- 1Leicester Medical School, University of Leicester, Leicester, United Kingdom
- 2Department of Audio-Vestibular Sciences, Institute of Health Sciences, Bhubaneswar, India
- 3Department of Psychology, Royal Holloway University of London, Egham, United Kingdom
- 4Department of Health and Human Performance, University of Houston, Houston, TX, United States
- 5University College London Medical School, London, United Kingdom
- 6Library and Learning Services, University of Leicester, Leicester, United Kingdom
- 7Cardiff School of Technologies, Cardiff Metropolitan University, Cardiff, United Kingdom
- 8San Jose State University-NASA Ames Research Center, Moffett Field, CA, United States
- 9Department of Psychological Sciences, Birkbeck University of London, London, United Kingdom
Introduction: Space Motion Sickness (SMS) is a syndrome that affects around 70% of astronauts and includes symptoms of nausea, dizziness, fatigue, vertigo, headaches, vomiting, and cold sweating. Consequences range from discomfort to severe sensorimotor and cognitive incapacitation, which might cause potential problems for mission-critical tasks and astronauts and cosmonauts' well-being. Both pharmacological and non-pharmacological countermeasures have been proposed to mitigate SMS. However, their effectiveness has not been systematically evaluated. Here we present the first systematic review of published peer-reviewed research on the effectiveness of pharmacological and non-pharmacological countermeasures to SMS.
Methods: We performed a double-blind title and abstract screening using the online Rayyan collaboration tool for systematic reviews, followed by a full-text screening. Eventually, only 23 peer-reviewed studies underwent data extraction.
Results: Both pharmacological and non-pharmacological countermeasures can help mitigate SMS symptoms.
Discussion: No definitive recommendation can be given regarding the superiority of any particular countermeasure approach. Importantly, there is considerable heterogeneity in the published research methods, lack of a standardized assessment approach, and small sample sizes. To allow for consistent comparisons between SMS countermeasures in the future, standardized testing protocols for spaceflight and ground-based analogs are needed. We believe that the data should be made openly available, given the uniqueness of the environment in which it is collected.
Systematic review registration: https://www.crd.york.ac.uk/prospero/display_record.php?ID=CRD42021244131.
1. Introduction
Deep space exploration to the Moon and Mars are no longer in the distant future. Since the first space missions, however, it has been clear that exposure to weightlessness (i.e., microgravity) leads to dramatic functional and structural changes in human physiology, including alterations in the musculoskeletal, cardiovascular, and neural systems (Buckey, 2006). Space Motion Sickness (SMS) is a clinical syndrome that affects around 70% of astronauts within the first 72 h of traveling to and returning from microgravity (Heer and Paloski, 2006). SMS symptoms include dizziness, vertigo, headaches, cold sweating, fatigue, nausea, and vomiting. Consequences range from discomfort to severe sensorimotor and cognitive incapacitation. SMS can therefore cause problems during re-entry and emergency exits from a spacecraft; for this reason, no extra-vehicular activities or spacewalks are allowed during the first few days of space missions (Souvestre and Landrock, 2005). Both pharmacological and non-pharmacological countermeasures have been proposed to mitigate SMS symptoms. However, the effectiveness of these countermeasures is still largely debated. The National Aeronautics and Space Administration's (NASA) Human Research Program has recently identified countermeasures against SMS as a critical unaddressed “knowledge gap” (NASA Human Resources Roadmap, 2022). Here we aim to bridge this gap in the knowledge by performing the first systematic review on the effectiveness of pharmacological and non-pharmacological SMS countermeasures. We have collated evidence from published peer-reviewed studies, critically evaluated the current findings, and highlighted potential further research.
Space is a hostile environment: microgravity, extreme temperatures, ionizing radiation and changes in ambient pressure are just some of the stressors space travelers encounter. That is, spaceflight exposes astronauts and cosmonauts to a number of environmental factors that are likely to cause short and long term consequences on human health. For example, exposure to cosmic radiation has dramatic effects on physiological processes (Gundel et al., 1997), and it is associated with a higher risk of cancer and cardiovascular diseases (Townsend, 2005). The lack of atmospheric pressure and solar light severely impacts the neurophysiology processes involved with circadian cycles (Gundel et al., 1997). Microgravity alters different aspects of bodily physiology, including changes in the central nervous system (CNS). Often, these changes occur during and post-flight in the form of neurovestibular problems (Van Ombergen et al., 2017). On Earth, sophisticated organs in the inner ear—the vestibular otoliths—detect gravitational acceleration. When the head moves with respect to terrestrial gravity, the vestibular otoliths shift with the direction of gravitational acceleration, moving the hair cell receptors and signaling to the brain where the head is with respect to the direction of gravity. Vestibular signals are integrated with sensory inputs from vision, and proprioception, to form an internal model of terrestrial gravity (Zago and Lacquaniti, 2005; Jörges and López-Moliner, 2017; Lacquaniti et al., 2017). Critically, the internal model created from integrating multiple sensory sources allows a subject to shape their behavior to adapt successfully to the terrestrial gravity environment.
Neurovestibular changes during spaceflight might account for the onset of SMS symptoms. Egorov and Samarin (1970) have considered the asymmetry in the vestibular otoliths as a potential explanation for SMS. In microgravity the vestibular cues for head tilt become irrelevant and lead to a reinterpretation of physical tilt into a translation sensation by the brain (Russomano et al., 2019). This distortion in vestibular signaling is due to asymmetries between the vestibular utricle and saccule on both sides of the head. Critically, the described physiological asymmetries might be aggravated in microgravity conditions leading the central nervous system to misinterpret the signal transmitted by the otolith organs and potentially lose the usual compensatory dynamics for these asymmetries. This might then induce SMS symptoms.
The fluid shifts induced by microgravity may also contribute to SMS (Parker et al., 1983). In space, bodily fluids (i.e., blood and lymph) are dramatically redistributed to the upper parts of the human body due to the absence of hydrostatic pressure. This fluid shift may affect the balance between endolymphatic and perilymphatic pressures in the vestibular labyrinth, causing SMS symptoms (Parker et al., 1983). According to this model, SMS symptoms such as nausea and vomiting might be triggered by unusual patterns of vestibular activity. Importantly, similar symptoms have been described in patients experiencing changes in vestibular structures, such as labyrinthine hydrops or rising intracranial pressure (Noskov and Grigoriev, 1994). However, an account for SMS solely based on fluids shift does not fully explain the symptoms reported by astronauts and cosmonauts. The most destabilizing effects of SMS last from the first to the fifth day of weightlessness and reoccur within the first 10 days after returning to Earth (Oman et al., 1986). In contrast, the shift in fluids develops immediately after entering into orbit and persists until the end of a mission, suggesting alternative etiologies for SMS (Oman et al., 1986).
So far, the most promising approach explaining SMS focuses on sensory conflict (Kohl, 1983). Similarly to terrestrial motion sickness, vestibular and visual cues for spatial orientation might conflict due to the lack of a gravitational reference. In microgravity, signals from the vestibular system no longer provide direct information about gravitational acceleration, which might affect the processing in the brain areas where sensory integration for orientation takes place between vision, proprioception, and vestibular information (e.g., the thalamus, insular cortex, temporoparietal junction, and somatosensory cortices). Accordingly, exposure to this sensory conflict results in SMS symptoms, vestibular illusions, and spatial disorientation (Weerts et al., 2015).
Individual factors such as age, sex, prior flight experience, and individual susceptibility have been suggested to impact the occurrence and severity of SMS. Few studies have explored these factors in SMS directly. Susceptibility to SMS has been shown to decline with flight experience (Golding et al., 2017). Studies have found that, age negatively correlates with motion sickness susceptibility, and females have a higher susceptibility to motion sickness than males (Dobie et al., 2001; Flanagan et al., 2005; Paillard et al., 2013). Differences in hormonal systems and stress expression may explain individual factors in susceptibility (Kohl, 1983), and hereditary susceptibility (Golding et al., 2005) to motion sickness.
Given its impact on space travelers' health, SMS has received much attention in the past years. While SMS is typically experienced in microgravity environments, ground-based research methods have been used to identify its characteristics and investigate potential countermeasures. These methods include centrifugation, parabolic flights, and rotating environments. Centrifugation creates altered gravities through centrifugal force by circular rotations. Parabolic flights elicit short periods of hypergravity (1.8 g), hypogravity (0.38 g, 0.16 g) and microgravity (0 g) through a series of accelerations and free-fall phases. Rotating environments, such as rotating rooms and chairs, disrupt the visual and vestibular information interpreted by the body and brain. Although these ground simulations cannot perfectly mimic spaceflight conditions, they are effective tools to explore SMS.
Is it possible to overcome SMS? Pharmacological methods of reducing SMS have been proposed and widely investigated. Several drugs from different pharmacological classes and with varying doses have been explored, including promethazine, scopolamine, dimenhydrinate, prochlorperazine, meclizine, metoclopramide, phenytoin, and lorazepam, among others. A reduction in SMS has been observed after consumption of promethazine or scopolamine during spaceflight (Graybiel, 1976, 1981; Oman et al., 1986; Davis et al., 1993a,b). Similar effects have been reported in parabolic flights (Norfleet et al., 1992; Golding et al., 2017), rotating environments (Graybiel, 1979; Hordinsky et al., 1982; Kohl et al., 1993; Lackner and Graybiel, 1994; Cowings et al., 2000; Dornhoffer et al., 2004; Weerts et al., 2012) and centrifugation (Weerts et al., 2015). However, side effects triggered by promethazine and scopolamine have also been reported, including fatigue, drowsiness, dry mouth, and problems with sensorimotor coordination. Clearly, these side effects are particularly detrimental in high-pressure environments where attention and coordination are critical for performance and survival. Some progress has been made to counteract the adverse side effects of SMS drugs, mainly drowsiness, with the addition of stimulants such as amphetamine (Graybiel, 1981; Hordinsky et al., 1982; Davis et al., 1993a; Kohl et al., 1993). However, more research is needed to understand the underlying mechanisms and effectiveness of using this combined pharmacological approach for the symptomatic treatment of SMS.
Alongside pharmacological approaches, non-pharmacological methods have been explored to mitigate SMS symptoms. These include the use of tilt-transition devices (Harm and Parker, 1994), autogenetic feedback training exercises (Cowings and Toscano, 2000), Virtual Reality training (Stroud et al., 2005), head or body rotation exercises (Reschke et al., 2006; Cloutier and Watt, 2007) and Galvanic Vestibular Stimulation (Dilda et al., 2014). These studies have predominantly focused on building tolerance against SMS. These and reported a reduction in SMS or motion sickness-associated symptoms (Cowings and Toscano, 2000). Thus, non-pharmacological countermeasures might be effective as a pre-flight training countermeasure, particularly in building resilience against SMS. However, the number of studies exploring non-pharmacological countermeasures is severely limited.
Here we performed a systematic review of the published peer-reviewed research on the effectiveness of pharmacological and non-pharmacological countermeasures of SMS. The non-pharmacological countermeasures considered in our systematic review included devices that exposed participants to the stimulus challenges of microgravity, biofeedback training, stroboscopic vision, torso rotation and Galvanic Vestibular Stimulation (Harm and Parker, 1994; Cowings and Toscano, 2000; Stroud et al., 2005; Reschke et al., 2006; Cloutier and Watt, 2007; Dilda et al., 2014). We initially identified 3,207 potential peer-reviewed studies containing SMS keywords, which was reduced to 23 peer-reviewed studies for inclusion following systematic screening (17 on pharmacological countermeasures, five on non-pharmacological countermeasures, and one study that compared both approaches). Results indicate that both pharmacological and non-pharmacological countermeasures have had some success in mitigating SMS symptoms. However, no definitive recommendation can be given about whether one type of countermeasure is more effective given the vast heterogeneity of research methods, the lack of a standardized research approach in evaluating outcomes, and the small sample size overall. Nonetheless, this review provides the first systematic summary of all of the peer-reviewed studies on SMS countermeasures. This can help clarify the state-of-the-art and inform future research on this critical unaddressed knowledge gap.
2. Methods
This systematic review was performed under the Preferred Reporting Items for Systematic Reviews and Meta-Analyses (PRISMA) statement (Page et al., 2021). The Librarian (KN), in collaboration with other team members, devised search keywords. Literature searches using Cinahl (via Ebsco), the Cochrane Library, Medline (via Ovid), Scopus, and Web of Science Core Collection databases were performed for relevant articles on 9th April 2021. A follow up search of PubSpace was conducted via PubMed Central on 17th May 2021. Full search strategies are reported in Supplementary material. References from the searches were exported to the Zotero reference management application Zotero (RRID:SCR_013784) to organize references and identify duplicate records, and then exported to Rayyan (Rayyan QCRI (RRID:SCR_017584), a software designed for the screening part of a systematic review through its features to label and sort papers by inclusion or exclusion criterion. Rayyan was therefore used for double-blind screening. A large number of references (3207) were screened for inclusion. Search restrictions were set for peer-reviewed literature, human studies, and the English language. Each article was evaluated for inclusion by four authors (AK, PPP, HEG, and IJ) independently, and all disagreements were resolved by consensus. Out of 3,207 abstracts, 41 full-length articles were screened out, and 23 articles were included as per the inclusion and exclusion criteria stated below. The PRISMA diagram outlining this process is presented in the Supplementary Figure 1.
The inclusion criteria were determined a priori and included specific search terms to ensure all peer-reviewed studies exploring pharmacological and non-pharmacological countermeasures for SMS were captured. Studies outside of our inclusion criteria were rejected, while studies that met the criteria underwent double-blind evaluation. This process ensured that the paper selection was unbiased and systematic. The inclusion criteria for the studies were stated in the form of the Population, Countermeasure, Comparison, Outcomes (PICO) format. They were as follows: (1) P: Adult humans (18 years of age and over) experiencing SMS in altered gravity (spaceflight and ground-based analogs) (2) I: Pharmacological/non-pharmacological (3) C: None (4) O: Miller and Graybiel scale (1968) and subjective self-ratings, or reduced severity/absence of SMS if the countermeasure was done prophylactically (5) Others: Quantitative/Qualitative countermeasure study. The exclusion criteria included: (1) P: People that have had/currently have a stroke, cerebrovascular accidents (CVAs), and peripheral vestibular disorders such as Benign Paroxysmal Positional Vertigo (BPPV), Vestibular Neuritis, Meniere's Disease, Mal de debarquement syndrome); Animal studies, (2) I: None, (3) C: None, (4) O: Terrestrial motion sickness, (5) Others: Non-English Language papers.
The following information was extracted: Title of Paper; Name of the journal; Author (Surname); Year; Funding source (e.g., NASA/European Space Agency [ESA], etc.); Publication Type (Journal article, conference, abstract); Method (Quantitative, Qualitative, Mixed Methods, Other); Altered Gravity Method (Space/Space Analog/Isolation, Confined and Extreme environments [ICE]/Centrifuge/Other); Number of total participants; Number of participants in the control group; Types of participants (Astronauts, cosmonaut, healthy adults, etc.); Participants (Male: Female ratio if available); Participant's age (mean age, standard deviation/range of age); Number of trained participants/naïve; Was any inclusion/exclusion criteria applied to participants? (Yes/No/Maybe); Group allocation; How were participants divided into groups (if applicable); Was there a control group (Yes/No/Not Sure); Duration of exposure in microgravity/altered gravity environment (making sure time is standardized, i.e., all in hours/days/weeks); Measurement timeline (e.g., pre-flight/in-flight/post-flight); How was SMS measured (self-report, biomarkers etc.); Note on any data exclusion and why; Type of countermeasure used for SMS (pharmacological or non-pharmacological); Name of countermeasure used for SMS (if pharmacological, include mode of administration and dose); Duration of countermeasure used for SMS; How was SMS affected (+ meaning reduced, – meaning increased, or = meaning negligible), Double signs with space between them if statistically significant; If effective, specify which symptoms were improved; Any side effects of countermeasure; Any secondary outcomes and if so, main result(s) of this; Advantages of countermeasure; Any limitations of the countermeasure itself. The type of study was also identified.
3. Results
Our systematic review focused on the effectiveness of pharmacological and non-pharmacological countermeasures to counteract SMS. Notably, only one study (Cowings and Toscano, 2000) directly compared both types of countermeasures. Overall, considerable heterogeneity emerged in the characteristics and methodologies of the studies (e.g., sample size, type, and duration of the altered gravity methods and how the effect of SMS was measured). Of the 23 studies, 17 looked at pharmacological countermeasures (Table 1). There were 15 different drugs or drug combinations used, which varied in dosage, timing, and mode of administration. The most studied drugs and their doses were 0.4 mg of oral scopolamine and 25 mg or 50 mg of oral or intramuscular promethazine. Every study reported a beneficial effect on SMS, defined mainly as a reduction in SMS symptoms experienced by participants. In 10 of the 17 studies, this result was reported as statistically significant. Golding et al. (2017) are notable for their study characteristics of a relatively large sample size of 246, including female participants, and choice of parabolic flight over a space analog (Golding et al., 2017). The most common side effect was drowsiness reported with promethazine consumption.
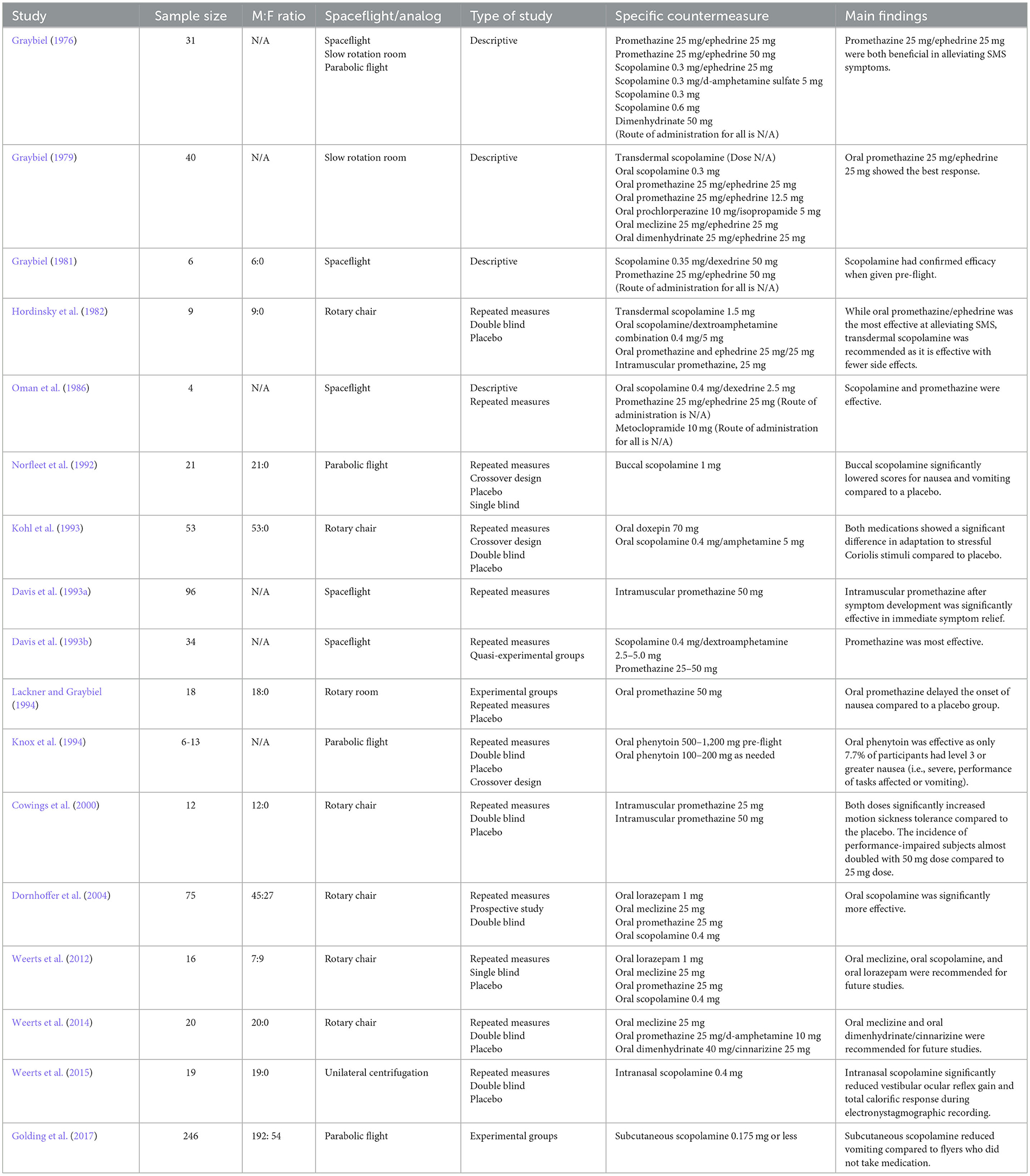
Table 1. Summary of study characteristics for pharmacological countermeasures in chronological order.
Of the 23 studies, six looked at non-pharmacological countermeasures (Table 2). The specific types were devices that exposed participants to the stimulus challenges of microgravity, biofeedback training, stroboscopic vision, torso rotation and Galvanic Vestibular Stimulation (Harm and Parker, 1994; Cowings and Toscano, 2000; Stroud et al., 2005; Reschke et al., 2006; Cloutier and Watt, 2007; Dilda et al., 2014). Unlike the pharmacological studies, all but the stroboscopic vision study carried out their countermeasure prophylactically rather than therapeutically (Harm and Parker, 1994; Cowings and Toscano, 2000; Stroud et al., 2005; Cloutier and Watt, 2007; Dilda et al., 2014). Like the pharmacological studies, each of the six non-pharmacological studies also reported a beneficial effect of SMS by reducing symptoms. In four of them, this result was reported as statistically significant.
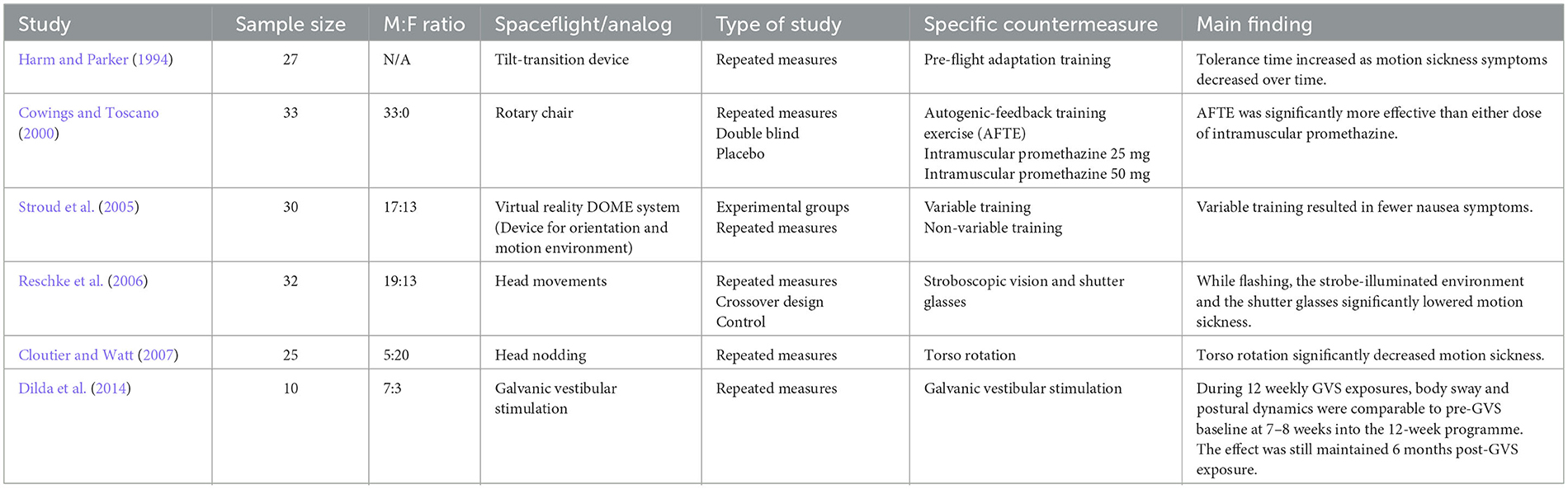
Table 2. Summary of study characteristics for non-pharmacological countermeasures in chronological order.
4. Discussion
Here we have systematically reviewed the available literature to explore the effectiveness of both pharmacological and non-pharmacological countermeasures against SMS. Despite the potential consequences of SMS on crewmember well-being and space mission success, findings remain inconsistent and contradictory in places. While positive results were found in both types of countermeasures, no clear and reliable evidence emerged about which countermeasure is most effective for addressing SMS symptoms. Evidently, across the pharmacological and non-pharmacological studies, a lack of a standardized protocol makes comparisons within and between the approaches almost impossible.
The pharmacology literature dramatically shows the lack of consistency and standardized approaches. Some studies have administered drugs such as scopolamine through transdermal means (Graybiel, 1979; Hordinsky et al., 1982) and others via oral intake (Oman et al., 1986; Davis et al., 1993a; Dornhoffer et al., 2004). Differences in administration may affect uptake and how quickly the drug impacts SMS symptoms. In addition, varying doses were reported in the literature with ambiguity around the time of administration, the number of doses, and how soon after SMS symptoms were measured. Little effort has been made to discriminate when the administration of drugs is most effective in relation to flight times, e.g., pre-, during, or post-flight. Research has also heavily focused on scopolamine and promethazine with little exploration of alternatives, given their side effects. It is, therefore, difficult to conclude which pharmaceutical drug is most effective in reducing SMS and when.
Similarly, drawing concrete conclusions or comparing non-pharmacological countermeasures is also tricky. A noticeable lack of replication or evaluation puts into question the reliability of these countermeasures in reducing SMS. More research and replication are needed, even using ground-based analogs that are more widely and relatively cheaply accessible, to explore the effectiveness of non-pharmacological countermeasures, to assess whether the resistance against SMS is genuinely achieved and whether a consistent reduction of symptoms is experienced.
SMS can be debilitating, and more efforts are needed to address symptoms. More consideration should be given to adopting a combined approach, not only of pharmaceuticals but also of pharmacological and non-pharmacological countermeasures. For instance, non-pharmacological countermeasures could increase tolerance to SMS during pre-flight training, while pharmacological countermeasures could be applied in-flight and post-flight to ease symptoms directly. Previous research has also primarily focused on addressing nausea-related symptoms of SMS. While this is crucial, SMS encompasses a range of symptoms that can affect the operational effectiveness of astronauts, including increased body warmth, sweating, loss of appetite, fatigue, and anorexia (Heer and Paloski, 2006). More efforts should be made to address other SMS symptoms as well.
Comparisons within each countermeasure domain are also complicated since some studies have explored SMS in actual spaceflight conditions, whereas others have used ground-based analogs. For example, comparing symptoms of SMS during an International Space Station (ISS) mission with a terrestrial rotary chair may not be a valid comparison given the stark differences in physiological, musculoskeletal, neurobiological factors and potentially different causative elements for SMS in both cases. Despite gravity always being present, ground-based analogs and simulations have been widely adopted across space research and could help develop effective countermeasures against SMS. However, standardization is required if fair comparisons are to be made.
Importantly, most of the current literature relies heavily on self-reported measures to capture the prevalence and severity of SMS symptoms (Hordinsky et al., 1982; Davis et al., 1993a; Kohl et al., 1993; Knox et al., 1994; Cowings and Toscano, 2000; Dornhoffer et al., 2004; Stroud et al., 2005; Reschke et al., 2006; Cloutier and Watt, 2007). Although widely used, this qualitative approach may be susceptible to bias impacting the accuracy and validity of the findings. More objective and quantitative methods of measuring SMS symptoms should be considered, such as physiological measures (e.g., heart rate, respiration, or skin conductance). These may act as precursors to actual SMS and could help develop targeted countermeasures while providing a more holistic and representative understanding of the onset and prevalence of SMS. If adequately validated, deviations in physiological measures can also be integrated into some early warning systems.
Our systematic review reveals that the current research suffers from severely restricted and biased samples. Participant samples are male-dominant and consist primarily of highly trained personnel, often recruited multiple times across different studies. As shown in Table 1, several studies consisted of all male participants. Addressing the lack of gender balance is critical since there are key hormonal and physiological differences between males and females that may impact the onset and severity of SMS symptoms (Reschke et al., 2014). With more efforts by space agencies and commercial companies to recruit females for crewed missions (Guzman, 2019), it is crucial to understand how SMS impacts both genders.
The reviewed literature provides a promising indication that both pharmacological and non-pharmacological approaches can be used to address SMS; however, given some of the challenges of doing space research mentioned above, our review paints an incomplete and inconsistent picture of the effectiveness of current countermeasures. Despite our systematic approach, no single countermeasure appears superior in addressing SMS. Importantly, there is an apparent lack of research into non-pharmacological studies, which might undermine its potential. Some consideration should also be given to combining the strengths of pharmacological and non-pharmacological approaches to reduce SMS symptoms maximally. Critically, the findings presented here are a snapshot of the current SMS literature. The vast majority of data generated by leading space agencies may not be published due to clinical confidentiality, time limitations, or non-significant results. To better understand SMS, researchers need to move toward an open science and transparent approach whereby data and peer-reviewed papers are made widely available sooner than later.
SMS's high prevalence and potentially lethal consequences reinforce the need to quickly find effective countermeasures to alleviate symptoms and ensure the success of human space missions. There is no doubt that long duration spaceflight and exposure to microgravity will increase the risk of developing SMS and, therefore symptoms that might very likely impact the physical and cognitive functioning of space travelers. Understanding the mechanisms of SMS and developing countermeasures is imperative for safety, health, and productivity of space crewmembers during future missions to the Moon, Mars, and beyond.
Data availability statement
The original contributions presented in the study are included in the article/Supplementary material, further inquiries can be directed to the corresponding author.
Author contributions
AK, PPP, IA, and IJ identified the keywords for scoping searches. KN carried out database searches using a search strategy. AK, PPP, IJ, and HG screened papers. AK, PPP, IA, HG, IJ, and BB completed data extraction on included papers and contributed to manuscript writing. EF and RG provided guidance throughout the systematic review and helped with manuscript editing. All authors read and approved the final manuscript.
Acknowledgments
We would like to acknowledge the Space Generation Advisory Council and UK Space Life and Biomedical Association. In particular, Dr. Rochelle Velho, Dr. Anthony Yuen, Zainab Mavani, Emma Barratt, Dr. Sergio Covarrubias-Castillo, Dr. Karan Ghatora, Ahmed Baraka, Bethany Evans, and Dr. Justin Koh.
Conflict of interest
The authors declare that the research was conducted in the absence of any commercial or financial relationships that could be construed as a potential conflict of interest.
Publisher's note
All claims expressed in this article are solely those of the authors and do not necessarily represent those of their affiliated organizations, or those of the publisher, the editors and the reviewers. Any product that may be evaluated in this article, or claim that may be made by its manufacturer, is not guaranteed or endorsed by the publisher.
Supplementary material
The Supplementary Material for this article can be found online at: https://www.frontiersin.org/articles/10.3389/fncir.2023.1150233/full#supplementary-material
References
Cloutier, A., and Watt, D. G. (2007). Adaptation to motion sickness from torso rotation affects symptoms from supine head nodding. Aviat. Space Environ. Med. 78, 764–769. Available online at: https://www.ingentaconnect.com/content/asma/asem/2007/00000078/00000008/art00003
Cowings, P. S., and Toscano, W. B. (2000). Autogenic-feedback training exercise is superior to promethazine for control of motion sickness symptoms. J. Clin. Pharmacol. 40, 1154–1165. doi: 10.1177/009127000004001010
Cowings, P. S., Toscano, W. B., DeRoshia, C., and Miller, N. E. (2000). Promethazine as a motion sickness treatment: impact on human performance and mood states. Aviat. Space Environ. Med. 71, 1013–1022.
Davis, J. R., Jennings, R. T., and Beck, B. G. (1993a). Comparison of treatment strategies for space motion sickness. Acta Astronaut. 29, 587–591. doi: 10.1016/0094-5765(93)90074-7
Davis, J. R., Jennings, R. T., Beck, B. G., and Bagian, J. P. (1993b). Treatment efficacy of intramuscular promethazine for space motion sickness. Aviat. Space Environ. Med. 64, 230–233.
Dilda, V., Morris, T. R., Yungher, D. A., MacDougall, H. G., and Moore, S. T. (2014). Central adaptation to repeated galvanic vestibular stimulation: implications for pre-flight astronaut training. PLoS ONE 9, e0112131. doi: 10.1371/journal.pone.0112131
Dobie, T., McBride, D., Dobie Jr, T., and May, J. (2001). The effects of age and sex on susceptibility to motion sickness. Aviat. Space Environ. Med. 72, 13–20.
Dornhoffer, J., Chelonis, J. J., and Blake, D. (2004). Stimulation of the semicircular canals via the rotary chair as a means to test pharmacologic countermeasures for space motion sickness. Otol. Neurotol. 25, 740–745. doi: 10.1097/00129492-200409000-00016
Egorov, B. B., and Samarin, G. I. (1970). Possible changes in paired working of vestibular system during weightlessness. Environ. Space Sci. USSR 4, 154-.
Flanagan, M. B., May, J. G., and Dobie, T. G. (2005). Sex differences in tolerance to visually-induced motion sickness. Aviat. Space Environ. Med. 76, 642–646. Available online at: https://www.ingentaconnect.com/contentone/asma/asem/2005/00000076/00000007/art00004
Golding, J. F., Kadzere, P., and Gresty, M. A. (2005). Motion sickness susceptibility fluctuates through the menstrual cycle. Aviat. Space Environ. Med. 76, 970–973. Available online at: https://www.ingentaconnect.com/content/asma/asem/2005/00000076/00000010/art00009
Golding, J. F., Paillard, A. C., Normand, H., Besnard, S., and Denise, P. (2017). Prevalence, predictors, and prevention of motion sickness in zero-G parabolic flights. Aerospace Med. Hum. Perform. 88, 3–9. doi: 10.3357/AMHP.4705.2017
Graybiel, A. (1976). The prevention of motion sickness in orbital flight. Life Sci. Space Res. 14, 109–118. doi: 10.1515/9783112516843-011
Graybiel, A. (1979). Prevention and treatment of space sickness in shuttle-orbiter missions. Aviat. Space Environ. Med. 50, 171–176.
Graybiel, A. (1981). Coping with space motion sickness in Spacelab missions. Acta Astronaut. 8, 1015–1018. doi: 10.1016/0094-5765(81)90073-4
Gundel, A., Polyakov, V. V., and Zulley, J. (1997). The alteration of human sleep and circadian rhythms during spaceflight. J. Sleep Res. 6, 1–8. doi: 10.1046/j.1365-2869.1997.00028.x
Guzman, A. (2019). Women's History Month: Most Recent Female Station Crew Members. NASA. Available online at: http://www.nasa.gov/mission_pages/station/research/news/whm-recent-female-astronauts (accessed December 23, 2022).
Harm, D. L., and Parker, D. E. (1994). Preflight adaptation training for spatial orientation and space motion sickness. J. Clin. Pharmacol. 34, 618–627. doi: 10.1002/j.1552-4604.1994.tb02015.x
Heer, M., and Paloski, W. H. (2006). Space motion sickness: incidence, etiology, and countermeasures. Autonom. Neurosci. Basic Clin. 129, 77–79. doi: 10.1016/j.autneu.2006.07.014
Hordinsky, J. R., Schwartz, E., Beier, J., Martin, J., and Aust, G. (1982). Relative efficacy of the proposed Space Shuttle antimotion sickness medications. Acta Astronaut. 9, 375–383. doi: 10.1016/0094-5765(82)90065-0
Jörges, B., and López-Moliner, J. (2017). Gravity as a strong prior: implications for perception and action. Front. Hum. Neurosci. 11, 203. doi: 10.3389/fnhum.2017.00203
Knox, G. W., Woodard, D., Chelen, W., Ferguson, R., and Johnson, L. (1994). Phenytoin for motion sickness: clinical evaluation. Laryngoscope 104, 935–939. doi: 10.1288/00005537-199408000-00005
Kohl, R. L. (1983). Sensory conflict theory of space motion sickness: an anatomical location for the neuroconflict. Aviat. Space Environ. Med. 54, 464–465.
Kohl, R. L., Sandoz, G. R., Reschke, M. F., Calkins, D. S., and Richelson, E. (1993). Facilitation of adaptation and acute tolerance to stressful sensory input by doxepin and scopolamine plus amphetamine. J Clin. Pharmacol. 33, 1092–1103. doi: 10.1002/j.1552-4604.1993.tb01946.x
Lackner, J. R., and Graybiel, A. (1994). Use of promethazine to hasten adaptation to provocative motion. J. Clin. Pharmacol. 34, 644–648. doi: 10.1002/j.1552-4604.1994.tb02018.x
Lacquaniti, F., Ivanenko, Y. P., Sylos-Labini, F., La Scaleia, V., La Scaleia, B., Willems, P. A., et al. (2017). Human locomotion in hypogravity: from basic research to clinical applications. Front. Physiol. 8, 893. doi: 10.3389/fphys.2017.00893
NASA Human Resources Roadmap (2022). SM-203: Develop and test SMS Countermeasures. Available online at: https://humanresearchroadmap.nasa.gov/gaps/gap.aspx?i=694 (accessed January 23, 2023).
Norfleet, W. T., Degioanni, J. J., Calkins, D. S., Reschke, M. F., Bungo, M. W., Kutyna, F. A., et al. (1992). Treatment of motion sickness in parabolic flight with buccal scopolamine. Aviat. Space Environ. Med. 63, 46–51.
Noskov, V. B., and Grigoriev, A. I. (1994). Diuretic as a means for rapid adaptation to weightlessness. Acta Astronaut. 32, 841–843. doi: 10.1016/0094-5765(94)90091-4
Oman, C. M., Lichtenberg, B. K., Money, K. E., and McCoy, R. K. (1986). M.I.T./Canadian vestibular experiments on the Spacelab-1 mission: 4. Space motion sickness: symptoms, stimuli, and predictability. Exp. Brain Res. 64, 316–334. doi: 10.1007/BF00237749
Page, M. J., McKenzie, J. E., Bossuyt, P. M., Boutron, I., Hoffmann, T. C., Mulrow, C. D., et al. (2021). The PRISMA 2020 statement: an updated guideline for reporting systematic reviews. BMJ 372, n71. doi: 10.1136/bmj.n71
Paillard, A. C., Quarck, G., Paolino, F., Denise, P., Paolino, M., Golding, J. F., et al. (2013). Motion sickness susceptibility in healthy subjects and vestibular patients: effects of gender, age and trait-anxiety. J. Vestib. Res. 23, 203–209. doi: 10.3233/VES-130501
Parker, D. E., Tjernstrom, O., Ivarsson, A., Gulledge, W. L., and Poston, R. L. (1983). Physiological and behavioral effects of tilt-induced body fluid shifts. Aviat. Space Environ. Med. 54, 402–409.
Reschke, M. F., Cohen, H. S., Cerisano, J. M., Clayton, J. A., Cromwell, R., Danielson, R. W., et al. (2014). Effects of sex and gender on adaptation to space: neurosensory systems. J. Women's Health 23, 959–962. doi: 10.1089/jwh.2014.4908
Reschke, M. F., Somers, J. T., and Ford, G. (2006). Stroboscopic vision as a treatment for motion sickness: strobe lighting vs. shutter glasses. Aviat. Space Environ. Med. 77, 2–7. Available online at: https://www.ingentaconnect.com/content/asma/asem/2006/00000077/00000001/art00001
Russomano, T., Da Rosa, M., and Dos Santos, M. (2019). Space motion sickness: A common neurovestibular dysfunction in microgravity. Neurol. India 67, S214–S218. doi: 10.4103/0028-3886.259127
Souvestre, P., and Landrock, C. (2005). Space flight biomedical deterioration prevention and correction using biophotonic technology: from postural deficiency syndrome to space adaptation syndrome. SAE Trans. 114, 13–25. doi: 10.4271/2005-01-2762
Stroud, K. J., Harm, D. L., and Klaus, D. M. (2005). Preflight virtual reality training as a countermeasure for space motion sickness and disorientation. Aviat. Space Environ. Med. 76, 352–356. Available online at: https://www.ingentaconnect.com/content/asma/asem/2005/00000076/00000004/art00006
Townsend, L. W. (2005). Implications of the space radiation environment for human exploration in deep space. Radiat. Prot. Dosimetry 115, 44–50. doi: 10.1093/rpd/nci141
Van Ombergen, A., Laureys, S., Sunaert, S., Tomilovskaya, E., Parizel, P. M., and Wuyts, F. L. (2017). Spaceflight-induced neuroplasticity in humans as measured by MRI: what do we know so far? NPJ Microgravity 3, 2. doi: 10.1038/s41526-016-0010-8
Weerts, A. P., De Meyer, G., Pauwels, G., Vanspauwen, R., Dornhoffer, J. L., Van De Heyning, P. H., et al. (2012). Pharmaceutical countermeasures have opposite effects on the utricles and semicircular canals in man. Audiol. Neurotol. 17, 235–242. doi: 10.1159/000337273
Weerts, A. P., Putcha, L., Hoag, S. W., Hallgren, E., Van Ombergen, A., Van de Heyning, P. H., et al. (2015). Intranasal scopolamine affects the semicircular canals centrally and peripherally. J. Appl. Physiol. 119, 213–218. doi: 10.1152/japplphysiol.00149.2015
Weerts, A. P., Vanspauwen, R., Fransen, E., Jorens, P. G., Van de Heyning, P. H., and Wuyts, F. L. (2014). Space motion sickness countermeasures: A pharmacological double-blind, placebo-controlled study. Aviat. Space Environ. Med. 85, 638–644. doi: 10.3357/asem.3865.2014
Keywords: Space Motion Sickness (SMS), pharmacological countermeasures, non-pharmacological countermeasures, human space flight, aerospace medicine
Citation: Khalid A, Prusty PP, Arshad I, Gustafson HE, Jalaly I, Nockels K, Bentley BL, Goel R and Ferrè ER (2023) Pharmacological and non-pharmacological countermeasures to Space Motion Sickness: a systematic review. Front. Neural Circuits 17:1150233. doi: 10.3389/fncir.2023.1150233
Received: 23 January 2023; Accepted: 19 May 2023;
Published: 16 June 2023.
Edited by:
Ronan Padraic Murphy, Dublin City University, IrelandReviewed by:
Charles R. Doarn, University of Cincinnati, United StatesMarc-Antoine Custaud, Université d'Angers, France
Copyright © 2023 Khalid, Prusty, Arshad, Gustafson, Jalaly, Nockels, Bentley, Goel and Ferrè. This is an open-access article distributed under the terms of the Creative Commons Attribution License (CC BY). The use, distribution or reproduction in other forums is permitted, provided the original author(s) and the copyright owner(s) are credited and that the original publication in this journal is cited, in accordance with accepted academic practice. No use, distribution or reproduction is permitted which does not comply with these terms.
*Correspondence: Elisa R. Ferrè, ZWxpc2EuZmVycmUmI3gwMDA0MDtiYmsuYWMudWs=
†These authors have contributed equally to this work and share first authorship
‡These authors have contributed equally to this work and share senior authorship