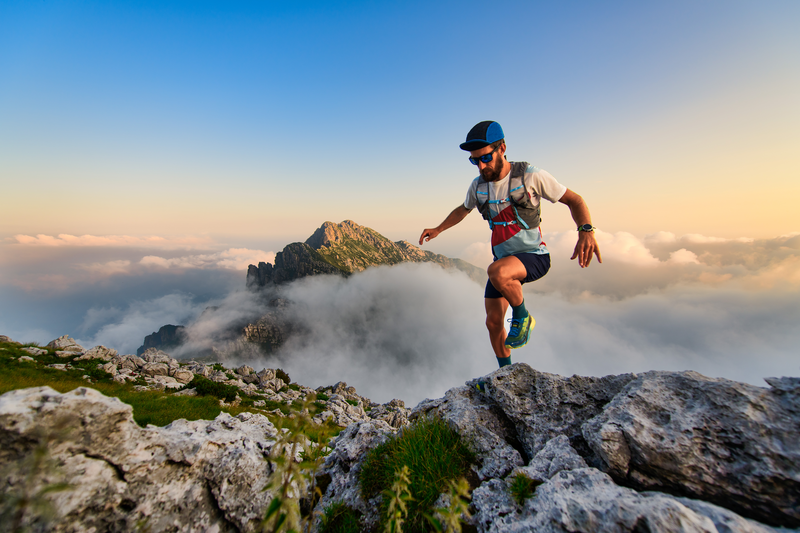
94% of researchers rate our articles as excellent or good
Learn more about the work of our research integrity team to safeguard the quality of each article we publish.
Find out more
ORIGINAL RESEARCH article
Front. Nephrol. , 06 November 2024
Sec. Critical Care Nephrology
Volume 4 - 2024 | https://doi.org/10.3389/fneph.2024.1398386
This article is part of the Research Topic Novel Diagnostic and Prognostic Methods in Acute Kidney Injury Among Patients in Intensive Care Unit View all 5 articles
Background: Fibrinogen plays a pivotal role in the inflammatory cascade and is intricately linked to the pathogenesis of sepsis. Nevertheless, its significance as a prognostic marker for sepsis-associated acute kidney injury (SA-AKI) remains uncertain. This study aimed to investigate the association between fibrinogen levels and 28-day mortality with sepsis-associated acute kidney injury.
Method: The fibrinogen levels of patients admitted to the intensive care unit of Beth Israel Deaconess Medical Center between 2008 and 2019 were retrospectively assessed, and those diagnosed with SA-AKI were divided into low, middle and high fibrinogen level groups according to tertiles. Multivariate Cox proportional hazards model was used to assess the 28-day mortality risk of the SA-AKI patients.
Results: A total of 3,479 patients with SA-AKI were included in the study. Fibrinogen demonstrated an independent association with 28-day mortality, yielding a hazard ratio (HR) of 0.961 (95% confidence interval [CI]: 0.923-0.999, P = 0.0471). Notably, a non-linear relationship between fibrinogen levels and 28-day mortality was observed, with the threshold observed at approximately 1.6 g/l. The effect sizes and corresponding CIs below and above this threshold were 0.509 (0.367, 0.707) and 1.011 (0.961, 1.064), respectively. Specifically, the risk of mortality among SA-AKI patients decreased by 49.1% for every 1 g/l increment in fibrinogen, provided that fibrinogen levels were less than 1.6 g/l.
Conclusion: In patients with SA-AKI, a non-linear relationship was identified between fibrinogen levels and 28-day mortality. Particularly, when their fibrinogen levels were less than 1.6 g/l, a concomitant decrease in 28-day mortality was observed as fibrinogen levels increased.
Acute kidney injury is defined as the abrupt deterioration of kidney function, characterized by elevated levels of creatinine and decreased urine output (1, 2). In the occurrence and progression of AKI, inflammation plays a crucial role (3, 4). Disruption of the inflammation-immune response is at the core of sepsis development (5), which can severely impair kidney function. The kidney is an important target organ in sepsis. If sepsis patients are not promptly treated, they are susceptible to deteriorating into sepsis-associated acute kidney injury within seven days (6, 7), accounting for approximately 45-70% of all critically ill patients (8–10). Furthermore, SA-AKI is closely associated with an increased risk of in-hospital mortality. Bagshaw et al. (11) reported that the in-hospital mortality rate of SA-AKI patients was 1.48 times higher than that of non-septic AKI patients, with a significantly longer average length of hospital stay for SA-AKI (37 days vs 21 days). Another study indicated that SA-AKI patients had a significantly higher in-hospital mortality rate compared to non-septic AKI patients (64.81% vs 22.22%) (12). Although many studies have revealed significant differences between SA-AKI and non-septic AKI, the prognostic indicators for SA-AKI still remain uncertain.
Fibrinogen, a precursor synthesized in the liver, plays a dual role as a clotting factor and an acute-phase inflammatory marker. It occurs actively during the period of sepsis and is associated with inflammatory response, consumptive coagulopathy, and microthrombus formation (13). Recent research reports a correlation between fibrinogen and prognosis in various clinical contexts, including tumor outcomes, cardiovascular surgery, and contrast-induced AKI (14–17). Moreover, propensity score matching studies have demonstrated a significant association between elevated fibrinogen levels and reduced risk of death in sepsis patients (HR 0.66, P < 0.001 and HR 0.73, P < 0.001) (18). Previous research shows that fibrinogen has different implications for AKI in different diseases. For instance, in liver transplant recipients, low levels of fibrinogen serve as a risk factor for the development of acute kidney injury (AKI) (19). Conversely, in patients undergoing heart valve replacement surgery, high fibrinogen levels are considered a risk factor for postoperative AKI (15). Additionally, there is a controversial relationship between fibrinogen and prognosis in sepsis as depicted by different literature sources. Studies based on Japanese databases demonstrate a J-shaped relationship, while the Mimic database shows a linear correlation (18, 20). In severe AKI cases, a linear relationship is observed (21). However, the significance of fibrinogen in the context of SA-AKI remains largely unknown due to the absence of relevant data. Therefore, the main objective of our study is to evaluate the predictive value of fibrinogen for the 28-day mortality in SA-AKI patients.
This is a retrospective observational cohort study based on data retrieved from the Medical Information Mart for Intensive Care-IV (MIMIC-IV) 2.0 database, which is managed by the Beth Israel Deaconess Medical Center. The study included over 50,000 first-time intensive care unit (ICU) admissions for sepsis between 2008 and 2019 (22). Author Chen successfully completed the training on “Protection of Human Subjects” and obtained authorization to access this database (certification number 51474639). Patient identifiers and data information were de-identified. The institutional review boards of Massachusetts Institute of Technology and Beth Israel Deaconess Medical Center approved the study protocol and use of the database for research purposes.
Data on individuals from the MIMIC-IV database who were diagnosed with sepsis according to the Sepsis-3 criteria (6) were retrieved. The KDIGO served as the basis for defining AKI (7). In this study, sepsis patients diagnosed with AKI within 2 days of admission to the ICU were selected as SA-AKI. The study inclusion criteria were:(1) only the first ICU admission was considered, and corresponding data were recorded; (2) patients aged ≥ 18 years old; (3) their length of ICU stays exceeded 24 hours; (4) data on important parameters such as fibrinogen levels and vital signs were completely recorded. Exclusion criteria:(1) Patients die in the first 24 h; (2) Patients without incomplete datasets. The final cohort comprised 3,479 participants (Figure 1). This study was approved by the Ethics Committee of Shenzhen People’s Hospital (Ethics number: LL-KT-201705001).
Figure 1. Flowchart of patient selection. MIMIC-IV, Medical Information Mart for Intensive Care-IV; AKI, Acute kidney injury; HR, Heart Rate; MAP, mean arterial pressure; RR, respiratory rate; ALB, albumin; WBC, white blood cell; Cr, creatinine; INR, international normalized ratio; PT, prolonged prothrombin time; PTT, partial thromboplastin time; LAC, lactate.
We used the Structured Query Language (SQL) to obtain fibrinogen data within the initial 24 hours of ICU admission. In cases where multiple measurements were available, we considered the lowest recorded fibrinogen level. Tertiles were used to classify the fibrinogens into the following three groups: low (fibrinogen ranging from 0.26 to 1.68 g/l), middle (fibrinogen ranging from 1.69 to 3.22 g/l), and high (fibrinogen ranging from 3.23 to 14.66 g/l).
On the basis of previous reports (18, 21, 23), the following variables were included to confirm the association between outcomes and fibrinogen: demographic characteristics such as age and gender, vital signs and laboratory tests including heart rate (HR), mean arterial pressure (MAP), respiratory rate, white blood cell count (WBC), serum albumin (ALB), platelet count (PLT), serum creatinine (Cr), international normalized ratio (INR), partial thromboplastin time (PTT), prothrombin time (PT), and lactate (LAC), as well as comorbidities such as myocardial infarction (MI), congestive heart failure (CHF), cerebrovascular disease, chronic obstructive pulmonary disease (COPD), diabetes (DM), chronic kidney disease (CKD), cancer, liver disease, and metastatic solid tumor (MST). Additionally, we considered severity assessment scores, including the Sequential Organ Failure Assessment (SOFA) score, Simplified Acute Physiology Score III (SAPS III), and the Charlson Comorbidity Index (CCI). Furthermore, we took into account treatments administered on the first day, encompassing mechanical ventilation (MV), renal replacement therapy (RRT), and norepinephrine (NE). Vital signs are represented by mean values, while laboratory test results are based on the most adverse values recorded on the initial day of ICU admission.
Sepsis‐induced coagulopathy (SIC) was diagnosed using International Society on Thrombosis and Hemostasis (ISTH)scoring systems (24). CKD was divided into 5 stages according to glomerular filtration rate (GFR). The GFR is estimated from Chronic Kidney Disease Epidemiology Collaboration (CKD- EPI) as follow (25):
GFR=a x (Cr/b/88.4)^c x (0.993)^age
a: female =144, male=141.
b: female =0.7, male =0.9.
c: female: Cr ≤ 61.88 umol/l, c=-0.329; Cr >61.88 umol/l, c=1.209.
male: Cr ≤ 61.88 umol/l,c =-0.411; Cr >61.88 umol/l, c =-1.20.
The primary outcome was 28-day mortality.
Descriptive analyses based on the fibrinogen tertials were performed. Categorical variables are presented using frequencies and percentages, and continuous variables are expressed as means with standard deviations (SD) for normally distributed data or medians with interquartile ranges (IQR) for skewed distributions. For statistical comparisons, we utilized the chi-square test for categorical variables, the one-way analysis of variance (ANOVA) for normally distributed data, and the Kruskal-Wallis test for skewed data. Survival analysis was performed using Kaplan-Meier curves and log-rank tests.
Univariate and multivariable Cox regression analyses were conducted to assess the relationship between fibrinogen levels and 28-day mortality. Confounding factors were addressed using multivariable-adjusted models. Model 1 adjusted for age and gender. Model 2 included adjustments from Model 1 and additional variables such as MAP, respiratory rate, HR, PLT, WBC, Cr, PT, PTT, INR, ALB and LAC. Model 3 included adjustments from Model 2 and additional variables such as MI, CHF, cerebrovascular disease, COPD, DM, CKD, cancer, liver disease, MST, SOFA, APS III, CCI, MV, RRT and NE.
We used a generalized additive model (GAM) to identify non-linear relationships. A two-piecewise linear regression model was used to assess the threshold effect of the fibrinogen and SA-AKI according to the smoothed curves. In order to ascertain the optimal threshold levels of fibrinogen, a recursive approach was employed, with the maximum model likelihood selected as the optimal outcome. A comparison was made between a one-line linear regression model and a two-piecewise linear model using a likelihood ratio test. Subgroup assessments were performed for sex, age, MAP, INR, PTT, PT, LAC, SOFA, COPD, DM, CKD, liver disease, MV, RRT and NE using a stratified Cox regression model. Likelihood ratio tests were employed to examine the interactions between subgroups. A sensitivity analysis was conducted to evaluate the relationship between fibrinogen and 28-day mortality in individuals with liver disease and SIC. Patients with SA-AKI with missing covariates were deleted, and baseline characteristics were compared between the missing and non-missing groups to assess the effect of missing data on outcomes.
All statistical analyses were carried out using R 3.3.2 from the R Foundation (http://www.R-project.org) and Free Statistics version 1.7. Statistical significance was determined using a two-sided test with a significance level of P < 0.05.
Among the 53,569 admissions reported in MIMIC-IV, a total of 15,671 patients with SA-AKI were identified. The study protocol is schematically represented in Figure 1. Based on the inclusion and exclusion criteria, a total of 3,479 patients with comprehensive data were included for analysis.
Table 1 presents the baseline characteristics of the participants, categorized into groups based on fibrinogen tertials: the low group (≥0.26 to ≤1.68 g/l), the middle group (≥1.69 to ≤3.22 g/l), and the high group (≥3.23 to ≤14.66 g/l). The average age of all participants was 62.5 ± 15.8 years, with 60.3% being male. In the low group, patients were younger and presented more severe conditions, as indicated by lower PLT, higher LAC levels, INR, PT, PTT, SOFA score and SAPS III. These patients also required more life support measures, including a higher utilization of MV and RRT. Conversely, the high group exhibited a higher prevalence of chronic comorbidities such as MI, CHF, COPD, DM, CKD, MST, and CCI. Supplementary Table S5 shows little differences in baseline characteristics between the missing and non-missing groups.
The Kaplan-Meier curve demonstrated that patients in the fibrinogen low group exhibited the highest 28-day mortality rate, while those in the middle group had the lowest mortality rate (Figure 2).
We examined the relationship between fibrinogen levels and 28-day mortality of SA-AKI using both univariate and multivariate Cox proportional hazards regression methods (Table 2). In the univariate analysis, several factors, including sex, age, MAP, respiratory rate, HR, fibrinogen, PLT, WBC, Cr, PT, PTT, INR, ALB, LAC, MI, CHF, CKD, cancer, liver disease, MST, SOFA, APS III, CCI, MV, RRT and NE showed significant association with 28-day mortality (P<0.001). However, on multivariate analysis, only age, respiratory rate, fibrinogen, WBC, PT, PTT, ALB, MI, liver disease, MST, APS III, MV and norepinephrine were identified as independent factors.
Table 2. Univariate Cox proportional hazards regression analysis the association between Fibrinogen and 28-day mortality in Patients With SA-AKI.
The association with fibrinogen as a continuous variable remained significant (HR, 0.961; 95% CI, 0.923-0.999; P=0.0471; Table 3), even after adjusting for all possible covariates (Table 3, model 3).
Table 3. Multivariable Cox proportional hazards regression models evaluating the association between Fibrinogen and 28-day mortality in patients with SA-AKI.
In the fully adjusted model, including fibrinogen as a categorical variable, the 28-day mortality risk was found to be 33% lower (HR, 0.67; 95% CI, 0.56-0.8; P <0.001) for patients in the middle fibrinogen group and 19% lower (HR, 0.81; 95% CI, 0.67-0.97; P =0.024) for those in the high fibrinogen group compared to patients in the low fibrinogen group. Trend analysis indicated a significant trend, with a P trend value of 0.024). All models showed consistent and strong statistical results (Table 3).
A non-linear dose-response relationship between fibrinogen and 28-day mortality was observed after adjusting for the indicated covariates (Figure 3). Using a two-piecewise linear regression model, we identified a threshold for fibrinogen at 1.6 g/l (Table 4). Below this threshold, there was a substantial decrease in 28-day mortality as fibrinogen levels increased (HR 0.509; 95% CI, 0.367-0.707; P < 0.001; Table 4 and Figure 2). However, above the threshold, the estimated dose-response curve appeared to be relatively flat (HR 1.011; 95% CI, 0.961-1.064; P = 0.675; Table 4).
Figure 3. Associations between Fibrinogen concentrations with 28-day mortality among participants with SA-AKI in MIMIC-IV. HRs were adjusted for Model 3 and only 99.5% of the data is shows.
Table 4. The non-linearity relationship between Fibrinogen and 28-day mortality in patients with SA- AKI.
The findings indicate a consistent and robust association between fibrinogen and 28-day mortality in sepsis patients with AKI, as demonstrated in Figure 4. Interactions were observed within specific subgroups, such as age, liver disease, and the use of renal replacement therapy (RRT) on day 1. we further divided the GFR into five categories, each representing different degrees of renal function (Supplementary Table S1). Subsequently, G 1 and G 2 were combined according to CKD risk stratification, while G 3, G 4, and G 5 were combined for stratification and interaction analysis. To assess the association of fibrinogen and SA-AKI with 28-day mortality outcomes in subgroups of GFR and CKD (Supplementary Table S2). After adjustment for all covariates, we observed similar results according to CKD (interaction P = 0.252) and GFR category subgroups (interaction P = 0.076). Furthermore, the sensitivity analysis conducted specifically on the liver disease and SIC populations provided reliable evidences regarding the relationship between fibrinogen and 28-day mortality, with an HR of 0.9 (95% CI: 0.82~0.99, P = 0.029) and HR of 0.97 (95% CI: 0.92~1.02, P = 0.214) (Supplementary Tables S3, S4).
Figure 4. Subgroup analysis of the association between Fibrinogen and 28-day mortality in patients with sepsis and AKI. MAP, mean arterial pressure; INR, international normalized ratio; PT, prolonged prothrombin time; PTT, partial thromboplastin time; LAC, lactate; SOFA, sequential organ failure assessment; COPD, chronic obstructive pulmonary disease; DM, diabetes; CKD; MV, mechanic ventilation; RRT, renal replacement therapy; NE, norepinephrine.
In this retrospective cohort study, we observed a non-linear relationship between fibrinogen and SA-AKI. Specifically, our findings indicate that when fibrinogen levels fell below 1.6 g/L, there was a pronounced reduction in mortality as fibrinogen levels increased. To the best of our knowledge, this is the first study elucidating such a non-linear association between fibrinogen and SA-AKI.
Previous studies have reported a positive association between elevated fibrinogen levels and an increased risk of developing AKI following cardiac valve replacement surgery (15). Fibrinogen, functioning as a coagulation factor, is known to elevate blood viscosity, potentially leading to renal hypoperfusion and hypoxia, thus contributing to the onset of AKI. Consequently, fibrinogen appears to exert a negative influence on both the development and outcomes of AKI. Nevertheless, a study focusing on living donor liver transplantation revealed that the occurrence of AKI was twice as frequent in the low fibrinogen group compared to the normal fibrinogen group (19). Further, our research reveals that individuals with SA-AKI and low fibrinogen levels had increased mortality rates compared to those with medium and high fibrinogen levels. These contrasting findings suggest that the influence of fibrinogen on AKI onset and progression may vary depending on the underlying disease etiology.
Sepsis emerges as the primary precipitating factor for AKI (5, 26). Fibrinogen’s role extends beyond conventional hemostasis in sepsis and plays a critical role in inflammatory responses, tissue injury, cellular migration, and gene expression (16). Research indicates a significant association between lower fibrinogen levels and increased mortality rates in sepsis (18, 20), and pediatric sepsis studies have also confirmed an inverse relationship between fibrinogen and in-hospital mortality rates (OR 0.767) (27). Fibrinogen, as a biomarker of coagulation factor consumption and an acute-phase reactant, rapidly increases during early-stage infections and inflammatory diseases, playing a crucial role in antimicrobial defense and hemostasis (28). Reports indicate sustained elevation of fibrinogen during the course of sepsis to counter inflammatory responses (29).Therefore, elevated fibrinogen levels early in sepsis may benefit host healing processes, while reduced levels could impair the ability to fight infection and regulate inflammation, thereby worsening sepsis prognosis (30). This study affirms the negative correlation (HR=0.961) between fibrinogen as a continuous variable and the 28-day mortality rate of SA-AKI. Compared to the low fibrinogen group, the medium and high fibrinogen groups experience respective reductions in the risk of mortality by 33% and 19%. Additionally, the low fibrinogen group demonstrates higher disease severity scores, including SOFA and APS III, indicating a more severe condition and a poorer prognosis.
Previous research has established a link between fibrinogen levels in sepsis patients and overall mortality, as well as associated organ dysfunction. Among individuals with septic shock, fibrinogen levels experience an average decline of 41% from day 1 to day 3, showing an inverse correlation with 30-day mortality risk (23). Furthermore, in the study on patients with SIC, the group exhibiting a declining trend in fibrinogen levels demonstrated the highest 28-day mortality rate compared to the group with a stable trend (46.7% vs. 10.0%, P=0.027), indicating a heightened risk of death (31). Similarly, our study showed the groups with intermediate and high fibrinogen levels displayed lower mortality rates compared with the low fibrinogen group(HR 0.67 vs. 0.81 vs. 1). Sepsis is characterized by inflammation and coagulation activation, with septic shock and coagulopathy signifying disease progression. During the inflammatory process, there is an increase in clotting factors and a decrease in fibrinolysis, resulting in fibrin deposition within the microcirculation, ultimately leading to thrombosis, microcirculatory disturbances, ischemia, and hypoxia, culminating in shock and organ dysfunction (32, 33). Moreover, excessive consumption of clotting factors leads to a significant reduction in fibrinogen and platelet count, contributing to adverse outcomes such as disseminated intravascular coagulation (DIC). Notably, the kidneys are particularly vulnerable to ischemia and hypoxia, with microcirculatory dysfunction potentially leading to SA-AKI (7, 34, 35). Therefore, the observed higher mortality rate among SA-AKI patients with low fibrinogen levels can be attributed to more severe vascular leakage, ischemia, and hypoxia. Additionally, in animal models of ischemia-reperfusion injury (36), the fibrinogen fragment Bβ15-42 has demonstrated protective effects by reducing inflammation and vascular leakage. Furthermore, in our study, the low fibrinogen group exhibited prolonged PTT and PT, as well as decreased platelet count, indicating an increased risk of bleeding and DIC, further emphasizing the association between low fibrinogen levels and adverse prognosis.
Contrary to previous findings associating high fibrinogen levels with cardiovascular diseases such as cardiac valve surgery and contrast-induced acute kidney injury (15, 17), our study reached the opposite conclusion. We discovered that SA-AKI patients with low fibrinogen levels have higher mortality rates, which may be related to the protective role of fibrinogen as an acute-phase protein in sepsis (28). Fundamental research has shown that fibrinogen protects cells from damage by binding to histones and fibrin released upon cell exposure (37). Therefore, increased fibrinogen concentration in the early stages of infection aids in preventing cell injury. However, during sepsis or trauma, when inflammation intensifies and immune function becomes excessively impaired, fibrinogen consumption exacerbates, diminishing its protective capacity. This leads to aggravated tissue injury, worsened prognosis, and increased mortality rates. Consistent with these observations, a study demonstrated that low fibrinogen levels remain a risk factor for in-hospital mortality in ICU AKI patients with sepsis (HR=1.29) (21). Our study yielded similar results. Moreover, our research revealed a nonlinear correlation between fibrinogen and SA-AKI, establishing a threshold of 1.6 g/l below which the risk of death significantly increases.
Patients with cirrhosis have an increased risk of developing AKI, which can eventually progress to hepatorenal syndrome and result in a significant risk of mortality (21). To account for potential interference from liver disease, we included liver disease as a confounder in the multivariate Cox analysis. Despite this adjustment, the association between fibrinogen and SA-AKI mortality remained robust. Subgroup analyses revealed that fibrinogen, as a continuous variable, exhibited a stronger association with 28-day mortality outcomes in patients with liver disease and SA-AKI than in those without liver disease (HR, 0.903;95% CI,0.825-0.990). As fibrinogen is produced by the liver, liver disease can result in reduced fibrinogen production and a poor prognosis for coagulopathy (38). Previous studies have demonstrated that low fibrinogen levels are an independent predictor of mortality in patients with acute-on-chronic hepatitis B liver failure (OR, 0.304; 95% CI: 0.094-0.983; P = 0.047) (39). Our subgroup results are consistent with these findings, indicating that fibrinogen levels are inversely associated with mortality risk in patients with liver disease, even in the context of SA-AKI. Patients undergoing RRT are at risk of bleeding from anticoagulant therapy (40). Fibrinogen is a predictor of the risk of bleeding prior to RRT. A reduction in fibrinogen levels is indicative of an elevated risk of bleeding and may portend a poor prognosis. As demonstrated in the subgroup analysis, fibrinogen exhibited a more pronounced inverse correlation in patients undergoing RRT compared to those not undergoing RRT (HR = 0.860, 95% CI: 0.757–0.977) (41). Although age is a risk factor for sepsis death, subgroup results show that compared with the elderly group ≥60 years, fibrinogen was more negatively associated with outcomes in younger than 60 years of age (HR: 0.861,95% CI: 0.788-0.940). This association warrants further investigation.
Nonetheless, this study has some limitations. Firstly, due to the limitations of the MIMIC database, the covariates (ALB, LAC) were excluded from the analysis owing to substantial missing data. However, a baseline comparison between groups with and without missing covariates was conducted, revealing minimal differences between the groups. Nevertheless, higher-quality prospective studies are needed in the future to validate our findings. Secondly, we only assessed fibrinogen levels within 24 hours after ICU admission without dynamic observation of patients’ fibrinogen levels, making it impossible to judge dynamic changes in fibrinogen for a comprehensive assessment of disease. Additionally, we did not consider the effect of fibrinogen infusion on outcomes. In the future, we will investigate the prognostic significance of changes in fibrinogen levels in patients with SA-AKI using the MIMIC database.
A non-linear association was observed between fibrinogen and SA-AKI. When fibrinogen was below 1.6 g/l, mortality risk decreased as fibrinogen increased.
The original contributions presented in the study are included in the article/Supplementary Material. Further inquiries can be directed to the corresponding authors.
The studies involving humans were approved by The institutional review boards of Massachusetts Institute of Technology and Beth Israel Deaconess Medical Center approved the study protocol and use of the database for research purposes. This study was approved by the Ethics Committee of Shenzhen People’s Hospital (Ethics number: LL-KT-201705001). The studies were conducted in accordance with the local legislation and institutional requirements. Written informed consent for participation was not required from the participants or the participants’ legal guardians/next of kin because patient identifiers and data information were de-identified.
MC: Writing – review & editing, Data curation, Formal analysis, Investigation, Writing – original draft. XC: Conceptualization, Formal analysis, Writing – review & editing. HL: Investigation, Writing – original draft. CB: Formal analysis, Writing – review & editing. LC: Formal analysis, Writing – review & editing. LZ: Data curation, Writing – review & editing. PG: Funding acquisition, Resources, Supervision, Writing – review & editing. FS: Project administration, Supervision, Writing – review & editing.
The author(s) declare financial support was received for the research, authorship, and/or publication of this article. This study was funded by Shenzhen Key Medical Discipline Construction Fund (SZXK046).
We thank Liu Jie (People’s Liberation Army of China General Hospital, Beijing, China) and Yang Qilin (The Second Affiliated Hospital of Guangzhou Medical University, Guangzhou, Guangdong, China) for helping in this revision.
The authors declare that the research was conducted in the absence of any commercial or financial relationships that could be construed as a potential conflict of interest.
All claims expressed in this article are solely those of the authors and do not necessarily represent those of their affiliated organizations, or those of the publisher, the editors and the reviewers. Any product that may be evaluated in this article, or claim that may be made by its manufacturer, is not guaranteed or endorsed by the publisher.
The Supplementary Material for this article can be found online at: https://www.frontiersin.org/articles/10.3389/fneph.2024.1398386/full#supplementary-material
1. Kellum JA, Romagnani P, Ashuntantang G, Ronco C, Zarbock A, Anders HJ. Acute kidney injury. Nat Rev Dis Primers. (2021) 7:52. doi: 10.1038/s41572-021-00284-z
2. Khwaja A. KDIGO clinical practice guidelines for acute kidney injury. Nephron Clin Pract. (2012) 120:c179–184. doi: 10.1159/000339789
3. Kinsey GR, Li L, Okusa MD. Inflammation in acute kidney injury. Nephron Exp Nephrol. (2008) 109:e102–107. doi: 10.1159/000142934
4. Jang HR, Rabb H. Immune cells in experimental acute kidney injury. Nat Rev Nephrol. (2015) 11:88–101. doi: 10.1038/nrneph.2014.180
5. Wang K, Xie S, Xiao K, Yan P, He W, Xie L. Biomarkers of sepsis-induced acute kidney injury. BioMed Res Int. (2018) 2018:6937947. doi: 10.1155/2018/6937947
6. Singer M, Deutschman CS, Seymour CW, Shankar-Hari M, Annane D, Bauer M, et al. The third international consensus definitions for sepsis and septic shock (Sepsis-3). Jama. (2016) 315:801–10. doi: 10.1001/jama.2016.0287
7. Zarbock A, Nadim MK, Pickkers P, Gomez H, Bell S, Joannidis M, et al. Sepsis-associated acute kidney injury: consensus report of the 28th Acute Disease Quality Initiative workgroup. Nat Rev Nephrol. (2023) 19:401–17. doi: 10.1038/s41581-023-00683-3
8. Doi K. Role of kidney injury in sepsis. J Intensive Care. (2016) 4:17. doi: 10.1186/s40560-016-0146-3
9. Hoste EA, Bagshaw SM, Bellomo R, Cely CM, Colman R, Cruz DN, et al. Epidemiology of acute kidney injury in critically ill patients: the multinational AKI-EPI study. Intensive Care Med. (2015) 41:1411–23. doi: 10.1007/s00134-015-3934-7
10. Poston JT, Koyner JL. Sepsis associated acute kidney injury. Bmj. (2019) 364:k4891. doi: 10.1136/bmj.k4891
11. Bagshaw SM, Uchino S, Bellomo R, Morimatsu H, Morgera S, Schetz M, et al. Septic acute kidney injury in critically ill patients: clinical characteristics and outcomes. Clin J Am Soc Nephrol. (2007) 2:431–9. doi: 10.2215/CJN.03681106
12. Cruz MG, Dantas JG, Levi TM, Rocha Mde S, de Souza SP, Boa-Sorte N, et al. Septic versus non-septic acute kidney injury in critically ill patients: characteristics and clinical outcomes. Rev Bras Ter Intensiva. (2014) 26:384–91. doi: 10.5935/0103-507X.20140059
13. Induruwa I, Moroi M, Bonna A, Malcor JD, Howes JM, Warburton EA, et al. Platelet collagen receptor Glycoprotein VI-dimer recognizes fibrinogen and fibrin through their D-domains, contributing to platelet adhesion and activation during thrombus formation. J Thromb Haemost. (2018) 16:389–404. doi: 10.1111/jth.13919
14. Mori S, Taki T, Murakami Y, Urata T, Okumura M, Akanabe H, et al. Low plasma fibrinogen levels are associated with poor prognosis in cutaneous angiosarcoma of the head and neck. Cancer Sci. (2021) 112:3924–7. doi: 10.1111/cas.v112.9
15. Yang JJ, Lei WH, Hu P, Wu BB, Chen JX, Ni YM, et al. Preoperative serum fibrinogen is associated with acute kidney injury after cardiac valve replacement surgery. Sci Rep. (2020) 10:6403. doi: 10.1038/s41598-020-63522-6
16. Guan XL, Li L, Jiang WJ, Gong M, Li HY, Liu YY, et al. Low preoperative serum fibrinogen level is associated with postoperative acute kidney injury in patients with in acute aortic dissection. J Cardiothorac Surg. (2023) 18:6. doi: 10.1186/s13019-023-02114-7
17. Li J, Wang Z, Zhang B, Zheng D, Lu Y, Li W. Predictive value of combining the level of fibrinogen and CHA2DS2-VASC Score for contrast-induced acute kidney injury in patients with acute coronary syndromes undergoing percutaneous coronary intervention. Int Urol Nephrol. (2022) 54:2385–92. doi: 10.1007/s11255-022-03149-w
18. Yao C, Zhang G, Zhang N, Li R, Sun S, Zhang L, et al. Fibrinogen is associated with prognosis of critically ill patients with sepsis: A study based on cox regression and propensity score matching. Mediators Inflammation. (2023) 2023:7312822. doi: 10.1155/2023/7312822
19. Park J, Joo MA, Choi HJ, Hong SH, Park CS, Choi JH, et al. Predictive utility of fibrinogen in acute kidney injury in living donor liver transplantation: A propensity score-matching analysis. PLoS One. (2021) 16:e0252715. doi: 10.1371/journal.pone.0252715
20. Matsubara T, Yamakawa K, Umemura Y, Gando S, Ogura H, Shiraishi A, et al. Significance of plasma fibrinogen level and antithrombin activity in sepsis: A multicenter cohort study using a cubic spline model. Thromb Res. (2019) 181:17–23. doi: 10.1016/j.thromres.2019.07.002
21. Zhang G, Zhang L, Si S, Jiang T, Xia Y, Zhu Y, et al. Fibrinogen and antithrombin III are associated with in-hospital mortality among critically ill patients with acute kidney injury. Ren Fail. (2022) 44:1938–47. doi: 10.1080/0886022X.2022.2142138
22. Johnson AEW, Bulgarelli L, Shen L, Gayles A, Shammout A, Horng S, et al. MIMIC-IV, a freely accessible electronic health record dataset. Sci Data. (2023) 10:1. doi: 10.1038/s41597-022-01899-x
23. Schupp T, Weidner K, Rusnak J, Jawhar S, Forner J, Dulatahu F, et al. Fibrinogen reflects severity and predicts outcomes in patients with sepsis and septic shock [J. Blood Coagul Fibrinolysis. (2023) 34:161–70. doi: 10.1097/MBC.0000000000001197
24. Iba T, Levy JH, Warkentin TE, Thachil J, van der Poll T, Levi M. Diagnosis and management of sepsis-induced coagulopathy and disseminated intravascular coagulation. J Thromb Haemost. (2019) 17:1989–94. doi: 10.1111/jth.14578
25. KDIGO 2021 clinical practice guideline for the management of glomerular diseases. Kidney Int. (2021) 100:S1–s276. doi: 10.1016/j.kint.2021.05.021
26. Zhi DY, Lin J, Zhuang HZ, Dong L, Ji XJ, Guo DC, et al. Acute kidney injury in critically ill patients with sepsis: clinical characteristics and outcomes. J Invest Surg. (2019) 32:689–96. doi: 10.1080/08941939.2018.1453891
27. Tang X, Shao L, Dou J, Zhou Y, Chen M, Cui Y, et al. Fibrinogen as a prognostic predictor in pediatric patients with sepsis: A database study. Mediators Inflammation. (2020) 2020:9153620. doi: 10.1155/2020/9153620
28. Ko YP, Flick MJ. Fibrinogen Is at the Interface of Host Defense and Pathogen Virulence in Staphylococcus aureus Infection. Semin Thromb Hemost. (2016) 42:408–21. doi: 10.1055/s-0036-1579635
29. Lorente JA, García-Frade LJ, Landín L, de Pablo R, Torrado C, Renes E, et al. Time course of hemostatic abnormalities in sepsis and its relation to outcome. Chest. (1993) 103:1536–42. doi: 10.1378/chest.103.5.1536
30. Orfanu A, Aramă V, Popescu C, Tilişcan C, Streinu-Cercel A, Aramă ŞS. The dynamical assessment of inflammatory biomarkers in predicting the outcome of septic patients and the response to antimicrobial therapy. J Crit Care Med (Targu Mures). (2020) 6:25–31. doi: 10.2478/jccm-2020-0004
31. Mori K, Tsujita Y, Yamane T, Eguchi Y. Decreasing plasma fibrinogen levels in the intensive care unit are associated with high mortality rates in patients with sepsis-induced coagulopathy. Clin Appl Thromb Hemost. (2022) 28:10760296221101386. doi: 10.1177/10760296221101386
32. Levi M. The coagulant response in sepsis and inflammation. Hamostaseologie. (2010) 30:10–12, 14-16.
33. Angus DC, van der Poll T. Severe sepsis and septic shock. N Engl J Med. (2013) 369:840–51. doi: 10.1056/NEJMra1208623
34. Ma S, Evans RG, Iguchi N, Tare M, Parkington HC, Bellomo R, et al. Sepsis-induced acute kidney injury: A disease of the microcirculation. Microcirculation. (2019) 26:e12483. doi: 10.1111/micc.2019.26.issue-2
35. Peerapornratana S, Manrique-Caballero CL, Gómez H, Kellum JA. Acute kidney injury from sepsis: current concepts, epidemiology, pathophysiology, prevention and treatment. Kidney Int. (2019) 96:1083–99. doi: 10.1016/j.kint.2019.05.026
36. Urbschat A, Rupprecht K, Zacharowski K, Obermüller N, Scheller B, Holfeld J, et al. Combined peri-ischemic administration of Bβ15-42 in treating ischemia reperfusion injury of the mouse kidney. Microvasc Res. (2015) 101:48–54. doi: 10.1016/j.mvr.2015.06.005
37. Locke M, Francis RJ, Tsaousi E, Longstaff C. Fibrinogen protects neutrophils from the cytotoxic effects of histones and delays neutrophil extracellular trap formation induced by ionomycin. Sci Rep. (2020) 10:11694. doi: 10.1038/s41598-020-68584-0
38. Guo HY, Zhang ZG, Zhang YY, Mei X, Liu Y, Wang JF, et al. Risks and predicting factors of bleeding complications in hepatitis B virus-related acute-on-chronic liver failure. Turk J Gastroenterol. (2020) 31:620–5. doi: 10.5152/tjg.2020.19307
39. Shao Z, Zhao Y, Feng L, Feng G, Zhang J, Zhang J. Association between plasma fibrinogen levels and mortality in acute-on-chronic hepatitis B liver failure. Dis Markers. (2015) 2015:468596. doi: 10.1155/2015/468596
40. Roe NA, Wiss AL, Volgas S, Hudson JQ. Review of anticoagulation in continuous renal replacement therapy. Crit Care Nurs Q. (2022) 45:144–55. doi: 10.1097/CNQ.0000000000000397
Keywords: fibrinogen, sepsis, acute kidney injury, sepsis-associated acute kidney injury, mortality, medical information mart for intensive care-IV
Citation: Chen M, Chen X, Ling H, Bai C, Chen L, Zhong L, Gong P and Shi F (2024) Prognostic significance of fibrinogen levels in sepsis-associated acute kidney injury: unveiling a nonlinear relationship and clinical implications. Front. Nephrol. 4:1398386. doi: 10.3389/fneph.2024.1398386
Received: 09 March 2024; Accepted: 11 October 2024;
Published: 06 November 2024.
Edited by:
Shengnan Li, Huazhong University of Science and Technology, ChinaReviewed by:
Liyan Cui, Peking University Third Hospital, ChinaCopyright © 2024 Chen, Chen, Ling, Bai, Chen, Zhong, Gong and Shi. This is an open-access article distributed under the terms of the Creative Commons Attribution License (CC BY). The use, distribution or reproduction in other forums is permitted, provided the original author(s) and the copyright owner(s) are credited and that the original publication in this journal is cited, in accordance with accepted academic practice. No use, distribution or reproduction is permitted which does not comply with these terms.
*Correspondence: Fei Shi, c2hpLmZlaUBzemhvc3BpdGFsLmNvbQ==; Ping Gong, Z29uZ3A4MjhAc2luYS5jbg==
†These authors share first authorship
Disclaimer: All claims expressed in this article are solely those of the authors and do not necessarily represent those of their affiliated organizations, or those of the publisher, the editors and the reviewers. Any product that may be evaluated in this article or claim that may be made by its manufacturer is not guaranteed or endorsed by the publisher.
Research integrity at Frontiers
Learn more about the work of our research integrity team to safeguard the quality of each article we publish.