- 1Dr. Kiran C. Patel College of Osteopathic Medicine, Institute for Neuro-Immune Medicine, Nova Southeastern University, Ft. Lauderdale, FL, United States
- 2College of Psychology, Nova Southeastern University, Ft. Lauderdale, FL, United States
- 3Miami VA Geriatric Research Education and Clinical Center (GRECC), Miami Veterans Affairs Healthcare System, Miami, FL, United States
- 4Department of Immunology, Tufts, University School of Medicine, Boston, MA, United States
Neurovascular unit (NVU) inflammation via activation of glial cells and neuronal damage plays a critical role in neurodegenerative diseases. Though the exact mechanism of disease pathogenesis is not understood, certain biomarkers provide valuable insight into the disease pathogenesis, severity, progression and therapeutic efficacy. These markers can be used to assess pathophysiological status of brain cells including neurons, astrocytes, microglia, oligodendrocytes, specialized microvascular endothelial cells, pericytes, NVU, and blood-brain barrier (BBB) disruption. Damage or derangements in tight junction (TJ), adherens junction (AdJ), and gap junction (GJ) components of the BBB lead to increased permeability and neuroinflammation in various brain disorders including neurodegenerative disorders. Thus, neuroinflammatory markers can be evaluated in blood, cerebrospinal fluid (CSF), or brain tissues to determine neurological disease severity, progression, and therapeutic responsiveness. Chronic inflammation is common in age-related neurodegenerative disorders including Alzheimer’s disease (AD), Parkinson’s disease (PD), and dementia. Neurotrauma/traumatic brain injury (TBI) also leads to acute and chronic neuroinflammatory responses. The expression of some markers may also be altered many years or even decades before the onset of neurodegenerative disorders. In this review, we discuss markers of neuroinflammation, and neurodegeneration associated with acute and chronic brain disorders, especially those associated with neurovascular pathologies. These biomarkers can be evaluated in CSF, or brain tissues. Neurofilament light (NfL), ubiquitin C-terminal hydrolase-L1 (UCHL1), glial fibrillary acidic protein (GFAP), Ionized calcium-binding adaptor molecule 1 (Iba-1), transmembrane protein 119 (TMEM119), aquaporin, endothelin-1, and platelet-derived growth factor receptor beta (PDGFRβ) are some important neuroinflammatory markers. Recent BBB-on-a-chip modeling offers promising potential for providing an in-depth understanding of brain disorders and neurotherapeutics. Integration of these markers in clinical practice could potentially enhance early diagnosis, monitor disease progression, and improve therapeutic outcomes.
Introduction
Neuroinflammatory and neurodegenerative disorders are characterized by the presence of acute and chronic neuroinflammatory responses in the brain. Neuroinflammatory response is the initial response to protect the brain against damage, infection such as microbial infections/sepsis or exposure to toxins by activated glial cells and neurons (Kempuraj et al., 2020a; Gao and Hernandes, 2021; Tran et al., 2022). However, excessive and persistent glial cell activation leads to chronic neuroinflammation-associated neurodegeneration and increases disease severity of neurodegenerative disorders (Le Thuc et al., 2015; Kempuraj et al., 2016). The neuroimmune system is implicated in the development, normal functioning, aging, and integrity of the central nervous system (CNS) (Hickman et al., 2018). Chronic disorders such as Alzheimer’s disease (AD), Parkinson’s disease (PD) and traumatic brain injury (TBI) are neuroinflammatory and neurodegenerative disorders with dysfunctional neurons, synapses, glial cells and their networks (Pathak et al., 2022). Conditions such as Gulf War Illness (GWI) and Myalgic encephalomyelitis/chronic fatigue syndrome (ME/CFS) are also chronic disorders that exhibit several neurological symptoms, neuroimmune dysfunction and neuroinflammation (Wirth et al., 2021; Cohen et al., 2024). The precise mechanisms underlying the pathogenesis of various neurodegenerative diseases are likely different and are currently not yet clearly understood. Different disease triggers can cause neuroinflammation and neuronal damage in different brain regions involving specific types of brain cells and pathways. Additionally, inflammatory mediators from peripheral inflammation can also influence neuroinflammation and neurodegeneration in the brain through a defective and vulnerable blood-brain barrier (BBB) (Kempuraj et al., 2017).
The BBB plays an important role in brain homeostasis by allowing selective molecules from peripheral blood into the brain parenchyma (Chin and Goh, 2018; Zapata-Acevedo et al., 2024). Neuroinflammation and neurodegenerative disorders disrupt the BBB, and increase permeability allowing the entry of immune cells, inflammatory mediators, toxic substances, and pathogens from the peripheral blood into the brain (Musafargani et al., 2020). Derangements and damage to the tight junction (TJ), adherens junction (AdJ), and gap junction (GJ) components of the BBB lead to increased BBB permeability, resulting in edema, increased neuroinflammation and neuronal damage in various brain disorders (Kempuraj et al., 2020a; Bhowmick et al., 2019). Neuroinflammation can lead to upregulation or downregulation of certain specific markers in different brain cells. Neuroinflammation can be beneficial by removing cellular debris and promoting the tissue repair process (Le Thuc et al., 2015). Neuroinflammation has also been shown to enable the proliferation and maturation of neuronal precursor cells, axonal regeneration, and remyelination over denuded axons (Yong et al., 2019). Damage/activation of glial cells, specialized brain endothelial cells, neurons, and BBB structure trigger the release of distinct markers from these cells into the cerebrospinal fluid (CSF) and blood that can be assayed by different procedures for the evaluation of disease status, progression and therapeutic efficacy. However, the dynamics of the BBB in various pathophysiological conditions are not yet clearly known. The development of BBB-on-a-chip modeling in the last decade has the potential for further understanding of BBB dynamics in pathophysiological conditions and neurotherapeutics (Peng et al., 2022; Ohbuchi et al., 2024). In this review, we present markers of neurons, glial cells, neurovascular unit (NVU), BBB proteins, neuroinflammation, and neurodegeneration associated with acute and chronic brain disorders.
Neuroinflammation and neurodegeneration markers
Neurogenesis is a turnover process that generates new neurons during adulthood, maintaining the integrity of the brain. Neurodegeneration is a slow and progressive dysfunction, loss of axons and neurons, which is accelerated by the aging process as well as the neuroinflammatory process (Culig et al., 2022). Mature neuronal markers include nuclear protein neuronal nuclei (NeuN; nuclei), neuron-specific enolase (NSE; cell bodies/soma), neurofilament light (NfL; axons), TUJ1 (class III beta-tubulin; cytoskeleton), tau (axon, cell body, dendrites), spectrin breakdown products (SBDPs; axons), and microtubule-associated protein 2 (MAP2; dendrites) which indicate specific parts of the neuron or damage (Zetterberg and Blennow, 2016; Figure 1). Synaptic markers include synaptosomal-associated protein (SNAP25), synaptophysin (SYP), and neuroligin (Zetterberg and Blennow, 2016). Neurodegeneration can be assessed by neuronal markers MAP2, NfL, TUJ1, and SYP. However, certain markers such as amyloid precursor protein (APP), amyloid β (Aβ) and tau are more specific to AD pathology. Synaptic disorder, synaptic loss and cognitive decline are common manifestations of neurodegenerative disorders (Dejanovic et al., 2024). Neuronal damage, neurodegeneration and neuronal loss have been reported in AD, PD and TBI. Nearly 19.5% of soldiers deployed in Operation Iraqi Freedom (OIF) and Operation Enduring Freedom (OEF) were exposed to blast traumatic brain injury (bTBI) (Kempuraj et al., 2020a). Certain conditions such as TBI and stress are risk factors for the onset of progressive neurodegenerative disorders including AD and PD or dementia or can exacerbate the existing AD, PD pathologies and dementia (Kempuraj et al., 2020b; Brett et al., 2022). The levels of ubiquitin C-terminal hydrolase-L1 (UCH-L1) and glial fibrillary acidic protein (GFAP) in the blood are U.S. Food and Drug Administration (FDA)-approved biomarkers for mild TBI (mTBI) (Wang et al., 2021a). Certain brain injury/TBI markers include UCH-L1, NSE, erythrocyte membrane protein band 4.1 (EPB41) for cell body/soma injury, NfL, tau, myelin basic protein (MBP) for axonal injury, SNCA for synaptic injury, GFAP, S100B for glial cell injury and inflammatory cytokines and neurotoxic mediators (for inflammation) (Silvestro et al., 2024; Zetterberg and Blennow, 2016). Certain chronic neuroimmune conditions such as ME/CFS and GWI are associated with neuroinflammation but may not have apparent neurodegeneration (Cohen et al., 2024; O’Callaghan and Miller, 2019). Positron emission tomography (PET) and magnetic resonance spectroscopic (MRS) neuroimaging allow for a non-invasive “read” of the brain for neuroinflammatory processes and neuronal integrity in brain diseases (Van Der Naalt, 2015; Lee et al., 2024).
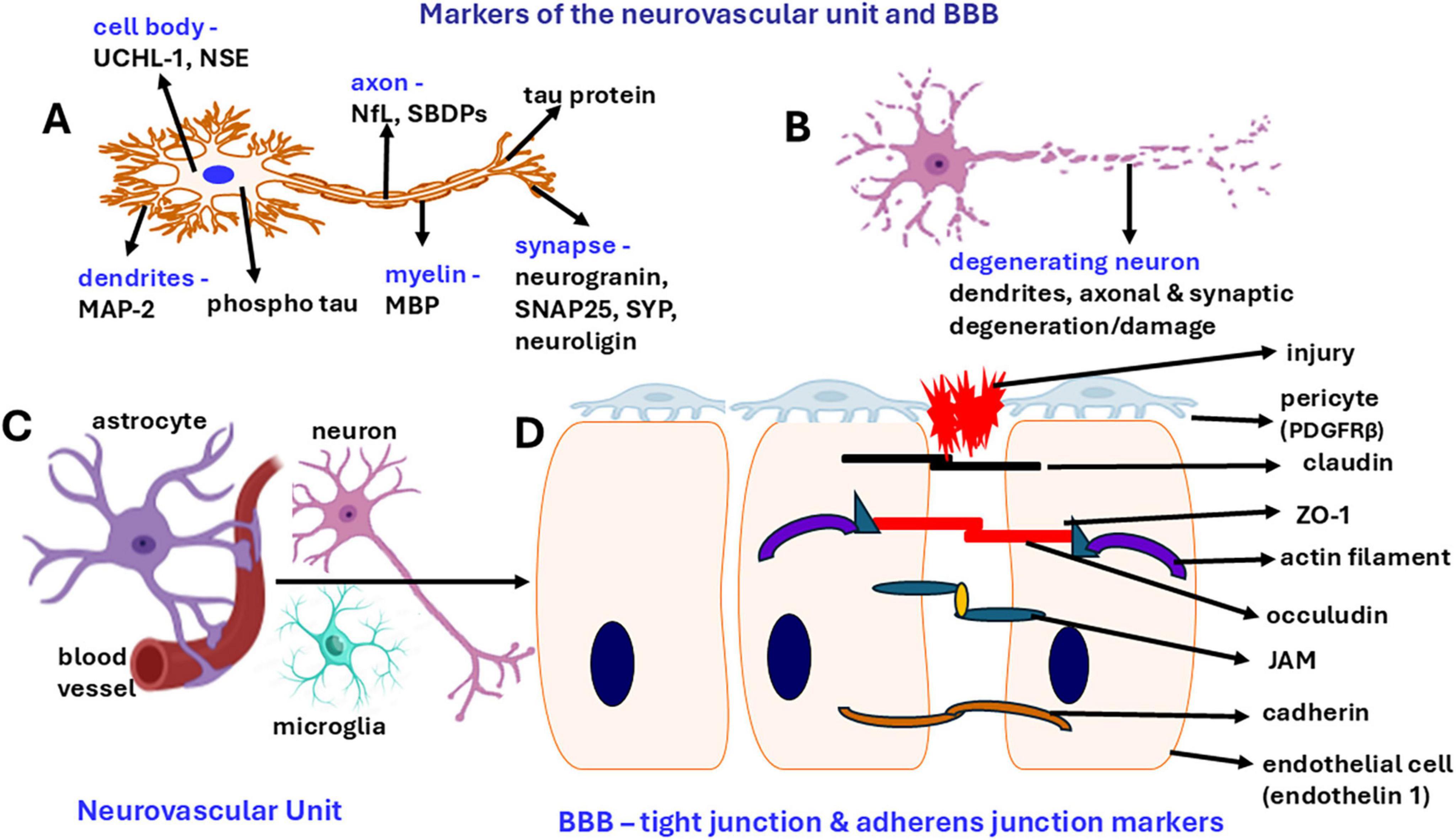
Figure 1. Schematic diagram shows neuronal markers in the neurovascular unit with BBB. BBB is a dynamic structure that functions as a gatekeeper. (A) Markers in neurons in different regions. (B) neurodegeneration of dendrites, axon, synaptic area. (C) NVU is comprised of micro blood vessels, endothelial cells, BBB, pericytes, neurons, astrocytes, and microglia. (D) BBB with endothelial cells, pericytes, tight junction and adherens junctions consisting of claudin, zonula occludens (ZO-1), occuludin, junctional adhesion molecule (JAM) and cadherin that regulates BBB permeability. The presence of increased levels of these markers in the blood or CFS indicates the damage to these structures. Derangement of BBB proteins can also cause BBB dysfunction.
Activation of glial cells such as microglia and astrocytes lead to the release of molecules that trigger neuroinflammatory response and neuroinflammation. Both microglia and astrocytes can function either as neurotoxic (proinflammatory) M1 microglia and A1 astrocytes or as anti-inflammatory (neuroprotective) M2 microglia and A2 astrocytes phenotypes (Kwon and Koh, 2020; Guo et al., 2022). M1 microglia and A1 astrocytes release proinflammatory and neurotoxic molecules, whereas M2 microglia and A2 astrocytes produce neurotrophic and neuroprotective molecules that support neuronal growth and survival (Kwon and Koh, 2020). The M1/A1 or M2/A2 status (phenotype) of these cells can change during disease progression and can alter the severity of neuroinflammatory and neurodegenerative diseases (Kwon and Koh, 2020). Resting astrocytes (A0) become functional astrocytes (A1 and A2) by stimulation (Ding et al., 2021; Figure 2). Senescent dystrophic microglia have abnormal morphology with deramification (thin and short branches) and fragmented cytoplasm (Woollacott et al., 2020). The number of dystrophic microglia increases in neurodegenerative disorders such as AD in which many microglia are dysfunctional and senescent (Woollacott et al., 2020; Shahidehpour et al., 2021). Neuroinflammatory and neurodegenerative conditions impact the NVU which consists of microvascular specialized endothelial cells with BBB complex, pericytes and astrocytes (Bhowmick et al., 2019; Kempuraj et al., 2020a; Kempuraj et al., 2024). Disruption of NUV and BBB, glial activation and dementia have been reported in the recent coronavirus disease 2019 (COVID-19)/Long COVID conditions caused by infection with severe acute respiratory syndrome coronavirus 2 (SARS-CoV-2) (Kempuraj et al., 2024; Owens et al., 2024; Theoharides and Kempuraj, 2023; Shi et al., 2023; Zingaropoli et al., 2022). Inflammation in the brain activates glial cells to release inflammatory mediators which activate endothelial cells to express adhesion molecules and attract the peripheral blood leukocytes to the inflammatory site in the brain. Activated endothelial cells lead to loss of vascular integrity, increased adhesion molecule expression and cytokine and chemokine release including C-C motif ligand 2 (CCL2), CCL3, and interleukin-8 (IL-8) (Theofilis et al., 2021; Alsbrook et al., 2023). Cerebral endothelial cells express toll-like receptors (TLRs), chemokine receptors C-X-C motif chemokine receptor 1 (CXCR1), CXCR2, CXCR3, CCR3, CXCR4, and tumor necrosis factor receptors (TNFRs) TNFR1 and TNFR2. Pericytes cover the micro vessels in the brain and express various contractile and cytoskeleton proteins such as α-smooth muscle actin, nestin, myosin, vimentin, and desmin, cell surface neural/glial antigen 2 (NG2), platelet derived growth factor receptor beta (PDGFRβ), cluster of differentiation 13 (CD13), and CD146 (Alarcon-Martinez et al., 2021). Pericytes play a role in regulating the BBB, angiogenesis, removal of toxins, blood flow, stem cells, and neuroinflammation (Bhowmick et al., 2019). Pericytes can differentiate into microglia-like cells with phagocytic activity indicating that pericyte loss may increase leukocyte infiltration (Alsbrook et al., 2023). Additionally, pericytes can express TLR4 and exert a proinflammatory response. Pericyte damage can lead to BBB dysfunction allowing the influx of neurotoxic molecules in the brain from the peripheral blood. Astrocytes are the most abundant cells in the brain and are involved in the formation, maintenance and BBB permeability (Schiera et al., 2024; Rauf et al., 2022). Increased GFAP expression, an astrocyte marker, activates astrocytes and releases IL-1β, IL-6 and TNF (Giovannoni and Quintana, 2020). Astrocytes also induce anti-inflammatory effects and regulate neurotransmitter homeostasis such as glutamate. Peripheral inflammation may lead to brain endothelial activation, allowing peripheral blood inflammatory factors to enter the brain, activate perivascular macrophages and microglia, and initiate neuroinflammation without any primary injury or disease in the brain (Mayer and Fischer, 2024). Microglia are the primary innate/resident immune cells in the brain that first respond to injuries in the brain (Rauf et al., 2022). Microglia constantly sense changes in the brain tissue microenvironment for housekeeping function that helps neuronal health and functions (Mayer and Fischer, 2024). Microglia can express inflammatory cytokines and chemokines such as TNF, IL-1, IL-6, CCL2, and IL-18 to stimuli and they also express activation marker sTREM2 (soluble triggering receptor expressed on myeloid cells 2).
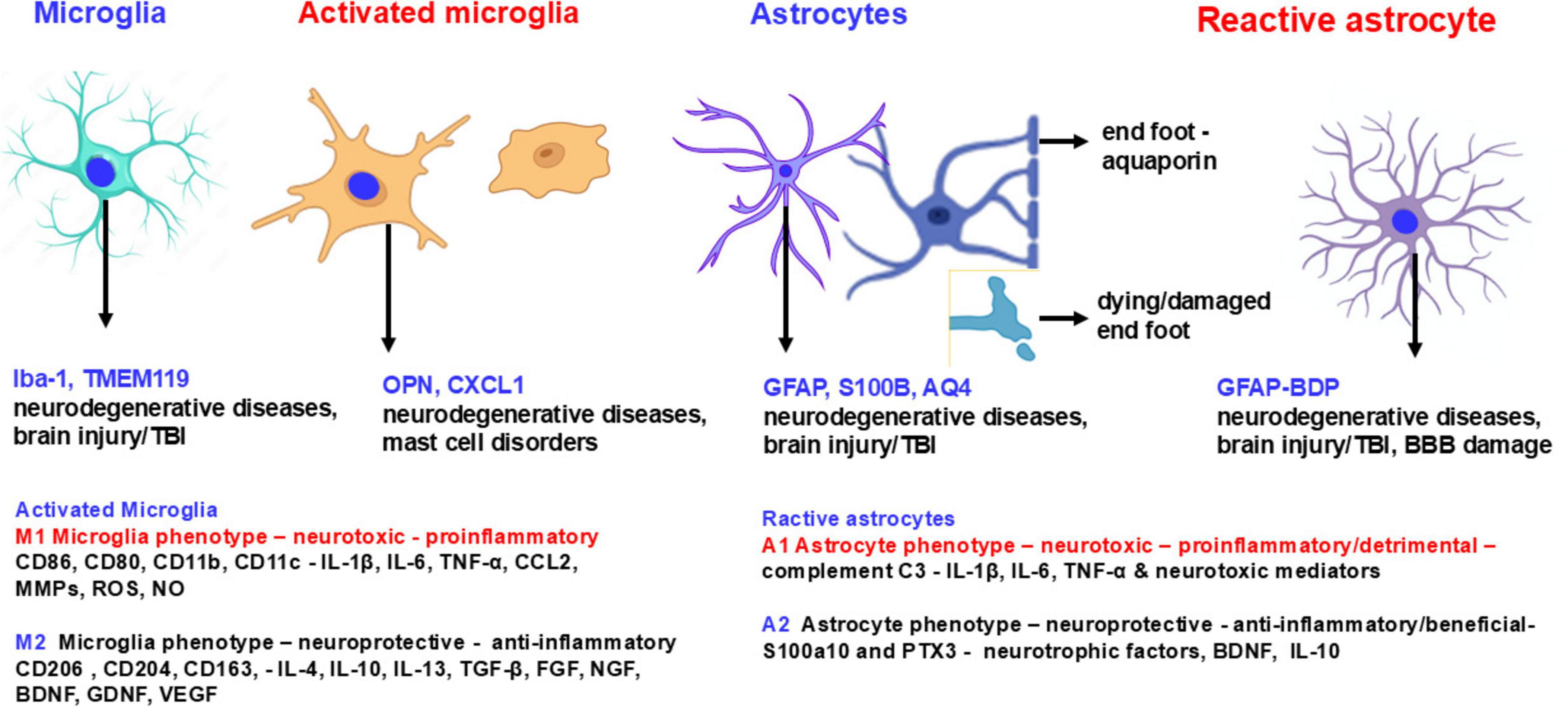
Figure 2. Schematic diagram shows microglial and astrocyte markers in normal, activated, anti-inflammatory and proinflammatory states. Microglia and astrocytes are activated in various neuroinflammatory and neurodegenerative disorders. Activated microglia and astrocytes express several markers that are also detected in biofluids. M1 microglia and A1 astrocytic phenotypes are neurotoxic and exacerbate neuroinflammation and neurodegeneration whereas M2 microglia and A2 astrocyte phenotypes are neuroprotective and protect the brain. These neurotoxic and neuroprotective glial cells express or secrete different molecules to exert detrimental or protective functions in the brain. AQ4, aquaporin 4; FGF, fibroblast growth factor; NO, nitric oxide; OPN, osteopontin; PTX3, pentraxin 3; ROS, reactive oxygen species; S100a10, S100 calcium-binding protein A10.
Understanding NVU/BBB dynamics in the brain’s pathophysiological conditions will improve the treatment options for brain disorders. In addition to the cells in the brain, infiltration of immunocytes, cytokines, chemokines and neurotoxic molecules from the periphery also activate glial cells, further releasing additional inflammatory mediators that accelerate neuroinflammation in the brain. Pathogenic substances that enter from the periphery to the brain also enhance inflammatory response in the brain (Kempuraj et al., 2017). There are several types of biomarkers including immunochemical analysis in tissues that involve tissue biopsy or post-mortem tissue, blood (minimally invasive)/CSF (invasive) based biomarkers, physical by physical examination such as cognitive test, urine, and brain imaging such as MRI (Chahine et al., 2014). Extracellular vehicles (EVs) released from brain cells can be detected in the blood and CSF and used as a marker for brain disorders (Gamez-Valero et al., 2019; Ollen-Bittle et al., 2022). Additionally, genomic and proteomic analysis provides molecular level biomarkers with next-generation sequencing and mass spectrometry procedures for neurological disorders (Chase Huizar et al., 2020). Abnormally activated glial cells can secrete disease-specific proteins that can be used as a novel biomarker (Kim et al., 2020). Recent progress in proteomic research has the potential for the development of novel
biomarkers for brain disorders (Kim et al., 2020). MicroRNAs (miRNAs) play an important role in inflammatory response in neuroinflammation (Su et al., 2016). Liquid biopsies such as exosomal miRNA are important biomarkers for many diseases including neurological diseases (Malhotra et al., 2023; Zhou et al., 2024).
Table 1 provides various markers of neurons, astrocytes, microglia, neuroinflammation, neurodegeneration, the NVU, and the BBB complex with their dysfunctions and associated neuropathology. This table also includes some membrane proteins, secreted proteins, signaling proteins and structural proteins associated with brain cells. Effective neurotherapeutic options should ideally target the BBB complex, address the damage and derangement of BBB proteins, and reduce BBB dysfunction.
The BBB is a crucial component of the NVU and plays an important role in the homeostasis of the brain. The NVU regulates BBB permeability, removal of toxic byproducts, and performs immune monitoring. BBB disruption and increased permeability are commonly observed in neurodegenerative disorders and neurotrauma, which increases BBB permeability causing or upregulating neuroinflammatory responses, neuroinflammation and neuronal loss (Yu et al., 2020). Therefore, we have highlighted recent advances in the study of BBB pathogenesis using the BBB-on-a-Chip model for CNS disorders and neurotherapeutics as briefly provided below.
BBB-on-a-Chip for CNS disorders and neurotherapeutics
The integrity of the BBB is maintained by astrocytes, pericytes, endothelial cells, and neurons, TJ, AdJ and GP proteins of the BBB. This integrity is crucial for normal brain function. However, chronic damage to NVU and BBB components leads to BBB dysfunction, increased BBB permeability/leakage, and neuroinflammation in many neurodegenerative diseases (Ohbuchi et al., 2024; Yoon et al., 2021). Therefore, the ability to model BBB behavior and pathogenesis is essential for the understanding of CNS disorders and neurotherapeutics. Vascularization in the brain organoids can be induced by modeling BBB micro environment using chip technology (Urrestizala-Arenaza et al., 2024). The BBB-on-a-chip (BBB chip) micro-engineered laboratory technology is a powerful in vitro model closely resembling human BBB structure to study normal and diseased states (Peng et al., 2022; Berjaoui et al., 2024). BBB-on-a-chip technology has significantly improved over the last decade and has been used to study various neurological diseases including AD, PD and Multiple Sclerosis (MS) (Berjaoui et al., 2024; Kawakita et al., 2022; Yoon et al., 2021; Palma-Florez et al., 2023). Recently neuroinflammation on-a-chip for studying MS (Berjaoui et al., 2024) and neuropathogenesis-on-chips (Amartumur et al., 2024) technology have been reported. The recently developed in vitro microfluidic/microfluidic human BBB-on-a-chip modeling using brain endothelial cells, pericytes, and astrocytes tri-culture model along with immune cell (T-cell) migration will be highly useful for understanding BBB functions, permeability, the pathogenesis of brain diseases, and evaluation of neurotherapeutic drugs that target the BBB (Ohbuchi et al., 2024). However, a fully efficient BBB-on-a-Chip model is still not available to date. A recent article described the use of built-in sensors to characterize BBB models via quasi-direct current and electrical impedance measurements, and various biosensors for the detection of metabolites, drugs, or toxic agents (Kincses et al., 2023). Microfluidic BBB-on-a-Chip provides an engineered physiological microenvironment necessary for real-time monitoring of barrier properties using human cells (Musafargani et al., 2020). The availability of AXION Maestro Edge multiwell microelectrode array (MEA) system (Axion BioSystems, Atlanta, GA) coupled with NETRI’s NeuroFluidics devices (NETRI, Lyon, France) could significantly enhance brain-on-a-Chip and BBB-on-a-Chip modeling in the study of brain disorders including neurotrauma/TBI, and development of drugs that target the BBB (Cohen et al., 2024; Ohbuchi et al., 2024). In a 3D microfluidic system, brain organoids are placed at the center chamber and endothelial cells and pericytes are placed on the side channels to create a micro vascularization system (Urrestizala-Arenaza et al., 2024) In a study, BBBs-on-chips were exposed to TNF-α and IL-1β to mimic neuroinflammation and studies the BBBs-on-chip’s barrier function, cell morphology, increased expression of cell adhesion molecules, increased permeability, and T cell adhesion, extravasation, and migration across BBB-on-chips (Nair et al., 2023). Even though brain-on-a-chip technology advanced the understanding of BBB pathophysiology, these models are still in a preliminary state, and the neurospheroids are still far from the human brain tissue. Thus, new and more advanced clinically relevant bioengineered models of human brain-on-a-chip for drug efficacy evaluation are required (Staicu et al., 2021; Cui and Cho, 2022). We are currently working on a BBB-on-a-Chip model to create disease-surrogate models for different brain disorders. Further research advancement in the BBB-on-a-Chip model could enhance the understanding of BBB dynamics in both health and disease conditions and assist in the development of treatments that target the BBB.
Conclusion
Neuroinflammation is a hallmark of many neurological disorders. Neuroinflammatory and neurodegenerative disorders are multifaceted processes involving the interaction of astrocytes, endothelial cells, neurons, microglia and infiltrating leukocytes as well as peripheral systems. Chronic release of neuroinflammatory mediators induces neuroinflammation, neurodegeneration, synaptic and neuronal loss and BBB dysfunction in the brain. Several molecules expressed by brain cells infiltrating peripheral leukocytes participate in the neuroinflammatory response in specific regions of the brain. Damage of NVU/BBB, TJ and AdJ proteins as well as neuroinflammatory markers could be assessed in the tissue as well as in CSF and blood though they are not specific to many brain disorders. Nevertheless, measuring such biomarkers is crucial for the diagnosis, severity assessment and treatment efficacy of various neurodegenerative disorders.
Author contributions
DK: Conceptualization, Writing – original draft, Writing – review and editing, Supervision. KD: Writing – review and editing. JC: Writing – review and editing. DV: Writing – review and editing. RJ: Writing – review and editing. SK: Writing – review and editing. TA: Writing – review and editing. BC: Writing – review and editing. AC: Writing – review and editing. NK: Writing – review and editing. TT: Writing – review and editing.
Funding
The author(s) declare that no financial support was received for the research, authorship, and/or publication of the article.
Conflict of interest
The authors declare that the research was conducted in the absence of any commercial or financial relationships that could be construed as a potential conflict of interest.
The author(s) declared that they were an editorial board member of Frontiers, at the time of submission. This had no impact on the peer review process and the final decision.
Publisher’s note
All claims expressed in this article are solely those of the authors and do not necessarily represent those of their affiliated organizations, or those of the publisher, the editors and the reviewers. Any product that may be evaluated in this article, or claim that may be made by its manufacturer, is not guaranteed or endorsed by the publisher.
References
Agarwal, K., Backler, W., Bayram, E., Bloom, L., Boeve, B. F., Cha, J. H., et al. (2024). Lewy body dementia: Overcoming barriers and identifying solutions. Alzheimers Dement. 20, 2298–2308. doi: 10.1002/alz.13674
Ahmad, S. J., Feigen, C. M., Vazquez, J. P., Kobets, A. J., and Altschul, D. J. (2022). Neurological Sequelae of COVID-19. J. Integr. Neurosci. 21, 77. doi: 10.31083/j.jin2103077
Ahmed, M. E., Selvakumar, G. P., Kempuraj, D., Raikwar, S. P., Thangavel, R., Bazley, K., et al. (2020). Neuroinflammation mediated by GMF exacerbates neuronal injury in an in vitro model of traumatic brain injury. J. Neurotrauma 37, 1645–1655. doi: 10.1089/neu.2019.6932
Aksnes, M., Capogna, E., Vidal-Pineiro, D., Chaudhry, F. A., Myrstad, M., Idland, A. V., et al. (2023). Matrix metalloproteinases are associated with brain atrophy in cognitively unimpaired individuals. Neurobiol. Aging 131, 11–23. doi: 10.1016/j.neurobiolaging.2023.05.012
Alarcon-Martinez, L., Yemisci, M., and Dalkara, T. (2021). Pericyte morphology and function. Histol. Histopathol. 36, 633–643. doi: 10.14670/HH-18-314
Albini, M., Krawczun-Rygmaczewska, A., and Cesca, F. (2023). Astrocytes and brain-derived neurotrophic factor (BDNF). Neurosci. Res. 197, 42–51. doi: 10.1016/j.neures.2023.02.001
Allen, S. J., Watson, J. J., Shoemark, D. K., Barua, N. U., and Patel, N. K. (2013). GDNF, NGF and BDNF as therapeutic options for neurodegeneration. Pharmacol. Ther. 138, 155–175. doi: 10.1016/j.pharmthera.2013.01.004
Alluri, H., Peddaboina, C. S., and Tharakan, B. (2024). Evaluation of Tight Junction Integrity in Brain Endothelial Cells Using Confocal Microscopy. Methods Mol. Biol. 2711, 257–262. doi: 10.1007/978-1-0716-3429-5_21
Alonso, A. D. C., El Idrissi, A., Candia, R., Morozova, V., and Kleiman, F. E. (2024). Tau: More than a microtubule-binding protein in neurons. Cytoskeleton 81, 71–77. doi: 10.1002/cm.21795
Alsbrook, D. L., Di Napoli, M., Bhatia, K., Biller, J., Andalib, S., Hinduja, A., et al. (2023). Neuroinflammation in acute ischemic and hemorrhagic stroke. Curr. Neurol. Neurosci. Rep. 23, 407–431. doi: 10.1007/s11910-023-01282-2
Al-Thomali, A. W., Al-Kuraishy, H. M., Al-Gareeb, A. I., De Waard, M., Sabatier, J. M., Khan Khalil, A. A., et al. (2022). Role of neuropilin 1 in COVID-19 patients with acute ischemic stroke. Biomedicines 10, 2032. doi: 10.3390/biomedicines10082032
Amartumur, S., Nguyen, H., Huynh, T., Kim, T. S., Woo, R. S., Oh, E., et al. (2024). Neuropathogenesis-on-chips for neurodegenerative diseases. Nat. Commun. 15, 2219. doi: 10.1038/s41467-024-46554-8
Amini Harandi, A., Siavoshi, F., Shirzadeh Barough, S., Amini Harandi, A., Pakdaman, H., Sahraian, M. A., et al. (2022). Vascular endothelial growth factor as a predictive and prognostic biomarker for multiple sclerosis. Neuroimmunomodulation 29, 476–485. doi: 10.1159/000525600
Antipova, D., and Bandopadhyay, R. (2017). Expression of DJ-1 in neurodegenerative disorders. Adv. Exp. Med. Biol. 1037, 25–43. doi: 10.1007/978-981-10-6583-5_3
Asghari, K., Niknam, Z., Mohammadpour-Asl, S., and Chodari, L. (2024). Cellular junction dynamics and Alzheimer’s disease: a comprehensive review. Mol Biol Rep. 51, 273. doi: 10.1007/s11033-024-09242-w
Aydogan Avsar, P., and Akkus, M. (2024). ZO-1 serum levels as a potential biomarker for psychotic disorder. Clin. Neuropharmacol. 47, 67–71. doi: 10.1097/WNF.0000000000000590
Babkina, A. S., Lyubomudrov, M. A., Golubev, M. A., Pisarev, M. V., and Golubev, A. M. (2024). Neuron-specific enolase-what are we measuring? Int. J. Mol. Sci. 25, 5040. doi: 10.3390/ijms25095040
Banecki, K., and Dora, K. A. (2023). Endothelin-1 in Health and Disease. Int. J. Mol. Sci. 24, 11295. doi: 10.3390/ijms241411295
Bei, J., Miranda-Morales, E. G., Gan, Q., Qiu, Y., Husseinzadeh, S., Liew, J. Y., et al. (2023). Circulating exosomes from Alzheimer’s Disease suppress vascular endothelial-cadherin expression and induce barrier dysfunction in recipient brain microvascular endothelial cell. J. Alzheimers Dis. 95, 869–885. doi: 10.3233/JAD-230347
Benveniste, E. N., Nguyen, V. T., and Wesemann, D. R. (2004). Molecular regulation of CD40 gene expression in macrophages and microglia. Brain Behav. Immun. 18, 7–12. doi: 10.1016/j.bbi.2003.09.001
Berjaoui, C., Kachouh, C., Joumaa, S., Hussein Ghayyad, M., Abate Bekele, B., Ajirenike, R., et al. (2024). Neuroinflammation-on-a-chip for multiple sclerosis research: a narrative review. Ann. Med. Surg. 86, 4053–4059. doi: 10.1097/MS9.0000000000002231
Bhend, M. E., Kempuraj, D., Sinha, N. R., Gupta, S., and Mohan, R. R. (2023). Role of aquaporins in corneal healing post chemical injury. Exp. Eye Res. 228, 109390. doi: 10.1016/j.exer.2023.109390
Bhowmick, S., D’Mello, V., Caruso, D., Wallerstein, A., and Abdul-Muneer, P. M. (2019). Impairment of pericyte-endothelium crosstalk leads to blood-brain barrier dysfunction following traumatic brain injury. Exp. Neurol. 317, 260–270. doi: 10.1016/j.expneurol.2019.03.014
Bohnert, S., Wirth, C., Schmitz, W., Trella, S., Monoranu, C. M., Ondruschka, B., et al. (2021). Myelin basic protein and neurofilament H in postmortem cerebrospinal fluid as surrogate markers of fatal traumatic brain injury. Int. J. Legal Med. 135, 1525–1535. doi: 10.1007/s00414-021-02606-y
Boulay, A. C., Mazeraud, A., Cisternino, S., Saubamea, B., Mailly, P., Jourdren, L., et al. (2015). Immune quiescence of the brain is set by astroglial connexin 43. J. Neurosci. 35, 4427–4439. doi: 10.1523/JNEUROSCI.2575-14.2015
Boutte, A. M., Deng-Bryant, Y., Johnson, D., Tortella, F. C., Dave, J. R., Shear, D. A., et al. (2016). Serum Glial Fibrillary Acidic Protein Predicts Tissue Glial Fibrillary Acidic Protein Break-Down Products and Therapeutic Efficacy after Penetrating Ballistic-Like Brain Injury. J. Neurotrauma 33, 147–156. doi: 10.1089/neu.2014.3672
Bozoyan, L., Dumas, A., Patenaude, A., and Vallieres, L. (2015). Interleukin-36gamma is expressed by neutrophils and can activate microglia, but has no role in experimental autoimmune encephalomyelitis. J. Neuroinflammation 12, 173. doi: 10.1186/s12974-015-0392-7
Brett, B. L., Gardner, R. C., Godbout, J., Dams-O’Connor, K., and Keene, C. D. (2022). Traumatic brain injury and risk of neurodegenerative disorder. Biol. Psychiatry 91, 498–507. doi: 10.1016/j.biopsych.2021.05.025
Bruno, F., Abondio, P., Montesanto, A., Luiselli, D., Bruni, A. C., and Maletta, R. (2023). The Nerve growth factor receptor (NGFR/p75(NTR)): a major player in Alzheimer’s disease. Int. J. Mol. Sci. 24:3200. doi: 10.3390/ijms24043200
Brunt, V. E., Ikoba, A. P., Ziemba, B. P., Ballak, D. B., Hoischen, A., Dinarello, C. A., et al. (2023). Circulating interleukin-37 declines with aging in healthy humans: relations to healthspan indicators and IL37 gene SNPs. Geroscience 45, 65–84. doi: 10.1007/s11357-022-00587-3
Buneeva, O., and Medvedev, A. (2024). Ubiquitin Carboxyl-Terminal Hydrolase L1 and Its Role in Parkinson’s Disease. Int. J. Mol. Sci. 25, 1303. doi: 10.3390/ijms25021303
Cantuti-Castelvetri, L., Ojha, R., Pedro, L. D., Djannatian, M., Franz, J., Kuivanen, S., et al. (2020). Neuropilin-1 facilitates SARS-CoV-2 cell entry and infectivity. Science 370, 856–860. doi: 10.1126/science.abd2985
Cao, K., Liao, X., Lu, J., Yao, S., Wu, F., Zhu, X., et al. (2018). IL-33/ST2 plays a critical role in endothelial cell activation and microglia-mediated neuroinflammation modulation. J. Neuroinflammation 15, 136. doi: 10.1186/s12974-018-1169-6
Cao, X., and Tabuchi, K. (2017). Functions of synapse adhesion molecules neurexin/neuroligins and neurodevelopmental disorders. Neurosci. Res. 116, 3–9. doi: 10.1016/j.neures.2016.09.005
Carniglia, L., Ramirez, D., Durand, D., Saba, J., Turati, J., Caruso, C., et al. (2017). Neuropeptides and microglial activation in inflammation, pain, and neurodegenerative diseases. Mediators Inflamm. 2017:5048616. doi: 10.1155/2017/5048616
Cattaneo, M. (2015). P2Y12 receptors: structure and function. J. Thromb. Haemost. 13(Suppl. 1), S10–S16. doi: 10.1111/jth.12952
Chahine, L. M., Stern, M. B., and Chen-Plotkin, A. (2014). Blood-based biomarkers for Parkinson’s disease. Parkinsonism Relat. Disord. 20, S99–S103. doi: 10.1016/S1353-8020(13)70025-7
Chase Huizar, C., Raphael, I., and Forsthuber, T. G. (2020). Genomic, proteomic, and systems biology approaches in biomarker discovery for multiple sclerosis. Cell Immunol. 358, 104219. doi: 10.1016/j.cellimm.2020.104219
Chen, Y., Wang, Y., Xu, J., Hou, T., Zhu, J., Jiang, Y., et al. (2023). Multiplex assessment of serum chemokines CCL2, CCL5, CXCL1, CXCL10, and CXCL13 following traumatic brain injury. Inflammation. 46, 244–255. doi: 10.1007/s10753-022-01729-7
Chin, E., and Goh, E. (2018). Blood-brain barrier on a chip. Methods Cell Biol. 146, 159–182. doi: 10.1016/bs.mcb.2018.06.003
Cho, S. J., Park, M. H., Han, C., Yoon, K., and Koh, Y. H. (2017). VEGFR2 alteration in Alzheimer’s disease. Sci. Rep. 7, 17713. doi: 10.1038/s41598-017-18042-1
Chu, P. H., Chen, S. C., Chen, H. Y., Wu, C. B., Huang, W. T., and Chiang, H. Y. (2023). Astrocyte-associated fibronectin promotes the proinflammatory phenotype of astrocytes through beta1 integrin activation. Mol. Cell Neurosci. 125, 103848. doi: 10.1016/j.mcn.2023.103848
Cibelli, A., Stout, R., Timmermann, A., de Menezes, L., Guo, P., Maass, K., et al. (2021). Cx43 carboxyl terminal domain determines AQP4 and Cx30 endfoot organization and blood brain barrier permeability. Sci. Rep. 11, 24334. doi: 10.1038/s41598-021-03694-x
Cintron-Colon, A. F., Almeida-Alves, G., Boynton, A. M., and Spitsbergen, J. M. (2020). GDNF synthesis, signaling, and retrograde transport in motor neurons. Cell Tissue Res. 382, 47–56. doi: 10.1007/s00441-020-03287-6
Cohen, J., Mathew, A., Dourvetakis, K. D., Sanchez-Guerrero, E., Pangeni, R. P., Gurusamy, N., et al. (2024). Recent research trends in neuroinflammatory and neurodegenerative disorders. Cells 13, 511. doi: 10.3390/cells13060511
Craig, A. M., and Kang, Y. (2007). Neurexin-neuroligin signaling in synapse development. Curr. Opin. Neurobiol. 17, 43–52. doi: 10.1016/j.conb.2007.01.011
Cui, B., and Cho, S. W. (2022). Blood-brain barrier-on-a-chip for brain disease modeling and drug testing. BMB Rep. 55, 213–219. doi: 10.5483/BMBRep.2022.55.5.043
Culig, L., Chu, X., and Bohr, V. A. (2022). Neurogenesis in aging and age-related neurodegenerative diseases. Ageing Res. Rev. 78, 101636. doi: 10.1016/j.arr.2022.101636
Custodia, A., Aramburu-Nunez, M., Rodriguez-Arrizabalaga, M., Pias-Peleteiro, J. M., Vazquez-Vazquez, L., Camino-Castineiras, J., et al. (2023). Biomarkers Assessing Endothelial Dysfunction in Alzheimer’s Disease. Cells 12, 962. doi: 10.3390/cells12060962
Dai, W., Zhan, M., Gao, Y., Sun, H., Zou, Y., Laurent, R., et al. (2024). Brain delivery of fibronectin through bioactive phosphorous dendrimers for Parkinson’s disease treatment via cooperative modulation of microglia. Bioact. Mater. 38, 45–54. doi: 10.1016/j.bioactmat.2024.04.005
Dejanovic, B., Sheng, M., and Hanson, J. E. (2024). Targeting synapse function and loss for treatment of neurodegenerative diseases. Nat. Rev. Drug Discov. 23, 23–42. doi: 10.1038/s41573-023-00823-1
Dichev, V., Kazakova, M., and Sarafian, V. (2020). YKL-40 and neuron-specific enolase in neurodegeneration and neuroinflammation. Rev. Neurosci. 31, 539–553. doi: 10.1515/revneuro-2019-0100
Ding, X. W., Li, R., Geetha, T., Tao, Y. X., and Babu, J. R. (2020). Nerve growth factor in metabolic complications and Alzheimer’s disease: Physiology and therapeutic potential. Biochim. Biophys. Acta Mol. Basis Dis. 1866, 165858. doi: 10.1016/j.bbadis.2020.165858
Ding, Z. B., Song, L. J., Wang, Q., Kumar, G., Yan, Y. Q., and Ma, C. G. (2021). Astrocytes: a double-edged sword in neurodegenerative diseases. Neural Regen. Res. 16, 1702–1710. doi: 10.4103/1673-5374.306064
Dithmer, S., Blasig, I. E., Fraser, P. A., Qin, Z., and Haseloff, R. F. (2024). The basic requirement of tight junction proteins in blood-brain barrier function and their role in pathologies. Int. J. Mol. Sci. 25, 5601. doi: 10.3390/ijms25115601
Domingues, A., and Fantin, A. (2021). Neuropilin 1 regulation of vascular permeability signaling. Biomolecules 11, 666. doi: 10.3390/biom11050666
D’Orleans-Juste, P., Akide Ndunge, O. B., Desbiens, L., Tanowitz, H. B., and Desruisseaux, M. S. (2019). Endothelins in inflammatory neurological diseases. Pharmacol. Ther. 194, 145–160. doi: 10.1016/j.pharmthera.2018.10.001
Duan, W., Zhang, Y. P., Hou, Z., Huang, C., Zhu, H., Zhang, C. Q., et al. (2016). Novel Insights into NeuN: from Neuronal Marker to Splicing Regulator. Mol. Neurobiol. 53, 1637–1647. doi: 10.1007/s12035-015-9122-5
Duly, A. M. P., Kao, F. C. L., Teo, W. S., and Kavallaris, M. (2022). betaIII-tubulin gene regulation in health and disease. Front. Cell Dev. Biol. 10:851542. doi: 10.3389/fcell.2022.851542
Echeverria, V., Barreto, G. E., Avila-Rodriguezc, M., Tarasov, V. V., and Aliev, G. (2017). Is VEGF a Key Target of Cotinine and Other Potential Therapies Against Alzheimer Disease? Curr. Alzheimer. Res. 14, 1155–1163. doi: 10.2174/1567205014666170329113007
Elahi, F. M., Casaletto, K. B., La Joie, R., Walters, S. M., Harvey, D., Wolf, A., et al. (2020). Plasma biomarkers of astrocytic and neuronal dysfunction in early- and late-onset Alzheimer’s disease. Alzheimers Dement. 16, 681–695. doi: 10.1016/j.jalz.2019.09.004
Elkabes, S., DiCicco-Bloom, E. M., and Black, I. B. (1996). Brain microglia/macrophages express neurotrophins that selectively regulate microglial proliferation and function. J. Neurosci. 16, 2508–2521. doi: 10.1523/JNEUROSCI.16-08-02508.1996
Erenler, A. K., and Baydin, A. (2020). Interleukin-33 (IL-33) as a diagnostic and prognostic factor in traumatic brain injury. Emerg. Med. Int. 2020, 1832345. doi: 10.1155/2020/1832345
Fan, J., Fong, T., Chen, X., Chen, C., Luo, P., and Xie, H. (2018). Glia maturation factor-beta: a potential therapeutic target in neurodegeneration and neuroinflammation. Neuropsychiatr. Dis. Treat. 14, 495–504. doi: 10.2147/NDT.S157099
Ford, M. M., George, B. E., Van Laar, V. S., Holleran, K. M., Naidoo, J., Hadaczek, P., et al. (2023). GDNF gene therapy for alcohol use disorder in male non-human primates. Nat. Med. 29, 2030–2040. doi: 10.1038/s41591-023-02463-9
Fu, A. K., Hung, K. W., Yuen, M. Y., Zhou, X., Mak, D. S., Chan, I. C., et al. (2016). IL-33 ameliorates Alzheimer’s disease-like pathology and cognitive decline. Proc. Natl. Acad. Sci. U. S. A. 113, E2705–E2713. doi: 10.1073/pnas.1604032113
Fusco, F. R., and Paldino, E. (2024). Is GDNF to Parkinson’s disease what BDNF is to Huntington’s disease? Neural Regen. Res. 19, 973–974. doi: 10.4103/1673-5374.385305
Gamez-Valero, A., Beyer, K., and Borras, F. E. (2019). Extracellular vesicles, new actors in the search for biomarkers of dementias. Neurobiol. Aging 74, 15–20. doi: 10.1016/j.neurobiolaging.2018.10.006
Gao, Q., and Hernandes, M. S. (2021). Sepsis-associated encephalopathy and blood-brain barrier dysfunction. Inflammation 44, 2143–2150. doi: 10.1007/s10753-021-01501-3
Geisert, E. E. Jr., Johnson, H. G., and Binder, L. I. (1990). Expression of microtubule-associated protein 2 by reactive astrocytes. Proc. Natl. Acad. Sci. U. S. A. 87, 3967–3971. doi: 10.1073/pnas.87.10.3967
George, N., and Geller, H. M. (2018). Extracellular matrix and traumatic brain injury. J. Neurosci. Res. 96, 573–588. doi: 10.1002/jnr.24151
Giovannoni, F., and Quintana, F. J. (2020). The Role of Astrocytes in CNS Inflammation. Trends Immunol. 41, 805–819. doi: 10.1016/j.it.2020.07.007
Gomez Morillas, A., Besson, V. C., and Lerouet, D. (2021). Microglia and Neuroinflammation: What Place for P2RY12? Int. J. Mol. Sci. 22, 1636. doi: 10.3390/ijms22041636
Granholm, A. C., and Hamlett, E. D. (2024). The Role of Tau Pathology in Alzheimer’s Disease and Down Syndrome. J. Clin. Med. 13, 1338. doi: 10.3390/jcm13051338
Gruel, R., Bijnens, B., Van Den Daele, J., Thys, S., Willems, R., Wuyts, D., et al. (2024). S100A8-enriched microglia populate the brain of tau-seeded and accelerated aging mice. Aging Cell. 23, e14120. doi: 10.1111/acel.14120
Guo, S., Wang, H., and Yin, Y. (2022). Microglia Polarization From M1 to M2 in Neurodegenerative Diseases. Front. Aging Neurosci. 14:815347. doi: 10.3389/fnagi.2022.815347
Gupta, T., Kumar, M., Kaur, U. J., Rao, A., and Bharti, R. (2024). Mapping ACE2 and TMPRSS2 co-expression in human brain tissue: implications for SARS-CoV-2 neurological manifestations. J. Neurovirol. doi: 10.1007/s13365-024-01206-x
Gusel’nikova, V. V., and Korzhevskiy, D. E. (2015). NeuN As a Neuronal Nuclear Antigen and Neuron Differentiation Marker. Acta Naturae. 7, 42–47.
Haffke, M., Freitag, H., Rudolf, G., Seifert, M., Doehner, W., Scherbakov, N., et al. (2022). Endothelial dysfunction and altered endothelial biomarkers in patients with post-COVID-19 syndrome and chronic fatigue syndrome (ME/CFS). J. Transl. Med. 20, 138. doi: 10.1186/s12967-022-03346-2
Haque, A., Ray, S. K., Cox, A., and Banik, N. L. (2016). Neuron specific enolase: a promising therapeutic target in acute spinal cord injury. Metab. Brain Dis. 31, 487–495. doi: 10.1007/s11011-016-9801-6
Harris, R., Miners, J. S., Allen, S., and Love, S. (2018). VEGFR1 and VEGFR2 in Alzheimer’s Disease. J. Alzheimers Dis. 61, 741–752. doi: 10.3233/JAD-170745
Haruwaka, K., Ikegami, A., Tachibana, Y., Ohno, N., Konishi, H., Hashimoto, A., et al. (2019). Dual microglia effects on blood brain barrier permeability induced by systemic inflammation. Nat. Commun. 10, 5816. doi: 10.1038/s41467-019-13812-z
Hashimoto, Y., Greene, C., Munnich, A., and Campbell, M. (2023). The CLDN5 gene at the blood-brain barrier in health and disease. Fluids Barriers CNS. 20, 22. doi: 10.1186/s12987-023-00424-5
Hausrat, T. J., Radwitz, J., Lombino, F. L., Breiden, P., and Kneussel, M. (2021). Alpha- and beta-tubulin isotypes are differentially expressed during brain development. Dev. Neurobiol. 81, 333–350. doi: 10.1002/dneu.22745
Hawksworth, J., Fernandez, E., and Gevaert, K. (2022). A new generation of AD biomarkers: 2019 to 2021. Ageing Res. Rev. 79, 101654. doi: 10.1016/j.arr.2022.101654
Hegen, A., Koidl, S., Weindel, K., Marme, D., Augustin, H. G., and Fiedler, U. (2004). Expression of angiopoietin-2 in endothelial cells is controlled by positive and negative regulatory promoter elements. Arterioscler. Thromb Vasc. Biol. 24, 1803–1809. doi: 10.1161/01.ATV.0000140819.81839.0e
Hellwig, K., Kvartsberg, H., Portelius, E., Andreasson, U., Oberstein, T. J., Lewczuk, P., et al. (2015). Neurogranin and YKL-40: independent markers of synaptic degeneration and neuroinflammation in Alzheimer’s disease. Alzheimers Res. Ther. 7, 74. doi: 10.1186/s13195-015-0161-y
Hickman, S., Izzy, S., Sen, P., Morsett, L., and El, K. J. (2018). Microglia in neurodegeneration. Nat. Neurosci. 21, 1359–1369.
Hossain, I., Marklund, N., Czeiter, E., Hutchinson, P., and Buki, A. (2024). Blood biomarkers for traumatic brain injury: A narrative review of current evidence. Brain Spine. 4, 102735. doi: 10.1016/j.bas.2023.102735
Hostenbach, S., D’Haeseleer, M., Kooijman, R., and De Keyser, J. (2016). The pathophysiological role of astrocytic endothelin-1. Prog. Neurobiol. 144, 88–102. doi: 10.1016/j.pneurobio.2016.04.009
Hu, X., Geng, P., Zhao, X., Wang, Q., Liu, C., Guo, C., et al. (2023). The NG2-glia is a potential target to maintain the integrity of neurovascular unit after acute ischemic stroke. Neurobiol. Dis. 180, 106076. doi: 10.1016/j.nbd.2023.106076
Huang, X., Guo, M., Zhang, Y., Xie, J., Huang, R., Zuo, Z., et al. (2023). Microglial IL-1RA ameliorates brain injury after ischemic stroke by inhibiting astrocytic CXCL1-mediated neutrophil recruitment and microvessel occlusion. Glia 71, 1607–1625. doi: 10.1002/glia.24359
Humes, C., Sic, A., and Knezevic, N. N. (2024). Substance P’s Impact on Chronic Pain and Psychiatric Conditions-A Narrative Review. Int. J. Mol. Sci. 25, 5905. doi: 10.3390/ijms25115905
Ikeshima-Kataoka, H. (2016). Neuroimmunological Implications of AQP4 in Astrocytes. Int. J. Mol. Sci. 17, 1306. doi: 10.3390/ijms17081306
Iyer, M. R., and Kunos, G. (2021). Therapeutic approaches targeting the neurotensin receptors. Expert. Opin. Ther. Pat. 31, 361–386. doi: 10.1080/13543776.2021.1866539
Jackson, R. J., Meltzer, J. C., Nguyen, H., Commins, C., Bennett, R. E., Hudry, E., et al. (2022). APOE4 derived from astrocytes leads to blood-brain barrier impairment. Brain. 145, 3582–3593. doi: 10.1093/brain/awab478
Jain, N., Lewis, C. A., Ulrich, J. D., and Holtzman, D. M. (2023). Chronic TREM2 activation exacerbates Abeta-associated tau seeding and spreading. J. Exp. Med. 220, 20654. doi: 10.1084/jem.20220654
Janelidze, S., Mattsson, N., Stomrud, E., Lindberg, O., Palmqvist, S., Zetterberg, H., et al. (2018). CSF biomarkers of neuroinflammation and cerebrovascular dysfunction in early Alzheimer disease. Neurology 91, e867–e877. doi: 10.1212/WNL.0000000000006082
Jiao, M., Li, X., Chen, L., Wang, X., Yuan, B., Liu, T., et al. (2020). Neuroprotective effect of astrocyte-derived IL-33 in neonatal hypoxic-ischemic brain injury. J. Neuroinflammation. 17, 251. doi: 10.1186/s12974-020-01932-z
Johnson, G. V., and Jope, R. S. (1992). The role of microtubule-associated protein 2 (MAP-2) in neuronal growth, plasticity, and degeneration. J. Neurosci. Res. 33, 505–512. doi: 10.1002/jnr.490330402
Ju, R., Zhuang, Z. W., Zhang, J., Lanahan, A. A., Kyriakides, T., Sessa, W. C., et al. (2014). Angiopoietin-2 secretion by endothelial cell exosomes: regulation by the phosphatidylinositol 3-kinase (PI3K)/Akt/endothelial nitric oxide synthase (eNOS) and syndecan-4/syntenin pathways. J. Biol. Chem. 289, 510–519. doi: 10.1074/jbc.M113.506899
Kadkova, A., Radecke, J., and Sorensen, J. B. (2019). The SNAP-25 Protein Family. Neuroscience 420, 50–71. doi: 10.1016/j.neuroscience.2018.09.020
Kassianidis, G., Siampanos, A., Poulakou, G., Adamis, G., Rapti, A., Milionis, H., et al. (2022). Calprotectin and imbalances between acute-phase mediators are associated with critical illness in COVID-19. Int. J. Mol. Sci. 23, 4894. doi: 10.3390/ijms23094894
Kawakita, S., Mandal, K., Mou, L., Mecwan, M. M., Zhu, Y., Li, S., et al. (2022). Organ-on-a-chip models of the blood-brain barrier: recent advances and future prospects. Small 18, e2201401. doi: 10.1002/smll.202201401
Keller, G. S., Medeiros, E. B., Dos Santos, M. L. C., Lidio, A. V., Kucharska, E., and Budni, J. (2023). COVID-19 and Brain Aging: What are the Implications of Immunosenescence? Curr. Aging Sci. 16, 89–96. doi: 10.2174/1874609816666221228103320
Kempuraj, D., Aenlle, K. K., Cohen, J., Mathew, A., Isler, D., Pangeni, R. P., et al. (2024). COVID-19 and Long COVID: disruption of the neurovascular unit, blood-brain barrier, and tight junctions. Neuroscientist 30, 421–439. doi: 10.1177/10738584231194927
Kempuraj, D., Ahmed, M. E., Selvakumar, G. P., Thangavel, R., Dhaliwal, A. S., Dubova, I., et al. (2020a). Brain injury-mediated neuroinflammatory response and Alzheimer’s disease. Neuroscientist 26, 134–155. doi: 10.1177/1073858419848293
Kempuraj, D., Ahmed, M. E., Selvakumar, G. P., Thangavel, R., Raikwar, S. P., Zaheer, S. A., et al. (2020b). Psychological stress-induced immune response and risk of Alzheimer’s disease in veterans from operation enduring freedom and operation Iraqi freedom. Clin. Ther. 42, 974–982. doi: 10.1016/j.clinthera.2020.02.018
Kempuraj, D., Ahmed, M. E., Selvakumar, G. P., Thangavel, R., Raikwar, S. P., Zaheer, S. A., et al. (2021). Acute Traumatic Brain Injury-Induced Neuroinflammatory Response and Neurovascular Disorders in the Brain. Neurotox Res. 39, 359–368. doi: 10.1007/s12640-020-00288-9
Kempuraj, D., Mentor, S., Thangavel, R., Ahmed, M. E., Selvakumar, G. P., Raikwar, S. P., et al. (2019). Mast Cells in Stress, Pain, Blood-Brain Barrier, Neuroinflammation and Alzheimer’s Disease. Front. Cell Neurosci. 13, 54.
Kempuraj, D., Selvakumar, G. P., Thangavel, R., Ahmed, M. E., Zaheer, S., Kumar, K. K., et al. (2018). Glia maturation factor and mast cell-dependent expression of inflammatory mediators and proteinase activated receptor-2 in neuroinflammation. J Alzheimers Dis. doi: 10.3233/JAD-180786 [Epub ahead of print].
Kempuraj, D., Thangavel, R., Natteru, P. A., Selvakumar, G. P., Saeed, D., Zahoor, H., et al. (2016). Neuroinflammation Induces Neurodegeneration. J. Neurol. Neurosurg. Spine. 1:1003.
Kempuraj, D., Thangavel, R., Selvakumar, G. P., Zaheer, S., Ahmed, M. E., Raikwar, S. P., et al. (2017). Brain and Peripheral Atypical Inflammatory Mediators Potentiate Neuroinflammation and Neurodegeneration. Front. Cell Neurosci. 11:216. doi: 10.3389/fncel.2017.00216
Kenkhuis, B., Somarakis, A., Kleindouwel, L. R. T., van Roon-Mom, W. M. C., Hollt, T., and van der Weerd, L. (2022). Co-expression patterns of microglia markers Iba1, TMEM119 and P2RY12 in Alzheimer’s disease. Neurobiol. Dis. 167, 105684. doi: 10.1016/j.nbd.2022.105684
Khalil, M., Teunissen, C. E., Otto, M., Piehl, F., Sormani, M. P., Gattringer, T., et al. (2018). Neurofilaments as biomarkers in neurological disorders. Nat. Rev. Neurol. 14, 577–589. doi: 10.1038/s41582-018-0058-z
Kim, D. S., and Kim, G. W. (2024). Biofluid-based Biomarkers in Traumatic Brain Injury: A Narrative Review. Brain Neurorehabil. 17, e8. doi: 10.12786/bn.2024.17.e8
Kim, J. H., Afridi, R., Lee, W. H., and Suk, K. (2020). Proteomic examination of the neuroglial secretome: lessons for the clinic. Expert. Rev. Proteomics 17, 207–220. doi: 10.1080/14789450.2020.1745069
Kincses, A., Vigh, J. P., Petrovszki, D., Valkai, S., Kocsis, A. E., Walter, F. R., et al. (2023). The use of sensors in blood-brain barrier-on-a-chip devices: current practice and future directions. Biosensors 13, 357. doi: 10.3390/bios13030357
Kitchen, P., Salman, M. M., Halsey, A. M., Clarke-Bland, C., MacDonald, J. A., Ishida, H., et al. (2020). Targeting aquaporin-4 subcellular localization to treat central nervous system edema. Cell 181, e19. doi: 10.1016/j.cell.2020.03.037
Kontsekova, S., Polcicova, K., Takacova, M., and Pastorekova, S. (2016). Endosialin: molecular and functional links to tumor angiogenesis. Neoplasma 63, 183–192. doi: 10.4149/202_15090N474
Korczyn, A. D., and Grinberg, L. T. (2024). Is Alzheimer disease a disease? Nat. Rev. Neurol. 20, 245–251. doi: 10.1038/s41582-024-00940-4
Korf, J. M., Honarpisheh, P., Mohan, E. C., Banerjee, A., Blasco-Conesa, M. P., Honarpisheh, P., et al. (2022). CD11b(high) B cells increase after stroke and regulate microglia. J. Immunol. 209, 288–300. doi: 10.4049/jimmunol.2100884
Korkmaz, O. T., Ay, H., Aytan, N., Carreras, I., Kowall, N. W., Dedeoglu, A., et al. (2019). Vasoactive intestinal peptide decreases beta-amyloid accumulation and prevents brain atrophy in the 5xFAD mouse model of Alzheimer’s disease. J. Mol. Neurosci. 68, 389–396. doi: 10.1007/s12031-018-1226-8
Korkmaz, O. T., and Tuncel, N. (2018). Advantages of Vasoactive Intestinal Peptide for the Future Treatment of Parkinson’s Disease. Curr. Pharm. Des. 24, 4693–4701. doi: 10.2174/1381612825666190111150953
Kumar, A., Stoica, B. A., Loane, D. J., Yang, M., Abulwerdi, G., Khan, N., et al. (2017). Microglial-derived microparticles mediate neuroinflammation after traumatic brain injury. J. Neuroinflammation 14, 47. doi: 10.1186/s12974-017-0819-4
Kwon, H. S., and Koh, S. H. (2020). Neuroinflammation in neurodegenerative disorders: the roles of microglia and astrocytes. Transl. Neurodegener. 9, 42. doi: 10.1186/s40035-020-00221-2
Kyriatzis, G., Khrestchatisky, M., Ferhat, L., and Chatzaki, E. A. (2024). Neurotensin and Neurotensin Receptors in Stress-related Disorders: Pathophysiology & Novel Drug Targets. Curr. Neuropharmacol. 22, 916–934. doi: 10.2174/1570159X21666230803101629
Lapshina, K. V., and Ekimova, I. V. (2024). Aquaporin-4 and Parkinson’s Disease. Int. J. Mol. Sci. 25, 672. doi: 10.3390/ijms25031672
Le Thuc, O., Blondeau, N., Nahon, J. L., and Rovere, C. (2015). The complex contribution of chemokines to neuroinflammation: switching from beneficial to detrimental effects. Ann. N. Y. Acad. Sci. 1351, 127–140. doi: 10.1111/nyas.12855
Lee, J. S., Sato, W., and Son, C. G. (2024). Brain-regional characteristics and neuroinflammation in ME/CFS patients from neuroimaging: A systematic review and meta-analysis. Autoimmun. Rev. 23, 103484. doi: 10.1016/j.autrev.2023.103484
Li, W., Chen, Z., Chin, I., Chen, Z., and Dai, H. (2018). The Role of VE-cadherin in Blood-brain Barrier Integrity Under Central Nervous System Pathological Conditions. Curr. Neuropharmacol. 16, 1375–1384. doi: 10.2174/1570159X16666180222164809
Li, X., Yan, B., Du, J., Xu, S., Liu, L., Pan, C., et al. (2022). Recent Advances in Progresses and Prospects of IL-37 in Central Nervous System Diseases. Brain Sci. 12, 723. doi: 10.3390/brainsci12060723
Liao, V., Cornman, H. L., Ma, E., and Kwatra, S. G. (2024). Prurigo nodularis: new insights into pathogenesis and novel therapeutics. Br. J. Dermatol. 190, 798–810. doi: 10.1093/bjd/ljae052
Lim, K. H., Yang, S., Kim, S. H., and Joo, J. Y. (2021). Identifying New COVID-19 Receptor Neuropilin-1 in Severe Alzheimer’s disease patients group brain using genome-wide association study approach. Front. Genet. 12:741175. doi: 10.3389/fgene.2021.741175
Lima Giacobbo, B., Doorduin, J., Klein, H. C., Dierckx, R., Bromberg, E., and de Vries, E. F. J. (2019). Brain-derived neurotrophic factor in brain disorders: focus on neuroinflammation. Mol. Neurobiol. 56, 3295–3312. doi: 10.1007/s12035-018-1283-6
Lin, E. Y., Xi, W., Aggarwal, N., and Shinohara, M. L. (2023). Osteopontin (OPN)/SPP1: from its biochemistry to biological functions in the innate immune system and the central nervous system (CNS). Int. Immunol. 35, 171–180. doi: 10.1093/intimm/dxac060
Lind-Holm Mogensen, F., Scafidi, A., Poli, A., and Michelucci, A. (2023). PARK7/DJ-1 in microglia: implications in Parkinson’s disease and relevance as a therapeutic target. J. Neuroinflammation 20, 95. doi: 10.1186/s12974-023-02776-z
Lista, S., and Hampel, H. (2017). Synaptic degeneration and neurogranin in the pathophysiology of Alzheimer’s disease. Expert. Rev. Neurother. 17, 47–57. doi: 10.1080/14737175.2016.1204234
Liu, F., Li, H., Hong, X., Liu, Y., and Yu, Z. (2024). Research progress of neuron-specific enolase in cognitive disorder: a mini review. Front. Hum. Neurosci. 18:1392519. doi: 10.3389/fnhum.2024.1392519
Lonnemann, N., Hosseini, S., Ohm, M., Geffers, R., Hiller, K., Dinarello, C. A., et al. (2022). IL-37 expression reduces acute and chronic neuroinflammation and rescues cognitive impairment in an Alzheimer’s disease mouse model. Elife 11, 75889. doi: 10.7554/eLife.75889
Lotankar, S., Prabhavalkar, K. S., and Bhatt, L. K. (2017). Biomarkers for Parkinson’s Disease: Recent Advancement. Neurosci. Bull. 33, 585–597. doi: 10.1007/s12264-017-0183-5
MacFadyen, J. R., Haworth, O., Roberston, D., Hardie, D., Webster, M. T., Morris, H. R., et al. (2005). Endosialin (TEM1, CD248) is a marker of stromal fibroblasts and is not selectively expressed on tumour endothelium. FEBS Lett. 579, 2569–2575. doi: 10.1016/j.febslet.2005.03.071
Malhotra, A. K., Ide, K., Salaheen, Z., Mahood, Q., Cunningham, J., Hutchison, J., et al. (2024). Acute fluid biomarkers for diagnosis and prognosis in children with mild traumatic brain injury: a systematic review. Mol. Diagn. Ther. 28, 169–187. doi: 10.1007/s40291-023-00685-8
Malhotra, S., Miras, M. C. M., Pappolla, A., Montalban, X., and Comabella, M. (2023). Liquid biopsy in neurological diseases. Cells 12, 1911. doi: 10.3390/cells12141911
Martin, L., Bouvet, P., Chounlamountri, N., Watrin, C., Besancon, R., Pinatel, D., et al. (2021). VEGF counteracts amyloid-beta-induced synaptic dysfunction. Cell Rep. 35, 109121. doi: 10.1016/j.celrep.2021.109121
Mary, A., Mancuso, R., and Heneka, M. T. (2024). Immune Activation in Alzheimer Disease. Annu. Rev. Immunol. 42, 585–613. doi: 10.1146/annurev-immunol-101921-035222
Mashaghi, A., Marmalidou, A., Tehrani, M., Grace, P. M., Pothoulakis, C., and Dana, R. (2016). Neuropeptide substance P and the immune response. Cell Mol. Life Sci. 73, 4249–4264. doi: 10.1007/s00018-016-2293-z
Matteoli, M. (2024). The role of microglial TREM2 in development: A path toward neurodegeneration? Glia 72, 1544–1554. doi: 10.1002/glia.24574
Mayer, M. G., and Fischer, T. (2024). Microglia at the blood brain barrier in health and disease. Front. Cell Neurosci. 18:1360195. doi: 10.3389/fncel.2024.1360195
McMahon, P. J., Panczykowski, D. M., Yue, J. K., Puccio, A. M., Inoue, T., Sorani, M. D., et al. (2015). Measurement of the glial fibrillary acidic protein and its breakdown products GFAP-BDP biomarker for the detection of traumatic brain injury compared to computed tomography and magnetic resonance imaging. J. Neurotrauma 32, 527–533. doi: 10.1089/neu.2014.3635
Mehta, R. I., and Mehta, R. I. (2023). The Vascular-Immune Hypothesis of Alzheimer’s Disease. Biomedicines 11, 408. doi: 10.3390/biomedicines11020408
Mi, Z., and Graham, S. H. (2023). Role of UCHL1 in the pathogenesis of neurodegenerative diseases and brain injury. Ageing Res. Rev. 86, 101856. doi: 10.1016/j.arr.2023.101856
Michael, B. D., Bricio-Moreno, L., Sorensen, E. W., Miyabe, Y., Lian, J., Solomon, T., et al. (2020). Astrocyte- and Neuron-Derived CXCL1 drives neutrophil transmigration and blood-brain barrier permeability in viral encephalitis. Cell Rep. 32, 108150. doi: 10.1016/j.celrep.2020.108150
Mina-Osorio, P., Winnicka, B., O’Conor, C., Grant, C. L., Vogel, L. K., Rodriguez-Pinto, D., et al. (2008). CD13 is a novel mediator of monocytic/endothelial cell adhesion. J. Leukoc. Biol. 84, 448–459. doi: 10.1189/jlb.1107802
Mira, R. G., Lira, M., and Cerpa, W. (2021). Traumatic brain injury: mechanisms of glial response. Front. Physiol. 12:740939. doi: 10.3389/fphys.2021.740939
Morales-Medina, J. C., Dumont, Y., and Quirion, R. (2010). A possible role of neuropeptide Y in depression and stress. Brain Res. 1314, 194–205. doi: 10.1016/j.brainres.2009.09.077
Morell, M., Souza-Moreira, L., and Gonzalez-Rey, E. (2012). VIP in neurological diseases: more than a neuropeptide. Endocr. Metab. Immune Disord. Drug Targets 12, 323–332. doi: 10.2174/187153012803832549
Morris, H. R., Spillantini, M. G., Sue, C. M., and Williams-Gray, C. H. (2024). The pathogenesis of Parkinson’s disease. Lancet 403, 293–304. doi: 10.1016/S0140-6736(23)01478-2
Mosley, R. L., Lu, Y., Olson, K. E., Machhi, J., Yan, W., Namminga, K. L., et al. (2019). A synthetic agonist to vasoactive intestinal peptide receptor-2 induces regulatory T cell neuroprotective activities in models of Parkinson’s disease. Front. Cell Neurosci. 13:421. doi: 10.3389/fncel.2019.00421
Mullard, A. (2023). NfL makes regulatory debut as neurodegenerative disease biomarker. Nat. Rev. Drug Discov. 22, 431–434. doi: 10.1038/d41573-023-00083-z
Munoz Pareja, J. C., de Rivero Vaccari, J. P., Chavez, M. M., Kerrigan, M., Pringle, C., Guthrie, K., et al. (2024). Prognostic and diagnostic utility of serum biomarkers in pediatric traumatic brain injury. J. Neurotrauma 41, 106–122. doi: 10.1089/neu.2023.0039
Musafargani, S., Mishra, S., Gulyas, M., Mahalakshmi, P., Archunan, G., Padmanabhan, P., et al. (2020). Blood brain barrier: A tissue engineered microfluidic chip. J. Neurosci. Methods 331, 108525. doi: 10.1016/j.jneumeth.2019.108525
Nabizadeh, F., Zafari, R., Alzheimer’s, and disease Neuroimaging, I. (2024). Progranulin and neuropathological features of Alzheimer’s disease: longitudinal study. Aging Clin. Exp. Res. 36, 55. doi: 10.1007/s40520-024-02715-9
Nagatsu, T., Nakashima, A., Ichinose, H., and Kobayashi, K. (2019). Human tyrosine hydroxylase in Parkinson’s disease and in related disorders. J. Neural Transm. 126, 397–409. doi: 10.1007/s00702-018-1903-3
Nagelhus, E. A., and Ottersen, O. P. (2013). Physiological roles of aquaporin-4 in brain. Physiol. Rev. 93, 1543–1562. doi: 10.1152/physrev.00011.2013
Nair, A. L., Groenendijk, L., Overdevest, R., Fowke, T. M., Annida, R., Mocellin, O., et al. (2023). Human BBB-on-a-chip reveals barrier disruption, endothelial inflammation, and T cell migration under neuroinflammatory conditions. Front. Mol. Neurosci. 16:1250123. doi: 10.3389/fnmol.2023.1250123
Negi, S., Khurana, N., and Duggal, N. (2024). The misfolding mystery: alpha-synuclein and the pathogenesis of Parkinson’s disease. Neurochem. Int. 177, 105760. doi: 10.1016/j.neuint.2024.105760
Nguyen, A. D., Malmstrom, T. K., Aggarwal, G., Miller, D. K., Vellas, B., and Morley, J. E. (2022). Serum neurofilament light levels are predictive of all-cause mortality in late middle-aged individuals. EBioMedicine. 82, 104146. doi: 10.1016/j.ebiom.2022.104146
Noor, A., and Zahid, S. (2017). A review of the role of synaptosomal-associated protein 25 (SNAP-25) in neurological disorders. Int. J. Neurosci. 127, 805–811. doi: 10.1080/00207454.2016.1248240
Norden, D. M., Trojanowski, P. J., Villanueva, E., Navarro, E., and Godbout, J. P. (2016). Sequential activation of microglia and astrocyte cytokine expression precedes increased Iba-1 or GFAP immunoreactivity following systemic immune challenge. Glia 64, 300–316. doi: 10.1002/glia.22930
O’Callaghan, J. P., and Miller, D. B. (2019). Neuroinflammation disorders exacerbated by environmental stressors. Metabolism 100S, 153951. doi: 10.1016/j.metabol.2019.153951
Ohbuchi, M., Shibuta, M., Tetsuka, K., Sasaki-Iwaoka, H., Oishi, M., Shimizu, F., et al. (2024). Modeling of Blood-Brain Barrier (BBB) dysfunction and immune cell migration using human BBB-on-a-chip for drug discovery research. Int. J. Mol. Sci. 25, 6496. doi: 10.3390/ijms25126496
Okonkwo, D. O., Yue, J. K., Puccio, A. M., Panczykowski, D. M., Inoue, T., McMahon, P. J., et al. (2013). GFAP-BDP as an acute diagnostic marker in traumatic brain injury: results from the prospective transforming research and clinical knowledge in traumatic brain injury study. J. Neurotrauma 30, 1490–1497. doi: 10.1089/neu.2013.2883
Ollen-Bittle, N., Roseborough, A. D., Wang, W., Wu, J. D., and Whitehead, S. N. (2022). Mechanisms and Biomarker Potential of Extracellular Vesicles in Stroke. Biology 11, 1231. doi: 10.3390/biology11081231
Ots, H. D., Tracz, J. A., Vinokuroff, K. E., and Musto, A. E. (2022). CD40-CD40L in Neurological Disease. Int. J. Mol. Sci. 23, 4115. doi: 10.3390/ijms23084115
Owens, C. D., Bonin Pinto, C., Detwiler, S., Olay, L., Pinaffi-Langley, A., Mukli, P., et al. (2024). Neurovascular coupling impairment as a mechanism for cognitive deficits in COVID-19. Brain Commun. 6, fcae080. doi: 10.1093/braincomms/fcae080
Palma-Florez, S., Lopez-Canosa, A., Moralez-Zavala, F., Castano, O., Kogan, M. J., Samitier, J., et al. (2023). BBB-on-a-chip with integrated micro-TEER for permeability evaluation of multi-functionalized gold nanorods against Alzheimer’s disease. J. Nanobiotechnology 21, 115. doi: 10.1186/s12951-023-01798-2
Papadopoulos, M. C., and Verkman, A. S. (2007). Aquaporin-4 and brain edema. Pediatr. Nephrol. 22, 778–784. doi: 10.1007/s00467-006-0411-0
Pathak, N., Vimal, S. K., Tandon, I., Agrawal, L., Hongyi, C., and Bhattacharyya, S. (2022). Neurodegenerative disorders of alzheimer, parkinsonism, amyotrophic lateral sclerosis and multiple sclerosis: an early diagnostic approach for precision treatment. Metab. Brain Dis. 37, 67–104. doi: 10.1007/s11011-021-00800-w
Patten, J., and Wang, K. (2021). Fibronectin in development and wound healing. Adv. Drug Deliv. Rev. 170, 353–368. doi: 10.1016/j.addr.2020.09.005
Peng, B., Hao, S., Tong, Z., Bai, H., Pan, S., Lim, K. L., et al. (2022). Blood-brain barrier (BBB)-on-a-chip: a promising breakthrough in brain disease research. Lab. Chip. 22, 3579–3602. doi: 10.1039/d2lc00305h
Pocock, J., Vasilopoulou, F., Svensson, E., and Cosker, K. (2024). Microglia and TREM2. Neuropharmacology 257, 110020. doi: 10.1016/j.neuropharm.2024.110020
Praschberger, R., Kuenen, S., Schoovaerts, N., Kaempf, N., Singh, J., Janssens, J., et al. (2023). Neuronal identity defines alpha-synuclein and tau toxicity. Neuron 111, e11. doi: 10.1016/j.neuron.2023.02.033
Ramani, S., Berard, J. A., and Walker, L. A. S. (2021). The relationship between neurofilament light chain and cognition in neurological disorders: A scoping review. J. Neurol. Sci. 420, 117229. doi: 10.1016/j.jns.2020.117229
Rangaraju, S., Raza, S. A., Li, N. X., Betarbet, R., Dammer, E. B., Duong, D., et al. (2018). Differential Phagocytic Properties of CD45(low) Microglia and CD45(high) Brain Mononuclear Phagocytes-Activation and Age-Related Effects. Front. Immunol. 9:405. doi: 10.3389/fimmu.2018.00405
Rao, X., Hua, F., Zhang, L., Lin, Y., Fang, P., Chen, S., et al. (2022). Dual roles of interleukin-33 in cognitive function by regulating central nervous system inflammation. J. Transl. Med. 20, 369. doi: 10.1186/s12967-022-03570-w
Rauf, A., Badoni, H., Abu-Izneid, T., Olatunde, A., Rahman, M. M., Painuli, S., et al. (2022). Neuroinflammatory Markers: Key Indicators in the Pathology of Neurodegenerative Diseases. Molecules 27, 3194. doi: 10.3390/molecules27103194
Reissner, C., Runkel, F., and Missler, M. (2013). Neurexins. Genome Biol. 14, 213. doi: 10.1186/gb-2013-14-9-213
Rempe, R. G., Hartz, A. M., and Bauer, B. (2016). Matrix metalloproteinases in the brain and blood-brain barrier: versatile breakers and makers. J. Cereb. Blood Flow Metab. 36, 1481–14507. doi: 10.1177/0271678X16655551
Rentsendorj, A., Sheyn, J., Fuchs, D. T., Daley, D., Salumbides, B. C., Schubloom, H. E., et al. (2018). A novel role for osteopontin in macrophage-mediated amyloid-beta clearance in Alzheimer’s models. Brain Behav. Immun. 67, 163–180. doi: 10.1016/j.bbi.2017.08.019
Repici, M., and Giorgini, F. (2019). DJ-1 in Parkinson’s disease: clinical insights and therapeutic perspectives. J. Clin. Med. 8, 377. doi: 10.3390/jcm8091377
Requena-Ocana, N., Flores-Lopez, M., Papaseit, E., Garcia-Marchena, N., Ruiz, J. J., Ortega-Pinazo, J., et al. (2022). Vascular endothelial growth factor as a potential biomarker of neuroinflammation and frontal cognitive impairment in patients with alcohol use disorder. Biomedicines. 10, 947. doi: 10.3390/biomedicines10050947
Reverchon, F., de Concini, V., Larrigaldie, V., Benmerzoug, S., Briault, S., Togbe, D., et al. (2020). Hippocampal interleukin-33 mediates neuroinflammation-induced cognitive impairments. J. Neuroinflammation 17, 268. doi: 10.1186/s12974-020-01939-6
Rho, S. S., Ando, K., and Fukuhara, S. (2017). Dynamic regulation of vascular permeability by vascular endothelial cadherin-mediated endothelial cell-cell junctions. J. Nippon Med. Sch. 84, 148–159. doi: 10.1272/jnms.84.148
Rigo, Y. R., Benvenutti, R., Portela, L. V., and Strogulski, N. R. (2024). Neurogenic potential of NG2 in neurotrauma: a systematic review. Neural Regen. Res. 19, 2673–2683. doi: 10.4103/NRR.NRR-D-23-01031
Robinson, C. R., Zhang, H., and Dougherty, P. M. (2014). Astrocytes, but not microglia, are activated in oxaliplatin and bortezomib-induced peripheral neuropathy in the rat. Neuroscience. 274, 308–317. doi: 10.1016/j.neuroscience.2014.05.051
Rocco, M. L., Soligo, M., Manni, L., and Aloe, L. (2018). Nerve Growth Factor: Early Studies and Recent Clinical Trials. Curr. Neuropharmacol. 16, 1455–1465. doi: 10.2174/1570159X16666180412092859
Rochfort, K. D., and Cummins, P. M. (2015). Cytokine-mediated dysregulation of zonula occludens-1 properties in human brain microvascular endothelium. Microvasc. Res. 100, 48–53. doi: 10.1016/j.mvr.2015.04.010
Rosmus, D. D., Lange, C., Ludwig, F., Ajami, B., and Wieghofer, P. (2022). The Role of Osteopontin in Microglia Biology: Current Concepts and Future Perspectives. Biomedicines 10, 840. doi: 10.3390/biomedicines10040840
Rothermundt, M., Peters, M., Prehn, J. H., and Arolt, V. (2003). S100B in brain damage and neurodegeneration. Microsc. Res. Tech. 60, 614–632. doi: 10.1002/jemt.10303
Ruan, C., and Elyaman, W. (2022). A New Understanding of TMEM119 as a Marker of Microglia. Front. Cell Neurosci. 16:902372. doi: 10.3389/fncel.2022.902372
Safwat, A., Helmy, A., and Gupta, A. (2023). The Role of Substance P Within Traumatic Brain Injury and Implications for Therapy. J. Neurotrauma 40, 1567–1583. doi: 10.1089/neu.2022.0510
Satoh, J., Kino, Y., Asahina, N., Takitani, M., Miyoshi, J., Ishida, T., et al. (2016). TMEM119 marks a subset of microglia in the human brain. Neuropathology 36, 39–49. doi: 10.1111/neup.12235
Schiera, G., Di Liegro, C. M., Schiro, G., Sorbello, G., and Di Liegro, I. (2024). Involvement of Astrocytes in the Formation, Maintenance, and Function of the Blood-Brain Barrier. Cells 13, 150. doi: 10.3390/cells13020150
Scholz, A., Plate, K. H., and Reiss, Y. (2015). Angiopoietin-2: a multifaceted cytokine that functions in both angiogenesis and inflammation. Ann. N. Y. Acad. Sci. 1347, 45–51. doi: 10.1111/nyas.12726
Selvakumar, G. P., Ahmed, M. E., Iyer, S. S., Thangavel, R., Kempuraj, D., Raikwar, S. P., et al. (2020a). Absence of glia maturation factor protects from axonal injury and motor behavioral impairments after traumatic brain injury. Exp. Neurobiol. 29, 230–248. doi: 10.5607/en20017
Selvakumar, G. P., Ahmed, M. E., Thangavel, R., Kempuraj, D., Dubova, I., Raikwar, S. P., et al. (2020b). A role for glia maturation factor dependent activation of mast cells and microglia in MPTP induced dopamine loss and behavioural deficits in mice. Brain Behav. Immun. doi: 10.1016/j.bbi.2020.01.013 [Epub ahead of print].
Shabani, F., Farasat, A., Mahdavi, M., and Gheibi, N. (2018). Calprotectin (S100A8/S100A9): a key protein between inflammation and cancer. Inflamm. Res. 67, 801–812. doi: 10.1007/s00011-018-1173-4
Shahidehpour, R. K., Higdon, R. E., Crawford, N. G., Neltner, J. H., Ighodaro, E. T., Patel, E., et al. (2021). Dystrophic microglia are associated with neurodegenerative disease and not healthy aging in the human brain. Neurobiol. Aging 99, 19–27. doi: 10.1016/j.neurobiolaging.2020.12.003
Shahim, P., Pham, D. L., van der Merwe, A. J., Moore, B., Chou, Y. Y., Lippa, S. M., et al. (2024). Serum NfL and GFAP as biomarkers of progressive neurodegeneration in TBI. Alzheimers Dement. 20, 4663–4676. doi: 10.1002/alz.13898
Shahim, P., Politis, A., van der Merwe, A., Moore, B., Chou, Y. Y., Pham, D. L., et al. (2020). Neurofilament light as a biomarker in traumatic brain injury. Neurology. 95, e610–e622. doi: 10.1212/WNL.0000000000009983
Sharma, C., Woo, H., and Kim, S. R. (2022). Addressing blood-brain barrier impairment in Alzheimer’s Disease. Biomedicines 10, 40742. doi: 10.3390/biomedicines10040742
Shende, P., and Desai, D. (2020). Physiological and therapeutic roles of neuropeptide Y on biological functions. Adv. Exp. Med. Biol. 1237, 37–47. doi: 10.1007/5584_2019_427
Shepherd, C. E., Goyette, J., Utter, V., Rahimi, F., Yang, Z., Geczy, C. L., et al. (2006). Inflammatory S100A9 and S100A12 proteins in Alzheimer’s disease. Neurobiol. Aging 27, 1554–1563. doi: 10.1016/j.neurobiolaging.2005.09.033
Shi, Q., Gutierrez, R. A., and Bhat, M. A. (2024). Microglia, Trem2, and Neurodegeneration. Neuroscientist doi: 10.1177/10738584241254118 [Epub ahead of print].
Shi, W., Jiang, D., Rando, H., Khanduja, S., Lin, Z., Hazel, K., et al. (2023). Blood-brain barrier breakdown in COVID-19 ICU survivors: an MRI pilot study. NeuroImmune Pharm. Ther. 2, 333–338. doi: 10.1515/nipt-2023-0018
Silvestro, S., Raffaele, I., Quartarone, A., and Mazzon, E. (2024). Innovative insights into traumatic brain injuries: biomarkers and new pharmacological targets. Int. J. Mol. Sci. 25, 2372. doi: 10.3390/ijms25042372
Sims, S. K., Wilken-Resman, B., Smith, C. J., Mitchell, A., McGonegal, L., and Sims-Robinson, C. (2022). Brain-derived neurotrophic factor and nerve growth factor therapeutics for brain injury: the current translational challenges in preclinical and clinical research. Neural Plast. 2022, 3889300. doi: 10.1155/2022/3889300
Sindi, I. A., Tannenberg, R. K., and Dodd, P. R. (2014). Role for the neurexin-neuroligin complex in Alzheimer’s disease. Neurobiol. Aging 35, 746–756. doi: 10.1016/j.neurobiolaging.2013.09.032
Song, P., and Krainc, D. (2024). Diverse functions of parkin in midbrain dopaminergic neurons. Mov. Disord. doi: 10.1002/mds.29890
Staicu, C. E., Jipa, F., Axente, E., Radu, M., Radu, B. M., and Sima, F. (2021). Lab-on-a-Chip Platforms as Tools for Drug Screening in Neuropathologies Associated with Blood-Brain Barrier Alterations. Biomolecules 11, 916. doi: 10.3390/biom11060916
Stogsdill, J. A., Ramirez, J., Liu, D., Kim, Y. H., Baldwin, K. T., Enustun, E., et al. (2017). Astrocytic neuroligins control astrocyte morphogenesis and synaptogenesis. Nature 551, 192–197. doi: 10.1038/nature24638
Su, W., Aloi, M. S., and Garden, G. A. (2016). MicroRNAs mediating CNS inflammation: Small regulators with powerful potential. Brain Behav. Immun. 52, 1–8. doi: 10.1016/j.bbi.2015.07.003
Sudhof, T. C. (2008). Neuroligins and neurexins link synaptic function to cognitive disease. Nature 455, 903–911. doi: 10.1038/nature07456
Sun, M., Baker, T. L., Wilson, C. T., Brady, R. D., Mychasiuk, R., Yamakawa, G. R., et al. (2022). Treatment with vascular endothelial growth factor-A worsens cognitive recovery in a rat model of mild traumatic brain injury. Front. Mol. Neurosci. 15:937350. doi: 10.3389/fnmol.2022.937350
Sun, M., Baker, T. L., Wilson, C. T., Brady, R. D., Yamakawa, G. R., Wright, D. K., et al. (2024). Treatment with the vascular endothelial growth factor-A antibody, bevacizumab, has sex-specific effects in a rat model of mild traumatic brain injury. J. Cereb. Blood Flow Metab. 44, 542–555. doi: 10.1177/0271678X231212377
Swanson, M. E. V., Mrkela, M., Murray, H. C., Cao, M. C., Turner, C., Curtis, M. A., et al. (2023). Microglial CD68 and L-ferritin upregulation in response to phosphorylated-TDP-43 pathology in the amyotrophic lateral sclerosis brain. Acta Neuropathol. Commun. 11, 69. doi: 10.1186/s40478-023-01561-6
Tachibana, K., Hirayama, R., Sato, N., Hattori, K., Kato, T., Takeda, H., et al. (2024). Association of Plasma Claudin-5 with Age and Alzheimer Disease. Int. J. Mol. Sci. 25, 1419. doi: 10.3390/ijms25031419
Tan, Y. J., Siow, I., Saffari, S. E., Ting, S. K. S., Li, Z., Kandiah, N., et al. (2023). Plasma Soluble ST2 levels are higher in neurodegenerative disorders and associated with poorer cognition. J. Alzheimers Dis. 92, 573–580. doi: 10.3233/JAD-221072
Taracanova, A., Tsilioni, I., Conti, P., Norwitz, E. R., Leeman, S. E., and Theoharides, T. C. (2018). Substance P and IL-33 administered together stimulate a marked secretion of IL-1beta from human mast cells, inhibited by methoxyluteolin. Proc. Natl. Acad. Sci. U. S. A. 115, E9381–E9390. doi: 10.1073/pnas.1810133115
Tarawneh, R., Kasper, R. S., Sanford, J., Phuah, C. L., Hassenstab, J., and Cruchaga, C. (2022). Vascular endothelial-cadherin as a marker of endothelial injury in preclinical Alzheimer disease. Ann. Clin. Transl. Neurol. 9, 1926–1940. doi: 10.1002/acn3.51685
Teunissen, C. E., Verberk, I. M. W., Thijssen, E. H., Vermunt, L., Hansson, O., Zetterberg, H., et al. (2022). Blood-based biomarkers for Alzheimer’s disease: towards clinical implementation. Lancet Neurol. 21, 66–77. doi: 10.1016/S1474-4422(21)00361-6
Thangavel, R., Kaur, H., Dubova, I., Selvakumar, G. P., Ahmed, M. E., Raikwar, S. P., et al. (2024). Parkinson’s disease dementia patients: expression of glia maturation factor in the brain. Int. J. Mol. Sci. 25, 1182. doi: 10.3390/ijms25021182
Thangavel, R., Kempuraj, D., Zaheer, S., Raikwar, S., Ahmed, M. E., Selvakumar, G. P., et al. (2017). Glia Maturation factor and mitochondrial uncoupling proteins 2 and 4 expression in the temporal cortex of Alzheimer’s disease brain. Front. Aging Neurosci. 9:150. doi: 10.3389/fnagi.2017.00150
Thangavel, R., Stolmeier, D., Yang, X., Anantharam, P., and Zaheer, A. (2012). Expression of glia maturation factor in neuropathological lesions of Alzheimer’s disease. Neuropathol. Appl. Neurobiol. 38, 572–581. doi: 10.1111/j.1365-2990.2011.01232.x
Theofilis, P., Sagris, M., Oikonomou, E., Antonopoulos, A. S., Siasos, G., Tsioufis, C., et al. (2021). Inflammatory mechanisms contributing to endothelial dysfunction. Biomedicines 9, 70781. doi: 10.3390/biomedicines9070781
Theoharides, T. C., and Kempuraj, D. (2023). Role of SARS-CoV-2 spike-protein-induced activation of microglia and mast cells in the pathogenesis of neuro-COVID. Cells 12, 688. doi: 10.3390/cells12050688
Theoharides, T. C., Stewart, J. M., Panagiotidou, S., and Melamed, I. (2016). Mast cells, brain inflammation and autism. Eur. J. Pharmacol. 778, 96–102. doi: 10.1016/j.ejphar.2015.03.086
Thorsell, A. (2010). Brain neuropeptide Y and corticotropin-releasing hormone in mediating stress and anxiety. Exp. Biol. Med. 235, 1163–1167. doi: 10.1258/ebm.2010.009331
Togawa, N., Ayaki, T., Yoshii, D., Maki, T., Sawamoto, N., and Takahashi, R. (2024). TMEM119-positive microglia were increased in the brains of patients with amyotrophic lateral sclerosis. Neurosci. Lett. 833, 137829. doi: 10.1016/j.neulet.2024.137829
Togo, T., Akiyama, H., Kondo, H., Ikeda, K., Kato, M., Iseki, E., et al. (2000). Expression of CD40 in the brain of Alzheimer’s disease and other neurological diseases. Brain Res. 885, 117–121. doi: 10.1016/s0006-8993(00)02984-x
Tomkowicz, B., Rybinski, K., Sebeck, D., Sass, P., Nicolaides, N. C., Grasso, L., et al. (2010). Endosialin/TEM-1/CD248 regulates pericyte proliferation through PDGF receptor signaling. Cancer Biol Ther. 9, 908–915. doi: 10.4161/cbt.9.11.11731
Tran, V. T. A., Lee, L. P., and Cho, H. (2022). Neuroinflammation in neurodegeneration via microbial infections. Front. Immunol. 13:907804. doi: 10.3389/fimmu.2022.907804
Tsilioni, I., Pantazopoulos, H., Conti, P., Leeman, S. E., and Theoharides, T. C. (2020). IL-38 inhibits microglial inflammatory mediators and is decreased in amygdala of children with autism spectrum disorder. Proc. Natl. Acad. Sci. U. S. A. 117, 16475–16480. doi: 10.1073/pnas.2004666117
Tsilioni, I., Patel, A. B., Pantazopoulos, H., Berretta, S., Conti, P., Leeman, S. E., et al. (2019). IL-37 is increased in brains of children with autism spectrum disorder and inhibits human microglia stimulated by neurotensin. Proc. Natl. Acad. Sci. U. S. A. 116, 21659–21665. doi: 10.1073/pnas.1906817116
Tyagi, K., Rai, P., Gautam, A., Kaur, H., Kapoor, S., Suttee, A., et al. (2023). Neurological manifestations of SARS-CoV-2: complexity, mechanism and associated disorders. Eur. J. Med. Res. 28, 307. doi: 10.1186/s40001-023-01293-2
Tziolos, N. R., Ioannou, P., Baliou, S., and Kofteridis, D. P. (2023). Long COVID-19 Pathophysiology: What Do We Know So Far? Microorganisms 1, 102458. doi: 10.3390/microorganisms11102458
Urrestizala-Arenaza, N., Cerchio, S., Cavaliere, F., and Magliaro, C. (2024). Limitations of human brain organoids to study neurodegenerative diseases: a manual to survive. Front. Cell Neurosci. 18:1419526. doi: 10.3389/fncel.2024.1419526
Vafadari, B., Salamian, A., and Kaczmarek, L. (2016). MMP-9 in translation: from molecule to brain physiology, pathology, and therapy. J. Neurochem. 139, 91–114. doi: 10.1111/jnc.13415
Vainchtein, I. D., Chin, G., Cho, F. S., Kelley, K. W., Miller, J. G., Chien, E. C., et al. (2018). Astrocyte-derived interleukin-33 promotes microglial synapse engulfment and neural circuit development. Science 359, 1269–1273. doi: 10.1126/science.aal3589
van de Veerdonk, F. L., de Graaf, D. M., Joosten, L. A., and Dinarello, C. A. (2018). Biology of IL-38 and its role in disease. Immunol. Rev. 281, 191–196. doi: 10.1111/imr.12612
Van Der Naalt, J. (2015). Resting functional imaging tools (MRS, SPECT, PET and PCT). Handb. Clin. Neurol. 127, 295–308. doi: 10.1016/B978-0-444-52892-6.00019-2
Van Hulle, C., Ince, S., Okonkwo, O. C., Bendlin, B. B., Johnson, S. C., Carlsson, C. M., et al. (2024). Elevated CSF angiopoietin-2 correlates with blood-brain barrier leakiness and markers of neuronal injury in early Alzheimer’s disease. Transl. Psychiatry 14, 3. doi: 10.1038/s41398-023-02706-w
Varesi, A., Carrara, A., Pires, V. G., Floris, V., Pierella, E., Savioli, G., et al. (2022). Blood-Based Biomarkers for Alzheimer’s Disease Diagnosis and Progression: An Overview. Cells 11, 1081367. doi: 10.3390/cells11081367
Varillas-Delgado, D., Jimenez-Antona, C., Lizcano-Alvarez, A., Cano-de-la-Cuerda, R., Molero-Sanchez, A., and Laguarta-Val, S. (2023). Predictive Factors and ACE-2 Gene Polymorphisms in Susceptibility to Long COVID-19 Syndrome. Int. J. Mol. Sci. 24, 16717. doi: 10.3390/ijms242316717
Vay, S. U., Olschewski, D. N., Petereit, H., Lange, F., Nazarzadeh, N., Gross, E., et al. (2021). Osteopontin regulates proliferation, migration, and survival of astrocytes depending on their activation phenotype. J. Neurosci. Res. 99, 2822–2843. doi: 10.1002/jnr.24954
Wakabayashi, K., Tanji, K., Odagiri, S., Miki, Y., Mori, F., and Takahashi, H. (2013). The Lewy body in Parkinson’s disease and related neurodegenerative disorders. Mol. Neurobiol. 47, 495–508. doi: 10.1007/s12035-012-8280-y
Wakayama, E., Kuzu, T., Tachibana, K., Hirayama, R., Okada, Y., and Kondoh, M. (2022). Modifying the blood-brain barrier by targeting claudin-5: Safety and risks. Ann. N. Y. Acad. Sci. 1514, 62–69. doi: 10.1111/nyas.14787
Waller, R., Baxter, L., Fillingham, D. J., Coelho, S., Pozo, J. M., Mozumder, M., et al. (2019). Iba-1-/CD68+ microglia are a prominent feature of age-associated deep subcortical white matter lesions. PLoS One 14:e0210888. doi: 10.1371/journal.pone.0210888
Wang, A., and He, B. P. (2009). Characteristics and functions of NG2 cells in normal brain and neuropathology. Neurol. Res. 31, 144–150. doi: 10.1179/174313209X393555
Wang, K. K., Munoz Pareja, J. C., Mondello, S., Diaz-Arrastia, R., Wellington, C., Kenney, K., et al. (2021a). Blood-based traumatic brain injury biomarkers - Clinical utilities and regulatory pathways in the United States, Europe and Canada. Expert. Rev. Mol. Diagn. 21, 1303–1321. doi: 10.1080/14737159.2021.2005583
Wang, X. M., Zeng, P., Fang, Y. Y., Zhang, T., and Tian, Q. (2021b). Progranulin in neurodegenerative dementia. J. Neurochem. 158, 119–137. doi: 10.1111/jnc.15378
Wasik, N., Sokol, B., Holysz, M., Manko, W., Juszkat, R., Jagodzinski, P. P., et al. (2020). Serum myelin basic protein as a marker of brain injury in aneurysmal subarachnoid haemorrhage. Acta Neurochir. 162, 545–552. doi: 10.1007/s00701-019-04185-9
Wei, S. S., Chen, L., Yang, F. Y., Wang, S. Q., and Wang, P. (2023a). The role of fibronectin in multiple sclerosis and the effect of drug delivery across the blood-brain barrier. Neural Regen. Res. 18, 2147–2155. doi: 10.4103/1673-5374.369102
Wei, Z. D., Liang, K., and Shetty, A. K. (2023b). Complications of COVID-19 on the Central Nervous System: Mechanisms and Potential Treatment for Easing Long COVID. Aging Dis. 14, 1492–1510. doi: 10.14336/AD.2023.0312
Wicher, G., Wallenquist, U., Lei, Y., Enoksson, M., Li, X., Fuchs, B., et al. (2017). Interleukin-33 Promotes Recruitment of Microglia/Macrophages in Response to Traumatic Brain Injury. J. Neurotrauma. 34, 3173–3182. doi: 10.1089/neu.2016.4900
Wirth, K. J., Scheibenbogen, C., and Paul, F. (2021). An attempt to explain the neurological symptoms of Myalgic Encephalomyelitis/Chronic Fatigue Syndrome. J. Transl. Med. 19, 471. doi: 10.1186/s12967-021-03143-3
Wolters, F. J., Boender, J., de Vries, P. S., Sonneveld, M. A., Koudstaal, P. J., de Maat, M. P., et al. (2018). Von Willebrand factor and ADAMTS13 activity in relation to risk of dementia: a population-based study. Sci. Rep. 8, 5474. doi: 10.1038/s41598-018-23865-7
Woollacott, I. O. C., Toomey, C. E., Strand, C., Courtney, R., Benson, B. C., Rohrer, J. D., et al. (2020). Microglial burden, activation and dystrophy patterns in frontotemporal lobar degeneration. J. Neuroinflammation 17, 234. doi: 10.1186/s12974-020-01907-0
Wysokinski, A. (2016). Serum levels of brain-derived neurotrophic factor (BDNF) and neurotrophin-3 (NT-3) in depressed patients with schizophrenia. Nord. J. Psychiatry 70, 267–271. doi: 10.3109/08039488.2015.1087592
Xia, P., Ji, X., Yan, L., Lian, S., Chen, Z., and Luo, Y. (2024). Roles of S100A8, S100A9 and S100A12 in infection, inflammation and immunity. Immunology 171, 365–376. doi: 10.1111/imm.13722
Xiang, Y., Xin, J., Le, W., and Yang, Y. (2020). Neurogranin: A Potential Biomarker of Neurological and Mental Diseases. Front. Aging Neurosci. 12:584743. doi: 10.3389/fnagi.2020.584743
Xie, D., Miao, W., Xu, F., Yuan, C., Li, S., Wang, C., et al. (2022). IL-33/ST2 Axis protects against traumatic brain injury through enhancing the function of regulatory T Cells. Front. Immunol. 13:860772. doi: 10.3389/fimmu.2022.860772
Xiong, Z., Thangavel, R., Kempuraj, D., Yang, E., Zaheer, S., and Zaheer, A. (2014). Alzheimer’s Disease: Evidence for the Expression of Interleukin-33 and Its Receptor ST2 in the Brain. J. Alzheimers Dis. doi: 10.3233/JAD-132081 [Epub ahead of print].
Xu, W. D., and Huang, A. F. (2018). Role of Interleukin-38 in Chronic Inflammatory Diseases: A Comprehensive Review. Fron. Immunol. 9:1462. doi: 10.3389/fimmu.2018.01462
Yadollahikhales, G., and Rojas, J. C. (2023). Anti-Amyloid Immunotherapies for Alzheimer’s Disease: A 2023 Clinical Update. Neurotherapeutics 20, 914–931. doi: 10.1007/s13311-023-01405-0
Yamaguchi, M., Nakao, S., Arima, M., Little, K., Singh, A., Wada, I., et al. (2024). Heterotypic macrophages/microglia differentially contribute to retinal ischaemia and neovascularisation. Diabetologia doi: 10.1007/s00125-024-06215-3
Yang, C., Huang, X., Huang, X., Mai, H., Li, J., Jiang, T., et al. (2016). Aquaporin-4 and Alzheimer’s Disease. J. Alzheimers Dis. 52, 391–402. doi: 10.3233/JAD-150949
Yang, C., Zheng, C., Zhuang, Y., Xu, S., Li, J., and Hu, C. (2024). Synaptic Vesicle-Related Proteins and Ubiquilin 2 in Cortical Synaptosomes Mediate Cognitive Impairment in Vascular Dementia Rats. Mol. Neurobiol. doi: 10.1007/s12035-024-04327-w
Yang, Y., Shen, L., Xu, M., Chen, L., Lu, W., and Wang, W. (2021). Serum calprotectin as a prognostic predictor in severe traumatic brain injury. Clin. Chim. Acta 520, 101–107. doi: 10.1016/j.cca.2021.06.009
Yeung, D., Manias, J. L., Stewart, D. J., and Nag, S. (2008). Decreased junctional adhesion molecule-A expression during blood-brain barrier breakdown. Acta Neuropathol. 115, 635–642. doi: 10.1007/s00401-008-0364-4
Yong, H. Y. F., Rawji, K. S., Ghorbani, S., Xue, M., and Yong, V. W. (2019). The benefits of neuroinflammation for the repair of the injured central nervous system. Cell Mol. Immunol. 16, 540–546. doi: 10.1038/s41423-019-0223-3
Yoon, J. K., Kim, J., Shah, Z., Awasthi, A., Mahajan, A., and Kim, Y. (2021). Advanced Human BBB-on-a-Chip: A New Platform for Alzheimer’s Disease Studies. Adv. Healthc. Mater. 10, e2002285. doi: 10.1002/adhm.202002285
Yu, X., Ji, C., and Shao, A. (2020). Neurovascular Unit Dysfunction and Neurodegenerative Disorders. Front. Neurosci. 14:334. doi: 10.3389/fnins.2020.00334
Yui, S., Nakatani, Y., and Mikami, M. (2003). Calprotectin (S100A8/S100A9), an inflammatory protein complex from neutrophils with a broad apoptosis-inducing activity. Biol. Pharm. Bull. 26, 753–760. doi: 10.1248/bpb.26.753
Zapata-Acevedo, J. F., Mantilla-Galindo, A., Vargas-Sanchez, K., and Gonzalez-Reyes, R. E. (2024). Blood-brain barrier biomarkers. Adv. Clin. Chem. 121, 1–88. doi: 10.1016/bs.acc.2024.04.004
Zare Rafie, M., Esmaeilzadeh, A., Ghoreishi, A., Tahmasebi, S., Faghihzadeh, E., and Elahi, R. (2021). IL-38 as an early predictor of the ischemic stroke prognosis. Cytokine 146, 155626. doi: 10.1016/j.cyto.2021.155626
Zetterberg, H., and Blennow, K. (2016). Fluid biomarkers for mild traumatic brain injury and related conditions. Nat. Rev. Neurol. 12, 563–574. doi: 10.1038/nrneurol.2016.127
Zhang, F., Pan, L., Lian, C., Xu, Z., Chen, H., Lai, W., et al. (2024). ICAM-1 may promote the loss of dopaminergic neurons by regulating inflammation in MPTP-induced Parkinson’s disease mouse models. Brain Res. Bull. 214, 110989. doi: 10.1016/j.brainresbull.2024.110989
Zhang, R., Jiang, H., Liu, Y., and He, G. (2023). Structure, function, and pathology of Neurexin-3. Genes Dis. 10, 1908–1919. doi: 10.1016/j.gendis.2022.04.008
Zhang, S. R., Nold, M. F., Tang, S. C., Bui, C. B., Nold, C. A., Arumugam, T. V., et al. (2019). IL-37 increases in patients after ischemic stroke and protects from inflammatory brain injury, motor impairment and lung infection in mice. Sci. Rep. 9, 6922. doi: 10.1038/s41598-019-43364-7
Zhang, X., Wang, L. P., Ziober, A., Zhang, P. J., and Bagg, A. (2021). Ionized Calcium Binding Adaptor Molecule 1 (IBA1). Am. J. Clin. Pathol. 156, 86–99. doi: 10.1093/ajcp/aqaa209
Zhang, Z., Li, X., Zhou, H., and Zhou, J. (2022). NG2-glia crosstalk with microglia in health and disease. CNS Neurosci. Ther. 28, 1663–1674. doi: 10.1111/cns.13948
Zhou, L., and Todorovic, V. (2021). Interleukin-36: Structure, Signaling and Function. Adv. Exp. Med. Biol. 21, 191–210. doi: 10.1007/5584_2020_488
Zhou, X., Shi, Q., Zhang, X., Gu, L., Li, J., Quan, S., et al. (2023). ApoE4-mediated blood-brain barrier damage in Alzheimer’s disease: Progress and prospects. Brain Res. Bull. 199, 110670. doi: 10.1016/j.brainresbull.2023.110670
Zhou, Y., Cai, G., Wang, Y., Guo, Y., Yang, Z., Wang, A., et al. (2024). Microarray Chip-Based High-Throughput Screening of Neurofilament Light Chain Self-Assembling Peptide for Noninvasive Monitoring of Alzheimer’s Disease. ACS Nano. 18, 18160–18175. doi: 10.1021/acsnano.3c09642
Zingaropoli, M. A., Iannetta, M., Piermatteo, L., Pasculli, P., Latronico, T., Mazzuti, L., et al. (2022). Neuro-Axonal Damage and Alteration of Blood-Brain Barrier Integrity in COVID-19 Patients. Cells 11, 2480. doi: 10.3390/cells11162480
Keywords: blood-brain barrier disruption, glial cells, neuroinflammatory biomarkers, neurodegenerative disorders, neurofilament light, neurovascular unit, tight junction proteins
Citation: Kempuraj D, Dourvetakis KD, Cohen J, Valladares DS, Joshi RS, Kothuru SP, Anderson T, Chinnappan B, Cheema AK, Klimas NG and Theoharides TC (2024) Neurovascular unit, neuroinflammation and neurodegeneration markers in brain disorders. Front. Cell. Neurosci. 18:1491952. doi: 10.3389/fncel.2024.1491952
Received: 05 September 2024; Accepted: 07 October 2024;
Published: 25 October 2024.
Edited by:
Arumugam R. Jayakumar, University of Miami, United StatesReviewed by:
Krishnapriya Thangaretnam, University of Miami, United StatesKumar Vaibhav, Augusta University, United States
Suresh Babu Rangasamy, University of Illinois Chicago, United States
Copyright © 2024 Kempuraj, Dourvetakis, Cohen, Valladares, Joshi, Kothuru, Anderson, Chinnappan, Cheema, Klimas and Theoharides. This is an open-access article distributed under the terms of the Creative Commons Attribution License (CC BY). The use, distribution or reproduction in other forums is permitted, provided the original author(s) and the copyright owner(s) are credited and that the original publication in this journal is cited, in accordance with accepted academic practice. No use, distribution or reproduction is permitted which does not comply with these terms.
*Correspondence: Duraisamy Kempuraj, kduraisa@nova.edu; orcid.org/0000-0003-1148-8681