- 1Member Research Committee, School of Medicine, Guilan University of Medical Sciences, Rasht, Iran
- 2Department of Neurology, Neurosciences Research Center, Poursina Hospital, School of Medicine, Guilan University of Medical Sciences, Rasht, Iran
- 3Department of Internal Medicine, Regenerative Medicine Research Center, Razi Hospital, School of Medicine, Guilan University of Medical Sciences, Rasht, Iran
Multiple sclerosis (MS) is an immune-mediated disorder involving the central nervous system (CNS), in which demyelination is caused. The initiation and progression of MS is thought to depend largely on CD4+ T lymphocytes, yet new data has emphasized the involvement of the innate immune system in the MS disease responses. Generally, several types of immune cells play a part, with natural killer (NK) cells being essential. Different subsets of natural killer cells function differently within the course of an autoimmune disease, such as MS. There are mainly two types of natural killers in humans: immature CD56bright CD16– and mature CD56dim CD16+ natural killers, together with their respective subtypes. Factors from natural killers expand the T cell population and control the process by which native CD4+ T cells differentiate into Th1 or Th2 lymphocytes, which affect autoimmune responses. Natural killer subsets CD56bright and CD56dim may have differing roles in MS development. The impact of these NK cell subsets is influenced by factors such as Granzymes, genetics, infections, TLR, and HSP. We reviewed and evaluated the relationship between natural killer cells and MS.
1 Introduction
The leading contributor to neurological disability in young people is multiple sclerosis (Mastorodemos et al., 2015). It refers to an immunological disorder affecting the CNS and involves inflammatory and demyelinating processes (Oh et al., 2018; Assari, 2020). Multiple sclerosis can affect any central nervous system component, leading to various disease manifestations (Doshi and Chataway, 2017). Both adaptive and innate immune systems are involved in MS pathogenesis (Liu et al., 2021). Different subsets of Natural killer cells function differently within the course of an autoimmune disease, such as MS (Gianchecchi et al., 2021).
MS may be affected or improved by natural killers through mechanisms of immunoregulation (Esmaeil Amini et al., 2020; Ahmadi et al., 2022). These cells are the main producers of cytokines, including TNF-α, GM-CSF, and IFN-γ. There are two major types of natural killer cells in humans: CD56bright and CD56dim NK cells (Schwane et al., 2020). CD56, which is often present in natural killers, is considered an important marker of treatment outcome (Laroni and Uccelli, 2020). Previously, research demonstrated that natural killer cells carry out various functions, including stimulating tissue and cell growth, exerting antitumor and antiviral activities, and modulating and modulating inflammatory responses (Poznanski and Ashkar, 2019; Bakhshi et al., 2023). Under particular conditions, NK can modulate autoimmune activity in the CNS.
Natural killer cells are multifunctional innate immune cells that are instrumental in providing the host defense and regulating the immunity of the host. The involvement of NK lymphocytes in MS disease course and treatment outcome is suggested by evidence from both individuals with multiple sclerosis and experimental autoimmune encephalomyelitis (EAE), an animal model of MS. However, their exact role is disputed. The impact of NK cell functions on MS pathogenesis remains unclear since research has primarily focused on peripheral natural killer cells instead of local tissue-resident natural killer cells and reported inconsistent results (Mimpen et al., 2020; Dhaiban et al., 2021; Mi et al., 2021). In our review study, we investigate the relationship between NK lymphocytes and MS and the impact of different NK cell subsets (protective or pathologic) on MS progression.
2 Characteristics and general features of multiple sclerosis
In addition to sensory impairments, bladder malfunctions, mental impairments, limb weakness, and incoordination could vary based on the location of the pathological changes in the central nervous system (Sadeghi Hassanabadi et al., 2022). It is common to find lesions around the brain’s ventricles in white and gray matter. The interplay of various effectors and immunity-regulatory cells may influence inflammatory processes in the CNS. MS can be divided into three subtypes: relapse remitting MS (RRMS), primary progressive MS (PPMS), and secondary progressive MS (SPMS) (Bakhshi et al., 2023). Typically, multiple sclerosis manifests as flare-ups, and then intermittent clinical stability for the majority of patients, but 10% of people progress slowly and have no neurological recovery from the onset (Kaur et al., 2013). A transient inflammatory response of the CNS causes damage to myelin and axons, leading to neurological dysfunction in the early stages (Beliën et al., 2022). Even though MS has axonal degeneration at an early stage, inflammation in MS is more extensive and chronic than that of primary neurodegenerative disorders or acute brain damage (Hemmer et al., 2002). T lymphocytes are the essential component in the development of the neurodegenerative MS in the CNS. The involvement of the innate immune system in the MS disease reaction has been emphasized by recent studies (Calahorra et al., 2022).
Multiple sclerosis involves Th lymphocytes that are suspected to be pro-inflammatory, such as Th1, Th17, and GM-CSF-secreting effector cells, as well as follicular Th cells. These effector cell subsets are likely to show abnormal activity because CD4+ regulatory T lymphocytes lack normal function. CD4+ T cells capable of cytotoxicity are thought to be involved in the process of multiple sclerosis, whether by causing damage or by helping through the controlled elimination of other pro-inflammatory cells. Follicular Th lymphocytes are recognized by their simultaneous expression of CXCR5 and PD-1 and through the release of IL-21 and IL-4, which are essential for B lymphocyte survival, proliferation, and plasma cell differentiation (Ahmadi et al., 2022). Follicular Th lymphocytes can enhance Th17 and B lymphocyte reactions, thus increasing neuroinflammatory responses. Even though CD4+ T lymphocytes might have a significant influence on the peripheral immune processes that lead to multiple sclerosis, it has been known for a long time that CD8+ T lymphocytes are more prevalent than CD4+ T lymphocytes in the brain lesions of MS patients. CSF CD8+ T lymphocytes associated with multiple sclerosis have active and memory phenotypes and can release effector cytokines, including IFN-γ, TNFα, and IL-17. Understanding the pathogenesis of multiple sclerosis requires a basic understanding of the blood-brain barrier (BBB), the integrity of which is compromised by MS lesions (Høglund and Maghazachi, 2014; Romaniuc et al., 2020). B cells primarily contribute to CNS autoimmunity by producing antibodies that react against myelin and cause demyelination and axonal damage. They are also responsible for presenting myelin components to T cells, which leads to the production of antibodies and promotes autoreactivity (Mastorodemos et al., 2015).
T lymphocytes that are activated can invade the CNS parenchyma, causing inflammation by secreting cytokines, including IL-17 and Interferon gamma (IFN-γ). As a result of these pro-inflammatory cytokines, other leukocytes are attracted to the site, and there is direct damage to neural tissue (Galicia and Gommerman, 2014). When properly stimulated, human invariant natural killer (iNKT) cells produce pro-inflammatory and anti-inflammatory cytokines, indicating they are a powerful immune control mechanism. iNKT cells predominantly produce pro-inflammatory molecules, notably IFN-γ and Tumor Necrosis Factor-alpha (TNF-α) (Yamamura et al., 2007), regarding the microglial cells, and depending on the inflammatory environment in the CNS. They can release anti-inflammatory substances (TGF-ß and IL-10). It has been suggested that microglia contribute to neurogenesis because they secrete neurotrophins, including brain-derived neurotrophic factor (BDNF), insulin-like growth factor-1 (IGF-1), and Neurotrophin 3 (NT-3) (Gandhi et al., 2010).
3 Natural killer cell
3.1 NK cell subsets
Natural killer cells are essential to immunity as a front-line immune response against viral pathogens and neoplasms (Kaur et al., 2013). Further to the cytotoxic functions of natural killers, which are essential to the innate immune system, they are capable of suppressing T lymphocytes either through the elimination of antigen-presenting cells or by the release of immunosuppressive substances, including IL-10. Anti-inflammatory cytokines are abundantly produced by the NK cell subset, which performs a “regulatory” function (Cooper et al., 2001). The chemokines released by these cells may further promote the accumulation of other regulatory leukocytes, namely FoxP3+ regulatory NK cells (Segal, 2007). NK cells are derived from CD34+ hematopoietic stem cells (HSCs), undergoing distinct developmental phases marked by the progressive expression of surface receptors and functional maturation. These progenitor cells, including lineage-committed precursors, may migrate from the bone marrow to peripheral tissues, where terminal differentiation occurs. Early precursors are characterized by the absence of CD34 and the presence of CD117, CD244, and CD161, but lack CD56 expression (Stabile et al., 2015). As maturation progresses, CD56 is acquired, followed by sequential expression of activating receptors such as NKp46, NKG2D, and DNAM-1. This transition culminates in a phenotype resembling peripheral blood (PB) CD56bright NK cells, which exhibit surface markers like NKp44 (Bozzano et al., 2015; Scoville et al., 2017).
Peripheral blood NK cells exist as heterogeneous populations, reflecting divergent differentiation states or functional specializations (Montaldo et al., 2013). The primary subsets are distinguished by surface density of CD56: CD56bright and CD56dim NK cells. Historically, CD56bright cells were associated with cytokine secretion, whereas CD56dim subsets were linked to cytotoxicity (Weber et al., 2024). Recent evidence, however, demonstrates that cytotoxic CD56dim cells also secrete substantial cytokines upon receptor activation (Cooper et al., 2001). A large percentage of circulating and spleen natural killers consist of CD56dim CD16+ NK cells. Upon interacting with tumor cells, these cytotoxic cells produce IFN-γ (Sadeghi Hassanabadi et al., 2022). When CD56bright NK cells encounter a dangerous or cancerous cell, they create an inflammatory condition that helps to eliminate the cancerous cells. However, this pro-inflammatory condition can also worsen autoimmune disorders, such as MS, if the immune system is reactivated (Mimpen et al., 2020). Most NK cells in a lymph node and tonsil are CD56brightCD16– NK cells and do not produce perforin; however, they release molecules, including IFN-γ, as a result of IL-12, 15, and 18 stimulation (Sadeghi Hassanabadi et al., 2022). CD56bright NK cells act as immunoregulators by producing cytokines as a result of chemical signals. As well as producing granzyme K, CD56bright NK cells eliminate active CD4+ T lymphocytes. Natural killers with high expression of CD56 antigen (CD56bright NK cells) do not exhibit killer-cell immunoglobulin-like receptors (KIR) (Fehniger et al., 2003; Rodriguez-Mogeda et al., 2024). They exhibit heightened responsiveness to cytokine signaling via receptors like IL-2R and IL-15R but reduced sensitivity to direct cellular interactions (Mimpen et al., 2020).
Multiple subsets of NK cells are believed to play a role in immunoregulatory processes in MS, based on studies of peripheral blood and cerebrospinal fluid. Dendritic cells (DCs), pivotal initiators of immune responses, modulate NK cell activity through direct contact and release of soluble mediators, including type I interferons, IL-12, IL-15, and IL-18. Among these, IL-12 is critical for enhancing NK cell proliferation and interferon-γ production, and had long been regarded as the central mediator amplifying signaling pathways critical to natural killer cell effector capabilities (Lagumersindez-Denis et al., 2017). Natural killer cells have various surface markers, which allow different methods to classify them into different groups. CD56dim NK cells have anti-tumor cytotoxic activity, while each group can produce cytokines. Both NK cell groups have NKp46- and NKp80-activating surface receptors. CD56bright NK cells differentiate CD56dim NK cells by the expression of CD16 and PEN5 (Roma, 2021; Chu et al., 2022). Human CD3–CD56+ NK subsets express low levels of CD8. The CD56+ CD8low subset of human NK cells is capable of a greater degree of cytolytic activity than CD8– subsets (Lowdell et al., 2002). Subsequently, this was expanded to include acute myeloid leukemia cases in complete remission following monotherapy chemotherapy (Addison et al., 2005). A second way to distinguish them is through their cytokine profiles, such as NK1 and NK2, which express IFN-γ and IL-10 (NK1) or IL-5 and IL-13 (NK2) (Lagumersindez-Denis et al., 2017). NK cells with CD56dim subsets have primarily cytotoxic functions, whereas NK cells with CD56bright subsets secrete an abundance of inflammatory cytokines that normally act as regulators (Campbell and Hasegawa, 2013). It is estimated that CD56dim NK cells produce ten times the amount of perforin and granzyme A in their granules than their CD56bright NK cells counterparts. So, CD56dim NK cells could have a more remarkable cytolytic ability due to this feature. However, NK cells with CD56bright NK cells surfaces secrete more immunological cytokines, including TNF-α, IFN-γ, and IL-10 (Jacobs et al., 2001).
3.2 NK Cell activator, inhibitor, and associated signaling pathway
Natural killer cells express numerous receptors that can activate their cytotoxic and secretory functions. These receptors identify ligands on the surface of infected, transformed, or stressed cells (NKG2D). Signaling through inhibitory receptors also equips NK cells with appropriate responsiveness via a process known as licensing (KIR) (Kumar, 2018). Different mechanisms are involved in regulating NK cell activation in order to minimize unwanted reactions. Initially, natural killer cells possess inhibitory receptors capable of recognizing a wide variety of ligands. In addition, upregulating host ligands for activating receptors may result in inadvertent damage (Fogel et al., 2013). The NK cell’s surface is coated with various germline-encoded inhibitory and activating receptors. HLA genes encode classic or nonclassical major histocompatibility complex (MHC) class I proteins, inhibiting NK cell function by binding to them (Paul and Lal, 2017). Some activated receptors recognize ligands similar to inhibitory receptors, and some identify molecules with a structure consistent with MHC class I molecules, which have been increased by cellular stress. Human tissues normally have low levels of MICA and MICB, yet these molecules are expressed at higher levels in epithelial tumors and stressed cells. MHC-like molecules, including MICA and RAE-Iβ, that increase in expression under stress bind to and are detected by the activating receptor NKG2D (Deng and Mariuzza, 2006).
As natural killers detect ligands that are produced in response to stress on their targets via natural cytotoxicity receptors, distressed cells activate them (Long and Rajagopalan, 2002). Various inhibitory receptors are present in activated cells, as demonstrated in previous studies. One is the killer-cell immunoglobulin-like receptor family (KIR), which interacts with HLA-I. Without the presence of “self” ligands, natural killers activate and kill their targets as a result (Pende et al., 2019). KIR receptors provide inhibitory and activating signals that determine NK cell responses, but cytokine stimulation also influences NK cell activation thresholds (Long et al., 2013). Ligands that are widely distributed on the host’s membrane, called MHC class I, prevent natural killer cells from responding. Nevertheless, a lower activation threshold can be observed when infection is present (Le Maux Chansac et al., 2005; Zucchini et al., 2008). Consequently, the increased production of natural killer triggers on host cells is minimized when cellular stress or infection is absent (Frank and Paust, 2020).
4 Demographic and immunological effects on NK cells in MS patients
4.1 Age and NK cell
As individuals age, their immune cell subpopulations undergo alterations in composition (Table 1). Immunosenescence refers to age-associated alterations in immune function that elevate susceptibility to age-related diseases and mortality risk in elderly populations. This progressive deterioration of immune competence alters key immunological functions, including dysregulation of inflammatory responses, impaired antimicrobial defenses, and heightened predisposition to autoimmune conditions such as multiple sclerosis (Dema et al., 2025). Age-associated modifications in NK cell populations and functional capacities may indirectly modulate MS pathogenesis.
The aging process, along with the proportion of different types of NK cells, possibly impacts the activity, secretion, and cytotoxicity of NK cells (Yan et al., 2010). This decrease in binding can be ascribed to an age-related impairment in secreting perforin, which can be attributed to an impaired alignment of lytic granules toward the immunological synapse (Hazeldine et al., 2012). The impact of aging on the production of cytokines and chemokines by CD56 NK cells remains unclear (Lutz et al., 2011). However, it can be speculated that decreased expression of CD94 may lead to more rigorously regulated NK cell activity and potentially a reduction in NK cell killing (Martínez-Rodríguez et al., 2010). In contrast, distinct activating receptors elicit the release of cytokines (such as IFN-γ and TNF-α). In the context of NK cell receptors, which can be either inhibitory or activating, the expression of certain receptors is influenced by aging (Fauriat et al., 2007). This is consistent with previous research that has demonstrated a reduction in CD56bright NK cells and an increase in CD56dim NK cells in healthy aging. Consequently, older subjects have a higher ratio of CD56dim to CD56bright NK cells (Kutzelnigg et al., 2005).
The precise role of NK cells in aging and their impact on MS remain incompletely understood. However, age-related shifts in NK cell subpopulations provide a basis for hypothesizing potential mechanisms linking Immunosenescence to MS pathophysiology. Age-associated declines in perforin secretion capacity, reduced CD94 receptor expression, and diminished cytotoxic activity of NK cells may attenuate neuroinflammation and decelerate autoimmune processes in MS. Conversely, decreased frequencies of s CD56bright NK cells might compromise their immunoregulatory functions, potentially exacerbating MS progression. While these theoretical pathways offer plausible explanations, rigorous experimental and clinical studies are necessary to elucidate the complex interplay between NK cell aging and MS pathophysiology.
4.2 NK cell frequency and rolls in MS types
Various subsets of NK cells appear to play varying roles in MS initiation or progress. CD56dim NK cells exhibit cytotoxic activity, whereas CD56bright NK cells release substantial quantities of anti-inflammatory cytokines, contributing to their modulatory activity (Cooper et al., 2001). The total number of NK cells in circulation was lower in untreated RR-MS patients in comparison with both healthy individuals and treated RRMS participants (Gayoso et al., 2011). Specifically, the subset of CD3–CD16– CD56bright NK cells showed a significant increase in treated RR-MS cases in comparison with healthy individuals and untreated RRMS patients. The production of IL-10 by the CD3–CD16–CD56bright NK cells was increased in treated RRMS individuals (Tahrali et al., 2018).
A higher percentage of CD3–CD56dim NK cells that produce perforin is observed in patients with PPMS and SPMS as opposed to those without these conditions, suggesting that this particular group is involved in the progression of these types of MS. These forms are characterized by a continuous progressing condition and more widespread damage to the axons, even in inactive lesions and normal areas of white matter (Ruder et al., 2021). Notably, no significant disparities were observed in the proportions of circulating CD3–CD56dim NK cells perforin+ natural killers between PPMS and SPMS participants. In an investigation comparing individuals diagnosed with PPMS and SPMS during remission phases to healthy subjects, Plantone and colleagues observed that the higher percentage of peripheral perforin-expressing CD56dim NK cells in PPMS and SPMS cases indicates that they may contribute to developing these MS forms. Additionally, the proportion of CD3–CD56bright NK cells showed a negative correlation with age in individuals with PPMS, while the proportion of CD3–CD56dim NK cells showed a positive correlation with age (Plantone et al., 2013). Previous studies have also reported a natural decline in the CD3– CD56bright NK cells subset and an increase in the CD3– CD56dim NK cells subset with healthy aging (Gayoso et al., 2011).
When considering their ratio, it appears that the proportion of natural killer cells and their subpopulations relative to CD4+ T cells and IL-17A+ CD4+ T lymphocytes play a significant role in determining pathogenic activity. In a study examining the clinical significance of immune cell ratios in multiple sclerosis, Mimpen and colleagues investigated NK cell and T lymphocyte subpopulations in peripheral blood samples to assess their association with disease progression. The analysis included baseline peripheral blood mononuclear cell specimens collected from 50 participants with relapsing-remitting multiple sclerosis. Researchers identified significantly reduced proportions of NK cells relative to CD4+ T lymphocytes, as well as diminished CD56dim NK cell-to-CD4+ T cell ratios, among patients demonstrating MRI-detectable inflammatory lesions. These findings propose that quantifying NK cell and CD4+ T lymphocyte balance could serve as a predictive biomarker for identifying subclinical or active neuroinflammatory processes in MS (Mimpen et al., 2021). However, further investigation using additional datasets from separate sources is necessary to validate the accuracy and usefulness of NK/CD4+ subpopulation ratios as indicators of prognosis in RRMS.
Through the phenotypic analysis of CD56dim NK cells, it was found that patients in remission exhibited higher frequencies of cells expressing HLA-DR and CD54, while the frequency of CD11c-expressing NK CD56dim NK cells was lower (Aranami et al., 2006). Recent findings suggest that remission RRMS patients, who are likely in an inactive disease state, have circulating CD56dim NK cells characterized by an activated phenotype marked by HLA-DR expression. Interestingly, remission RRMS patients displayed increased frequencies of CD54– expressing NK cells, indicating that NK cells may have the ability to migrate to sites of inflammation. For a more comprehensive understanding, it would be beneficial to investigate the expression of a broader range of molecules to better elucidate the functional characteristics of NK cells and their subpopulations (Darlington et al., 2018). Furthermore, additional research is needed to assess the direct involvement of CD56 NK cells in the detrimental effects on axons within MS lesions and their influence on disease activity across different forms of MS (Beliën et al., 2022).
CD56bright NK cells eliminate (autologous) active T lymphocytes, severely restricting T lymphocyte activity (Jiang et al., 2011). It appears that CD56bright NK cells proliferate more rapidly than Treg. It proves how CD56bright NK cells are immunoregulatory and protective in MS (Caruana et al., 2017). In addition to CD56bright NK cells, CD56dim NK cells eliminate autologous antigen-activated CD4+ T lymphocytes. A variety of stimulants activate natural killers to restrain T lymphocyte proliferation or to increase their cytotoxicity (Gross et al., 2016; Figure 1).
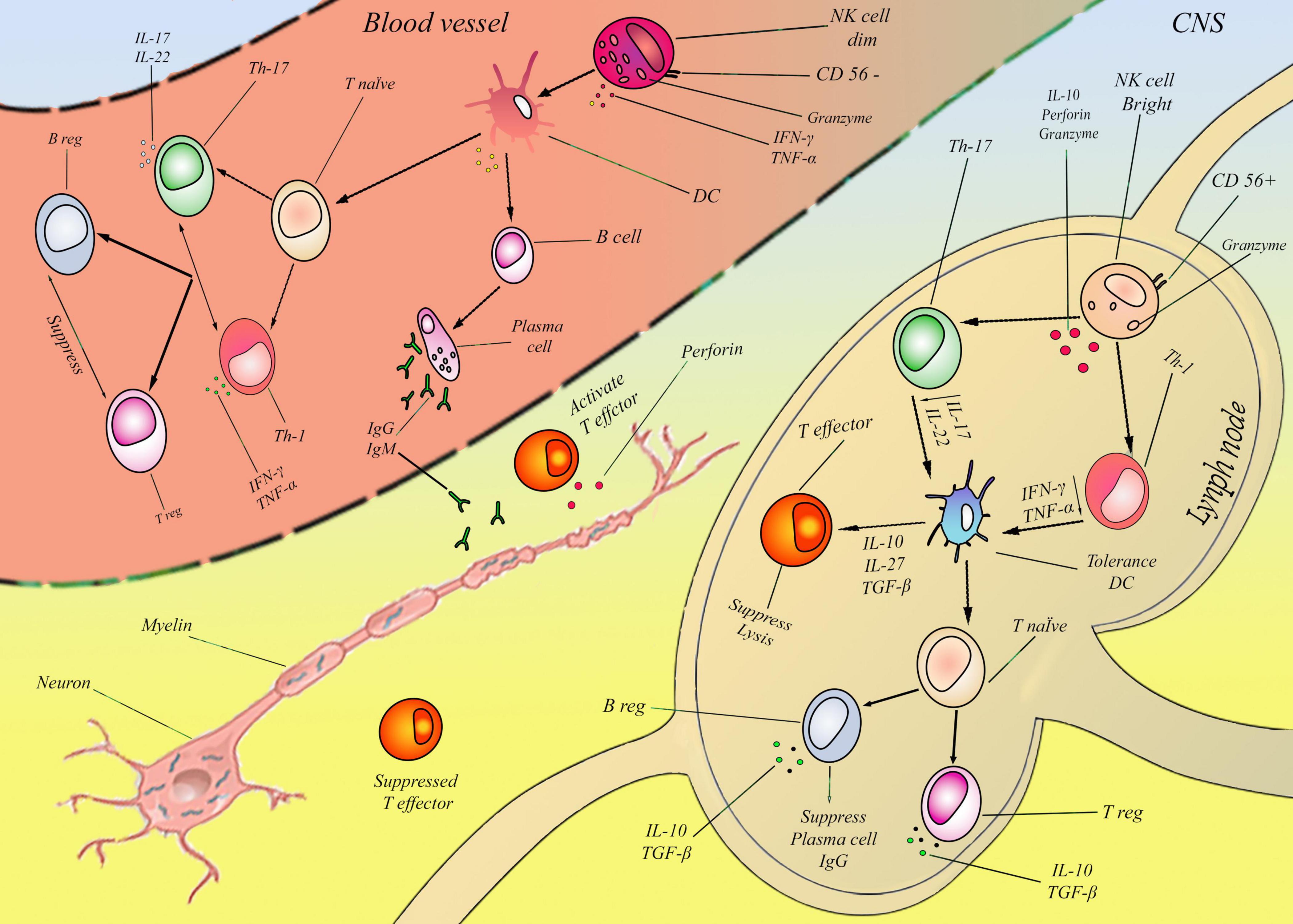
Figure 1. According to previous studies, myelin degeneration may be associated with NK cells in two ways: bright and dim NKs. These two cells’ effect on MS was proved, but their detailed mechanism is almost unknown. In this figure, a new mechanism is presented for two NK cells. Lymph node: In the first step CD56bright NK cells produce Granzyme and IL-10 by default. IL-10 suppresses Th-1,17 and subsequently reduces IFN-γ, TNF-α, and IL-17, IL-22 in the second step. Reduction of these cytokines tolerated DCs and induced IL-10, 27, and TGF-β in the third step. In the fourth step, in cellular immunity, the T effector and cell lysis mechanism are suppressed, and T native cells differentiate to T-reg, consequently inducing IL-10 and intensifying this cycle. Also, in humoral immunity, the plasma cell was suppressed and induced B-reg with TGF- β. In sum, CD56brightcells suppressed two mains humoral (Plasma cell/IgG) and cellular (effector T) pathways, mainly by IL-10 and TGF- β. Blood vessels: CD56dim NK cells in the first step produce IFN-Y and TNF-a. Subsequently, in the second step, these two cytokines directly activated DCs and continuously affected cellular and humoral systems by inflammatory cytokines in the third step. In the cellular immune system, T-native cells differentiated to Th-1, 17 and induced the production of IFN-γ, TNF-α, and IL-17, IL-22. These factors activated T-effectors and suppressed T-reg cells. Also, in the humoral immunity system, B cells are converted to plasma cells and induced IgG and IgM. In sum, CD56dim cells activated two main humoral (Plasma cell/IgG, IgM) and cellular (effector T) pathways.CD56dim NK cells developed demyelination by activating auto-antibody and perforin from plasma cells and effector T cells, and CD56brightcells inhibit demyelination by suppressing plasma cells and effector T cells. It is important to emphasize that this figure only outlines the fundamental and overarching pathways involving natural killer (NK) cells in the context of multiple sclerosis (MS), while omitting various influential factors such as therapeutic interventions.
The CD56dim CD16+ NK cells secretes type 1 cytokines like IFN-γ and TNF-α, while the CD56bright CD16– NK cells secretes type 2 cytokines like IL-4 and IL-5 (Takeyama et al., 2021). CD56bright CD16– NK cells produce Th2 cytokines, including IL-4, IL-5, and IL-13, and are abundant in Fas (CD95) (Chen and Wang, 2019). Anti-CD3 and anti-CD52 monoclonal antibodies co-stimulate the ex vivo growth of natural killer cells from human peripheral blood mononuclear cells. Additional data showed the modulating role of natural killer cells, which is linked to CD95 expression reduction before relapse (Takahashi et al., 2004). It has been shown that MS patients in remission have a higher CD56bright CD16– NK cell population than those with relapse, implying that this subpopulation is beneficial to the remission process. In addition, CD56bright CD16– NK cells seem to influence antigen-specific autoreactive T-cell activation negatively (Takahashi et al., 2004).
4.3 Infection and NK cell
Viral infections are among the main environmental risk elements associated with MS, and NK lymphocytes are essential for the host’s defense against them (Table 1). The role of NKG2C+ NK lymphocyte proliferation in MS, which may be caused by Human Cytomegalovirus (HCMV) infection or other immune effects, is unclear (Hammer et al., 2018). CMV may have a protective effect on MS pathogenesis due to its ability to configure the immune system (Waggoner et al., 2011). For instance, one hypothesis suggests that CMV infection generates memory-like natural killer cells that are very effective against herpesviruses, such as EBV. CMV infection also appears to cause various modifications in the immunity, especially in NK and T lymphocytes (Moreira et al., 2019). Some studies have shown that NK lymphocytes work as immunoregulators and that the NKG2C+ NK lymphocyte subpopulation could influence the adaptive immune processes involved in MS development (Picarda and Benedict, 2018). Thus, HCMV could have a positive impact on MS, reducing disability worsening risk in patients with NKG2C+ NK lymphocyte proliferation induced by the virus. In a study assessing the immunological effects of latent CMV infection in individuals with MS, Prii and colleagues conducted flow cytometric immunophenotyping of NK cells, CD8+ T lymphocytes, and NKT-like cells in peripheral blood samples. Their analyses revealed distinct phenotypic profiles in these immune subsets among CMV-seropositive MS patients, marked by elevated expression of the activating NKG2C surface receptor. Furthermore, higher NKG2C receptor density was positively correlated with elevated Expanded Disability Status Scale (EDSS) scores, suggesting a potential link between CMV-driven immune adaptations and neurological disability progression in MS (Perri et al., 2024).
NK lymphocytes may interact with EBV through several mechanisms. As key components of the innate immunity against viruses, NK cells participate in the initial defense against EBV infection. An increase of NK lymphocytes is observed in infectious mononucleosis, which is related to a lower count of viruses in some cases (Bakhshi et al., 2024). The infection also seems to induce phenotype alterations in NK lymphocytes, such as an increase of NKG2A expression but not a memory-like NK cell phenotype (Chijioke et al., 2013).
The heterogeneous clinical outcomes of HSV-1 (Herpes simplex virus) infections indicate that host genes affecting susceptibility play a role in its regulation. MS patients who have the KIR2DL2 gene showed reduced activation of NK lymphocytes and slower elimination of HSV-1, according to a prior investigation (Cassidy et al., 2015). KIR2DL2 and the subsequent SHP1-SHP2 signaling pathway impair the efficiency of NK lymphocytes in controlling HSV-1 development. In contrast, the lack of KIR2DL2 or the inhibition of the SHP1-SHP2 signaling pathway is thought to protect against viral infections by facilitating an immune response to counteract viral invasion. The first investigation of the cytokine profile changes due to KIR2DL2 expression on NK lymphocytes revealed distinct cytokine secretion in KIR2DL2+ MS patients. KIR2DL2+ NK lymphocytes from individuals with MS produced a significant amount of Th17 cytokines, primarily IL-17A, throughout the HSV-1 infection course (Rizzo et al., 2016; Hedayati-Ch et al., 2024). Conversely, MS patients’ KIR2DL2- NK lymphocytes and NK lymphocytes from both KIR2DL2+ and KIR2DL2– control subjects secreted Th1 cytokines, mainly IFN-γ. Interestingly, the variation in cytokine release among individuals with multiple sclerosis does not appear to be correlated with a distinct distribution of CD56bright CD56dim NK lymphocyte subpopulations in MS subjects who possess the KIR2DL2 receptor and those who do not (Bortolotti et al., 2022).
Herpesviruses that may influence MS development have been shown to modify NK phenotype, indicating the relevance of their phenotypes in predicting specific pathogens infections in MS. EBV infection could promote the expansion of early differentiated NK cells (CD56dim CD57–) (Azzi et al., 2014).
4.4 HSP and NK cells
We demonstrated in a previous paper that the development of EAE was inhibited by pretreatment of mice with Hsp70 (Heat shock protein) complexed with CNS tissue-isolated non-characterized peptides and that this mechanism hinged on NK lymphocyte activity. In addition, the immunoregulation of EAE resulted from alterations in Dendritic Cell (DC) antigen presentation, which was caused by interactions involving Hsp70–peptide complexes and NKG2D receptor (Galazka et al., 2007). Tolerance to this autoimmune disease was induced by non-characterized peptide/Hsp70 complexes derived from the CNS of EAE mice. The activation of NK lymphocytes and the involvement of the NKG2D receptor and its ligand, H60, were the basis of the tolerogenic effect of the peptides complexed with Hsp70 (Galazka et al., 2006). A CNS-derived peptide, HINT138–57, which was administered in complexed form with Hsp70 by pretreatment, modulated NK cell-dependent immunity in animals sensitized to EAE. The immunoregulation mediated by NK lymphocytes was based on dual receptor stimulation (CD94 and NKG2D) on the membrane of NK lymphocytes (Galazka et al., 2014). This new mechanism of immunoregulation could deliver significant consequences for the control of autoimmune responses in the CNS and potential treatment strategies in autoimmunity and MS.
4.5 Granzymes and NK cells
Five granzymes are present in the human body: A, B, H, K, and M. The biological effects of granzyme A (GrA) and granzyme B (GrB) are comprehensively understood, but the biological role of the other granzymes is largely unknown. GrB causes rapid cell degeneration, mainly by activating caspases. GrA, on the other hand, causes cell death that does not depend on caspases (Boivin et al., 2009). NK cells cause an apoptotic process, independent of caspases, that involves NK cell degranulation and mitochondrial damage in activated T lymphocytes. GrA and granzyme K (GrK) can both cause this type of cell death; however, GrK is transferred to target cells more preferentially (Jiang et al., 2011). GrK causes cell death that does not depend on caspases and is very similar to GrA-induced cell death in terms of morphology and biochemistry, although GrA and GrK have very different substrate specificity fields (Hay and Slansky, 2022).
Studies involving granzyme transfer suggested that GrK was a critical factor in NK cell-mediated cytotoxicity in response to activated T lymphocytes. This agreed with the findings that GrK was expressed preferentially in CD56bright in NK lymphocytes (Jiang et al., 2011). Cell death in activated T lymphocytes primarily resulted from mitochondrial apoptosis linked to the production of Reactive Oxygen Species (ROS). GrA and GrK were the main inducers of this type of death. There was a much greater level of expression of GrK in the CD56bright NK cell subpopulation of patients who had received treatment (Peng et al., 2021). The findings of this study supported the function of GrK in modulating T-cell responses by CD56bright NK cells. CD56bright NK cells that regulate immunity eliminate autologous activated T cells using a perforin-dependent degranulation pathway that involves two related granzymes, GrK and GrA (Melsen et al., 2016). Also, granzyme B-mediated cell death was reported by Tr1 cells, which were comparable to CD56bright NK cells, on other counts, including the secretion of IFN-gamma and IL-10 at the same time and their induction by IL-27 (Jiang et al., 2011).
CD56bright NK cells were initially regarded as having no cytotoxic ability because they expressed much less perforin and almost no GrB in comparison to CD56dim NK cells (Carrega et al., 2014). CD56bright NK cells expressed levels of GrA that were comparable with CD56dim NK cells, virtually all of which were positive for GrK, whereas CD56dim NK cells (and almost all CD4+ T cells) did not express GrK.
5 Multiple sclerosis and natural killer cell
5.1 Genetic and non-genetic relationship between MS and NK cells
A systematic review thoroughly examined multiple susceptibility loci for multiple sclerosis and their genomic risks (Kemppinen et al., 2011). In addition to genes affecting adaptive immune cells, other genes also affect the innate immune system, including natural killer cells (Li et al., 2021). Consequently, gene variants involved in natural killer lymphocyte function are known to exist in multiple sclerosis. Cell surface glycoproteins such as CD226 appear on cytotoxic lymphocytes, including natural killers (Liu et al., 2017). The CD226 level is generally reduced in MS patients compared to the control group (Liu et al., 2017). In addition, CD226 plays a crucial part in NK cell-mediated T-cell elimination (Ardolino et al., 2011). Many MHC class II risk alleles are associated with MS (such as HLA-DRB1*15:01, HLA-DRB1*13:03, and HLA-DRB1*03:01), while MHC class I alleles may confer protective effects (such as HLA-A*02:01, HLA-B*44:02, and HLA-B*38:01) (Moutsianas et al., 2015).
The environment is considered a factor linked to MS; Vitamin D deficiency, infection with EBV and CMV, smoking, and obesity in adolescents are the most impactful and better understood among the factors related to environmental exposure (Beliën et al., 2022). Vitamin D maintains immune equilibrium by suppressing inflammatory cytokines produced by T lymphocytes (Smolders and Damoiseaux, 2011; Smolders et al., 2011). In vitro, cytotoxicity increases with 1,25-dihydroxy vitamin D treatment of NK cells without altering proliferation (Balogh et al., 1999). Adolescents and adults with EBV infection may develop infectious mononucleosis associated with multiple sclerosis (Cohen, 2000; Loosen et al., 2022). Natural killer cells are major players in the first line of immunity against EBV infection as they participate in innate immunity to the virus (Williams et al., 2005). Additionally, positive CMV serology indicates an increased MS risk, suggesting CMV contributes to disease development (Sundqvist et al., 2014). Tobacco use is associated with an increased risk of developing MS, possibly as a result of its inflammatory properties in the respiratory tracts, which can lead to systemic and chronic inflammatory responses. Smoking continues to play a role in MS pathology once the disease develops by increasing the frequency of relapses and reducing the time to progressive form (Ashare and Wetherill, 2018). The NK cells are affected by smoking in several ways, but an association between altered NK cells and MS currently can’t be established. Conversely, obesity negatively impacts the immunity (Faner et al., 2014; Gerriets and MacIver, 2014; Castoldi et al., 2016). Obesity and decreased physical activity independently increase the risk of developing MS. Obesity is associated with chronic inflammation that may be involved in the pathogenesis of MS. There is a lack of evidence regarding the relevance of these mechanisms to the development of MS (Varghese et al., 2019). Obesity also alters the NK cell population, demonstrating reduced cytotoxicity and cytokine production (Bähr et al., 2017). In this regard, it is impossible to establish a specific link between MS and obesity’s effect on NK cells. As a general rule, both smoking and obesity lead to pro-inflammatory conditions (Mimpen et al., 2020).
5.2 Involvement of different NK cell receptors in MS
NK cell activating and inhibiting receptors are a complex group of receptors that use opposing signaling patterns to enhance or suppress activation. Natural killer cells can eliminate abnormal cells, marked by high expression of stress-responsive ligands, enhancing activating interactions, and by decreased expression of HLA class I molecules, lowering the inhibition caused by HLA-specific inhibitory receptors (Schuster et al., 2014). Natural killer lymphocyte receptors on the surface consist of inhibiting as well as activating molecules, some expressing a variety of unpredictable, diverse, and overlapping forms. The interaction of these receptors on the surface with their corresponding substances controls the functionality of natural killers, and the combination of signals from these receptors determines the activation state of the natural killer lymphocyte (Pegram et al., 2011). Changes in natural killer lymphocyte counts and functionality have been associated with various human autoimmune disorders. For instance, reduced numbers of natural killer cells in the blood, decreased receptor expression, or decreased natural killer function have been seen among individuals suffering from type 1 diabetes and systemic lupus erythematosus (Kucuksezer et al., 2021).
There has been a new perspective on multiple sclerosis predisposition emerging since T cell receptors, and KIRs recognize HLA class I molecules in parallel. Since HLA-A3- restricted CD8+ T lymphocytes play an important role in its activation in a humanized mouse model, it is important to consider the role of natural killer lymphocyte receptors such as KIR3DL2, which are capable of detecting HLA-A3 (Crux and Elahi, 2017). Recently, we have gained further insight into the role of HLA-B and HLA-C alleles as KIR ligands in multiple sclerosis. There are a number of receptors that recognize HLA-Cw5, including KIR2DL1, KIR2DS1, and perhaps also KIR2DL2 and KIR2DL3 located on natural killer cells or a subpopulation of T lymphocytes, indicating that the HLA-KIR pathway may play a role in immune function modulation. There has been a similar protective effect noted for HLA-Bw4 ligands of KIR3DL1 (Jiao et al., 2008).
A family of genes known as CD94/NKG2 can be found on human chromosome 12 as part of the natural killer gene complex. Four NKG2 genes in humans (NKG2A, C, E, and F) were associated with the expression of the NK-activating receptors NKp30, NKp46, and CD94/NKG2D on the surface (Kaur et al., 2013). T-cell activation is reported to be regulated by CD94/NKG2A receptors in EAE. Using antibodies that block Qa1 NKG2A interactions, there is a reduction in cellular infiltrates and activation of microglia, as well as an alteration in the cytokine profile (namely, a reduction in IL-17 and IFN-γ and an increase in IL-4 and IL-10) of CD4+ T cells in the central nervous system (Chewning et al., 2007). Moreover, Qa1 interaction with CD94-NKG2A receptors on CD8+ T cells gives rise to an anti-suppressive response that weakens suppressive functions of CD8+ regulatory T cells; thereby, Qa1-NKG2A interaction disruption results in improved CD8 regulation and decreased EAE progression (Kim and Cantor, 2011; Kaur et al., 2013).
As part of the leukocyte receptor complex on chromosome 19q13.4, there are genes encoding leukocyte immunoglobulin-like receptors (LILRs, LIRs, or CD85) closely related to the KIR genes. Soluble LILRA3 poses a potential competitive characteristic since it contains no transmembrane and cytoplasmic regions. Those with progressive multiple sclerosis are reported to have a larger number of circulating LILRB1+ CD8+ T lymphocytes and LILRB1+ natural killer cells in comparison to patients with relapsing-remitting multiple sclerosis (Storm et al., 2021). During active relapse-remitting multiple sclerosis, there is a reduction in LILRB4 levels in monocytes in the blood. While it is unclear what its ligand is, LILRB4 is capable of inhibiting CD4+ T cell proliferation when expressed on antigen-presenting cells (Liu et al., 2020).
5.3 Mechanisms of immune regulation by natural killers in MS and EAE
Contact-dependent cytotoxicity is a crucial function of NK cells. During this process, cytolytic granules that contain proteases, granzymes, and perforins are released onto the targets through lytic synapses. Additionally, target cells are subjected to caspase-dependent apoptosis by stimulating natural cytotoxic receptors (NCRs) (Nielsen et al., 2012; Laroni et al., 2016; Gauthier et al., 2019). Natural killer cells are thought to prevent autoimmune disorders by reducing autoreactive adaptive immune responses. Mimpen et al. examined the significance of this ratio in the context of individuals with multiple sclerosis by analyzing natural killers and T lymphocyte subsets, in addition to their prognostic significance in terms of disease course (Mimpen et al., 2021). According to them, the CD56bright NK lymphocyte subset is usually regarded as the regulating subset and has an inverse correlation with IL-17A+ CD4+ T cell percentages, but this concept seems to be contradicted by clinical findings (Poli et al., 2009). Activated T lymphocytes can be eliminated by both CD56dim and CD56bright NK cells. Research on natural killer cells in MS has primarily focused on CD56bright natural killers over the past few years, but our findings indicate that CD56dim NK cells have the potential to be of considerable significance (Mimpen et al., 2021).
MS is usually defined as a condition caused by autoimmune inflammation and involving CNS demyelination caused by autoreactive T cells (Thompson et al., 2018). Previously, studies have demonstrated that natural killer cells regulate adaptive immune reactions early in the process of an immune challenge in lymphoid organs. NK cells activated by cytokines possess potent lytic capacity against autologous CD4+ T cells and macrophages when they engage TRAIL receptors on their surfaces (Nielsen et al., 2012; Laroni et al., 2016). Schuster et al. defined an unexpected interaction involving natural killer cells and CD4+ T lymphocytes following chronic viral infections. This interaction occurs in a nonlymphoid tissue, specifically involving TRAIL-expressing NK cells. They demonstrated a novel and unexpected capacity of NK cells in the context of chronic viral infection. In one sense, effector CD4+ T lymphocytes are major components of the immune response to chronic viral infections; however, some subtypes of natural killer cells selectively eliminate effector CD4+ T cells, which in turn reduces autoimmune responses. Taken together, although NK cell-mediated deletion of CD4+ T cells prolongs the chronicity of infection, it also limits the development of autoimmunity in response to viral infections (Schuster et al., 2014). HLA-E detection at high levels in CNS plaques has been linked to increased inactivity and a reduction in NK cytotoxicity among MS patients (Morandi et al., 2013). Lünemann et al. analyzed CD56dim CD56bright subsets of NK cells and the activity of these cells in peripheral blood samples from relapsing-remitting multiple sclerosis patients and healthy donors. Their results indicated that cytokine-stimulated expansion under in vitro conditions, along with IFN-γ secretory capacity, was markedly impaired in CD56brightCD16– NK cells. In contrast, CD56dimCD16+ NK subsets exhibited no such functional compromise. This selective dysfunction in CD56brightCD16– cells was attributed to a cell-autonomous mechanism, as the defect persisted in isolated NK cell populations tested in a separate validation cohort comprising both MS patients and healthy controls. Certain activities of CD56bright NK cells, including the expansion of natural killer cells in response to cytokines and IFN-γ release, are impaired in MS patients’ NK cells (Lünemann et al., 2011).
EAE represents a great animal model that describes how self-reactive CD4+ cells may contribute to MS. EAE is initiated through immunization with antigen-specific T cells for myelin oligodendrocyte glycoprotein (MOG) (Constantinescu et al., 2011). In patients suffering from multiple sclerosis and EAE models, Th17 cells induce disease (Komuczki et al., 2019; Wagner et al., 2020). It is hypothesized that natural killers contribute to the prevention of EAE by inhibiting Th17 responses by killing monocytes that infiltrate the CNS (Jiang et al., 2017).
5.4 Natural killer cells in therapies for MS
Despite the promising therapeutic properties of CD56bright NK cells, there are no treatments available for disease based on this mechanism. Even though other drugs have not been developed to affect NK cells, they may be beneficial. Several drugs were discussed in connection with NK cells. One of the earliest treatments for MS was interferon-1b, a ligand that inhibits the activity of CD56 NK cells. The immunoregulatory effect of interferon-1b may be attributed in part to the reduction of cytotoxicity in CD56dim NK cells (Kaur et al., 2013). Moreover, interferon-1b appears to alter the ratio between CD56bright CD56dim NK cells in peripheral blood by increasing the number of CD56bright of lymphocytes (Poli et al., 2009).
Natalizumab prevents immune cells from migrating by blocking the very late antigen-4 (VLA-4). Natalizumab increases the number of circulating NK cells, possibly due to its decreased migration capacity to tissues, such as the central nervous system (Schlesinger and Bendas, 2015). A decrease in CD56bright NK cells seems to be more significant than the depletion of T lymphocytes in the CNS, which is consistent with the favorable outcomes of natalizumab (Krumbholz et al., 2008). So far, NK cells have been suggested to have both positive and negative impacts on MS. According to studies of the past 40 years, MS progression is linked to a low NK cell function. Nevertheless, other research revealed a connection between NK cell activity and central nervous system pathologies, implying that the impact of NK lymphocytes on MS may vary depending on their subtype or mechanism of action (Mimpen et al., 2020; Kucuksezer et al., 2021).
Glatiramer acetate (GA) is a product consisting of four amino acids found within myelin basic protein (MBP) (Glu, Ala, Lys, Tyr) (Schrempf and Ziemssen, 2007). Originally, the substance was intended to induce EAE as a synthetic antigen. Studies in vitro indicate that glatiramer acetate promotes NK cell cytolytic activity, targeting immature and mature dendritic cells (DCs) and activating Th-1 cells (Wens et al., 2021). GA does not appear to enhance or alter NK cells directly, but they may play an essential role in clearing GA-altered DC fields (Maghazachi et al., 2016).
The fingolimod drug works by antagonistically opposing the effects of sphingosine-1-phosphate (S1P), which inhibits the release from lymph nodes of self-reactive lymphocytes (Groves et al., 2013). As a result of lymphocyte accumulation in the lymph nodes, fingolimod causes lymphopenia; however, Natural killer cells appear to be more resistant than B- and T-lymphocytes (Schwichtenberg et al., 2021). Fingolimod appears to affect the NK cell population (Kowarik et al., 2011). CD56bright NK cells enrichment within the CSF may be responsible for at least some of the effects of fingolimod (Schwichtenberg et al., 2021).
Dimethyl Fumarate (DMF) is an efficient therapeutic agent for MS. However, its effect on the immune system remains unclear. There is evidence that the number of CD56bright NK cells, have increased among MS patients who received DMF. Lymphopenia is a major concern for MS patients in those who use DMF as a treatment because of an increased risk of progressive multifocal leukoencephalopathy.
Multiple sclerosis treatment can affect natural killer cell numbers (Laroni and Uccelli, 2020). In an integrated analysis of in vivo clinical data and in vitro mechanistic studies derived from two phase II clinical trials involving 22 individuals with MS, Bielekova and colleagues proposed a model in which daclizumab exerts its therapeutic effects through an immunological cross-regulatory pathway linking innate and adaptive immunity. Their findings posit that this mechanism is mediated by immunoregulatory CD56bright NK cells modulating T-cell activity, as evidenced by both trial-derived observations and controlled laboratory assays. Also, they found that daclizumab was able to expand CD56–positive NK cells, possibly contributing to its beneficial effects. Additionally, they improved their cytotoxic and regulatory abilities (Bielekova et al., 2006). Recent studies have shown that CD56bright NK cells expand after daclizumab due to IL-2 binding to their intermediate receptor. Daclizumab does not target this receptor (Sheridan et al., 2011). In addition, some MS sufferers who receive daclizumab develop autoimmune encephalitis. A reduction in regulatory T lymphocytes may contribute to the withdrawal of the drug (Bianchi and Ciccarelli, 2019).
It is important to isolate immune subsets, as demonstrated by daclizumab and fingolimod. Therefore, the treatment propagates its beneficial effects and prevents unwanted ones. In a wide range of autoimmune diseases, NK cells have been identified as potential biomarkers and therapeutic targets (Gianchecchi et al., 2021). Research is currently being conducted to determine whether the levels of natural killers and their functional capacity are prognostic and indicative of treatment outcomes in MS. Nevertheless, further validation is required to provide a mechanistic explanation for the patterns observed (Mimpen et al., 2021).
6 Perspective findings on the NK and MS
As the earlier studies demonstrate, MS models can experience both protection and pathogenic impact from NK cells. Different functions of natural killers or a difference in the subset of targeted natural killer cells may have caused these divergent findings. Multiple mechanisms exist through which natural killer cells are capable of modulating self-reactive T lymphocytes by inhibition of these cells (Pallmer and Oxenius, 2016). To promote autoreactive T lymphocyte production, natural killers secrete substances to regulate native CD4+ T lymphocyte differentiation into Th1 or Th2 cells, thereby influencing autoimmune reactions (Poggi and Zocchi, 2014). natural killer cells secrete IFN-γ, which is thought to contribute to Th1 polarization. It could manifest as a subset of NK cells that express antigen-presenting machinery (such as MHC class II) and directly activate self-reactive T lymphocytes (Paul and Lal, 2017). In spite of this, cells may be interacting with auto-reactive T lymphocytes in multiple mechanisms, with direct control demonstrated recently on activated T cells by natural killer cells. Conversely, natural killer cells’ degeneration,’ which is characterized by the loss of the number or function of these cells (a reduction in cytotoxicity), is often reported in MS patients’ peripheral blood (Gross et al., 2016). In these settings, reduced cytotoxicity of natural killer cells can impair the restraint of immunopathogenic cells. In contrast, a decrease in circulating natural killer cells may indicate increased recruitment to inflammatory sites (Yang et al., 2021).
NK cells have an essential and influential part in the progression of MS. Some evidence suggests that removing or deactivating NK cells is beneficial to MS disease progression. In contrast, there has been some suggestion that more NK cells are beneficial to the condition (Ahmadi et al., 2022). The discrepancy can result from the fact that subsets of natural killers still need to be fully characterized. Increasing CD56bright NK cells or reducing CD56dim NK cells in MS has been demonstrated to result in successful treatment outcomes (Laroni et al., 2016). Thus, CD56bright NK cells may modulate immune responses by increasing cytokine production associated with decreased adaptive immunity. CD56bright NK cells have the potential to suppress the effects of autoreactive T lymphocytes, one of the primary causes of inflammation in MS. In this way, autoimmunity may be limited. On the other hand, the medication that increases CD56dim NK cells can have adverse effects on the patient. However, further research is necessary to validate these findings and determine the mechanism of action (Ahmadi et al., 2022).
After observing favorable results in MS patients following treatment with daclizumab, a monoclonal antibody that blocks the alpha chain of IL-2 receptors (IL-2Ra; CD25), natural killer cells began to receive attention due to their IL-2 receptors (Giovannoni et al., 2014). Even so, the function of natural killer cells remains controversial, as demonstrated by studies demonstrating both their beneficial and detrimental effects in MS and experimental autoimmune encephalomyelitis (EAE) induced in rodents (Gandhi et al., 2010). Natural killer cells exhibit their functions by balancing their stimulatory and inhibitory receptors (Trachtenberg, 2009). Therefore, some studies have determined the natural killer cell receptor (NKR) expression profiles in multiple sclerosis and evaluated their contribution to the development of the disease. An initial study investigating how KIRs and their HLA ligands contribute to the progression of multiple sclerosis showed that patient HLA-Bw4 levels were significantly lower than those of healthy individuals, suggesting that HLA-Bw4 may protect against the development of MS (Lorentzen et al., 2009). The HLA-Bw4 ligand binds to inhibitory receptors on NK cells, thereby conferring NK cells’ functional proficiency in humans. According to a second study, the expression of KIR2DL2, an inhibitory receptor associated with infection by viruses, increased in people with multiple sclerosis afflicted with herpes simplex virus (HSV)-1 (Rizzo et al., 2012). Furthermore, NK cells expressing KIR2DL2 failed to control HSV-1 infection (Rizzo et al., 2016). Overall, the data suggest that KIRs and their ligands may play a significant role in MS. Yet, additional research is needed to understand their allelic variants’ functions in greater detail (Kucuksezer et al., 2021).
7 Conclusion
Available data indicate NK cells’ involvement in autoimmune diseases. An inflammatory environment promotes the migration of circulating NK cells into the central nervous system, influencing their function. NK cells release cytokines that attract and stimulate other immune cells, such as macrophages, neutrophils, and autoreactive T and B cells, contributing to inflammatory responses and tissue damage. Nevertheless, data suggests that specific subsets of NK cells can prevent autoimmune disorders by directly targeting autoreactive B and T cells. Furthermore, CD56dim and CD56bright NK cells can behave favorably or adversely under different conditions regarding similar autoimmune diseases. CD56dim NK cells developed demyelination by activating auto-antibody and perforin from plasma cells and effector T cells, and CD56bright NK cells inhibit demyelination by suppressing plasma cells and effector T cells.
Author contributions
FA: Visualization, Writing – original draft. MA: Visualization, Writing – original draft. SA: Conceptualization, Validation, Writing – original draft. AS: Conceptualization, Writing – review & editing. AF: Investigation, Visualization, Writing – review & editing. AB: Conceptualization, Software, Writing – review & editing.
Funding
The author(s) declare that no financial support was received for the research and/or publication of this article.
Acknowledgments
We would like to thank Reza Shakib, immunologist at Guilan University of Medical Science.
Conflict of interest
The author declares that the research was conducted in the absence of any commercial or financial relationships that could be construed as a potential conflict of interest.
Publisher’s note
All claims expressed in this article are solely those of the authors and do not necessarily represent those of their affiliated organizations, or those of the publisher, the editors and the reviewers. Any product that may be evaluated in this article, or claim that may be made by its manufacturer, is not guaranteed or endorsed by the publisher.
Abbreviations
NK, Natural Killer cell; RRMS, relapse remitting MS; PPMS, primary progressive MS; SPMS, secondary progressive MS; EAE, experimental autoimmune encephalomyelitis; PEN5, A sulfated polylactosamine carbohydrate epitope first described in a subpopulation of mature natural killer cells. TRAIL, TNF-related apoptosis-inducing ligand.
References
Acar, N., Tuncer, A., Ozkazanc, D., Ozbay, F., Karaosmanoglu, B., Goksen, S., et al. (2020). An immunological and transcriptomics approach on differential modulation of NK cells in multiple sclerosis patients under interferon-β1 and fingolimod therapy. J. Neuroimmunol. 347:577353. doi: 10.1016/j.jneuroim.2020.577353
Addison, E., North, J., Bakhsh, I., Marden, C., Haq, S., Al-Sarraj, S., et al. (2005). Ligation of CD8alpha on human natural killer cells prevents activation-induced apoptosis and enhances cytolytic activity. Immunology 116, 354–361. doi: 10.1111/j.1365-2567.2005.02235.x
Ahmadi, A., Fallah Vastani, Z., Abounoori, M., Azizi, M., Labani-Motlagh, A., Mami, S., et al. (2022). The role of NK and NKT cells in the pathogenesis and improvement of multiple sclerosis following disease-modifying therapies. Health Sci. Rep. 5:e489. doi: 10.1002/hsr2.489
Aranami, T., Miyake, S., and Yamamura, T. (2006). Differential expression of CD11c by peripheral blood NK cells reflects temporal activity of multiple sclerosis. J. Immunol. 177, 5659–5667. doi: 10.4049/jimmunol.177.8.5659
Ardolino, M., Zingoni, A., Cerboni, C., Cecere, F., Soriani, A., Iannitto, M., et al. (2011). DNAM-1 ligand expression on Ag-stimulated T lymphocytes is mediated by ROS-dependent activation of DNA-damage response: Relevance for NK-T cell interaction. Blood 117, 4778–4786. doi: 10.1182/blood-2010-08-300954
Ashare, R., and Wetherill, R. (2018). The intersection of sex differences, tobacco use, and inflammation: Implications for psychiatric disorders. Curr. Psychiatry Rep. 20:75. doi: 10.1007/s11920-018-0946-3
Assari, S. (2020). Social determinants of delayed gratification among American children. Casp J. Neurol. Sci. 6, 181–189. doi: 10.32598/cjns.6.22.2
Azzi, T., Lünemann, A., Murer, A., Ueda, S., Béziat, V., Malmberg, K., et al. (2014). Role for early-differentiated natural killer cells in infectious mononucleosis. Blood 124, 2533–2543. doi: 10.1182/blood-2014-01-553024
Bähr, I., Goritz, V., Doberstein, H., Hiller, G., Rosenstock, P., Jahn, J., et al. (2017). Diet-induced obesity is associated with an impaired NK cell function and an increased colon cancer incidence. J. Nutr. Metab. 2017:4297025. doi: 10.1155/2017/4297025
Bakhshi, A., Eslami, N., Norouzi, N., Letafatkar, N., Amini-Salehi, E., and Hassanipour, S. (2024). The association between various viral infections and multiple sclerosis: An umbrella review on systematic review and meta-analysis. Rev. Med. Virol. 34:e2494. doi: 10.1002/rmv.2494
Bakhshi, A., Saberi, A., Norouzi, N., and Sedighi, N. (2023). Association between multiple sclerosis and Helicobacter pylori in the acute and chronic phases of infection: A systematic review and meta-analysis. Roman. J. Neurol. 22, 11–17. doi: 10.37897/RJN.2023.1.2
Balogh, G., de Boland, A., Boland, R., and Barja, P. (1999). Effect of 1,25(OH)(2)-vitamin D(3) on the activation of natural killer cells: Role of protein kinase C and extracellular calcium. Exp. Mol. Pathol. 67, 63–74. doi: 10.1006/exmp.1999.2264
Beliën, J., Goris, A., and Matthys, P. (2022). Natural killer cells in multiple sclerosis: Entering the stage. Front. Immunol. 13:869447. doi: 10.3389/fimmu.2022.869447
Bianchi, A., and Ciccarelli, O. (2019). Daclizumab-induced encephalitis in multiple sclerosis. Mult. Scler. 25, 1557–1559. doi: 10.1177/1352458519845079
Bielekova, B., Catalfamo, M., Reichert-Scrivner, S., Packer, A., Cerna, M., Waldmann, T., et al. (2006). Regulatory CD56(bright) natural killer cells mediate immunomodulatory effects of IL-2Ralpha-targeted therapy (daclizumab) in multiple sclerosis. Proc. Natl. Acad. Sci. U. S. A. 103, 5941–5946. doi: 10.1073/pnas.0601335103
Boivin, W., Cooper, D., Hiebert, P., and Granville, D. (2009). Intracellular versus extracellular granzyme B in immunity and disease: Challenging the dogma. Lab. Invest. 89, 1195–1220. doi: 10.1038/labinvest.2009.91
Bortolotti, D., Gentili, V., Bortoluzzi, A., Govoni, M., Schiuma, G., Beltrami, S., et al. (2022). Herpesvirus infections in KIR2DL2-positive multiple sclerosis patients: Mechanisms triggering autoimmunity. Microorganisms 10:494. doi: 10.3390/microorganisms10030494
Bozzano, F., Marras, F., Ascierto, M., Cantoni, C., Cenderello, G., Dentone, C., et al. (2015). ‘Emergency exit’ of bone-marrow-resident CD34(+)DNAM-1(bright)CXCR4(+)-committed lymphoid precursors during chronic infection and inflammation. Nat. Commun. 6:8109. doi: 10.1038/ncomms9109
Calahorra, L., Camacho-Toledano, C., Serrano-Regal, M., Ortega, M., and Clemente, D. (2022). Regulatory cells in multiple sclerosis: From blood to brain. Biomedicines 10:335. doi: 10.3390/biomedicines10020335
Campbell, K., and Hasegawa, J. (2013). Natural killer cell biology: An update and future directions. J. Allergy Clin. Immunol. 132, 536–544. doi: 10.1016/j.jaci.2013.07.006
Carrega, P., Bonaccorsi, I., Di Carlo, E., Morandi, B., Paul, P., Rizzello, V., et al. (2014). CD56(bright)perforin(low) noncytotoxic human NK cells are abundant in both healthy and neoplastic solid tissues and recirculate to secondary lymphoid organs via afferent lymph. J. Immunol. 192, 3805–3815. doi: 10.4049/jimmunol.1301889
Caruana, P., Lemmert, K., Ribbons, K., Lea, R., and Lechner-Scott, J. (2017). Natural killer cell subpopulations are associated with MRI activity in a relapsing-remitting multiple sclerosis patient cohort from Australia. Mult. Scler. 23, 1479–1487. doi: 10.1177/1352458516679267
Cassidy, S., Mukherjee, S., Myint, T., Mbiribindi, B., North, H., Traherne, J., et al. (2015). Peptide selectivity discriminates NK cells from KIR2DL2- and KIR2DL3-positive individuals. Eur. J. Immunol. 45, 492–500. doi: 10.1002/eji.201444613
Castoldi, A., Naffah de Souza, C., Câmara, N., and Moraes-Vieira, P. (2016). The macrophage switch in obesity development. Front. Immunol. 6:637. doi: 10.3389/fimmu.2015.00637
Chen, Z., and Wang, L. (2019). Ovalbumin induces natural killer cells to secrete Th2 cytokines IL-5 and IL-13 in a mouse model of asthma. Mol. Med. Rep. 19, 3210–3216. doi: 10.3892/mmr.2019.9966
Chewning, J., Gudme, C., Hsu, K., Selvakumar, A., and Dupont, B. (2007). KIR2DS1-positive NK cells mediate alloresponse against the C2 HLA-KIR ligand group in vitro. J. Immunol. 179, 854–868. doi: 10.4049/jimmunol.179.2.854
Chijioke, O., Müller, A., Feederle, R., Barros, M., Krieg, C., Emmel, V., et al. (2013). Human natural killer cells prevent infectious mononucleosis features by targeting lytic Epstein-Barr virus infection. Cell Rep. 5, 1489–1498. doi: 10.1016/j.celrep.2013.11.041
Chu, J., Gao, F., Yan, M., Zhao, S., Yan, Z., Shi, B., et al. (2022). Natural killer cells: A promising immunotherapy for cancer. J. Transl. Med. 20:240. doi: 10.1186/s12967-022-03437-0
Cohen, J. (2000). Epstein-Barr virus infection. N. Engl. J. Med. 343, 481–492. doi: 10.1056/NEJM200008173430707
Constantinescu, C., Farooqi, N., O’Brien, K., and Gran, B. (2011). Experimental autoimmune encephalomyelitis (EAE) as a model for multiple sclerosis (MS). Br. J. Pharmacol. 164, 1079–1076. doi: 10.1111/j.1476-5381.2011.01302.x
Cooper, M., Fehniger, T., and Caligiuri, M. (2001). The biology of human natural killer-cell subsets. Trends Immunol. 22, 633–640. doi: 10.1016/s1471-4906(01)02060-9
Crux, N., and Elahi, S. (2017). Human leukocyte antigen (HLA) and immune regulation: How do classical and non-classical HLA alleles modulate immune response to human immunodeficiency virus and hepatitis C virus infections? Front. Immunol. 8:832. doi: 10.3389/fimmu.2017.00832
Darlington, P., Stopnicki, B., Touil, T., Doucet, J., Fawaz, L., Roberts, M., et al. (2018). Natural killer cells regulate Th17 cells after autologous hematopoietic stem cell transplantation for relapsing remitting multiple sclerosis. Front. Immunol. 9:834. doi: 10.3389/fimmu.2018.00834
Deeba, E., Lambrianides, A., Pantzaris, M., Krashias, G., and Christodoulou, C. (2020). The expression profile of virus-recognizing toll-like receptors in natural killer cells of Cypriot multiple sclerosis patients. BMC Res. Notes 13:460. doi: 10.1186/s13104-020-05300-1
Dema, M., Eixarch, H., Hervera, A., Castillo, M., Villar, L., Montalban, X., et al. (2025). Disease aggravation with age in an experimental model of multiple sclerosis: Role of immunosenescence. Aging Cell [Online ahead of print]. doi: 10.1111/acel.14491
Deng, L., and Mariuzza, R. (2006). Structural basis for recognition of MHC and MHC-like ligands by natural killer cell receptors. Semin. Immunol. 18, 159–166. doi: 10.1016/j.smim.2006.03.004
Dhaiban, S., Al-Ani, M., Elemam, N., Al-Aawad, M., Al-Rawi, Z., and Maghazachi, A. (2021). Role of peripheral immune cells in multiple sclerosis and experimental autoimmune encephalomyelitis. Science 3:2.
Doshi, A., and Chataway, J. (2017). Multiple sclerosis, a treatable disease. Clin. Med. 17, 530–536. doi: 10.7861/clinmedicine.17-6-530
Esmaeil Amini, M., Shomali, N., Bakhshi, A., Rezaei, S., Hemmatzadeh, M., Hosseinzadeh, R., et al. (2020). Gut microbiome and multiple sclerosis: New insights and perspective. Int. Immunopharmacol. 88:107024. doi: 10.1016/j.intimp.2020.107024
Faner, R., Gonzalez, N., Cruz, T., Kalko, S., and Agustí, A. (2014). Systemic inflammatory response to smoking in chronic obstructive pulmonary disease: Evidence of a gender effect. PLoS One 9:e97491. doi: 10.1371/journal.pone.0097491
Fauriat, C., Just-Landi, S., Mallet, F., Arnoulet, C., Sainty, D., Olive, D., et al. (2007). Deficient expression of NCR in NK cells from acute myeloid leukemia: Evolution during leukemia treatment and impact of leukemia cells in NCRdull phenotype induction. Blood 109, 323–330. doi: 10.1182/blood-2005-08-027979
Fehniger, T., Cooper, M., Nuovo, G., Cella, M., Facchetti, F., Colonna, M., et al. (2003). CD56bright natural killer cells are present in human lymph nodes and are activated by T cell-derived IL-2: A potential new link between adaptive and innate immunity. Blood 101, 3052–3057. doi: 10.1182/blood-2002-09-2876
Fogel, L., Yokoyama, W., and French, A. (2013). Natural killer cells in human autoimmune disorders. Arthritis Res. Ther. 15:216. doi: 10.1186/ar4232
Frank, K., and Paust, S. (2020). Dynamic natural killer cell and T cell responses to influenza infection. Front. Cell Infect. Microbiol. 10:425. doi: 10.3389/fcimb.2020.00425
Galazka, G., Jurewicz, A., Domowicz, M., Cannella, B., Raine, C., and Selmaj, K. (2014). HINT1 peptide/Hsp70 complex induces NK-cell-dependent immunoregulation in a model of autoimmune demyelination. Eur. J. Immunol. 44, 3026–3044. doi: 10.1002/eji.201444694
Galazka, G., Jurewicz, A., Orlowski, W., Stasiolek, M., Brosnan, C., Raine, C., et al. (2007). EAE tolerance induction with Hsp70-peptide complexes depends on H60 and NKG2D activity. J. Immunol. 179, 4503–4512. doi: 10.4049/jimmunol.179.7.4503
Galazka, G., Stasiolek, M., Walczak, A., Jurewicz, A., Zylicz, A., Brosnan, C., et al. (2006). Brain-derived heat shock protein 70-peptide complexes induce NK cell-dependent tolerance to experimental autoimmune encephalomyelitis. J. Immunol. 176, 1588–1599. doi: 10.4049/jimmunol.176.3.1588
Galicia, G., and Gommerman, J. (2014). Plasmacytoid dendritic cells and autoimmune inflammation. Biol. Chem. 395, 335–346. doi: 10.1515/hsz-2013-0213
Gandhi, R., Laroni, A., and Weiner, H. (2010). Role of the innate immune system in the pathogenesis of multiple sclerosis. J. Neuroimmunol. 221, 7–14. doi: 10.1016/j.jneuroim.2009.10.015
Gauthier, L., Morel, A., Anceriz, N., Rossi, B., Blanchard-Alvarez, A., Grondin, G., et al. (2019). Multifunctional natural killer cell engagers targeting NKp46 trigger protective tumor immunity. Cell 177, 1701–1713.e16. doi: 10.1016/j.cell.2019.04.041
Gayoso, I., Sanchez-Correa, B., Campos, C., Alonso, C., Pera, A., Casado, J., et al. (2011). Immunosenescence of human natural killer cells. J. Innate Immun. 3, 337–343. doi: 10.1159/000328005
Gerriets, V., and MacIver, N. (2014). Role of T cells in malnutrition and obesity. Front. Immunol. 5:379. doi: 10.3389/fimmu.2014.00379
Gianchecchi, E., Delfino, D., and Fierabracci, A. (2021). Natural killer cells: Potential biomarkers and therapeutic target in autoimmune diseases? Front. Immunol. 12:616853. doi: 10.3389/fimmu.2021.616853
Giovannoni, G., Gold, R., Selmaj, K., Havrdova, E., Montalban, X., Radue, E., et al. (2014). Daclizumab high-yield process in relapsing-remitting multiple sclerosis (SELECTION): A multicentre, randomised, double-blind extension trial. Lancet Neurol. 13, 472–481. doi: 10.1016/S1474-4422(14)70039-0
Gross, C., Schulte-Mecklenbeck, A., Rünzi, A., Kuhlmann, T., Posevitz-Fejfár, A., Schwab, N., et al. (2016). Impaired NK-mediated regulation of T-cell activity in multiple sclerosis is reconstituted by IL-2 receptor modulation. Proc. Natl. Acad. Sci. U. S. A. 113, E2973–E2982. doi: 10.1073/pnas.1524924113
Groves, A., Kihara, Y., and Chun, J. (2013). Fingolimod: Direct CNS effects of sphingosine 1-phosphate (S1P) receptor modulation and implications in multiple sclerosis therapy. J. Neurol. Sci. 328, 9–18. doi: 10.1016/j.jns.2013.02.011
Hammer, Q., Rückert, T., and Romagnani, C. (2018). Natural killer cell specificity for viral infections. Nat Immunol. 19, 800–808. doi: 10.1038/s41590-018-0163-6
Hao, J., Campagnolo, D., Liu, R., Piao, W., Shi, S., Hu, B., et al. (2011). Interleukin-2/interleukin-2 antibody therapy induces target organ natural killer cells that inhibit central nervous system inflammation. Ann. Neurol. 69, 721–734. doi: 10.1002/ana.22339
Hay, Z., and Slansky, J. (2022). Granzymes: The molecular executors of immune-mediated cytotoxicity. Int. J. Mol. Sci. 23:1833. doi: 10.3390/ijms23031833
Hazeldine, J., Hampson, P., and Lord, J. (2012). Reduced release and binding of perforin at the immunological synapse underlies the age-related decline in natural killer cell cytotoxicity. Aging Cell 11, 751–759. doi: 10.1111/j.1474-9726.2012.00839.x
Hedayati-Ch, M., Ebrahim-Saraie, H., and Bakhshi, A. (2024). Clinical and immunological comparison of COVID-19 disease between critical and non-critical courses: A systematic review and meta-analysis. Front. Immunol. 15:1341168. doi: 10.3389/fimmu.2024.1341168
Hemmer, B., Cepok, S., Nessler, S., and Sommer, N. (2002). Pathogenesis of multiple sclerosis: An update on immunology. Curr. Opin. Neurol. 15, 227–231. doi: 10.1097/00019052-200206000-00001
Høglund, R., and Maghazachi, A. (2014). Multiple sclerosis and the role of immune cells. World J. Exp. Med. 4, 27–37. doi: 10.5493/wjem.v4.i3.27
Huth, T., Brenu, E., Ramos, S., Nguyen, T., Broadley, S., Staines, D., et al. (2016). Pilot study of natural killer cells in chronic fatigue syndrome/myalgic encephalomyelitis and multiple sclerosis. Scand. J. Immunol. 83, 44–51. doi: 10.1111/sji.12388
Jacobs, R., Hintzen, G., Kemper, A., Beul, K., Kempf, S., Behrens, G., et al. (2001). CD56bright cells differ in their KIR repertoire and cytotoxic features from CD56dim NK cells. Eur. J. Immunol. 31, 3121–3127. doi: 10.1002/1521-4141(2001010)31:10
Jiang, W., Chai, N., Maric, D., and Bielekova, B. (2011). Unexpected role for granzyme K in CD56bright NK cell-mediated immunoregulation of multiple sclerosis. J. Immunol. 187, 781–790. doi: 10.4049/jimmunol.1100789
Jiang, W., Li, D., Han, R., Zhang, C., Jin, W., Wood, K., et al. (2017). Acetylcholine-producing NK cells attenuate CNS inflammation via modulation of infiltrating monocytes/macrophages. Proc. Natl. Acad. Sci. U. S. A. 114, E6202–E6211. doi: 10.1073/pnas.1705491114
Jiao, Y., Ma, C., Wang, L., Cui, B., Zhang, J., You, L., et al. (2008). Polymorphisms of KIRs gene and HLA-C alleles in patients with ankylosing spondylitis: Possible association with susceptibility to the disease. J. Clin. Immunol. 28, 343–349. doi: 10.1007/s10875-008-9183-6
Kaur, G., Trowsdale, J., and Fugger, L. (2013). Natural killer cells and their receptors in multiple sclerosis. Brain 136(Pt 9), 2657–2676. doi: 10.1093/brain/aws159
Kemppinen, A., Kaprio, J., Palotie, A., and Saarela, J. (2011). Systematic review of genome-wide expression studies in multiple sclerosis. BMJ Open 1:e000053. doi: 10.1136/bmjopen-2011-000053
Kim, H., and Cantor, H. (2011). Regulation of self-tolerance by Qa-1-restricted CD8(+) regulatory T cells. Semin. Immunol. 23, 446–452. doi: 10.1016/j.smim.2011.06.001
Komuczki, J., Tuzlak, S., Friebel, E., Hartwig, T., Spath, S., Rosenstiel, P., et al. (2019). Fate-mapping of GM-CSF expression identifies a discrete subset of inflammation-driving T helper cells regulated by cytokines IL-23 and IL-1β. Immunity 50, 1289–1304.e6. doi: 10.1016/j.immuni.2019.04.006
Kowarik, M., Pellkofer, H., Cepok, S., Korn, T., Kümpfel, T., Buck, D., et al. (2011). Differential effects of fingolimod (FTY720) on immune cells in the CSF and blood of patients with MS. Neurology 76, 1214–1221. doi: 10.1212/WNL.0b013e3182143564
Krumbholz, M., Meinl, I., Kümpfel, T., Hohlfeld, R., and Meinl, E. (2008). Natalizumab disproportionately increases circulating pre-B and B cells in multiple sclerosis. Neurology 71, 1350–1354. doi: 10.1212/01.wnl.0000327671.91357.96
Kucuksezer, U., Aktas Cetin, E., Esen, F., Tahrali, I., Akdeniz, N., Gelmez, M., et al. (2021). The role of natural killer cells in autoimmune diseases. Front. Immunol. 12:622306. doi: 10.3389/fimmu.2021.622306
Kumar, S. (2018). Natural killer cell cytotoxicity and its regulation by inhibitory receptors. Immunology 154, 383–393. doi: 10.1111/imm.12921
Kutzelnigg, A., Lucchinetti, C., Stadelmann, C., Brück, W., Rauschka, H., Bergmann, M., et al. (2005). Cortical demyelination and diffuse white matter injury in multiple sclerosis. Brain 128(Pt 11), 2705–2712. doi: 10.1093/brain/awh641
Lagumersindez-Denis, N., Wrzos, C., Mack, M., Winkler, A., van der Meer, F., Reinert, M., et al. (2017). Differential contribution of immune effector mechanisms to cortical demyelination in multiple sclerosis. Acta Neuropathol. 134, 15–34. doi: 10.1007/s00401-017-1706-x
Laroni, A., and Uccelli, A. (2020). CD56bright natural killer cells: A possible biomarker of different treatments in multiple sclerosis. J. Clin. Med. 9:1450. doi: 10.3390/jcm9051450
Laroni, A., Armentani, E., Kerlero de Rosbo, N., Ivaldi, F., Marcenaro, E., Sivori, S., et al. (2016). Dysregulation of regulatory CD56(bright) NK cells/T cells interactions in multiple sclerosis. J. Autoimmun. 72, 8–18. doi: 10.1016/j.jaut.2016.04.003
Le Maux Chansac, B., Moretta, A., Vergnon, I., Opolon, P., Lécluse, Y., Grunenwald, D., et al. (2005). NK cells infiltrating a MHC class I-deficient lung adenocarcinoma display impaired cytotoxic activity toward autologous tumor cells associated with altered NK cell-triggering receptors. J. Immunol. 175, 5790–5798. doi: 10.4049/jimmunol.175.9.5790
Li, H., Hou, X., Liang, Y., Xu, F., Zhang, X., Cui, P., et al. (2021). Gene-based tests of a genome-wide association study dataset highlight novel multiple sclerosis risk genes. Front. Neurosci. 15:614528. doi: 10.3389/fnins.2021.614528
Liu, G., Hu, Y., Jin, S., and Jiang, Q. (2017). Genetic variant rs763361 regulates multiple sclerosis CD226 gene expression. Proc. Natl. Acad. Sci. U. S. A. 114, E906–E907. doi: 10.1073/pnas.1618520114
Liu, J., Wu, Q., Shi, J., Guo, W., Jiang, X., Zhou, B., et al. (2020). LILRB4, from the immune system to the disease target. Am. J. Transl. Res. 12, 3149–3166.
Liu, M., Liang, S., and Zhang, C. N. K. (2021). Cells in autoimmune diseases: Protective or pathogenic? Front. Immunol. 12:624687. doi: 10.3389/fimmu.2021.624687
Long, E., and Rajagopalan, S. (2002). Stress signals activate natural killer cells. J. Exp. Med. 196, 1399–1402. doi: 10.1084/jem.20021747
Long, E., Kim, H., Liu, D., Peterson, M., and Rajagopalan, S. (2013). Controlling natural killer cell responses: Integration of signals for activation and inhibition. Annu. Rev. Immunol. 31, 227–258. doi: 10.1146/annurev-immunol-020711-075005
Loosen, S., Doege, C., Meuth, S., Luedde, T., Kostev, K., and Roderburg, C. (2022). Infectious mononucleosis is associated with an increased incidence of multiple sclerosis: Results from a cohort study of 32,116 outpatients in Germany. Front. Immunol. 13:937583. doi: 10.3389/fimmu.2022.937583
Lorentzen, A., Karlsen, T., Olsson, M., Smestad, C., Mero, I., Woldseth, B., et al. (2009). Killer immunoglobulin-like receptor ligand HLA-Bw4 protects against multiple sclerosis. Ann. Neurol. 65, 658–666. doi: 10.1002/ana.21695
Lowdell, M., Craston, R., Samuel, D., Wood, M., O’Neill, E., Saha, V., et al. (2002). Evidence that continued remission in patients treated for acute leukaemia is dependent upon autologous natural killer cells. Br. J. Haematol. 117, 821–827. doi: 10.1046/j.1365-2141.2002.03495.x
Lünemann, A., Tackenberg, B., DeAngelis, T., da Silva, R., Messmer, B., Vanoaica, L., et al. (2011). Impaired IFN-γ production and proliferation of NK cells in multiple sclerosis. Int. Immunol. 23, 139–148. doi: 10.1093/intimm/dxq463
Lutz, C., Karapetyan, A., Al-Attar, A., Shelton, B., Holt, K., Tucker, J., et al. (2011). Human NK cells proliferate and die in vivo more rapidly than T cells in healthy young and elderly adults. J. Immunol. 186, 4590–4598. doi: 10.4049/jimmunol.1002732
Maghazachi, A., Sand, K., and Al-Jaderi, Z. (2016). Glatiramer acetate, dimethyl fumarate, and monomethyl fumarate upregulate the expression of CCR10 on the surface of natural killer cells and enhance their chemotaxis and cytotoxicity. Front. Immunol. 7:437. doi: 10.3389/fimmu.2016.00437
Martínez-Rodríguez, J., Cobo-Calvo, A., Villar, L., Munteis, E., Blanco, Y., Rasal, R., et al. (2016). Adaptive natural killer cell response to cytomegalovirus and disability progression in multiple sclerosis. Mult. Scler. 22, 741–752. doi: 10.1177/1352458515601215
Martínez-Rodríguez, J., López-Botet, M., Munteis, E., Rio, J., Roquer, J., Montalban, X., et al. (2011). Natural killer cell phenotype and clinical response to interferon-beta therapy in multiple sclerosis. Clin. Immunol. 141, 348–356. doi: 10.1016/j.clim.2011.09.006
Martínez-Rodríguez, J., Saez-Borderías, A., Munteis, E., Romo, N., Roquer, J., and López-Botet, M. (2010). Natural killer receptors distribution in multiple sclerosis: Relation to clinical course and interferon-beta therapy. Clin. Immunol. 137, 41–50. doi: 10.1016/j.clim.2010.06.002
Mastorodemos, V., Ioannou, M., and Verginis, P. (2015). Cell-based modulation of autoimmune responses in multiple sclerosis and experimental autoimmmune encephalomyelitis: Therapeutic implications. Neuroimmunomodulation 22, 181–195. doi: 10.1159/000362370
Mellergård, J., Edström, M., Jenmalm, M., Dahle, C., Vrethem, M., and Ernerudh, J. (2013). Increased B cell and cytotoxic NK cell proportions and increased T cell responsiveness in blood of natalizumab-treated multiple sclerosis patients. PLoS One 8:e81685. doi: 10.1371/journal.pone.0081685
Melsen, J., Lugthart, G., Lankester, A., and Schilham, M. (2016). Human circulating and tissue-resident CD56(bright) natural killer cell populations. Front. Immunol. 7:262. doi: 10.3389/fimmu.2016.00262
Mi, Y., Han, J., Zhu, J., and Jin, T. (2021). Role of the PD-1/PD-L1 signaling in multiple sclerosis and experimental autoimmune encephalomyelitis: Recent insights and future directions. Mol. Neurobiol. 58, 6249–6271. doi: 10.1007/s12035-021-02495-7
Mimpen, M., Muris, A., Rolf, L., Gerlach, O., Kuhle, J., Hupperts, R., et al. (2021). Prognostic value of natural killer cell/T cell ratios for disease activity in multiple sclerosis. Eur. J. Neurol. 28, 901–909. doi: 10.1111/ene.14680
Mimpen, M., Smolders, J., Hupperts, R., and Damoiseaux, J. (2020). Natural killer cells in multiple sclerosis: A review. Immunol. Lett. 222, 1–11. doi: 10.1016/j.imlet.2020.02.012
Montaldo, E., Del Zotto, G., Della Chiesa, M., Mingari, M., Moretta, A., De Maria, A., et al. (2013). Human NK cell receptors/markers: A tool to analyze NK cell development, subsets and function. Cytometry A 83, 702–713. doi: 10.1002/cyto.a.22302
Morandi, F., Venturi, C., Rizzo, R., Castellazzi, M., Baldi, E., Caniatti, M., et al. (2013). Intrathecal soluble HLA-E correlates with disease activity in patients with multiple sclerosis and may cooperate with soluble HLA-G in the resolution of neuroinflammation. J. Neuroimmune Pharmacol. 8, 944–955. doi: 10.1007/s11481-013-9459-3
Moreira, A., Alari-Pahissa, E., Munteis, E., Vera, A., Zabalza, A., Llop, M., et al. (2019). Adaptive features of natural killer cells in multiple sclerosis. Front. Immunol. 10:2403. doi: 10.3389/fimmu.2019.02403
Moutsianas, L., Jostins, L., Beecham, A., Dilthey, A., Xifara, D., Ban, M., et al. (2015). Class II HLA interactions modulate genetic risk for multiple sclerosis. Nat. Genet. 47, 1107–1113. doi: 10.1038/ng.3395
Nielsen, N., Ødum, N., Ursø, B., Lanier, L., and Spee, P. (2012). Cytotoxicity of CD56(bright) NK cells towards autologous activated CD4+ T cells is mediated through NKG2D, LFA-1 and TRAIL and dampened via CD94/NKG2A. PLoS One 7:e31959. doi: 10.1371/journal.pone.0031959
Oh, J., Vidal-Jordana, A., and Montalban, X. (2018). Multiple sclerosis: Clinical aspects. Curr. Opin. Neurol. 31, 752–759. doi: 10.1097/WCO.0000000000000622
Pallmer, K., and Oxenius, A. (2016). Recognition and regulation of T cells by NK cells. Front. Immunol. 7:251. doi: 10.3389/fimmu.2016.00251
Paul, S., and Lal, G. (2017). The molecular mechanism of natural killer cells function and its importance in cancer immunotherapy. Front. Immunol. 8:1124. doi: 10.3389/fimmu.2017.01124
Pegram, H., Andrews, D., Smyth, M., Darcy, P., and Kershaw, M. (2011). Activating and inhibitory receptors of natural killer cells. Immunol. Cell Biol. 89, 216–224. doi: 10.1038/icb.2010.78
Pende, D., Falco, M., Vitale, M., Cantoni, C., Vitale, C., Munari, E., et al. (2019). Killer Ig-like receptors (KIRs): Their role in NK cell modulation and developments leading to their clinical exploitation. Front. Immunol. 10:1179. doi: 10.3389/fimmu.2019.01179
Peng, H., Lucavs, J., Ballard, D., Das, J., Kumar, A., Wang, L., et al. (2021). Metabolic reprogramming and reactive oxygen species in T cell immunity. Front. Immunol. 12:652687. doi: 10.3389/fimmu.2021.652687
Perri, V., Zingaropoli, M., Pasculli, P., Ciccone, F., Tartaglia, M., Baione, V., et al. (2024). The impact of cytomegalovirus infection on natural killer and CD8+ T cell phenotype in multiple sclerosis. Biology 13:154. doi: 10.3390/biology13030154
Picarda, G., and Benedict, C. (2018). Cytomegalovirus: Shape-shifting the immune system. J. Immunol. 200, 3881–3889. doi: 10.4049/jimmunol.1800171
Plantone, D., Marti, A., Frisullo, G., Iorio, R., Damato, V., Nociti, V., et al. (2013). Circulating CD56dim NK cells expressing perforin are increased in progressive multiple sclerosis. J. Neuroimmunol. 265, 124–127. doi: 10.1016/j.jneuroim.2013.10.004
Poggi, A., and Zocchi, M. (2014). NK cell autoreactivity and autoimmune diseases. Front. Immunol. 5:27. doi: 10.3389/fimmu.2014.00027
Poli, A., Michel, T., Thérésine, M., Andrès, E., Hentges, F., and Zimmer, J. (2009). CD56bright natural killer (NK) cells: An important NK cell subset. Immunology 126, 458–465. doi: 10.1111/j.1365-2567.2008.03027.x
Poznanski, S., and Ashkar, A. (2019). What defines NK cell functional fate: Phenotype or metabolism? Front. Immunol. 10:1414. doi: 10.3389/fimmu.2019.01414
Rizzo, R., Bortolotti, D., Fainardi, E., Gentili, V., Bolzani, S., Baldi, E., et al. (2016). KIR2DL2 inhibitory pathway enhances Th17 cytokine secretion by NK cells in response to herpesvirus infection in multiple sclerosis patients. J. Neuroimmunol. 294, 1–5. doi: 10.1016/j.jneuroim.2016.03.007
Rizzo, R., Gentili, V., Casetta, I., Caselli, E., De Gennaro, R., Granieri, E., et al. (2012). Altered natural killer cells’ response to herpes virus infection in multiple sclerosis involves KIR2DL2 expression. J. Neuroimmunol. 251, 55–64. doi: 10.1016/j.jneuroim.2012.07.004
Rodríguez-Martín, E., Picón, C., Costa-Frossard, L., Alenda, R., Sainz de la Maza, S., Roldán, E., et al. (2015). Natural killer cell subsets in cerebrospinal fluid of patients with multiple sclerosis. Clin. Exp. Immunol. 180, 243–249. doi: 10.1111/cei.12580
Rodriguez-Mogeda, C., van Ansenwoude, C., van der Molen, L., Strijbis, E., Mebius, R., and de Vries, H. (2024). The role of CD56bright NK cells in neurodegenerative disorders. J. Neuroinflamm. 21:48. doi: 10.1186/s12974-024-03040-8
Roma, S. (2021). Role and Regulation of Human Innate Lymphoid Cells and Natural Killer Cells in Lymphoma. Milano, MI: European Institute of Oncology.
Romaniuc, A., Bălaşa, R., Ştirbu, N., Maier, S., Andone, S., Bajko, Z., et al. (2020). The main determinants for suicidal ideation in a Romanian cohort of multiple sclerosis patients. Behav. Neurol. 2020:2594702. doi: 10.1155/2020/2594702
Ruder, J., Rex, J., Obahor, S., Docampo, M., Müller, A., Schanz, U., et al. (2021). NK cells and innate-like T cells after autologous hematopoietic stem cell transplantation in multiple sclerosis. Front. Immunol. 12:794077. doi: 10.3389/fimmu.2021.794077
Sadeghi Hassanabadi, N., Broux, B., Marinović, S., and Gotthardt, D. (2022). Innate lymphoid cells - neglected players in multiple sclerosis. Front. Immunol. 13:909275. doi: 10.3389/fimmu.2022.909275
Sasson, S. (2009). Dysregulation of the IL-7/IL-7R System: Implications for T-cell Homeostasis in HIV-1 Infection and pre-B Cell Oncogenesis: UNSW Sydney. Sydney: Centre for Immunology Research Seminar.
Schlesinger, M., and Bendas, G. (2015). Contribution of very late antigen-4 (VLA-4) integrin to cancer progression and metastasis. Cancer Metastasis Rev. 34, 575–591. doi: 10.1007/s10555-014-9545-x
Schrempf, W., and Ziemssen, T. (2007). Glatiramer acetate: Mechanisms of action in multiple sclerosis. Autoimmun. Rev. 6, 469–475. doi: 10.1016/j.autrev.2007.02.003
Schuster, I., Wikstrom, M., Brizard, G., Coudert, J., Estcourt, M., Manzur, M., et al. (2014). TRAIL+ NK cells control CD4+ T cell responses during chronic viral infection to limit autoimmunity. Immunity 41, 646–656. doi: 10.1016/j.immuni.2014.09.013
Schwane, V., Huynh-Tran, V., Vollmers, S., Yakup, V., Sauter, J., Schmidt, A., et al. (2020). Distinct signatures in the receptor repertoire discriminate CD56bright and CD56dim natural killer cells. Front. Immunol. 11:568927. doi: 10.3389/fimmu.2020.568927
Schwichtenberg, S., Wisgalla, A., Schroeder-Castagno, M., Alvarez-González, C., Schlickeiser, S., Siebert, N., et al. (2021). Fingolimod therapy in multiple sclerosis leads to the enrichment of a subpopulation of aged NK cells. Neurotherapeutics 18, 1783–1797. doi: 10.1007/s13311-021-01078-7
Scoville, S., Freud, A., and Caligiuri, M. (2017). Modeling human natural killer cell development in the era of innate lymphoid cells. Front. Immunol. 8:360. doi: 10.3389/fimmu.2017.00360
Segal, B. (2007). The role of natural killer cells in curbing neuroinflammation. J. Neuroimmunol. 191, 2–7. doi: 10.1016/j.jneuroim.2007.09.006
Sheridan, J., Zhang, Y., Riester, K., Tang, M., Efros, L., Shi, J., et al. (2011). Intermediate-affinity interleukin-2 receptor expression predicts CD56(bright) natural killer cell expansion after daclizumab treatment in the CHOICE study of patients with multiple sclerosis. Mult. Scler. 17, 1441–1448. doi: 10.1177/1352458511414755
Smolders, J., and Damoiseaux, J. (2011). Vitamin D as a T-cell modulator in multiple sclerosis. Vitam. Horm. 86, 401–428. doi: 10.1016/B978-0-12-386960-9.00018-6
Smolders, J., Moen, S., Damoiseaux, J., Huitinga, I., and Holmøy, T. (2011). Vitamin D in the healthy and inflamed central nervous system: Access and function. J. Neurol. Sci. 311, 37–43. doi: 10.1016/j.jns.2011.07.033
Stabile, H., Nisti, P., Morrone, S., Pagliara, D., Bertaina, A., Locatelli, F., et al. (2015). Multifunctional human CD56 low CD16 low natural killer cells are the prominent subset in bone marrow of both healthy pediatric donors and leukemic patients. Haematologica 100, 489–498. doi: 10.3324/haematol.2014.116053
Storm, L., Bruijnesteijn, J., de Groot, N., and Bontrop, R. (2021). The genomic organization of the LILR region remained largely conserved throughout primate evolution: Implications for health and disease. Front. Immunol. 12:716289. doi: 10.3389/fimmu.2021.716289
Sundqvist, E., Bergström, T., Daialhosein, H., Nyström, M., Sundström, P., Hillert, J., et al. (2014). Cytomegalovirus seropositivity is negatively associated with multiple sclerosis. Mult. Scler. 20, 165–173. doi: 10.1177/1352458513494489
Tahrali, I., Kucuksezer, U., Altintas, A., Uygunoglu, U., Akdeniz, N., Aktas-Cetin, E., et al. (2018). Dysfunction of CD3-CD16+CD56dim and CD3-CD16-CD56bright NK cell subsets in RR-MS patients. Clin. Immunol. 193, 88–97. doi: 10.1016/j.clim.2018.02.005
Takahashi, K., Aranami, T., Endoh, M., Miyake, S., and Yamamura, T. (2004). The regulatory role of natural killer cells in multiple sclerosis. Brain 127(Pt 9), 1917–1927. doi: 10.1093/brain/awh219
Takeyama, R., Fukui, A., Mai, C., Yamamoto, M., Saeki, S., Yamaya, A., et al. (2021). Co-expression of NKp46 with activating or inhibitory receptors on, and cytokine production by, uterine endometrial NK cells in recurrent pregnancy loss. J. Reprod. Immunol. 145:103324. doi: 10.1016/j.jri.2021.103324
Thompson, A., Baranzini, S., Geurts, J., Hemmer, B., and Ciccarelli, O. (2018). Multiple sclerosis. Lancet 391, 1622–1636. doi: 10.1016/S0140-6736(18)30481-1
Trachtenberg, E. (2009). Understanding the role of natural killer cell receptors and their human leukocyte antigen ligands in multiple sclerosis. Ann. Neurol. 65, 626–628. doi: 10.1002/ana.21747
Varghese, M., Griffin, C., McKernan, K., Eter, L., Lanzetta, N., Agarwal, D., et al. (2019). Sex differences in inflammatory responses to adipose tissue lipolysis in diet-induced obesity. Endocrinology 160, 293–312. doi: 10.1210/en.2018-00797
Waggoner, S., Cornberg, M., Selin, L., and Welsh, R. (2011). Natural killer cells act as rheostats modulating antiviral T cells. Nature 481, 394–398. doi: 10.1038/nature10624
Wagner, C., Roqué, P., and Goverman, J. (2020). Pathogenic T cell cytokines in multiple sclerosis. J. Exp. Med. 217:e20190460. doi: 10.1084/jem.20190460
Weber, S., Menees, K., Park, J., Agin-Liebes, J., Lin, C., Alcalay, R., et al. (2024). Distinctive CD56dim NK subset profiles and increased NKG2D expression in blood NK cells of Parkinson’s disease patients. NPJ Park. Dis. 10:36. doi: 10.1038/s41531-024-00652-y
Wens, I., Janssens, I., Derdelinckx, J., Meena, M., Willekens, B., and Cools, N. (2021). Made to measure: Patient-tailored treatment of multiple sclerosis using cell-based therapies. Int. J. Mol. Sci. 22:7536. doi: 10.3390/ijms22147536
Williams, H., McAulay, K., Macsween, K., Gallacher, N., Higgins, C., Harrison, N., et al. (2005). The immune response to primary EBV infection: A role for natural killer cells. Br. J. Haematol. 129, 266–274. doi: 10.1111/j.1365-2141.2005.05452.x
Yamamura, T., Sakuishi, K., Illés, Z., and Miyake, S. (2007). Understanding the behavior of invariant NKT cells in autoimmune diseases. J. Neuroimmunol. 191, 8–15. doi: 10.1016/j.jneuroim.2007.09.014
Yan, J., Greer, J., Hull, R., O’Sullivan, J., Henderson, R., Read, S., et al. (2010). The effect of ageing on human lymphocyte subsets: Comparison of males and females. Immun. Ageing 7:4. doi: 10.1186/1742-4933-7-4
Yang, Y., Day, J., Souza-Fonseca Guimaraes, F., Wicks, I., and Louis, C. (2021). Natural killer cells in inflammatory autoimmune diseases. Clin. Transl. Immunol. 10:e1250. doi: 10.1002/cti2.1250
Keywords: multiple sclerosis, natural killer, inflammation, immunity, protection
Citation: Aghaee F, Abedinpour M, Anvari S, Saberi A, Fallah A and Bakhshi A (2025) Natural killer cells in multiple sclerosis: foe or friends? Front. Cell. Neurosci. 19:1500770. doi: 10.3389/fncel.2025.1500770
Received: 23 September 2024; Accepted: 20 March 2025;
Published: 04 April 2025.
Edited by:
Junhui Wang, University of Toronto, CanadaReviewed by:
Ana Mafalda Fonseca, University of Beira Interior, PortugalXueping Chen, West China Hospital, Sichuan University, China
Everardo Hegewisch-Solloa, ImmuneBridge, Inc, United States
Copyright © 2025 Aghaee, Abedinpour, Anvari, Saberi, Fallah and Bakhshi. This is an open-access article distributed under the terms of the Creative Commons Attribution License (CC BY). The use, distribution or reproduction in other forums is permitted, provided the original author(s) and the copyright owner(s) are credited and that the original publication in this journal is cited, in accordance with accepted academic practice. No use, distribution or reproduction is permitted which does not comply with these terms.
*Correspondence: Arash Bakhshi, QS5iYWtoc2hpLmIxM0BnbWFpbC5jb20=
†ORCID: Arash Bakhshi, orcid.org/0000-0002-0642-4651