage due to ischemia (43). However, whether these cells become properly integrated with the existing circuitry remains unclear. Another concern as to the potential use of these cells in terms of their translational value relates to the possibility of tumor development, which could have negative ramifications regarding their future use in a clinical setting. On the other hand, use of autologous transplantation as an option, involving the neurosurgical isolation of NSCs from patients with head injury cannot be disregarded and needs to be fully engaged as a viable option. However, whether these cells remain viable for a significant period of time post-surgery while retaining the capacity to improve functional outcome in patients with VS remains an unanswered question that needs to be addressed in the future.
Bone Marrow Cells
Mesenchymal bone marrow stromal cells (BMSCs) are a mixture of undifferentiated cells that includes stem and progenitor cells. Their ability for self-renewal and multipotential capability provides for applications in tissue engineering and regenerative medicine. These cells can be readily isolated from bone marrow and grown in culture without ethical and technical concerns. Several studies have reported that transplanted BMSCs accelerate neuroplasticity and facilitate neuronal regeneration and functional recovery (44–47). In addition, they exhibit an ability to enhance the proliferation of endogenous NSCs (48). Such attributes make them an attractive candidate for the treatment of VS, particularly given their low antigenicity due to reduced expression of the major histocompatibility complex (class II) antigens (49). These cells produce high levels of growth factors, cytokines, and extracellular matrix molecules that could have potential neurotrophic or neuroprotective effect in the injured brain. Indeed, studies have demonstrated that the beneficial effects of transplanted BMSCs are attributed to their neurotrophic or neuroprotective effect rather than direct cell replacement (50).
Transplantation of human BMSCs into rats following TBI led to an improvement in sensorimotor and spatial learning along with a reduction in brain injury volume and improved angiogenesis (51, 52). This improved sensorimotor function was evident even when treatment with these cells was delayed by 2 months following brain injury (53), indicative of the long-term viability of these cells and their ability to ameliorate the extent of cerebral damage following administration at later times. This suggests that treatment of cases of VS with these cells has the potential to benefit patients even if treatment is delayed following the insult. In a recent study, 45% of cases of VS treated with autologous BMSCs showed an improvement in consciousness (54). Allogenic transplantation of hematopoietic stem cells (HSCs) from bone marrow has also been promising in the treatment of X-linked adrenoleukodystrophy (ALD), a progressive disease presenting most commonly in males, in which cerebral demyelination due to improper fatty acid metabolism in early childhood can rapidly lead to a VS (55). Autologous treatment of HSCs in combination with gene therapy, involving an introduction of wild-type cDNA of the mutated gene into these cells has provided additional benefits for young children with ALD, in which arrest of cerebral demyelination was achieved (56). Altogether, these findings support the idea that BMSCs provide an important potential for treatment of the injured brain and strongly points to benefits from BMSC treatment, highlighting the notion for future use of these cells in the treatment of VS.
Umbilical Cord Blood
Several key features make umbilical cord blood an attractive prospect for treatment of VS. Cord blood provides large numbers of HSCs, mesenchymal stem cells, unrestricted somatic stem cells, and embryonic-like stem cells. These cells can be easily harvested at birth without ethical controversy, can be administered with no risk of allograft rejection, and without ethical complications (57). In a landmark study, autologous intravenous cord blood transplantation in a two and a half year old child was shown to considerably improve neurologic function over a period of 40 months following cardiac arrest and development of VS, representing the first reported case of a successful cell therapy treatment of pediatric cerebral palsy (58) (Figure 2). In an earlier report, treatment of a 16-month-old child in VS with cord blood led to lesser though significant clinical improvement over a 6-month period (59). In addition, allogenic cord blood treatment of an 8-year-old boy with ALD prevented neurological deterioration and halted disease progression (60). Notably, one study involving an 8-month-old infant in VS suggests that it may be possible to influence the movement of these cells in the brains of such patients when labeled with paramagnetic iron oxide particles and using an external magnetic field following their transplantation into the ventricular system (61). Such an approach could be useful in helping to direct transplanted stem cells toward the site of a lesion. These findings strengthen the case that it is possible to use stem cells as a viable, major therapeutic strategy for the treatment of patients in VS, one that should be engaged, not ignored.
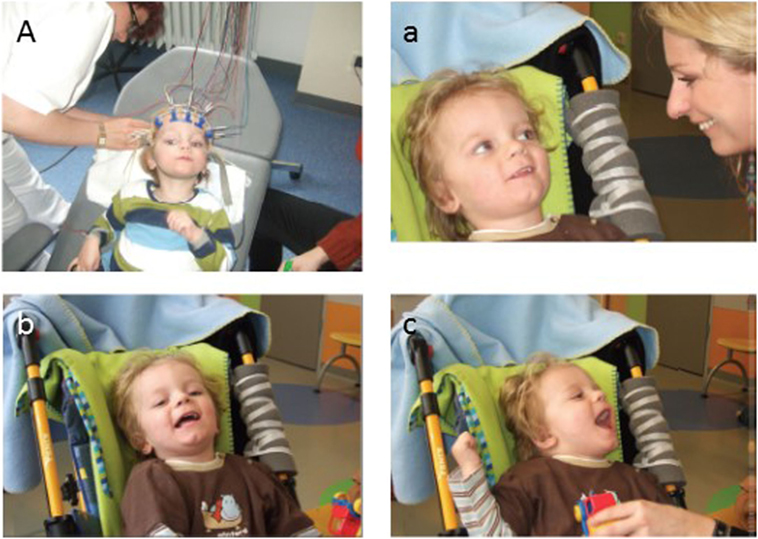
Figure 2. The patient (L.B.) before and after autologous umbilical cord blood transplantation. (A) The patient during EEG recording is in a VS 9 weeks after the insult before transplantation of cord blood cells. Note the dilated, unresponsive pupils in spite of bright light from the ceiling. (a–c) Two-month follow-up. (a) First social smiling of the patient (L.B.) toward his mother and (b,c) laughing, when played with, 2 months after transplantation of cord blood cells (i.e., 4 months and 1 week after severe brain damage caused by cardiac arrest). Reproduced from Jensen and Hamelmann (58) with permission.
Induced Pluripotent Stem Cells
The development of iPSCs (62), involving somatic cell reprograming, represents an important watershed, providing the potential for novel neural replacement strategies. The main characteristics of iPSCs are unlimited self-renewal and pluripotency (63), defining the ability to differentiate into three germ layers and multilineage cell types. Significantly, a major advantage of the use of patient-specific iPSCs is that it can be safely administered as an autologous treatment without the potential for rejection. Such properties of these reprogramed cells help provide considerable hope that VS and other disease states in which neurodegeneration is a feature might be successfully treated in the future. Indeed, in the 10 years since the seminal work of Takahashi and Yamanaka (62), significant progress has already occurred in deriving iPSCs suitable for clinical use (64).
Conclusion
For decades, the VS has been considered to represent a severe neurological condition, recovery from which was extremely unlikely, if at all. Patients might remain in this condition for years without much chance for significant recovery. However, recent advances in our understanding of the ability of stem cells to positively influence repair of the damaged brain suggests that the VS may no longer be the largely irreversible maladie of years past. Although in the absence of an established animal model, the question of how stem cell treatment might modify consequences of the VS has remained unaddressed for the most part, a few recent case studies involving the use of these cells have been reported. Their findings suggest that stem cell treatment may offer new hope to effect significant recovery in terms of the level of consciousness, and potentially overall cerebral function. To further our understanding of how this might come about, development of viable animal models of the VS represents an issue that should be pursued in the future, particularly in terms of the potential for stem cell intervention. Currently, no well established in vivo models of this condition exist, mainly due to the difficulty of keeping the animal alive for extended periods of time following an insult of this magnitude. A stronger push toward development of such experimental models is likely to pave the way toward identification, and a better understanding, of potential therapeutic interventions that may improve the overall condition of patients in VS over the long term.
Author Contributions
The author confirms being the sole contributor to this manuscript and approved it for publication.
Conflict of Interest Statement
The author declares that the research was conducted in the absence of any commercial or financial relationships that could be construed as a potential conflict of interest.
Funding
The author’s laboratory is supported by the Canadian Institutes of Health Research and Fundação de Amparo à Pesquisa do Estado de São Paulo (FAPESP) in Brazil.
References
2. Kotchoubey B, Lang S, Mezger G, Schmalohr D, Schneck M, Semmler A, et al. Information processing in severe disorders of consciousness: vegetative state and minimally conscious state. Clin Neurophysiol (2005) 116:2441–53. doi:10.1016/j.clinph.2005.03.028
3. Gantner IS, Bodart O, Laureys S, Demertzi A. Our rapidly changing understanding of acute and chronic disorders of consciousness: challenges for neurologists. Future Neurol (2013) 8:43–54. doi:10.2217/fnl.12.77
4. Harrison AH, Connolly JF. Finding a way in: a review and practical evaluation of fMRI and EEG for detection and assessment in disorders of consciousness. Neurosci Biobehav Rev (2013) 37:1403–19. doi:10.1016/j.neubiorev.2013.05.004
5. Monti MM. Cognition in the vegetative state. Annu Rev Clin Psychol (2012) 8:431–54. doi:10.1146/annurev-clinpsy-032511-143050
6. Steppacher I, Eickhoff S, Jordanov T, Kaps M, Witzke W, Kissler J. N400 predicts recovery from disorders of consciousness. Ann Neurol (2013) 73:594–602. doi:10.1002/ana.23835
7. Panksepp J, Fuchs T, Garcia VA, Lesiak A. Does any aspect of mind survive brain damage that typically leads to a persistent vegetative state? Ethical considerations. Philos Ethics Humanit Med (2007) 2:32. doi:10.1186/1747-5341-2-32
8. Laureys S, Celesia GG, Cohadon F, Lavrijsen J, Leon-Carrrion J, Sannita WG, et al. Unresponsive wakefulness syndrome: a new name for the vegetative state or apallic syndrome. BMC Med (2010) 8:68. doi:10.1186/1741-7015-8-68
9. Giacino JT, Ashwal S, Childs N, Cranford R, Jennett B, Katz DI, et al. The minimally conscious state: definition and diagnostic criteria. Neurology (2002) 58:349–53. doi:10.1212/WNL.58.3.349
10. Schnakers C, Vanhaudenhuyse A, Giacino JT, Ventura M, Boly M, Majerus S, et al. Diagnostic accuracy of the vegetative and minimally conscious state: clinical consensus versus standardized neurobehavioral assessment. BMC Neurol (2009) 9:35. doi:10.1186/1471-2377-9-35
11. Beaumont JG, Kenealy PM. Incidence and prevalence of the vegetative and minimally conscious state. Neuropsychol Rehabil (2005) 15:184–9. doi:10.1080/09602010443000489
12. Multi-society task force on PVS. Medical aspects of the persistent vegetative state. N Engl J Med (1994) 330:1572–9. doi:10.1056/NEJM199406023302206
13. Multi-society task force on PVS. Medical aspects of the persistent vegetative state. N Engl J Med (1994) 330:1499–508. doi:10.1056/NEJM199405263302107
14. Van Erp WS, Lavrijsen JC, Van de Laar FA, Vos PE, Laureys S, Koopmans RT. The vegetative state/unresponsive wakefulness syndrome: a systematic review of prevalence studies. Eur J Neurol (2014) 21:1361–8. doi:10.1111/ene.12483
15. Van Erp WS, Lavrijsen JC, Vos PE, Bor H, Laureys S, Koopmans RT. The vegetative state: prevalence, misdiagnosis, and treatment limitations. J Am Med Dir Assoc (2015) 16:.e9–85. doi:10.1016/j.jamda.2014.10.014
16. Adams JH, Graham DI, Jennett B. The neuropathology of the vegetative state after acute insult. Brain (2000) 123:1327–38. doi:10.1093/brain/123.7.1327
17. Maxwell WL, MacKinnon MA, Smith DH, McIntosh TK, Graham DI. Thalamic nuclei after human blunt head injury. J Neuropathol Exp Neurol (2006) 65:478–88. doi:10.1097/01.jnen.0000229241.28619.75
18. Schiff ND. Central thalamic contributions to arousal regulation and neurological disorders of consciousness. Ann N Y Acad Sci (2008) 1129:105–18. doi:10.1196/annals.1417.029
19. Van der Werf YD, Witter MP, Groenewegen HJ. The intralaminar and midline nuclei of the thalamus. Anatomical and functional evidence for participation in processes of arousal and awareness. Brain Res Brain Res Rev (2002) 39:107–40. doi:10.1016/S0165-0173(02)00181-9
20. Kinney HC, Samuels MA. Neuropathology of the persistent vegetative state: a review. J Neuropathol Exp Neurol (1994) 53:548–58. doi:10.1097/00005072-199411000-00002
21. Graham DI, Adams JH, Murray LS, Jennett B. Neuropathology of the vegetative state after head injury. Neuropsychol Rehabil (2005) 15:198–213. doi:10.1080/09602010443000452
22. Gage FH, Ray J, Fisher LJ. Isolation, characterization, and use of stem cells from the CNS. Annu Rev Neurosci (1995) 18:159–92. doi:10.1146/annurev.ne.18.030195.001111
23. McKay R. Stem cells in the central nervous system. Science (1997) 276:66–71. doi:10.1126/science.276.5309.66
24. Altman J. Autoradiographic and histological studies of postnatal neurogenesis. IV. Cell proliferation and migration in the anterior forebrain, with special reference to persisting neurogenesis in the olfactory bulb. J Comp Neurol (1969) 137:433–57. doi:10.1002/cne.901370404
25. Corotto FS, Henegar JA, Maruniak JA. Neurogenesis persists in the subependymal layer of the adult mouse brain. Neurosci Lett (1993) 149:111–4. doi:10.1016/0304-3940(93)90748-A
26. Morshead CM, Reynolds BA, Craig CG, McBurney MW, Staines WA, Morassutti D, et al. Neural stem cells in the adult mammalian forebrain: a relatively quiescent subpopulation of subependymal cells. Neuron (1994) 13:1071–82. doi:10.1016/0896-6273(94)90046-9
27. Patel K, Sun D. Strategies targeting endogenous neurogenic cell response to improve recovery following traumatic brain injury. Brain Res (2016) 1640:104–13. doi:10.1016/j.brainres.2016.01.055
28. Naylor M, Bowen KK, Sailor KA, Dempsey RJ, Vemuganti R. Preconditioning-induced ischemic tolerance stimulates growth factor expression and neurogenesis in adult rat hippocampus. Neurochem Int (2005) 47:565–72. doi:10.1016/j.neuint.2005.07.003
29. Parent JM, Yu TW, Leibowitz RT, Geschwind DH, Sloviter RS, Lowenstein DH. Dentate granule cell neurogenesis is increased by seizures and contributes to aberrant network reorganization in the adult rat hippocampus. J Neurosci (1997) 17:3727–38.
30. Nakagawa E, Aimi Y, Yasuhara O, Tooyama I, Shimada M, McGeer PL, et al. Enhancement of progenitor cell division in the dentate gyrus triggered by initial limbic seizures in rat models of epilepsy. Epilepsia (2000) 41:10–8. doi:10.1111/j.1528-1157.2000.tb01498.x
31. Picard-Riera N, Decker L, Delarasse C, Goude K, Nait-Oumesmar B, Liblau R, et al. Experimental autoimmune encephalomyelitis mobilizes neural progenitors from the subventricular zone to undergo oligodendrogenesis in adult mice. Proc Natl Acad Sci U S A (2002) 99:13211–6. doi:10.1073/pnas.192314199
32. Sun D, Bullock MR, McGinn MJ, Zhou Z, Altememi N, Hagood S, et al. Basic fibroblast growth factor-enhanced neurogenesis contributes to cognitive recovery in rats following traumatic brain injury. Exp Neurol (2009) 216:56–65. doi:10.1016/j.expneurol.2008.11.011
33. Sun D, Bullock MR, Altememi N, Zhou Z, Hagood S, Rolfe A, et al. The effect of epidermal growth factor in the injured brain after trauma in rats. J Neurotrauma (2010) 27:923–38. doi:10.1089/neu.2009.1209
34. Lu D, Qu C, Goussev A, Jiang H, Lu C, Schallert T, et al. Statins increase neurogenesis in the dentate gyrus, reduce delayed neuronal death in the hippocampal CA3 region, and improve spatial learning in rat after traumatic brain injury. J Neurotrauma (2007) 24:1132–46. doi:10.1089/neu.2007.0288
35. Barha CK, Ishrat T, Epp JR, Galea LA, Stein DG. Progesterone treatment normalizes the levels of cell proliferation and cell death in the dentate gyrus of the hippocampus after traumatic brain injury. Exp Neurol (2011) 231:72–81. doi:10.1016/j.expneurol.2011.05.016
36. Lu D, Mahmood A, Qu C, Goussev A, Schallert T, Chopp M. Erythropoietin enhances neurogenesis and restores spatial memory in rats after traumatic brain injury. J Neurotrauma (2005) 22:1011–7. doi:10.1089/neu.2005.22.1011
37. Xiong Y, Mahmood A, Meng Y, Zhang Y, Qu C, Schallert T, et al. Delayed administration of erythropoietin reducing hippocampal cell loss, enhancing angiogenesis and neurogenesis, and improving functional outcome following traumatic brain injury in rats: comparison of treatment with single and triple dose. J Neurosurg (2010) 113:598–608. doi:10.3171/2009.9.JNS09844
38. Han X, Tong J, Zhang J, Farahvar A, Wang E, Yang J, et al. Imipramine treatment improves cognitive outcome associated with enhanced hippocampal neurogenesis after traumatic brain injury in mice. J Neurotrauma (2011) 28:995–1007. doi:10.1089/neu.2010.1563
39. Rolfe A, Sun D. Chapter 42 stem cell therapy in brain trauma: implications for repair and regeneration of injured brain in experimental TBI models. In: Kobeissy FH, editor. Brain Neurotrauma: Molecular, Neuropsychological, and Rehabilitation Aspects. Boca Raton, FL: CRC Press/Taylor & Francis (2015).
40. Kukekov VG, Laywell ED, Suslov O, Davies K, Scheffler B, Thomas LB, et al. Multipotent stem/progenitor cells with similar properties arise from two neurogenic regions of adult human brain. Exp Neurol (1999) 156:333–44. doi:10.1006/exnr.1999.7028
41. Brunet JF, Pellerin L, Arsenijevic Y, Magistretti P, Villemure JG. A novel method for in vitro production of human glial-like cells from neurosurgical resection tissue. Lab Invest (2002) 82:809–12. doi:10.1097/01.LAB.0000017166.26718.BB
42. Richardson RM, Holloway KL, Bullock MR, Broaddus WC, Fillmore HL. Isolation of neuronal progenitor cells from the adult human neocortex. Acta Neurochir (Wien) (2006) 148:773–7. doi:10.1007/s00701-006-0778-5
43. Olstorn H, Moe MC, Roste GK, Bueters T, Langmoen IA. Transplantation of stem cells from the adult human brain to the adult rat brain. Neurosurgery (2007) 60:1089–98. doi:10.1227/01.NEU.0000255461.91892.0D
44. Pavlichenko N, Sokolova I, Vijde S, Shvedova E, Alexandrov G, Krouglyakov P, et al. Mesenchymal stem cells transplantation could be beneficial for treatment of experimental ischemic stroke in rats. Brain Res (2008) 1233:203–13. doi:10.1016/j.brainres.2008.06.123
45. Keimpema E, Fokkens MR, Nagy Z, Agoston V, Luiten PG, Nyakas C, et al. Early transient presence of implanted bone marrow stem cells reduces lesion size after cerebral ischaemia in adult rats. Neuropathol Appl Neurobiol (2009) 35:89–102. doi:10.1111/j.1365-2990.2008.00961.x
46. Andrews EM, Tsai SY, Johnson SC, Farrer JR, Wagner JP, Kopen GC, et al. Human adult bone marrow-derived somatic cell therapy results in functional recovery and axonal plasticity following stroke in the rat. Exp Neurol (2008) 211:588–92. doi:10.1016/j.expneurol.2008.02.027
47. Jang DK, Park SI, Han YM, Jang KS, Park MS, Chung YA, et al. Motor-evoked potential confirmation of functional improvement by transplanted bone marrow mesenchymal stem cell in the ischemic rat brain. J Biomed Biotechnol (2011) 2011:238409. doi:10.1155/2011/238409
48. Yoo SW, Kim SS, Lee SY, Lee HS, Kim HS, Lee YD, et al. Mesenchymal stem cells promote proliferation of endogenous neural stem cells and survival of newborn cells in a rat stroke model. Exp Mol Med (2008) 40:387–97. doi:10.3858/emm.2008.40.4.387
49. Le BK, Ringden O. Immunobiology of human mesenchymal stem cells and future use in hematopoietic stem cell transplantation. Biol Blood Marrow Transplant (2005) 11:321–34. doi:10.1016/j.bbmt.2005.01.005
50. Li Y, Chopp M. Marrow stromal cell transplantation in stroke and traumatic brain injury. Neurosci Lett (2009) 456:120–3. doi:10.1016/j.neulet.2008.03.096
51. Lu D, Mahmood A, Qu C, Hong X, Kaplan D, Chopp M. Collagen scaffolds populated with human marrow stromal cells reduce lesion volume and improve functional outcome after traumatic brain injury. Neurosurgery (2007) 61:596–602. doi:10.1227/01.NEU.0000290908.38438.B2
52. Xiong Y, Qu C, Mahmood A, Liu Z, Ning R, Li Y, et al. Delayed transplantation of human marrow stromal cell-seeded scaffolds increases transcallosal neural fiber length, angiogenesis, and hippocampal neuronal survival and improves functional outcome after traumatic brain injury in rats. Brain Res (2009) 1263:183–91. doi:10.1016/j.brainres.2009.01.032
53. Bonilla C, Zurita M, Otero L, Aguayo C, Vaquero J. Delayed intralesional transplantation of bone marrow stromal cells increases endogenous neurogenesis and promotes functional recovery after severe traumatic brain injury. Brain Inj (2009) 23:760–9. doi:10.1080/02699050903133970
54. Tian C, Wang X, Wang X, Wang L, Wang X, Wu S, et al. Autologous bone marrow mesenchymal stem cell therapy in the subacute stage of traumatic brain injury by lumbar puncture. Exp Clin Transplant (2013) 11:176–81. doi:10.6002/ect.2012.0053
55. Borker A, Yu LC. Unrelated allogeneic bone marrow transplant in adrenoleukodystrophy using CD34+ stem cell selection. Metab Brain Dis (2002) 17:139–42. doi:10.1023/A:1019969820308
56. Cartier N, Hacein-Bey-Abina S, Bartholomae CC, Bougnères P, Schmidt M, Kalle CV, et al. Lentiviral hematopoietic cell gene therapy for X-linked adrenoleukodystrophy. Methods Enzymol (2012) 507:187–98. doi:10.1016/B978-0-12-386509-0.00010-7
57. Romanov YA, Svintsitskaya VA, Smirnov VN. Searching for alternative sources of postnatal human mesenchymal stem cells: candidate MSC-like cells from umbilical cord. Stem Cells (2003) 21:105–10. doi:10.1634/stemcells.21-1-105
58. Jensen A, Hamelmann E. First autologous cell therapy of cerebral palsy caused by hypoxic-ischemic brain damage in a child after cardiac arrest-individual treatment with cord blood. Case Rep Transplant (2013) 2013:951827. doi:10.1155/2013/951827
59. Jozwiak S, Habich A, Kotulska K, Sarnowska A, Kropiwnicki T, Janowski M, et al. Intracerebroventricular transplantation of cord blood-derived neural progenitors in a child with severe global brain ischemic injury. Cell Med (2010) 1:71–80. doi:10.3727/215517910X536618
60. Awaya T, Kato T, Niwa A, Hiramatsu H, Umeda K, Watanabe K, et al. Successful cord blood transplantation using a reduced-intensity conditioning regimen for advanced childhood-onset cerebral adrenoleukodystrophy. Pediatr Transplant (2011) 15:E116–20. doi:10.1111/j.1399-3046.2009.01188.x
61. Janowski M, Walczak P, Kropiwnicki T, Jurkiewicz E, Domanska-Janik K, Bulte JW, et al. Long-term MRI cell tracking after intraventricular delivery in a patient with global cerebral ischemia and prospects for magnetic navigation of stem cells within the CSF. PLoS One (2014) 9(2):e97631. doi:10.1371/journal.pone.0097631
62. Takahashi K, Yamanaka S. Induction of pluripotent stem cells from mouse embryonic and adult fibroblast cultures by defined factors. Cell (2006) 126:663–76. doi:10.1016/j.cell.2006.07.024
63. Singh VK, Kalsan M, Kumar N, Saini A, Chandra R. Induced pluripotent stem cells: applications in regenerative medicine, disease modeling, and drug discovery. Front Cell Dev Biol (2015) 3:2. doi:10.3389/fcell.2015.00002
64. Cefalo MG, Carai A, Miele E, Po A, Ferretti E, Mastronuzzi A, et al. Human iPSC for therapeutic approaches to the nervous system: present and future applications. Stem Cells Int (2016) 2016:4869071. doi:10.1155/2016/4869071
Keywords: consciousness, neuroimaging, traumatic brain injury, hypoxia-ischemia, unresponsive wakefulness syndrome, stem cell, transplantation, induced pluripotent stem cell
Citation: Hazell AS (2016) The Vegetative State and Stem Cells: Therapeutic Considerations. Front. Neurol. 7:118. doi: 10.3389/fneur.2016.00118
Received: 25 February 2016; Accepted: 07 July 2016;
Published: 23 August 2016
Edited by:
Alexandre Leite Rodrigues Oliveira, University of Campinas, BrazilReviewed by:
Namas Chandra, New Jersey Institute of Technology, USASharon Leah Juliano, Uniformed Services University of the Health Sciences, USA
Copyright: © 2016 Hazell. This is an open-access article distributed under the terms of the Creative Commons Attribution License (CC BY). The use, distribution or reproduction in other forums is permitted, provided the original author(s) or licensor are credited and that the original publication in this journal is cited, in accordance with accepted academic practice. No use, distribution or reproduction is permitted which does not comply with these terms.
*Correspondence: Alan S. Hazell, YWxhbi5zdGV3YXJ0LmhhemVsbEB1bW9udHJlYWwuY2E=