- 1College of Health Sciences, University of Memphis, Memphis, TN, United States
- 2Division of Endocrinology, University of Tennessee Health Sciences Center (UTHSC), Memphis, TN, United States
- 3Department of Biological Sciences, University of Memphis, Memphis, TN, United States
- 4Department of Clinical Medicine, Aarhus University Hospital, Aarhus, Denmark
- 5College of Nursing, Resonance, Memphis, TN, United States
- 6Department of Health Promotion and Disease Prevention, University of Tennessee Health Science Center, Memphis, TN, United States
Introduction: Early identification of compromised renal clearance caused by high-dose methotrexate (HDMTX) is essential for initiating timely interventions that can reduce acute kidney injury and MTX-induced systemic toxicity.
Methods: We induced acute kidney injury (AKI) by infusing 42 juvenile pigs with 4 g/kg (80 g/m2) of MTX over 4 hours without high-volume alkalinizing hydration therapy. Concentrations of serum creatinine and MTX were measured at 15 time points up to 148 hours, with 10 samples collected during the first 24 hours after the start of the HDMTX infusion.
Results: During the first 28 hours, 81% of the pigs had increases in the concentrations of serum creatinine in one or more samples indicative of AKI (i.e., > 0.3g/dL increase). A rate of plasma MTX clearance of less than 90% during the initial 4 hours after the HDMTX infusion and a total serum creatinine increase at 6 and 8 hours after starting the infusion greater than 0.3 g/dL were predictive of AKI at 28 hours (p < 0.05 and p < 0.001, respectively). At conclusion of the infusion, pigs with a creatinine concentration more than 0.3 g/dL higher than baseline or serum MTX greater than 5,000 μmol/L had an increased risk of severe AKI.
Conclusions: Our findings suggest that serum samples collected at conclusion and shortly after HDMTX infusion can be used to predict impending AKI. The pig model can be used to identify biological, environmental, and iatrogenic risk factors for HDMTX-induced AKI and to evaluate interventions to preserve renal functions, minimize acute kidney injury, and reduce systemic toxicity.
1 Introduction
High-dose methotrexate (HDMTX), defined as doses of 1 g/m2 of body surface area and higher, is used to treat a variety of cancers, including acute lymphoblastic leukemia, non-Hodgkin lymphoma, and osteosarcoma (1). Supportive care during and after HDMTX is critical to reduce the risk of toxicity and especially acute kidney injury (AKI). Methotrexate (MTX)-induced AKI is thought to be caused when MTX crystalizes in the renal tubular lumen, leading to tubular toxicity (1–3). Because low urine pH decreases the solubility of MTX and the metabolite 7-hydroxy-methotrexate (7OH-MTX) and increases MTX uptake by renal tubular epithelial cells, hyperhydration and urine alkalinization are used to ensure a urine pH ≥ 7.0 until the serum MTX level is < 0.2 µM to reduce both crystal-induced injury and cellular damage after internalization and polyglutamation within urothelial cells (1, 4, 5).
In children treated with HDMTX, the incidence of AKI (1.5-fold increase in plasma creatinine concentration) is seen in up to 40% of all children receiving 24-hour infusions of HDMTX at 8 g/m² (6). Most children recover from HDMTX-induced AKI (7), but renal functions can be compromised for weeks (8) and cause delays in subsequent cancer treatment. Early detection of nephrotoxicity for immediate initiation of effective interventions is essential to avoid irreversible damage and permanent nephron destruction. Currently, an increase in serum creatinine concentration or a persistently high level of serum MTX after the HDMTX infusion are the most commonly used indicators of impaired renal function.
Serum creatinine remains a widely used biomarker for AKI (9, 10). However, creatinine has limitations as an indicator of renal clearance and injury (11–13) and significant increases in concentration may not occur for 24 hours or longer after the onset of renal injury. Alternative markers of renal injury (14–18) suffer the same shortcomings as creatinine, in that appearance in the serum or urine occurs after renal injury has already occurred.
The impact of early detection of MTX-induced AKI and the efficacy of early interventions have not been methodically studied, in part because no relevant large animal model has been developed in which MTX-induced impaired renal clearance can be reliably produced. This includes determining if early treatment with glucarpidase, an enzyme that converts MTX into non-toxic metabolites and is currently used as a rescue drug in cases with severely delayed MTX elimination and AKI (7, 19, 20), will prevent the further deterioration of renal functions.
Rodent models using mostly mice and rats have dominated HDMTX research. Despite the insights gained into the mechanisms of toxicity, differences in renal characteristics and the small size of rodent models limit the translation of findings into clinical care. Non-human primates share similarities with humans, but high costs, and specialized care and caging requirements limit their use for preclinical studies. This study used juvenile pigs as a translational large animal model that is relevant for patients receiving HDMTX. Pigs have a renal structure and renal functions that are similar to humans, as evident from recent pig-to-human kidney transplants. Pigs are sufficiently large to allow for central venous access and serial blood sampling, and reliably develop AKI compatible with the HDMTX-induced renal toxicity in humans (as shown in the present study). Furthermore, pigs are suitable for replicating the chemotherapy and supportive care procedures used in patients and for evaluating interventions. Pigs have been used to evaluate the pharmacokinetics of low-dose MTX (21), as a preclinical model to assess the safety of MTX administration directly into the fourth ventricle (22), and to study the relationship between renal clearance and glomerular filtration (23). Moreover, porcine models for gastrointestinal damage associated with the chemotherapeutic agent doxorubicin replicate the conditions in humans (24, 25).
2 Materials and methods
2.1 Pigs and their care
All aspects of the research involving animals were approved by the University of Memphis Institutional Animal Care and Use Committee (protocol #0745). A total of 56 weaned, specific pathogen-free male pigs of a consistent genetic lineage were obtained at approximately 20 days of age from a commercial source. Male pigs were used because of the possible increased risk of nephrotoxicity caused by HDMTX (26). The pigs were initially group housed in a Polydome Litter Saver Pig Nursery with environmental enrichment during a 3- to 5-day period of acclimation to laboratory settings, the experimental diet, and human contact. The room was maintained at 22°C ± 1°C, with a 12 : 12 hour light : dark cycle. The pigs were weighed daily and had frequent human interactions to facilitate chemotherapy administration, handling, and sampling.
To mimic nutrition support for cancer patients and reduce the magnitude of weight loss, the pigs were fed a premium dog food (Purina Pro Plan®) to increase the protein and energy supply relative to standard pig feed. Prior to dosing, a catheter (3.5 French single-lumen Umbili-Cath™; Utah Medical Products, Inc., Midvale, UT, USA) was surgically placed in the right jugular vein, then tunneled to, and exteriorized on, the dorsal surface at the base of the neck, and secured using a Statlock® Foley Stabilization Device (Bard Medical, Covington, GA, USA). The catheter was used for administration of MTX, the antiemetic ondansetron [Pfizer, Inc., NY, USA; 0.15 mg/kg, IV (intravenously)], and leucovorin (0.5 mg/kg, IV), and for the collection of blood samples. For the surgery, the pigs were fasted overnight and sedated [Telazol®; Fort Dodge Animal Health, Fort Dodge, IA, USA; 3–4 mg/kg, IM (intramuscularly)]. A sterile field was prepared, and anesthesia was induced and maintained using isoflurane (1%–5% with oxygen). The pigs received postoperative analgesia immediately after surgery [Carprofen®; Fort Dodge Animal Health, Fort Dodge, IA, USA; 2-4 mg/kg, IM and after 24 hours [Metacam®; Boehringer Ingelheim Animal Health, Duluth, GA, USA; 0.2 mg/kg, per os (orally)]. The pigs were individually housed after the surgery. A prophylactic antibiotic (cefazolin; Bayer Animal Health; 10 mg/kg, IV) was given at the conclusion of the surgery and for 2 days post surgery. The catheters were flushed 2 or 3 times daily with heparinized saline (50 units/mL).
2.2 Administration of MTX
The pigs were allowed to recover from surgery and were feeding and gaining weight before starting MTX dosing. After collection of a baseline blood sample and administration of the antiemetic ondansetron (0.15 mg/kg; IV), the dose of MTX was infused over a 4-hour period (Abbott Plus XL3) during which the pigs were awake and resting in slings. To reduce the risk of gastrointestinal and hematologic toxicities, leucovorin (0.5 mg.kg, IV) was provided to all pigs via the jugular catheter at hours 28 and 52 after the start of the MTX infusion.
The dose of MTX necessary for a single dose to induce renal toxicity was determined using 14 pigs and by escalating the dose from 0.4 g/kg (n = 2), which is equivalent to a human dose of 8 g/m2, to 1.2 g/kg (n = 4) and then to 2 g/kg (n = 8), which still did not cause an increase in serum creatinine concentration within 48 hours (data not reported). Escalation of the MTX dose to 4 g/kg (equivalent to 80 g/m²) induced renal toxicity based on increased serum creatinine concentration and delayed clearance of MTX. The MTX solutions were prepared by solubilizing MTX powder of the desired concentration (2.4, 4, or 8 g/L) in 800 mL of deionized water while stirring and slowly adding 10% sodium hydroxide (NaOH) until most of the MTX was dissolved (solution pH was 7–9). To dissolve the remaining MTX, a 1% NaOH solution was gradually added together with 2.6 g of NaCl and 9 g of benzyl alcohol while continuously stirring until all components were dissolved. The final pH was adjusted to 7.4 using 1% hydrochloric acid (HCl) and the volume was increased to 1 L using deionized water. The prepared MTX solution was placed in intravenous bags and infused using IV sets with a 0.22-micron inline filter at a rate of 12.5 mL/kg/h (250 mL/m2/h) for 4 hours. This infusion rate for 4 hours exceeds the hyperhydration of most pediatric protocols, which recommend 125 mL/m²/h during the HDMTX infusion (1). Unlike in pediatric protocols, additional IV hydration was not provided to the pigs before or after the MTX infusion. The volume of water the pigs were allowed to drink after the MTX infusion was limited to 5 mL/kg every hour for 12 hours. Thereafter water was available ad libitum. Urine pH was recorded by pH meter before and after administration of the MTX.
A second dose of MTX was provided to 10 pigs at hour 76 after the start of the first HDMTX infusion. These pigs included seven without evidence of AKI and one each with mild, moderate, and severe AKI at 28 hours after the start of the first dose of MTX.
2.3 Assessment of responses to MTX
The pigs were observed a minimum of five times each day to monitor general health (eating, drinking, activity, responsiveness to care staff, and vomiting), and stool consistency (using a Likert scale from 0 for normal to 3 for severe diarrhea). Body weight was recorded daily.
Blood samples (1 mL) were collected in serum separator tubes immediately before (hour 0) and at conclusion of the MTX administration (hour 4) and at hours 5, 6, 8, 10, 12, 14, 16, 22, and 28. Thereafter blood samples were collected daily until day 6 (hour 148) after start of the MTX infusion. The serum was isolated and used for measurement of MTX and creatinine (Vet Axcel® Chemistry Analyzer; Alfa Wassermann). The diagnosis of AKI was defined as a ≥ 0.3 mg/dL increase in serum creatinine concentration relative to the start of the infusion (12). The severity of AKI was characterized as mild, moderate, and severe based on creatinine concentration increases above baseline of 0.3–0.5 (mild), 0.5–1.0 (moderate), and > 1.0 mg.dL (severe).
Serum MTX was measured using an enzyme-based method that was adapted for a microplate assay (27). Briefly, 20 μL of serum (with and without serial dilution in water) was added to 130 μL of human dihydrofolate reductase solution (DHFR; R&D Systems, Inc., Minneapolis, MN, USA; two units in 0.05 M Tris buffer pH 7.5) and incubated for 1 minute at 37°C in the plate reader (Synergy 2; BioTek, Winooski, VT, USA) before adding 50 μL of a mixture of dihydrofolate (Sigma Aldrich, St Louis, MO; 50 mg in 15 mL of 0.5M Tris buffer pH 7.5, with 20% 2-mercaptoethanol) and nicotinamide adenine dinucleotide phosphate (NADPH; Sigma Aldrich, St. Louis, MO, USA; 50 mg in 10 mL of 0.5M Tris buffer pH 7.5). The reaction mixture was incubated for 60 minutes at 37°C, during which the change in absorbance at 340 nm was recorded every 30 seconds. A standard curve was prepared for the initial rate of decline in absorbance in the presence of MTX concentrations ranging from 0 to 20 μmol/L.
The majority of pigs (n = 31) were euthanized at hour 148 (Euthasol®; Virbac Animal Health, Fort Worth, TX, USA; 1 mL/4.5 kg). It was necessary to humanely euthanize eight pigs before 148 hours because they had become moribund (unresponsive, weight loss greater than 20%) or had an exceedingly high serum concentration of creatinine (> 7 mg/dL). Another three pigs died suddenly between 24 and 72 hours. The kidneys were removed from all pigs and were inspected externally and internally for gross signs of injury and for visual accumulation of MTX crystals. Other organs were visually inspected.
2.4 Statistical analysis
Values presented in tables are means and standard deviations. The rate of decline in the levels of serum MTX (CSC) was defined as change between the MTX concentration at a specific time point and the MTX concentration at the end of the MTX infusion:
Higher CSC values represent faster rates of MTX elimination from the blood. A generalized linear model with mixed effects was used to model CSC values with AKI at 24 hours for the pigs that received the highest MTX infusion. A p-value less than 0.05 was considered statistically significant.
3 Results
The 42 pigs treated with the highest dose of MTX (4 g/kg) survived the infusion, but 11 pigs died or were euthanized before day 6 after the infusion. The high volume of fluid infused with the MTX resulted in high urine output during the MTX infusion. The pH of urine collected prior to and immediately after the MTX infusion averaged 5.8 (± 0.61; range 4.9–7.1), which is similar to previously published pH measurements of pig urine (28). The presence of MTX crystals in the urine during and after administration of the MTX and in the kidneys of pigs that were necropsied at or before hour 28 (Figure 1) indicates that the concentration of MTX in the urine exceeded the solubility limit. Diarrhea and vomiting were observed for only a few animals during the first 24 hours after the MTX infusion and for no pigs after 24 hours. The feces collected during the first 24 hours after the MTX infusion had a yellow tinge. An analysis of stool samples revealed the presence of MTX, although concentrations were not determined. Soon after the start of the MTX infusion, the skin of the pigs developed a yellowish appearance, which gradually disappeared during the next 24 hours. Most pigs that received the 4 g/kg of MTX were lethargic for 6 to 12 hours after the infusion, but readily drank the restricted volume of water. Intrarenal accumulation of MTX crystals was seen in the kidneys of all pigs that died or were euthanized within 52 hours after the MTX infusion (Figure 1), but not in kidneys from the pigs that were necropsied at 148 hours.
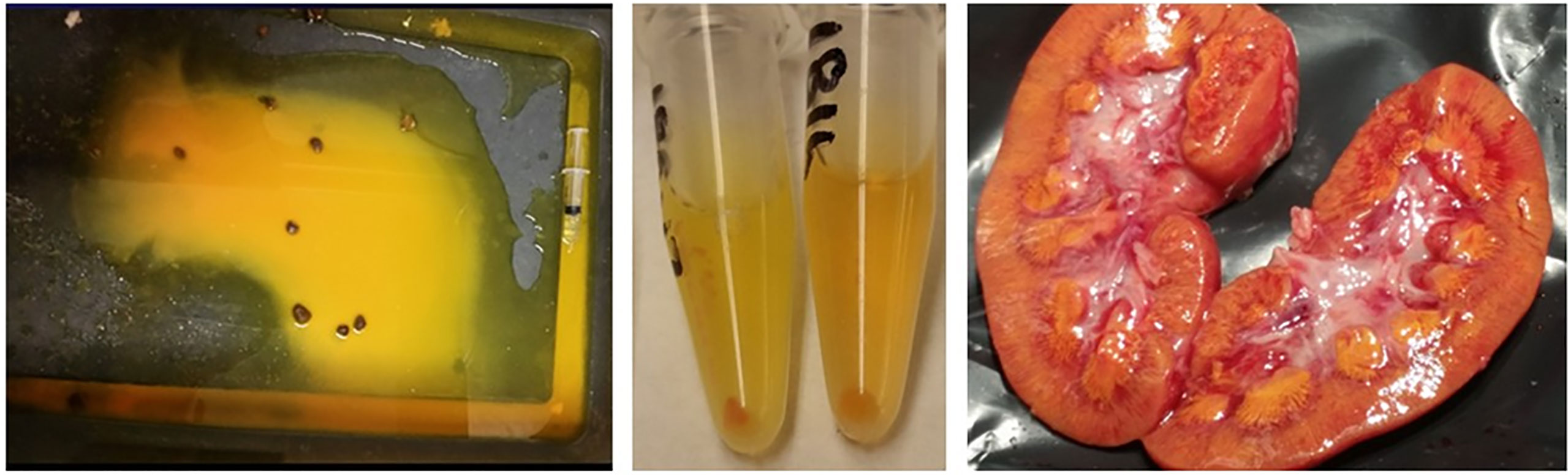
Figure 1 Macroscopic methotrexate crystals were evident in the pen (left panel) and sedimented in the urine (middle panel) during the infusion and in the kidneys (right panel) of animals with acute kidney injury after administering 4 g methotrexate/kg body weight.
3.1 Body weight gain before and after administration of 4 g/kg of MTX
Most of the pigs (92%) gained weight during the 3 days prior to MTX administration (Table 1). The average gain of 2.8% of body weight per day (+ 1.8%) is lower than in production settings but was not unexpected, as the pigs had just been weaned, were recovering from surgery, and were isolated after surgery. During the 3 days after the MTX infusion, 30 pigs (70%) lost weight (–0.90%/day ± 1.98%). This is similar to the growth depression of rabbits after high doses of methotrexate (29). The majority of the pigs (72%) began to gain weight again from days 3 to 6 after the MTX infusion (2.28%/d + 3.26%).
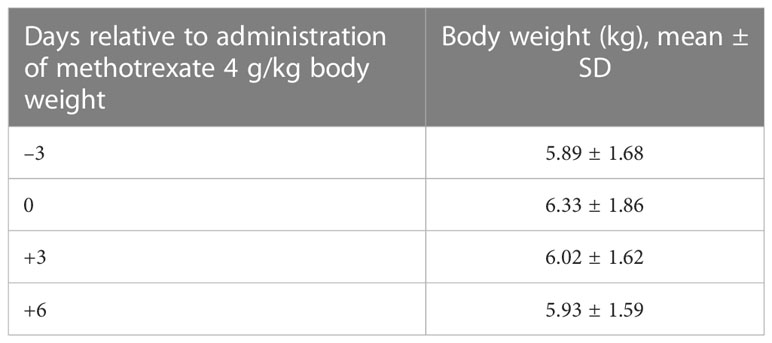
Table 1 Body weights (kg) of experimental pigs used to evaluate responses to administration of 4 g/kg methotrexate.
3.2 Serum creatinine and incidence of acute kidney injury
None of the pigs at the start of the MTX infusion had an elevated serum creatinine concentration indicative of AKI. The percentage of pigs that developed AKI increased after the MTX infusion (Figure 2). By 6 hours, nearly 40% of the pigs had an elevated creatinine concentration indicative of AKI. The percentage of pigs with one or more incidences of AKI increased to 70% at 16 hours and 76% at 28 hours, with 81% of the pigs eventually developing AKI of varying severity and duration. Eight pigs (19%) never developed AKI, including three that received the second dose of MTX at 76 hours.
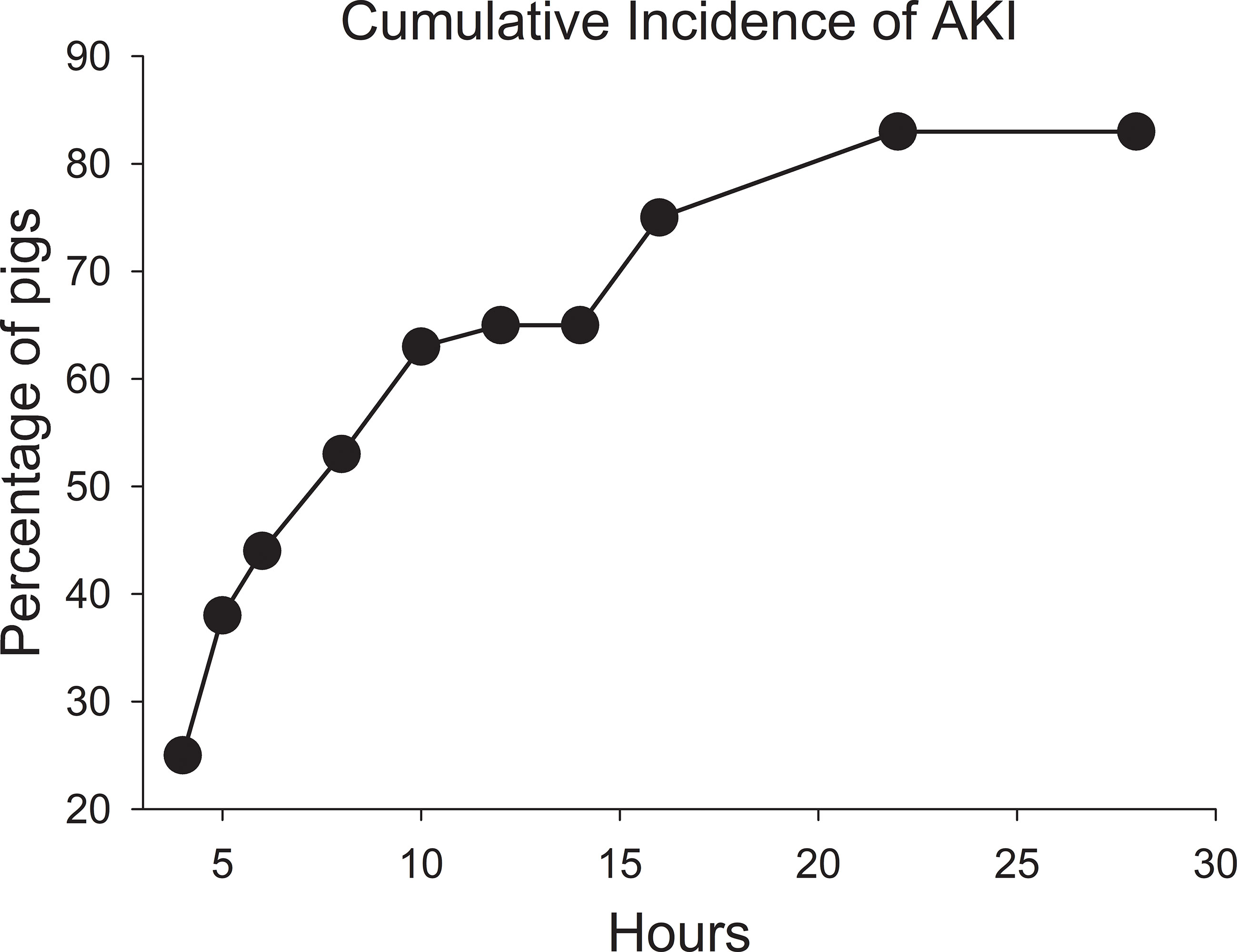
Figure 2 Cumulative incidence of acute kidney injury after starting the infusion of high-dose methotrexate at 4 g/kg body weight.
On a population basis, the mean serum creatinine concentration at the end of the 4-hour MTX infusion was unchanged compared with the mean pre-infusion concentration (Table 2). Because of the high volume of fluid that was infused (250 mL/m2/h) to administer the dose of MTX, at conclusion of the infusion serum creatinine concentration had decreased in 14 pigs (32%). Of these, only four had evidence of AKI at 28 hours. Despite the large fluid volume infused with the HDMTX, eight pigs (19%) had evidence of AKI at conclusion of the infusion, with an average creatinine concentration increase of 0.41 mg/dL (±0.13 mg/dL). Five of these eight pigs developed severe AKI by 28 hours and only two survived to complete the study but with persistent severe AKI. The remaining three pigs developed moderate AKI and two had recovered before hour 148. Among the 26 pigs that developed AKI after hour 4 and conclusion of the first MTX infusion, at 28 hours eight had recovered from earlier evidence of AKI at one or more sampling times and 14 pigs had AKI of similar or worsening severity. The remaining four pigs developed AKI of mild severity after 28 hours (Figure 3).
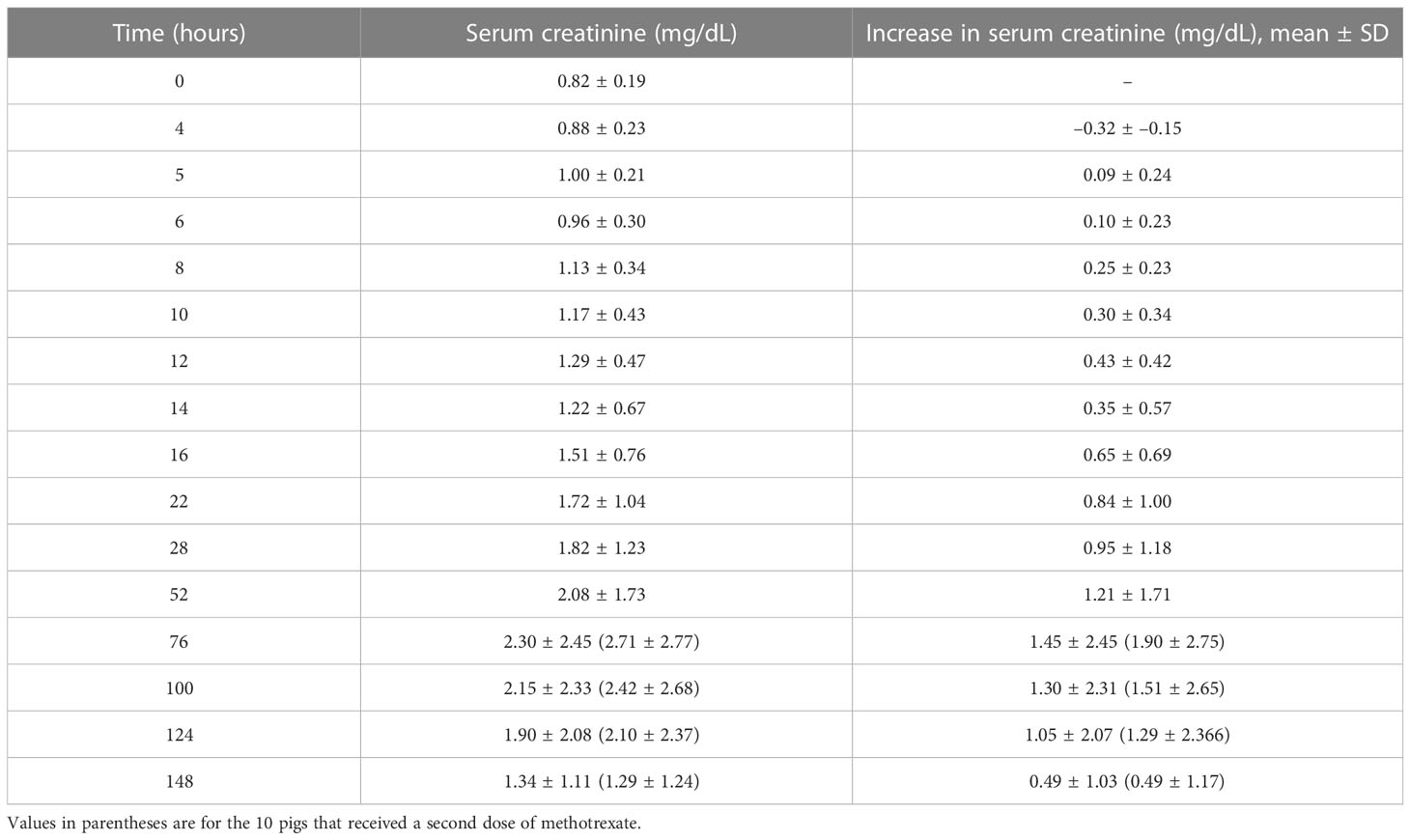
Table 2 Serum creatinine concentrations before and after the 4-hour infusion of methotrexate at 4 g/kg with the corresponding relative increases.
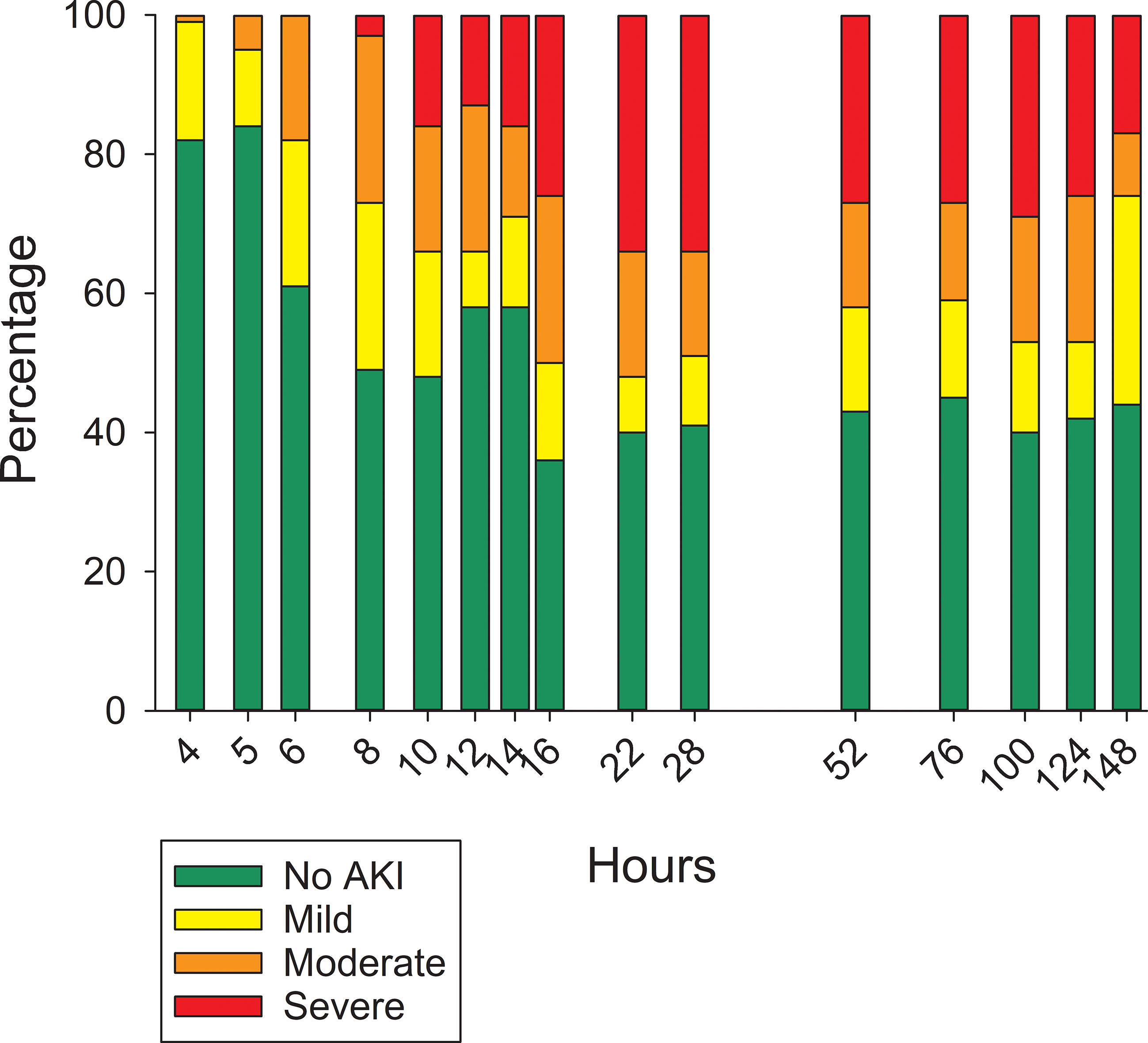
Figure 3 Percentage of pigs with varying severity of acute kidney injury at different time points after initiating the 4-hour infusion of methotrexate at 4 g/kg body weight.
Of the seven pigs with normal serum creatinine concentrations before starting the second dose of MTX at 76 hours, three developed AKI of moderate severity. The two pigs with mild and moderate AKI at the time of the second dose developed moderate and severe AKI, respectively. The pig with severe AKI at the time of the second dose continued to have severely compromised clearance until 124 hours, when it had moderate AKI, and by 148 hours it no longer had AKI based on serum creatinine concentration.
The percentage increases in serum creatinine concentration from baseline were calculated at 16, 28, and 52 hours after the start of the MTX infusion (Table 3). There was a 90%–120% increase in serum creatinine concentration for pigs with moderate AKI, defined as a 0.5 - 1.0 mg/dL increase in serum creatinine. Among the pigs with evidence of AKI at 16 hours, those with an increase in creatinine concentration of 50% or less compared with baseline did not have a further increase at 28 and 52 hours. Pigs with more than twofold increase in creatinine concentration at 16 hours had further increases in creatinine concentration at 28 hours. Pigs with severe AKI at 16 hours experienced even higher percentage increases at 28 hours and again at 52 hours, indicating that the severity of AKI continued to increase. This is consistent with the deaths of the pigs that had severe AKI at 16 hours.
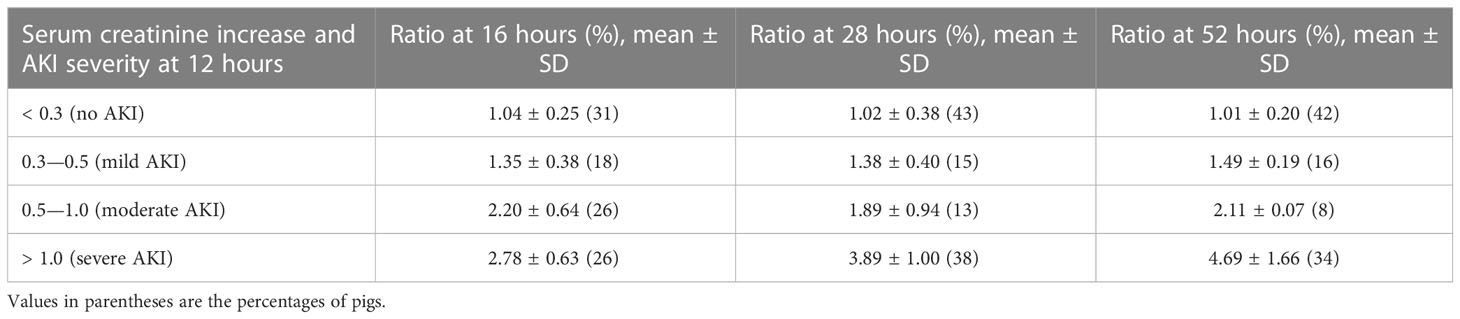
Table 3 Ratios for the increase in serum creatinine at 16, 28 and 52 hours compared with baseline for pigs with varying severities of acute kidney injury at 12 hours.
Increases in creatinine concentration at 6, 8, and 10 hours relative to 4 hours were not predictive of whether or not a pig would have AKI at 28 hours. However, the sum of creatine concentration increases at 6 and 8 hours from 4 hours was significantly higher for pigs with AKI at 28 hours (Figure 4), but did not identify pigs that would develop mild, moderate, or severe AKI. Including the increase in creatinine concentration at 10 hours did not improve predictability and, from a clinical perspective, would delay identifying pigs at risk of developing AKI that would benefit from early intervention to minimize renal damage and systemic toxicity.
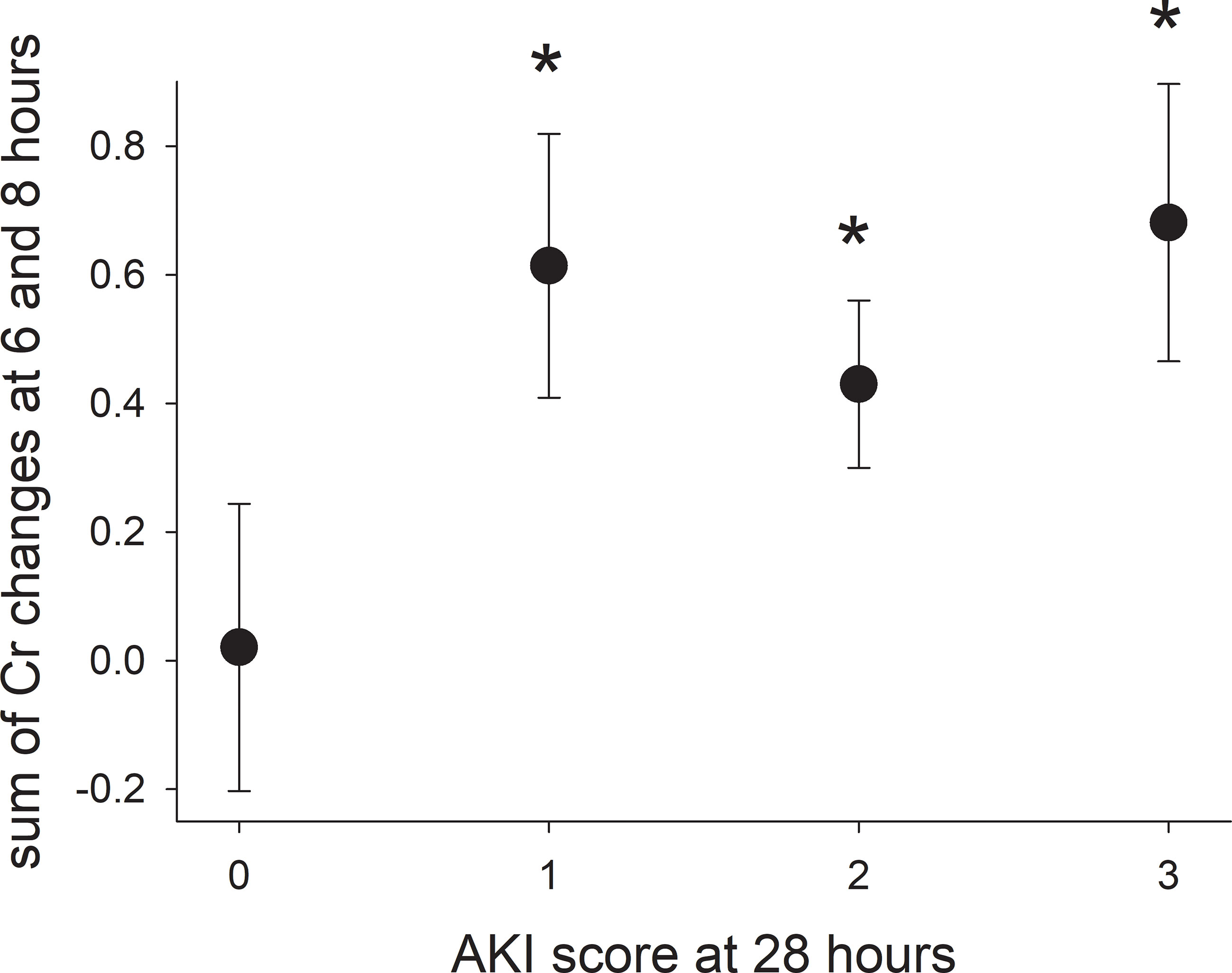
Figure 4 The sum of the increases in serum creatinine concentration at hours 6 and 8 (2 and 4 hours after ending the infusion) for pigs with varying severity of acute kidney injury. Asterisks indicate a significant difference (p-value < 0.05) compared with pigs without acute kidney injury (score of 0).
3.3 Serum methotrexate concentrations
There was rapid decline in serum MTX concentration during the first 24 hours after the infusion followed by a slower decline thereafter (Figure 5A), which is consistent with published two-compartment and three-compartment models of MTX elimination. At 52 hours, the mean serum MTX concentration was 28 μmol/L (± 27 μmol/L) and 81% of the pigs had serum MTX concentrations higher than the 1 μM concentration considered as evidence of delayed clearance among children receiving HDMTX. At 76 hours after the start of the HDMTX infusion, 18 (69%) out of 26 pigs had a serum MTX concentration > 0.1 μM, which is comparable to the 17 (61%) that had an elevated serum creatinine concentration indicative of AKI.
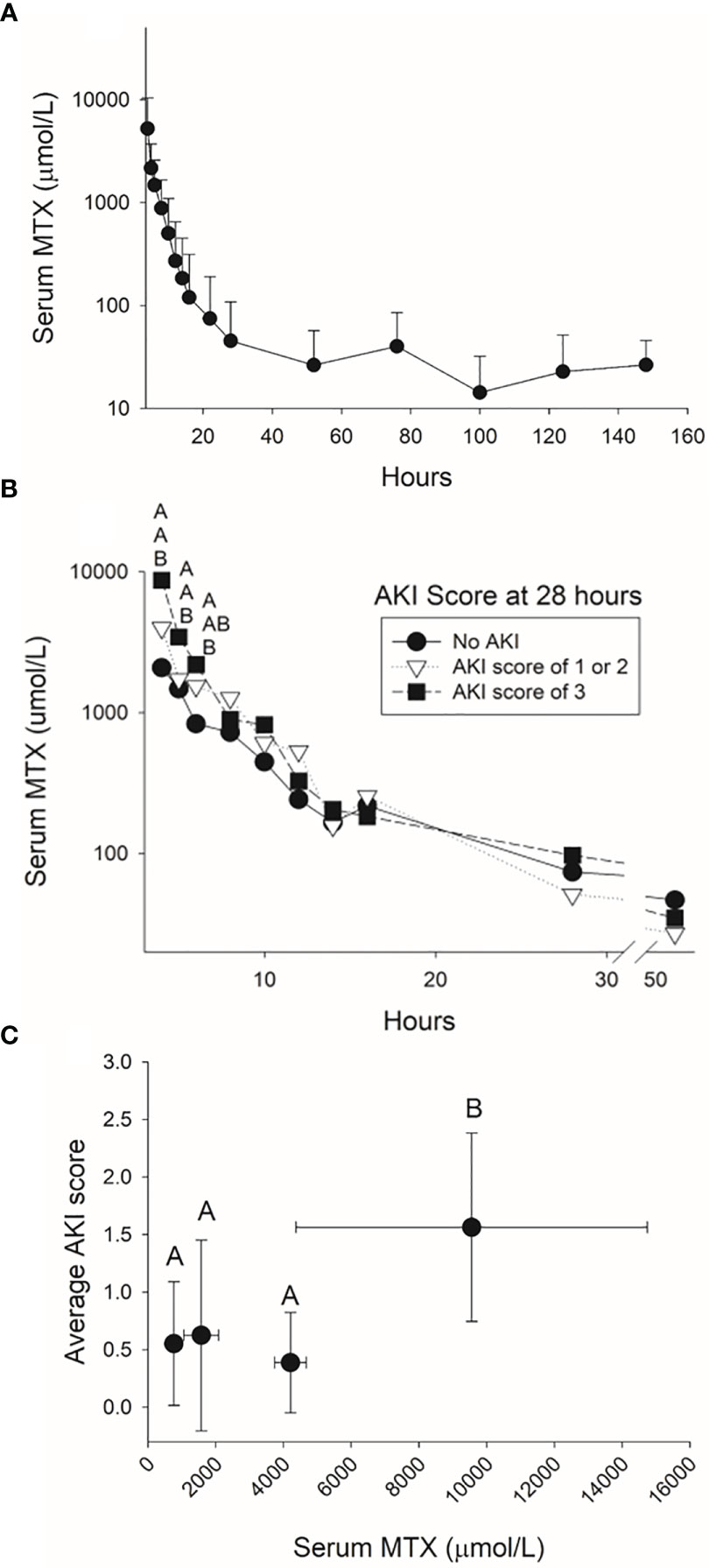
Figure 5 Serum methotrexate concentrations measured in the pig for 144 hours after the infusion of 4 g/kg of methotrexate (A) and for the first 48 hours after the infusion for pigs with varying severities of acute kidney injury at 28 hours (B). The average acute kidney injury score based on the increases in serum creatinine concentration during the 24 hours after completing the 4-hour methotrexate infusion (C) was higher when serum concentrations at the end of the infusion were higher than 5,000 µmol/L.
Pigs that developed mild, moderate, or severe AKI at 28 hours had significantly higher serum methotrexate concentrations at conclusion of the infusion (hour 4) than pigs without AKI (Figure 5B). For this model of HDMTX renal toxicity, serum MTX concentrations greater than 5,000 µM at conclusion of the infusion resulted in a higher AKI score averaged from the first 10 measurements of creatinine concentration after the infusion (Figure 5C).
The rate of MTX elimination during the initial 4 hours after the end of the infusion was associated with the presence of AKI at 24 hours. Specifically, pigs with serum MTX concentrations at hour 8 that were greater than 10% of the serum MTX concentration at conclusion of the infusion were at greater risk of AKI (Figure 6; p = 0.03; n = 38; OR 0.46; 95% CI 0.22–0.94). In line with this, pigs with severe AKI at 28 hours had cleared less MTX at hour 8. The percentage decreases of MTX concentration at hours 10 and 16 hours were less predictive (p-values of 0.14 and 0.23, respectively). Among the pigs given a second dose of MTX, the rate of decrease in serum MTX concentration during the 4 hours after the second infusion was slower in pigs with AKI at the time of dosing than in pigs without AKI, particularly when compared with pigs that did not develop AKI after the second dose.
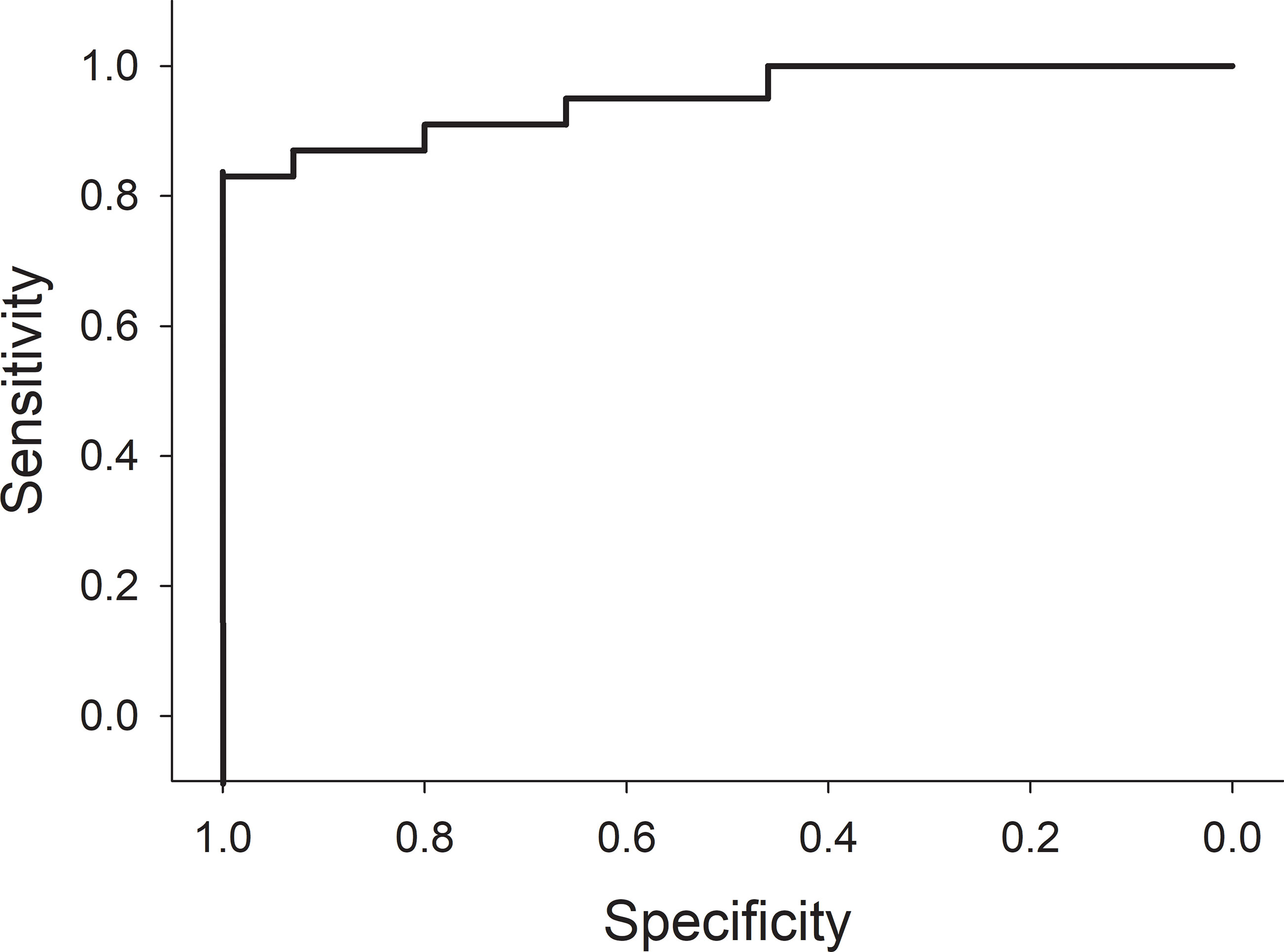
Figure 6 Receiver operator curve using a decrease of 90% or less in serum methotrexate levels within 4 hours from the time of completion of the 4-hour methotrexate infusion to predict acute kidney injury at 24 hours.
4 Discussion
Renal clearance is the major route for MTX elimination and the early identification of impaired MTX clearance is essential for beginning interventions to prevent the further deterioration of renal functions. It has been suggested that intrarenal precipitation of MTX is the leading pathological mechanism responsible for AKI in patients treated with high-dose MTX, especially when a very high MTX dose is infused over a few hours, as it is in patients treated for osteosarcomas. Hyperhydration and urine alkalinization are used as supportive care to prevent the formation of MTX crystals. However, the degree of alkalinization together with hydration volume and duration differs between cancer treatment protocols (30, 31). Some protocols increase the hydration volume in case of AKI, but it has never been confirmed if this prevents further deterioration of renal functions. It is also common to administer extra bicarbonate to patients with low urine pH during MTX infusion and it has even been suggested urine alkalinization alone can prevent MTX-induced AKI (32).
This study infused weaned pigs over 4 hours with a high volume (i.e., 250 mL/m2/h) of high-dose MTX (4 g/kg of MTX; equivalent to 80 g/m²) as a translational model for studying MTX-induced AKI. The 4 g/kg of MTX exceeded the 1–12 g/m² used in current cancer treatment protocols for leukemia and osteosarcoma, but it is similar to the 88 g/m2 administered to children with cancer (33, 34) and was infused without urine alkalinization to increase the risk of AKI. This approach caused AKI in 76% of the pigs within 28 hours after the start of the HDMTX infusion. The presence of MTX crystals in the kidneys of pigs that were euthanized before 52 hours suggests that this animal model is relevant to AKI in patients caused by infusions of HDMTX. Interestingly, no pigs had MTX crystals in their kidneys at autopsy 6 days after the HDMTX infusions. The high incidence of HDMTX-induced AKI among the pigs exceeds the rate of 2%–12% found in patients (35). The increases in serum creatinine concentration measured in the pigs mimic clinical data (36) and the 0.3 to 0.5 mg/dL increase for pigs with mild AKI is similar to the 1.5-fold increase for humans considered to have delayed elimination induced by HDMTX (37).
Different clinical parameters are considered to influence the risk of MTX-induced AKI. These include age, sex, genetics, serum total protein at the time of dosing, concomitant drugs, previous or subclinical episodes of AKI, and other unknown contributing factors (38–40). Variation among patients for these parameters confound clinical studies of MTX renal toxicity and complicate interpretations. These variables were minimized by using juvenile pigs of a similar age and body weight, and of the same genetic lineage that were given identical care and nutrition, and by not co-administering other chemotherapeutic agents. Still, there was variation among pigs in serum MTX concentrations, increases in serum creatinine concentration, and presence and severity of AKI. This replicates the unexplained variation in serum MTX concentrations and incidences and in severities of systemic toxicity and AKI among patients treated with HDMTX (10, 38), including pediatric patients treated with 33.6 g/m2 (33). The pig model provides an opportunity to identify factors that contribute to AKI risk and evaluate the efficacy of interventions.
4.1 Serum creatinine and AKI
The recovery of nearly 50% of the pigs from AKI mimics the potential of patients to regain normal renal clearance after MTX-induced injury (41, 42). However, only 4 of the 21 pigs (19%) that had moderate or severe AKI at or before 28 hours recovered before 148 hours. Of concern is that nephrons have a limited capacity for recovery and tubular dysfunction may persist for months (8). Any permanent loss of nephrons during HDMTX therapy depletes renal reserve capacity and increases the risk of AKI in subsequent HDMTX infusions and the future risk of chronic kidney disease. An unresolved question is what components of the nephron are capable of repair and recovery of functions. A strength of the pig model is the ability to address these questions and the need to understand the impact of HDMTX therapy during childhood on renal functions later in life (43). Of interest is how the cumulative increase in creatinine concentration during the initial hours after completion of the infusion may be an early determinant of AKI.
4.2 Serum MTX and AKI
The 4,751 µmol/L (+ 4,764 µmol/L) average MTX serum concentration at the end of the 4-hour HDMTX infusion exceeds the > 700 µM considered necessary for treating osteosarcoma (44). Only three pigs did not achieve this concentration at conclusion of the infusion. Methotrexate concentrations exceeded 1,500 µM at conclusion of the infusion for 69% of the pigs and five pigs had a serum MTX concentration greater than 10,000 µM. The wide variation in serum MTX concentrations and clearance among the pigs mimics the several-fold range of serum MTX concentrations and variable pharmacokinetics among pediatric patients [ (6, 45–47) (6, 46–48)]. The high MTX concentrations, especially without hyperhydration and urine alkalinization, increase the risk of toxicity and impaired renal clearance, and (10) certainly contributed to the high incidence of AKI among the pigs.
There was a clear association between serum MTX concentration at the end of infusion and risk of AKI within the first 28 hours after start of the HDMTX infusion. Concentrations of MTX at the end of the infusion that exceeded 5,000 µmol/L corresponded with higher AKI scores, particularly after hour 14. This highlights the importance of monitoring MTX steady-state concentrations during the infusion to adjust dosing, and, thereby, reduce toxicity and the incidence and severity of AKI (46). Of the seven pigs with MTX concentrations less than 1,000 µmol/L at the end of the infusion, four developed transient mild-to-moderate AKI during the first 28 hours, with one pig continuing to have moderate AKI at hours 28 and 52.
The majority of MTX is eliminated by glomerular filtration and renal tubular secretion (48, 49). Although glomerular filtration is responsible for the initial exponential decline in serum MTX concentration, tubular secretion is a saturable pathway with an increasing role in the elimination of MTX at lower concentrations (37). A novel finding from the dense sampling of the pigs after the infusion is that a slower exponential decline in serum MTX concentration during the first 4 hours after the infusion is a sensitive and early indicator of impaired renal clearance and increased risk of AKI. This has not been previously recognized because pharmacokinetic modeling of MTX clearance has relied largely on samples collected from patients at or after 24 hours to identify patients with delayed elimination and renal damage (50). This approach has had limited success predicting impending renal injury, impaired clearance, and systemic toxicity (45, 51, 52). Samples collected 6 hours after ending the infusion (44) may still not be early enough to characterize the steep exponential decline in serum MTX concentration that occurs during the initial hours after the infusion (44, 53). Our findings with the pig model indicate that the initial exponential decline in MTX concentration can be used in monitoring of serum MTX concentration during the infusion for earlier identification of patients with delayed elimination and renal damage.
The half-life of serum MTX during the first 24 hours after dosing has been estimated at about 150 min (42). In the present study, the hour 5 serum MTX concentrations averaged 60% (±30%) of those measured at the end of administration (hour 4) and at hour 6 were 37% (±20%), implying that the half-life during the initial 2 hours after the infusion is shorter than 2 hours. The shorter half-life may reflect the high initial concentrations (33). The reduction in MTX clearance, hence longer half-life, and increase in serum creatinine during the first 4 hours after the infusion is consistent with MTX crystals obstructing nephron tubules and decreasing glomerular filtration without an apparent relationship with renal blood flow (54).
When sufficient numbers of nephrons are compromised and filtration capacity is diminished, the ability to rapidly clear MTX, creatinine, and other filtered molecules declines (55). This prolongs the exposure of the renal tubular epithelium to toxic concentrations of MTX, which can compromise tubule transport and the elimination of toxins and other drugs (56). The strategy of hyperhydration and urine alkalinization increases MTX solubility in urine, but it does not prevent the formation of MTX crystals in the nephrons (57), even without high serum concentrations (11), nor does it enhance filtration by remaining glomeruli. Although high-dose leucovorin rescue addresses the systemic toxicity for patients with MTX nephrotoxicity and reduced clearance, there is little or no evidence that this intervention reduces MTX concentrations in the nephrons (58). The pig model can be used to evaluate interventions that preserve glomerular filtration and tubular secretion, minimize renal and systemic damage, and will enhance renal recovery from current and future therapy regimens.
The presence of MTX in the feces of the pigs corroborates the presence of hepatic clearance associated with biliary secretion (59). This alternative pathway of elimination is responsible for 10%–30% of MTX elimination and is mediated by transporters in the intestine and liver (60, 61). Hepatotoxicity associated with HDMTX (62) has the potential to limit this pathway of elimination.
4.3 Identifying patients at risk of MTX-induced renal injury—a new paradigm
Identifying factors that increase the risk of HDMTX-induced renal damage is of paramount importance. The variations in serum MTX concentration and AKI, despite the minimization of known contributing variables in this experimental model, highlights the need for new biomarkers of delayed elimination of MTX and development of AKI. In this regard, the rate of decline in MTX concentrations during the first 4 hours after completion of the infusion (hours 4 through 8 in this model) was highly predictive of development of AKI, with a receiver operator curve that has large areas with either perfect sensitivity or perfect specificity (Figure 6). The half-life of MTX in juvenile pigs is approximately 1.3 hours, so the elimination of 90% of MTX would be expected in 4 hours (slightly more than three half-lives). Indeed, all animals with lower rates of MTX elimination than 90% during the first 4 hours after stopping the infusion developed AKI. This leads to a new paradigm for early detection of delayed MTX elimination that is (1) based on very early measurements when renal protective measures, such as increased hyperhydration, more aggressive urine alkalinization and glucarpidase will be most beneficial; (2) based on the rate of MTX elimination rather than any single level taken at a single time point, which serves as a surrogate marker of decreased glomerular filtration rate (GFR), which can be measured in real time without waiting for the rise in creatinine concentration, which will inevitably occur 1–2 days after renal injury; and (3) independent of the MTX dose, duration of infusion, and patient characteristics, as the rate of decrease of MTX concentration during the first 4 hours reflects GFR during those 4 hours and obviates the need for complex nomograms and single-time-point thresholds that differ based on MTX dose, MTX infusion duration, time from the start of MTX infusion, patient sex, and serum creatinine concentration (Figure 7).
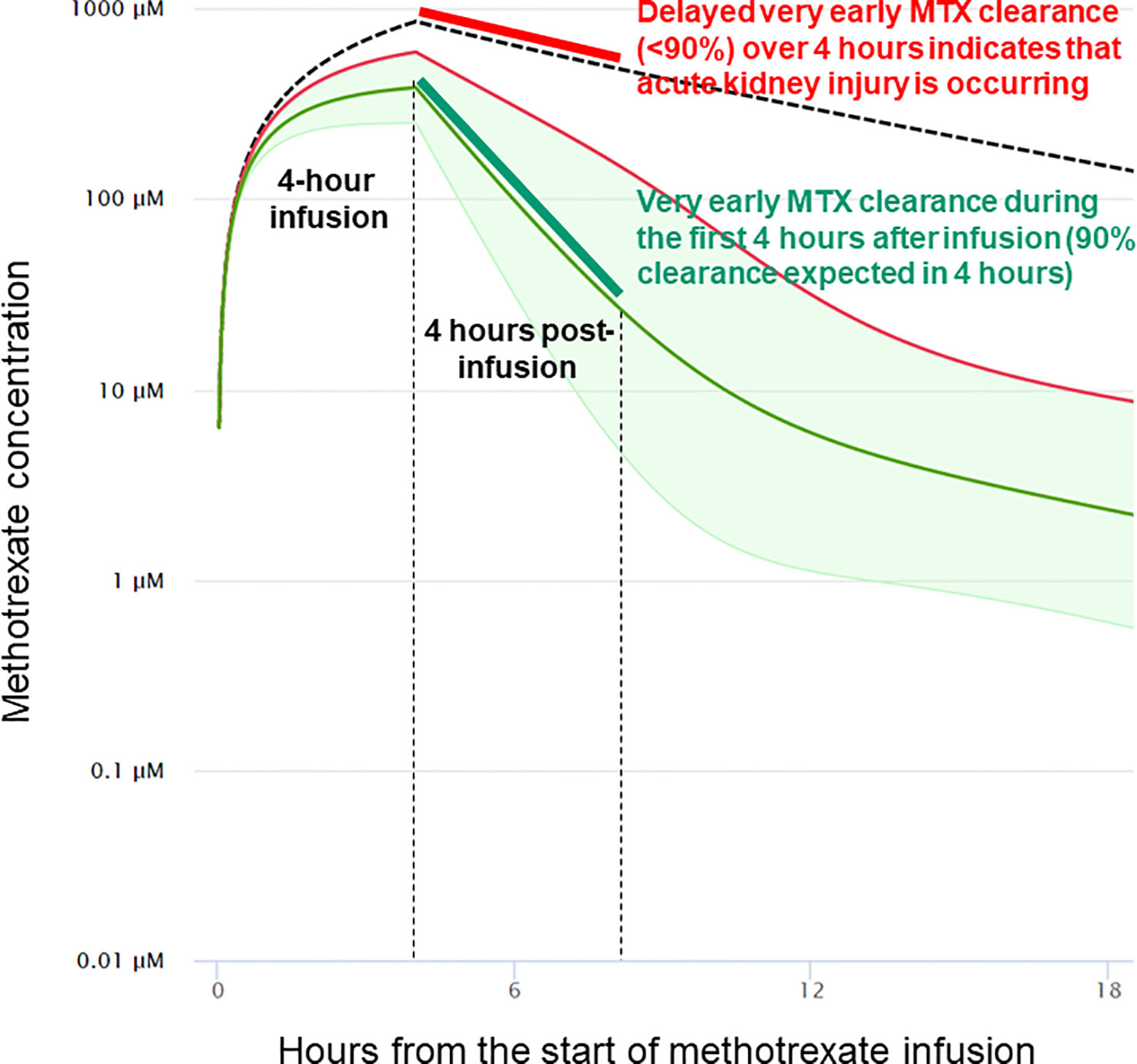
Figure 7 Impact of very early clearance of methotrexate on the probability of delayed elimination and acute kidney injury. In this example from models validated in humans, the 4-hour methotrexate clearance analyzed in the pig model is estimated to accurately predict methotrexate clearance and acute kidney injury at 24 and 48 hours from the start of the methotrexate infusion. The model used is taken from Resonance Study Manager (ResonanceHealth.org), which uses the validated model at MTXPK.org.
Renal clearance varies among individual humans and pigs owing to differences in numbers of functional nephrons (63) that can originate during nephrogenesis (64, 65). Corresponding with this, the normal decline in nephron numbers that occurs with aging (66) coincides with the greater risk in adults than in children of delayed MTX clearance and higher peak serum creatinine concentration, even at lower MTX doses (67). There is growing interest in pharmacogenomics for identifying genes and gene products that contribute to MTX clearance (53, 68). These efforts combined with metabolomic analysis of urine samples at the start and conclusion of MTX therapy (16) may reveal genetic factors that are predictive of risks.
5 Conclusions
Weaned juvenile pigs given 4-hour HDMTX infusions represent a valuable preclinical model for MTX-induced AKI and can be used to study biological, environmental, and iatrogenic factors that increase the risk of AKI. This includes administration of pantoprazole (69), cisplatin, and other conventional chemotherapeutic agents that are nephrotoxic (70, 71) and could increase the incidence and severity of AKI caused by HDMTX. Other drug–HDMTX combinations can be evaluated as potential risk factors for increased incidence and severity of AKI in pediatric patients (72, 73). This preclinical animal model can potentially be used to evaluate how the current supportive care with hyperhydration and urine alkalization can be optimized. Furthermore, the model can be used to identify new biomarkers and protocols for early identification of reduced renal clearance and to study the underlying pathophysiological mechanisms of MTX-induced AKI. Once validated in humans through measurement of MTX at two early time points (0 and 4 hours after completing the infusion), the rate of MTX elimination during this time should be the only biomarker needed for very early detection of potential AKI. The model needs to be extended to include responses to 24-hour infusion. Early mitigation using increased hyperhydration, alkalinization, and glucarpidase will prevent extensive and permanent renal dysfunction, reduce the systemic MTX toxicity, and lower the costs associated with delaying treatment (69).
Data availability statement
The original contributions presented in the study are included in the article/supplementary material. Further inquiries can be directed to the corresponding author.
Ethics statement
The animal study was reviewed and approved by the University of Memphis Institutional Animal Care and Use Committee.
Author contributions
Conceptualization and funding acquisition: RB and SH. Methodology: RB, SH, and TW. Conduct of study phases using animals: RB, KB, and TW. Sample analysis: RB and TW. Data analysis and interpretation: RB, SH, TM, and XC. Preparation of the manuscript: RB, SH, and TM. All authors contributed to the article and approved the submitted version.
Funding
Funding for the research described herein was provided by BTG.
Acknowledgments
We want to thank Donny Ray and the numerous animal care staff and research assistants who participated in the animals’ care, handling, surgeries and postoperative care, and the collection of samples.
Conflict of interest
The authors declare that the research was conducted in the absence of any commercial or financial relationships that could be construed as a potential conflict of interest.
Publisher’s note
All claims expressed in this article are solely those of the authors and do not necessarily represent those of their affiliated organizations, or those of the publisher, the editors and the reviewers. Any product that may be evaluated in this article, or claim that may be made by its manufacturer, is not guaranteed or endorsed by the publisher.
References
1. Howard SC, McCormick J, Pui CH, Buddington RK, Harvey RD. Preventing and managing toxicities of high-dose methotrexate. Oncologist (2016) 21(12):1471–82. doi: 10.1634/theoncologist.2015-0164
2. Boelens AD, Mathôt RAA, Vlaar APJ, Bouman CSC. Glucarpidase treatment for methotrexate intoxication: a case report and review of the literature. Neth J Med (2018) 76(1):36–9.
3. Mallipattu SK, Ross MJ. Methotrexate in the urine. Kidney Int (2011) 80(2):226. doi: 10.1038/ki.2011.97
4. Jacobs SA, Stoller RG, Chabner BA, Johns DG. Dose-dependent metabolism of methotrexate in man and rhesus monkeys. Cancer Treat Rep (1977) 61(4):651–6.
5. Sand TE, Jacobsen S. Effect of urine pH and flow on renal clearance of methotrexate. Eur J Clin Pharmacol (1981) 19(6):453–6. doi: 10.1007/BF00548590
6. Mikkelsen TS, Mamoudou AD, Tuckuviene R, Wehner PS, Schroeder H. Extended duration of prehydration does not prevent nephrotoxicity or delayed drug elimination in high-dose methotrexate infusions: a prospectively randomized cross-over study. Pediatr Blood Cancer (2014) 61(2):297–301. doi: 10.1002/pbc.24623
7. Christensen AM, Pauley JL, Molinelli AR, Panetta JC, Ward DA, Stewart CF, et al. Resumption of high-dose methotrexate after acute kidney injury and glucarpidase use in pediatric oncology patients. Cancer (2012) 118(17):4321–30. doi: 10.1002/cncr.27378
8. Ragab SM, Badr EA. Evaluation of serum and urine fetuin-a levels in children with acute lymphoblastic leukemia during and after high-dose methotrexate therapy: relation to toxicity. Hematology (2016) 21(2):78–91. doi: 10.1179/1607845415Y.0000000042
9. Xu WQ, Zhang LY, Chen XY, Pan BH, Mao JQ, Song H, et al. Serum creatinine and creatinine clearance for predicting plasma methotrexate concentrations after high-dose methotrexate chemotherapy for the treatment for childhood lymphoblastic malignancies. Cancer Chemother Pharmacol (2014) 73(1):79–86. doi: 10.1007/s00280-013-2319-2
10. Yang Y, Wang X, Tian J, Wang Z. Renal function and plasma methotrexate concentrations predict toxicities in adults receiving high-dose methotrexate. Med Sci Monit (2018) 24:7719–26. doi: 10.12659/MSM.912999
11. Garneau AP, Riopel J, Isenring P. Acute methotrexate-induced crystal nephropathy. N Engl J Med (2015) 373(27):2691–3. doi: 10.1056/NEJMc1507547
12. Ostermann M, Joannidis M. Acute kidney injury 2016: diagnosis and diagnostic workup. Crit Care (2016) 20(1):299. doi: 10.1186/s13054-016-1478-z
13. Wasung ME, Chawla LS, Madero M. Biomarkers of renal function, which and when? Clin Chim Acta (2015) 438:350–7. doi: 10.1016/j.cca.2014.08.039
14. Barreto JN, McClanahan AL, Rule AD, Thompson CA, Frazee E. Incorporating cystatin c to predict methotrexate elimination in patients with CNS lymphoma and suspicious renal function. Case Rep Hematol (2018) 2018:7169897. doi: 10.1155/2018/7169897
15. Gu L, Shi H, Zhang R, Wei Z, Bi KS, Chen XH. Simultaneous determination of five specific and sensitive nephrotoxicity biomarkers in serum and urine samples of four drug-induced kidney injury models. J Chromatogr Sci (2017) 55(1):60–8. doi: 10.1093/chromsci/bmw150
16. Muhrez K, Benz-de Bretagne I, Nadal-Desbarats L, Blasco H, Gyan E, Choquet S, et al. Endogenous metabolites that are substrates of organic anion transporter's (OATs) predict methotrexate clearance. Pharmacol Res (2017) 118:121–32. doi: 10.1016/j.phrs.2016.05.021
17. Severin MJ, Campagno RV, Brandoni A, Torres AM. Time evolution of methotrexate-induced kidney injury: a comparative study between different biomarkers of renal damage in rats. Clin Exp Pharmacol Physiol (2019) 46(9):828–36. doi: 10.1111/1440-1681.13122
18. Ylinen E, Jahnukainen K, Saarinen-Pihkala UM, Jahnukainen T. Assessment of renal function during high-dose methotrexate treatment in children with acute lymphoblastic leukemia. Pediatr Blood Cancer (2014) 61(12):2199–202. doi: 10.1002/pbc.25137
19. Ramsey LB, Balis FM, O'Brien MM, Schmiegelow K, Pauley JL, Bleyer A, et al. Consensus guideline for use of glucarpidase in patients with high-dose methotrexate induced acute kidney injury and delayed methotrexate clearance. Oncologist (2018) 23(1):52–61. doi: 10.1634/theoncologist.2017-0243
20. Svahn T, Mellgren K, Harila-Saari A, Åsberg A, Kanerva J, Jónsson Ó, et al. Delayed elimination of high-dose methotrexate and use of carboxypeptidase G2 in pediatric patients during treatment for acute lymphoblastic leukemia. Pediatr Blood Cancer (2017) 64(7). doi: 10.1002/pbc.26395
21. Prémaud A, Rousseau A, Gicquel M, Ragot S, Manceau J, Laurentie M, et al. An animal model for the study of chronopharmacokinetics of drugs and application to methotrexate and vinorelbine. Toxicol Appl Pharmacol (2002) 183(3):189–97. doi: 10.1006/taap.2002.9467
22. Sandberg DI, Solano J, Petito CK, Mian A, Mou C, Koru-Sengul T, et al. Safety and pharmacokinetic analysis of methotrexate administered directly into the fourth ventricle in a piglet model. J Neurooncol (2010) 100(3):397–406. doi: 10.1007/s11060-010-0210-0
23. Cermanová J, Chládek J, Sispera L, Martínková J. [Renal excretion of methotrexate in an in vivo model in minipigs]. Acta Med (Hradec Kralove) Suppl (2000) 43(2):139–47.
24. Martin J, Howard SC, Pillai A, Vogel P, Naren AP, Davis S, et al. The weaned pig as a model for doxorubicin-induced mucositis. Chemotherapy (2014) 60(1):24–36. doi: 10.1159/000365725
25. Sangild PT, Shen RL, Pontoppidan P, Rathe M. Animal models of chemotherapy-induced mucositis: translational relevance and challenges. Am J Physiol Gastrointest Liver Physiol (2018) 314(2):G231–g46. doi: 10.1152/ajpgi.00204.2017
26. Wiczer T, Dotson E, Tuten A, Phillips G, Maddocks K. Evaluation of incidence and risk factors for high-dose methotrexate-induced nephrotoxicity. J Oncol Pharm Pract (2016) 22(3):430–6. doi: 10.1177/1078155215594417
27. Widemann BC, Balis FM, Adamson PC. Dihydrofolate reductase enzyme inhibition assay for plasma methotrexate determination using a 96-well microplate reader. Clin Chem (1999) 45(2):223–8. doi: 10.1093/clinchem/45.2.223
28. Handa RK, Lingeman JE, Bledsoe SB, Evan AP, Connors BA, Johnson CD. Intraluminal measurement of papillary duct urine pH, in vivo: a pilot study in the swine kidney. Urolithiasis (2016) 44(3):211–7. doi: 10.1007/s00240-015-0834-9
29. Moëll C, Garwicz S. High-dose methotrexate causes short-term suppression of growth in rabbits. Acta Paediatr (1995) 84(11):1237–40. doi: 10.1111/j.1651-2227.1995.tb13540.x
30. Mikkelsen TS, Sparreboom A, Cheng C, Zhou Y, Boyett JM, Raimondi SC, et al. Shortening infusion time for high-dose methotrexate alters antileukemic effects: a randomized prospective clinical trial. J Clin Oncol (2011) 29(13):1771–8. doi: 10.1200/JCO.2010.32.5340
31. Schmiegelow K, Attarbaschi A, Barzilai S, Escherich G, Frandsen TL, Halsey C, et al. Consensus definitions of 14 severe acute toxic effects for childhood lymphoblastic leukaemia treatment: a Delphi consensus. Lancet Oncol (2016) 17(6):e231–e9. doi: 10.1016/S1470-2045(16)30035-3
32. Mir O, Ropert S, Babinet A, Alexandre J, Larousserie F, Durand JP, et al. Hyper-alkalinization without hyper-hydration for the prevention of high-dose methotrexate acute nephrotoxicity in patients with osteosarcoma. Cancer Chemother Pharmacol (2010) 66(6):1059–63. doi: 10.1007/s00280-010-1259-3
33. Borsi JD, Moe PJ. A comparative study on the pharmacokinetics of methotrexate in a dose range of 0.5 g to 33.6 g/m2 in children with acute lymphoblastic leukemia. Cancer (1987) 60(1):5–13. doi: 10.1002/1097-0142(19870701)60:1<5::aid-cncr2820600103>3.0.co;2-d
34. Nathan PC, Whitcomb T, Wolters PL, Steinberg SM, Balis FM, Brouwers P, et al. Very high-dose methotrexate (33.6 g/m(2)) as central nervous system preventive therapy for childhood acute lymphoblastic leukemia: results of national cancer Institute/Children's cancer group trials CCG-191P, CCG-134P and CCG-144P. Leuk Lymphoma (2006) 47(12):2488–504. doi: 10.1080/10428190600942769
35. Schmidt JA, Rinaldi S, Scalbert A, Ferrari P, Achaintre D, Gunter MJ, et al. Plasma concentrations and intakes of amino acids in male meat-eaters, fish-eaters, vegetarians and vegans: a cross-sectional analysis in the EPIC-Oxford cohort. Eur J Clin Nutr (2016) 70(3):306–12. doi: 10.1038/ejcn.2015.144
36. Tiwari P, Thomas MK, Pathania S, Dhawan D, Gupta YK, Vishnubhatla S, et al. Serum creatinine versus plasma methotrexate levels to predict toxicities in children receiving high-dose methotrexate. Pediatr Hematol Oncol (2015) 32(8):576–84. doi: 10.3109/08880018.2015.1087612
37. Skärby T, Jönsson P, Hjorth L, Behrentz M, Björk O, Forestier E, et al. High-dose methotrexate: on the relationship of methotrexate elimination time vs renal function and serum methotrexate levels in 1164 courses in 264 Swedish children with acute lymphoblastic leukaemia (ALL). Cancer Chemother Pharmacol (2003) 51(4):311–20. doi: 10.1007/s00280-002-0552-1
38. Al-Turkmani MR, Law T, Narla A, Kellogg MD. Difficulty measuring methotrexate in a patient with high-dose methotrexate-induced nephrotoxicity. Clin Chem (2010) 56(12):1792–4. doi: 10.1373/clinchem.2010.144824
39. Cheng DH, Lu H, Liu TT, Zou XQ, Pang HM. Identification of risk factors in high-dose methotrexate-induced acute kidney injury in childhood acute lymphoblastic leukemia. Chemotherapy (2018) 63(2):101–7. doi: 10.1159/000486823
40. Yetgin S, Olgar S, Aras T, Cetin M, Düzova A, Beylergil V, et al. Evaluation of kidney damage in patients with acute lymphoblastic leukemia in long-term follow-up: value of renal scan. Am J Hematol (2004) 77(2):132–9. doi: 10.1002/ajh.20146
41. Harms J, Khawaja A, Taylor M, Han X, Mrug M. Recovery of methotrexate-induced anuric acute kidney injury after glucarpidase therapy. SAGE Open Med Case Rep (2017) 5. doi: 10.1177/2050313X17705050
42. Hempel L, Misselwitz J, Fleck C, Kentouche K, Leder C, Appenroth D, et al. Influence of high-dose methotrexate therapy (HD-MTX) on glomerular and tubular kidney function. Med Pediatr Oncol (2003) 40(6):348–54. doi: 10.1002/mpo.10293
43. Grönroos MH, Jahnukainen T, Möttönen M, Perkkiö M, Irjala K, Salmi TT. Long-term follow-up of renal function after high-dose methotrexate treatment in children. Pediatr Blood Cancer (2008) 51(4):535–9. doi: 10.1002/pbc.21650
44. Colom H, Farré R, Soy D, Peraire C, Cendros JM, Pardo N, et al. Population pharmacokinetics of high-dose methotrexate after intravenous administration in pediatric patients with osteosarcoma. Ther Drug Monit (2009) 31(1):76–85. doi: 10.1097/FTD.0b013e3181945624
45. Aumente D, Buelga DS, Lukas JC, Gomez P, Torres A, García MJ. Population pharmacokinetics of high-dose methotrexate in children with acute lymphoblastic leukaemia. Clin Pharmacokinet (2006) 45(12):1227–38. doi: 10.2165/00003088-200645120-00007
46. Foster JH, Thompson PA, Bernhardt MB, Margolin JF, Hilsenbeck SG, Jo E, et al. A prospective study of a simple algorithm to individually dose high-dose methotrexate for children with leukemia at risk for methotrexate toxicities. Cancer Chemother Pharmacol (2019) 83(2):349–60. doi: 10.1007/s00280-018-3733-2
47. Seidel H, Andersen A, Kvaløy JT, Nygaard R, Moe PJ, Jacobsen G, et al. Variability in methotrexate serum and cerebrospinal fluid pharmacokinetics in children with acute lymphocytic leukemia: relation to assay methodology and physiological variables. Leuk Res (2000) 24(3):193–9. doi: 10.1016/S0145-2126(99)00181-2
48. Ogungbenro K, Aarons L. Physiologically based pharmacokinetic modelling of methotrexate and 6-mercaptopurine in adults and children. part 1: methotrexate. J Pharmacokinet Pharmacodyn (2014) 41(2):159–71. doi: 10.1080/10428190600942769
49. Perazella MA. Drug-induced acute kidney injury: diverse mechanisms of tubular injury. Curr Opin Crit Care (2019) 25(6):550–7. doi: 10.1097/MCC.0000000000000653
50. Borsi JD, Sagen E, Romslo I, Moe PJ. Pharmacokinetics and metabolism of methotrexate: an example for the use of clinical pharmacology in pediatric oncology. Pediatr Hematol Oncol (1990) 7(1):13–33. doi: 10.3109/08880019009034317
51. Li X, Sui Z, Jing F, Xu W, Li X, Guo Q, et al. Identifying risk factors for high-dose methotrexate-induced toxicities in children with acute lymphoblastic leukemia. Cancer Manag Res (2019) 11:6265–74. doi: 10.2147/CMAR.S207959
52. Mei S, Li X, Jiang X, Yu K, Lin S, Zhao Z. Population pharmacokinetics of high-dose methotrexate in patients with primary central nervous system lymphoma. J Pharm Sci (2018) 107(5):1454–60. doi: 10.1016/j.xphs.2018.01.004
53. Lui G, Treluyer JM, Fresneau B, Piperno-Neumann S, Gaspar N, Corradini N, et al. A pharmacokinetic and pharmacogenetic analysis of osteosarcoma patients treated with high-dose methotrexate: data from the OS2006/Sarcoma-09 trial. J Clin Pharmacol (2018) 58(12):1541–9. doi: 10.1002/jcph.1252
54. Prowle JR, Ishikawa K, May CN, Bellomo R. Renal plasma flow and glomerular filtration rate during acute kidney injury in man. Ren Fail (2010) 32(3):349–55. doi: 10.3109/08860221003611695
55. Koren G. The nephrotoxic potential of drugs and chemicals. pharmacological basis and clinical relevance. Med Toxicol Adverse Drug Exp (1989) 4(1):59–72. doi: 10.1007/bf03259903
56. Takeuchi A, Masuda S, Saito H, Doi T, Inui K. Role of kidney-specific organic anion transporters in the urinary excretion of methotrexate. Kidney Int (2001) 60(3):1058–68. doi: 10.1046/j.1523-1755.2001.0600031058.x
57. Luciano RL, Perazella MA. Crystalline-induced kidney disease: a case for urine microscopy. Clin Kidney J (2015) 8(2):131–6. doi: 10.1093/ckj/sfu105
58. Flombaum CD, Liu D, Yan SQ, Chan A, Mathew S, Meyers PA, et al. Management of patients with acute methotrexate nephrotoxicity with high-dose leucovorin. Pharmacotherapy (2018) 38(7):714–24. doi: 10.1002/phar.2145
59. Creaven PJ, Hansen HH, Alford DA, Allen LM. Methotrexate in liver and bile after intravenous dosage in man. Br J Cancer (1973) 28(6):589–91. doi: 10.1038/bjc.1973.190
60. Lohitnavy M, Yasong L, Lohitnavy O, Yang RSH. A physiologically-based pharmacokinetic model of methotrexate incorporating hepatic excretion via multidrug-resistance-associated protein 2 (Mrp2) in mice, rats, dogs, and humans. Annu Int Conf IEEE Eng Med Biol Soc (2017) 2017:2728–31. doi: 10.1109/EMBC.2017.8037421
61. Vlaming ML, van Esch A, Pala Z, Wagenaar E, van de Wetering K, van Tellingen O, et al. Abcc2 (Mrp2), Abcc3 (Mrp3), and Abcg2 (Bcrp1) are the main determinants for rapid elimination of methotrexate and its toxic metabolite 7-hydroxymethotrexate in vivo. Mol Cancer Ther (2009) 8(12):3350–9. doi: 10.1158/1535-7163.MCT-09-0668
62. Özdemir ZC, Bozkurt Turhan A, Düzenli Kar Y, Bör Ö. The frequency of hepatotoxicity and myelotoxicity in leukemic children with different high doses of methotrexate. Int J Pediatr Adolesc Med (2016) 3(4):162–8. doi: 10.1016/j.ijpam.2016.08.008
63. Puelles VG, Hoy WE, Hughson MD, Diouf B, Douglas-Denton RN, Bertram JF. Glomerular number and size variability and risk for kidney disease. Curr Opin Nephrol Hypertens (2011) 20(1):7–15. doi: 10.1097/MNH.0b013e3283410a7d
64. Fanni D, Gerosa C, Nemolato S, Mocci C, Pichiri G, Coni P, et al. "Physiological" renal regenerating medicine in VLBW preterm infants: could a dream come true? J Matern Fetal Neonatal Med (2012) 25 Suppl 3:41–8. doi: 10.3109/14767058.2012.712339
65. Ryan D, Sutherland MR, Flores TJ, Kent AL, Dahlstrom JE, Puelles VG, et al. Development of the human fetal kidney from mid to late gestation in Male and female infants. EBioMedicine (2018) 27:275–83. doi: 10.1016/j.ebiom.2017.12.016
66. Hommos MS, Glassock RJ, Rule AD. Structural and functional changes in human kidneys with healthy aging. J Am Soc Nephrol (2017) 28(10):2838–44. doi: 10.1681/ASN.2017040421
67. Wippel B, Gundle KR, Dang T, Paxton J, Bubalo J, Stork L, et al. Safety and efficacy of high-dose methotrexate for osteosarcoma in adolescents compared with young adults. Cancer Med (2019) 8(1):111–6. doi: 10.1002/cam4.1898
68. Hegyi M, Arany A, Semsei AF, Csordas K, Eipel O, Gezsi A, et al. Pharmacogenetic analysis of high-dose methotrexate treatment in children with osteosarcoma. Oncotarget (2017) 8(6):9388–98. doi: 10.18632/oncotarget.11543
69. Ranchon F, Vantard N, Henin E, Bachy E, Sarkozy C, Karlin L, et al. Delayed methotrexate elimination: incidence, interaction with antacid drugs, and clinical consequences? Hematol Oncol (2018) 36(2):399–406. doi: 10.1002/hon.2479
70. ?>Gupta S, Portales-Castillo I, Daher A, Kitchlu A. Conventional chemotherapy nephrotoxicity. Adv Chronic Kidney Dis (2021) 28(5):402–14.e1. doi: 10.1053/j.ackd.2021.08.001
71. Mahmoud AAS, Elsalam HBA, El-Deeb SM, Zanaty FM, Aboelghar HM, Elharoun MS. Evaluation of kidney dysfunction in childhood cancer survivors. Pediatr Res (2022) 92(6):1689–94. doi: 10.1038/s41390-022-02015-w
72. Pommert L, Liberio N, Ng JS, Egelund TA, Siver MJ, Katzenstein HM, et al. Concurrent imatinib dosing with high-dose methotrexate leads to acute kidney injury and delayed methotrexate clearance in pediatric patients with Philadelphia chromosome-positive b-cell acute lymphoblastic leukemia. J Pediatr Hematol Oncol (2021) 43(2):e296–300. doi: 10.1097/MPH.0000000000001816
Keywords: methotrexate, creatinine, acute kidney injury, chemotherapy, pig, animal model, clearance
Citation: Buddington RK, Wong T, Buddington KK, Mikkelsen TS, Cao X and Howard SC (2023) Early clinical indicators of acute kidney injury caused by administering high-dose methotrexate therapy to juvenile pigs. Front. Nephrol. 3:1193494. doi: 10.3389/fneph.2023.1193494
Received: 24 March 2023; Accepted: 08 June 2023;
Published: 12 September 2023.
Edited by:
Jerzy Chudek, Medical University of Silesia, PolandReviewed by:
Winfried Alsdorf, University Medical Center Hamburg-Eppendorf, GermanyMara Medeiros, Federico Gómez Children’s Hospital, Mexico
Copyright © 2023 Buddington, Wong, Buddington, Mikkelsen, Cao and Howard. This is an open-access article distributed under the terms of the Creative Commons Attribution License (CC BY). The use, distribution or reproduction in other forums is permitted, provided the original author(s) and the copyright owner(s) are credited and that the original publication in this journal is cited, in accordance with accepted academic practice. No use, distribution or reproduction is permitted which does not comply with these terms.
*Correspondence: Randal K. Buddington, cmFuZGFsLmJ1ZGRpbmd0b25AbHN1aHMuZWR1