- 1Department of Cell and Developmental Biology, University College London, London, UK
- 2Champalimaud Neuroscience Programme, Champalimaud Centre for the Unknown, Lisbon, Portugal
Adult zebrafish are robustly social animals whereas larva is not. We designed an assay to determine at what stage of development zebrafish begin to interact with and prefer other fish. One week old zebrafish do not show significant social preference whereas most 3 weeks old zebrafish strongly prefer to remain in a compartment where they can view conspecifics. However, for some individuals, the presence of conspecifics drives avoidance instead of attraction. Social preference is dependent on vision and requires viewing fish of a similar age/size. In addition, over the same 1–3 weeks period larval zebrafish increasingly tend to coordinate their movements, a simple form of social interaction. Finally, social preference and coupled interactions are differentially modified by an NMDAR antagonist and acute exposure to ethanol, both of which are known to alter social behavior in adult zebrafish.
Introduction
Human infants exhibit social behaviors from birth (Xiao et al., 2014). Throughout life these innate social drives provide the substrate for learning more complex forms of human interaction. Disruptions to early social behavior may impair the development of normal adult sociality, and may contribute to disorders such as autism (Banerjee et al., 2014). Since the neural circuitry that underlies human innate social behavior is established in utero, very little is understood about its normal and pathological development, anatomy, and function.
Early developing social behaviors, such as the preference to observe and mimic conspecifics, are common to many other mammals (Ferrari et al., 2006) and non-mammalian vertebrates (Engeszer et al., 2004, 2007; Mooney, 2014). Animal models are much more amenable to detailed investigation and share many of the same anatomical and functional neural systems that underlie innate social behavior in humans (O'Connell and Hofmann, 2011, 2012). Consequently, we sought a model system for which neural circuits can be assessed throughout development, and for which social behavior is an important component of the organism's behavioral repertoire (Oliveira, 2013).
Zebrafish adults are social animals (Oliveira, 2013), exhibiting a range of group (shoaling and schooling) (Krause et al., 2000; Green et al., 2012; Miller and Gerlai, 2012), conspecific directed aggression (Jones and Norton, 2015), mating (Engeszer et al., 2008), and other behaviors (Arganda et al., 2012). Larval zebrafish, however, do not exhibit the overt shoaling and schooling behaviors that are readily apparent in adults. In order to shoal fish must prefer to approach and remain near conspecifics. There is some evidence that this preference might develop as early as 1 week (Hinz et al., 2013) whereas shoaling appears only in early flexion larva (~13–15 days post fertilization (dpf, 6 mm length) (Engeszer et al., 2007). Schooling, however, requires the group to move in a polarized and coordinated manner and has thus far only been described in adults (Miller and Gerlai, 2012).
Social behavior encompasses more than simply preferring to be near members of the same species. For instance, individuals may coordinate their behavior with other members of the same social group. Such coordination is obvious in the case of schooling fish, where individuals align their body orientation and synchronize their movements, but it is also present in social mammals. For example, humans will unconsciously coordinate a diverse range of behaviors, such as yawning, eye blinks, and posture (Sebanz et al., 2006; Richardson et al., 2007), and this is thought to provide a foundation for more elaborate forms of social communication and cooperation.
Here we set out to investigate early social interactions in zebrafish and to determine if the establishment of preference for the presence of conspecifics is contemporaneous with individuals beginning to coordinate their behavior. We have designed a novel social preference/interaction assay for zebrafish larva that continuously monitors, with high temporal resolution, the detailed behavior of individuals freely choosing to observe or avoid a conspecific. This assay demonstrates that social behaviors develop gradually and are robust by 3 weeks post-fertilization. We have also used the assay to characterize the effects of substances known to influence the social behavior of adults.
Materials and Methods
Zebrafish Husbandry
AB strain zebrafish (Danio rerio) to be tested were bred, raised and housed in the same environment. All fish were obtained from natural spawning and housed in groups of roughly 50 fish, and kept at 14 h light/10 h dark cycle. Fish were fed two times per day from 4 dpf with dry food diet from SAFE Diets (particle size 50–100) and twice with salt water rotifer (Brachionus Plicatilis) until 10 dpf; then twice a day with dry food diet (particle size 100–200), and with a combination of salt water rotifer and brine shrimp (Artemia salina) until 15 days; finally twice a day with dry food diet (particle size 200–300) and with brine shrimp until used in the experiments. All the fish run in the behavioral assay were fed in the morning. All test fish were paired with age-matched siblings as the social cue. The experiments described were approved by local ethical committee (AWERB Bloomsbury Campus UCL) and the UK Home Office.
Behavioral Assay
Experiments were performed in a custom-built behavioral setup (Figure 1A) that was assembled from structural framing (Misumi, DE) and optomechanics (Thorlabs, USA). The videography system comprised a high-speed camera (Flea3, PointGrey, CA), infrared LED backlight (Advanced Illumination, USA, 880 nm), infrared filter (R70, Hoya, JP), and a vari-focal lens (Fujinon, JP). Fish were imaged in a custom-built behavioral arena that was fabricated with a laser-cutter from 5 mm thick opaque white acrylic, sealed with silicone, and with glass window partitions; the multi-chamber design is shown in Figure 1A. The dimensions of a single behavioral arena were 4 × 3.2 cm. The viewing chamber that contained a single or multiple SC fish was 1.5 cm square, and the width of the passage between the two arms of the arena was 6 mm. The water level height was 5 mm, the temperature of the water was around 25′C, pH was 7.0, and the conductivity was 445 μS. The arena was supported on a transparent base covered on one side with diffusive gel paper (Rosco Cinegel, USA). It was illuminated with visible light by homogenously projecting a white rectangle, via a 45° infrared cold mirror positioned between the chamber and IR illuminator, onto the base of the assay using a laser light projector (Microvision, ShowwX+, USA). For all experiments, the entire behavioral apparatus was enclosed in a light-tight enclosure, and for the dark experiments, the visible background illumination was removed.
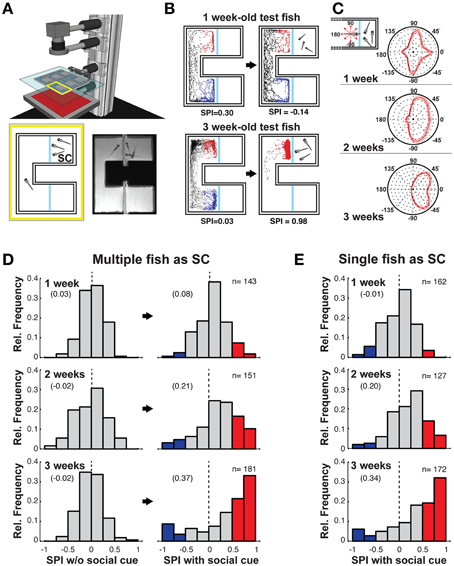
Figure 1. Social preference is robust in 3 weeks old zebrafish. (A) Schematic of the behavioral setup (top). Infrared light homogeneously illuminates the behavioral arenas. Schematic of a single choice chamber (bottom left) with an observer (test) fish and multiple conspecifics as the social cue (SC). The blue lines are clear glass windows. Single frame from high-speed video recording of an experiment with 3 weeks old fish (bottom right). (B) Examples of tracking of a 1 week old (top) and 3 weeks old (bottom) fish, in the absence (left), and presence (right) of the SC. The blue and red portions of the movement tracks are used to calculate the social preference index (SPI, indicated below). (C) Schematic depicting body orientation of the observer test fish relative to the SC chamber (inset—top left). Polar histograms, averaged across all tested fish, of body orientations of the observer fish when within the SC side of the chamber. From 1 to 3 weeks a preference emerges for the observer fish to view the SC with either the left (−45°) or right (+45°) eye. Thin lines indicate two standard errors from the mean (SEM) (1 week: n = 143, 2 weeks: n = 151, 3 weeks: n = 181). (D) Histograms of all SPIs during acclimation (left column) and SC (right column) periods across different developmental stages [1 week (6–8 dpf); 2 weeks (13–15 dpf), 3 weeks (20–22 dpf)]. A range of positive and negative preferences are observed. For presentation clarity, red bars (SPI > 0.5) highlight strong preference for the SC, while blue bars (SPI < –0.5) highlight strong aversion for the SC (zero is marked with a dashed vertical line). (E) Histogram of all SPIs when a single conspecific served as the SC across different developmental stages. Numbers in brackets indicate Mean SPI.
Acquisition Software
Fish in six individual chambers were contemporaneously tracked in real-time using custom written workflows in Bonsai, an open-source C# data stream processing framework (Lopes et al., 2015). For each chamber, the image was cropped, background subtracted, thresholded, and the centroid found, characterized (position, orientation, and heading), and stored in a CSV file. The video was also saved with H.264 compression for subsequent offline analysis (http://www.dreo-sci.com/resources/).
Data Analysis
Social Preference Index (SPI) was calculated by subtracting the number of frames in which the fish was located within the region near the conspecific SC (area highlighted by the red tracking in Figure 1B) by the number of frames spent in the equivalent region on the opposite side of the chamber (blue tracking in Figure 1B). This difference was then divided by the total number of frames recorded in the two analysis compartments during the experiment, resulting in a value varying between −1 and 1 [SPI = (SC frames – Non SC frames)/Total frames]. The SPI during the acclimation period, for which there is no SC, was computed with reference to the randomized side of the chamber on which the SC would be added in the subsequent experimental phase.
The compressed video from each experiment could be repeatedly re-analyzed using custom written bulk-processing routines in Python (https://www.dreo-sci.com/resources/). A motion trajectory for each fish was computed by first segmenting the binary particle for each fish from the background and then measuring the change in pixel values, for that particle, from one frame to the next. This resultant frame-by-frame segmented motion value provided a very stable time series for identifying the peaks of individual bouts and then testing for interaction between the observer and SC fish.
Statistical Analysis
The SPI distributions for many Ac vs. SC conditions (1 week old, MK-801, EtOH, etc.) are normally distributed, however some conditions (in which the value approaches the SPI bound of ±1), are clearly non-normal. We therefore used the same non-parametric statistic test for all comparisons in the study: a Wilcoxon signed-rank test of paired samples.
Drug Treatments
MK-801: 100 mM stock solution was prepared by dissolving MK-801 (M107; Sigma-Aldrich) in 100% DMSO (D2650; Sigma-Aldrich) and stored at −20°C. The drug was administered for 1 h prior the experiments by diluting the stock solution in fish water in order to obtain a working concentration of 100 μM. Zebrafish were washed with fish water before placing them in the chamber for recordings.
For ethanol experiments, low (0.125%) or a high ethanol (0.5%) concentrations were obtained by diluting ethanol in fish water. Fish were exposed with one of the two ethanol concentrations for 1 h prior to, and during experiments.
Results
Fish Develop Strong Social Preference and Interactions by 3 Weeks of Age
We designed a behavioral chamber in which zebrafish fish could swim freely between two arms, but in only one could they view conspecific siblings through a glass partition. Six such chambers were simultaneously monitored with an infrared high-speed camera and automated tracking software recorded the behavior (position and orientation) of the observer fish (Figure 1A, Supplementary Movie 1). Following 15 min in the chamber without conspecifics [acclimation (Ac) period], a single or three conspecifics were added to one of the adjacent compartments, randomly selected, and the behavior of the observer fish was monitored for an additional 15 min [social cue (SC) period]. There was no bias between compartment arms in the acclimation phase for fish at any age, nor if the fish were monitored for a further 15 min following the acclimation phase without adding the SC (Supplementary Figures 1E,F).
Three weeks old zebrafish consistently showed a very strong bias to remain in the arm of the chamber adjacent to the SC [Figure 1B, compare Supplementary Movie 2 (1 week)–Supplementary Movie 3 (3 weeks)]. To quantify the tendency for each tested fish to spend time in one or the other arm of the chamber, we defined a social preference index (SPI) (see Section Materials and Methods). A positive SPI indicates a preference for the chamber arm with the SC and a negative SPI indicates an aversion for the SC. The SPI was computed for all tested one, 2 and 3 weeks old fish with and without the presence of multiple (Figure 1D) or a single conspecific (Figure 1E). One week old larva exhibited a very weak, but significant, preference for an SC arm (Figure 1D, top; Ac vs. SC p = 0.006) containing multiple conspecifics, however, this preference bias was absent when only a single fish was placed in the SC arm (Figure 1E, top; Ac vs. SC p = 0.9). In contrast, the SPI of 2 weeks old larva was strongly shifted toward positive values when viewing both multiple (Figure 1D, middle; Ac vs. SC, p = 4.4 × 10−10) and single conspecifics (Figure 1E, middle; Ac vs. SC, p = 1.4 × 10−11, Supplementary Figure 1D). By 3 weeks, SC preference strengthened further, with many values close to 1, reflecting the strong bias of some observer fish to remain almost entirely on the side of the conspecifics (multiple: Figure 1D, bottom; Ac vs. SC, p = 2.0 × 10−13, single: Figure 1E, bottom; Ac vs. SC, p = 1.4 × 10−15).
A small minority of 3 weeks old fish had strong negative SPIs (Figure 1D, bottom). These fish exhibited an aversive response to the conspecifics, preferring to stay in the chamber away from the SC (Supplementary Figure 1B, Supplementary Movie 4). Such aversive behavior was rarely observed in younger fish suggesting that, as for positive social interaction, social aversion also increases throughout development.
The behavior of the 3 weeks old zebrafish when viewing the SC consisted of alternating body orientation such that the left or right eye directly viewed the SC compartment (Supplementary Movie 3). This behavior was quantified in a histogram of all orientations of the fish body axis while in the SC arm of the chamber (Figure 1C, Supplementary Figure 1C). A gradual transition from primarily orienting along the axes of the chamber (cardinal directions: 0°, ±90°, and 180°) to orienting for visual observation (±45°) occurred over the first 3 weeks of development. No strong bias for observing with either the left or right eye (Sovrano and Andrew, 2006) was found in this assay.
We next set out to investigate what sensory cues contribute to the displayed social preference.
Social Preference Requires Visual Observation of Conspecifics with a Similar Age
Although visual stimulation seemed the most likely source of the preference for the SC, it was possible that some olfactory or tactile cues may pass between the chambers of the observer and SC fish. Consequently, we compared preference behavior for 3 weeks old fish tested in the dark to those tested in light (Figure 2A).
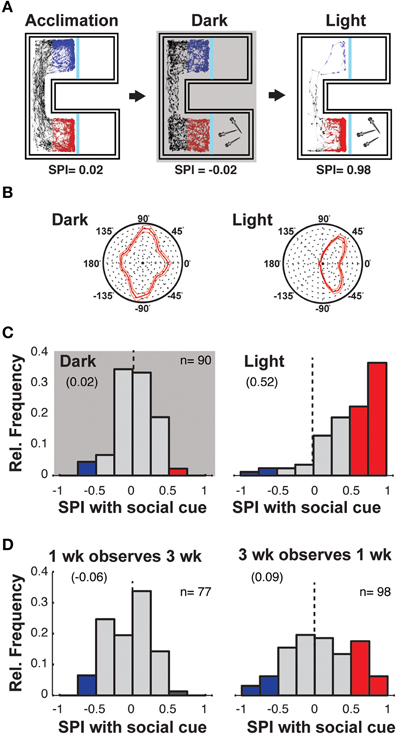
Figure 2. Social preference requires visual observation of similarly aged fish. (A) Schematic of the experiment to assess whether visual information is required for fish to show social preference. Following the acclimation period, 3 weeks old zebrafish are presented with a SC and monitored under both normal illumination and darkness, where the order of exposure to each condition was randomized. SPIs resulting from such experiments are indicated below schematics. (B) Polar histograms of body orientations of the observer when on the SC side of the chamber during both light and dark sessions. The preference for the observer fish to orient at 45° to the SC chamber is not present in darkness (thin lines indicate 2 × SEM, n = 90), supporting the inference that such orientation in the light represents monocular viewing of conspecifics. (C) Histograms of SPIs for all individuals during the dark and light conditions in the presence of a single fish as SC. (D) Histograms of the SPIs of 1 week old fish observing 3 weeks old fish (left), and the SPIs of 3 weeks old fish observing 1 week old fish (right). See Supplementary Figure 2C for the SPIs in the absence of SC. Numbers in brackets indicate Mean SPI.
Removal of background illumination completely abolished the tendency of observer fish to orient toward the conspecific viewing chamber (Figure 2B) and social preference was abolished, as evidenced by the distribution of SPIs (Figure 2C, Supplementary Figure 2A; Ac vs. SC, p = 0.57). Furthermore, with normal background illumination, replacing the transparent window with an opaque barrier also eliminated preference for the SC (Supplementary Figure 2B). These experiments provide strong evidence that the social preference behavior of 3 weeks old zebrafish depends on vision.
The data above indicates that during the first 3 weeks of their life, zebrafish develop a robust social preference to view age-matched conspecifics. However, during this time they also change significantly in size, doubling their head to tail length (Supplementary Figure 1A). To assay whether the size/age of the SC fish influences social preference, we monitored the behavior of 1 and 3 weeks old zebrafish presented with larger/older or smaller/younger conspecifics as the SC (Figure 2D, Supplementary Figure 2C).
One week old fish not only showed no preference for 3 weeks old fish, but also a slight aversion to viewing the larger fish, supporting the conclusion that the development of social preference reflects maturation of the observer and is not simply dependent on the age/size of the stimulus [Figure 2D left; Ac vs. SC, p = 0.002; SPI difference (Ac-SC) = −0.111]. Three weeks old fish also did not exhibit a strong social preference when presented with 1 week old fish as the SC [Figure 2D, right; Ac vs. SC, p = 0.02; SPI difference (Ac-SC) = 0.106]. However, the broader distribution of SPIs suggests that the smaller/younger fish may still influence the behavior of the larger/older observing fish, which could be due to fish becoming progressively more responsive to any moving objects within their environment.
Zebrafish Coordinate Their Movement
Three weeks old fish display robust visually-driven social preference; high-speed videography additionally allowed us to investigate the extent to which the behavior of the SC fish influenced the behavior of the observer (Supplementary Movie 5).
Young zebrafish tend to move in small bouts of activity consisting of discrete swims or turns (Orger et al., 2008). Individual bouts were detected by identifying a peak in the motion tracking signal (Figure 3A, top trace). Averaging all of these bout time-courses (Figure 3B) revealed a pre- and post-bout quiescent period, the timings of which reflected the periodicity of movement. These quiescent periods shortened from 1 to 3 weeks of age as the mean bout frequency increased ~50%, from 0.79 Hz at 1 week to 1.22 Hz at 3 weeks. As observed in other behavioral contexts, these movement bouts were composed of a mixture of forward swims and orienting turns (Figure 3C) (Orger et al., 2008).
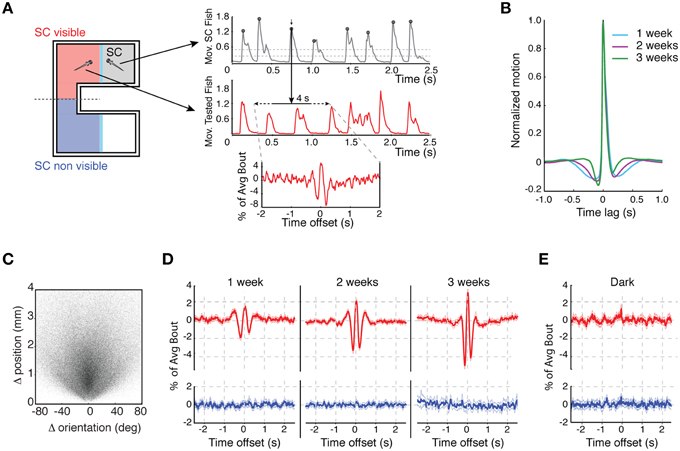
Figure 3. Development of the dynamics of social interaction. (A) Example of the motion bout detection and alignment analysis: the left schematic shows the test chamber indicating in red the side in which the SC fish is visible and in blue the side in which it is not. The plots on the right indicate how movement bouts were analyzed. Top plot shows movements bouts of the SC fish. Peaks in movement trajectories were identified with a dual-threshold algorithm (upper threshold dotted line is 3 × standard deviation (3 × SD) and lower threshold dotted line is 2 × SD from baseline). The middle plot shows the movement bouts of the observer, test fish. The movement peaks of the SC fish were used to extract short time windows of the movement trajectories of the observer fish trajectory (2 s either side of the SC fish movement peak). The bottom plot shows the “bout-triggered-average” (BTA) movement for the observer fish which was computed by averaging movements across all of the 4 s time windows aligned to the SC peak movement. BTAs were computed separately depending on whether the observer fish could view the SC or not (left schematic). (B) The average bout motion time-course for all SC fish, normalized to the peak movement of each fish, at different developmental stages. The average bouts are overlaid to highlight changes in the kinetics between 1 and 3 weeks of age. (C) Scatter plot presentation of all bouts, where each bout is represented by a single point that indicates the change (Δ) in position and orientation that occurred during that bout (n = 247, 779 bouts). (D) BTAs of 1–3 weeks old observer fish motion aligned to movement bouts of single SC fish (red) or plotted when the SC was not visible (blue) (1 week: n = 106, 2 weeks: n = 136, 3 weeks: n = 163). (E) BTAs for fish monitored in darkness when on the same (red) or opposite side (blue) of the SC (n = 90).
We next asked whether a motion bout produced by the SC fish influenced the movement of the observer. Short time windows of the motion trajectories from the observer fish, normalized by each individual's average motion peak, were extracted and aligned to the bouts of the SC fish (Figure 3A, middle trace) and were then averaged over all bouts (Figure 3A, bottom trace). This generated a “bout triggered average” (BTA), which is an estimate of how the motion bout of the SC influences movement of the observer.
A clear interaction between the movement of the SC fish and the observer was present at all stages of development. Notably, a bout of motion by a SC fish was, on average, coincident with a synchronous increase in motion by the observer fish. The strength of this motion coupling increased substantially over development (Figure 3D), correlating with the enhancement in positive social preference. This visual coupling behavior was, unsurprisingly, absent for fish in the dark (Figure 3E). These results indicate that not only do 3 weeks old fish prefer to be with conspecifics, but that their behavior is more strongly coupled with that of their social partners.
Social Preference and Interaction are Differentially Impaired by Drug Exposure
We next assayed whether pharmacological manipulations that affect sociality in adult animals similarly influence the manifestation of social behavior in young zebrafish.
Social learning in adult zebrafish is dependent upon N-methyl-D-Aspartate (NMDA) Receptor signaling (Maaswinkel et al., 2013) and we first assessed whether manipulating this pathway altered the social preference and interaction behavior of zebrafish larva. The NMDA receptor antagonist MK-801 was acutely administered at a concentration of 100 μM for 1 h prior to assaying 3 weeks old zebrafish (Figures 4A–D). Although MK-801 can lead to increased locomotor activity (Menezes et al., 2015), at this concentration and age treated zebrafish showed no overt change in the overall amount of swimming. However, we found that 3 weeks old fish exhibited no social preference (Figure 4A, Supplementary Figure 2D; Ac vs. SC period, p = 0.51). This result suggests that blocking NMDA receptors interferes with circuitry required for social interactions, both in larva and in adult fish. In addition, video-tracking revealed a significant alteration of movement dynamics in MK-801 treated larva. The treated fish produced swim bouts lacking the pre- and post-bout quiescent periods (Figure 4B), and a near total loss of conventional forward swims (Figure 4C). Every bout involved a change in orientation (i.e., turning), which is consistent with the “erratic movements” observed in MK-801 treated adult fish (Sison and Gerlai, 2011) and reveals a substantial disruption to the movement control system after drug treatment. These altered bout dynamics also produced an asymmetry in the pattern of social interaction (Figure 4D); the motion of the observer fish strongly influenced the movement of the untreated SC fish (dip prior to 0 s), but the drug-treated observer was much less influenced by the movement of the SC fish (smaller dip after 0 s; Figure 4D). Furthermore, the synchronicity peak at 0 s lag was abolished.
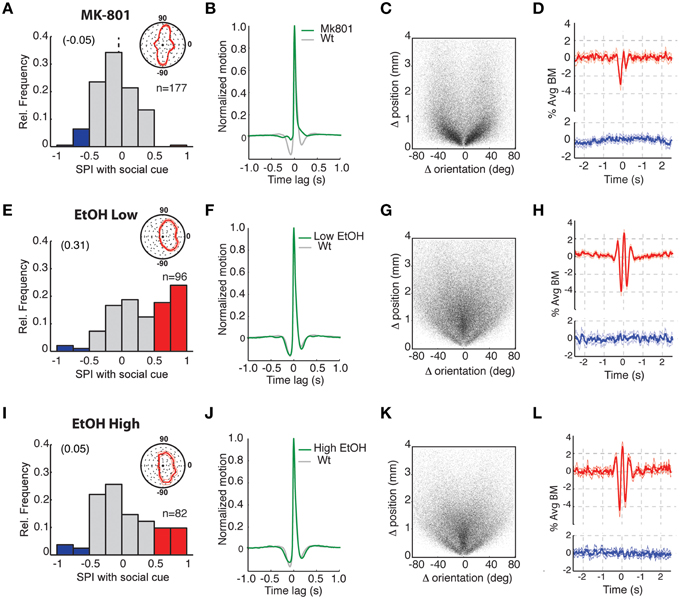
Figure 4. Exposure to NMDA receptor antagonist or ethanol disrupts social preference and differentially impairs social interactions. (A–D) Analysis of fish treated with 100 μM MK-801 NMDA receptor antagonist. (A) Histogram of SPIs revealing no apparent preference for the SC and (inset) body orientations showed little or no direction toward the SC chamber (zero position). SPIs during the acclimation periods are shown in Supplementary Figure 2D. (B) Average motion bout profile for MK-801 treated fish. Relative to untreated controls (gray plot), there is a reduction in the pre- and post-bout quiescent periods and consequently the periodicity of bout generation. (C) Scatter plot presentation of all bouts (n = 85.275 bouts) from all tested fish, where each bout is represented by single point based on the position and body orientation change that occurred for that bout. MK-801 treatment results in a conspicuous reduction in forward swimming bouts (“0” position on X-axis). (D) Bout-triggered averages (BTA) of MK-801-treated observer fish when the SC fish was visible (red plot) or not (blue plot). There is a disruption of normal movement interactions before and after 0 s offset (compare to equivalent plots in Figure 3 or in h and l below) and the abolishment of behavioral synchrony at 0 s offset (see results text for further explanation). (E–H) Comparable analyses as in (A–D) of fish treated with 0.125% alcohol. (E) Plot of SPIs showing that social preference (red) remains and (inset) body orientations were directed toward the SC chamber. (F–H) Average motion bout profiles (F), bout distributions (n = 101, 167 bouts) (G), and BTA plots (H) are all similar to untreated 3 weeks old zebrafish. (I–L) Comparable analyses as in (A–D) of fish treated with 0.5% alcohol. (I) Analysis of SPIs showing social preference is severely disrupted and (inset) body orientations are less strongly directed toward the SC chamber. (J–L) Average motion bout profiles (J), bout distributions (n = 69, 675 bouts) (K), and BTA plots (L) are all similar to untreated 3 weeks old zebrafish.
Acute exposure to high concentrations of ethanol is also known to influence the social behavior of adult zebrafish (Gerlai et al., 2000; Ladu et al., 2014). Consequently, we exposed 3 weeks old fish to low (0.125%) and high (0.5%) levels of ethanol 1 h prior to and during testing in the social assay (Figures 4E–L). The influence of ethanol exposure was concentration dependent. Fish exposed to low ethanol retained a strong SPI (Figure 4E, and Supplementary Figure 2D; Ac vs. SC period p = 5.8 × 10−8) and their bout dynamics (Figure 4F) and composition (swims vs. turns) (Figure 4G) were unaffected. Furthermore, the strength of their BTA interaction was similar to age-equivalent untreated fish. In contrast, upon exposure to a higher concentration of ethanol, social preference was greatly reduced and the SPI distribution was not significantly different from the acclimation period (Figure 4L, Supplementary Figure 2D, Ac vs. SC p = 0.07). Remarkably, despite this loss of social preference by zebrafish exposed to high ethanol concentrations, movement dynamics (Figure 4J), distributions of swim turns (Figure 4K), and the strength of movement coupling with other fish (Figure 4L) were not substantially affected. These intriguing results suggest that social preference and interactions with other individuals, each a fundamental component of social behavior, can be decoupled by pharmacological, and likely other, manipulations.
Discussion
The Development of Social Preference
We have shown that zebrafish gradually develop a “social” preference, which we define as the tendency to remain in a chamber that provides visual access to conspecifics; a behavior that is not significant in 1 week old fish, begins to emerge by 2 weeks, and is very robust at 3 weeks. This preference is visually driven and does not solely depend on the age/size of the conspecific partners as 1 week old larva show no interest in larger 3 weeks old fish. These results suggest that social preference emerges with the development of neural systems that appear or mature during the second and third weeks of life. For instance, it is known that some brain areas, such as the pallium (Dirian et al., 2014), undergo extensive growth during this period.
Whether the preference to observe conspecifics reflects a drive to shoal/school or aggression (Gerlai et al., 2000) is difficult to distinguish with this assay. Both of these behaviors involve multi-modal stimuli (olfactory and tactile), which are prevented in our assay by the transparent barrier dividing the observer and social cue. Nevertheless, our assay demonstrates that visual cues alone can drive social behaviors and is thus easily translated to experimental setups that require restrained fish, but allow for detailed investigation of the underlying neural activity, e.g., two-photon microscopy.
We have not yet fully characterized the specific visual features that drive social preference. However, a simple preference for moving stimuli is unlikely to explain the response since 3 weeks old fish show little interest in viewing moving younger/smaller conspecifics. Our assay has demonstrated that visual stimulation is sufficient to drive social behavior at 3 weeks of age. The presentation of visual “social” stimuli to restrained fish is much more straightforward than attempting to recapitulate the complex tactile and olfactory stimuli that are also involved in schooling/shoaling interactions (Miller and Gerlai, 2012). Therefore, this social behavior assay will considerably facilitate future studies to characterize the changes in neural circuitry that correlate with this fundamental behavioral transition.
It is intriguing that not all fish develop a positive response to conspecifics as some individuals exhibit avoidance behavior when other fish come into view. This result warrants further investigation. For instance, our assay could be used to determine whether fish exhibiting aversive behavior retain this negative social bias after multiple presentations of the SC or whether different environmental, pharmacological, and genetic manipulations predispose developing zebrafish to express more aversive or attractive social behaviors.
Social Interaction as a Coupled Dynamic System
We found that when a fish observes the movement of a conspecific, its own swimming is affected. This visually-mediated coupling of movement is already present in 1 week old larva, but strengthens considerably over the following weeks. Coupling the motion of one fish to that of another is an important prerequisite for the coordinated behavior that predominates in groups of schooling fish (Miller and Gerlai, 2012). The temporal profile of this movement coupling, notably its remarkable synchronicity, is reminiscent of the coordinated movements apparent in other social organisms, including humans (Sebanz et al., 2006; Richardson et al., 2007).
Indeed, synchronization of behavior is observed in many physical systems with coupled dynamics, such as two metronomes on a shared surface (Pantaleone, 2002) as well as for many biological rhythms (Winfree, 1967). If any two periodic movement generators are sensitive to the motion of one another, then they will act as coupled oscillators and will have a natural tendency to synchronize. We have found such a coupling of observation of movement to movement generation in young zebrafish. Whether such coupling dynamics are important for the shoaling/schooling interactions of adult fish, or any other species demonstrating coordinated synchronous movements, warrants further investigation.
Disruptions to the coordination of behaviors, such as the loss of synchronized eye-blinking in autistic subjects (Sears et al., 1994; Senju et al., 2007), are now being identified as potentially important biomarkers of disease that may facilitate early diagnosis and intervention.
Pharmacological Manipulation of Early Social Behavior
The NMDA receptor antagonist MK-801 disrupted both social preference and interaction, whereas alcohol exposure disrupted only preference, leaving intact the ability of fish to couple their movements. This suggests that these two aspects of the social behavior can be at least partially disassociated.
In addition to observing that acute treatment with 100 μM MK-801 disrupts social preference in larva, as was previously shown in adults (Sison and Gerlai, 2011), we also found that it greatly alters underlying movement dynamics. MK-801 treated larva show strongly reduced bout periodicity and do not produce conventional forward swims, which could underlie the observed deficit in coordinated behavior. Such movement impairments will also affect the ability of treated fish to shoal, and might explain why adult zebrafish exposed to a lower concentration (5 μM) of MK-801 exhibited disrupted shoal cohesion (Maaswinkel et al., 2013).
In contrast to NMDA receptor blockade, fish exposed to high concentrations of ethanol exhibited no disruption of intrinsic movement dynamics and show wild-type levels of movement interaction, but social preference was severely disrupted. These results highlight our assay's sensitivity to distinguish components of social behavior, preference, and interaction, which could be separately impaired by different pathologies. Consequently this assay should be well suited for analysis of a range of genetic (Pietri et al., 2013) and pharmacological (Scerbina et al., 2012) manipulations that have been linked to developmental disorders affecting social behavior.
Future Directions
If we are to identify and characterize the neural circuits that underlie the development of social behavior in zebrafish, it will be necessary to adapt our assay to enable the monitoring and manipulation of neural activity in vivo during social interactions. Fortunately, the brains of zebrafish are still small and relatively transparent during the developmental stages at which we observe the onset of social preference and coupled interactions, and are thus amenable to optical and optogenetic techniques for anatomical and functional investigation of whole-brain circuitry (Ahrens and Engert, 2015). Leveraging the power of these optical and genetic tools in young zebrafish, detailed comparison of the neural circuits established during normal and atypical development is likely to produce fundamental insights into the neural basis of social behavior and its associated pathologies.
Author Contributions
ED, SWW, and ARK designed the project; ED performed experiments; GL created the software to acquire and analyze the data; ED and ARK analyzed data; ED, ARK, and SWW wrote the paper.
Conflict of Interest Statement
The authors declare that the research was conducted in the absence of any commercial or financial relationships that could be construed as a potential conflict of interest.
Acknowledgments
We thank Isaac Bianco, Jason Rihel, David Tingley, and David Schoppik for helpful advice and comments on the manuscript, Carole Wilson, Heather Callaway, Karen Dunford, Jenna Hakkesteeg, and Matthew Wicks for fish care. This work was supported by Wellcome Trust Funding to SW and ED and by the Champalimaud Foundation to GL and AK. The authors declare no competing financial interests.
Supplementary Material
The Supplementary Material for this article can be found online at: http://journal.frontiersin.org/article/10.3389/fncir.2015.00039
References
Ahrens, M. B., and Engert, F. (2015). Large-scale imaging in small brains. Curr. Opin. Neurobiol. 32C, 78–86. doi: 10.1016/j.conb.2015.01.007
Arganda, S., Pérez-Escudero, A., and de Polavieja, G. G. (2012). A common rule for decision making in animal collectives across species. Proc. Natl. Acad. Sci. U.S.A. 109, 20508–20513. doi: 10.1073/pnas.1210664109
Banerjee, S., Riordan, M., and Bhat, M. A. (2014). Genetic aspects of autism spectrum disorders: insights from animal models. Front. Cell. Neurosci. 8:58. doi: 10.3389/fncel.2014.00058
Dirian, L., Galant, S., Coolen, M., Chen, W., Bedu, S., Houart, C., et al. (2014). Spatial regionalization and heterochrony in the formation of adult pallial neural stem cells. Dev. Cell 30, 123–136. doi: 10.1016/j.devcel.2014.05.012
Engeszer, R. E., Barbiano, L. A. D., Ryan, M. J., and Parichy, D. M. (2007). Timing and plasticity of shoaling behaviour in the zebrafish, Danio rerio. Anim. Behav. 74, 1269–1275. doi: 10.1016/j.anbehav.2007.01.032
Engeszer, R. E., Ryan, M. J., and Parichy, D. M. (2004). Learned social preference in zebrafish. Curr. Biol. 14, 881–884. doi: 10.1016/j.cub.2004.04.042
Engeszer, R. E., Wang, G., Ryan, M. J., and Parichy, D. M. (2008). Sex-specific perceptual spaces for a vertebrate basal social aggregative behavior. Proc. Natl. Acad. Sci. U.S.A. 105, 929–933. doi: 10.1073/pnas.0708778105
Ferrari, P. F., Visalberghi, E., Paukner, A., Fogassi, L., Ruggiero, A., and Suomi, S. J. (2006). Neonatal imitation in rhesus macaques. PLoS Biol. 4:e302. doi: 10.1371/journal.pbio.0040302
Gerlai, R., Lahav, M., Guo, S., and Rosenthal, A. (2000). Drinks like a fish: zebra fish (Danio rerio) as a behavior genetic model to study alcohol effects. Pharmacol. Biochem. Behav. 67, 773–782. doi: 10.1016/S0091-3057(00)00422-6
Green, J., Collins, C., Kyzar, E. J., Pham, M., Roth, A., Gaikwad, S., et al. (2012). Automated high-throughput neurophenotyping of zebrafish social behavior. J. Neurosci. Methods 210, 266–271. doi: 10.1016/j.jneumeth.2012.07.017
Hinz, F. I., Aizenberg, M., Tushev, G., and Schuman, E. M. (2013). Protein synthesis-dependent associative long-term memory in larval zebrafish. J. Neurosci. 33, 15382–15387. doi: 10.1523/JNEUROSCI.0560-13.2013
Jones, L. J., and Norton, W. H. J. (2015). Using zebrafish to uncover the genetic and neural basis of aggression, a frequent comorbid symptom of psychiatric disorders. Behav. Brain Res. 276, 171–180. doi: 10.1016/j.bbr.2014.05.055
Krause, J., Butlin, R. K., Peuhkuri, N., and Pritchard, V. L. (2000). The social organization of fish shoals: a test of the predictive power of laboratory experiments for the field. Biol. Rev. Camb. Philos. Soc. 75, 477–501. doi: 10.1111/j.1469-185X.2000.tb00052.x
Ladu, F., Butail, S., Macrí, S., and Porfiri, M. (2014). Sociality modulates the effects of ethanol in zebra fish. Alcohol. Clin. Exp. Res. 38, 2096–2104. doi: 10.1111/acer.12432
Lopes, G., Bonacchi, N., Frazão, J., Neto, J. P., Atallah, B. V., Soares, S., et al. (2015). Bonsai: an event-based framework for processing and controlling data streams. Front. Neuroinform. 9:7. doi: 10.3389/fninf.2015.00007
Maaswinkel, H., Zhu, L., and Weng, W. (2013). Assessing social engagement in heterogeneous groups of zebrafish: a new paradigm for autism-like behavioral responses. PLoS ONE 8:e75955. doi: 10.1371/journal.pone.0075955
Menezes, F. P., Kist, L. W., Bogo, M. R., Bonan, C. D., and Da Silva, R. S. (2015). Evaluation of Age-Dependent Response to NMDA Receptor Antagonism in Zebrafish. Zebrafish 12, 137–143. doi: 10.1089/zeb.2014.1018
Miller, N., and Gerlai, R. (2012). From schooling to shoaling: patterns of collective motion in zebrafish (Danio rerio). PLoS ONE 7:e48865. doi: 10.1371/journal.pone.0048865
Mooney, R. (2014). Auditory-vocal mirroring in songbirds. Philos. Trans. R. Soc. Lond. B. Biol. Sci. 369, 20130179. doi: 10.1098/rstb.2013.0179
O'Connell, L. A., and Hofmann, H. A. (2011). The vertebrate mesolimbic reward system and social behavior network: a comparative synthesis. J. Comp. Neurol. 519, 3599–3639. doi: 10.1002/cne.22735
O'Connell, L. A., and Hofmann, H. A. (2012). Evolution of a vertebrate social decision-making network. Science 336, 1154–1157. doi: 10.1126/science.1218889
Oliveira, R. F. (2013). Mind the fish: zebrafish as a model in cognitive social neuroscience. Front. Neural Circuits 7:131. doi: 10.3389/fncir.2013.00131
Orger, M. B., Kampff, A. R., Severi, K. E., Bollmann, J. H., and Engert, F. (2008). Control of visually guided behavior by distinct populations of spinal projection neurons. Nat. Neurosci. 11, 327–333. doi: 10.1038/nn2048
Pantaleone, J. (2002). Synchronization of metronomes. Am. J. Phys. 70, 992–1000. doi: 10.1119/1.1501118
Pietri, T., Roman, A.-C., Guyon, N., Romano, S. A., Washbourne, P., Moens, C. B., et al. (2013). The first mecp2-null zebrafish model shows altered motor behaviors. Front. Neural Circuits 7:118. doi: 10.3389/fncir.2013.00118
Richardson, M. J., Marsh, K. L., Isenhower, R. W., Goodman, J. R. L., and Schmidt, R. C. (2007). Rocking together: dynamics of intentional and unintentional interpersonal coordination. Hum. Mov. Sci. 26, 867–891. doi: 10.1016/j.humov.2007.07.002
Scerbina, T., Chatterjee, D., and Gerlai, R. (2012). Dopamine receptor antagonism disrupts social preference in zebrafish: a strain comparison study. Amino Acids 43, 2059–2072. doi: 10.1007/s00726-012-1284-0
Sears, L. L., Finn, P. R., and Steinmetz, J. E. (1994). Abnormal classical eye-blink conditioning in autism. J. Autism Dev. Disord. 24, 737–751. doi: 10.1007/BF02172283
Sebanz, N., Bekkering, H., and Knoblich, G. (2006). Joint action: bodies and minds moving together. Trends Cogn. Sci. 10, 70–76. doi: 10.1016/j.tics.2005.12.009
Senju, A., Maeda, M., Kikuchi, Y., Hasegawa, T., Tojo, Y., and Osanai, H. (2007). Absence of contagious yawning in children with autism spectrum disorder. Biol. Lett. 3, 706–708. doi: 10.1098/rsbl.2007.0337
Sison, M., and Gerlai, R. (2011). Behavioral performance altering effects of MK-801 in zebrafish (Danio rerio). Behav. Brain Res. 220, 331–337. doi: 10.1016/j.bbr.2011.02.019
Sovrano, V. A., and Andrew, R. J. (2006). Eye use during viewing a reflection: behavioural lateralisation in zebrafish larvae. Behav. Brain Res. 167, 226–231. doi: 10.1016/j.bbr.2005.09.021
Winfree, A. T. (1967). Biological rhythms and the behavior of populations of coupled oscillators. J. Theor. Biol. 16, 15–42. doi: 10.1016/0022-5193(67)90051-3
Keywords: social behavior, zebrafish model system, NMDA receptors, ethanol, visual behavior
Citation: Dreosti E, Lopes G, Kampff AR and Wilson SW (2015) Development of social behavior in young zebrafish. Front. Neural Circuits 9:39. doi: 10.3389/fncir.2015.00039
Received: 12 June 2015; Accepted: 23 July 2015;
Published: 18 August 2015.
Edited by:
Claire Wyart, Brain and Spinal Cord Institute, FranceReviewed by:
Filippo Del Bene, Institut Curie, FranceHarold Burgess, National Institute for Child Health and Human Development, USA
William Norton, University of Leicester, UK
Copyright © 2015 Dreosti, Lopes, Kampff and Wilson. This is an open-access article distributed under the terms of the Creative Commons Attribution License (CC BY). The use, distribution or reproduction in other forums is permitted, provided the original author(s) or licensor are credited and that the original publication in this journal is cited, in accordance with accepted academic practice. No use, distribution or reproduction is permitted which does not comply with these terms.
*Correspondence: Elena Dreosti and Steve W. Wilson, Department of Cell and Developmental Biology, University College London, Gower Street, London WC1E 6BT, UK, e.dreosti@ucl.ac.uk; s.wilson@ucl.ac.uk