- 1Departamento de Neurociencia, Facultad de Medicina, Universidad de Chile, Santiago, Chile
- 2Programa de Doctorado en Neurociencias, Centro Interdisciplinario de Neurociencia UC, Pontificia Universidad Católica de Chile, Santiago, Chile
- 3Departamento de Fonoaudiología, Facultad de Medicina, Universidad de Chile, Santiago, Chile
- 4Biomedical Neuroscience Institute, Facultad de Medicina, Universidad de Chile, Santiago, Chile
- 5Latin American Brain Health Institute (BrainLat), Universidad Adolfo Ibáñez, Santiago, Chile
- 6Centro Avanzado de Ingeniería Eléctrica y Electrónica, Advanced Center for Electrical and Electronic Engineering (AC3E), Universidad Técnica Federico Santa María, Valparaíso, Chile
- 7Servicio de Otorrinolaringología, Hospital Clínico Universidad de Chile, Santiago, Chile
Introduction: The mechanisms underlying tinnitus perception are still under research. One of the proposed hypotheses involves an alteration in top-down processing of auditory activity. Low-frequency oscillations in the delta and theta bands have been recently described in brain and cochlear infrasonic signals during selective attention paradigms in normal hearing controls. Here, we propose that the top-down oscillatory activity observed in brain and cochlear signals during auditory and visual selective attention in normal subjects, is altered in tinnitus patients, reflecting an abnormal functioning of the corticofugal pathways that connect brain circuits with the cochlear receptor.
Methods: To test this hypothesis, we used a behavioral task that alternates between auditory and visual top-down attention while we simultaneously measured electroencephalogram (EEG) and distortion-product otoacoustic emissions (DPOAE) signals in 14 tinnitus and 14 control subjects.
Results: We found oscillatory activity in the delta and theta bands in cortical and cochlear channels in control and tinnitus patients. There were significant decreases in the DPOAE oscillatory amplitude during the visual attention period as compared to the auditory attention period in tinnitus and control groups. We did not find significant differences when using a between-subjects statistical approach comparing tinnitus and control groups. On the other hand, we found a significant cluster in the delta band in tinnitus when using within-group statistics to compare the difference between auditory and visual DPOAE oscillatory power.
Conclusion: These results confirm the presence of top-down infrasonic low-frequency cochlear oscillatory activity in the delta and theta bands in tinnitus patients, showing that the corticofugal suppression of cochlear oscillations during visual and auditory attention in tinnitus patients is preserved.
Introduction
Tinnitus is the perception of a phantom sound in the absence of external acoustic stimulation (Elgoyhen et al., 2015). It is a prevalent condition ranging from 11 to 30% of the general population, while for a subset of individuals, it becomes a chronic and distressful symptom (McCormack et al., 2016), affecting auditory, cognitive, and emotional brain networks (Husain, 2016; De Ridder et al., 2021). Chronic tinnitus sufferers have a higher prevalence of neuropsychiatric conditions, mainly anxiety and depressive symptoms (Geocze et al., 2013; Ziai et al., 2017). In addition, cognitive abilities, such as selective attention, can be affected by tinnitus (Roberts et al., 2013), as the perception of the phantom stimulus could be perceived as an annoying distractor that constantly disrupts attention, altering performance in auditory and visual attention tasks (Araneda et al., 2015).
Although several hypotheses have been raised to understand the neurobiology of tinnitus, to date, the neural mechanisms of tinnitus are still elusive (Knipper et al., 2020). One of the proposed mechanisms involves alterations in top-down processing at different levels of the auditory pathway (Knipper et al., 2021). In this line, the auditory efferent system connects central auditory structures with cochlear hair cells and auditory-nerve neurons by multiple neuroanatomical feedback circuits (Elgueda and Delano, 2020) that might be altered in tinnitus sufferers (Knipper et al., 2020). The auditory efferent system is organized into (i) brainstem circuits (medial and lateral olivocochlear neurons) (Warr and Guinan, 1979), and (ii) corticofugal descending pathways connecting the auditory cortex with subcortical nuclei (Terreros and Delano, 2015; Lauer et al., 2022). One of the functions that has been attributed to these corticofugal pathways is to suppress auditory responses during selective attention tasks (Oatman, 1971; Delano et al., 2007; Srinivasan et al., 2012; Wittekindt et al., 2014; Terreros et al., 2016). The latter is particularly important in the context of tinnitus. Evidence has shown that attention impairments are involved in the inability of patients to divert attention from the phantom percept (Khan and Husain, 2020). This suggests that the corticofugal functioning of auditory efferent pathways could be altered in tinnitus sufferers (Araneda et al., 2015; Knipper et al., 2020).
A possible alteration in the auditory efferent functioning in tinnitus patients has been studied by several researchers with contradictory results (Riga et al., 2015). However, it is important to highlight that previous studies searching for a role of the auditory efferent system in tinnitus have only studied brainstem circuits, specifically the activation of the medial olivocochlear reflex with contralateral acoustic stimulation (Riga et al., 2015). Thus, it is still unknown whether there is a functional alteration of the auditory efferent corticofugal projections during attention paradigms in tinnitus sufferers. In this article, we aim to elucidate possible disruptions of these corticofugal pathways in the context of tinnitus during a cross-modal attention task.
Recently, in non-tinnitus subjects, we described a top-down mechanism involving the corticofugal oscillatory modulation of cochlear responses at theta and delta bands (< 10 Hz), during visual and auditory attention, using simultaneous electroencephalogram (EEG) and distortion-product otoacoustic emissions (DPOAE) recordings (Dragicevic et al., 2019). Furthermore, similar oscillatory corticofugal modulations have been observed in measurements of spontaneous external ear canal pressure (Köhler et al., 2021; Köhler and Weisz, 2023) and auditory-nerve responses recorded with cochlear implants (Gehmacher et al., 2022), during visual and auditory selective attention tasks. Taken together, these independent works provide evidence of a top-down oscillatory mechanism in delta and theta frequency bands during selective visual and auditory attention that modulates cochlear and auditory-nerve responses.
Here, we propose that the corticofugal oscillatory activity observed during auditory and visual selective attention in normal subjects (Dragicevic et al., 2019; Köhler et al., 2021; Gehmacher et al., 2022) is altered in tinnitus patients, reflecting an abnormal functioning of the corticofugal pathways that connect the auditory cortex with the cochlear receptor. To test this hypothesis, we used the same attentional paradigm as in Dragicevic et al. (2019), simultaneously measuring EEG and DPOAE signals in tinnitus and control subjects. These two integrated recording methods allowed us to evaluate top-down oscillatory mechanisms -from cerebral cortex to cochlea- in a frequency range (1–40 Hz) in which cognitive effects are commonly observed in neural networks (Buzsáki and Draguhn, 2004).
Materials and methods
Subjects
Subjects were recruited from the Otolaryngology Department of the Clinical Hospital of the Universidad de Chile, which were invited to participate voluntarily, including 14 participants as the tinnitus group, and 14 subjects as the control group. Tinnitus group inclusion criteria were (i) 18–60 years old, (ii) either unilateral or bilateral non-pulsatile tinnitus (> 3 months of duration), and (iii) audiogram thresholds ≤ 25 dB HL. All procedures were approved by the Institutional Scientific Ethic Committee of the Clinical Hospital of the Universidad de Chile (approval number OAIC 016/20042016), and all participants signed a written informed consent.
Audiology
Hearing thresholds were measured using air-conduction pure-tone audiometry (AC40, Interacoustics, Denmark). We calculated the pure tone average (PTA) for each ear by averaging hearing thresholds at 0.5, 1, 2 and 4 kHz frequencies. To discard conductive hearing loss, we also measured the external ear canal acoustic immittance in both ears with a clinical device (AT235H, Interacoustics, Denmark).
Behavioral task alternating between visual and auditory attention
Subjects performed the same behavioral paradigm described in Dragicevic et al., 2019, in which selective attention to visual and auditory modality alternated consecutively across trials. The participants had to complete 4 blocks of 44 trials each (about 8 minutes per block). Additionally, once the instructions were explained and the recordings were confirmed to be robust (low impedance in the EEG signal and clear DPOAEs with respect to the surrounding noise), at least one training block was carried out to ensure the quality of the behavioral reports. Visual task. The visual task started with a passive (no attention) pseudo-random period (lasting between 2 and 2.5 s). As depicted in Figure 1A (left), during visual attention trials, subjects had to focus their attention on the pointer of a clockwise-revolving clock (1 Hz), passing through 100 tick marks, and report the offset time of a visual cue while ignoring DPOAE eliciting tones (f1 and f2) (that served as distractors). The onset of this visual cue (color change on the rim of the clock) indicated the period of focused visual attention (variable duration between 1.5 and 2.5 s) to the time indicated by the position of the clock’s pointer. No silent gaps were presented during the visual task. Subjects gave their visual task response by first pressing a button, which triggered opposite and slower (1/3 Hz) rotation of the clock and releasing the button at the desired position. No feedback was provided to the subjects regarding performance. Immediately following button release, the task switches to auditory attention, and the clock hand no longer moves but jumps to random positions without coherent motion. Initial training of this response modality was given until motor error was minimized to about 3.6°, corresponding to the minimum angular step programmed in our display (360°/100). Auditory task. As depicted in Figure 1A (right), a brief gap of silence interrupted the DPOAE-eliciting tones appearing in a period of variable duration (between 1.5 and 2.5 s), and subjects had to report gap detection while looking at the center of the clock and ignoring the clock. After the behavioral response to the gap in DPOAE, the clock recovered its clockwise motion, and after a period of variable duration (between 2 and 2.5 s), the visual cue started, indicating a new period of visual attention.
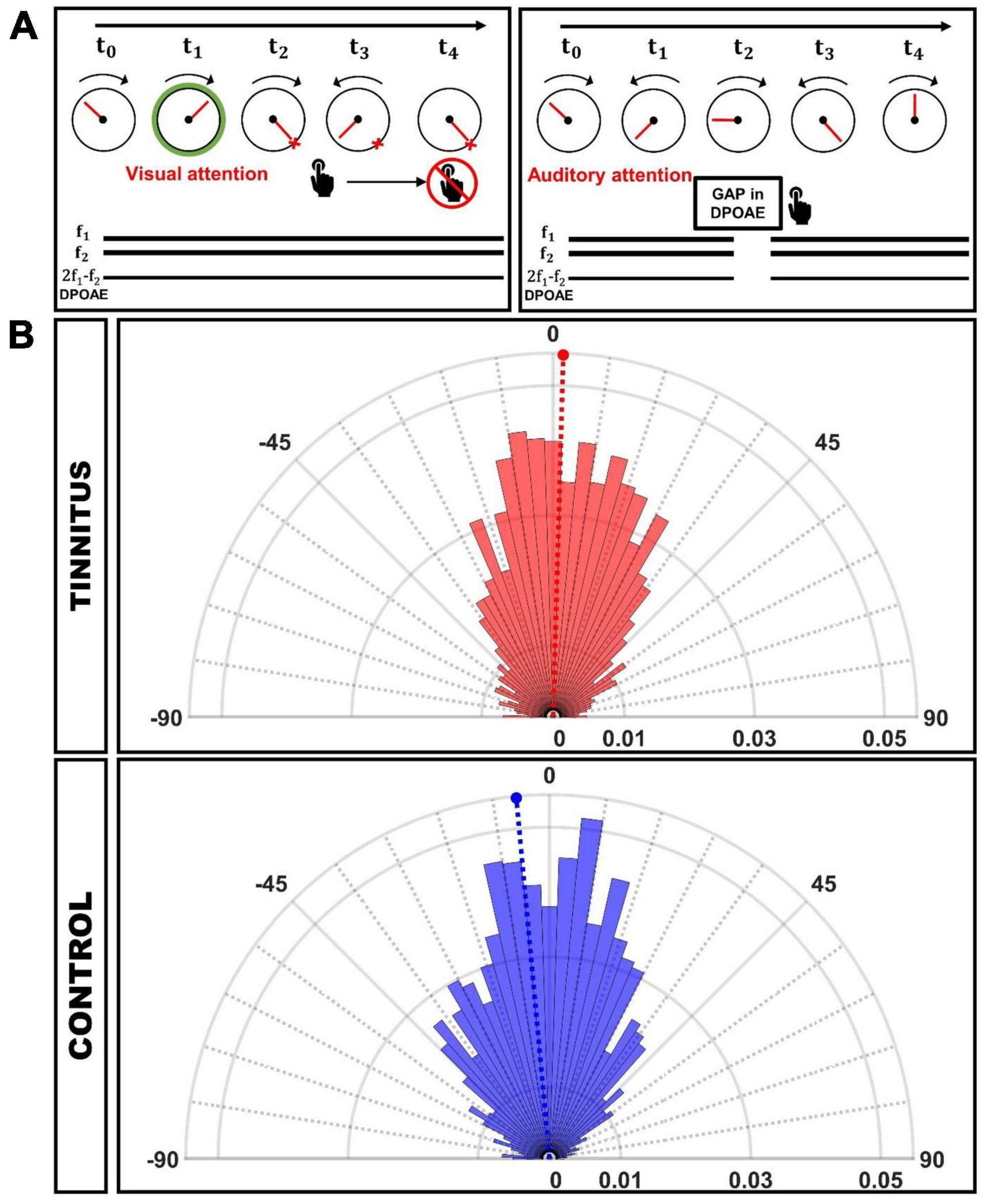
Figure 1. (A) Behavioral task used to evaluate visual and auditory attention. Left: Visual attention: Subjects had to report the position of the clock’s tick at the offset of the green circle (visual attention cue). Simultaneously two tones (f1 and f2) that elicit DPOAEs were presented as auditory distractors. Right: Auditory attention: Individuals had to respond to the gap of silence embedded in the f1 and f2 stimuli while the clock‘s tick is moving randomly. DPOAE were recorded continuously during the whole task. (B) Circular histograms of behavioral responses during the visual attention task, in which 0° represents the clock’s tick target position. The magnitude of the column bars illustrates the probability of a given response position in the clock referenced to the visual target offset. The dashed lines mark the mean angle for each group (Tinnitus: 0.98°, CI-95%: [–1.10°, 3.07°]; Control: –5.03°, CI-95%: [–6.79°, –3.26°]).
Data acquisition system and general procedures
Continuous 32-channels EEG and 4-channels electro-oculogram (EOG) signals were recorded using Tucker-Davis Technologies hardware (PZ3 for EEG and RA4PA for EOG). Ring shaped Ag/AgCl electrodes were positioned in an elastic headcap (size 56 or 58, EasyCap, Germany) that was secured with velcro under the chin area. EEG electrode positions complied with the 10–20 EEG standard system. The ground electrode was positioned on AFz. Scalp contacts were cleaned with alcohol and electroconductive gel was applied to keep impedances < 5 kΩ. Data were digitally filtered using a band pass filter (0.1–100 Hz) and a notch filter at 50 Hz. The output of this filtered data was saved with a sampling rate of 1 kHz. Simultaneously, the DPOAE signal was recorded using an Etymotic Research microphone (ER10-C). EEG, EOG, and DPOAE signals were synchronized with a desktop computer running a custom software written in LabWindows/CVI 9.0 via a National Instruments A/D converter that controlled visual stimuli on a high refresh rate screen (100 Hz) and auditory stimuli by Transistor-Transistor Logic (TTL) pulses. Volunteers were prepared for EEG and DPOAE recordings, inspection of the external ear canal to assess the presence of earwax, selection and calibration of f1 and f2 primary tones to elicit DPOAE. Training blocks were presented before data acquisition to ensure the full comprehension of the behavioral task.
Data pre-processing
Electroencephalogram (EEG) and DPOAE single trial channels were evaluated by means of visual inspection. We carried out the rejection of trials considering the amplitude of the complete DPOAE signal for each recording block, this was done through cursors that delimited the allowed amplitudes. The trials that passed the described visual inspection process were individually inspected using the ELAN software (Aguera et al., 2011). For this procedure, the DPOAE channels, vertical EOG, horizontal EOG, and the EEG channels Fz, F3, Fc1, Cz, Fc2, F4, P3, O1, Pz, O2 and P4 were considered. Additionally, we applied the independent component analysis (ICA) technique to EEG channels to remove artifacts related to blinking (EOG recordings were used to improve eye blink detection for data pre-processing) and cardiac rhythm using the EEGLAB toolbox (v. 14.1.2) for Matlab.
DPOAE virtual channel
We generated a DPOAE “virtual” channel using a Hilbert-based approach to obtain the oscillation amplitude of the 2f1-f2 DPOAE component in the 1–50 Hz band. The method uses a band-pass filter of the signal with an attenuation value > 100 dB and centered at the frequency of the DPOAE (2f1-f2). After that, the envelope of the filtered signal was calculated using the Hilbert method. The signal envelope of the 2f1-f2 DPOAE amplitude was subsequently processed in the frequency domain in conjunction with EEG channels.
Data analysis
For the EEG and DPOAE channels, time and frequency domain averages across trials were calculated, we used time windows of ± 1,500 ms aligned to the onset of visual or auditory attention periods. In the case of the visual modality, the trials were locked to the onset of the visual cue (0 ms for visual attention) that marked the beginning of the visual selective attention period. For the auditory modality, the onset of the selective attention period (0 ms for auditory attention) was determined by the end of the visual period.
Spectrograms were analyzed between 1 and 50 Hz at 1 Hz resolution. For each subject and channel, the spectrum of the single trials was obtained using Morlet wavelets (recommended because of their ideal balance between time and frequency resolution necessary for the dynamics of the cortical and cochlear oscillations). Then, frequency-specific z-scores were obtained based on each trial baseline (−1,500 to 0 ms); that is, for each trial and frequency, the mean and standard deviation of the baseline was calculated, and the resulting spectrogram between 1 and 50 Hz was represented in z-scores. Using Z-scores in this way helped us to rescue the individual relevance of single trials instead of averaging the raw trials, and then performing Z-scoring. Finally, the spectrograms were averaged for each subject.
To evaluate possible significant differences in time spectrograms between auditory and visual attention in control and tinnitus groups, we performed permutation tests (Maris and Oostenveld, 2007) using within-group and between-groups approaches. The general procedure consisted of computing the subtraction between the time-frequency matrices represented as z-scores. Then, a mask generated by the permutations test (from the two groups of n = 14) was performed to identify significant differences in the differential spectrograms. We used a threshold of 0.05 for significance, 16,000 permutations, a maximum number of clusters of 3, and the two-sided option for detecting negative and positive clusters.
The differences in the time courses of the cochlear and electroencephalographic oscillations were evaluated by implementing a slope-to-peak analysis. This was done for each subject by calculating the point-to-point derivatives of the means of the z-score spectrograms in the 1–8 Hz band during the auditory and visual attention periods. The derivatives were calculated from 0 ms to the point at which it changed sign, then these values were averaged. Then, individual values were averaged for each channel (by modality and group). The statistically significant differences in the mean slope values were assessed with the Mann-Whitney test. In all the procedures mentioned above, the EEG channels chosen as relevant for the detailed analysis were Cz, Fz, and O1. These channels were selected as a measure of oscillatory activity in the auditory cortex, prefrontal cortex, and visual cortex, respectively, that were also used in Dragicevic et al., 2019.
Angular response analysis of the visual selective attention task
The behavioral responses in the visual period were recorded as the angular deviation relative to the correct answer, independent of the absolute target angle, such that a perfect response corresponded to 0° of deviation. It is important to note that in our protocol, a response represented by a positive angle means that the subject released the button before the clock pointer reached the target angle for that trial. Conversely, a negative response means that the subject release after the clock pointer pass the target angle. We analyzed the angular responses using the CircStat Toolbox for Circular Statistics (Berens, 2009). Statistical comparisons between tinnitus and control groups were performed using the Watson-Williams test.
Results
We recorded simultaneous EEG and DPOAE signals in 28 subjects, including 14 individuals with tinnitus [mean age 38.1 ± 9.1 years (mean ± SD)], and 14 controls (mean age 34.2 ± 8.0 years; p = 0.12, t-test) while they were performing a behavioral task that alternates between visual and auditory attention (Dragicevic et al., 2019). There were non-significant differences in hearing thresholds between tinnitus and control groups as evaluated by audiograms obtained from 0.125 to 16 kHz (Figure 2). The PTA (0.5 to 4 kHz) for the tinnitus group was: 9.20 ± 0.28 dB HL (mean ± SD), while for the control group was: 9.02 ± 0.30 dB HL, p = 0.45, t-test. Regarding anxiety levels, there were non-significant differences between tinnitus and control groups, as evaluated by the State-Trait Anxiety Inventory (STAI) scores (STAI tinnitus: 37.1 ± 9.3; STAI controls: 34.4 ± 12.0; p = 0.25, t-test). The mean Tinnitus Handicap Inventory (THI) for the group of tinnitus patients was 31.1 ± 19.7 points, ranging between 8 and 72 points. Table 1 summarizes individual demographic and audiological data from the 14 tinnitus and 14 controls included in this study.
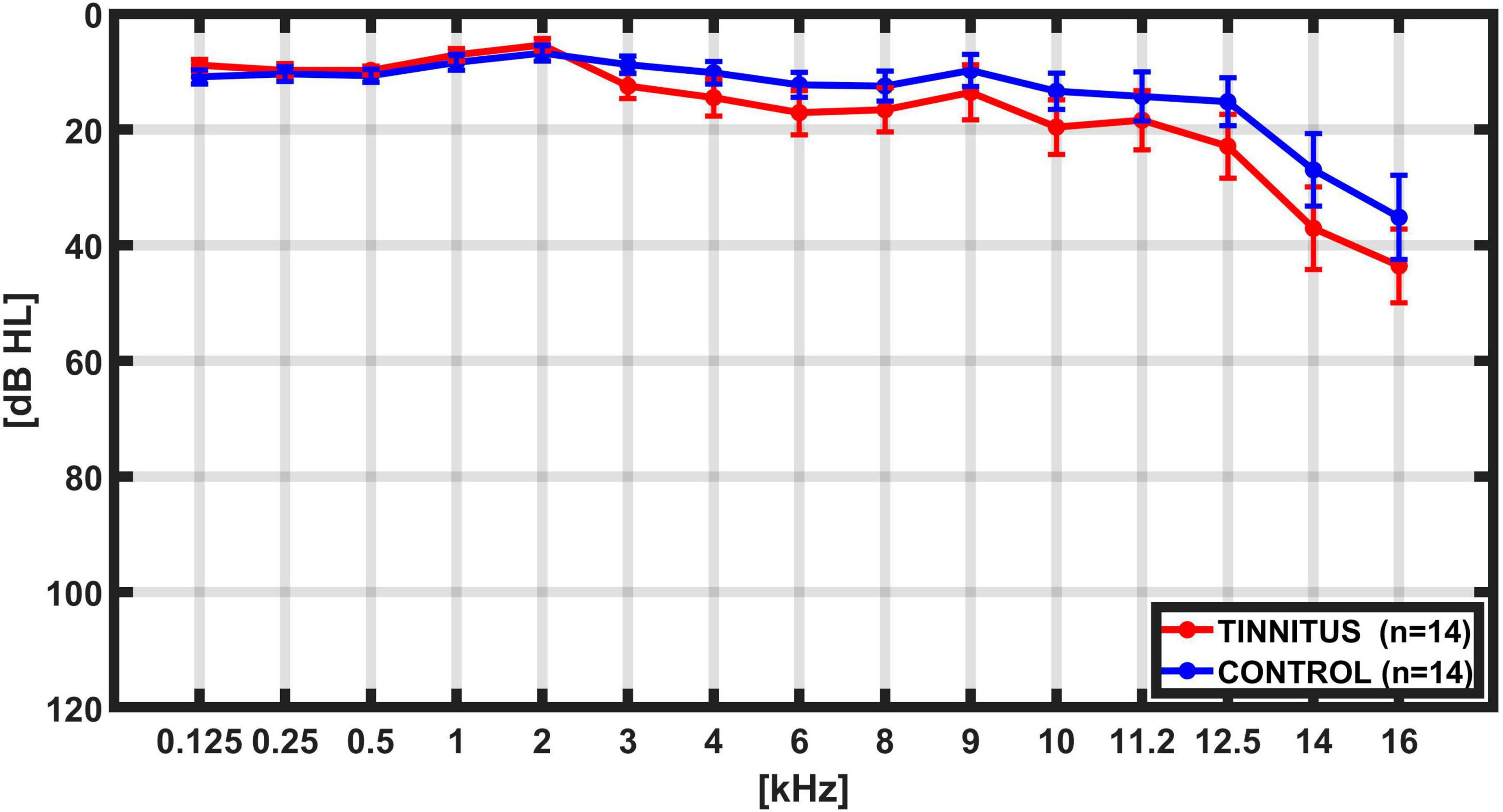
Figure 2. Grand average hearing thresholds. Bilateral average audiogram thresholds from 0.125 kHz to 16 kHz in controls (blue, n = 14) and tinnitus (red, n = 14) subjects. Error bars represents SEM. Although high-frequency hearing threshold tended to be worse in tinnitus, this difference did not reach statistical significance in the present sample.
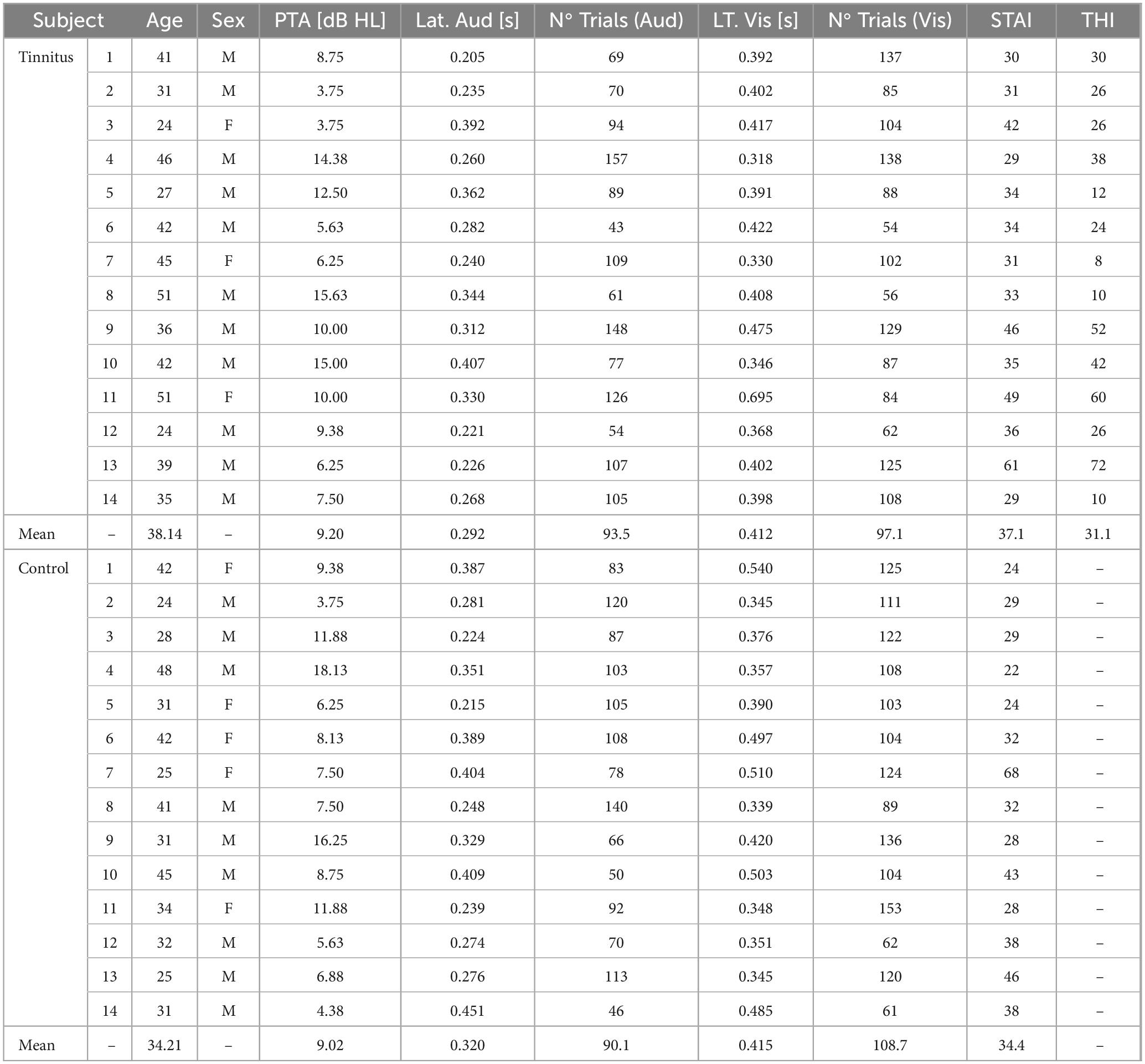
Table 1. Summary of individual demographic, audiological and behavioral data in control (n = 14) and tinnitus (n = 14) subjects.
Behavioral responses
In relation to the behavioral performance, the mean response latency to the auditory target (silent gap in DPOAE) was shorter (306 ± 73 ms) than the mean response latency to the visual cue (413 ± 82 ms; p < 0.01, t-test). When comparing the behavioral performance between tinnitus and control groups, we found non-significant differences in the mean response latencies to the visual and auditory targets. Tinnitus [auditory task: 292 ± 46 ms; visual task: 412 ± 33 ms] and control groups [auditory task: 320 ± 40 ms (p = 0.32, t-test); visual task: 415 ± 33 ms (p = 0.92, t-test)] (Table 1 and Figure 3).
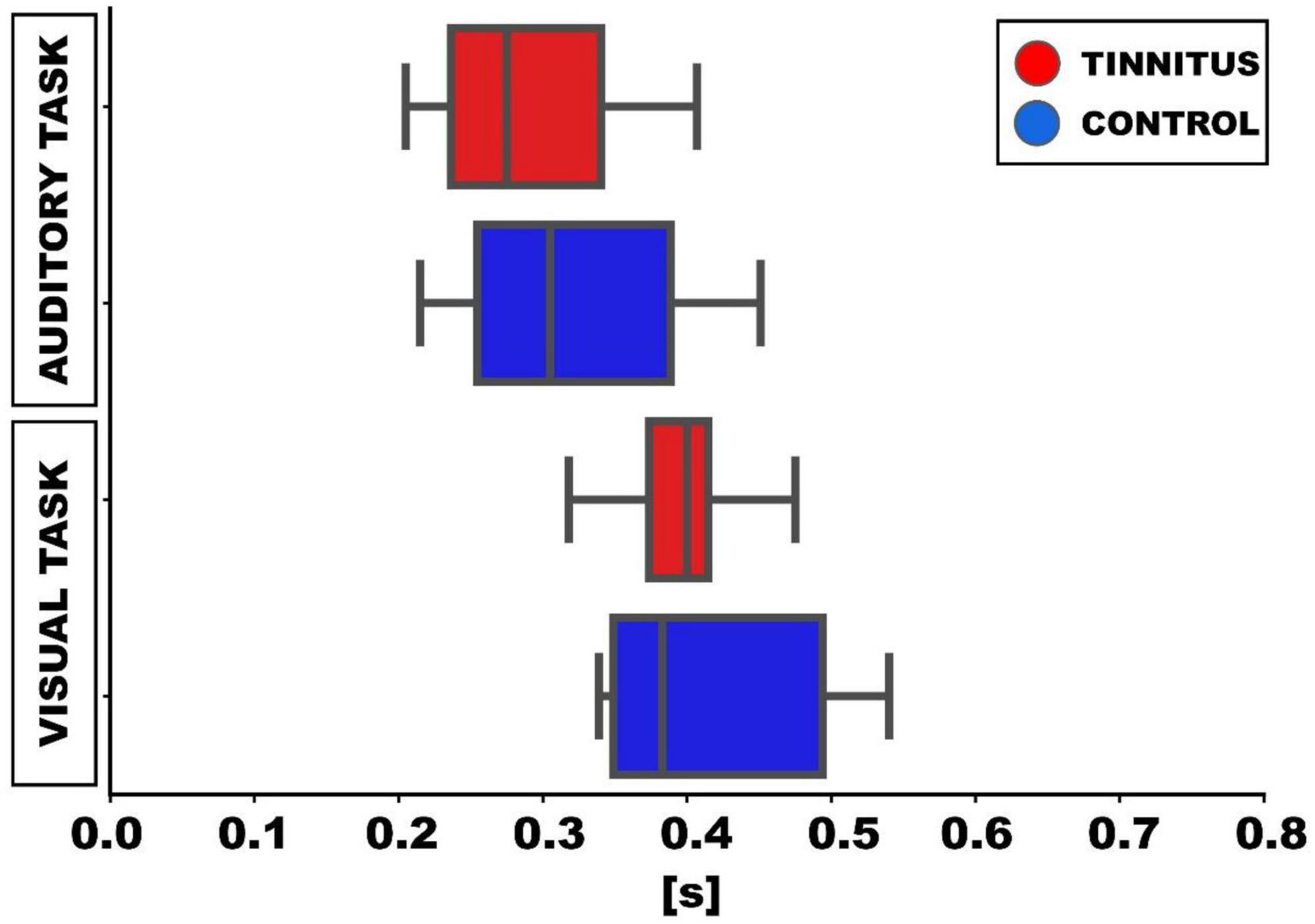
Figure 3. Box-plots showing the median latencies of responses to visual and auditory targets in the behavioral task. In the auditory task, the latencies for detecting the gap in DPOAE are shown. Meanwhile, in the visual task in the visual task, the latencies correspond to the report of the visual cue (green circle) offset.
We performed circular analyses statistics of the behavioral responses during the visual selective attention period (angular responses). The 14 subjects of the tinnitus group contributed with 2,388 responses, while 14 individuals of the control group provided 2,383 angular responses. The mean angle for the tinnitus group was 0.98° with a confidence interval (CI-95%) of [−1.10°, 3.07°], while for the control group was −5.03° with CI-95% of [−6.79°,−3.26°]. Note that the confidence intervals do not overlap. The Watson-Williams test, a circular analog of the two-sample t-test shows that the differences in angular responses between controls and tinnitus groups was significant (p = 6.24 × 10–6).
The resultant vector length of the circle distribution of behavioral responses in the visual task can be used as a measure of dispersion (of value 1 when all responses are associated with the same angle), being 0.68 for the tinnitus group and 0.77 for the controls. We used the Von Mises distribution (μ: location, the data is clustered around this value; κ: concentration, the reciprocal measure of dispersion) to fit data. The values for the tinnitus group were (μT, κT) = (0.98°, 1.90), and for controls (μC, κC) = (−5.03°, 2.57). κT < κC indicates that the control group data are more concentrated around μC, and therefore we can conclude that the individuals in the tinnitus group presented less precise responses than controls in the visual task. The normalized polar histograms for both groups can be found in Figure 1B.
Oscillatory activity
Next, we evaluated the oscillatory activity in EEG and DPOAE signals during auditory and visual attention periods in the control and tinnitus groups. Similar to our previous work (Dragicevic et al., 2019), we selected four regions of interest (ROIs), including Cz, Fz, O1, and DPOAE channels for frequency analyses. In agreement with previous works (Dragicevic et al., 2019; Köhler et al., 2021; Gehmacher et al., 2022), the grand average of the EEG and DPOAE spectra confirmed the presence of low-frequency oscillations in theta and delta frequency bands (< 10 Hz) during the auditory and visual attention periods in the control and tinnitus groups (Figure 4).
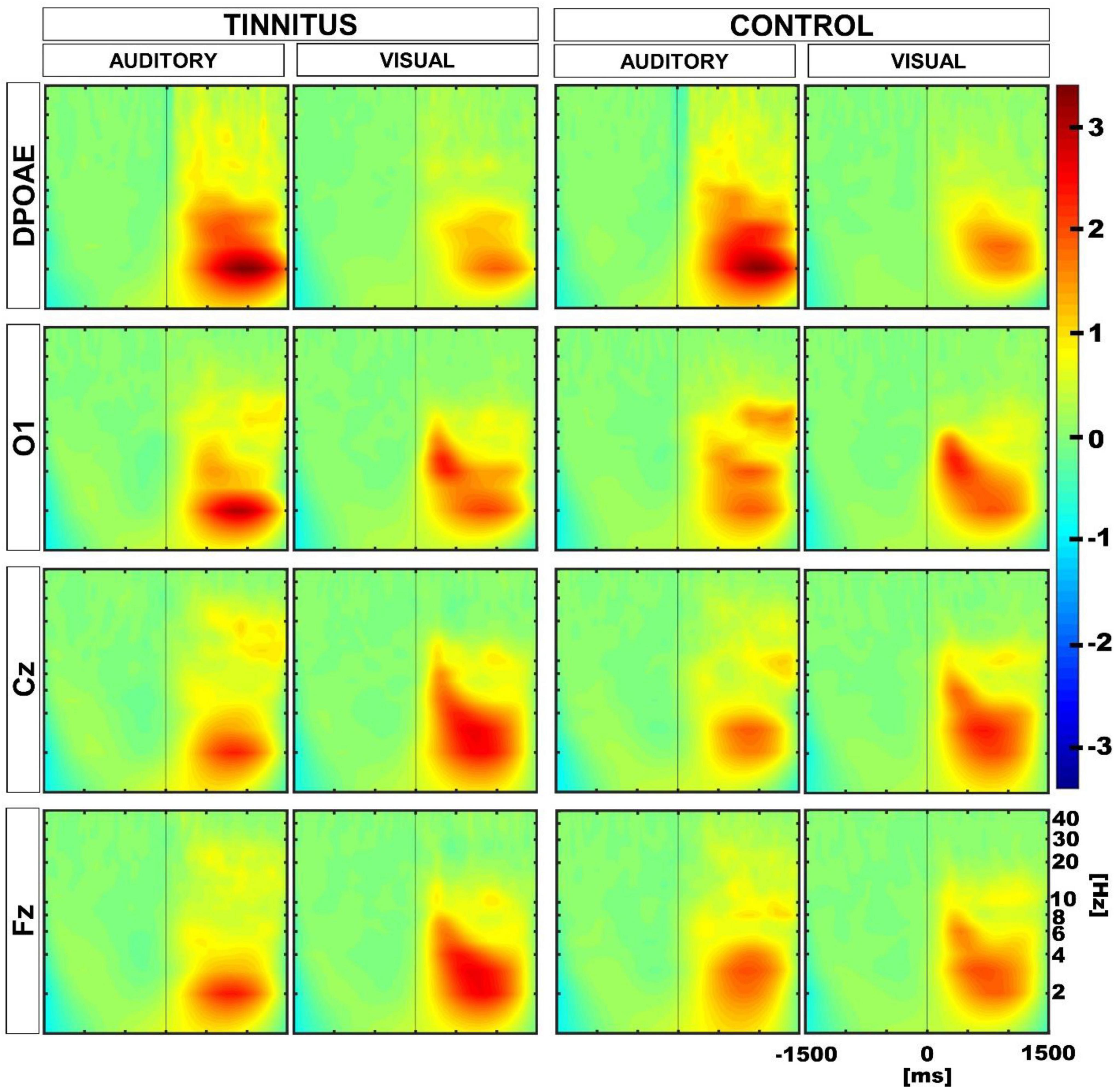
Figure 4. Grand average time spectra calculated for control and tinnitus groups during visual and auditory attention tasks. The color intensity scale was normalized to z-scores in all conditions. The vertical line in the panels illustrate time “0” in auditory and visual tasks. Note the presence of low-frequency oscillatory activity in theta and delta bands (< 10 Hz) in EEG and DPOAE channels during the selective attention period (from 0 to 1,500 ms). A significant reduction in the magnitude of the oscillatory activity in the cochlear channel (DPOAE) was observed in control and tinnitus groups during the visual attention period as compared to the auditory attention period. An increase in the theta power is observed in the Fz channel during the visual attention period in tinnitus.
Oscillatory power in the 1–8 Hz band in the auditory versus visual attention periods
To evaluate differences in the oscillatory power of the delta and theta frequency bands in the ROIs between the auditory and visual attention periods, we considered the auditory and visual trials in the 1–8 Hz frequency band in the period between 200 and 1,500 ms after the cue onset. The choice of the temporal interval was made after inspection of the individual time-frequency charts, noting that the highest magnitude oscillatory activity was located mainly after 200 ms. Performing a paired t-test, we found a significant reduction of the oscillatory activity in the DPOAE channel during the visual attention period in the control and tinnitus groups, compared to the oscillatory activity in the auditory attention period (DPOAE-tinnitus: visual: 0.632, auditory: 1.185, p = 0.0256; DPOAE-control: visual: 0.638, auditory: 1.255, p = 0.0483; values on Z-score). Using the same criteria, we found a significant increase in the oscillatory power during the visual period in the Fz EEG channel for the tinnitus group (Fz-tinnitus: visual: 1.030, auditory: 0.688, p = 0.0483; values on Z-score), but not for the control group (Fz-control: visual: 0.827, auditory: 0.815, p = 0.4775). In addition, we found significant increases in the oscillatory power in Cz channels for the visual attention period in both groups (Cz-tinnitus: visual: 1.051, auditory: 0.688, p = 0.0456; Cz-control: visual: 0.940, auditory: 0.619, p = 0.0348; values on Z-score). There were non-significant differences between visual and auditory oscillatory amplitudes in the occipital channel in both groups (O1-tinnitus: visual: 0.878, auditory: 0.834, p = 0.4399; O1-control: visual: 0.847, auditory: 0.875, p = 0.4399; values on Z-score).
Oscillatory power in the 1–8 Hz band between control and tinnitus
Afterward, we compared group differences between tinnitus and controls performing two-sample t-tests. We found a significant difference in the Fz channel for the visual task with a higher value for the tinnitus group (visual: Fz-tinnitus: 1.030, Fz-control: 0.827, p = 0.040; values on Z-score. p = 0.32 after correction), however, after correction for multiple comparisons, this result was not significant. All the other comparisons between ROIs in tinnitus and control groups were not significant.
Permutation tests to compare individual differences
Next, to evaluate whether the differences observed in the amplitude of DPOAE and EEG low-frequency oscillations during the auditory and visual attention periods were different in tinnitus and controls at the individual level, we performed permutation tests using a within-subjects approach. Figure 5 shows the results of these permutation tests, uncorrected and corrected for multiple comparisons. Note that after multiple comparison correction, there is a significant positive cluster in the delta band in the DPOAE channel of the tinnitus individuals, illustrating that the suppression of the DPOAE oscillatory activity during visual attention in the delta band is stronger in tinnitus. We also found a significant negative cluster in the theta band in the Cz channel of the control group.
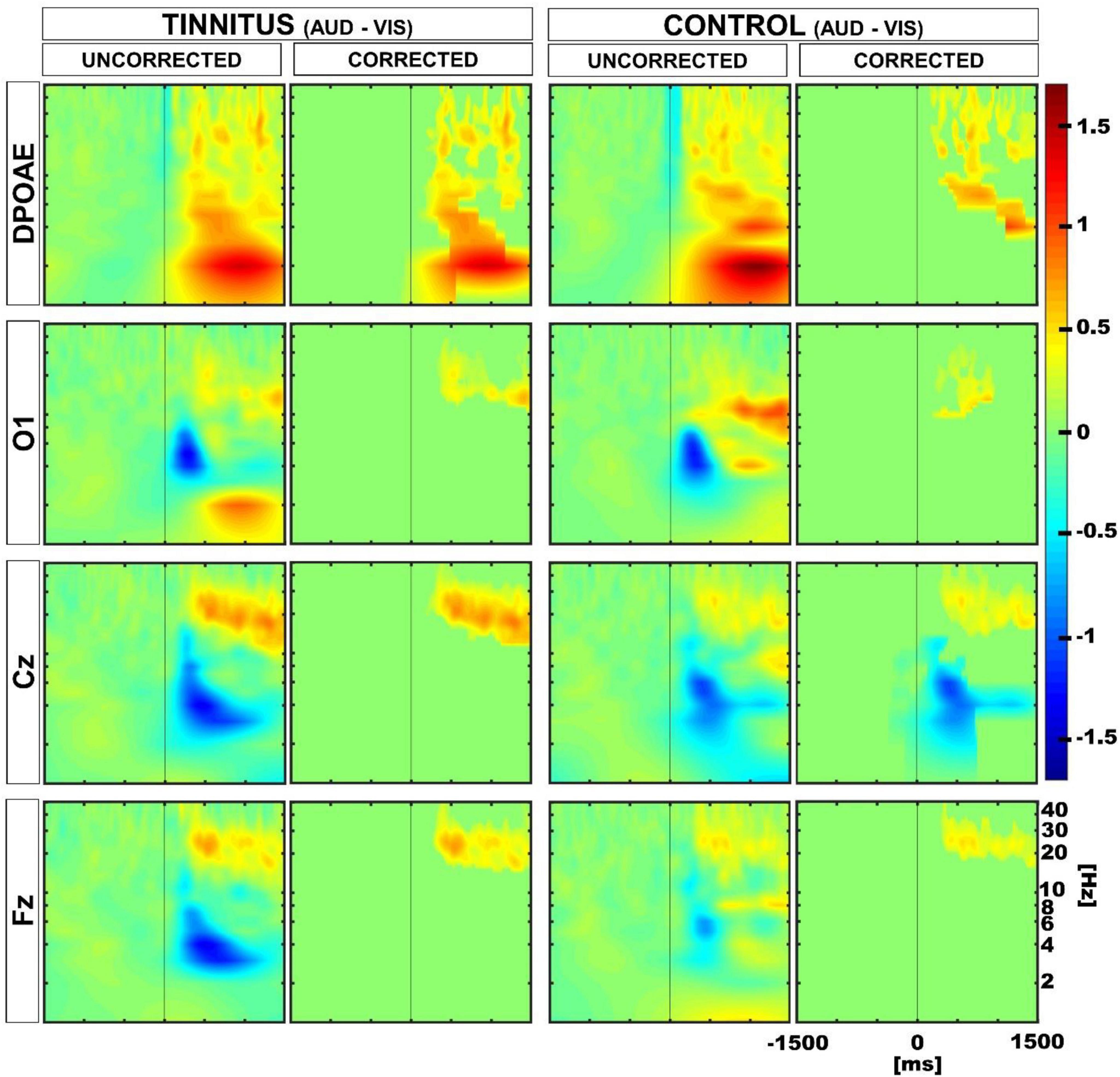
Figure 5. Time spectra average differences between auditory and visual periods using permutation tests in a within subjects approach. Color represents the grand average of the differences in Z-score. Red and blue clusters represent significant differences in the permutation test, while non-significant differences are shown in green. The first and third column show uncorrected results, while the second and fourth illustrate multiple comparison corrected results. Positive significant differences are represented in red (i.e., auditory > visual), while negative differences are illustrated in blue (i.e., auditory < visual). Note that after correction, there is a significant positive cluster in the delta-theta band in the DPOAE channel of the tinnitus group, and a significant negative cluster in the theta band in the Cz channel of the control group.
Afterward, we compared the differences between auditory and visual modalities (aud-vis) for both tinnitus and control groups using a between-subjects approach. Although we initially found distinctions in the delta and theta bands between the control and tinnitus groups, subsequent multiple comparisons corrections revealed that these differences were non-significant (Figure 6).
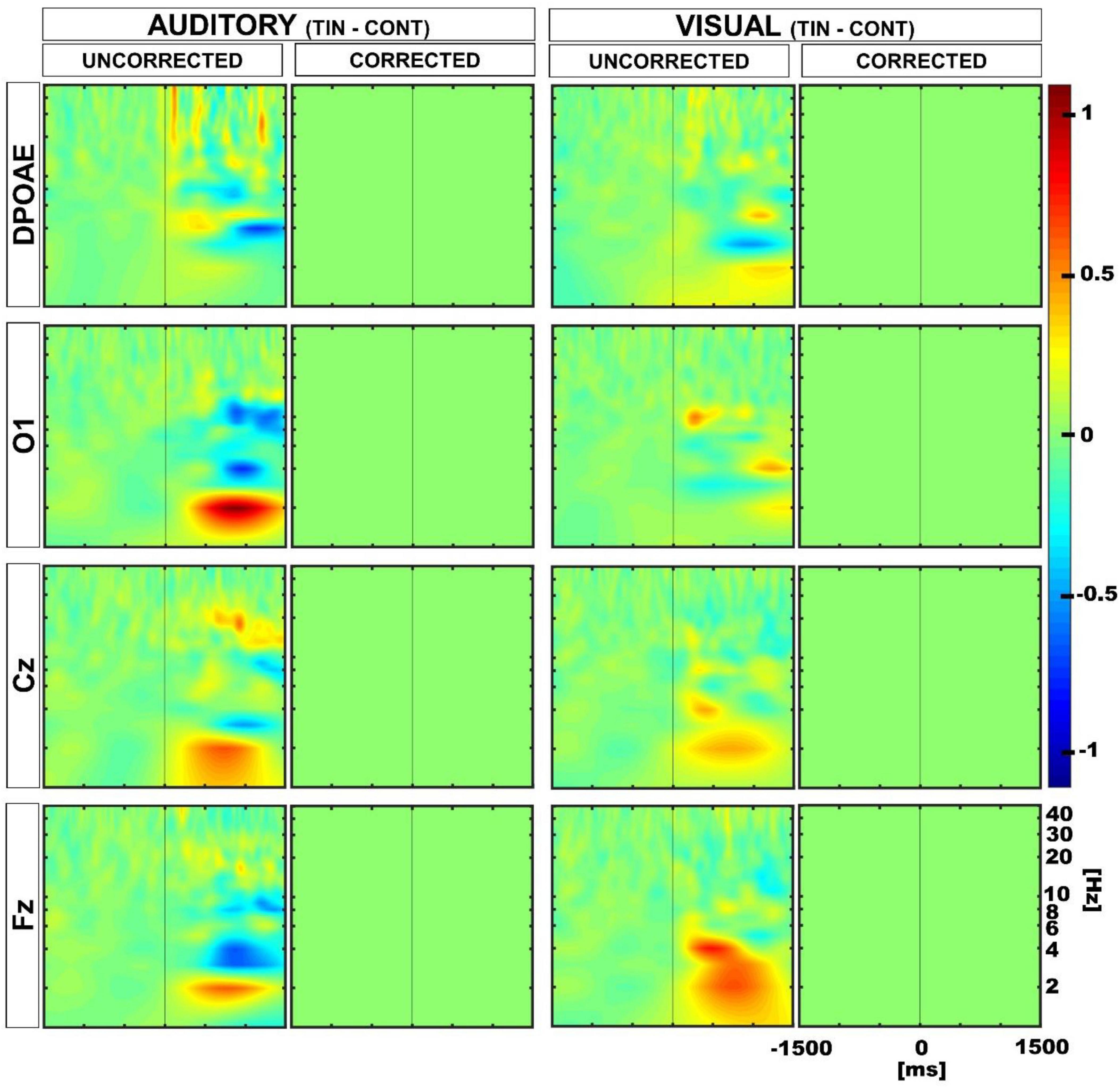
Figure 6. Time spectra average differences between auditory and visual periods using permutation tests in a between subjects approach. Color represents the grand average of the differences in Z-score. The first and third columns show uncorrected results, while the second and fourth columns illustrate multiple comparison corrected results. The uncorrected results reveal differences in the delta and theta bands in EEG channels, whereas the multiple comparison corrected analyses yielded non-significant differences (non-significant differences are depicted in green).
Time course of the oscillatory changes in the 1–8 Hz frequency band
Finally, we explored the temporal course of the oscillatory power of the 1–8 Hz band in the auditory and visual attentional periods, in control and tinnitus groups. Figure 7 displays the grand averages of the temporal course of the power increase in the 1–8 Hz band (mean ± SEM, 14 tinnitus, 14 controls for each modality) for the DPOAE, O1, Cz, and Fz channels. To assess the differences between the temporal profiles of each channel, we performed a curve slope analysis, computing the mean slope values between 0 ms and the point where the slope began to decrease. The analysis showed that for the auditory modality, the mean slopes were steeper for the DPOAE channel in both groups compared to the mean slope for the EEG channels [tinnitus mean slope and p-value for DPOAE vs. EEG channel: DPOAE: 0.0023, O1: 0.0017 (p = 0.162), Cz: 0.0014 (p = 0.047), Fz: 0.0013 (p = 0.015); control mean slope and p-value for DPOAE vs. EEG channel: DPOAE: 0.0026, O1: 0.0017 (p = 0.0183), Cz: 0.0014 (p = 0.0007), Fz: 0.0016 (p = 0.0031)]. Regarding the visual modality, the mean DPOAE slope values were lower than the mean slope of EEG channels in both groups [tinnitus mean slope and p-value for DPOAE vs. EEG channel: DPOAE: 0.0011, O1: 0.0035 (p = 0.00031), Cz: 0.0033 (p = 0.00004), Fz: 0.0030 (p = 0.00006); control mean slope and p-value for DPOAE vs. EEG channel: DPOAE: 0.0014, O1: 0.0036 (p = 0.0003), Cz: 0.0027 (p = 0.0013), Fz: 0.0023 (p = 0.0348)].
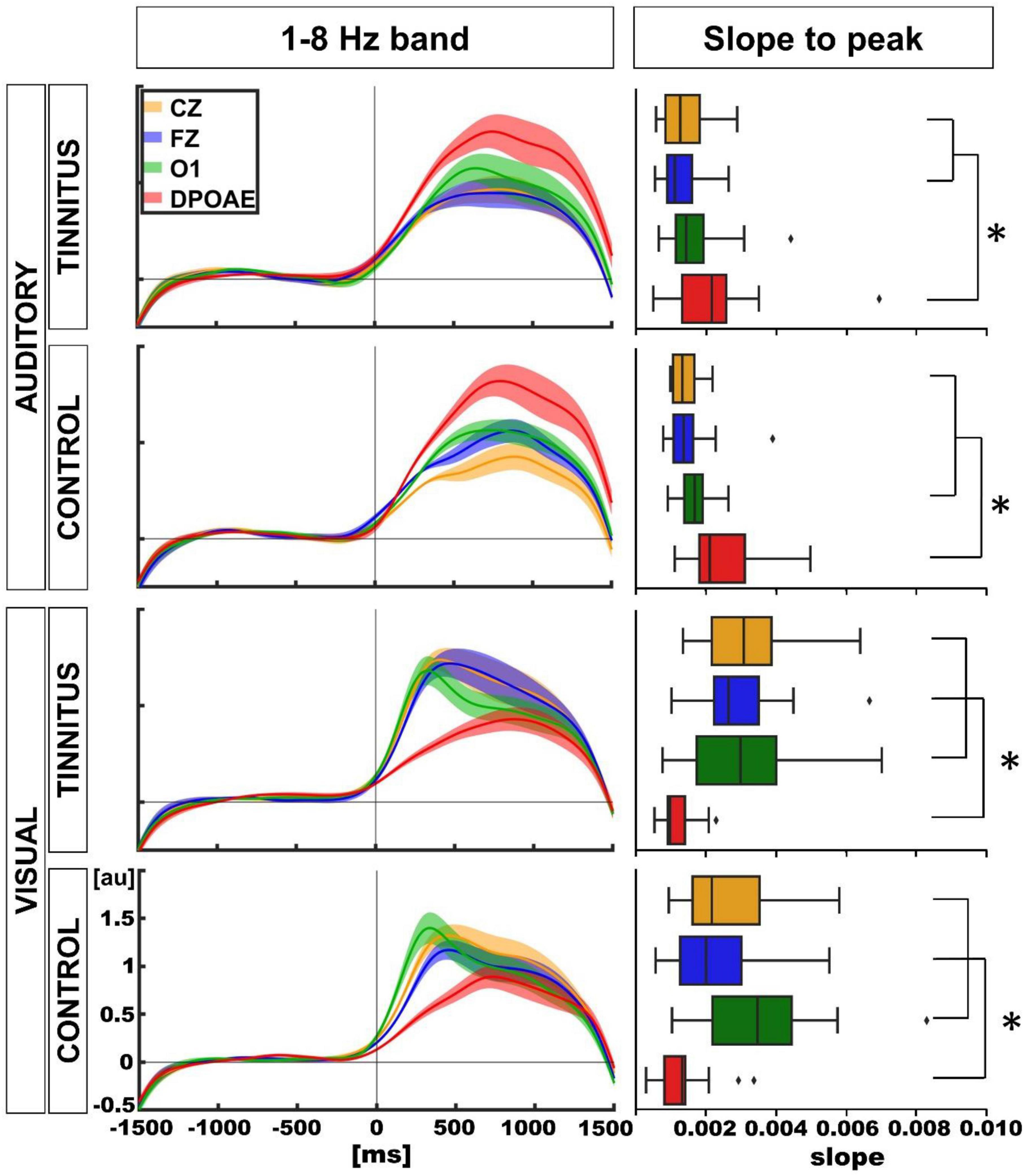
Figure 7. Temporal course of the amplitude of 1–8 Hz frequency band between EEG and DPOAE channels and comparison of slope values between individuals (*p < 0.05).
Mean slope differences comparisons between tinnitus and control groups for visual and auditory modalities were non-significant (auditory: tinnitus vs. control: DPOAE: p = 0.22, O1: p = 0.27, Cz: p = 0.47, Fz: p = 0.13; visual: tinnitus vs. control: DPOAE: p = 0.35, O1: p = 0.30, Cz: p = 0.16, Fz: p = 0.10).
Discussion
We found that cortical and cochlear oscillatory activity in the delta/theta bands were present in the tinnitus group, and similar to the control group, there was a significant decrease in DPOAE oscillatory amplitude during the visual attention period as compared to the auditory attention period. We did not find significant clusters when using a between-subjects approach to directly compare between tinnitus and control groups. On the other hand, we found a significant cluster in the delta band in tinnitus when using within-group statistics to compare the difference between auditory and visual DPOAE oscillatory power. Finally, there was a significant difference in the temporal course of the oscillatory power ascending slope (in the 1–8 Hz band) when comparing the auditory and visual attention periods, but not between control and tinnitus groups.
Behavioral differences between control and tinnitus
Behavioral comparisons between control and tinnitus yielded non-significant differences in the auditory task, and only subtle differences in visual attention. In the latter task, the mean angle values for both groups showed that subjects in the tinnitus group tended to report their responses before the clock pointer reached the target. In addition, the angular dispersion showed that the control group tended to respond more accurately than the tinnitus group. Together, these results might be explained by the known elevated anxiety levels of tinnitus sufferers (Kleinstäuber and Weise, 2020; Chen et al., 2023). The literature reports that tinnitus sufferers have psychological profiles showing more anxiety and with greater distractibility than the general population (Araneda et al., 2015; Kleinstäuber and Weise, 2020; Chen et al., 2023). However, unexpectedly, our analysis of the anxious traits (STAI) did not show significant differences between the two groups. This might be explained by the high level of anxiety observed in the general population, especially after the COVID-19 pandemic (Phalswal et al., 2023), which might have masked anxiety differences between control and tinnitus.
Low-frequency cochlear oscillations are suppressed during visual attention
Previous works using DPOAE, external ear canal pressure and cochlear implant low frequency signals have evidenced the presence of low-frequency oscillations in the delta and theta bands at the most peripheral level of the auditory pathway during selective attention paradigms (Dragicevic et al., 2019; Köhler et al., 2021; Gehmacher et al., 2022; Köhler and Weisz, 2023). Here, we used the same paradigm as Dragicevic et al., 2019, confirming the presence of these infrasonic oscillations in a new cohort of volunteers with and without tinnitus. Moreover, our data also confirmed a reliable suppressive effect of cochlear oscillatory activity during visual attention as compared to the auditory modality (Dragicevic et al., 2019; Köhler et al., 2021; Gehmacher et al., 2022). Altogether, these results show that the magnitude of cochlear oscillatory activity (delta and theta frequency bands) depends on the attentional modality, with increased amplitude during auditory attention and diminished amplitude with visual attention. Whether this effect is limited to the attentional mechanisms or is a more general cognitive mechanism is still an open question (Marcenaro et al., 2021).
Oscillatory power in the 1–8 Hz band in the between control and tinnitus
The next question was whether cochlear and cortical oscillatory activity were altered in tinnitus patients as compared to control patients during visual and auditory attention. We used two approaches, (i) a comparison between control and tinnitus groups in the different conditions, and (ii) a second approach using within-subjects permutation tests for studying individual differences in tinnitus and control subjects between the auditory and visual attention periods.
All significant differences at the DPOAE level were found when using the within-subjects approach, while when using the group-average approach there were non-significant differences between controls and tinnitus. These findings indicate that corticofugal oscillatory modulation of the cochlear receptor during auditory and visual attention is preserved in the tinnitus group. Only when using an individual comparison (within subject approach), the difference between the cochlear oscillatory activity during auditory and visual attention conditions in the delta band was stronger for the tinnitus group (Figure 5, corrected DPOAE spectrograms). A speculative explanation for this finding could be the presence of a compensatory top-down mechanism for suppressing the phantom sound. Importantly, this result was concomitant to an increase in the theta band in frontal regions, which has been reported in more severe tinnitus (Czornik et al., 2022). A speculative explanation could be that the higher oscillatory activity in the theta band in the frontal region might indicate a greater compensatory top-down suppression to the cochlear receptor in the delta band during visual attention in tinnitus patients. However, this proposition needs to be tested in future experiments, including a task that does not demand top-down attention.
Time course of the oscillatory changes in the 1–8 Hz frequency band
Finally, we studied the temporal course of the oscillatory amplitudes changes by measuring the differences in slopes along time of EEG and DPOAE oscillations. Our findings confirmed a temporal shift of the ascending phase of the cochlear oscillatory activity in the low frequency band with auditory and visual attention (Dragicevic et al., 2019), showing that during auditory attention, brain oscillations were preceded by the cochlear oscillations, while during visual attention, brain oscillatory activity precedes cochlear oscillations (Figure 7). These results are indicative of a peripheral correlate of the attentional switch at the delta-theta band that occurs in the transition between the auditory and visual modality. Importantly a similar neural correlate of attentional switching has been obtained by other groups in EEG recordings (Phillips et al., 2014; Wang et al., 2016). On the other hand, when comparing the slopes of the temporal course of EEG and DPOAE low-frequency oscillations in tinnitus and control groups, we did not find significant differences, suggesting that the temporal dynamics of these oscillatory activity was not disrupted in tinnitus sufferers.
Limitations
Our study has a relatively small sample size, and consequently conclusions should be taken carefully. More studies on tinnitus with larger sample sizes are needed to clarify whether the corticofugal oscillatory modulation of cochlear responses is enhanced (as suggested by results in Figure 5) in a specific subset of tinnitus patients or in other perceptual (e.g., residual inhibition of tinnitus) or cognitive (e.g., working memory) conditions.
Conclusion
We confirm the presence of infrasonic low-frequency cochlear oscillations in the delta and theta bands in tinnitus patients, evidencing that the corticofugal suppression of cochlear oscillations during visual attention is preserved in the tinnitus group.
Data availability statement
The raw data supporting the conclusions of this article will be made available by the authors, without undue reservation.
Ethics statement
The studies involving humans were approved by the Institutional Scientific Ethic Committee of the Clinical Hospital of the Universidad de Chile. The studies were conducted in accordance with the local legislation and institutional requirements. The participants provided their written informed consent to participate in this study.
Author contributons
RD-S: Writing – original draft, Conceptualization, Data curation, Formal analysis, Investigation, Methodology, Project administration, Supervision, Validation, Visualization, Writing – review & editing. AL: Writing – review & editing, Investigation, Project administration. CD: Writing – review & editing, Conceptualization, Formal analysis, Methodology, Software, Supervision, Validation, Funding acquisition. VM: Writing – review & editing, Conceptualization, Methodology, Validation, Funding acquisition. PHD: Writing – review & editing, Conceptualization, Data curation, Funding acquisition, Investigation, Methodology, Project administration, Supervision, Validation, Writing – original draft.
Funding
The authors declare financial support was received for the research, authorship, and/or publication of this article. This research was funded by ANID Proyecto Fondecyt Regular 1220607 to PHD, Fondecyt Postdoctorado 3230557 to VM, Fondecyt Postdoctorado 3200735 to CD. Proyecto Basal ANID FB0008, Proyecto Milenio ICN09_015, and Fundación Guillermo Puelma to PHD.
Acknowledgments
We would like to thank Fernando Vergara for his technical contributions.
Conflict of interest
The authors declare that the research was conducted in the absence of any commercial or financial relationships that could be construed as a potential conflict of interest.
Publisher’s note
All claims expressed in this article are solely those of the authors and do not necessarily represent those of their affiliated organizations, or those of the publisher, the editors and the reviewers. Any product that may be evaluated in this article, or claim that may be made by its manufacturer, is not guaranteed or endorsed by the publisher.
References
Aguera, P., Jerbi, K., Caclin, A., and Bertrand, O. (2011). ELAN: A software package for analysis and visualization of MEG, EEG, and LFP signals. Comput. Intell. Neurosci. 2011:158970. doi: 10.1155/2011/158970
Araneda, R., De Volder, A., Deggouj, N., and Renier, L. (2015). Altered inhibitory control and increased sensitivity to cross-modal interference in tinnitus during auditory and visual tasks. PLoS One 10:e0120387. doi: 10.1371/journal.pone.0120387
Berens, P. (2009). CircStat: A MATLAB toolbox for circular statistics. J. Stat. Softw. 31, 1–21. doi: 10.18637/jss.v031.i10
Buzsáki, G., and Draguhn, A. (2004). Neuronal oscillations in cortical networks. Science 304, 1926–1929. doi: 10.1126/science.1099745
Chen, X., Ren, L., Xue, X., Yu, N., Liu, P., Shen, W., et al. (2023). The comorbidity of depression and anxiety symptoms in tinnitus sufferers: A network analysis. Brain Sci. 13:583. doi: 10.3390/brainsci13040583
Czornik, M., Birbaumer, N., Braun, C., Hautzinger, M., Wolpert, S., Löwenheim, H., et al. (2022). Neural substrates of tinnitus severity. Int. J. Psychophysiol. 181, 40–49. doi: 10.1016/j.ijpsycho.2022.08.009
De Ridder, D., Schlee, W., Vanneste, S., Londero, A., Weisz, N., Kleinjung, T., et al. (2021). Tinnitus and tinnitus disorder: Theoretical and operational definitions (an international multidisciplinary proposal). Prog. Brain Res. 260, 1–25. doi: 10.1016/bs.pbr.2020.12.002
Delano, P., Elgueda, D., Hamame, C., and Robles, L. (2007). Selective attention to visual stimuli reduces cochlear sensitivity in chinchillas. J. Neurosci. 27, 4146–4153. doi: 10.1523/JNEUROSCI.3702-06.2007
Dragicevic, C., Marcenaro, B., Navarrete, M., Robles, L., and Delano, P. (2019). Oscillatory infrasonic modulation of the cochlear amplifier by selective attention. PLoS One 14:e0208939. doi: 10.1371/journal.pone.0208939
Elgoyhen, A., Langguth, B., De Ridder, D., and Vanneste, S. (2015). Tinnitus: Perspectives from human. Nat. Rev. Neurosci. 16, 632–642. doi: 10.1038/nrn4003
Elgueda, D., and Delano, P. (2020). Corticofugal modulation of audition. Curr. Opin. Physiol. 18, 73–78. doi: 10.1016/j.cophys.2020.08.016
Gehmacher, Q., Reisinger, P., Hartmann, T., Keintzel, T., Rösch, S., Schwarz, K., et al. (2022). Direct cochlear recordings in humans show a theta rhythmic modulation of auditory nerve activity by selective attention. J. Neurosci. 42, 1343–1351. doi: 10.1523/JNEUROSCI.0665-21.2021
Geocze, L., Mucci, S., Abranches, D., Marco, M., and Penido Nde, O. (2013). Systematic review on the evidences of an association between tinnitus and depression. Braz. J. Otorhinolaryngol. 79, 106–111. doi: 10.5935/1808-8694.20130018
Husain, F. (2016). Neural networks of tinnitus in humans: Elucidating severity and habituation. Hear. Res. 334, 37–48. doi: 10.1016/j.heares.2015.09.010
Khan, R. A., and Husain, F. T. (2020). Tinnitus and cognition: Can load theory help us refine our understanding? Laryngosc. Investig. Otolaryngol. 5, 1197–1204. doi: 10.1002/lio2.501
Kleinstäuber, M., and Weise, C. (2020). “Psychosocial variables that predict chronic and disabling tinnitus: A systematic review,” in The Behavioral Neuroscience of Tinnitus, eds G. D. Searchfield and J. Zhang (Cham: Springer International Publishing), 361–380.
Knipper, M., Mazurek, B., van Dijk, P., and Schulze, H. (2021). Too blind to see the elephant? Why neuroscientists ought to be interested in tinnitus. J. Assoc. Res. Otolaryngol. 22, 609–621. doi: 10.1007/s10162-021-00815-1
Knipper, M., van Dijk, P., Schulze, H., Mazurek, B., Krauss, P., Scheper, V., et al. (2020). The neural bases of tinnitus: Lessons from deafness and cochlear implants. J. Neurosci. 40, 7190–7202. doi: 10.1523/JNEUROSCI.1314-19.2020
Köhler, M., Demarchi, G., and Weisz, N. (2021). Cochlear activity in silent cue-target intervals shows a theta-rhythmic pattern and is correlated to attentional alpha and theta modulations. BMC Biol. 19:48. doi: 10.1186/s12915-021-00992-8
Köhler, M., and Weisz, N. (2023). Cochlear theta activity oscillates in phase opposition during interaural attention. J. Cogn. Neurosci. 35, 588–602. doi: 10.1162/jocn_a_01959
Lauer, A., Jimenez, S., and Delano, P. (2022). Olivocochlear efferent effects on perception and behavior. Hear. Res. 419:108207. doi: 10.1016/j.heares.2021.108207
Marcenaro, B., Leiva, A., Dragicevic, C., López, V., and Delano, P. (2021). The medial olivocochlear reflex strength is modulated during a visual working memory task. J. Neurophysiol. 125, 2309–2321. doi: 10.1152/jn.00032.2020
Maris, E., and Oostenveld, R. (2007). Nonparametric statistical testing of EEG- and MEG-data. J. Neurosci. Methods 164, 177–190. doi: 10.1016/j.jneumeth.2007.03.024
McCormack, A., Edmondson-Jones, M., Somerset, S., and Hall, D. (2016). A systematic review of the reporting of tinnitus prevalence and severity. Hear. Res. 337, 70–79. doi: 10.1016/j.heares.2016.05.009
Oatman, L. (1971). Role of visual attention on auditory evoked potentials in unanesthetized cats. Exp. Neurol. 32, 341–356. doi: 10.1016/0014-4886(71)90003-3
Phalswal, U., Pujari, V., Sethi, R., and Verma, R. (2023). Impact of social media on mental health of the general population during Covid-19 pandemic: A systematic review. J. Educ. Health Promot. 12:23. doi: 10.4103/jehp.jehp_460_22
Phillips, J., Vinck, M., Everling, S., and Womelsdorf, T. (2014). A long-range fronto-parietal 5- to 10-Hz network predicts “top-down” controlled guidance in a task-switch paradigm. Cereb. Cortex 24, 1996–2008. doi: 10.1093/cercor/bht050
Riga, M., Katotomichelakis, M., and Danielides, V. (2015). The potential role of the medial olivocochlear bundle in the generation of tinnitus: Controversies and weaknesses in the existing clinical studies. Otol. Neurotol. 36, 201–208. doi: 10.1097/MAO.0000000000000384
Roberts, L., Husain, F., and Eggermont, J. (2013). Role of attention in the generation and modulation of tinnitus. Neurosci. Biobehav. Rev. 37, 1754–1773. doi: 10.1016/j.neubiorev.2013.07.007
Srinivasan, S., Keil, A., Stratis, K., Woodruff Carr, K., and Smith, D. (2012). Effects of cross-modal selective attention on the sensory periphery: Cochlear sensitivity is altered by selective attention. Neuroscience 223, 325–332. doi: 10.1016/j.neuroscience.2012.07.062
Terreros, G., and Delano, P. (2015). Corticofugal modulation of peripheral auditory responses. Front. Syst. Neurosci. 9:134. doi: 10.3389/fnsys.2015.00134
Terreros, G., Jorratt, P., Aedo, C., Elgoyhen, A., and Delano, P. (2016). Selective attention to visual stimuli using auditory distractors is altered in alpha-9 nicotinic receptor subunit knock-out mice. J. Neurosci. 36, 7198–7209. doi: 10.1523/JNEUROSCI.4031-15.2016
Wang, W., Viswanathan, S., Lee, T., and Grafton, S. (2016). Coupling between theta oscillations and cognitive control network during cross-modal visual and auditory attention: Supramodal vs modality-specific mechanisms. PLoS One 11:e0158465. doi: 10.1371/journal.pone.0158465
Warr, W., and Guinan, J. Jr. (1979). Efferent innervation of the organ of corti: Two separate systems. Brain Res. 173, 152–155. doi: 10.1016/0006-8993(79)91104-1
Wittekindt, A., Kaiser, J., and Abel, C. (2014). Attentional modulation of the inner ear: A combined otoacoustic emission and EEG study. J. Neurosci. 34, 9995–10002. doi: 10.1523/JNEUROSCI.4861-13.2014
Keywords: auditory efferent, corticofugal, tinnitus, EEG, attention, oscillations
Citation: Donoso-San Martín R, Leiva A, Dragicevic CD, Medel V and Delano PH (2024) The corticofugal oscillatory modulation of the cochlear receptor during auditory and visual attention is preserved in tinnitus. Front. Neural Circuits 17:1301962. doi: 10.3389/fncir.2023.1301962
Received: 25 September 2023; Accepted: 08 December 2023;
Published: 04 January 2024.
Edited by:
Cristina Martins E. Silva, Universidade Federal do Espírito Santo, BrazilReviewed by:
Calvin Wu, University of Michigan, United StatesMario Treviño, University of Guadalajara, Mexico
Copyright © 2024 Donoso-San Martín, Leiva, Dragicevic, Medel and Delano. This is an open-access article distributed under the terms of the Creative Commons Attribution License (CC BY). The use, distribution or reproduction in other forums is permitted, provided the original author(s) and the copyright owner(s) are credited and that the original publication in this journal is cited, in accordance with accepted academic practice. No use, distribution or reproduction is permitted which does not comply with these terms.
*Correspondence: Paul H. Delano, cGRlbGFub0BoY3VjaC5jbA==