- 1Division of Life Science and Applied Life Science (BK21 FOUR), College of Natural Sciences, Gyeongsang National University, Jinju-si, Republic of Korea
- 2Haemato-Oncology/Systems Medicine Group, Paul O'Gorman Leukaemia Research Centre, Institute of Cancer Sciences, MVLS, University of Glasgow, Glasgow, United Kingdom
- 3Department of Psychiatry and Neuropsychology, School for Mental Health and Neuroscience (MHeNs), Maastricht University, Maastricht, Netherlands
- 4Alz-Dementia Korea Co., Jinju-si, Republic of Korea
Alzheimer's disease (AD) is a progressive neurodegenerative disorder pathologically characterized by the deposition of amyloid beta (Aβ) plaques and neurofibrillary tangles (NFTs) in the brain. The accumulation of these aggregated proteins causes memory and synaptic dysfunction, neuroinflammation, and oxidative stress. This research study is significant as it aims to assess the neuroprotective properties of vitamin E (VE) analog Trolox in an Aβ1 − 42-induced AD mouse model. Aβ1 − 42 5μL/5min/mouse was injected intracerebroventricularly (i.c.v.) into wild-type adult mice brain to induce AD-like neurotoxicity. For biochemical analysis, Western blotting and confocal microscopy were performed. Remarkably, intraperitoneal (i.p.) treatment of Trolox (30 mg/kg/mouse for 2 weeks) reduced the AD pathology by reducing the expression of Aβ, phosphorylated tau (p-tau), and β-site amyloid precursor protein cleaving enzyme1 (BACE1) in both cortex and hippocampus regions of mice brain. Furthermore, Trolox-treatment decreased neuroinflammation by inhibiting Toll-like receptor 4 (TLR4), phosphorylated nuclear factor-κB (pNF-κB) and interleukin-1β (IL-1β), and other inflammatory biomarkers of glial cells [ionized calcium-binding adaptor molecule 1 (Iba1) and glial fibrillary acidic protein (GFAP)]. Moreover, Trolox reduced oxidative stress by enhancing the expression of nuclear factor erythroid-related factor 2 (NRF2) and heme oxygenase 1 (HO1). Similarly, Trolox-induced synaptic markers, including synaptosomal associated protein 23 (SNAP23), synaptophysin (SYN), and post-synaptic density protein 95 (PSD-95), and memory functions in AD mice. Our findings could provide a useful and novel strategy for investigating new medications to treat AD-associated neurodegenerative diseases.
1 Introduction
Alzheimer's disease (AD) is the primary contributor to the onset of dementia, a devastating neurodegenerative disorder categorized by the growing loss of cholinergic neurons (Godoy et al., 2014; Stefanova et al., 2015). This neurodegeneration results in a decline in cognitive abilities, typically evident through changes in thinking, learning, memory, and daily life activities (Denny et al., 2021). Despite extensive research, the exact cause of AD remains unknown. The two pathological hallmarks of AD, first categorized by Alois Alzheimer, are the deposition of amyloid plaques and neurofibrillary tangles (NFTs) in the female patient (Auguste D) brain having the age of 51 years old. The two transmembrane enzymes (β-site amyloid precursor protein cleaving enzyme1 (BACE1) and α-secretase) undergo proteolysis of amyloid precursor protein (APP) into amyloid β-peptides (Aβ1 − 42). On the other side, hyperphosphorylation of the microtubule-associated tau protein leads to forming NFTs of varying lengths and shapes. The deposition of Aβ1 − 42 peptides and NFTs in the brain leads to neuroinflammation, oxidative stress, and synaptic and memory dysfunctions, followed by neurodegeneration (Miranda et al., 2000; Behl et al., 1994; Koppal et al., 1998; Leissring et al., 2002; Schieber and Chandel, 2014; Badshah et al., 2019). Both the cytotoxic proteins (Aβ1 − 42 peptides and p-Tau) in the brain trigger immune responses through glial cells, specifically microglia and astrocytes, leading to subsequent neuroinflammation and release different proinflammatory mediators and cytokines (phosphorylated nuclear factor kappa B, interleukin 1β and Toll-like receptor 4). The neurotoxic effect of Aβ1 − 42 has been observed and supported by different in vitro studies that are mediated by free radical mechanisms (Miranda et al., 2000; Behl et al., 1994; Koppal et al., 1998) and variation in calcium ion (Ca2+) homeostasis in neuronal cells (Leissring et al., 2002). In addition, AD progression involves oxidative stress induced by reactive oxygen species (ROS), which are free radical molecules produced abnormally during cellular metabolism. This oxidative stress is associated with cellular damage, affecting DNA, proteins, and lipids, contributing to various diseases such as neurodegenerative disorders, cardiovascular diseases, and cancer (Schieber and Chandel, 2014; Badshah et al., 2019; Agostinho and Oliveira, 2010). Under natural conditions, reactive antioxidants develop in a biologically controlled environment, impacting cellular processes such as autophagy, inflammation, immunological response, and cell division. Some of the significant antioxidant genes, including nuclear factor erythroid-related factor 2 (NRF2), have a detrimental effect and regulate neuroinflammation, oxidative stress, and synapse formation in the brain (Khan et al., 2018; Huang et al., 2005). Numerous studies have proposed that enhanced oxidative stress in neuronal cells disrupts the internal antioxidant system, leading to downregulation of NRF2 and its target gene heme oxygenase 1 (HO1) protein expression levels (Giordano et al., 2020; Khan et al., 2019).
A growing number of plant-derived natural products are being investigated as therapeutic agents for preventing and treating neurological disorders such as AD and Parkinson's disease (Huang and Mucke, 2012). Vitamins are essential micronutrients for strengthening the immune system and fighting infections. They contribute to neurogenesis, the defense mechanism of neurons, and are involved in metabolic reactions, neuron survival, and transmission (Kumar et al., 2022). Additionally, vitamin E (VE) is recognized as a vital micronutrient in the diet of most animals. It comprises tocopherols and tocotrienols, with α-tocopherol being the most potent homolog (Xu et al., 2023). Several previous studies have revealed that VE performs various functions, such as improving animal growth and reproduction, sustaining cell membrane homeostasis, and enhancing body immunity and anti-inflammatory and antioxidant capabilities (Rengaraj and Hong, 2015; El-Sayed and Izquierdo, 2022; Sattler et al., 2003; Kim et al., 2017). In this study, Trolox (6-hydroxy-2, 5, 7, 8-tetramethylchroman-2-carboxylic acid), a hydrophilic counterpart to α-tocopherol and a prominent form of tocopherols in the human body, was employed against Aβ1 − 42-induced AD mouse model (Massey and Burton, 1990; Lúcio et al., 2009). Trolox, like α-tocopherol, acts as a potent lipid antioxidant by scavenging lipid peroxyl free radicals, preventing peroxidation of polyunsaturated fatty acids, and protecting cell membranes from oxidative damage. Unlike α-tocopherol, Trolox is water-soluble and lipophilic, reaching both water and lipid segments of cells. It has become more significant than α-tocopherol in several biochemical assays due to its superior radical scavenging efficacy against peroxyl and alkoxyl radicals (Giordano et al., 2020). Trolox has been previously reported as a potent antioxidant and anti-inflammatory against 1-methyl-4-phenyl-1,2,3,6-tetrahydropyridine (MPTP)-induced Parkinson's disease in a mouse model (Atiq et al., 2023), a potent antioxidant and inflammatory candidate that alleviates inflammation and oxidative stress in human and murine primary alveolar type II cells from injury (Messier et al., 2013) and preventing oxidative stress-induced apoptosis in mouse thymocytes (Forrest et al., 1994), and renal normal rat kidney 52e cells) (Guo et al., 2012). At present, we hypothesized that Trolox might rescue mice brains from the Aβ1 − 42-induced neuroinflammation mediated neurodegeneration and oxidative stress in the Aβ1 − 42-induced AD mouse model. Different neuroprotective properties, including antioxidant, anti-inflammatory, and synaptic properties, were assessed by biochemical, cognitive, and immunohistochemical assays.
2 Materials and methods
2.1 Animals
Wild-type male mice (C57BL/6N n = 32, 8 weeks old, 25–30 g body weight) were purchased from Samtako Biolabs (Ulsan, South Korea). Under a 12-h light/dark cycle, all mice were housed for habituation for 7 days in temperature- and humidity-controlled settings with free access to food and water. All the experimental protocols for animal care and treatment were approved (approval ID: 125, animal ethics code: GNU-200331-M0020) by the Animal Ethics Committee of the Division of Applied Life Sciences, Department of Biology, Gyeongsang National University, South Korea.
2.2 Drug treatment with animal groupings
A sterile saline solution was used as a solvent to prepare a stock solution of human-derived Aβ1 − 42 peptide (Cat. #PP69, Sigma–Aldrich, St. Louis, MO, USA) at 1 mg/ml concentration. This solution was then incubated at 37°C for 4 days to allow appropriate accumulation of Aβ1 − 42 oligomers. Mice were anesthetized with a combination of Rompun (xylazine, 10 mg/kg) and Zoletil (ketamine, 90 mg/kg) dissolved in 0.9% saline and injected intraperitoneally (i.p.). The Aβ1 − 42 peptide or vehicle (5 μL or 0.9% NaCl/5 min/mouse) was stereotaxically injected intracerebroventricular (i.c.v.) into the mice brain ventricles by using a Hamilton microsyringe in different measurements of −2.4 mm dorsoventral (DV), −0.2-mm anteroposterior (AP) and 1-mm mediolateral (ML) to the bregma point. The stereotaxic surgical procedure was conducted in a room equipped with a temperature control system to maintain the animals' body temperature between 36°C and 37°C. The body temperature was regularly monitored with a thermometer due to the hypothermic effects of anesthesia, which can induce tau phosphorylation (Ali et al., 2015). The animals were assigned into four groups: (1) control group (0.9% saline as control mice), (2) Aβ1 − 42 group (5 μL/5 min/mouse), (3) Aβ1 − 42 + Trolox group [30 mg/kg (i.p.) per day for 2 weeks] and (4) Trolox alone group as a sham group [30 mg/kg (i.p.) per day for 2 weeks, dissolved in phosphate buffer saline (pH 7.4) (Figure 1)]. The dose of Trolox was selected as reported previously (Sharma and Sayyed, 2006). Each group of animals contained eight mice, and each of them was allocated for morphological study and Western blot analysis.
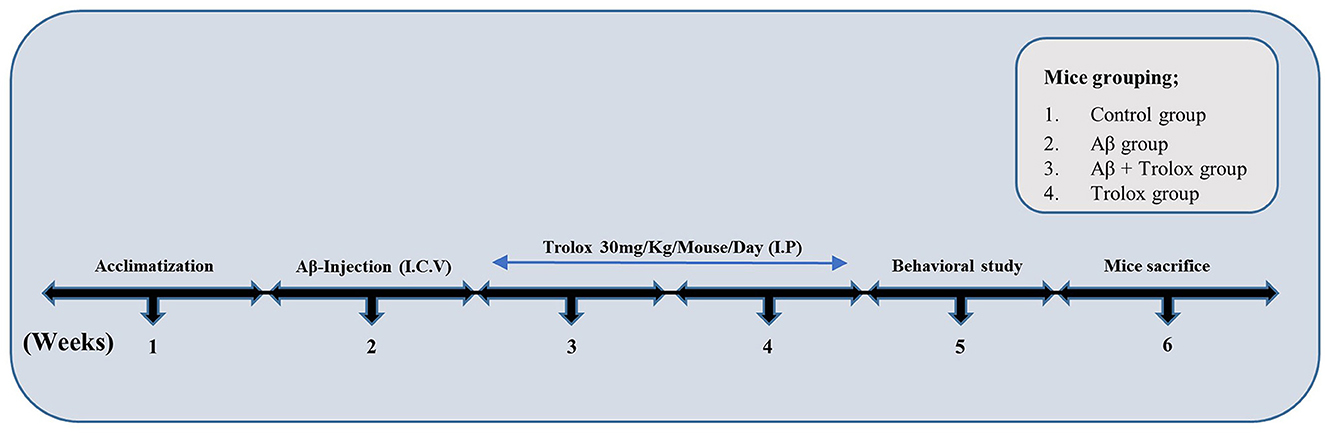
Figure 1. Experimental plan of Trolox against Aβ1 − 42-induced Alzheimer's disease mouse model. The experimental paradigm consisted of 6 weeks. Mice were acclimatized in the first week, Aβ-Intracerebroventricular (i.c.v.) injection was administered in the second week, and Trolox (30 mg) drug was treated in the third and fourth weeks. Behavioral analysis (MWM, Y-maze) was performed on the fifth week, and the mice were euthanized on the sixth week.
2.3 Behavioral study
2.3.1 Y-maze test
The Y-maze was performed as described previously (Ikram et al., 2019). The translucent plexiglass sheets made up of the Y-maze apparatus were each 50 cm long, 20 cm high, and 10 cm wide at the bottom and top. The mice were kept in the middle of the instrument for 8-min phases, allowing free movement to explore the maze. The consecutive entry of the mice into the three arms in an overlapping triplet was detected as one of the spontaneous changes. Spontaneous alternation behavior in the form of higher percentage values was considered to improve cognitive capabilities. The alteration behavior has been presented in percentage using the formula: (entries into three arms consecutively/total number of arm entries – 2) × 100.
2.3.2 Morris water maze test
The cognitive behavior of mice was observed by distributing mice into four groups, n = 8 for each group. The Morris water maze (MWM) was performed as previously described (Yan and Vassar, 2014; Vassar, 2014). MWM consisted of a circular tank (100 cm in diameter, 40 cm in height) containing water (23 ± 1°C) with a depth of 15.5 cm, and a non-toxic white-colored ink was dissolved by making transparent water opaque. The transparent escape platform was kept in the center of one quadrant 1 cm below the water's surface to make it invisible. It measures 10 cm in diameter and 20 cm in height. Each mouse was trained once daily for 5 days consecutively on a single hidden platform in one of the three rotating quadrants. For the valuation of memory, a probe test was conducted the next day of training by removing the hidden platform, and mice were allowed to swim freely for the time scale of 60 s. The number of crossovers where the hidden platform was present during the training session and the time spent in the target quadrant were recorded. A video-recording software (SMART Pan lab, Harvard Apparatus, Holliston, MA, USA) was used for data recording purposes.
2.4 Protein extraction
The protein extraction was performed as previously described (Chuang et al., 2017). Using ketamine and xylazine, the mice were anesthetized intramuscularly (i.m.), euthanized, and removed. The brain, the cortex, and hippocampus regions were carefully dissected and were preserved at −80°C for further experiments. The cortex and hippocampus regions were homogenized in PRO-PREPTM extraction solution (iNtRON Biotechnology, Inc., Sungnam, South Korea) and centrifuged at 13,000 g for 30 min at 4°C. After centrifugation, the supernatant was collected and kept at −80°C for the Western blot analysis.
2.5 Western blot analysis
The immunoblotting analysis followed established protocols from previous studies (Khan et al., 2021). Briefly, a Bio-Rad assay kit (Bio-Rad Laboratories, Irvine, CA, USA) was used to determine protein concentrations. Proteins extracted from the brains of all experimental mice groups in equal amounts were subjected to SDS-PAGE on 4–15% gels with a prestained protein marker (GangNam-STAIN, iNtRON Biotechnology, Dallas, TX, USA) and then transferred to polyvinylidene difluoride (PVDF) membranes (Immobilon-PSQ, Transfer membrane, Merck Millipore, Burlington, MA, USA). After PVDF membrane transfer, all membranes were blocked with 5% skim milk (Difco™ Skim Milk, BD, France) and then incubated with primary antibodies overnight at 4°C. Furthermore, after incubation, the membranes were probed with horseradish peroxidase (HRP)-conjugated secondary antibodies. The protein bands were detected by applying an Enhanced chemiluminescent (ECL) detecting solution (ATTO Corporation, Tokyo, Japan), further scanning the X-ray films. A densitometric analysis of the protein bands was performed using ImageJ software (v.1.50, NIH, Bethesda, MD, USA).
2.6 Sample preparation for morphological analysis of brain specimens
The brain specimens for morphological examination were prepared as described previously (Khan et al., 2023). All the mice were anesthetized (ketamine and xylazine) (i.m.) and transcardially perfused with 0.9% normal saline and 4% paraformaldehyde solution. The brains were carefully removed and fixed in ice–cold 4% neutral buffer paraformaldehyde at 4°C for 72 h, and then all the brains were dehydrated in 20% sucrose for 72 h. Furthermore, the brains were placed in an optimum cutting temperature (OCT) compound obtained from Finetek Japan Co., Ltd., Tokyo, Japan, and then frozen. Brain sections of 14 μm were obtained on gelatin-coated slides using a microtome (CM 3050C cryostat, Leica, Germany).
2.7 Immunofluorescence staining
Immunofluorescence analysis was carried out as conducted previously (Amin et al., 2017). All slides were washed twice for 10 min with phosphate-buffered saline (PBS, 1%). The slides were then incubated at room temperature for 5 min with proteinase K. A blocking solution containing 0.3% Triton X-100 and 2% normal serum dissolved in 1% PBS was applied to each slide for 1 h after washing. All slides were treated with primary antibodies overnight at 4°C. Following incubation, brain sections were washed with PBS and exposed to secondary antibodies that were tetramethylrhodamine isothiocyanate (TRITC) or fluorescein isothiocyanate (FITC) (antirabbit, antigoat, or antimouse) diluted 1:50 in PBS for 90 min at room temperature. After secondary antibody treatment, the tissue slides were counterstained for 8 min with 4′,6-diamidino-2-phenylindole (DAPI) nucleus solution. Then, the slides were covered with a coverslip using a mounting media by applying DPX (Distyrene Plasticizer Xylene). Immunofluorescence imaging was performed using a confocal laser scanning microscope (FV 1000MPE, Olympus, Japan).
2.8 Antibodies
Table 1 The primary antibodies used in this study.
3 Statistical data analysis
The reported result data was analyzed as mean ± standard error of the mean (SEM) using one-way ANOVA followed by Tukey's test for comparing various treatment groups of mice for the replicate of three experiments. For this purpose, eight mice/group were taken, and the p-value (p < 0.05) was set as a standard for the significant differences among the groups. Significance: #p ≤ 0.05, ##p ≤ 0.01, and ###p ≤ 0.001; *p ≤ 0.05, **p ≤ 0.01, and ***p ≤ 0.001. ImageJ software was used for the immunohistological quantitative study which is shown as arbitrary units (AU).
4 Results
4.1 Trolox-treatment reversed Aβ1 − 42-induced cognitive impairment
We performed neurobehavioral analysis (Y-maze and Morris water maze (MWM) tests) to examine the effects of Trolox on the memory and learning assessment of different experimental mice groups. First, we tested spatial working memory using the Y-maze (Figures 2A, B). Cognitive performance was assumed to be enhanced by a higher percentage (%) of spontaneous alteration behavior. Working memory was reduced in the Aβ1 − 42-injected mice group, presenting a significantly lower rate (21.68 %) of spontaneous alteration compared to the normal control mouse group (48.66 %) and Trolox-treated group (48.91 %), showing significant memory functions. Conversely, Trolox + Aβ1 − 42-treatment indicated a significant increase (41.97%) in spontaneous-alteration behavior compared with Aβ1 − 42-injected mice group, which demonstrated that Trolox mitigated short-term memory discrepancies in the Aβ1 − 42-injected mice group (Figures 2A, B). In the training phase of MWM, all groups' mice were allowed to search the hidden platform located in one quadrant of the water tank, which showed that AD mice took more time as compared to saline-treated control mice while treatment with Trolox reduced the time (Figure 2C). Furthermore, a probe test was performed after the training phase by removing the hidden platform. The results showed that Aβ1 − 42-induced mice spent less time and several crossings in a zone that previously had hidden platforms (Figures 2D, E). Time spent in the target quadrant and crossings over the hidden platform were significantly increased after treatment with Trolox. These results revealed that Trolox improved memory and learning functions.
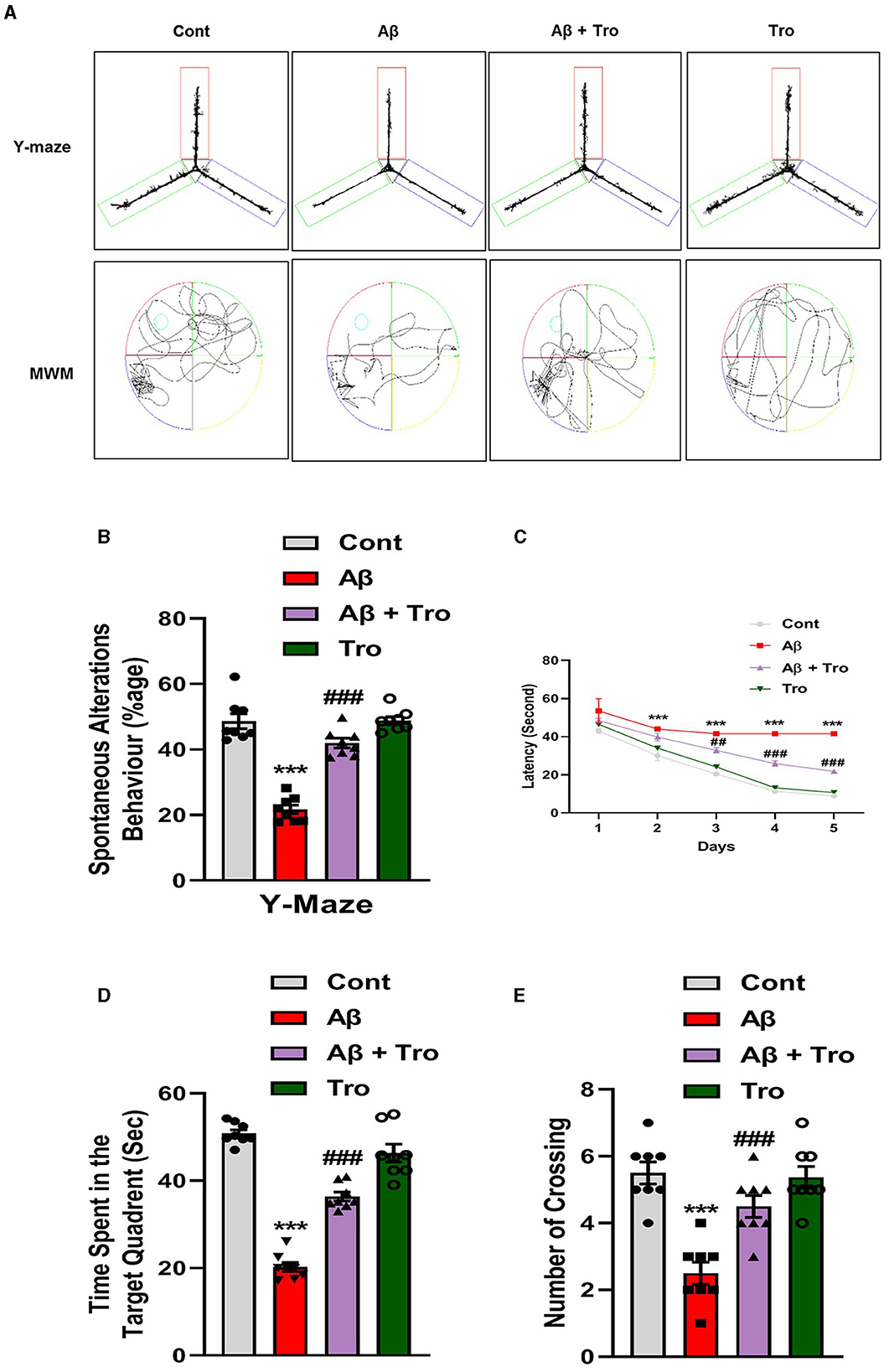
Figure 2. Neuroprotective effects of Trolox on memory and learning of mice brain. (A) The trajectory map of mice in Y-maze and MWM. (B) Y-maze test shows the percentage of spontaneous alteration behavior of experimental mice. (C) Line graph that represents average escape latency to reach the hidden platform until the fifth day. (D) Time spent by the mice in the quadrant where the platform was in training; (E) indicates the probe test. The data are presented as the mean ± SEM from eight mice per group, for Western blot and confocal microscopy, with four mice per group used in each assay, and are representative of three independent experiments. Scale bar = 50 μm, magnification 10×. Asterisks denote a significant difference from saline-injected control mice, and # indicates from Aβ1 − 42-injected mice. ***p < 0.001,###p < 0.001, and p < 0.05.
4.2 Trolox-treatment downregulated the Aβ1 − 42, p-Tau and BACE1 expression causing Alzheimer's disease in mouse brain
According to the previous study, it has been investigated that a single dose of intracerebroventricular (i.c.v.) injection of Aβ1 − 42 peptides induces memory and cognitive impairment, causes deposition of Aβ and induces AD in healthy mice brains analogous to the indications detected in humans' brains (Khan et al., 2021; Liang et al., 2010). In line with these studies, our Western blot results exhibited higher expression for Aβ in the AD-induced mice's brain cortex and hippocampus than saline-treated control mice. Trolox treatment significantly reduced the expression of Aβ in the brain cortex and hippocampus in the AD mouse model (Figure 3A). Similarly, the tau protein is essential for stabilizing microtubules and plays a significant role in providing structural support and facilitating intracellular transport in neurons (Rawat et al., 2022). Our Western blot results demonstrated higher expression for p-Tau in both the cortex and hippocampus of AD-induced mice's brains as compared to saline-treated control mice. Interestingly, Trolox treatment significantly decreased the expression of p-Tau in the brain of AD-induced mice. β-secretase, or β-site amyloid precursor protein cleaving enzyme 1 (BACE1), plays an essential role in AD, which causes the formation of Aβ1 − 42 peptides by splitting the amyloid precursor protein (APP) (Mateos-Aparicio and Rodríguez-Moreno, 2019). Similarly, a higher expression level of BACE1 was found in both cortex and hippocampus in the brain of the Aβ1 − 42-injected mice group, which was reversed with the treatment of Trolox (Figure 3A). To further confirm the Aβ and p-Tau Western blot results, we performed confocal microscopy for the examination of immunoreactivity of Aβ and p-Tau in the AD-induced mice brain, which showed enhanced immunoreactivity of Aβ and p-Tau in the AD-induced mice brain compared to a control group of mice. At the same time, Trolox treatment effectively reduced the expression of Aβ in both the cortex and hippocampus (Figure 3B).
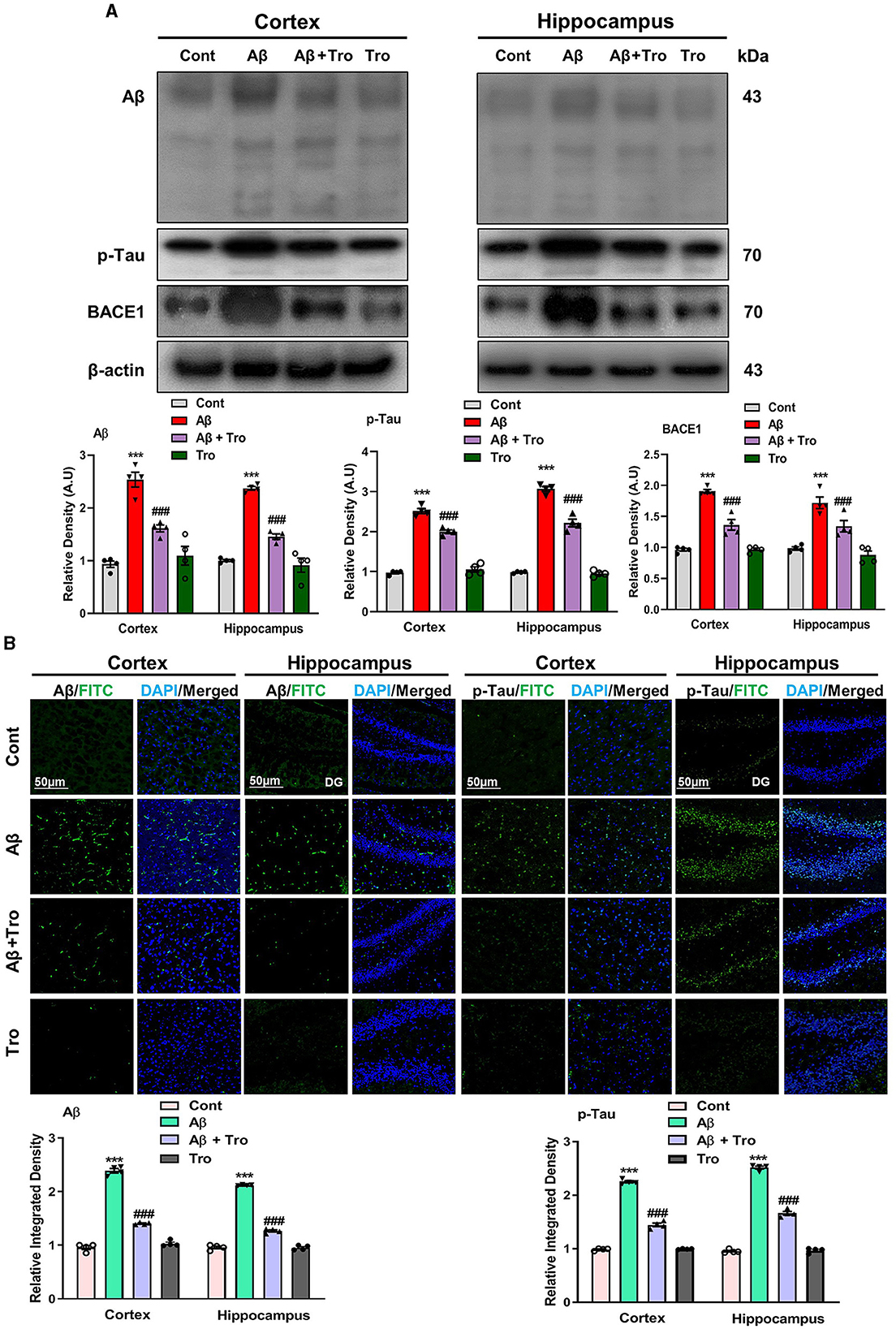
Figure 3. Trolox mitigated AD pathology by decreasing the Aβ, p-Tau, and BACE1 protein expressions. (A) Western blot analysis of Aβ, p-Tau, and BACE1 protein expressions in the cortex and hippocampus of mice brain. As a loading control, β-actin was used. Band quantification among the groups was performed using ImageJ software. (B) Confocal microscopy results show the immunofluorescence of Aβ and p-Tau (green) along with their respective histograms and DAPI staining (blue) in the cortex and hippocampus [dentate gyrus (DG)] of adult mice. The values of relative density compared with the control group are measured in arbitrary units (AU). The present data are measured as the mean ± SEM of eight mice/group, every four mice per group for Western blot and confocal microscopy, respectively, and are representative of three independent experiments. Scale bar = 50 μm, magnification 10×. Significance levels are indicated as ***p < 0.001 ###p < 0.001, and p < 0.05. Asterisks denote significant differences from saline-injected control mice, while hashtags indicate differences from Aβ1 − 42-injected mice.
4.3 Trolox abrogated Aβ1 − 42-induced glial cell activation in AD mice brains
Within the brain, the glial cells (microglia and astrocytes) play a vital role in maintaining neuronal homeostasis. The accumulation of Aβ in the brain induces an excessive response from glial cells, triggering neuroinflammation and neurodegenerative (Kim et al., 2018). In our study, we examined the effects of Trolox in AD-induced activated glial cells, which showed higher expressions of Iba1 (Ionized calcium-binding adaptor molecule 1, a marker of activated microglia) and GFAP, (glial fibrillary acidic protein, a marker of activated astrocytes) in the AD-induced mice cortex and hippocampus as compared to saline-treated normal mice. At the same time, treatment with Trolox reduced the expression of activated glial cells (Figure 4A). For further confirmation of immunoblot results, we performed confocal microscopy to examine the immunoreactivity of GFAP and Iba1 in Aβ-induced mice brains. Immunofluorescence results revealed more immunoreactivity of GFAP and Iba1 in the cortex and hippocampus regions in the Aβ1 − 42 mice group compared with the control group. In contrast, co-administration of Trolox + Aβ1 − 42 significantly reduced the immunoreactivity of GFAP and Iba1 in the cortex and hippocampus regions in AD-induced mice (Figure 4B).
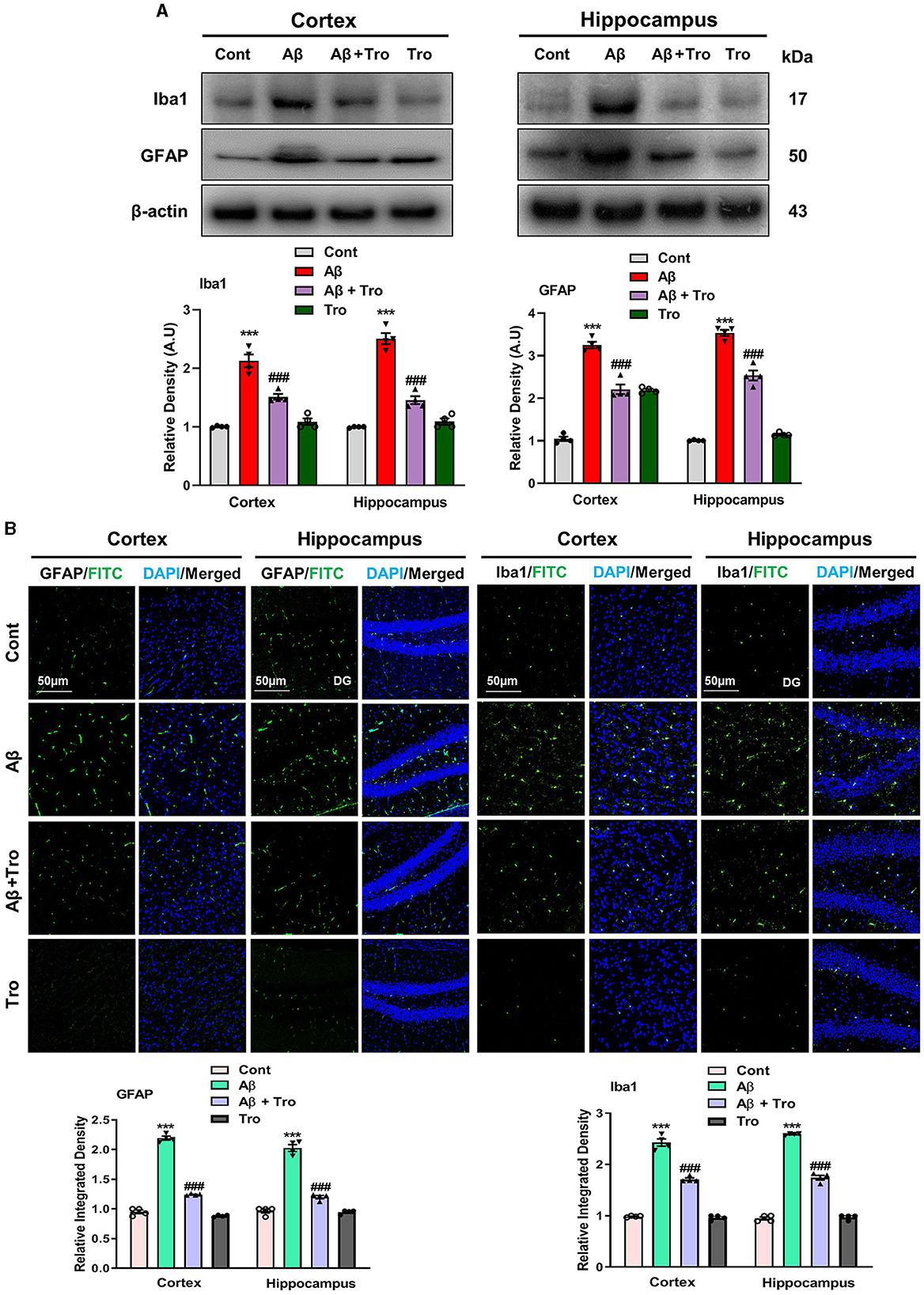
Figure 4. Effects of Trolox on reduction of glial cell activation. (A) Analysis of protein expression of Iba1 and GFAP in mice brain cortex and hippocampus, β-actin was used as a loading control. Bands were quantified among different mice groups by ImageJ software. (B) Confocal microscopy shows the immunoreactivity of GFAP and Iba1 (green) and DAPI staining (blue) in the cortex and hippocampus (DG) as well as their respective histograms. The values of relative density compared with the control group are measured in arbitrary units (AU). The data are presented as the mean ± SEM from eight mice per group for Western blot and confocal microscopy, with four mice per group used in each assay, and are representative of three independent experiments. Scale bar = 50 μm, magnification 10×. Significance levels are indicated as *** p < 0.001, ###p < 0.001, and p < 0.05. Asterisks (*) denote significant differences from saline-injected control mice, while hashtags (#) indicate differences from Aβ1 − 42-injected mice.
4.4 Trolox mitigated Aβ1 − 42-induced neuroinflammation by suppressing inflammatory cytokines in the AD mice brain
Toll-like receptor 4 (TLR4) is the surface receptor of glial cells (Iba1 and GFAP). We evaluated the effect of Trolox on these inflammatory cytokines (TLR4, pNF-kB, and IL-1β) using Western blotting. The expression of TLR4 is upregulated in the Aβ1 − 42 injected group, which was significantly downregulated in the Aβ1 − 42 + Trolox co-treated group compared to a control group of mice. We investigated the expression of phosphorylated nuclear factor kappa B (pNF-kB) in the experimental groups of mice. Since pNF-kB is crucial in the etiology of AD. Additionally, the interleukin 1β (IL-1β) is also released by the activated pNF-kB, which is responsible for neuroinflammation (Muhammad et al., 2019). The immunoblot analysis demonstrated the elevated expression of these biomarkers in both the cortex and hippocampus in the Aβ1 − 42-induced AD mouse model. Whereas Trolox treatment reduced the expressions of these inflammatory cytokines in the cortex and hippocampus of AD-induced mice brains (Figure 5). These investigations revealed that Trolox is a potent candidate for neuroinflammation.
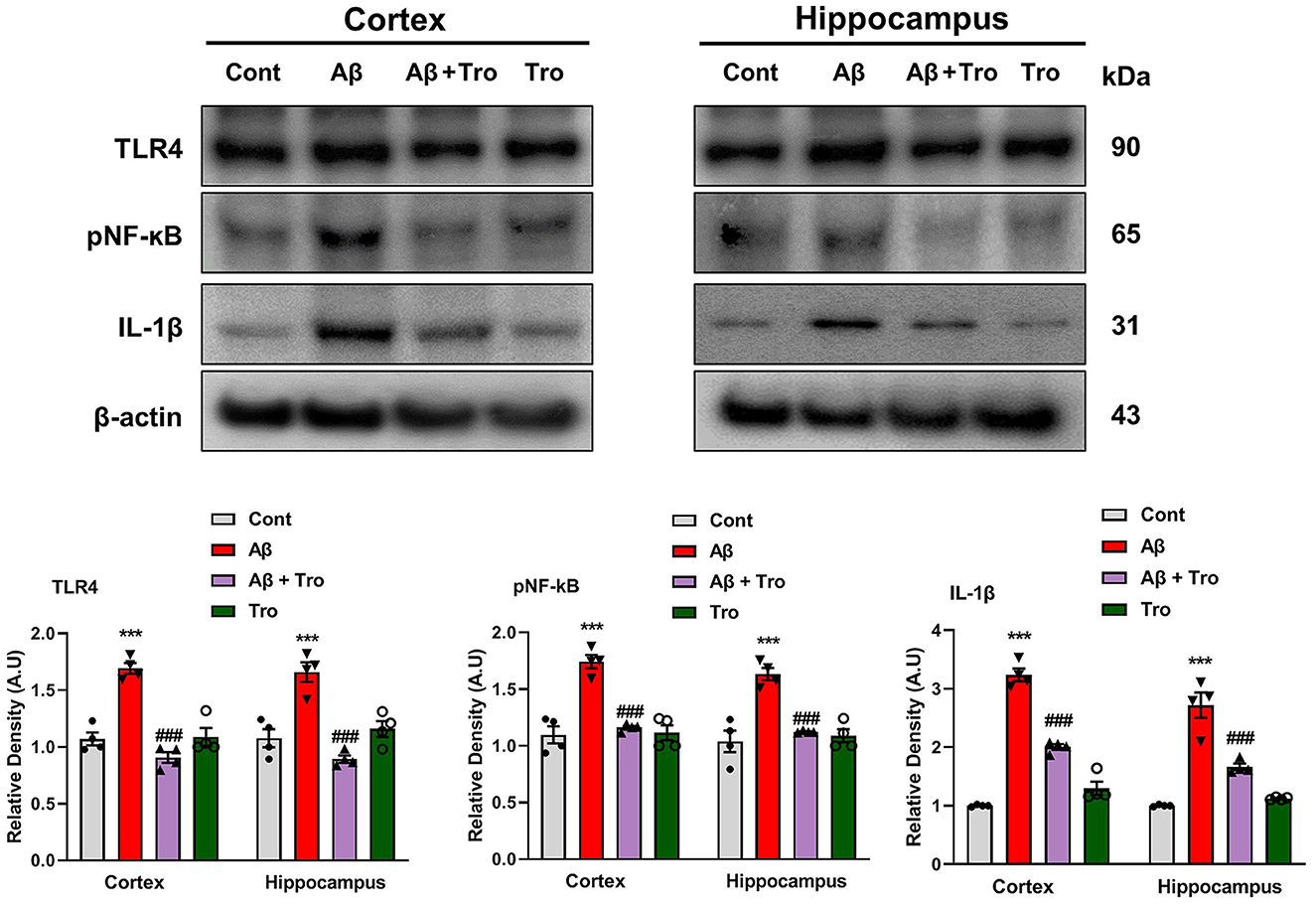
Figure 5. Trolox reduced inflammatory mediators in Aβ1 − 42-induced mice brains. Immunoblotting Analysis of TLR4, pNF-kB, and IL-1β proteins expressed in mice's brain cortex and hippocampus, β-actin was used as a loading control. ImageJ software was used to quantify bands across mouse groups along with their respective histograms. The values of relative density compared with the control group are measured in arbitrary units (AU). The data are presented as the mean ± SEM from eight mice per group for Western blot and confocal microscopy, with four mice per group used in each assay, and are representative of three independent experiments. Scale bar = 50 μm, magnification 10×. Significance levels are indicated as ***p < 0.001, ###p < 0.001, and p < 0.05. Asterisks denote significant differences from saline-injected control mice, while hashtags indicate differences from Aβ1 − 42-injected mice.
4.5 Trolox suppressed the Aβ1 − 42-induced oxidative stress by elevating NRF2/HO1 level in AD mouse brain
Trolox has shown strong scavenging activity against various free radicals across multiple cellular model systems and has been approved as a reference compound as an antioxidant. The two eminent markers responsible for oxidative stress measurement are nuclear factor erythroid 2-related factor (NRF2) and heme oxygenase 1 (HO1), which perform defensive characteristics against oxidative stress in neurodegenerative conditions (Zhang et al., 2021). We also examined the antioxidative effects of Trolox against Aβ1 − 42-induced oxidative stress in the mouse brain cortex and hippocampus. Our Western blot analysis revealed significantly reduced levels of NRF2 and HO1 in both cortex and hippocampus in Aβ1 − 42-injected animals; surprisingly, Trolox treatment significantly enhanced the expression of NRF2/HO1 in the AD-induced mice brain (Figure 6A). We performed immunofluorescence analysis to confirm further our investigations, which showed a decreased level of NRF2 in the Aβ1 − 42-injected mice brains compared to saline-treated control mice and was upregulated with Trolox-treated mice brains (Figure 6B).
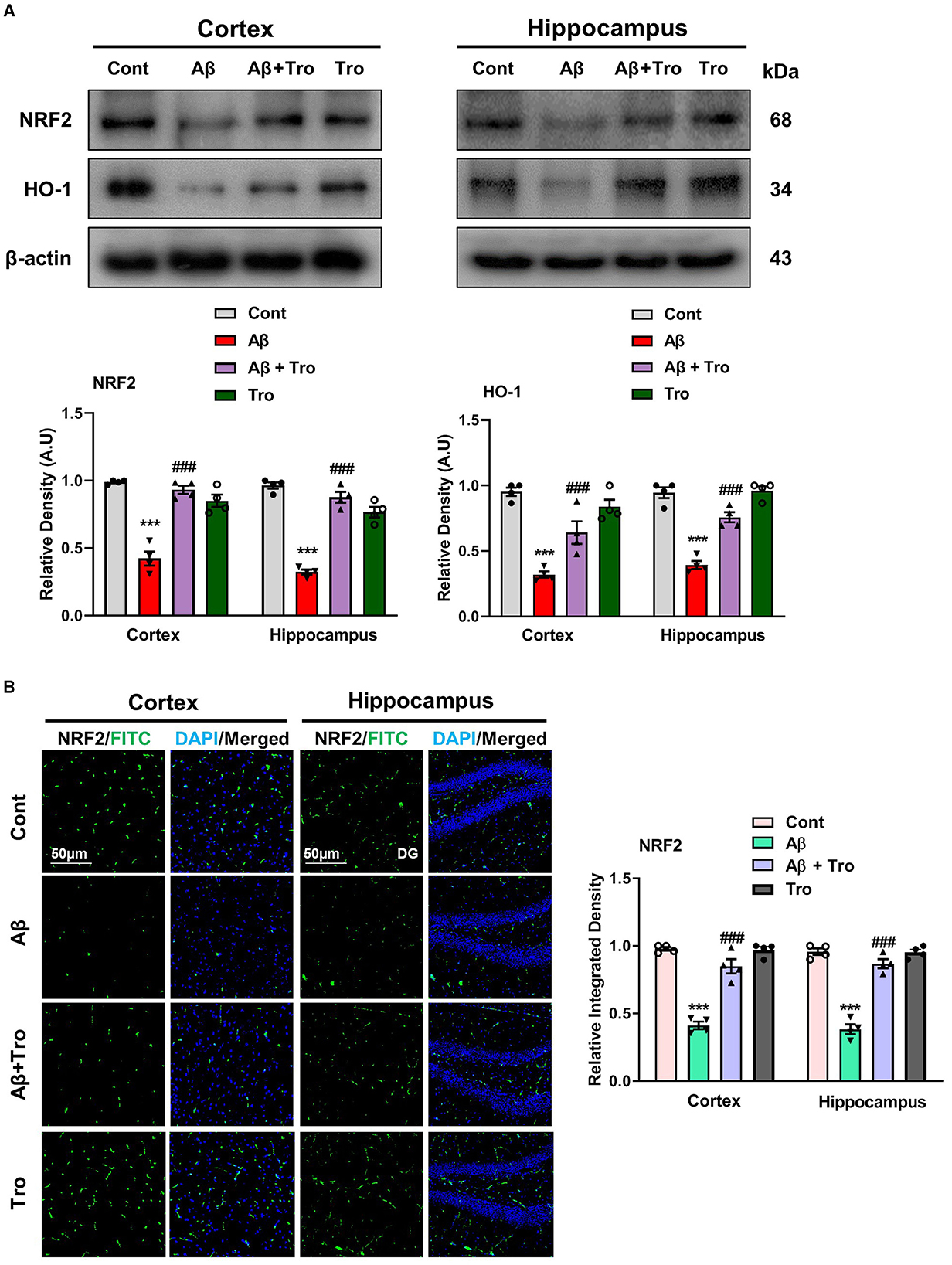
Figure 6. Neuroprotective effects of Trolox on oxidative stress. (A) Immunoblot analysis of the proteins NRF2 and HO-1 expression in cortex and hippocampus of adult mice brain. β-Actin as a loading control was used. (B) Immunofluorescence of NRF2 (green) along with their respective histograms and DAPI staining (blue) in both cortex and hippocampus (DG) of mice brain. The values of relative density compared with the control group are measured in arbitrary units (AU). The data are presented as the mean ± SEM from eight mice per group for Western blot and confocal microscopy, with four mice per group used in each assay, and are representative of three independent experiments. Magnification 10×, scale bar = 50 μm. Significance levels are indicated as ***p < 0.001, ###p < 0.001, and p < 0.05. Asterisks denote significant differences from saline-injected control mice, while hashtags indicate differences from Aβ1 − 42-injected mice.
4.6 Trolox administration improved synaptic proteins in AD mouse brains
It is reported that Aβ is toxic to synaptic function (Chen et al., 2014). For synaptic integrity analysis, we measured the synaptic proteins such as post-synaptic density protein 95 (PSD-95) and pre-synaptic proteins such as synaptosomal associated protein 23 (SNAP23) and synaptophysin (SYN). Our Western blot results showed reduced expression of these synaptic proteins compared to saline-treated control mice. On the contrary, Trolox treatment increases the expression levels of these synaptic markers in the AD mouse brain (Figure 7).
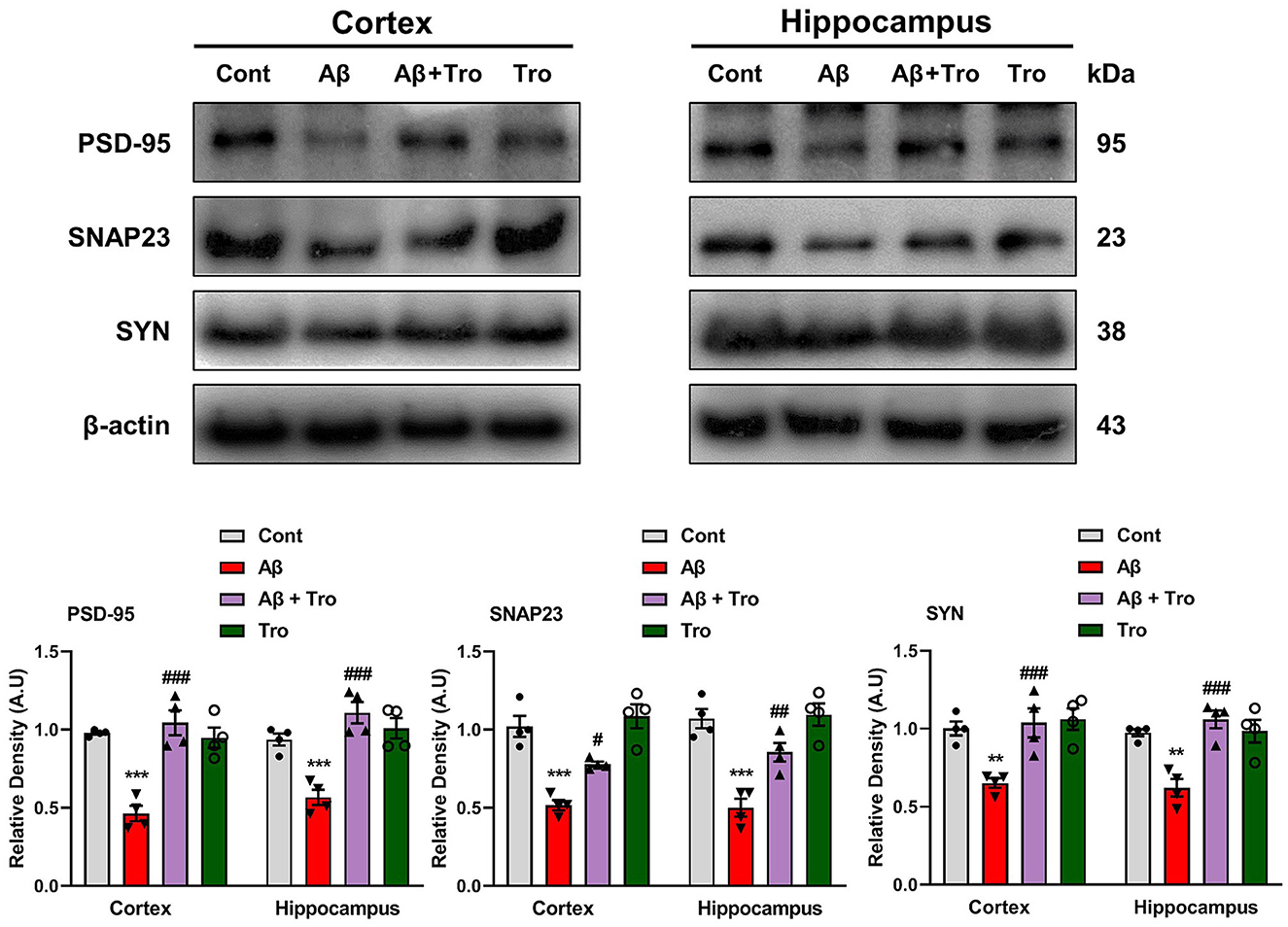
Figure 7. Trolox treatment enhanced the expression of synaptic proteins in the Aβ1 − 42-induced mice brain. Mouse cortex and hippocampus PSD-95, SNAP23, and SYP, protein expression analysis by Western blotting. β-actin was used as a loading control. Quantification of bands and histograms across mouse groups was carried out using ImageJ software. Compared to the control group, relative densities are expressed as arbitrary units (AU). The data are presented as the mean ± SEM from eight mice per group for Western blot and confocal microscopy, with four mice per group used in each assay, and are representative of three independent experiments. Scale bar = 50 μm, magnification 10×. Significance levels are indicated as ***p < 0.001, ###p < 0.001, and p < 0.05. Asterisks denote significant differences from saline-injected control mice, while hashtags indicate differences from Aβ1 − 42-injected mice.
5 Discussion
The Aβ1 − 42 peptide synthesis and accumulation in the brain are the primary pathophysiological indicators of Alzheimer's disease (AD), which is the leading cause of dementia and neurodegenerative conditions (Ali et al., 2021). The Aβ accumulation in the brain induces neuroinflammation and oxidative stress, which further causes synaptic and memory dysfunction. Currently, numerous research studies are underway to lower the formation and content of Aβ in the brain of AD, and there is no proper treatment available for the patients. In this regard, therapeutic agents that showed anti-inflammatory and antioxidative properties were suitable candidates to reduce the inflammation and oxidative stress in the brain of AD patients. In our current study, we reported that Trolox possesses anti-inflammatory and antioxidant properties against Aβ1 − 42-induced Alzheimer's disease (AD) mouse model. Trolox reduced amyloid beta (Aβ) burden, neuroinflammation, and oxidative stress and enhanced synaptic memory and cognitive functions (Figure 8).
First, we examine the protective effects of Trolox in Aβ1 − 42-induced mice brains by performing behavioral tests, which showed that Trolox treatment in AD-induced mice significantly enhanced memory and cognitive functions. Tau protein is responsible for stabilizing microtubules to maintain the structural integrity of neurons. In AD conditions, tau protein is hyperphosphorylated, causing the formation of abnormal assemblies known as neurofibrillary tangles (NFTs). This hyperphosphorylation of tau protein causes it to separate from microtubules and then aggregate to form NFTs, which are related to neuronal dysfunction and cell death (Noble et al., 2013). Various studies have reported tau protein as a negative regulator in diseased conditions such as AD and Parkinson's disease. In AD and other tauopathies, tau is abnormally phosphorylated and results in neuronal and synaptic loss in the brain. Tau phosphorylation in the AD brain is considered by at least a three-fold increase in phosphorylation (Neddens et al., 2018). Our results showed upregulation of phosphorylated-tau (p-Tau) protein in both cortical and hippocampal regions of Aβ1 − 42-induced AD mice brain while Trolox treatment significantly reversed the p-tau expression as compared to the saline-treated mice group. Similarly, β-site amyloid precursor protein cleaving enzyme 1 (BACE1) is the vital enzyme that recruits the formation of Aβ from amyloid beta precursor protein (APP), leading to Aβ accumulation in the brain (Hajdú et al., 2023). Various conditions, such as AD hypoxia and oxidative stress, cause an increase in BACE1 expression (Cai et al., 2023). The findings of our current study exhibited elevated expression of BACE1 in the AD-induced mice. However, Trolox treatment significantly reduced the BACE1 expression.
The accumulation of Aβ in the brain is responsible for the activation of microglia and astrocytes, which further leads to neuroinflammation, oxidative stress, and neurodegeneration (Söllvander et al., 2016). We also observed a high expression level of ionized calcium-binding adaptor protein 1(Iba1), glial fibrillary acidic protein (GFAP), and other inflammatory mediators and cytokines (phosphorylated nuclear factor kappa B, interleukin 1β and Toll-like receptor 4) in AD-induced mice brains. In our current study Trolox treatment reduced the activated microglia and astrocytes and decreased the elevated inflammatory cytokines. On the other side, Aβ and neuroinflammation deteriorate the antioxidant enzymes in the brain, specifically the nuclear factor erythroid-related factor 2 (NFR2) and heme oxygenase 1 (HO1) pathway, which fights against oxidative damage. When this defense mechanism alters, it can harm brain cells, which further contributes to the progression of neurodegenerative conditions (Suzen et al., 2022). Our results showed that Trolox has strong antioxidative properties and prevents the mouse brain from oxidative stress by activating the NFR2/HO1 pathways.
Synaptic plasticity is important to the brain's development and normal functions, and its failure is a cardinal feature of AD (Mateos-Aparicio and Rodríguez-Moreno, 2019). The synaptic proteins such as post-synaptic density protein 95 (PSD-95), synaptophysin (SYN), and synaptosomal associated protein 23 (SNAP23) are essential for regulating various neurotransmitters and have been found to be modified in neurodegenerative diseases (Bereczki et al., 2018; Hsieh et al., 2006). In our study, we also found decreased expression levels of these synaptic proteins in Aβ1 − 42-induced AD mice brains, while the Trolox treatment significantly improved their expressions. In conclusion, our research findings revealed that Trolox exhibited multidimensional effects by reducing Aβ, p-tau, and BACE1 expressions both in cortical and hippocampal regions and mitigating memory, learning, and cognitive impairments in AD-induced mice. Further, it is demonstrated that Trolox has potent anti-inflammatory, antioxidative, and neuroprotective properties in AD mouse models. These findings underscore that Trolox is a promising therapeutic target for AD and related neurodegenerative disorders. However, further research studies should be needed to know the mechanistic role of Trolox in AD as well as clinical trials on humans in the future.
Data availability statement
The original contributions presented in the study are included in the article/Supplementary material, further inquiries can be directed to the corresponding author.
Ethics statement
The animal study was approved by Animal Ethics Committee of the Division of Applied Life Sciences, Department of Biology, Gyeongsang National University, South Korea. The study was conducted in accordance with the local legislation and institutional requirements.
Author contributions
MT: Conceptualization, Formal analysis, Methodology, Software, Writing – original draft, Writing – review & editing. MHK: Conceptualization, Formal analysis, Methodology, Writing – review & editing. TJP: Conceptualization, Data curation, Formal analysis, Methodology, Writing – review & editing. JA: Data curation, Formal analysis, Investigation, Writing – review & editing. KC: Data curation, Formal analysis, Methodology, Writing – review & editing. JSP: Data curation, Formal analysis, Investigation, Methodology, Writing – review & editing. MOK: Data curation, Funding acquisition, Investigation, Supervision, Validation, Writing – review & editing, Project administration.
Funding
The author(s) declare financial support was received for the research, authorship, and/or publication of this article. This research was supported by the Bio & Medical Technology Development Program of the National Research Foundation (NRF) funded by the Korean Government (MSIT) (RS-2024-00441331).
Conflict of interest
MOK was employed by Alz-Dementia Korea Co.
The remaining authors declare that the research was conducted in the absence of any commercial or financial relationships that could be construed as a potential conflict of interest.
Publisher's note
All claims expressed in this article are solely those of the authors and do not necessarily represent those of their affiliated organizations, or those of the publisher, the editors and the reviewers. Any product that may be evaluated in this article, or claim that may be made by its manufacturer, is not guaranteed or endorsed by the publisher.
Supplementary material
The Supplementary Material for this article can be found online at: https://www.frontiersin.org/articles/10.3389/fncel.2024.1453038/full#supplementary-material
References
Agostinho, C. A., and Oliveira, C. (2010). Neuroinflammation, oxidative stress and the pathogenesis of alzheimers disease. Curr. Pharm. Des. 16, 2766–2778. doi: 10.2174/138161210793176572
Ali, T., Rehman, S. U., Khan, A., Badshah, H., Abid, N. B., Kim, M. W., et al. (2021). Adiponectin-mimetic novel nonapeptide rescues aberrant neuronal metabolic-associated memory deficits in Alzheimer's disease. Mol. Neurodegener. 16, 1–22. doi: 10.1186/s13024-021-00445-4
Ali, T., Yoon, G. H., Shah, S. A., Lee, H. Y., and Kim, M. O. (2015). Osmotin attenuates amyloid beta-induced memory impairment, tau phosphorylation and neurodegeneration in the mouse hippocampus. Sci. Rep. 5, 1–17. doi: 10.1038/srep11708
Amin, F. U., Shah, S. A., and Kim, M. O. (2017). Vanillic acid attenuates Aβ1-42-induced oxidative stress and cognitive impairment in mice. Sci. Rep. 7:40753. doi: 10.1038/srep40753
Atiq, A., Lee, H. J., Khan, A., Kang, M. H., Rehman, I. U., Ahmad, R., et al. (2023). Vitamin E analog trolox attenuates MPTP-induced Parkinson's disease in mice, mitigating oxidative stress, neuroinflammation, and motor impairment. Int. J. Mol. Sci. 24:9942. doi: 10.3390/ijms24129942
Badshah, H., Ikram, M., Ali, W., Ahmad, S., Hahm, J. R., and Kim, M. O. (2019). Caffeine may abrogate LPS-induced oxidative stress and neuroinflammation by regulating Nrf2/TLR4 in adult mouse brains. Biomolecules 9:719. doi: 10.3390/biom9110719
Behl, C., Davis, J. B., Lesley, R., and Schubert, D. (1994). Hydrogen peroxide mediates amyloid β protein toxicity. Cell 77, 817–827. doi: 10.1016/0092-8674(94)90131-7
Bereczki, E., Branca, R. M., Francis, P. T., Pereira, J. B., Baek, J. H., Hortobágyi, T., et al. (2018). Synaptic markers of cognitive decline in neurodegenerative diseases: a proteomic approach. Brain 141, 582–595. doi: 10.1093/brain/awx352
Cai, W., Li, L., Sang, S., Pan, X., and Zhong, C. (2023). Physiological roles of β-amyloid in regulating synaptic function: implications for AD pathophysiology. Neurosci. Bull. 39, 1289–1308. doi: 10.1007/s12264-022-00985-9
Chen, Y., Wang, B., Liu, D., Li, J. J., Xue, Y., Sakata, K., et al. (2014). Hsp90 chaperone inhibitor 17-AAG attenuates Aβ-induced synaptic toxicity and memory impairment. J. Neurosci. 34, 2464–2470. doi: 10.1523/JNEUROSCI.0151-13.2014
Chuang, K.-A., Li, M. H., Lin, N. H., Chang, C. H., Lu, I. H., Pan, I. H., et al. (2017). Rhinacanthin c alleviates amyloid-β fibrils' toxicity on neurons and attenuates neuroinflammation triggered by lps, amyloid-β, and interferon-γ in glial cells. Oxid. Med. Cell. Longev. 2017:5414297. doi: 10.1155/2017/5414297
Denny, A., Bartley, K., Edwards, S., Webbe, F., and LoGalbo, A. (2021). AD8 patient–informant discrepancy predicts insight and cognitive impairment in Alzheimer's disease. Geriatr. Nurs. 42, 262–267. doi: 10.1016/j.gerinurse.2020.08.009
El-Sayed, A. F. M., and Izquierdo, M. (2022). The importance of vitamin E for farmed fish—A review. Rev. Aquacult. 14, 688–703. doi: 10.1111/raq.12619
Forrest, V. J., Kang, Y. H., McClain, D. E., Robinson, D. H., and Ramakrishnan, N. (1994). Oxidative stress-induced apoptosis prevented by Trolox. Free Radical Biol. Med. 16, 675–684. doi: 10.1016/0891-5849(94)90182-1
Giordano, M. E., Caricato, R., and Lionetto, M. G. (2020). Concentration dependence of the antioxidant and prooxidant activity of trolox in hela cells: Involvement in the induction of apoptotic volume decrease. Antioxidants 9:1058. doi: 10.3390/antiox9111058
Godoy, J. A., Rios, J. A., Zolezzi, J. M., Braidy, N., and Inestrosa, N. C. (2014). Signaling pathway cross talk in Alzheimer's disease. Cell Commun. Signal. 12, 1–12. doi: 10.1186/1478-811X-12-23
Guo, C., He, Z., Wen, L., Zhu, L., Lu, Y., Deng, S., et al. (2012). Cytoprotective effect of trolox against oxidative damage and apoptosis in the NRK-52e cells induced by melamine. Cell Biol. Int. 36, 183–188. doi: 10.1042/CBI20110036
Hajdú, I., Végh, B. M., Szilágyi, A., and Závodszky, P. (2023). Beta-secretase 1 recruits amyloid-beta precursor protein to ROCK2 kinase, resulting in erroneous phosphorylation and beta-amyloid plaque formation. Int. J. Mol. Sci. 24:10416. doi: 10.3390/ijms241310416
Hsieh, H., Boehm, J., Sato, C., Iwatsubo, T., Tomita, T., Sisodia, S., et al. (2006). AMPAR removal underlies Aβ-induced synaptic depression and dendritic spine loss. Neuron 52, 831–843. doi: 10.1016/j.neuron.2006.10.035
Huang, D., Ou, B., and Prior, R. L. (2005). The chemistry behind antioxidant capacity assays. J. Agric. Food Chem. 53, 1841–1856. doi: 10.1021/jf030723c
Huang, Y., and Mucke, L. (2012). Alzheimer mechanisms and therapeutic strategies. Cell 148, 1204–1222. doi: 10.1016/j.cell.2012.02.040
Ikram, M., Muhammad, T., Rehman, S. U., Khan, A., Jo, M. G., Ali, T., et al. (2019). Hesperetin confers neuroprotection by regulating Nrf2/TLR4/NF-κB signaling in an Aβ mouse model. Mol. Neurobiol. 56, 6293–6309. doi: 10.1007/s12035-019-1512-7
Khan, A., Ali, T., Rehman, S. U., Khan, M. S., Alam, S. I., Ikram, M., et al. (2018). Neuroprotective effect of quercetin against the detrimental effects of LPS in the adult mouse brain. Front. Pharmacol. 9:1383. doi: 10.3389/fphar.2018.01383
Khan, A., Ikram, M., Muhammad, T., Park, J., and Kim, M. O. (2019). Caffeine modulates cadmium-induced oxidative stress, neuroinflammation, and cognitive impairments by regulating Nrf-2/HO-1 in vivo and in vitro. J. Clin. Med. 8:680. doi: 10.3390/jcm8050680
Khan, A., Park, J. S., Kang, M. H., Lee, H. J., Ali, J., Tahir, M., et al. (2023). Caffeic acid, a polyphenolic micronutrient rescues mice brains against Aβ-induced neurodegeneration and memory impairment. Antioxidants 12:1284. doi: 10.3390/antiox12061284
Khan, A., Park, T. J., Ikram, M., Ahmad, S., Ahmad, R., Jo, M. G., et al. (2021). Antioxidative and anti-inflammatory effects of kojic acid in aβ-induced mouse model of Alzheimer's disease. Mol. Neurobiol. 58, 5127–5140. doi: 10.1007/s12035-021-02460-4
Kim, M. S., Hur, D., Lee, S., Jeaong, E., Jeong, H., and Hong, S. (2017). Effects of vitamin E with different levels or sources of dietary lipid on the growth and expression of inflammatory, oxidative stress, and apoptotic genes in the head kidney of olive flounder, Paralichthys olivaceus. J. World Aquac. Soc. 48, 518–530. doi: 10.1111/jwas.12359
Kim, Y. S., Jung, H. M., and Yoon, B.-E. (2018). Exploring glia to better understand Alzheimer's disease. Animal Cells Syst. 22, 213–218. doi: 10.1080/19768354.2018.1508498
Koppal, T., Subramaniam, R., Drake, J., Prasad, M. R., Dhillon, H., and Butterfield, D. A. (1998). Vitamin E protects against Alzheimer's amyloid peptide (25–35)-induced changes in neocortical synaptosomal membrane lipid structure and composition. Brain Res. 786, 270–273. doi: 10.1016/S0006-8993(97)01466-2
Kumar, R. R., Singh, L., Thakur, A., Singh, S., and Kumar, B. (2022). Role of vitamins in neurodegenerative diseases: a review. CNS Neurol. Disord.-Drug Targ. 21, 766–773. doi: 10.2174/1871527320666211119122150
Leissring, M. A., Murphy, M. P., Mead, T. R., Akbari, Y., Sugarman, M. C., Jannatipour, M., et al. (2002). A physiologic signaling role for the γ-secretase-derived intracellular fragment of AP. Proc. Nat. Acad. Sci. 99, 4697–4702. doi: 10.1073/pnas.072033799
Liang, B., Duan, B.-Y., Zhou, X.-P., Gong, J.-X., and Luo, Z.-G. (2010). Calpain activation promotes BACE1 expression, amyloid precursor protein processing, and amyloid plaque formation in a transgenic mouse model of Alzheimer disease. J. Biol. Chem. 285, 27737–27744. doi: 10.1074/jbc.M110.117960
Lúcio, M., Nunes, C., Gaspar, D., Ferreira, H., Lima, J., and Reis, S. (2009). Antioxidant activity of vitamin E and Trolox: understanding of the factors that govern lipid peroxidation studies in vitro. Food Biophys. 4, 312–320. doi: 10.1007/s11483-009-9129-4
Massey, K. D., and Burton, K. (1990). Free radical damage in neonatal rat cardiac myocyte cultures: effects of α-tocopherol, Trolox, and phytol. Free Radical Biol. Med. 8, 449–458. doi: 10.1016/0891-5849(90)90058-Q
Mateos-Aparicio, P., and Rodríguez-Moreno, A. (2019). The impact of studying brain plasticity. Front. Cell. Neurosci. 13:66. doi: 10.3389/fncel.2019.00066
Messier, E. M., Bahmed, K., Tuder, R. M., Chu, H. W., Bowler, R. P., and Kosmider, B. (2013). Trolox contributes to Nrf2-mediated protection of human and murine primary alveolar type II cells from injury by cigarette smoke. Cell Death Dis. 4, e573–e573. doi: 10.1038/cddis.2013.96
Miranda, S., Opazo, C., Larrondo, L. F., Muñoz, F. J., Ruiz, F., Leighton, F., et al. (2000). The role of oxidative stress in the toxicity induced by amyloid β-peptide in Alzheimer's disease. Prog. Neurobiol. 62, 633–648. doi: 10.1016/S0301-0082(00)00015-0
Muhammad, T., Ali, T., Ikram, M., Khan, A., Alam, S. I., and Kim, M. O. (2019). Melatonin rescue oxidative stress-mediated neuroinflammation/neurodegeneration and memory impairment in scopolamine-induced amnesia mice model. J. Neuroimmune Pharmacol. 14, 278–294. doi: 10.1007/s11481-018-9824-3
Neddens, J., Temmel, M., Flunkert, S., Kerschbaumer, B., Hoeller, C., Loeffler, T., et al. (2018). Phosphorylation of different tau sites during progression of Alzheimer's disease. Acta Neuropathol. Commun. 6, 1–15. doi: 10.1186/s40478-018-0557-6
Noble, W., Hanger, D. P., Miller, C. C., and Lovestone, S. (2013). The importance of tau phosphorylation for neurodegenerative diseases. Front. Neurol. 4:83. doi: 10.3389/fneur.2013.00083
Rawat, P., Sehar, U., Bisht, J., Selman, A., Culberson, J., and Reddy, P. H. (2022). Phosphorylated tau in Alzheimer's disease and other tauopathies. Int. J. Mol. Sci. 23:12841. doi: 10.3390/ijms232112841
Rengaraj, D., and Hong, Y. H. (2015). Effects of dietary vitamin E on fertility functions in poultry species. Int. J. Mol. Sci. 16, 9910–9921. doi: 10.3390/ijms16059910
Sattler, S. E., Cahoon, E. B., Coughlan, S. J., and DellaPenna, D. (2003). Characterization of tocopherol cyclases from higher plants and cyanobacteria. Evolutionary implications for tocopherol synthesis and function. Plant Physiol. 132, 2184–2195. doi: 10.1104/pp.103.024257
Schieber, M., and Chandel, N. S. (2014). ROS function in redox signaling and oxidative stress. Curr. Biol. 24, R453–R462. doi: 10.1016/j.cub.2014.03.034
Sharma, S. S., and Sayyed, S. G. (2006). Effects of trolox on nerve dysfunction, thermal hyperalgesia and oxidative stress in experimental diabetic neuropathy. Clini. Exp. Pharmacol. Physiol. 33:11. doi: 10.1111/j.1440-1681.2006.04481.x
Söllvander, S., Nikitidou, E., Brolin, R., Söderberg, L., Sehlin, D., Lannfelt, L., et al. (2016). Accumulation of amyloid-β by astrocytes result in enlarged endosomes and microvesicle-induced apoptosis of neurons. Mol. Neurodegener. 11, 1–19. doi: 10.1186/s13024-016-0098-z
Stefanova, N. A., Maksimova, K. Y., Kiseleva, E., Rudnitskaya, E. A., Muraleva, N. A., and Kolosova, N. G. (2015). Melatonin attenuates impairments of structural hippocampal neuroplasticity in OXYS rats during active progression of A lzheimer's disease-like pathology. J. Pineal Res. 59, 163–177. doi: 10.1111/jpi.12248
Suzen, S., Tucci, P., Profumo, E., Buttari, B., and Saso, L. (2022). A pivotal role of Nrf2 in neurodegenerative disorders: a new way for therapeutic strategies. Pharmaceuticals 15:692. doi: 10.3390/ph15060692
Vassar, R. (2014). BACE1 inhibitor drugs in clinical trials for Alzheimer's disease. Alzheimer's Res. Therapy 6, 1–14. doi: 10.1186/s13195-014-0089-7
Xu, A., Shang-Guan, J., Li, Z., Huang, Z., Shi, S., and Ye, Y. (2023). Effects of dietary vitamin E on the growth performance, immunity and digestion of Epinephelus fuscoguttatus♀ × Epinephelus lanceolatus♂ by physiology, pathology and RNA-seq. Aquaculture 2023:739752. doi: 10.1016/j.aquaculture.2023.739752
Yan, R., and Vassar, R. (2014). Targeting the β secretase BACE1 for Alzheimer's disease therapy. Lancet Neurol. 13, 319–329. doi: 10.1016/S1474-4422(13)70276-X
Zhang, Q., Liu, J., Duan, H., Li, R., Peng, W., and Wu, C. (2021). Activation of Nrf2/HO-1 signaling: An important molecular mechanism of herbal medicine in the treatment of atherosclerosis via the protection of vascular endothelial cells from oxidative stress. J. Adv. Res. 34, 43–63. doi: 10.1016/j.jare.2021.06.023
Keywords: Alzheimer's disease (AD), amyloid beta plaques (Aβ), neurofibrillary tangles (NFTs), Trolox, neuroinflammation, oxidative stress and neurodegeneration
Citation: Tahir M, Kang MH, Park TJ, Ali J, Choe K, Park JS and Kim MO (2024) Multifaceted neuroprotective approach of Trolox in Alzheimer's disease mouse model: targeting Aβ pathology, neuroinflammation, oxidative stress, and synaptic dysfunction. Front. Cell. Neurosci. 18:1453038. doi: 10.3389/fncel.2024.1453038
Received: 22 June 2024; Accepted: 23 August 2024;
Published: 17 September 2024.
Edited by:
Giulio Nittari, University of Camerino, ItalyReviewed by:
Nataraj Jagadeesan, Chapman University, United StatesSoumyabrata Banerjee, Vidyasagar University, India
Copyright © 2024 Tahir, Kang, Park, Ali, Choe, Park and Kim. This is an open-access article distributed under the terms of the Creative Commons Attribution License (CC BY). The use, distribution or reproduction in other forums is permitted, provided the original author(s) and the copyright owner(s) are credited and that the original publication in this journal is cited, in accordance with accepted academic practice. No use, distribution or reproduction is permitted which does not comply with these terms.
*Correspondence: Myeong Ok Kim, mokim@gnu.ac.kr
†These authors have contributed equally to this work