- 1Department of Neurosurgery, University of Maryland School of Medicine, Baltimore, MD, United States
- 2The College of Arts and Sciences, Cornell University, Ithaca, NY, United States
- 3Department of Neurosurgery, University of Michigan, Ann Arbor, MI, United States
- 4Surgical Neurology Branch, National Institute of Neurological Disorders and Stroke (NINDS), National Institutes of Health, Bethesda, MD, United States
Glioma-related epilepsy (GRE) is a hallmark clinical presentation of gliomas with significant impacts on patient quality of life. The current standard of care for seizure management is comprised of anti-seizure medications (ASMs) and surgical resection. Seizures in glioma patients are often drug-resistant and can often recur after surgery despite total tumor resection. Therefore, current research is focused on the pro-epileptic pathological changes occurring in tumor cells and the peritumoral environment. One important contribution to seizures in GRE patients is metabolic reprogramming in tumor and surrounding cells. This is most evident by the significantly heightened seizure rate in patients with isocitrate dehydrogenase mutated (IDHmut) tumors compared to patients with IDH wildtype (IDHwt) gliomas. To gain further insight into glioma metabolism in epileptogenesis, this review compares the metabolic changes inherent to IDHmut vs. IDHwt tumors and describes the pro-epileptic effects these changes have on both the tumor cells and the peritumoral environment. Understanding alterations in glioma metabolism can help to uncover novel therapeutic interventions for seizure management in GRE patients.
1. Introduction
Approximately 90% of patients with low-grade gliomas (LGG) and 50% with high-grade gliomas (HGG) experience glioma-related epilepsy (GRE), defined by the occurrence of spontaneous seizures with clear glioma correlation (Prakash et al., 2012; You et al., 2020). The presence of seizures in a glioma patient is a positive predictor of reduced mortality (Vecht et al., 2014; Ge et al., 2022). However, worsening seizures, specifically seizure recurrence following a period of seizure freedom or increased seizure frequency, is a positive predictor of glioma progression in patients with established GRE (Smits and Duffau, 2011; Prakash et al., 2012). This is supported by recent findings suggesting that gliomas, and other brain tumors, promote epileptogenesis in the surrounding peritumoral brain tissue through structural alterations of existing neural connections and through molecules released by tumors (Adhikari, 2021; Komiyama et al., 2022). Furthermore, recent observations from intracranial electrocorticography (ECoG) studies in glioma patients have shown that seizure onset zones (SOZs) are often located 1.5 cm beyond the tumor margin (Mittal et al., 2016). Thus, understanding the impact of gliomas on the peritumoral environment will allow us to better elucidate the pathophysiology of GRE and inform targeted therapeutics.
The peritumoral neuronal hyperexcitability associated with gliomas has been attributed to a large number of factors such as mass effect, disruption of ion homeostasis, blood brain barrier breakdown, neuroinflammation, genetic mutations, and metabolic alterations (Hills et al., 2022; Goethe et al., 2023; Tobochnik et al., 2023). One major mechanism through which tumors promote epileptogenicity is by metabolic reprogramming in tumor cells and in the peritumoral environment. Glioma cells require a significant energy supply to sustain a higher metabolic rate (Bi et al., 2020). In response to this increased energy demand, tumor cells reprogram their carbohydrate metabolism to primarily utilize glycolysis in lieu of oxidative phosphorylation, regardless of tissue oxygenation. This phenomenon is known as aerobic glycolysis, or the Warburg effect, and results in more rapid adenosine triphosphate (ATP) production. In addition to providing an energy source, metabolic changes in tumoral cells can impact the peritumoral environment through the release of downstream metabolic byproducts, as well as through other alterations such as relative peritumoral hypoxia (You et al., 2012; Mortazavi et al., 2022; Giambra et al., 2023).
In the context of gliomas, metabolic reprogramming in tumor and peritumoral cells has significant clinical implications in patient symptomology and prognosis. One example of this is the identification of mutations in isocitrate dehydrogenase 1 (IDH1) and/or 2 (IDH2) as critical biomarkers for glioma classification and prognosis (Weller et al., 2015). Interestingly, IDHmut glioma patients having a preoperative seizure rate of 59-74%, compared to 18-34% seen in IDHwt glioma patients (Chen et al., 2017). The higher frequency of seizures in IDHmut tumors suggests that understanding the metabolic differences between IDHwt and IDHmut glioma cells could reveal new mechanisms causing epilepsy and lead to targeted advancements in GRE detection and seizure management. This review will focus specifically on the metabolic reprogramming that occurs in tumoral cells based on IDH mutation status (Figure 1) and describe the corresponding pro-epileptic effects on the surrounding brain tissue.
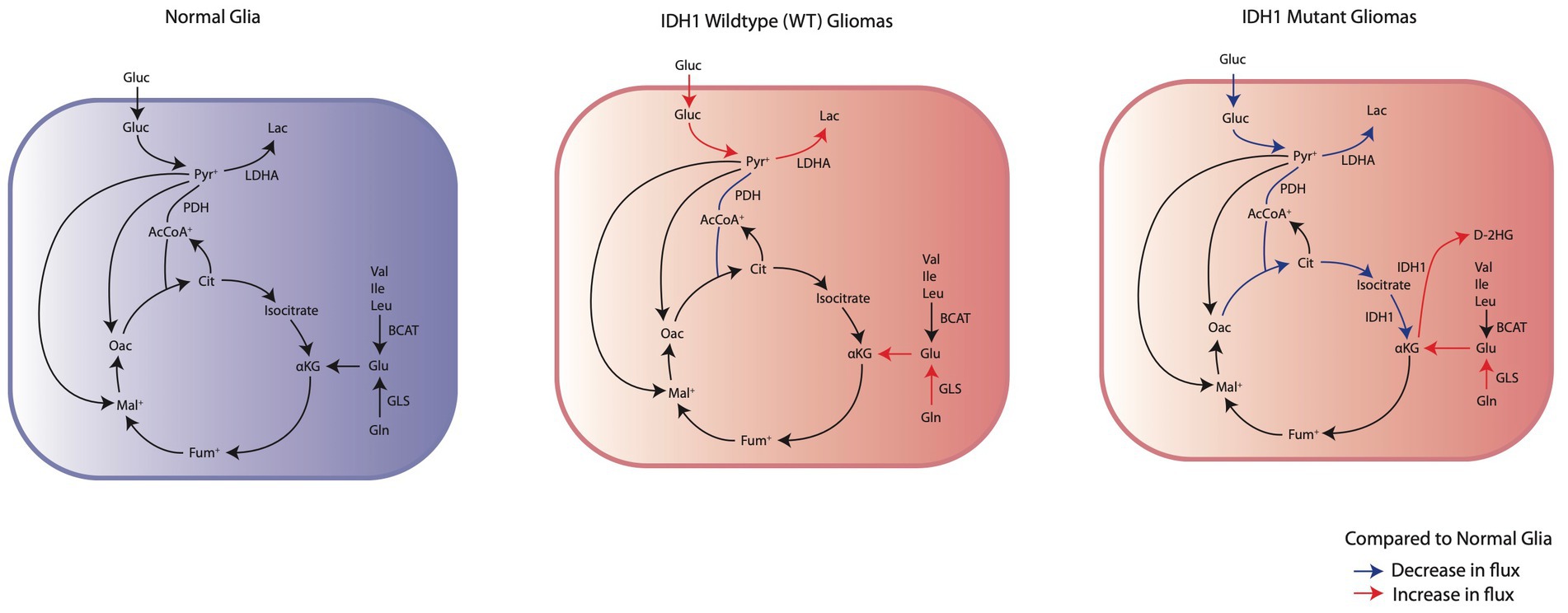
Figure 1. Comparison of the changes in IDHwt glioma cells and IDH1mut glioma cells energy metabolism to normal glia. IDHwt glioma cells have an upregulation of aerobic glycolysis through increased glucose consumption and LDHA expression. IDH1mut glioma cells demonstrate suppression of glycolysis and subsequent reliance on oxidative phosphorylation through glutaminolysis for anaplerosis.
2. Pro-seizure metabolic reprogramming in isocitrate dehydrogenase wildtype glioma
Increased energy needs in glioma cells contribute to epileptogenicity by altering the metabolism of both tumor and surrounding cells. Stabilization of hypoxia inducible factor 1α (HIF-1α) is a primary metabolic response in IDHwt cells (Strickland and Stoll, 2017). While hypoxia usually activates HIF-1α, in gliomas, HIF-1α stabilizes even before hypoxia occurs in the tumor or surrounding cells, indicating its independence from hypoxia (Woolf and Scheck, 2012; Strickland and Stoll, 2017). This stabilization of HIF-1α fosters metabolic adjustments, including higher glucose uptake and increased aerobic glycolysis, while reducing oxidative phosphorylation (Rempel et al., 1996).
In normal glucose metabolism, glucose enters neurons mainly through facilitated diffusion glucose transporters (GLUTs) and is converted to pyruvate through a series of glycolytic steps (Yu et al., 2016). Pyruvate is then preferentially converted to acetyl-CoA by pyruvate dehydrogenase (PDH) which enters the tricarboxylic acid (TCA) cycle to generate electron carriers NADH and FADH2 for ATP production by the electron transport chain through oxidative phosphorylation (Yu et al., 2016). Normally, in anaerobic conditions, pyruvate can also be converted to lactate through the enzyme lactate dehydrogenase (LDH) to produce NAD+ needed to sustain glycolytic production of ATP (Yu et al., 2016). In glioma cells, however, HIF-1α stabilization causes pyruvate to be preferentially converted to lactate, even in aerobic conditions (Figure 2; Yu et al., 2016; Han et al., 2020). HIF-1α stabilization induces inactivation of PDH through increased phosphorylation by pyruvate dehydrogenase kinase (PDK) and upregulation of LDHA, the subunit of LDH that preferentially converts pyruvate to lactate (Kim et al., 2006, 2015; Strickland and Stoll, 2017; Han et al., 2020). This causes glioma cells to preferentially utilize glycolysis for ATP production in lieu of the TCA cycle and oxidative phosphorylation. Furthermore, HIF-1α stabilization also upregulates GLUT1 and GLUT3 transporter expression and phosphofructokinase (PFK1) expression, allowing for elevated glucose uptake, increased glycolytic capacity, and rapid production of the ATP needed to sustain the high metabolic demand of glioma cells (Han et al., 2020). In addition to the shunting of pyruvate away from the TCA cycle, the electron transport chain (ETC) required for oxidative phosphorylation is impaired in hypoxic glioma cells (Papandreou et al., 2006; Grassian et al., 2014). Dysfunction of all four ETC complexes is implicated in glioblastoma, whereas often complexes II and IV are preferentially involved in low grade gliomas (Strickland and Stoll, 2017). Similarly, these impairments are also reported in epilepsy patients with temporal lobe epilepsy (TLE), suggesting that ETC impairment may play a role in GRE (Waldbaum and Patel, 2010).
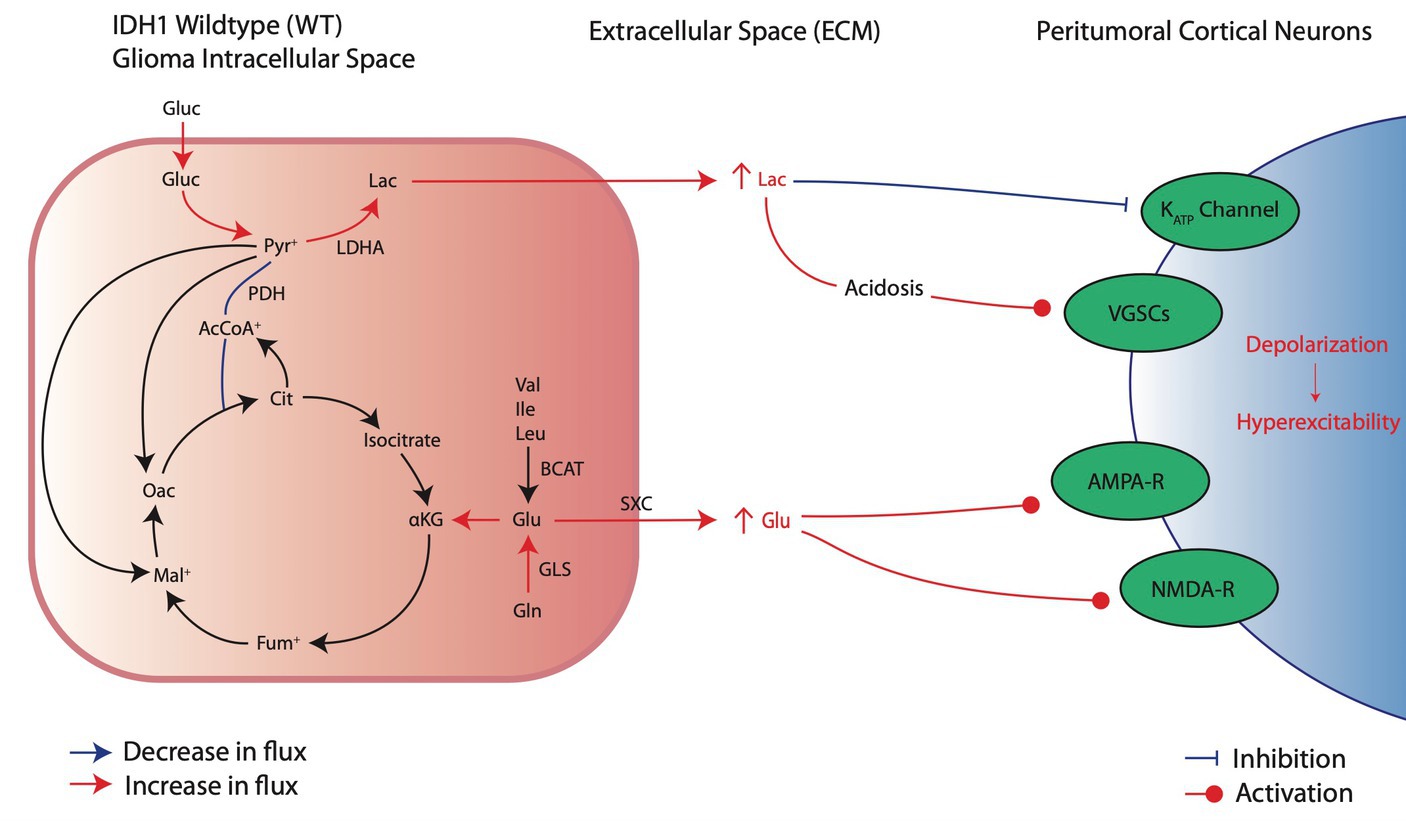
Figure 2. Metabolic reprogramming in IDHwt glioma cells and their pro-convulsive effects. IDHwt cells upregulate aerobic glycolysis and increase extracellular lactate through increased LDHA activity, leading to local acidosis and peritumoral neuronal depolarization of local cortical neurons. In addition, increased glutamate production and release induces local activation of excitatory receptors AMPA and NMDA.
Metabolic shifts in glioma cells, such as LDHA upregulation, lead to pro-epileptic effects by releasing metabolites like lactate into the surrounding area, which induces excitatory changes in nearby neurons (Figure 2; Valvona et al., 2016). Lactate’s role in causing hyperexcitability is indicated by mechanisms also found in epilepsy (Angamo et al., 2017). In both tumoral cells and epileptic neurons, metabolic reprogramming mediated by HIF-1α leads to increased LDHA levels, boosting lactate production and release into the extracellular environment (Sada et al., 2020; Ksendzovsky et al., 2023). Furthermore, although still an area of active investigation, there is literature to suggest that LDHA inhibition may reduce seizure susceptibility (Sada et al., 2015). In the context of gliomas, increased lactate release by tumor cells may similarly contribute to seizure initiation. Although controversial in current literature, substantial evidence suggests that extracellular lactate elevations can induce peritumoral neuron hyperexcitation (Beaumont and Whittle, 2000; Schaller, 2005; Shamji et al., 2009; You et al., 2012). Specifically, the acidic environment that results from lactic acid buildup has been associated with neuronal membrane depolarization through increased voltage-gated sodium channel (VGSC) activation (Rudà et al., 2012; Monteiro et al., 2017; Stanke et al., 2021). Additionally, lactate may promote seizures through closure of ATP-sensitive potassium (KATP) channels that normally act as an energy sensor to protect neurons from excessive depolarization (Karagiannis et al., 2021). Lactate is also often the initial fuel source during seizure activity; thus, excess lactate in the peritumoral environment may increase available energy and sustain increased activity in healthy peritumoral cells (Yang et al., 2013).
In addition to lactate, glutamate is another key metabolite released by glioma cells into the peritumoral environment in response to metabolic reprogramming (Figure 2). Although glycolysis becomes the main fate of glucose metabolism after metabolic reprogramming, glioma cells also increasingly rely on glutaminolysis to maintain some TCA cycle activity (Maus and Peters, 2017; Strickland and Stoll, 2017). The deamination of glutamine by glutaminase (GLS) produces glutamate, which then undergoes a series of reactions to replenish depleted TCA cycle substrates, through generation of α-ketoglutarate (αKG), and continue oxidative phosphorylation (Maus and Peters, 2017). Compared to normal astrocytes, glioma cells express increased levels of GLS and produce large amounts of glutamate (Yao et al., 2014). Additionally, glutamate can be synthesized from branched amino acids (valine, leucine, and isoleucine) by branched-chain amino acid transaminases (BCAT; Maus and Peters, 2017). Both pathways lead to increased intracellular glutamate synthesis for TCA cycle anaplerosis. Excess glutamate is released by glioma cells through system xc- (SXC), an amino acid antiporter that exchanges intracellular glutamate for extracellular cysteine (Maus and Peters, 2017). Intracellular cysteine can be used for glutathione (GSH) production for protection against ROS (Maus and Peters, 2017). Thus, it is favorable for glioma cells to upregulate SXC activity at the expense of releasing excess glutamate generated due to metabolic reprogramming into the extracellular environment. The overall result is excessive extracellular glutamate that can lead to increased tumor proliferation (Lange et al., 2021), neuronal hyperexcitability, and seizures (Robert and Sontheimer, 2014).
Besides causing metabolic shifts in glioma cells, tumoral HIF-1α stabilization also triggers pro-convulsive alterations in the surrounding tissue. Specifically, HIF-1α-induced VEGF signaling stimulates local angiogenesis to nourish both the tumor and adjacent brain areas (Schaller, 2005; Colwell et al., 2017; Monteiro et al., 2017; Strickland and Stoll, 2017). Despite neovascularization, the tumor and surrounding tissue remain relatively hypoxic due to the new vascular supply’s disorganization and fragility (Dubois et al., 2014; Wang et al., 2021). Insufficient oxygen delivery in these regions hampers oxidative phosphorylation, leading to cellular hypoxia in tumor and surrounding cells, and further stabilizing HIF-1α (Kaur et al., 2005). Fragility in newly formed peritumoral vessels also leads to focal BBB permeability changes, which can promote seizure activity through vasogenic edema, inflammatory changes, and other alterations that affect ion balance in neurons (Dubois et al., 2014; Adhikari, 2021). Additionally, poor tissue perfusion also results in hypoxia and a further energy deficit ultimately leading to sodium-potassium pump (Na+-K+-ATPase) dysfunction (Gusarova et al., 2011; Xu and Fan, 2022). The Na-K-ATPase is the key transporter maintaining neuronal resting membrane potential; therefore, loss of proper Na+-K+-ATPase function leads to a more depolarized state in peritumoral neurons and increased probability of neurons to reach action potential threshold, and thus pathologic hyperactivity (Bazzigaluppi et al., 2017; Xu and Fan, 2022). Lastly, hypoxia in glioma cells is linked to excitatory effects in the peritumoral environment through expression of thrombospondins (TSP) (Wang et al., 2021). TSPs are a family of signaling molecules that regulate synaptogenesis and plasticity and are released by glial cells in ischemia (He et al., 2022). Similarly, TSP2 expression is significantly elevated in human glioma cells with marginal increases noted in the peritumoral environment, corresponding to hyperexcitability and increased excitatory synapse formation in peritumoral neuronal populations (Wang et al., 2021). Overall, HIF-1α stabilization in glioma cells induces hypoxic alterations in the peritumoral environment which lead to a pro-convulsive state.
3. Pro-seizure metabolic reprogramming in isocitrate dehydrogenase mutant glioma
Patients with IDHmut gliomas have an increased propensity to develop GRE (preoperative seizure rate of 59-74%) compared to IDHwt (18-34%) (Liubinas et al., 2014; Chen et al., 2017). In addition to the tumor-specific mechanisms described above for IDHwt glioma cells, there are metabolic adaptations seen in IDHmut gliomas that are distinct from those in IDHwt gliomas (Figure 3). The IDH complex is usually composed of either a homodimerized complex of IDH1 subunits, in the cytoplasm, or IDH2 subunits, in the mitochondria (Han et al., 2020). In addition, there are heterotetrametric complexes that include IDH3 that also reside in the mitochondria (Yan et al., 2009). Both IDH1 and IDH2 reversibly oxidize isocitrate to αKG and reduce NADP+ to NADPH in the process (Dang et al., 2009; Han et al., 2020). However, mutations in either IDH1 and IDH2 decrease the binding affinity of isocitrate, increase the affinity for NADPH, and promote an irreversible neoplastic reaction that oxidizes NADPH to convert αKG to the oncometabolite D-2-Hydroxglutarate (D2HG) (Lu et al., 2012). Clinically, the vast majority (~95%) of mutations are in IDH1 rather than IDH2 (Hartmann et al., 2009). Therefore, most studies have focused on IDH1mut metabolic reprogramming as opposed to IDH2. IDH1mut cells can demonstrate greater than 25-fold upregulation in D2HG (Grassian et al., 2014), which can lead to many intracellular and extracellular implications in cellular metabolism and peritumoral excitability.
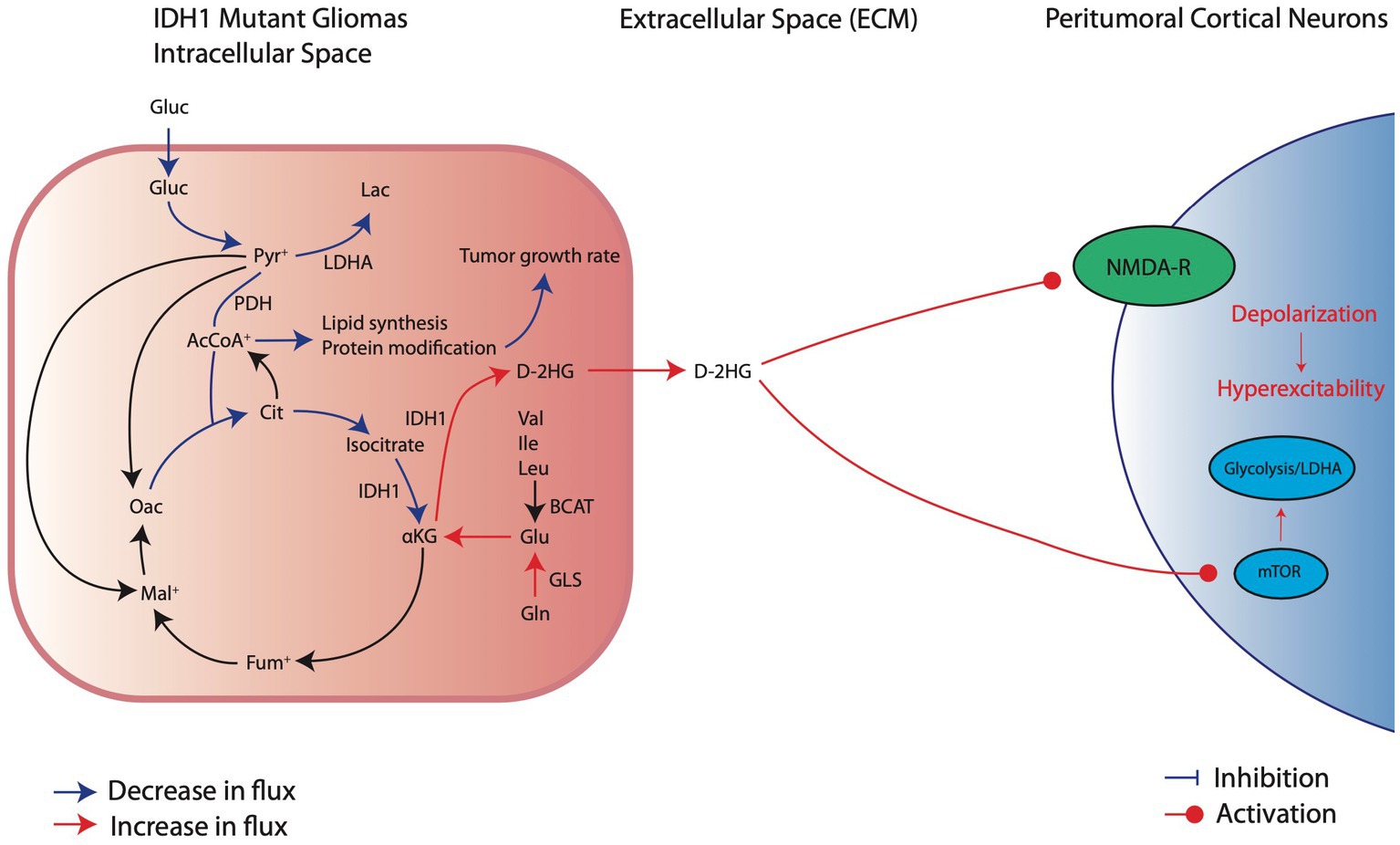
Figure 3. Metabolic reprogramming in IDH1mut glioma cells and their pro-convulsive effects. IDHmut cells have reduction in glucose consumption and downregulation of glycolytic factors, such as LDHA, which decreases carboxylic entry into the TCA cycle. Thus, IDH1mut cells rely on intracellular glutamate breakdown to aKG to sustain oxidative phosphorylation. At the same time, IDH1 mutations significantly overproduce D-2HG which exerts its pro-excitatory effects through activation of NMDA receptors and the mTOR pathway of local cortical neurons.
Unlike IDHwt glioma cells, which favor mainly glycolysis with minor contribution from glutaminolysis for energy production, IDH1mut cells have significantly increased reliance on glutamine breakdown to αKG for anaplerosis and maintenance of oxidative phosphorylation (Figure 3; Grassian et al., 2014). Mutations in IDH lead to silencing, rather than expression, of glycolysis related genes such as LDHA, CA9, and VEGFA, supporting recent findings which demonstrated a reduction in glucose consumption in IDH1mut cells (Han et al., 2020; Liu et al., 2021). This silencing effect on glycolytic genes may be due to epigenetic alterations associated with D2HG accumulation or due to decreased HIF1α stabilization in IDH1mut conditions, as opposed to increased HIF1α stabilization seen in IDHwt cells (Zhao et al., 2009). However, there is conflicting evidence on whether HIF1α expression is increased or decreased with IDHmut in glioma and more work is needed to establish the role of HIF1α in IDHmut metabolic reprogramming (Han et al., 2020).
In hypoxic conditions, IDH1mut cells exhibit slower growth compared to IDHwt cells, which maintain their growth rate, suggesting that HIF1α responsiveness is lost in IDH1mut cells (Grassian et al., 2014). This corresponds with the clinical observation that IDH mutations are present in approximately 70-80% of slower growing LGGs but only 10% of rapidly dividing glioblastomas and suggests a direct link between metabolic reprogramming and tumor growth rate (Hartmann et al., 2009; Kim et al., 2010; Turkalp et al., 2014; Louis et al., 2016). As such, IDH mutations may reduce tumor growth rate either because of reliance on a slower metabolic process in oxidative phosphorylation rather than the more rapid glycolysis, or because D2HG prevents complete reductive carboxylation of glutamine to citrate and ultimately lowers cytosolic acetyl-coA availability for cholesterol and phospholipid synthesis, amino acid modifications, and histone acetylation needed to undergo rapid cellular division (Grassian et al., 2014; Fack et al., 2017; Han et al., 2020). Some studies suggest that the slow growing nature of IDHmut gliomas may lead to seizures because it induces indolent changes, rather than rapid disruptions, in peritumoral neural network connections and allows time for reorganization of tumoral and peritumoral vasculature to occur (Adhikari, 2021). Aligning with this idea, studies have found that 90% of patients with LLG experience GRE versus up to 50% of patients with HGG, and that epilepsy incidence prior to glioma diagnosis is also increased in LGG (Prakash et al., 2012; You et al., 2012; Vecht et al., 2014; Van Opijnen et al., 2023). In fact, seizures are used an independent clinical predictor of LLG, while prolonged focal neurological changes, cognitive deficits, or headaches predict HGG (Rasmussen et al., 2017). This demonstrates that metabolic adaptations associated with IDHmut gliomas have direct implications on GRE development.
Although IDH1mut leads to reliance on oxidative phosphorylation, these tumor cells rely predominantly on glutamine and/or glutamate rather than glucose as a primary carbon source for metabolism (Figure 3; Lange et al., 2021). Because IDH1mut leads to reduced αKG for TCA function at the expense of D2HG production, these cells restore αKG by either converting glutamate directly into αKG or utilizing glutamine for αKG production indirectly by first breaking down glutamine into glutamate (Han et al., 2020). The net result of this process is that, compared to IDHwt tumors, glutamate levels in IDHmut tumors are substantially reduced (Han et al., 2020). This suggests that, unlike IDHwt cells which may promote epileptogenesis through excess glutamate release into the peritumoral environment, IDHmut cells likely do not substantially release glutamate. However, IDHmut cells may promote epileptogenesis in a similar manner through release of D2HG (Figure 3).
Glutamate and D2HG are structurally similar molecules (Figure 4), therefore when D2HG is released into the peritumoral environment, it can bind to and activate glutamate receptors in a manner similar to glutamate (Stockhammer et al., 2012; Mortazavi et al., 2022). Activation of glutamate receptors by D2HG in turn leads to hyperexcitability and synaptic plasticity, which promote seizures and epileptic network formation (Kölker et al., 2002; Cavazos and Cross, 2006; Gong et al., 2006; Chen et al., 2017). Additionally, D2HG release by IDHmut glioma cells can promote epileptogenesis independently of glutamate signaling by inducing metabolic alterations in peritumoral neurons (Mortazavi et al., 2022). Specifically, release of D2HG by tumor cells can induce aerobic glycolysis in peritumoral neurons signified by LDHA upregulation through an mTOR mediated mechanism, which in turn leads to neuronal hyperactivity and promotes seizures (Mortazavi et al., 2022). Clinically, the pro-epileptogenic effects of D2HG are supported by a related disease called 2-hydroxyglutarate-acidurea, a condition in which D2HG is elevated in the serum, cerebrospinal fluid, and urine. These patients endure persistent seizures as an initial symptom and worsen seizure burden over the disease course (Stockhammer et al., 2012; Zübarioğlu et al., 2020), suggesting that elevation of D2HG in glioma patients may contribute to GRE.
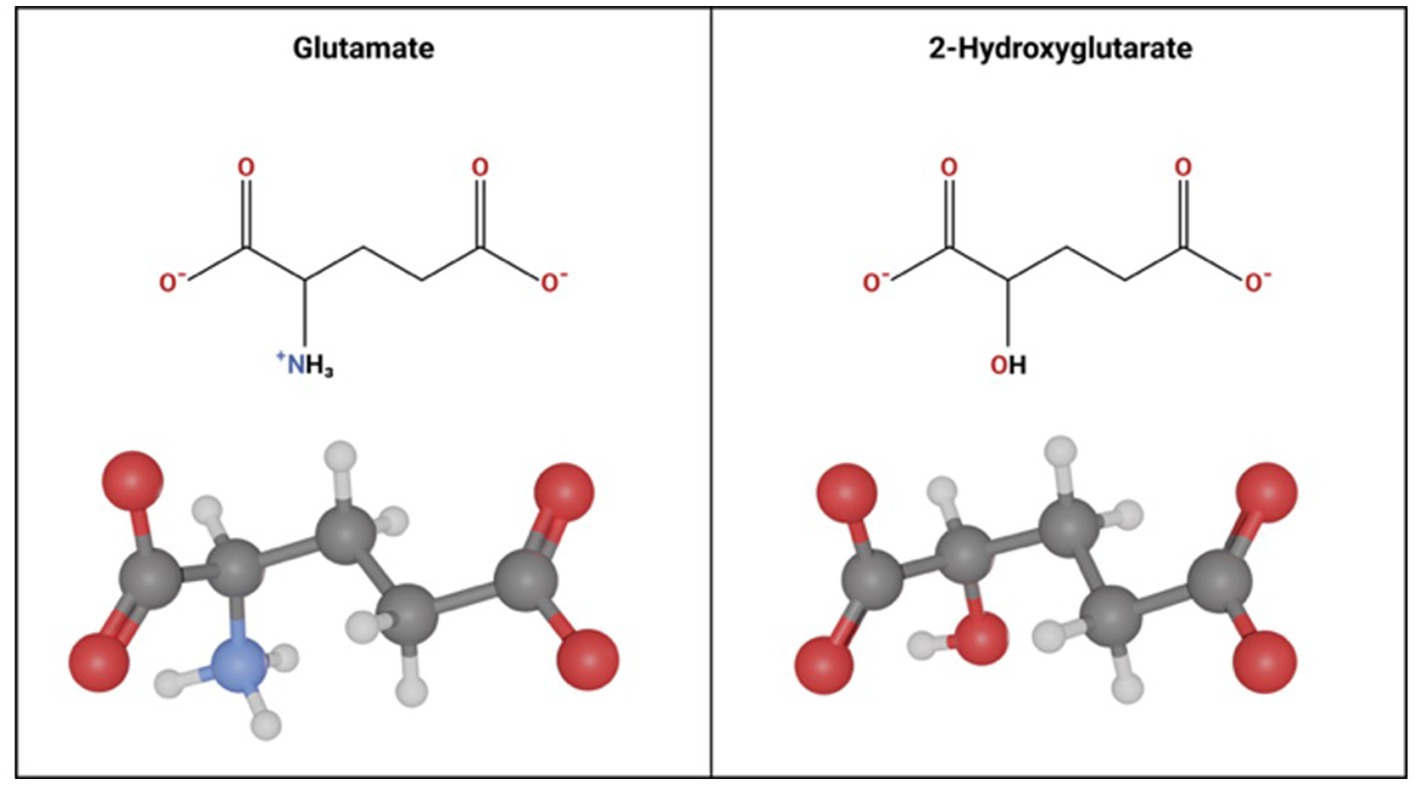
Figure 4. Molecular Similarity between glutamate and 2-hydroxyglutarate. Glutamate and 2 Hydroxyglutarate follow a similar molecular structure. The only difference is a hydroxyl group instead of an amine group on carbon-2 in 2-hydroxyglutarate.
4. Clinical implications and future metabolism-focused innovations in GRE management
Current seizure treatment in GRE involves the use of anti-seizure medications (ASM), similar to the approach for non-tumor epilepsy patients (Chen et al., 2018). However, standard ASMs do not address the root cause and are ineffective in controlling seizures for up to 50% of GRE patients (Aaronson et al., 2011; Mittal et al., 2016). Even with complete tumor resection as an alternative when ASMs fail, 30-40% of GRE patients continue to experience seizures (Aaronson et al., 2011; Mittal et al., 2016). The continued seizures after complete tumor resection suggest either residual pathological changes in the peritumoral cortex that need post-resection treatment, or the need for improved tumor localization methods. Despite understanding the role of metabolic reprogramming in GRE-related seizure susceptibility, metabolism-specific biomarkers and therapies remain underdeveloped. We propose several approaches for clinically targeting these metabolic disruptions to identify epileptic tissue, predict outcomes, and manage seizures in GRE patients.
4.1. Biomarkers and treatment of peritumoral changes in IDHmut GRE
Advancements in quantification of IDHmut specific peritumoral changes can guide GRE prognosis and monitoring. As of now, tumor biopsy is required to determine IDH genetic status. Recently, however, less invasive measures, such as positron emission tomography (PET), have been investigated to predict IDH genotype (Lohmann et al., 2018; Liu et al., 2021). Similar research is needed to predict seizure susceptibility in glioma patients, especially IDHmut glioma patients. For example, liquid chromatography–tandem mass spectrometry (MS) has been used on resected tissue to stratify patient risk for preoperative seizures based on 2HG concentration (Ohno et al., 2021). The authors identified a cutoff value of 1,190 ng/mg, above which preoperative seizures could be predicted within IDHmut glioma patients. Thus, elevated 2HG values were associated with seizures. Ongoing research is exploring less invasive methods for metabolite quantification, such as cerebrospinal fluid sampling or non-invasive imaging, to assess oncogenic metabolites released by IDHmut glioma cells into the peritumoral environment (Xiao et al., 2020; Balana et al., 2022). For example, MS of CSF fluid can detect and quantify 2HG levels and stratify patient’s with IDHmut (Kalinina et al., 2016). Additionally, magnetic resonance imaging (MRI) and hyperpolarized MRI (hpMRI) identification of D2HG accumulation has already been used for the non-invasive classification of IDHmut gliomas (Choi et al., 2012; Leather et al., 2017; Salamanca-Cardona et al., 2017; Tietze et al., 2018). The next steps involve using CSF detection to identify predictive 2HG values for predicting seizure susceptibility and employing hpMRI to non-invasively map the peritumoral cortex affected by IDHmut gliomas. This would facilitate the precise removal of epileptic zones beyond the tumor margin in GRE patients. Additionally, tracking 2HG concentrations in CSF over time could help monitor treatment efficacy and adjust ASM dosing.
While recent research findings on IDHmut pathology are promising, more clinical studies focused on targeting IDHmut to manage seizures in GRE patients are warranted. One important case report described a patient with refractory GRE due to an IDH1mut oligodendroglioma in which the IDH1 inhibitor ivosidenib was effective in reducing the patient’s seizure frequency (Vo et al., 2022). Cases like this demonstrate the potential for metabolically-targeted anti-seizure therapies in GRE. Similarly, mTOR inhibitors have been shown to reduce IDHmut glioma growth rate and D2HG levels (Batsios et al., 2019). Thus, mTOR inhibitors can potentially reduce the hyperactive effects of D2HG and may reduce seizures induced by IDHmut gliomas. All in all, because IDHmut gliomas carry the highest rate of seizures, IDH-focused therapies for GRE patients should be further investigated.
4.2. Biomarkers and treatment of peritumoral changes in IDHwt GRE
In IDHwt glioma patients, tumor cells’ increased reliance on glycolysis results in the release of two pro-convulsive metabolites, glutamate and lactate, into the peritumoral cortex. While some studies dispute elevated glutamate levels or their link to seizures, the majority of research using Magnetic Resonance Spectroscopy (MRS) or microdialysis indicates that glutamate is significantly elevated in both glioma tumors and the surrounding cortex. One study even associates elevated glutamate in these regions with seizures (Rijpkema et al., 2003; Roslin et al., 2003; Fan et al., 2004; Marcus et al., 2010; Yuen et al., 2012). This implies that, much like D2HG in IDHmut patients, glutamate could serve as a biomarker in IDHwt patients. Non-invasive imaging techniques like MRS could identify epileptic zones in the surrounding tumor tissue for surgical planning. Additionally, small studies have shown that the glutamate-sensitive AMPA receptor antagonist perampanel is effective in reducing seizures in GRE patients, suggesting that neutralizing elevated extracellular glutamate may be a viable treatment for glioma-related seizures (Vecht et al., 2017; Dunn-Pirio et al., 2018; Izumoto et al., 2018; Maschio et al., 2019; Chonan et al., 2020; Coppola et al., 2020; Lange et al., 2021). Blocking the SXC transporter responsible for glutamate release from glioma cells may also be a viable therapeutic strategy, as inhibitors of SXC have been found in preclinical epilepsy studies to be anti-convulsant (Leclercq et al., 2019). However, it remains to be seen if SXC inhibition can reduce seizure susceptibility in glioma patients.
The increased reliance on glycolysis by IDHwt cells, characterized by LDH upregulation, and the LDH upregulation seen in peritumoral cells around IDHmut tumors, resembles that seen in neurons within non-glioma epilepsy patient tissue (Ksendzovsky et al., 2023). As such, these shared mechanisms could guide novel treatments. The LDHA inhibitor stiropentol has been shown to reduce seizures in preclinical studies and is an FDA approved treatment option for seizure management in Dravet syndrome (Sada et al., 2015; Nickels and Wirrell, 2017) Although LDH inhibition has been proven to reduce tumor growth, its anti-convulsive effects in GRE patients have not yet been explored (Guyon et al., 2022). Furthermore, metabolic imaging of lactate with 13C-hpMRI, already used for tumor localization, may also be useful for mapping epileptic zones around tumors for surgical resection (Zaccagna et al., 2022).
5. Conclusion
Seizures are a common and debilitating symptom in brain tumor patients, particularly those with IDHmut low-grade gliomas. Despite treatment with ASM’s, many patients continue to experience seizures even after medication use and complete tumor removal. A growing body of literature has focused on the pro-convulsive effects of metabolic reprogramming in glioma cells and has begun to shed light on potential disease-specific targeted detection and treatment options for patients with GRE. Importantly, current evidence suggests that IDHwt and IDHmut genotypes differentially affect tumoral metabolism and consequentially have different pathological mechanisms that cause excitability in peritumoral neuronal populations. IDHwt tumors upregulate glycolysis and subsequentially release excessive glutamate and lactate into the peritumoral environment, both of which can mediate hyperexcitability in the surrounding neurons. In addition to the IDHwt mechanisms, IDHmut tumors, on the other hand, rely further on oxidative phosphorylation driven by glutamine/glutamate metabolism, which contributes to slower growth of IDHmut tumors and release of tumoral D2HG. D2HG release promotes peritumoral hyperexcitation by stimulation of glutamate receptors and induction of glycolysis in peritumoral neurons, which causes hyperactivation via lactate production similar to that seen in IDHwt tumors.
Although not the only mechanism contributing to neuroexcitability, the presence of an IDH1 mutation has demonstrated significant clinical susceptibility to seizures. Given the varying underlying pathologies due to metabolic reprogramming in tumor cells, it’s crucial to tailor diagnostic and therapeutic strategies for managing seizures in patients with IDHwt versus IDHmut tumors. Patients with IDHwt tumors may benefit more from detection of glutamate as a biomarker of epileptogenesis, whereas D2HG detection should be used in patients with IDHmut tumors. Similarly, therapies targeting glutamate release (SXC inhibitors) and action on glutamate receptors (AMPA/NMDAR receptor inhibitors) should be further explored in IDHwt patients, whereas IDHmut may benefit more from IDH inhibitors to prevent D2HG production. In addition, LDH detection and inhibitors may be useful in both IDHwt and IDHmut patients. Exploring these avenues and identifying new pro-convulsive mechanisms in tumoral metabolic reprogramming will enable clinicians to more precisely classify, monitor, and treat GRE patients, leading to earlier and improved clinical decisions that will significantly enhance patient quality of life.
Author contributions
DM: Conceptualization, Writing – original draft, Writing – review & editing. MM: Conceptualization, Writing – original draft, Writing – review & editing. JQ: Writing – original draft. AM: Conceptualization, Writing – review & editing. UB: Visualization, Writing – original draft. MB: Supervision, Writing – review & editing. KZ: Supervision, Writing – review & editing. AK: Conceptualization, Supervision, Writing – review & editing.
Funding
The author(s) declare that no financial support was received for the research, authorship, and/or publication of this article.
Conflict of interest
The authors declare that the research was conducted in the absence of any commercial or financial relationships that could be construed as a potential conflict of interest.
Publisher’s note
All claims expressed in this article are solely those of the authors and do not necessarily represent those of their affiliated organizations, or those of the publisher, the editors and the reviewers. Any product that may be evaluated in this article, or claim that may be made by its manufacturer, is not guaranteed or endorsed by the publisher.
References
Aaronson, N. K., Taphoorn, M. J. B., Heimans, J. J., Postma, T. J., Gundy, C. M., Beute, G. N., et al. (2011). Compromised health-related quality of life in patients with low-grade glioma. J. Clin. Oncol. 29, 4430–4435. doi: 10.1200/JCO.2011.35.5750
Adhikari, S. (2021). “Pathogenesis and management of brain tumor-related epilepsy” in Gliomas. eds. B. C. Walker and S. Mittal (Brisbane, AU: Exon Publications)
Angamo, E. A., ul Haq, R., Rösner, J., Gabriel, S., Gerevich, Z., Heinemann, U., et al. (2017). Contribution of intrinsic lactate to maintenance of seizure activity in neocortical slices from patients with temporal lobe epilepsy and in rat entorhinal cortex. Int. J. Mol. Sci. 18:1835. doi: 10.3390/ijms18091835
Balana, C., Castañer, S., Carrato, C., Moran, T., Lopez-Paradís, A., Domenech, M., et al. (2022). Preoperative diagnosis and molecular characterization of gliomas with liquid biopsy and Radiogenomics. Front. Neurol. 13:865171. doi: 10.3389/fneur.2022.865171
Batsios, G., Viswanath, P., Subramani, E., Najac, C., Gillespie, A. M., Santos, R. D., et al. (2019). PI3K/mTOR inhibition of IDH1 mutant glioma leads to reduced 2HG production that is associated with increased survival. Sci. Rep. 9:10521. doi: 10.1038/s41598-019-47021-x
Bazzigaluppi, P., Ebrahim Amini, A., Weisspapir, I., Stefanovic, B., and Carlen, P. L. (2017). Hungry neurons: metabolic insights on seizure dynamics. Int. J. Mol. Sci. 18:112269. doi: 10.3390/ijms18112269
Beaumont, A., and Whittle, I. R. (2000). The pathogenesis of tumour associated epilepsy. Acta Neurochir. 142, 1–15. doi: 10.1007/s007010050001
Bi, J., Chowdhry, S., Wu, S., Zhang, W., Masui, K., and Mischel, P. S. (2020). Altered cellular metabolism in gliomas - an emerging landscape of actionable co-dependency targets. Nat. Rev. Cancer 20, 57–70. doi: 10.1038/s41568-019-0226-5
Cavazos, J. E., and Cross, D. J. (2006). The role of synaptic reorganization in mesial temporal lobe epilepsy. Epilepsy Behav. 8, 483–493. doi: 10.1016/j.yebeh.2006.01.011
Chen, D. Y., Chen, C. C., Crawford, J. R., and Wang, S. G. (2018). Tumor-related epilepsy: epidemiology, pathogenesis and management. J. Neuro-Oncol. 139, 13–21. doi: 10.1007/s11060-018-2862-0
Chen, H., Judkins, J., Thomas, C., Wu, M., Khoury, L., Benjamin, C. G., et al. (2017). Mutant IDH1 and seizures in patients with glioma. Neurology 88, 1805–1813. doi: 10.1212/WNL.0000000000003911
Choi, C., Ganji, S. K., DeBerardinis, R. J., Hatanpaa, K. J., Rakheja, D., Kovacs, Z., et al. (2012). 2-hydroxyglutarate detection by magnetic resonance spectroscopy in IDH-mutated patients with gliomas. Nat. Med. 18, 624–629. doi: 10.1038/nm.2682
Chonan, M., Saito, R., Kanamori, M., Osawa, S. I., Watanabe, M., Suzuki, H., et al. (2020). Experience of low dose Perampanel to add-on in glioma patients with Levetiracetam-uncontrollable epilepsy. Neurol. Med. Chir. (Tokyo) 60, 37–44. doi: 10.2176/nmc.oa.2018-0245
Colwell, N., Larion, M., Giles, A. J., Seldomridge, A. N., Sizdahkhani, S., Gilbert, M. R., et al. (2017). Hypoxia in the glioblastoma microenvironment: shaping the phenotype of cancer stem-like cells. Neuro Oncol. 19, 887–896. doi: 10.1093/neuonc/now258
Coppola, A., Zarabla, A., Maialetti, A., Villani, V., Koudriavtseva, T., Russo, E., et al. (2020). Perampanel confirms to be effective and well-tolerated as an add-on treatment in patients with brain tumor-related epilepsy (PERADET study). Front. Neurol. 11:592. doi: 10.3389/fneur.2020.00592
Dang, L., White, D. W., Gross, S., Bennett, B. D., Bittinger, M. A., Driggers, E. M., et al. (2009). Cancer-associated IDH1 mutations produce 2-hydroxyglutarate. Nature 462, 739–744. doi: 10.1038/nature08617
Dubois, L. G., Campanati, L., Righy, C., D’Andrea-Meira, I., Spohr, T. C., Porto-Carreiro, I., et al. (2014). Gliomas and the vascular fragility of the blood brain barrier. Front. Cell. Neurosci. 8:418. doi: 10.3389/fncel.2014.00418
Dunn-Pirio, A. M., Woodring, S., Lipp, E., Herndon, J. E., Healy, P., Weant, M., et al. (2018). Adjunctive perampanel for glioma-associated epilepsy. Epilepsy Behav. Case Rep. 10, 114–117. doi: 10.1016/j.ebcr.2018.09.003
Fack, F., Tardito, S., Hochart, G., Oudin, A., Zheng, L., Fritah, S., et al. (2017). Altered metabolic landscape in IDH-mutant gliomas affects phospholipid, energy, and oxidative stress pathways. EMBO Mol. Med. 9, 1681–1695. doi: 10.15252/emmm.201707729
Fan, G., Sun, B., Wu, Z., Guo, Q., and Guo, Y. (2004). In vivo single-voxel proton MR spectroscopy in the differentiation of high-grade gliomas and solitary metastases. Clin. Radiol. 59, 77–85. doi: 10.1016/j.crad.2003.08.006
Ge, H., di, G., Yan, Z., Liu, D., Liu, Y., Song, K., et al. (2022). Does epilepsy always indicate worse outcomes? A longitudinal follow-up analysis of 485 glioma patients. World J. Surg. Oncol. 20:297. doi: 10.1186/s12957-022-02772-2
Giambra, M., di Cristofori, A., Valtorta, S., Manfrellotti, R., Bigiogera, V., Basso, G., et al. (2023). The peritumoral brain zone in glioblastoma: where we are and where we are going. J. Neurosci. Res. 101, 199–216. doi: 10.1002/jnr.25134
Goethe, E. A., Deneen, B., Noebels, J., and Rao, G. (2023). The role of Hyperexcitability in Gliomagenesis. Int. J. Mol. Sci. 24:749. doi: 10.3390/ijms24010749
Gong, R., Park, C. S., Abbassi, N. R., and Tang, S. J. (2006). Roles of glutamate receptors and the mammalian target of rapamycin (mTOR) signaling pathway in activity-dependent dendritic protein synthesis in hippocampal neurons. J. Biol. Chem. 281, 18802–18815. doi: 10.1074/jbc.M512524200
Grassian, A. R., Parker, S. J., Davidson, S. M., Divakaruni, A. S., Green, C. R., Zhang, X., et al. (2014). IDH1 mutations alter citric acid cycle metabolism and increase dependence on oxidative mitochondrial metabolism. Cancer Res. 74, 3317–3331. doi: 10.1158/0008-5472.CAN-14-0772-T
Gusarova, G. A., Trejo, H. E., Dada, L. A., Briva, A., Welch, L. C., Hamanaka, R. B., et al. (2011). Hypoxia leads to Na,K-ATPase downregulation via ca(2+) release-activated ca(2+) channels and AMPK activation. Mol. Cell. Biol. 31, 3546–3556. doi: 10.1128/MCB.05114-11
Guyon, J., Fernandez-Moncada, I., Larrieu, C. M., Bouchez, C. L., Pagano Zottola, A. C., Galvis, J., et al. (2022). Lactate dehydrogenases promote glioblastoma growth and invasion via a metabolic symbiosis. EMBO Mol. Med. 14:e15343. doi: 10.15252/emmm.202115343
Han, S., Liu, Y., Cai, S. J., Qian, M., Ding, J., Larion, M., et al. (2020). IDH mutation in glioma: molecular mechanisms and potential therapeutic targets. Br. J. Cancer 122, 1580–1589. doi: 10.1038/s41416-020-0814-x
Han, W., Shi, J., Cao, J., Dong, B., and Guan, W. (2020). Emerging roles and therapeutic interventions of aerobic glycolysis in glioma. Onco. Targets. Ther. 13, 6937–6955. doi: 10.2147/OTT.S260376
Hartmann, C., Meyer, J., Balss, J., Capper, D., Mueller, W., Christians, A., et al. (2009). Type and frequency of IDH1 and IDH2 mutations are related to astrocytic and oligodendroglial differentiation and age: a study of 1,010 diffuse gliomas. Acta Neuropathol. 118, 469–474. doi: 10.1007/s00401-009-0561-9
He, T., Yang, G. Y., and Zhang, Z. (2022). Crosstalk of astrocytes and other cells during ischemic stroke. Life (Basel). 12:60910. doi: 10.3390/life12060910
Hills, K. E., Kostarelos, K., and Wykes, R. C. (2022). Converging mechanisms of Epileptogenesis and their insight in glioblastoma. Front. Mol. Neurosci. 15:903115. doi: 10.3389/fnmol.2022.903115
Izumoto, S., Miyauchi, M., Tasaki, T., Okuda, T., Nakagawa, N., Nakano, N., et al. (2018). Seizures and tumor progression in glioma patients with uncontrollable epilepsy treated with Perampanel. Anticancer Res. 38, 4361–4366. doi: 10.21873/anticanres.12737
Kalinina, J., Ahn, J., Devi, N. S., Wang, L., Li, Y., Olson, J. J., et al. (2016). Selective detection of the D-enantiomer of 2-Hydroxyglutarate in the CSF of glioma patients with mutated Isocitrate dehydrogenase. Clin. Cancer Res. 22, 6256–6265. doi: 10.1158/1078-0432.CCR-15-2965
Karagiannis, A., Gallopin, T., Lacroix, A., Plaisier, F., Piquet, J., Geoffroy, H., et al. (2021). Lactate is an energy substrate for rodent cortical neurons and enhances their firing activity. Elife 10:10. doi: 10.7554/eLife.71424
Kaur, B., Khwaja, F. W., Severson, E. A., Matheny, S. L., Brat, D. J., and Van Meir, E. G. (2005). Hypoxia and the hypoxia-inducible-factor pathway in glioma growth and angiogenesis. Neuro-Oncology 7, 134–153. doi: 10.1215/S1152851704001115
KIM, J., HAN, J., JANG, Y., KIM, S. J., LEE, M. J., RYU, M. J., et al. (2015). High-capacity glycolytic and mitochondrial oxidative metabolisms mediate the growth ability of glioblastoma. Int. J. Oncol. 47, 1009–1016. doi: 10.3892/ijo.2015.3101
Kim, Y. H., Nobusawa, S., Mittelbronn, M., Paulus, W., Brokinkel, B., Keyvani, K., et al. (2010). Molecular classification of low-grade diffuse gliomas. Am. J. Pathol. 177, 2708–2714. doi: 10.2353/ajpath.2010.100680
Kim, J., Tchernyshyov, I., Semenza, G. L., and Dang, C. V. (2006). HIF-1-mediated expression of pyruvate dehydrogenase kinase: a metabolic switch required for cellular adaptation to hypoxia. Cell Metab. 3, 177–185. doi: 10.1016/j.cmet.2006.02.002
Kölker, S., Pawlak, V., Ahlemeyer, B., Okun, J. G., Hörster, F., Mayatepek, E., et al. (2002). NMDA receptor activation and respiratory chain complex V inhibition contribute to neurodegeneration in d-2-hydroxyglutaric aciduria. Eur. J. Neurosci. 16, 21–28. doi: 10.1046/j.1460-9568.2002.02055.x
Komiyama, K., Iijima, K., Kawabata-Iwakawa, R., Fujihara, K., Kakizaki, T., Yanagawa, Y., et al. (2022). Glioma facilitates the epileptic and tumor-suppressive gene expressions in the surrounding region. Sci. Rep. 12:6805. doi: 10.1038/s41598-022-10753-4
Ksendzovsky, A., Bachani, M., Altshuler, M., Walbridge, S., Mortazavi, A., Moyer, M., et al. (2023). Chronic neuronal activation leads to elevated lactate dehydrogenase a through the AMP-activated protein kinase/hypoxia-inducible factor-1α hypoxia pathway. Brain Commun. 5:298. doi: 10.1093/braincomms/fcac298
Lange, F., Hörnschemeyer, J., and Kirschstein, T. (2021). Glutamatergic mechanisms in glioblastoma and tumor-associated epilepsy. Cells 10:1226. doi: 10.3390/cells10051226
Leather, T., Jenkinson, M. D., Das, K., and Poptani, H. (2017). Magnetic resonance spectroscopy for detection of 2-Hydroxyglutarate as a biomarker for IDH mutation in gliomas. Meta 7:29. doi: 10.3390/metabo7020029
Leclercq, K., Van Liefferinge, J., Albertini, G., Neveux, M., Dardenne, S., Mairet-Coello, G., et al. (2019). Anticonvulsant and antiepileptogenic effects of system xc-inactivation in chronic epilepsy models. Epilepsia 60, 1412–1423. doi: 10.1111/epi.16055
Liu, F. M., Gao, Y. F., Kong, Y., Guan, Y., Zhang, J., Li, S. H., et al. (2021). The diagnostic value of lower glucose consumption for IDH1 mutated gliomas on FDG-PET. BMC Cancer 21:83. doi: 10.1186/s12885-021-07797-6
Liubinas, S. V., D’Abaco, G. M., Moffat, B. M., Gonzales, M., Feleppa, F., Nowell, C. J., et al. (2014). IDH1 mutation is associated with seizures and protoplasmic subtype in patients with low-grade gliomas. Epilepsia 55, 1438–1443. doi: 10.1111/epi.12662
Lohmann, P., Lerche, C., Bauer, E. K., Steger, J., Stoffels, G., Blau, T., et al. (2018). Predicting IDH genotype in gliomas using FET PET radiomics. Sci. Rep. 8:13328. doi: 10.1038/s41598-018-31806-7
Louis, D. N., Perry, A., Reifenberger, G., von Deimling, A., Figarella-Branger, D., Cavenee, W. K., et al. (2016). The 2016 World Health Organization classification of tumors of the central nervous system: a summary. Acta Neuropathol. 131, 803–820. doi: 10.1007/s00401-016-1545-1
Lu, C., Ward, P. S., Kapoor, G. S., Rohle, D., Turcan, S., Abdel-Wahab, O., et al. (2012). IDH mutation impairs histone demethylation and results in a block to cell differentiation. Nature 483, 474–478. doi: 10.1038/nature10860
Marcus, H. J., Carpenter, K. L. H., Price, S. J., and Hutchinson, P. J. (2010). In vivo assessment of high-grade glioma biochemistry using microdialysis: a study of energy-related molecules, growth factors and cytokines. J. Neuro-Oncol. 97, 11–23. doi: 10.1007/s11060-009-9990-5
Maschio, M., Pauletto, G., Zarabla, A., Maialetti, A., Ius, T., Villani, V., et al. (2019). Perampanel in patients with brain tumor-related epilepsy in real-life clinical practice: a retrospective analysis. Int. J. Neurosci. 129, 593–597. doi: 10.1080/00207454.2018.1555160
Maus, A., and Peters, G. J. (2017). Glutamate and α-ketoglutarate: key players in glioma metabolism. Amino Acids 49, 21–32. doi: 10.1007/s00726-016-2342-9
Mittal, S., Barkmeier, D., Hua, J., Pai, D. S., Fuerst, D., Basha, M., et al. (2016). Intracranial EEG analysis in tumor-related epilepsy: evidence of distant epileptic abnormalities. Clin. Neurophysiol. 127, 238–244. doi: 10.1016/j.clinph.2015.06.028
Monteiro, A. R., Hill, R., Pilkington, G. J., and Madureira, P. A. (2017). The role of hypoxia in glioblastoma invasion. Cells 6:45. doi: 10.3390/cells6040045
Mortazavi, A., Fayed, I., Bachani, M., Dowdy, T., Jahanipour, J., Khan, A., et al. (2022). IDH-mutated gliomas promote epileptogenesis through d-2-hydroxyglutarate-dependent mTOR hyperactivation. Neuro-Oncology 24, 1423–1435. doi: 10.1093/neuonc/noac003
Nickels, K. C., and Wirrell, E. C. (2017). Stiripentol in the management of epilepsy. CNS Drugs 31, 405–416. doi: 10.1007/s40263-017-0432-1
Ohno, M., Hayashi, Y., Aikawa, H., Hayashi, M., Miyakita, Y., Takahashi, M., et al. (2021). Tissue 2-Hydroxyglutarate and preoperative seizures in patients with diffuse gliomas. Neurology 97, e2114–e2123. doi: 10.1212/WNL.0000000000012893
Papandreou, I., Cairns, R. A., Fontana, L., Lim, A. L., and Denko, N. C. (2006). HIF-1 mediates adaptation to hypoxia by actively downregulating mitochondrial oxygen consumption. Cell Metab. 3, 187–197. doi: 10.1016/j.cmet.2006.01.012
Prakash, O., Lukiw, W. J., Peruzzi, F., Reiss, K., and Musto, A. E. (2012). Gliomas and seizures. Med. Hypotheses 79, 622–626. doi: 10.1016/j.mehy.2012.07.037
Rasmussen, B. K., Hansen, S., Laursen, R. J., Kosteljanetz, M., Schultz, H., Nørgård, B. M., et al. (2017). Epidemiology of glioma: clinical characteristics, symptoms, and predictors of glioma patients grade I-IV in the the Danish neuro-oncology registry. J. Neuro-Oncol. 135, 571–579. doi: 10.1007/s11060-017-2607-5
Rempel, A., Mathupala, S. P., Griffin, C. A., Hawkins, A. L., and Pedersen, P. L. (1996). Glucose catabolism in cancer cells: amplification of the gene encoding type II hexokinase. Cancer Res. 56, 2468–2471.
Rijpkema, M., Schuuring, J., Van der Meulen, Y., Van der Graaf, M., Bernsen, H., Boerman, R., et al. (2003). Characterization of oligodendrogliomas using short echo time 1H MR spectroscopic imaging. NMR Biomed. 16, 12–18. doi: 10.1002/nbm.807
Robert, S. M., and Sontheimer, H. (2014). Glutamate transporters in the biology of malignant gliomas. Cell. Mol. Life Sci. 71, 1839–1854. doi: 10.1007/s00018-013-1521-z
Roslin, M., Henriksson, R., Bergström, P., Ungerstedt, U., and Bergenheim, A. T. (2003). Baseline levels of glucose metabolites, glutamate and glycerol in malignant glioma assessed by stereotactic microdialysis. J. Neuro-Oncol. 61, 151–160. doi: 10.1023/A:1022106910017
Rudà, R., Bello, L., Duffau, H., and Soffietti, R. (2012). Seizures in low-grade gliomas: natural history, pathogenesis, and outcome after treatments. Neuro Oncol. 14, iv55–iv64. doi: 10.1093/neuonc/nos199
Sada, N., Lee, S., Katsu, T., Otsuki, T., and Inoue, T. (2015). Epilepsy treatment. Targeting LDH enzymes with a stiripentol analog to treat epilepsy. Science 347, 1362–1367. doi: 10.1126/science.aaa1299
Sada, N., Suto, S., Suzuki, M., Usui, S., and Inoue, T. (2020). Upregulation of lactate dehydrogenase a in a chronic model of temporal lobe epilepsy. Epilepsia 61, e37–e42. doi: 10.1111/epi.16488
Salamanca-Cardona, L., Shah, H., Poot, A. J., Correa, F. M., Di Gialleonardo, V., Lui, H., et al. (2017). In vivo imaging of glutamine metabolism to the Oncometabolite 2-Hydroxyglutarate in IDH1/2 mutant tumors. Cell Metab. 26, 830–841.e3. doi: 10.1016/j.cmet.2017.10.001
Schaller, B. (2005). Influences of brain tumor-associated pH changes and hypoxia on epileptogenesis. Acta Neurol. Scand. 111, 75–83. doi: 10.1111/j.1600-0404.2004.00355.x
Shamji, M. F., Fric-Shamji, E. C., and Benoit, B. G. (2009). Brain tumors and epilepsy: pathophysiology of peritumoral changes. Neurosurg. Rev. 32, 275–285. doi: 10.1007/s10143-009-0191-7
Smits, A., and Duffau, H. (2011). Seizures and the natural history of World Health Organization grade II gliomas: a review. Neurosurgery 68, 1326–1333. doi: 10.1227/NEU.0b013e31820c3419
Stanke, K. M., Wilson, C., and Kidambi, S. (2021). High expression of glycolytic genes in clinical glioblastoma patients correlates with lower survival. Front. Mol. Biosci. 8:752404. doi: 10.3389/fmolb.2021.752404
Stockhammer, F., Misch, M., Helms, H. J., Lengler, U., Prall, F., von Deimling, A., et al. (2012). IDH1/2 mutations in WHO grade II astrocytomas associated with localization and seizure as the initial symptom. Seizure 21, 194–197. doi: 10.1016/j.seizure.2011.12.007
Strickland, M., and Stoll, E. A. (2017). Metabolic reprogramming in glioma. Front. Cell Dev. Biol. 5:43. doi: 10.3389/fcell.2017.00043
Tietze, A., Choi, C., Mickey, B., Maher, E. A., Parm Ulhøi, B., Sangill, R., et al. (2018). Noninvasive assessment of isocitrate dehydrogenase mutation status in cerebral gliomas by magnetic resonance spectroscopy in a clinical setting. J. Neurosurg. 128, 391–398. doi: 10.3171/2016.10.JNS161793
Tobochnik, S., Dorotan, M. K. C., Ghosh, H. S., Lapinskas, E., Vogelzang, J., Reardon, D. A., et al. (2023). Glioma genetic profiles associated with electrophysiologic hyperexcitability. medRxiv 2023:23285841. doi: 10.1101/2023.02.22.23285841
Turkalp, Z., Karamchandani, J., and Das, S. (2014). IDH mutation in glioma: new insights and promises for the future. JAMA Neurol. 71, 1319–1325. doi: 10.1001/jamaneurol.2014.1205
Valvona, C. J., Fillmore, H. L., Nunn, P. B., and Pilkington, G. J. (2016). The regulation and function of lactate dehydrogenase a: therapeutic potential in brain tumor. Brain Pathol. 26, 3–17. doi: 10.1111/bpa.12299
Van Opijnen, M. P., Tesileanu, C. M. S., Dirven, L., Van der Meer, P. B., Wijnenga, M. M. J., Vincent, A. J. P. E., et al. (2023). IDH1/2 wildtype gliomas grade 2 and 3 with molecular glioblastoma-like profile have a distinct course of epilepsy compared to IDH1/2 wildtype glioblastomas. Neuro-Oncology 25, 701–709. doi: 10.1093/neuonc/noac197
Vecht, C., Duran-Peña, A., Houillier, C., Durand, T., Capelle, L., and Huberfeld, G. (2017). Seizure response to perampanel in drug-resistant epilepsy with gliomas: early observations. J. Neuro-Oncol. 133, 603–607. doi: 10.1007/s11060-017-2473-1
Vecht, C. J., Kerkhof, M., and Duran-Pena, A. (2014). Seizure prognosis in brain tumors: new insights and evidence-based management. Oncologist 19, 751–759. doi: 10.1634/theoncologist.2014-0060
Vo, A. H., Ambady, P., and Spencer, D. (2022). The IDH1 inhibitor ivosidenib improved seizures in a patient with drug-resistant epilepsy from IDH1 mutant oligodendroglioma. Epilepsy Behav. Rep. 18:100526. doi: 10.1016/j.ebr.2022.100526
Waldbaum, S., and Patel, M. (2010). Mitochondria, oxidative stress, and temporal lobe epilepsy. Epilepsy Res. 88, 23–45. doi: 10.1016/j.eplepsyres.2009.09.020
Wang, Y. H., Huang, T. L., Chen, X., Yu, S. X., Li, W., Chen, T., et al. (2021). Glioma-derived TSP2 promotes excitatory synapse formation and results in Hyperexcitability in the Peritumoral cortex of glioma. J. Neuropathol. Exp. Neurol. 80, 137–149. doi: 10.1093/jnen/nlaa149
Wang, Y., Zhang, F., Xiong, N., Xu, H., Chai, S., Wang, H., et al. (2021). Remodelling and treatment of the blood-brain barrier in glioma. Cancer Manag. Res. 13, 4217–4232. doi: 10.2147/CMAR.S288720
Weller, M., Wick, W., Aldape, K., Brada, M., Berger, M., Pfister, S. M., et al. (2015). Glioma. Nat. Rev. Dis. Primers. 1:15017. doi: 10.1038/nrdp.2015.17
Woolf, E. C., and Scheck, A. C. (2012). Metabolism and glioma therapy. CNS Oncol. 1, 7–10. doi: 10.2217/cns.12.9
Xiao, F., Lv, S., Zong, Z., Wu, L., Tang, X., Kuang, W., et al. (2020). Cerebrospinal fluid biomarkers for brain tumor detection: clinical roles and current progress. Am. J. Transl. Res. 12, 1379–1396.
Xu, Y., and Fan, Q. (2022). Relationship between chronic hypoxia and seizure susceptibility. CNS Neurosci. Ther. 28, 1689–1705. doi: 10.1111/cns.13942
Yan, H., Parsons, D. W., Jin, G., McLendon, R., Rasheed, B. A., Yuan, W., et al. (2009). IDH1 and IDH2 mutations in gliomas. N. Engl. J. Med. 360, 765–773. doi: 10.1056/NEJMoa0808710
Yang, H., Wu, J., Guo, R., Peng, Y., Zheng, W., Liu, D., et al. (2013). Glycolysis in energy metabolism during seizures. Neural Regen. Res. 8, 1316–1326. doi: 10.3969/j.issn.1673-5374.2013.14.008
Yao, P. S., Kang, D. Z., Lin, R. Y., Ye, B., Wang, W., and Ye, Z. C. (2014). Glutamate/glutamine metabolism coupling between astrocytes and glioma cells: neuroprotection and inhibition of glioma growth. Biochem. Biophys. Res. Commun. 450, 295–299. doi: 10.1016/j.bbrc.2014.05.120
You, G., Sha, Z., and Jiang, T. (2012). The pathogenesis of tumor-related epilepsy and its implications for clinical treatment. Seizure 21, 153–159. doi: 10.1016/j.seizure.2011.12.016
You, G., Sha, Z., and Jiang, T. (2020). Clinical diagnosis and perioperative Management of Glioma-Related Epilepsy. Front. Oncol. 10:550353. doi: 10.3389/fonc.2020.550353
Yu, L., Chen, X., Wang, L., and Chen, S. (2016). The sweet trap in tumors: aerobic glycolysis and potential targets for therapy. Oncotarget 7, 38908–38926. doi: 10.18632/oncotarget.7676
Yuen, T. I., Morokoff, A. P., Bjorksten, A., D’Abaco, G., Paradiso, L., Finch, S., et al. (2012). Glutamate is associated with a higher risk of seizures in patients with gliomas. Neurology 79, 883–889. doi: 10.1212/WNL.0b013e318266fa89
Zaccagna, F., McLean, M. A., Grist, J. T., Kaggie, J., Mair, R., Riemer, F., et al. (2022). Imaging glioblastoma metabolism by using hyperpolarized [1-13C]pyruvate demonstrates heterogeneity in lactate labeling: a proof of principle study. Radiol. Imaging Cancer 4:e210076. doi: 10.1148/rycan.210076
Zhao, S., Lin, Y., Xu, W., Jiang, W., Zha, Z., Wang, P., et al. (2009). Glioma-derived mutations in IDH1 dominantly inhibit IDH1 catalytic activity and induce HIF-1alpha. Science 324, 261–265. doi: 10.1126/science.1170944
Keywords: tumor-related epilepsy, brain, glioma, seizure development, tumoral metabolism, peritumoral excitability
Citation: McAfee D, Moyer M, Queen J, Mortazavi A, Boddeti U, Bachani M, Zaghloul K and Ksendzovsky A (2023) Differential metabolic alterations in IDH1 mutant vs. wildtype glioma cells promote epileptogenesis through distinctive mechanisms. Front. Cell. Neurosci. 17:1288918. doi: 10.3389/fncel.2023.1288918
Edited by:
Mioara Larion, National Institutes of Health, United StatesReviewed by:
Giuseppina D’Alessandro, Sapienza University of Rome, ItalyRobert C. Wykes, University College London, United Kingdom
Copyright © 2023 McAfee, Moyer, Queen, Mortazavi, Boddeti, Bachani, Zaghloul and Ksendzovsky. This is an open-access article distributed under the terms of the Creative Commons Attribution License (CC BY). The use, distribution or reproduction in other forums is permitted, provided the original author(s) and the copyright owner(s) are credited and that the original publication in this journal is cited, in accordance with accepted academic practice. No use, distribution or reproduction is permitted which does not comply with these terms.
*Correspondence: Alexander Ksendzovsky, YWtzZW5kem92c2t5QHNvbS51bWFyeWxhbmQuZWR1
†These authors have contributed equally to this work and share first authorship