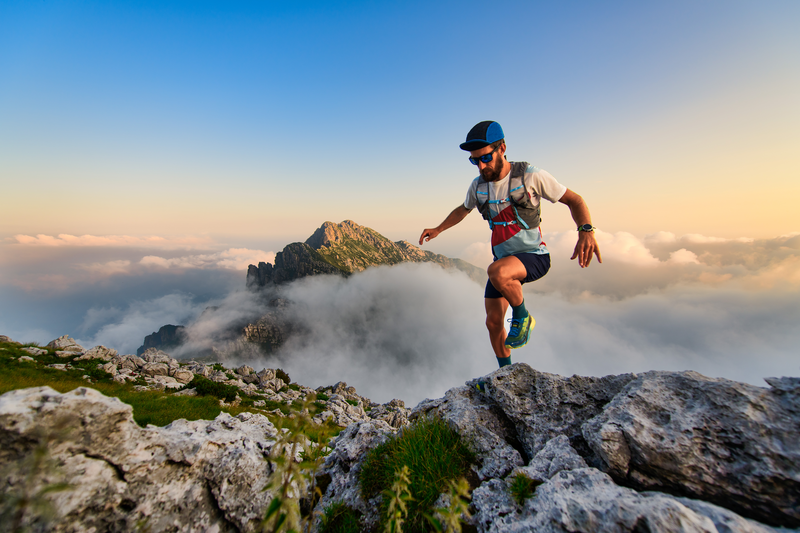
94% of researchers rate our articles as excellent or good
Learn more about the work of our research integrity team to safeguard the quality of each article we publish.
Find out more
REVIEW article
Front. Nephrol. , 19 October 2022
Sec. Onconephrology
Volume 2 - 2022 | https://doi.org/10.3389/fneph.2022.1017921
This article is part of the Research Topic Women in Onconephrology: 2022 View all 6 articles
Immune checkpoint inhibitors (ICIs) are used increasingly to treat more than 17 cancers and have shown promising therapeutic results. However, ICI use can result in a variety of immune-related adverse events (IRAEs) which can occur in any organ, including the kidneys. Acute kidney injury (AKI) is the most common nephrotoxicity, classically related to acute interstitial nephritis. Much more diverse patterns and presentations of ICI-related kidney injury can occur, and have implications for diagnostic and therapeutic management approaches. In this review, we summarize the recently approved ICIs for cancer, the incidence and risk factors for nephrotoxicity, our current understanding of the pathophysiological mechanisms and the key clinicopathological features of ICI-related AKI, and therapeutic strategies. We also explore important knowledge that require further investigation, such as the risks/benefits of ICI rechallenge in patients who recover from an episode of ICI-related AKI, and the application of liquid biopsy and microbiome to identify noninvasive biomarkers to diagnose and predict kidney injury and guide ICI therapy.
Immune checkpoint inhibitors (ICIs) are monoclonal antibodies designed to interfere with surface receptors that mediate a negative feedback loop in the immune system. These receptors are mainly present on immune cells such as T and B cells, but are also expressed by certain tumor cells. By binding to these receptors, ICIs can inhibit and/or remove “the brakes” of the immune system. As a consequence, ICIs enhance activation of T cells and exert antitumor activity (1–3). Cytotoxic T lymphocyte–associated protein 4 (CTLA-4), also known as CD152, is a CD28 homolog that is predominantly expressed on T cells. It has a high binding activity for B7 (CD80/86) mainly present on antigen-presenting cells, and interaction of CTLA-4 and B7 can decrease and/or prevent T cell activation (4). Ipilimumab, a CTLA-4 inhibitor, was the first ICI drug approved by the U.S. Food and Drug Administration (FDA) for the treatment of melanoma in 2011 (5). Since the approval of ipilimumab, the list of the FDA-approved ICIs is increasing (Table 1); subsequently, six ICI inhibitors that target programmed cell death protein 1 (PD-1) (cemiplimab, nivolumab, pembrolizumab), or PD-ligand 1 (PD-L1) (atezolizumab, avelumab, durvalumab) have been approved by FDA (1, 6, 7). PD-1 is a receptor mainly localized on the plasma membrane of T cells, while PD-L1 is expressed on the surface of tumor and antigen-presenting cells. PD-1 and PD-L1 interaction can inhibit activation of T cells, thus maintain a balance of immune homeostasis and result in blockade of anti-tumor immune response (8–10). Recently, based on data from a global, randomized phase 2-3 study, RELATIVITY-047 (11), the FDA approved relatlimab, the first immunotherapy that targets the lymphocyte activation gene-3 (LAG-3) for patients aged 12 and older with untreated metastatic or unresectable melanoma, combined with the PD-1 inhibitor nivolumab. The indications for ICIs have also been expanded to many types of malignancies (i.e., melanoma, renal cell carcinoma, non-small cell lung carcinoma, metastatic colorectal cancer, breast, and classic Hodgkin lymphoma) (Table 1) (12).
The success of ICIs has come at the cost of a distinct spectrum of side effects known as immune-related adverse events (IRAEs). IRAEs can occur in any organ in patients receiving ICIs, with a wide range in incidence from 59% to 85% overall (13, 14). IRAEs present with various degrees of severity from mild to life-threatening. The organs most commonly affected by IRAEs are the skin, gastrointestinal tract, and endocrine system; the kidneys are less frequently affected (15). Acute kidney injury (AKI) caused by acute interstitial nephritis (AIN) is the major type of ICI-related kidney injury or nephrotoxicity (6), but increasing evidence has also demonstrated other patterns of ICI-related nephrotoxicity, such as glomerular diseases (e.g., pauci-immune glomerulonephritis, C3 glomerulonephritis, renal vasculitis, and podocytopathy), acute tubular necrosis (ATN), and electrolyte abnormalities (e.g., hyponatremia, hypocalcemia, renal tubular acidosis [RTA]). Due to different mechanisms of action among ICI classes, the clinicopathological presentations might vary among patients treated with different ICIs. ICI-related kidney IRAEs pose unique diagnostic and management challenges.
In the current review, we discuss our understanding of the incidence, pathophysiological mechanisms, risk factors, key clinicopathological features, and therapeutic strategies and outcomes of ICI-related nephrotoxicity. We also discuss on-going studies and the gaps in knowledge that require further investigation, such as ICI rechallenge in patients whose kidney function recovered from an episode of ICI-related AKI, and the application of liquid biopsy and microbiome to identify noninvasive biomarkers for diagnosis and prediction of kidney injury as well as guidance of ICI therapy.
Precise data on the incidence of ICI-related adverse kidney effects such as AKI remain limited as large population-based studies across diverse international cohorts are not available. Available data, however, indicate that the incidence of AKI (defined by at least 1.5 times increase in serum creatinine relative to the baseline) in patients receiving ICI is approximately 17% (16–20). Early cohort studies reported an estimated incidence of AKI from 10% to 30% (21), but most recent studies noted a much smaller risk in the order of 3 to 5% (16, 22–25). In agreement, a systematic meta-analysis study including 48 clinical trials and 11,482 patients treated with PD-1 inhibitors demonstrated that the overall incidence of AKI was 4.2% (26). Furthermore, a retrospective study in 1766 patients receiving an ICI between 2014 and 2018 found that 7.6% (n=123) developed AKI within one year of administration of the first dose, but the incidence of AKI directly related with ICI use was only 0.8% (27). While most studies support that there is no difference in AKI risk with CTLA-4 and PD-1 inhibitor monotherapy (16–18, 22–26, 28, 29), PD-L1 inhibitors such as atezolizumab, durvalumab and avelumab may have a lower risk of AKI (<1%) (30). However, combination of the CTLA-4 inhibitor ipilimumab and the PD-1 inhibitor nivolumab carries a higher risk of ICI-related AKI (4.9%) than monotherapy with either ipilimumab (2%), nivolumab (1.9%), or pembrolizumab (1.4%) (28). Data from some clinical trials also shows less organ-toxicity such as pneumonitis and colitis in patients receiving PD-L1 inhibitors than PD-1 and CTLA-4 inhibitors (6, 31, 32). However, post-marketing surveillance data indicate that atezolizumab may have the highest risk of kidney side effects among all ICI monotherapies but confirm a low risk of ICI-related AKI with avelumab (33). So far, data on the “real-world” incidence of LAG-3 inhibitor-related AKI or other kidney side effects are unavailable. In the global phase 2-3 RELATIVITY-047 trial the incidence of ICI-related kidney adverse effects, such as nephritis and renal dysfunction that occurred within 100 days after the last dose, was only 2% in patients receiving combination therapy of relatlimab and nivolumab, compared to 1.4% in those receiving nivolumab alone (11).
In summary, the incidence of AKI directly related to ICI is low, but ranges from 0.8% to 5%. This is likely due to a variety of factors, such as patients’ demographics, definitions used to categorize AKI, different half-life of ICIs, single or combination use of ICIs, and cancer types. The epidemiology and/or incidence of ICI-related AKI and other kidney side effects, particularly in those patients with the single ICI use need be further investigated and validated by large, prospective, multicenter cohort studies.
AKI is common in patients with cancer, but ICI-related AKI that is directly caused by the use of ICI, not by other alternative etiologies, is less common. Unfortunately, clinical characteristics that can be used to distinguish ICI-related AKI from other causes-related AKI remain unavailable or unreliable, although there are some features suggestive of ICI-related AKI. A multicenter study of 138 patients with ICI-related AKI (defined by ≥2 times increase in serum creatinine or new dialysis requirement) found that most patients had sub-nephrotic proteinuria, about half presented with sterile pyuria, and a small portion of patients (~21%) had eosinophilia (24), similar to the findings from other studies (25, 28, 34, 35). Nevertheless, these clinical manifestations are not specific enough to confirm or rule out ICI-related AKI because other causes such as drug-related AKI have similar features.
A multicenter study shows that approximately half of patients with ICI-related AKI had an extrarenal IRAE (i.e., colitis, dermatitis, hypophysitis, rash, or thyroiditis) that occurred before or at the time of AKI, and rash was considered as the most common manifestation of extrarenal IRAEs (24). Studies by Seethapathy et al. and Cortazar et al. showed that the incidence of ≥1 extrarenal IRAEs in patients with ICI-related AKI was up to 62% and 87%, respectively (16, 28), and the most common coexisting IRAEs were thyroiditis and colitis (16). Thus, the presence of extrarenal IRAEs may be a crucial clinical clue when ICI-related AKI is suspected. In addition, it should be noted that AKI following ICI initiation often occurs later than other commonly reported IRAEs (36–38). As outlined in a multicenter study, the median interval from the initiation of ICI therapy to the occurrence of AKI was 14 weeks with an interquartile (IQR) range of 6-47 weeks (24). This is similar to the findings (median interval: 13 and 14 weeks, IQR range: 3-35 and 6-56 weeks, respectively) of two small studies with 13 and 16 patients, respectively (25, 28). Of note, ICI-related AKI may occur at any time after initiation of ICI therapy, from a few days after the first dose of ICI use to more than 10 weeks after the last dose given (24). Importantly, however, as shown in our studies, ICI-related AKI occurred earlier than AKI due to other causes in cancer patients undergoing ICI therapy (median [IQR], 4 months [1.2, 11.4] vs. 8.5 months [5.3, 10.4], p = 0.03), and use of proton pump inhibitors (PPI) or other AIN-associated drugs led to more rapid onset of AKI (22). Therefore, the variation of the latency period from ICI initiation to AKI occurrence is large, probably caused by long, different half-lives (6-27 days) of ICI therapy (39). Additional data are needed to clarify the interval between ICIs administration and AKI development.
Although ICI-related AKI is the most common kidney IRAE, ICI-related electrolyte abnormalities have also been described, such as hyponatremia, hypokalemia, hyperkalemia, hypophosphatemia, and hypomagnesemia (14, 21, 40–47). A narrative review covering all ICI-related renal toxicities that have been reported to the FDA adverse event reporting system between July 2011 and June 2015, confirmed that hyponatremia was the second most common type of ICI-related renal toxicities, accounting for 33.9% of reports (21). Hyponatremia was more common with the CTLA-4 inhibitor ipilimumab than the PD-1 inhibitors nivolumab and pembrolizumab (21). It needs to be recognized though that hyponatremia in general is not uncommon in patients with cancer (up to 50%), likely due to volume depletion, cirrhosis, and syndrome of inappropriate anti-diuretic hormone (48–50). As ICI can cause autoimmune adrenalitis or hypophysitis (7), ICI hyponatremia may also be caused by primary or secondary adrenal insufficiency (7). Severe hyponatremia (<124 mEq/L) due to ICI-related endocrinopathy, however, is rare, affecting only 0.3% of patients treated with ICIs (51). Regarding hypercalcemia, a systematic meta-analysis study including 48 clinical trials with 11,482 patients shows that the overall incidence in patients who received a PD-1 inhibitor is 1% (95% confidential interval (CI) 0.6-1.8%) (26). Severe hypocalcemia defined by a corrected calcium <7.0 mg/dL is a rare event, estimated to occur in only 0.2% in patients receiving ICIs (51).
Cases of distal RTA have been described in patients receiving ICIs who develop AIN. In 2018, the first presentation of RTA as a renal IRAE was reported in a 79-year-old female with metastatic non-small cell lung cancer following treatment with the PD-1 inhibitor nivolumab (52). In a 59-year-old male patient with a stage IV bronchopulmonary cancer, tubular dysfunction developed and preceded AKI following initiation of the PD-1 inhibitors nivolumab and pembrolizumab (53). It has also been shown that distal RTA persisted in some cases with ICIs therapy although kidney function had already improved (54, 55). A study analyzing kidney biopsies by immunofluorescent staining with specific antibodies found that expression of type 1 and B1 anion exchanger and vacuolar-type H+-ATPase α4 subunit was decreased in the intercalated cells in comparison with controls (54). This suggests at least partially that in the context of ICI-related autoimmune response, expression of H+-ATPase and/or Cl-/HCO3- was altered in the type A intercalated cells.
Finally, ICIs can also reactivate pre-existing autoimmune diseases (56). It is estimated that 27-50% of patients with preexisting autoimmune disease (i.e., inflammatory bowel disease, lupus, and rheumatoid arthritis), experienced worsening of their disease following treatment with an ICI (57). Based on our knowledge, there is only one case report of ICI-related primary membranous nephropathy reactivation. In this case with pleural mesothelioma, primary membranous nephropathy was reactivated following nivolumab treatment, and rituximab, specifically targeting B-lymphocytes, was effective in treating reoccurrence of membranous nephropathy. The patient was rechallenged with nivolumab and kidney function remained stable (58). There is also a case of a 69-year-old patient with metastatic melanoma who had pre-existing anti-neutrophil cytoplasmic autoantibody (ANCA) vasculitis and ipilimumab-related colitis. In this case, his vasculitis did not flare following PD-1 inhibitor pembrolizumab therapy (59).
Many studies have demonstrated that the most common pathologic lesion of ICI-related AKI is AIN, which was reported in nearly 90% of kidney biopsies (24, 25, 28, 34). However, other pathologic entities that occur either alone or in conjunction with AIN also have been described, including various glomerulonephritis and acute tubular injury or ATN (16, 18, 25, 28, 60, 61). The incidence of these glomerular diseases remains to be defined; but our estimate is that these pathologic lesions account for <10% of all cases of ICI-related AKI.
Glomerular disease is less common than AIN, but remains a crucial adverse effect of ICIs. In a large, multicenter series study, AIN was reported in 93% (n=56/60) of patients with ICI-related AKI who underwent biopsy. The remaining patients were diagnosed with minimal change disease with acute tubular injury, ANCA-negative pauci-immune crescentic glomerulonephritis, anti-glomerular basement membrane disease, and C3 glomerulonephritis (24). Another international multicenter study that included > 400 cases of ICI-related AKI showed that among patients who underwent kidney biopsy, the final diagnosis was AIN, ATN, IgA and membranous nephropathy for 82.7%, 6.3%, 2.5% and 2% of the cases, respectively (35). Smaller series in patients with advanced solid or hematologic malignancy who developed ICI-related AKI indicated the same: 88% (14 out of 16 patients) presented with AIN on kidney biopsy either as the major pathologic form or as the mild interstitial inflammation associated with other glomerular diseases (i.e., membranous glomerulonephritis, pauci-immune glomerulonephritis, C3 glomerulonephritis, IgA nephropathy, and amyloid A amyloidosis) (25). Minimal change disease was described in one patient receiving the anti-PD-1 antibody pembrolizumab for Hodgkin lymphoma and two patients receiving the CTLA-4 antibody ipilimumab for melanoma, respectively (62, 63). In addition, focal segmental glomerulosclerosis, lupus nephritis, and renal vasculitis after ICIs have been reported in several case reports (25, 60, 64, 65). A case series reported that renal vasculitis or pauci-immune glomerulonephritis occurred in four patients after ICI therapy. Interestingly, three patients presented with small to medium vessel vasculitis, but had negative ANCA testing (60). A recent study of 45 patients with biopsy-proven glomerular disease following ICIs therapy suggested that the most common kidney lesions were pauci-immune glomerulonephritis/vasculitis (n=12/45, 27%), podocytopathy (n=9/45, 20%), and C3 glomerulopathy (n=5/45, 11%) (61). Similar to ICI-related AIN, the median time from the ICI initiation to the diagnosis of glomerular disease was about 3 months. It should be noted that among patients with glomerular diseases, 40% also had AIN on biopsy (61). All of the 12 patients with pauci-immune glomerulonephritis had negative ANCA testing results, and of note, and none were taking medications such as hydralazine and/or minocycline that are typically associated with occurrence of ANCA vasculitis (61).
Finally, ATN is a noteworthy pathological feature associated found in kidney biopsies of patients who develop AKI while on ICI therapy. However, it is unclear if ATN is truly an IRAE. A small case series of 12 patients who received pembrolizumab therapy showed 5 cases with ATN, 4 with AIN and 2 with minimal change disease (66). In another series of 15 patients with AKI following ICI therapy and renal biopsy results, 9 patients had AIN and 6 had ATN (67).
In summary, the histopathological patterns of kidney injury in patients with ICIs therapy are largely heterogenous. Therefore, kidney biopsy is very important in those patients with a clinical history that highly suggests ICI-related AKI.
Several risk factors have been reported to be related to ICI-related AKI. Particularly PPI use, which is also associated to AIN in the general population, is a significant risk factor for ICI-related AKI (68). Two large retrospective studies show that the PPI use was significantly associated with ICI-related AKI, and both studies show that the hazard ratio was 2.85 (95% CI 1.81 and 4.48 to 1.34 and 6.08, respectively) (16, 24). Other drugs that have been reported to increase the risk of ICI-related AKI include non-steroidal anti-inflammatory drugs (NSAIDs) and antibiotics (16, 24). Current hypotheses suggest that administration of drugs that potentially increase the risk of AIN may initiate the immune reaction triggered by drug-specific T cells (69). These latent drug-specific T cells may be reactivated following ICIs therapy, leading to loss of immune tolerance (34, 70). As such, drugs known to cause or increase the risk of AIN should be used cautiously in patients who are receiving ICIs therapy. Interestingly, a large, multicenter cohort study shows that patients with ICI-related AKI who received AIN-associated drugs more likely recovered from AKI, possibly due to discontinuation of these nephrotoxic medications (24, 35). Other studies also show that additional risks for ICI-related AKI included lower estimated glomerular function rate at baseline, combination therapy of ICIs, and presence of extrarenal IRAEs (16, 24, 35).
To date, the precise mechanisms of ICI-related AKI have not been well defined. Immune checkpoints play a key role in maintaining normal immune responses and self-tolerance. After activation, PD-1 expression is increased on T, B, and natural killer T cells, as well as the activated monocytes and dendritic cells (71). Its ligand PD-L1 is localized on the membrane of tumor cells (72). The PD-1 and PD-L1 interaction results in blockade of anti-tumor immune responses (8–10). CTLA-4, specifically expressed on the surface of T cells, can prevent or decrease immune response by competing the binding of CD28 to CD80 or CD86 on dendritic cells (73). Furthermore, CTLA-4 can regulate the interactions between T and the antigen-presenting cells in the secondary lymphedema (74). As a result, the ICI-induced blockade of the PD-1/PD-L1 and CTLA-4 axis can cause activation of T cells in tumor tissue, which then kill tumor cells by producing inflammatory factors and cytokines (Figure 1).
Figure 1 Proposed mechanisms of immune checkpoint inhibitor-related kidney injury. (A) CTLA-4 competes with CD28 receptor binding to CD80/CD86 on dendritic cells, leading to inhibition of T cell activation and thereby avoiding autoimmune response. Anti-CTLA-4 (i.e., ipilimumab) blocks CD80/CD86 binding to the CTLA-4 receptor, which allows CD28 binding and induces activation of T cells. (B) PD-L1, the ligand of PD-1, is expressed on certain tumor cells. PD-L1 binds PD-1 and blunt T cell function. Blocking PD-L1 binding to PD-1 with anti-PD-1 or anti-PD-L1 monoclonal antibodies (i.e., nivolumab and atezolizumab) further activates T cells or suppresses deactivation of T cells and promotes tumor cell killing. (C) Activated T cells increase proinflammatory cytokines/chemokines in kidney tissue and generate autoantibodies against kidney tissue. Reactivation of drug specific effector T cells by ICIs leads to loss of tolerance. The elevation of PD-L1 on renal tubular epithelial cells can cause kidney injury through locally infiltrated effector T cells. CTLA-4, cytotoxic T lymphocyte–associated protein 4; PD-1, programmed cell death protein 1; PD-L1, programmed death ligand 1; MHC-Ag: major histocompatibility complex with tumor antigen; TCR, T cell receptor; PPI, proton pump inhibitors; NSAID, non-steroidal anti-inflammatory drug; AIN, acute interstitial nephritis; ICIs: immune checkpoint inhibitors; AKI, acute kidney injury; MCD, minimal change disease; MN, membranous nephropathy; GN, glomerulonephritis; RTA, renal tubular acidosis.
However, the initiation of this blockade may result in release of tissue-specific self-reactive T cells, which can reduce or disturb immune tolerance against renal antigens. When circulate into kidney, the aberrant activated T lymphocytes infiltrate into the parenchyma and release cytokines, leading to inflammatory reaction and kidney injury (75–77) (Figure 1). In a preclinical study, a chronic systemic inflammation and a lupus glomerulonephritis-like kidney lesion developed in mice with PD-1 knockout mice (78), providing evidence that loss or inhibition of PD-1 has kidney side effects. In addition, due to changing the immune microenvironment, exposure of ICIs may produce drug-specific antibodies against renal tissue or circulating antibodies against native antigens (79). This may lead to immune complex-related AIN. Exposure of ICIs may reactivate drug-specific T cells and then decrease their tolerance to AIN-associated drugs. The reduction of tolerance may increase the likelihood of nephrotoxicity in patients with combination therapies of ICIs and PPI or NSAIDs.
Haptenization may be another potential mechanism of ICI-related AKI. Small molecular weight drug compounds such as ICIs or their metabolized components can be recognized as an antigen by mesangial cells, kidney tubular cells, or glomerular podocytes, leading to formation of antigen-antibody complex (80). Also, ICIs can be trapped and recognized as a hapten by dendric cells in the parenchyma of kidney, leading to tubular damage and kidney injury (81).
In addition, PD-L1 is expressed both on the membrane of tumor cells and normal kidney tubule, especially the proximal tubular cells (82). In a murine model of nephrotoxic nephritis, infiltration of Foxp3+ Tregs expressing PD-L1 was detected in the kidneys (83). Inhibition of PD-L1 signaling by PD-L1 knockout or by anti- PD-L1 antibody increased Treg cells in the inflamed kidney, but more severe kidney injury was observed (83). This is likely associated with increase of kidney Th1 immune response. Notably, the gene expression profile of Tregs in kidney inflammation was significantly changed in PD-L1 knockout mice (83). Moreover, the inhibitory ability of Tregs isolated from kidney PD-L1 knockout mice were impaired in vitro. These Tregs did not protect against ATN in vivo (83). In a kidney ischemia-reperfusion injury model, administration of anti-PD-L1 or anti-PD-L2 antibodies significantly abrogated Treg-mediated protection function and exacerbated kidney inflammation and tubular injury (84). These data suggests that PD-L1 plays a protective role in kidney injury, which might be associated with Treg-mediated inhibition of the Th1 immune response. Just recently, acute rejection was observed in kidney transplant mice that received anti-PD-1 and anti-PD-L1 antibodies. Interestingly, increased infiltration of T-cells and expression of urinary lipocalin 2 were detected in these mice (85). Clinically, increased PD-L1 expression was reported in patients who underwent rejection after a kidney transplant (86); this might be a compensative upregulation. These findings suggest that PD-L1 inhibitors may execute different effects through regulating function of multiple types of cells in kidney injury (87).
Generally, patients receiving ICI therapy have routine laboratory tests such as complete blood count and comprehensive metabolic panel before initiation of ICIs; urinalysis is not currently recommended, but should be considered as a part of the initial baseline tests in patients at risk for kidney disease. As such, physicians may have more baseline information about kidney function for comparison during ICIs therapy. The following tests should be considered to improve clinical diagnosis, prognosis, and therapeutic efficacy in patients who develop ICI-related kidney injury.
The gold standard for diagnosis of ICI-related AIN or glomerular disease is kidney biopsy. First, alternative etiologies for AKI should be excluded, such as contrast-related nephropathy, volume depletion, obstructive nephropathy, and chemotherapy related renal tubular injury. Current guidelines recommend that empiric immunosuppressive therapy of suspected ICI-related AIN is acceptable without a kidney biopsy if other causes and glomerular disease can be excluded, unless there are moderate or severe life-threatening kidney side effects defined as Grade 2 to 3 or higher by Common Terminology Criteria for Adverse Events (CTCAE) (88, 89). However, this poses a potential risk to patients if unnecessary immunosuppression is initiated. Therefore, besides a complete and thorough kidney function assessment, kidney biopsy is often required to diagnose the precise kidney lesion and provide optimal therapy (7). Of note, additional histological stains may be helpful to in the diagnosis of ICI-related AKI. A study shows that positive PD-L1 staining in tubular epithelial cells can help differentiate PD-1-related AIN from other causes-related AIN (67). Nevertheless, further studies are still needed to validate the diagnostic role of PD-L1 staining in PD-1-related AIN (6).
Noninvasive tests are needed to diagnosis AIN in patients who cannot undergo kidney biopsy; however, so far, their diagnostic utility is limited. A few reports have shown that uptake of 18F-fluorodeoxyglucose (FDG) on positron emission tomography (PET) scan can be increased in renal cortex in patients with ICI-related AIN (90, 91). However, patients with ICI-related AKI caused by other non-AIN causes can also have mild FDG uptake (90, 92). As such, PET scan may not be as diagnostic in patients with mild AIN, especially in those who do not have a baseline PET scan image for comparison. But for those patients who cannot undergo kidney biopsy, PET scan may be used as an adjuvant diagnostic tool for ICI-related AIN when other causes-related AKI are excluded (7).
Kidney biopsy is the gold standard for diagnosis of ICI-related AKI providing guidance for therapeutic decisions. However, its big limitation is the invasive nature of the procedure. Furthermore, kidney biopsy cannot be repeated multiple times. Based on a small tissue, the information derived from the biopsy samples are likely incomplete and unable to reveal the whole kidney histopathology. To overcome these challenges, minimally-invasive liquid biopsy of blood or urine is an attractive alternative for identification of biomarkers (93).
It has been well known that systemic inflammatory status correlates with the occurrence of AKI. Some inflammatory factors, such as serum C-reactive protein (CRP), IL-6, IL-10, IL-17, TGF-β1, lactate dehydrogenase (LDH), lymphocyte number, and the ratio of neutrophil relative to lymphocyte, may be potentially used as the biomarkers for IRAEs. A study shows that the gene expression profile of peripheral blood before ipilimumab treatment was changed and associated with the occurrence of gastrointestinal IRAEs in patients with advanced melanoma (94). Subsequently, it has been reported that baseline serum IL-17 level was significantly correlated with severe diarrhea and colitis, while the combination use of baseline TGF-β1 and IL-10 levels was significantly (hazard ratio 2.66, p = 0.035) associated with therapeutic clinical outcome such as progression free survival after ipilimumab (95). In a phase II clinical trial including 27 patients with metastatic prostate cancer receiving ipilimumab, CD8+ T cell clones was found to be increased, which preceded the development of severe IRAEs such as rash, hypophysitis and diarrhea (96). Later on, some studies suggested that the baseline derived neutrophils/(leukocytes minus neutrophils) ratio and LDH level could be used as prognostic biomarkers in patients with advanced or metastatic non-small cell lung cancer (97, 98). It has been reported that the decreased ratios of neutrophil or platelet relative to lymphocyte can predict IRAEs (i.e., pneumonitis, diarrhea, increase lipase/amylase, increase transaminase, skin-related events, and arthralgies/myalgies) in patients with advanced non-small cell lung cancer (99). The levels of CRP, a type of acute phase protein, was reported to be increased prior to the occurrence of IRAEs in patients with ICIs therapy (100). Additionally, early increased CRP and IL-6 after the administration of nivolumab or pembrolizumab is also an indicator of cancer response in patients with non-small cell lung cancer (101). Thereafter, it has been shown that CRP, IL-6, and the neutrophil-to-lymphocyte ratio were negatively correlated with the overall survival in patients with metastatic melanoma who received nivolumab or ipilimumab, suggesting that they could be used as the prognostic markers (102). Interestingly, a recent study found that the levels of serum CRP and the ratio of urine retinol binding protein (URBP) relative to creatinine were significantly higher in patients with ICI-related AKI compared to those with non-ICI-related AKI (22). Additionally, a multivariate analysis of 38 patients with advanced non-small cell lung cancer with 32 paired serum samples revealed that follistatin (also known as activin-binding protein) and IFN-γ-inducible protein-10 (IP-10, also known as CXCL10) were statistically associated with durable clinic benefit defined by partial response and stable disease that lasted for more than 6 months while CCL5 (RANTES) was significantly associated with IRAEs onset. Reduction of CCL5 levels was also detected after initiation of corticosteroid treatment (103). Furthermore, a study shows that baseline level of CD16+ monocytes were positively associated with therapeutic response rate, but negatively correlated with Treg cell number in patients with ipilimumab therapy (104). Recently, a study including 1746 patients with metastatic breast cancer receiving ICIs therapy shows that increased infiltrations of lymphocytes and CD8+ T cells in tumor tissue can predict progression free survival and the overall survival (105).
It has been reported that urine TNF-α and IL-9 levels were persistently elevated in patients with AIN compared to those with other diagnoses (i.e., acute tubular injury, glomerular diseases, and diabetic kidney disease) and those without any known kidney diseases (106). This suggests that currently available tests of urinary TNF-α and IL-9 can be used to improve discrimination for AIN diagnosis before kidney biopsy. However, these studies included only a few patients treated with ICIs. A recent study including 37 patients with ICI-related AKI and 13 with non-ICI-related AKI found that at the time of AKI diagnosis, the ratio of URBP relative to urine creatinine was significantly higher (median [IQR] 1927 [1174, 46,522] vs. 233 [127, 989]) in patients with ICI-related AKI compared to those with the non-ICI-related AKI (22).
As described above, some serum and urine markers have shown promise for diagnosing IRAEs including AIN in a small series of patients (Table 2). Notably, the application of only one biomarker can be nonspecific for individual IRAEs. But multi-positive biomarkers can highly increase the sensitivity of diagnosis of renal IRAEs. Isik et al., showed that both the log (CRP & URBP/creatinine) is higher in patients with presumed ICI-AKI; therefore, helping with the decision on performing the kidney biopsy, particularly when other infectious diseases and other obvious causes are ruled out (22). Conversely, when both biomarkers are negative and patients are not already on any immunosuppression, the presence of ICI-related AKI is less likely (22). However, further validation is still needed in large, multicenter, prospective studies. In addition, the study of urinary proteomics may identify more potential biomarkers for kidney disease (93). Prospective studies that better defined the mechanisms of IRAEs may also identify more specific noninvasive biomarker candidates for ICI-related AKI.
Table 2 Potential noninvasive biomarkers for IRAEs and clinical outcomes in patients on ICI therapy.
Microbiota regulates multiple physiological processes, and have an important role in various pathologic conditions, including obesity, diabetes, inflammatory bowel disease, neurodegenerative disease, and cancer (107). It has been reported that the gut microbiota may have a critical role in chronic kidney disease and AKI; but the current results are inconsistent (108, 109). Recently, a study found that the gut microbiota involved immune system regulation and tumorigenesis, and its abundance and composition influenced the efficacy of ICIs treatment (107). In addition, it has been reported that certain toxicities produced following ICI therapy were associated with the intestinal microbiome, particularly in patients with colitis (110). A study of patients with melanoma found that incidence of colitis was lower in those with rich Bacteroidetes following the treatment of anti-CTLA-4 antibody ipilimumab (111). However, the role of altered microbiome in ICI-related AKI remains unstudied, and the further investigation is needed.
The severity of ICI-related AKI varies. It has been indicated that ICI-related AKI was not related to increased mortality (112); however, non-recovery of AKI has been shown to be a significant predictor for mortality (24). Therefore, early identification and prompt treatment appears to be important for management of IRAEs including ICI-related AKI.
Currently, the CTCAE grade ≥ 2 kidney toxicity (grade 2: 2-3x baseline creatinine; grade 3: >3x baseline creatinine; grade 4: >6x baseline creatinine or dialysis needed), the ASCO (American Society of Clinical Oncology), NCCN (National Comprehensive Cancer Network) and SITC (Society for Immunotherapy of Cancer) guidelines recommend temporary cessation of immunotherapy when an alternative AKI etiology cannot be identified, and initiation of corticosteroids at a dose of 0.5 to 1 mg/kg/day prednisone equivalents tapered over 4 to 6 weeks (88, 89, 113). For patients with sustained stage 1 AKI and those with stage 2 or 3 AKI, kidney biopsy is strongly recommended if there are no contraindications in order to determine the exact cause of AKI (Figure 2). Once the diagnosis of ICI-related AKI is confirmed, corticosteroids treatment should be initiated (Table 3). A study in 429 patients with ICI-related AKI demonstrated that starting corticosteroids within 14 days following diagnosis of ICI-related AKI was associated with higher likelihood of renal recovery (odds ratio 2.64; 95% CI 1.58-4.41) (35). Recently, Baker et al., demonstrated that mortality was lower in ICI-treated patients who developed ICI-related AKI as compared to those where the underlying etiology of AKI was unrelated to ICI therapy (112).
Figure 2 Management of immune checkpoint inhibitor related nephrotoxicity. AIN, acute interstitial nephritis; AKI, acute kidney injury; ATI, acute tubular injury; ATN, acute tubular necrosis; Cr, creatinine; CRP, C-reactive protein; GN, glomerulonephritis; ICIs, immune checkpoint inhibitors; IRAE, immune-related adverse event; RBC, red blood cell; RTA, renal tubular acidosis; UA, urinalysis; uRBP, urine retinol-binding protein; WBC, white blood cell.
Table 3 Proposed management of patients with presumed immune checkpoint inhibitors-related acute kidney injury (after other causes have been ruled-out).
As previously described (7), immunosuppression with prednisone (starting dose 0.8-1.0 mg/kg and maximal dose 60-80 mg daily) for stage 1 to 2 AKI, can generally obtain excellent therapeutic efficacy. Pulse-dose intravenous corticosteroids (e.g., methylprednisolone, 0.5-1 g/day for 2 to 3 days) are usually recommended for hospitalized patients with stage 3 AKI, followed by treatment of oral prednisone. Oral prednisone treatment for 8 to 12 weeks is recommended similar to other drug-indued AKI (65); but the duration of prednisone taper should depend on therapeutic efficacy. A retrospective single-center pilot study examined a small number of patients with ICI-related AKI and found that a shorter course of corticosteroids tapered withing 3 weeks yielded similar kidney outcomes compared with a longer course tapered within 6 weeks (114). However, larger randomized clinical trials with predominantly biopsy-proven ICI-related AKI (e.g., AIN) should be performed to confirm these findings. It should be noted that corticosteroid therapy in ICI-related AKI may need to be longer in patients with higher chance of relapse. This could be partially caused by the long half-life of ICIs, which be as high as 27 days for pembrolizumab (39).
In addition, a study shows that corticosteroid dosages were higher in patients with a complete response compared to those with a partial response (23). Although it is hard to compare the corticosteroid outcomes in retrospective studies, this suggests that the initial dose of prednisone may play a key role in AKI recovery. There is also a subset of patients with ICI-related AIN that do not respond to corticosteroid treatment (28). In such patients who presented with corticosteroid refractory or dependent, immunosuppression regimen should be promptly adjusted by switching or adding other immunosuppressant drugs (i.e., azathioprine, cyclophosphamide, cyclosporine, infliximab, mycophenolate mofetil, or rituximab) (25, 88, 89, 115–117), but the data is limited so far. A case series study including 10 patients with refractory or relapsed ICI-related AKI shows that majority of patients (80%) obtained a long period complete or partial remission following treatment with infliximab (118). However, the median time of corticosteroid therapy in this study was 3.5 weeks only which may explain the relapse. Mycophenolate mofetil, which is commonly used as a steroid-sparing agent in other forms of AIN, may also be effective in ICI-related AKI that is steroid refractory (119, 120).
Some studies have suggested that the outcomes are poor in patients with ICI-related glomerular diseases (112). However, a systematic review study including 45 patients with biopsy-proven ICI-related glomerular disease shows that almost all patients received corticosteroids, and 73% experienced complete or partial recovery of AKI (61).
Immunosuppression therapy for IRAEs may decrease anti-tumor efficacy of ICIs and the overall outcomes (121–123). There is mixed data on whether corticosteroid use for IRAEs worsen cancer survival (121–123); some studies have indicated that corticosteroids may reduce survival (124). In patients with non-small cell lung cancer who were treated with PD-L1 inhibitors, cancer outcomes were worse in those with prednisone doses ≥10 mg (125, 126). Therefore, corticosteroids should be also used cautiously.
The ICIs are typically withheld until AKI recovers, and some patients with severe AKI that does not fully recover need permanently discontinuation of ICI. Nevertheless, ICIs may be the only effective treatment option in some cancers, and ICI rechallenge has to be considered in those who recover from an episode of ICI-related AKI (127) (Figure 2). Patients with severe kidney dysfunction before ICI administration and whose kidney function takes longer (>6 weeks) to recover from an episode of ICI-related AKI very likely developed permanent kidney dysfunction (39). Some studies show that the recurrence rate of ICI-related AKI ranges from 5.1% to 40% after ICI rechallenge (16, 18, 22, 24, 29, 128, 129). Recently, a large, multicenter study shows that of the 121 patients rechallenged, only 20 (16.5%) developed recurrent ICI-related AKI. All patients with recurrent ICI-related AKI held their ICI therapy, and 70% (14 out of 20) were treated with corticosteroids, and 60% (12 out of 20) achieved renal recovery (35). Notably, it has been shown that the incidence of recurrent ICI-related AKI is relatively low, but patients who recovery from ICI-related AKI may be at risk of developing other IRAEs after rechallenge with ICIs. In addition, patients who developed recurrent AKI after ICI rechallenge were often receiving a concomitant AIN-inducible drug such as PPI, NSAIDs, and antibiotics (23, 24, 130). Patients who were receiving a potential offending drug (PPI, NSAID, antibiotic) at the time they developed ICI-related AKI may be good candidates for rechallenge, as long as they can strictly avoid these agents during ICI rechallenge. Before initiating rechallenge therapy, a detailed discussion about the risks and benefits should be discussed between the multidisciplinary team of oncologists and nephrologist with the patient. Once rechallenge is started, it is important to closely monitor for AKI recurrence in order to early recognize and take prompt intervention strategies. In addition, further studies are also required to identify specific biomarkers and risk factors that can predict IRAEs during ICIs rechallenge.
The incidence of ICI-related AKI is rising due to increasing use of ICIs in patients with cancer. Early and accurate diagnosis of ICI-related AKI is important to guide therapeutic strategies. The most commonly reported kidney lesion is AIN. Nevertheless, glomerular disease, acute tubular injury, and electrolyte abnormalities have also been described. The differential diagnosis of AKI in patients receiving ICIs is broad and due to the lack of sensitive clinicopathologic and laboratory findings, future experimental and clinical studies are urgently needed to identify noninvasive biomarkers that can be used to diagnose and predict ICI-related AIN. The underlying molecular mechanisms of AKI caused by ICIs should be further investigated and clarified. Patients with ICI-related AIN should temporarily withhold ICI therapy and start corticosteroid therapy, whereas pure ICI-related ATN does not require discontinuation of ICI therapy. For rechallenge of ICI after ICI-related AKI, future studies are needed to compare immunosuppression strategies and determine the optimal timing of ICI rechallenge. The risk factors and the long-term outcomes of ICI-related AKI need be better understood.
All authors contributed to the research, development, and content of the manuscript. All authors contributed to the article and approved the submitted version.
Author MS is supported by NIH R01 DK130839. Author SH is supported by National Institute of Health K08 DK118120 from the NIDDK and by Mayo CCaTS grant number UL1TR002377.
MES has served as a scientific advisory board member to Mallinckrodt.
The remaining authors declare that the research was conducted in the absence of any commercial or financial relationships that could be construed as a potential conflict of interest.
All claims expressed in this article are solely those of the authors and do not necessarily represent those of their affiliated organizations, or those of the publisher, the editors and the reviewers. Any product that may be evaluated in this article, or claim that may be made by its manufacturer, is not guaranteed or endorsed by the publisher.
1. Gupta S, Cortazar FB, Riella LV, Leaf DE. Immune checkpoint inhibitor nephrotoxicity: Update 2020. Kidney360 (2020) 1(2):130–40. doi: 10.34067/KID.0000852019
2. Leach DR, Krummel MF, Allison JP. Enhancement of antitumor immunity by CTLA-4 blockade. Science (1996) 271(5256):1734–6. doi: 10.1126/science.271.5256.1734
3. Boussiotis VA. Molecular and biochemical aspects of the PD-1 checkpoint pathway. N Engl J Med (2016) 375(18):1767–78. doi: 10.1056/NEJMra1514296
4. Pentcheva-Hoang T, Egen JG, Wojnoonski K, Allison JP. B7-1 and B7-2 selectively recruit CTLA-4 and CD28 to the immunological synapse. Immunity (2004) 21(3):401–13. doi: 10.1016/j.immuni.2004.06.017
5. Wolchok JD, Neyns B, Linette G, Negrier S, Lutzky J, Thomas L, et al. Ipilimumab monotherapy in patients with pretreated advanced melanoma: a randomised, double-blind, multicentre, phase 2, dose-ranging study. Lancet Oncol (2010) 11(2):155–64. doi: 10.1016/S1470-2045(09)70334-1
6. Seethapathy H, Herrmann SM, Sise ME. Immune checkpoint inhibitors and kidney toxicity: Advances in diagnosis and management. Kidney Med (2021) 3(6):1074–81. doi: 10.1016/j.xkme.2021.08.008
7. Herrmann SM, Perazella MA. Immune checkpoint inhibitors and immune-related adverse renal events. Kidney Int Rep (2020) 5(8):1139–48. doi: 10.1016/j.ekir.2020.04.018
8. Pardoll DM. The blockade of immune checkpoints in cancer immunotherapy. Nat Rev Cancer (2012) 12(4):252–64. doi: 10.1038/nrc3239
9. Agata Y, Kawasaki A, Nishimura H, Ishida Y, Tsubata T, Yagita H, et al. Expression of the PD-1 antigen on the surface of stimulated mouse T and b lymphocytes. Int Immunol (1996) 8(5):765–72. doi: 10.1093/intimm/8.5.765
10. Aguiar PN Jr., Santoro IL, Tadokoro H, de Lima Lopes G, Filardi BA, Oliveira P, et al. The role of PD-L1 expression as a predictive biomarker in advanced non-small-cell lung cancer: a network meta-analysis. Immunotherapy (2016) 8(4):479–88. doi: 10.2217/imt-2015-0002
11. Tawbi HA, Schadendorf D, Lipson EJ, Ascierto PA, Matamala L, Castillo Gutierrez E, et al. Relatlimab and nivolumab versus nivolumab in untreated advanced melanoma. N Engl J Med (2022) 386(1):24–34. doi: 10.1056/NEJMoa2109970
12. Marin-Acevedo JA, Kimbrough EO, Manochakian R, Zhao Y, Lou Y. Immunotherapies targeting stimulatory pathways and beyond. J Hematol Oncol (2021) 14(1):78. doi: 10.1186/s13045-021-01085-3
13. Postow MA, Sidlow R, Hellmann MD. Immune-related adverse events associated with immune checkpoint blockade. N Engl J Med (2018) 378(2):158–68. doi: 10.1056/NEJMra1703481
14. Larkin J, Chiarion-Sileni V, Gonzalez R, Grob JJ, Cowey CL, Lao CD, et al. Combined nivolumab and ipilimumab or monotherapy in untreated melanoma. N Engl J Med (2015) 373(1):23–34. doi: 10.1056/NEJMoa1504030
15. Champiat S, Lambotte O, Barreau E, Belkhir R, Berdelou A, Carbonnel F, et al. Management of immune checkpoint blockade dysimmune toxicities: a collaborative position paper. Ann Oncol (2016) 27(4):559–74. doi: 10.1093/annonc/mdv623
16. Seethapathy H, Zhao S, Chute DF, Zubiri L, Oppong Y, Strohbehn I, et al. The incidence, causes, and risk factors of acute kidney injury in patients receiving immune checkpoint inhibitors. Clin J Am Soc Nephrol (2019) 14(12):1692–700. doi: 10.2215/CJN.00990119
17. Stein C, Burtey S, Mancini J, Pelletier M, Sallee M, Brunet P, et al. Acute kidney injury in patients treated with anti-programmed death receptor-1 for advanced melanoma: a real-life study in a single-centre cohort. Nephrol Dial Transplant (2021) 36(9):1664–74. doi: 10.1093/ndt/gfaa137
18. Meraz-Munoz A, Amir E, Ng P, Avila-Casado C, Ragobar C, Chan C, et al. Acute kidney injury associated with immune checkpoint inhibitor therapy: incidence, risk factors and outcomes. J Immunother Cancer (2020) 8(1):e000467. doi: 10.1136/jitc-2019-000467
19. Garcia-Carro C, Bolufer M, Bury R, Castaneda Z, Munoz E, Felip E, et al. Acute kidney injury as a risk factor for mortality in oncological patients receiving checkpoint inhibitors. Nephrol Dial Transplant (2022) 37(5):887–94. doi: 10.1093/ndt/gfab034
20. Shimamura Y, Watanabe S, Maeda T, Abe K, Ogawa Y, Takizawa H. Incidence and risk factors of acute kidney injury, and its effect on mortality among Japanese patients receiving immune check point inhibitors: a single-center observational study. Clin Exp Nephrol (2021) 25(5):479–87. doi: 10.1007/s10157-020-02008-1
21. Wanchoo R, Karam S, Uppal NN, Barta VS, Deray G, Devoe C, et al. Adverse renal effects of immune checkpoint inhibitors: A narrative review. Am J Nephrol (2017) 45(2):160–9. doi: 10.1159/000455014
22. Isik B, Alexander MP, Manohar S, Vaughan L, Kottschade L, Markovic S, et al. Biomarkers, clinical features, and rechallenge for immune checkpoint inhibitor renal immune-related adverse events. Kidney Int Rep (2021) 6(4):1022–31. doi: 10.1016/j.ekir.2021.01.013
23. Manohar S, Ghamrawi R, Chengappa M, Goksu BNB, Kottschade L, Finnes H, et al. Acute interstitial nephritis and checkpoint inhibitor therapy: Single center experience of management and drug rechallenge. Kidney360 (2020) 1(1):16–24. doi: 10.34067/KID.0000152019
24. Cortazar FB, Kibbelaar ZA, Glezerman IG, Abudayyeh A, Mamlouk O, Motwani SS, et al. Clinical features and outcomes of immune checkpoint inhibitor-associated AKI: A multicenter study. J Am Soc Nephrol (2020) 31(2):435–46. doi: 10.1681/ASN.2019070676
25. Mamlouk O, Selamet U, Machado S, Abdelrahim M, Glass WF, Tchakarov A, et al. Nephrotoxicity of immune checkpoint inhibitors beyond tubulointerstitial nephritis: single-center experience. J Immunother Cancer (2019) 7(1):2. doi: 10.1186/s40425-018-0478-8
26. Manohar S, Kompotiatis P, Thongprayoon C, Cheungpasitporn W, Herrmann J, Herrmann SM. Programmed cell death protein 1 inhibitor treatment is associated with acute kidney injury and hypocalcemia: meta-analysis. Nephrol Dial Transplant (2019) 34(1):108–17. doi: 10.1093/ndt/gfy105
27. Sorah JD, Rose TL, Radhakrishna R, Derebail VK, Milowsky MI. Incidence and prediction of immune checkpoint inhibitor-related nephrotoxicity. J Immunother (2021) 44(3):127–31. doi: 10.1097/CJI.0000000000000338
28. Cortazar FB, Marrone KA, Troxell ML, Ralto KM, Hoenig MP, Brahmer JR, et al. Clinicopathological features of acute kidney injury associated with immune checkpoint inhibitors. Kidney Int (2016) 90(3):638–47. doi: 10.1016/j.kint.2016.04.008
29. Espi M, Teuma C, Novel-Catin E, Maillet D, Souquet PJ, Dalle S, et al. Renal adverse effects of immune checkpoints inhibitors in clinical practice: ImmuNoTox study. Eur J Cancer (2021) 147:29–39. doi: 10.1016/j.ejca.2021.01.005
30. Seethapathy H, Zhao S, Strohbehn IA, Lee M, Chute DF, Bates H, et al. Incidence and clinical features of immune-related acute kidney injury in patients receiving programmed cell death ligand-1 inhibitors. Kidney Int Rep (2020) 5(10):1700–5. doi: 10.1016/j.ekir.2020.07.011
31. Sonpavde GP, Grivas P, Lin Y, Hennessy D, Hunt JD. Immune-related adverse events with PD-1 versus PD-L1 inhibitors: a meta-analysis of 8730 patients from clinical trials. Future Oncol (2021) 17(19):2545–58. doi: 10.2217/fon-2020-1222
32. Martins F, Sofiya L, Sykiotis GP, Lamine F, Maillard M, Fraga M, et al. Adverse effects of immune-checkpoint inhibitors: epidemiology, management and surveillance. Nat Rev Clin Oncol (2019) 16(9):563–80. doi: 10.1038/s41571-019-0218-0
33. Chen G, Qin Y, Fan QQ, Zhao B, Mei D, Li XM. Renal adverse effects following the use of different immune checkpoint inhibitor regimens: A real-world pharmacoepidemiology study of post-marketing surveillance data. Cancer Med (2020) 9(18):6576–85. doi: 10.1002/cam4.3198
34. Shirali AC, Perazella MA, Gettinger S. Association of acute interstitial nephritis with programmed cell death 1 inhibitor therapy in lung cancer patients. Am J Kidney Dis (2016) 68(2):287–91. doi: 10.1053/j.ajkd.2016.02.057
35. Gupta S, Short SAP, Sise ME, Prosek JM, Madhavan SM, Soler MJ, et al. Acute kidney injury in patients treated with immune checkpoint inhibitors. J Immunother Cancer (2021) 9(10):e003467. doi: 10.1136/jitc-2021-003467
36. Weber J, Mandala M, Del Vecchio M, Gogas HJ, Arance AM, Cowey CL, et al. Adjuvant nivolumab versus ipilimumab in resected stage III or IV melanoma. N Engl J Med (2017) 377(19):1824–35. doi: 10.1056/NEJMoa1709030
37. Weber JS, Kahler KC, Hauschild A. Management of immune-related adverse events and kinetics of response with ipilimumab. J Clin Oncol (2012) 30(21):2691–7. doi: 10.1200/JCO.2012.41.6750
38. Weber JS, Hodi FS, Wolchok JD, Topalian SL, Schadendorf D, Larkin J, et al. Safety profile of nivolumab monotherapy: A pooled analysis of patients with advanced melanoma. J Clin Oncol (2017) 35(7):785–92. doi: 10.1200/JCO.2015.66.1389
39. Perazella MA, Shirali AC. Immune checkpoint inhibitor nephrotoxicity: what do we know and what should we do? Kidney Int (2020) 97(1):62–74. doi: 10.1016/j.kint.2019.07.022
40. Barnard ZR, Walcott BP, Kahle KT, Nahed BV, Coumans JV. Hyponatremia associated with ipilimumab-induced hypophysitis. Med Oncol (2012) 29(1):374–7. doi: 10.1007/s12032-010-9794-7
41. Chodakiewitz Y, Brown S, Boxerman JL, Brody JM, Rogg JM. Ipilimumab treatment associated pituitary hypophysitis: clinical presentation and imaging diagnosis. Clin Neurol Neurosurg (2014) 125:125–30. doi: 10.1016/j.clineuro.2014.06.011
42. Vandiver JW, Singer Z, Harshberger C. Severe hyponatremia and immune nephritis following an initial infusion of nivolumab. Target Oncol (2016) 11(4):553–6. doi: 10.1007/s11523-016-0426-9
43. Topalian SL, Sznol M, McDermott DF, Kluger HM, Carvajal RD, Sharfman WH, et al. Survival, durable tumor remission, and long-term safety in patients with advanced melanoma receiving nivolumab. J Clin Oncol (2014) 32(10):1020–30. doi: 10.1200/JCO.2013.53.0105
44. Weber JS, D'Angelo SP, Minor D, Hodi FS, Gutzmer R, Neyns B, et al. Nivolumab versus chemotherapy in patients with advanced melanoma who progressed after anti-CTLA-4 treatment (CheckMate 037): a randomised, controlled, open-label, phase 3 trial. Lancet Oncol (2015) 16(4):375–84. doi: 10.1016/S1470-2045(15)70076-8
45. Postow MA, Chesney J, Pavlick AC, Robert C, Grossmann K, McDermott D, et al. Nivolumab and ipilimumab versus ipilimumab in untreated melanoma. N Engl J Med (2015) 372(21):2006–17. doi: 10.1056/NEJMoa1414428
46. Wolchok JD, Kluger H, Callahan MK, Postow MA, Rizvi NA, Lesokhin AM, et al. Nivolumab plus ipilimumab in advanced melanoma. N Engl J Med (2013) 369(2):122–33. doi: 10.1056/NEJMoa1302369
47. Murakami N, Borges TJ, Yamashita M, Riella LV. Severe acute interstitial nephritis after combination immune-checkpoint inhibitor therapy for metastatic melanoma. Clin Kidney J (2016) 9(3):411–7. doi: 10.1093/ckj/sfw024
48. Girotra M, Hansen A, Farooki A, Byun DJ, Min L, Creelan BC, et al. The current understanding of the endocrine effects from immune checkpoint inhibitors and recommendations for management. JNCI Cancer Spectr (2018) 2(3):pky021. doi: 10.1093/jncics/pky021
49. Trainer H, Hulse P, Higham CE, Trainer P, Lorigan P. Hyponatraemia secondary to nivolumab-induced primary adrenal failure. Endocrinol Diabetes Metab Case Rep (2016) 2016:16–0108. doi: 10.1530/EDM-16-0108
50. Faje AT, Sullivan R, Lawrence D, Tritos NA, Fadden R, Klibanski A, et al. Ipilimumab-induced hypophysitis: a detailed longitudinal analysis in a large cohort of patients with metastatic melanoma. J Clin Endocrinol Metab (2014) 99(11):4078–85. doi: 10.1210/jc.2014-2306
51. Seethapathy H, Rusibamayila N, Chute DF, Lee M, Strohbehn I, Zubiri L, et al. Hyponatremia and other electrolyte abnormalities in patients receiving immune checkpoint inhibitors. Nephrol Dial Transplant (2021) 36(12):2241–7. doi: 10.1093/ndt/gfaa272
52. El Bitar S, Weerasinghe C, El-Charabaty E, Odaimi M. Renal tubular acidosis an adverse effect of PD-1 inhibitor immunotherapy. Case Rep Oncol Med (2018) 2018:8408015. doi: 10.1155/2018/8408015
53. Charmetant X, Teuma C, Lake J, Dijoud F, Frochot V, Deeb A. A new expression of immune checkpoint inhibitors' renal toxicity: When distal tubular acidosis precedes creatinine elevation. Clin Kidney J (2020) 13(1):42–5. doi: 10.1093/ckj/sfz051
54. Herrmann SM, Alexander MP, Romero MF, Zand L. Renal tubular acidosis and immune checkpoint inhibitor therapy: An immune-related adverse event of PD-1 inhibitor-a report of 3 cases. Kidney Med (2020) 2(5):657–62. doi: 10.1016/j.xkme.2020.05.015
55. Atiq SO, Gokhale T, Atiq Z, Holmes R, Sparks MA. A case of pembrolizumab induced distal renal tubular acidosis. J Onco-Nephrology (2021) 5(1):23–6. doi: 10.1177/2399369321992776
56. Abdel-Wahab N, Shah M, Lopez-Olivo MA, Suarez-Almazor ME. Use of immune checkpoint inhibitors in the treatment of patients with cancer and preexisting autoimmune disease: A systematic review. Ann Intern Med (2018) 168(2):121–30. doi: 10.7326/M17-2073
57. Johnson DB, Beckermann KE, Wang DY. Immune checkpoint inhibitor therapy in patients with autoimmune disease. Oncol (Williston Park) (2018) 32(4):190–4.
58. Lin JS, Wang DY, Mamlouk O, Glass WF, Abdelrahim M, Yee C, et al. Immune checkpoint inhibitor associated reactivation of primary membranous nephropathy responsive to rituximab. J Immunother Cancer (2020) 8(2):e001287. doi: 10.1136/jitc-2020-001287
59. Maul LV, Weichenthal M, Kahler KC, Hauschild A. Successful anti-PD-1 antibody treatment in a metastatic melanoma patient with known severe autoimmune disease. J Immunother (2016) 39(4):188–90. doi: 10.1097/CJI.0000000000000118
60. Gallan AJ, Alexander E, Reid P, Kutuby F, Chang A, Henriksen KJ. Renal vasculitis and pauci-immune glomerulonephritis associated with immune checkpoint inhibitors. Am J Kidney Dis (2019) 74(6):853–6. doi: 10.1053/j.ajkd.2019.04.016
61. Kitchlu A, Jhaveri KD, Wadhwani S, Deshpande P, Harel Z, Kishibe T, et al. A systematic review of immune checkpoint inhibitor-associated glomerular disease. Kidney Int Rep (2021) 6(1):66–77. doi: 10.1016/j.ekir.2020.10.002
62. Kitchlu A, Fingrut W, Avila-Casado C, Chan CT, Crump M, Hogg D, et al. Nephrotic syndrome with cancer immunotherapies: A report of 2 cases. Am J Kidney Dis (2017) 70(4):581–5. doi: 10.1053/j.ajkd.2017.04.026
63. Kidd JM, Gizaw AB. Ipilimumab-associated minimal-change disease. Kidney Int (2016) 89(3):720. doi: 10.1016/j.kint.2015.11.028
64. Daanen RA, Maas RJH, Koornstra RHT, Steenbergen EJ, van Herpen CML, Willemsen A. Nivolumab-associated nephrotic syndrome in a patient with renal cell carcinoma: A case report. J Immunother (2017) 40(9):345–8. doi: 10.1097/CJI.0000000000000189
65. Fadel F, El Karoui K, Knebelmann B. Anti-CTLA4 antibody-induced lupus nephritis. N Engl J Med (2009) 361(2):211–2. doi: 10.1056/NEJMc0904283
66. Izzedine H, Mathian A, Champiat S, Picard C, Mateus C, Routier E, et al. Renal toxicities associated with pembrolizumab. Clin Kidney J (2019) 12(1):81–8. doi: 10.1093/ckj/sfy100
67. Cassol C, Satoskar A, Lozanski G, Rovin B, Hebert L, Nadasdy T, et al. Anti-PD-1 immunotherapy may induce interstitial nephritis with increased tubular epithelial expression of PD-L1. Kidney Int Rep (2019) 4(8):1152–60. doi: 10.1016/j.ekir.2019.06.001
68. Blank ML, Parkin L, Paul C, Herbison P. A nationwide nested case-control study indicates an increased risk of acute interstitial nephritis with proton pump inhibitor use. Kidney Int (2014) 86(4):837–44. doi: 10.1038/ki.2014.74
69. Spanou Z, Keller M, Britschgi M, Yawalkar N, Fehr T, Neuweiler J, et al. Involvement of drug-specific T cells in acute drug-induced interstitial nephritis. J Am Soc Nephrol (2006) 17(10):2919–27. doi: 10.1681/ASN.2006050418
70. Kwiatkowska E, Domanski L, Dziedziejko V, Kajdy A, Stefanska K, Kwiatkowski S. The mechanism of drug nephrotoxicity and the methods for preventing kidney damage. Int J Mol Sci (2021) 22(11):6109. doi: 10.3390/ijms22116109
71. Keir ME, Butte MJ, Freeman GJ, Sharpe AH. PD-1 and its ligands in tolerance and immunity. Annu Rev Immunol (2008) 26:677–704. doi: 10.1146/annurev.immunol.26.021607.090331
72. Dermani FK, Samadi P, Rahmani G, Kohlan AK, Najafi R. PD-1/PD-L1 immune checkpoint: Potential target for cancer therapy. J Cell Physiol (2019) 234(2):1313–25. doi: 10.1002/jcp.27172
73. Hosseini A, Gharibi T, Marofi F, Babaloo Z, Baradaran B. CTLA-4: From mechanism to autoimmune therapy. Int Immunopharmacol (2020) 80:106221. doi: 10.1016/j.intimp.2020.106221
74. Lo B, Abdel-Motal UM. Lessons from CTLA-4 deficiency and checkpoint inhibition. Curr Opin Immunol (2017) 49:14–9. doi: 10.1016/j.coi.2017.07.014
75. de Miguel M, Calvo E. Clinical challenges of immune checkpoint inhibitors. Cancer Cell (2020) 38(3):326–33. doi: 10.1016/j.ccell.2020.07.004
76. Galluzzi L, Humeau J, Buque A, Zitvogel L, Kroemer G. Immunostimulation with chemotherapy in the era of immune checkpoint inhibitors. Nat Rev Clin Oncol (2020) 17(12):725–41. doi: 10.1038/s41571-020-0413-z
77. Perazella MA, Shirali AC. Nephrotoxicity of cancer immunotherapies: Past, present and future. J Am Soc Nephrol (2018) 29(8):2039–52. doi: 10.1681/ASN.2018050488
78. Nishimura H, Nose M, Hiai H, Minato N, Honjo T. Development of lupus-like autoimmune diseases by disruption of the PD-1 gene encoding an ITIM motif-carrying immunoreceptor. Immunity (1999) 11(2):141–51. doi: 10.1016/S1074-7613(00)80089-8
79. Marco T, Anna P, Annalisa T, Francesco M, Stefania SL, Stella D, et al. The mechanisms of acute interstitial nephritis in the era of immune checkpoint inhibitors in melanoma. Ther Adv Med Oncol (2019) 11:1758835919875549. doi: 10.1177/1758835919875549
80. Izzedine H, Gueutin V, Gharbi C, Mateus C, Robert C, Routier E, et al. Kidney injuries related to ipilimumab. Invest New Drugs (2014) 32(4):769–73. doi: 10.1007/s10637-014-0092-7
81. Hofmann L, Forschner A, Loquai C, Goldinger SM, Zimmer L, Ugurel S, et al. Cutaneous, gastrointestinal, hepatic, endocrine, and renal side-effects of anti-PD-1 therapy. Eur J Cancer (2016) 60:190–209. doi: 10.1016/j.ejca.2016.02.025
82. Ding H, Wu X, Gao W. PD-L1 is expressed by human renal tubular epithelial cells and suppresses T cell cytokine synthesis. Clin Immunol (2005) 115(2):184–91. doi: 10.1016/j.clim.2005.01.005
83. Neumann K, Ostmann A, Breda PC, Ochel A, Tacke F, Paust HJ, et al. The co-inhibitory molecule PD-L1 contributes to regulatory T cell-mediated protection in murine crescentic glomerulonephritis. Sci Rep (2019) 9(1):2038. doi: 10.1038/s41598-018-38432-3
84. Jaworska K, Ratajczak J, Huang L, Whalen K, Yang M, Stevens BK, et al. Both PD-1 ligands protect the kidney from ischemia reperfusion injury. J Immunol (2015) 194(1):325–33. doi: 10.4049/jimmunol.1400497
85. Shim YJ, Khedraki R, Dhar J, Fan R, Dvorina N, Valujskikh A, et al. Early T cell infiltration is modulated by programed cell death-1 protein and its ligand (PD-1/PD-L1) interactions in murine kidney transplants. Kidney Int (2020) 98(4):897–905. doi: 10.1016/j.kint.2020.03.037
86. Starke A, Lindenmeyer MT, Segerer S, Neusser MA, Rusi B, Schmid DM, et al. Renal tubular PD-L1 (CD274) suppresses alloreactive human T-cell responses. Kidney Int (2010) 78(1):38–47. doi: 10.1038/ki.2010.97
87. Tian R, Liang J, Li R, Zhou X. Acute kidney injury induced by immune checkpoint inhibitors. Kidney Dis (2022) 8:190–201. doi: 10.1159/000520798
88. Brahmer JR, Lacchetti C, Schneider BJ, Atkins MB, Brassil KJ, Caterino JM, et al. Management of immune-related adverse events in patients treated with immune checkpoint inhibitor therapy: American society of clinical oncology clinical practice guideline. J Clin Oncol (2018) 36(17):1714–68. doi: 10.1200/JCO.2017.77.6385
89. Thompson JA, Schneider BJ, Brahmer J, Andrews S, Armand P, Bhatia S, et al. Management of immunotherapy-related toxicities, version 1.2019. J Natl Compr Canc Netw (2019) 17(3):255–89. doi: 10.6004/jnccn.2019.0013
90. Qualls D, Seethapathy H, Bates H, Tajmir S, Heidari P, Endres P, et al. Positron emission tomography as an adjuvant diagnostic test in the evaluation of checkpoint inhibitor-associated acute interstitial nephritis. J Immunother Cancer (2019) 7(1):356. doi: 10.1186/s40425-019-0820-9
91. Heybeli C, Nathan MA, Herrmann SM. Renal injury in the setting of immune checkpoint inhibitor: report of a case of hypothyroidism and the role of positron emission tomography. J Onco-Nephrology (2020) 4(3):112–6. doi: 10.1177/2399369320945724
92. Adams MC, Turkington TG, Wilson JM, Wong TZ. A systematic review of the factors affecting accuracy of SUV measurements. AJR Am J Roentgenol (2010) 195(2):310–20. doi: 10.2214/AJR.10.4923
93. Mischak H. Pro: urine proteomics as a liquid kidney biopsy: no more kidney punctures! Nephrol Dial Transplant (2015) 30(4):532–7. doi: 10.1093/ndt/gfv046
94. Shahabi V, Berman D, Chasalow SD, Wang L, Tsuchihashi Z, Hu B, et al. Gene expression profiling of whole blood in ipilimumab-treated patients for identification of potential biomarkers of immune-related gastrointestinal adverse events. J Transl Med (2013) 11:75. doi: 10.1186/1479-5876-11-75
95. Tarhini AA, Zahoor H, Lin Y, Malhotra U, Sander C, Butterfield LH, et al. Baseline circulating IL-17 predicts toxicity while TGF-beta1 and IL-10 are prognostic of relapse in ipilimumab neoadjuvant therapy of melanoma. J Immunother Cancer (2015) 3:39. doi: 10.1186/s40425-015-0081-1
96. Subudhi SK, Aparicio A, Gao J, Zurita AJ, Araujo JC, Logothetis CJ, et al. Clonal expansion of CD8 T cells in the systemic circulation precedes development of ipilimumab-induced toxicities. Proc Natl Acad Sci USA (2016) 113(42):11919–24. doi: 10.1073/pnas.1611421113
97. Mezquita L, Auclin E, Ferrara R, Charrier M, Remon J, Planchard D, et al. Association of the lung immune prognostic index with immune checkpoint inhibitor outcomes in patients with advanced non-small cell lung cancer. JAMA Oncol (2018) 4(3):351–7. doi: 10.1001/jamaoncol.2017.4771
98. Kazandjian D, Gong Y, Keegan P, Pazdur R, Blumenthal GM. Prognostic value of the lung immune prognostic index for patients treated for metastatic non-small cell lung cancer. JAMA Oncol (2019) 5(10):1481–5. doi: 10.1001/jamaoncol.2019.1747
99. Pavan A, Calvetti L, Dal Maso A, Attili I, Del Bianco P, Pasello G, et al. Peripheral blood markers identify risk of immune-related toxicity in advanced non-small cell lung cancer treated with immune-checkpoint inhibitors. Oncologist (2019) 24(8):1128–36. doi: 10.1634/theoncologist.2018-0563
100. Abolhassani AR, Schuler G, Kirchberger MC, Heinzerling L. C-reactive protein as an early marker of immune-related adverse events. J Cancer Res Clin Oncol (2019) 145(10):2625–31. doi: 10.1007/s00432-019-03002-1
101. Ozawa Y, Amano Y, Kanata K, Hasegwa H, Matsui T, Kakutani T, et al. Impact of early inflammatory cytokine elevation after commencement of PD-1 inhibitors to predict efficacy in patients with non-small cell lung cancer. Med Oncol (2019) 36(4):33. doi: 10.1007/s12032-019-1255-3
102. Laino AS, Woods D, Vassallo M, Qian X, Tang H, Wind-Rotolo M, et al. Serum interleukin-6 and c-reactive protein are associated with survival in melanoma patients receiving immune checkpoint inhibition. J Immunother Cancer (2020) 8(1):e000842. doi: 10.1136/jitc-2020-000842
103. Oyanagi J, Koh Y, Sato K, Mori K, Teraoka S, Akamatsu H, et al. Predictive value of serum protein levels in patients with advanced non-small cell lung cancer treated with nivolumab. Lung Cancer (2019) 132:107–13. doi: 10.1016/j.lungcan.2019.03.020
104. Romano E, Kusio-Kobialka M, Foukas PG, Baumgaertner P, Meyer C, Ballabeni P, et al. Ipilimumab-dependent cell-mediated cytotoxicity of regulatory T cells ex vivo by nonclassical monocytes in melanoma patients. Proc Natl Acad Sci USA (2015) 112(19):6140–5. doi: 10.1073/pnas.1417320112
105. Zou Y, Zou X, Zheng S, Tang H, Zhang L, Liu P, et al. Efficacy and predictive factors of immune checkpoint inhibitors in metastatic breast cancer: a systematic review and meta-analysis. Ther Adv Med Oncol (2020) 12:1758835920940928. doi: 10.1177/1758835920940928
106. Moledina DG, Wilson FP, Pober JS, Perazella MA, Singh N, Luciano RL, et al. Urine TNF-alpha and IL-9 for clinical diagnosis of acute interstitial nephritis. JCI Insight (2019) 4(10):e127456. doi: 10.1172/jci.insight.127456
107. Wu J, Wang S, Zheng B, Qiu X, Wang H, Chen L. Modulation of gut microbiota to enhance effect of checkpoint inhibitor immunotherapy. Front Immunol (2021) 12:669150. doi: 10.3389/fimmu.2021.669150
108. Kobayashi T, Iwata Y, Nakade Y, Wada T. Significance of the gut microbiota in acute kidney injury. Toxins (Basel) (2021) 13(6):369. doi: 10.3390/toxins13060369
110. Hayase E, Jenq RR. Role of the intestinal microbiome and microbial-derived metabolites in immune checkpoint blockade immunotherapy of cancer. Genome Med (2021) 13(1):107. doi: 10.1186/s13073-021-00923-w
111. Dubin K, Callahan MK, Ren B, Khanin R, Viale A, Ling L, et al. Intestinal microbiome analyses identify melanoma patients at risk for checkpoint-blockade-induced colitis. Nat Commun (2016) 7:10391. doi: 10.1038/ncomms10391
112. Baker ML, Yamamoto Y, Perazella MA, Dizman N, Shirali AC, Hafez N, et al. Mortality after acute kidney injury and acute interstitial nephritis in patients prescribed immune checkpoint inhibitor therapy. J Immunother Cancer (2022) 10(3):e004421. doi: 10.1136/jitc-2021-004421
113. Puzanov I, Diab A, Abdallah K, Bingham CO 3rd, Brogdon C, Dadu R, et al. Managing toxicities associated with immune checkpoint inhibitors: consensus recommendations from the society for immunotherapy of cancer (SITC) toxicity management working group. J Immunother Cancer (2017) 5(1):95. doi: 10.1186/s40425-017-0300-z
114. Lee MD, Seethapathy H, Strohbehn IA, Zhao SH, Boland GM, Fadden R, et al. Rapid corticosteroid taper versus standard of care for immune checkpoint inhibitor induced nephritis: a single-center retrospective cohort study. J Immunother Cancer (2021) 9(4):e002292. doi: 10.1136/jitc-2020-002292
115. Spain L, Diem S, Larkin J. Management of toxicities of immune checkpoint inhibitors. Cancer Treat Rev (2016) 44:51–60. doi: 10.1016/j.ctrv.2016.02.001
116. Carlos CA, Hsu RK. Acute kidney injury with immune checkpoint inhibitors: A push beyond case reports. Clin J Am Soc Nephrol (2019) 14(12):1679–81. doi: 10.2215/CJN.12621019
117. Kyriazis P, Tiwary A, Freeman J, Landry D, Braden G. Atypical anti-glomerular basement membrane glomerulonephritis in a patient with metastatic melanoma treated with mitogen-activated protein kinase and immune checkpoint inhibitors: a case report. J Med Case Rep (2021) 15(1):186. doi: 10.1186/s13256-021-02766-w
118. Lin JS, Mamlouk O, Selamet U, Tchakarov A, Glass WF, Sheth RA, et al. Infliximab for the treatment of patients with checkpoint inhibitor-associated acute tubular interstitial nephritis. Oncoimmunology (2021) 10(1):1877415. doi: 10.1080/2162402X.2021.1877415
119. Jessel S, Austin M, Kluger HM. Mycophenolate as primary treatment for immune checkpoint inhibitor induced acute kidney injury in a patient with concurrent immunotherapy-associated diabetes: A case report. Clin Oncol Case Rep (2021) 4(1):156.
120. Omori G, Takada K, Murase K, Hayasaka N, Nakamura H, Iyama S, et al. Successful mycophenolate mofetil treatment of a patient with severe steroid-refractory hepatitis evoked by nivolumab plus ipilimumab treatment for relapsed bladder cancer. Clin Case Rep (2021) 9(2):654–9. doi: 10.1002/ccr3.3597
121. Horvat TZ, Adel NG, Dang TO, Momtaz P, Postow MA, Callahan MK, et al. Immune-related adverse events, need for systemic immunosuppression, and effects on survival and time to treatment failure in patients with melanoma treated with ipilimumab at memorial Sloan Kettering cancer center. J Clin Oncol (2015) 33(28):3193–8. doi: 10.1200/JCO.2015.60.8448
122. Johnson DB, Friedman DL, Berry E, Decker I, Ye F, Zhao S, et al. Survivorship in immune therapy: Assessing chronic immune toxicities, health outcomes, and functional status among long-term ipilimumab survivors at a single referral center. Cancer Immunol Res (2015) 3(5):464–9. doi: 10.1158/2326-6066.CIR-14-0217
123. Toi Y, Sugawara S, Sugisaka J, Ono H, Kawashima Y, Aiba T, et al. Profiling preexisting antibodies in patients treated with anti-PD-1 therapy for advanced non-small cell lung cancer. JAMA Oncol (2019) 5(3):376–83. doi: 10.1001/jamaoncol.2018.5860
124. Petrelli F, Signorelli D, Ghidini M, Ghidini A, Pizzutilo EG, Ruggieri L, et al. Association of steroids use with survival in patients treated with immune checkpoint inhibitors: A systematic review and meta-analysis. Cancers (Basel) (2020) 12(3):546. doi: 10.3390/cancers12030546
125. Faje AT, Lawrence D, Flaherty K, Freedman C, Fadden R, Rubin K, et al. High-dose glucocorticoids for the treatment of ipilimumab-induced hypophysitis is associated with reduced survival in patients with melanoma. Cancer (2018) 124(18):3706–14. doi: 10.1002/cncr.31629
126. Arbour KC, Mezquita L, Long N, Rizvi H, Auclin E, Ni A, et al. Impact of baseline steroids on efficacy of programmed cell death-1 and programmed death-ligand 1 blockade in patients with non-Small-Cell lung cancer. J Clin Oncol (2018) 36(28):2872–8. doi: 10.1200/JCO.2018.79.0006
127. Herrmann SM. Is rechallenge appropriate in patients that develop immune checkpoint inhibitor-associated AKI?: PRO. Kidney360 (2022) 3:799–802. doi: 10.34067/KID.0003962021
128. Dolladille C, Ederhy S, Sassier M, Cautela J, Thuny F, Cohen AA, et al. Immune checkpoint inhibitor rechallenge after immune-related adverse events in patients with cancer. JAMA Oncol (2020) 6(6):865–71. doi: 10.1001/jamaoncol.2020.0726
129. Hultin S, Nahar K, Menzies AM, Long GV, Fernando SL, Atkinson V, et al. Histological diagnosis of immune checkpoint inhibitor induced acute renal injury in patients with metastatic melanoma: a retrospective case series report. BMC Nephrol (2020) 21(1):391. doi: 10.1186/s12882-020-02044-9
130. Koda R, Watanabe H, Tsuchida M, Iino N, Suzuki K, Hasegawa G, et al. Immune checkpoint inhibitor (nivolumab)-associated kidney injury and the importance of recognizing concomitant medications known to cause acute tubulointerstitial nephritis: a case report. BMC Nephrol (2018) 19(1):48. doi: 10.1186/s12882-018-0848-y
Keywords: immune checkpoint inhibitors, immune-related adverse events, nephrotoxicity, acute kidney injury, acute interstitial nephritis, liquid biopsy, noninvasive biomarkers, rechallenge
Citation: Miao J, Sise ME and Herrmann SM (2022) Immune checkpoint inhibitor related nephrotoxicity: Advances in clinicopathologic features, noninvasive approaches, and therapeutic strategy and rechallenge. Front. Nephrol. 2:1017921. doi: 10.3389/fneph.2022.1017921
Received: 12 August 2022; Accepted: 28 September 2022;
Published: 19 October 2022.
Edited by:
Sabine Karam, University of Minnesota Health Twin Cities, United StatesReviewed by:
Javier Molina-Cerrillo, Ramón y Cajal University Hospital, SpainCopyright © 2022 Miao, Sise and Herrmann. This is an open-access article distributed under the terms of the Creative Commons Attribution License (CC BY). The use, distribution or reproduction in other forums is permitted, provided the original author(s) and the copyright owner(s) are credited and that the original publication in this journal is cited, in accordance with accepted academic practice. No use, distribution or reproduction is permitted which does not comply with these terms.
*Correspondence: Sandra M. Herrmann, aGVycm1hbm4uc2FuZHJhQG1heW8uZWR1
Disclaimer: All claims expressed in this article are solely those of the authors and do not necessarily represent those of their affiliated organizations, or those of the publisher, the editors and the reviewers. Any product that may be evaluated in this article or claim that may be made by its manufacturer is not guaranteed or endorsed by the publisher.
Research integrity at Frontiers
Learn more about the work of our research integrity team to safeguard the quality of each article we publish.