- 1Beilun District People’s Hospital, Ningbo, China
- 2Tianjin Union Medical Center, Tianjin, China
- 3First Teaching Hospital of Tianjin University of Traditional Chinese Medicine, Tianjin, China
- 4National Clinical Research Center for Chinese Medicine Acupuncture and Moxibustion, Tianjin, China
- 5National Anti-Drug Laboratory Beijing Regional Center, Beijing, China
Peripheral nerve injury (PNI) is a structural event with harmful consequences worldwide. Due to the limited intrinsic regenerative capacity of the peripheral nerve in adults, neural restoration after PNI is difficult. Neurological remodeling has a crucial effect on the repair of the form and function during the regeneration of the peripheral nerve after the peripheral nerve is injured. Several studies have demonstrated that acupuncture is effective for PNI-induced neurologic deficits, and the potential mechanisms responsible for its effects involve the nervous system remodeling in the process of nerve repair. Moreover, acupuncture promotes neural regeneration and axon sprouting by activating related neurotrophins retrograde transport, such as nerve growth factor (NGF), brain-derived neurotrophic factor (BDNF), glial cell-derived neurotrophic factor (GDNF), N-cadherin, and MicroRNAs. Peripheral nerve injury enhances the perceptual response of the central nervous system to pain, causing central sensitization and accelerating neuronal cell apoptosis. Together with this, the remodeling of synaptic transmission function would worsen pain discomfort. Neuroimaging studies have shown remodeling changes in both gray and white matter after peripheral nerve injury. Acupuncture not only reverses the poor remodeling of the nervous system but also stimulates the release of neurotrophic substances such as nerve growth factors in the nervous system to ameliorate pain and promote the regeneration and repair of nerve fibers. In conclusion, the neurological remodeling at the peripheral and central levels in the process of acupuncture treatment accelerates nerve regeneration and repair. These findings provide novel insights enabling the clinical application of acupuncture in the treatment of PNI.
1. Introduction
Peripheral nerve injury caused by compression, traction, ischemia, and laceration can cause motor impairment of the limb, disuse atrophy of the limb muscles, and other nutritional disorders and sensory disturbances in the damaged innervated areas. With a 13–23 per 100,000 person-years estimated incidence rate, peripheral nerve damage (PNI) can cause sensory loss, persistent discomfort, motor impairment, or even amputation (Asplund et al., 2009; Sullivan et al., 2016). The most popular nerve injury categorization system is Seddon’s original one based on neurophysiological alterations: grade 1 nerve damage is a neurapraxia disease, grade 2 is axonal degeneration, and grade 3 is nerve transection. Peripheral nerve injury functional recovery is a slow process. The amount of time required for functional reconstruction and neural regeneration may be the reason for this, but the treatment method used also may be a factor in how long it takes to recover. Before the nerve injury becomes irreparable, early detection enables the start of neuroanastomosis, a suitable rehabilitation program, and adjustment of biomechanics, all of which benefit to encourage nerve repair. For instance, peripheral nerve injuries are more frequent in the upper extremities than the lower, tend to be sport-specific, and frequently include a biomechanical component. According to earlier research, people with peripheral nerve injury of the upper limbs did not have a high quality of life because of the acute neuropathic pain and potential for impairment (Pan et al., 2019). At present, there are gradually reconstructive operations clinically, but many patients experience a loss of their upper limbs’ normal function after the operation. Long-term nerve injury and inability to recover will also result in a lifetime impairment of patients. Therefore, further postoperative recovery is required to treat them (Bruyns et al., 2003; Magistroni et al., 2020). The main strategies for the treatment of PNI include pharmacologic interventions, behavioral therapy, physical stimulation, and supportive therapy in addition to surgery. In the numerous interventions for peripheral nerve injury, we need to carry out precision treatment according to the patient’s condition. Among them, acupuncture has its unique advantages in that acupuncture plays an important role in anti-inflammation, analgesia, and speeding up nerve repair. This makes the sequence of postoperative rehabilitation treatment of patients can be acupuncture first and rehabilitation manipulation later, to reduce the pain during rehabilitation manipulation and maximize the analgesic efficiency of acupuncture. Moreover, acupuncture is a safe, effective, and less painful treatment method. But there is still much uncertainty about how acupuncture works in PNI.
Acupuncture is a procedure involving the insertion of a fine needle into the skin or deeper tissues at specific locations of the body (acupoints) to prevent and treat diseases. Several lines of neuroanatomical and neurological evidence have demonstrated the abundant distribution of nerve endings in human meridians and acupoints, and the involvement of the nervous system is indispensable for the effects of acupuncture. Acupuncture is regarded as a potential adjuvant treatment for acupuncture analgesia, neural regeneration, and functional restoration in addition to conventional therapy of nerve anastomosis (Hao et al., 1995; Balogun et al., 1998; Zhang et al., 2018). Some studies showed that electroacupuncture (EA) helped individuals with peripheral nerve injuries improve their motor or sensory function (Dimitrova et al., 2017). Some researchers have indicated that rehabilitation training together with Jiaji electroacupuncture can greatly facilitate the recovery of muscle group function and improve the quality of life of patients with upper limb peripheral nerve injury (Li H. et al., 2021). With the rapid development of research on acupuncture’s central effect, accumulating evidence showed acupuncture or electro-acupuncture also played a key role in brain remodeling, which suggested a potentially positive impact on axon regeneration and synapse formation (Xiao et al., 2018). Some research suggests acupuncture and electroacupuncture can encourage nerve regeneration and enhance nerve function (Inoue et al., 2003; Lu et al., 2008; Chen et al., 2013). What’s more, acupuncture is green and harmless, which has this beauty, that accelerates the recovery after PNI.
Research on the mechanism of acupuncture in peripheral nerve injury is currently mostly concentrated at the level of individual molecules and/or signaling cascades. Acupuncture has the potential to control a complex network of several signaling molecules and pathways due to the wide range of interaction communication that exists between various signaling pathways. This notion is in line with acupuncture’s holistic regulation features, which involve numerous targets, linkages, techniques, and levels. And more research is needed to describe how acupuncture affects this intricate network. Therefore, this paper reviews the effects and mechanisms of acupuncture on nerve regeneration and repair from the perspective of nervous system remodeling.
2. Clinical efficacy of acupuncture or electro-acupuncture on nerve regeneration of PNI patients
Acupuncture or EA affects nerve system according to several studies. Researchers showed that low-intensity ES at the ST36 site could activate brainstem vagal efferent neurons or drive catecholamine release from adrenal glands to achieve the target that suppressed systemic inflammation induced by bacterial endotoxins (Liu et al., 2021). Some results suggested that electroacupuncture may be able to modulate extracellular adenosine triphosphate (ATP) levels in the prefrontal cortex of depressive-like maternal separation rats, potentially contributing to its antidepressant effects (Zheng et al., 2023). It is demonstrated that EA pretreatment resulted in increased ambient endocannabinoid (eCB) levels and subsequent activation of ischemic penumbral astroglial cannabinoid type 1 receptors (CB1R) in a middle cerebral artery occlusion (MCAO) model which led to moderate upregulation of extracellular glutamate that protected neurons from cerebral ischemic injury (Yang et al., 2021). Researchers found that EA markedly improved acute lumbar sprain, and EA better improved the rehabilitation and regeneration of force-displacement values and temperature index of the infrared thermogram of the muscle (Fan and Wu, 2015). Acupuncture or EA has been found to have a beneficial clinical effect in treating PNI, according to some researchers. Accelerated nerve regeneration caused by electroacupuncture with intermittent direct current may contribute to the recovery of PNI (Inoue et al., 2011). Some studies demonstrated that short-term acupuncture treatment may result in long-term improvement in mild-to-moderate idiopathic CTS. Acupuncture treatment can be considered an alternative therapy to other conservative treatments for those who do not opt for early surgical decompression (Yang et al., 2011). Some findings indicated that electroacupuncture could improve symptomatology for CTS patients, as evidenced by improved symptomatology, grip strength, electrophysiological function, and physical provocation sign (Ho et al., 2014). The grip strength of CTS patients’ primary symptomatic side dramatically increased following acupuncture treatment indicating an improvement in median nerve motor function obstacle (Mathiowetz et al., 1985). As CTS-induced paresthesias constitute diffuse, synchronized, multidigit symptomatology, researchers found that maladaptive change and correction are consistent with Hebbian plasticity mechanisms. Acupuncture shows promise in inducing beneficial cortical plasticity manifested by more focused digital representations (Napadow et al., 2007b). The acupoints for PNI treatment are listed in Tables 1–5 and shown in Figures 1–5. Table 1 summarizes the evidence of the effect of acupuncture on the repair of peripheral nerve injury.
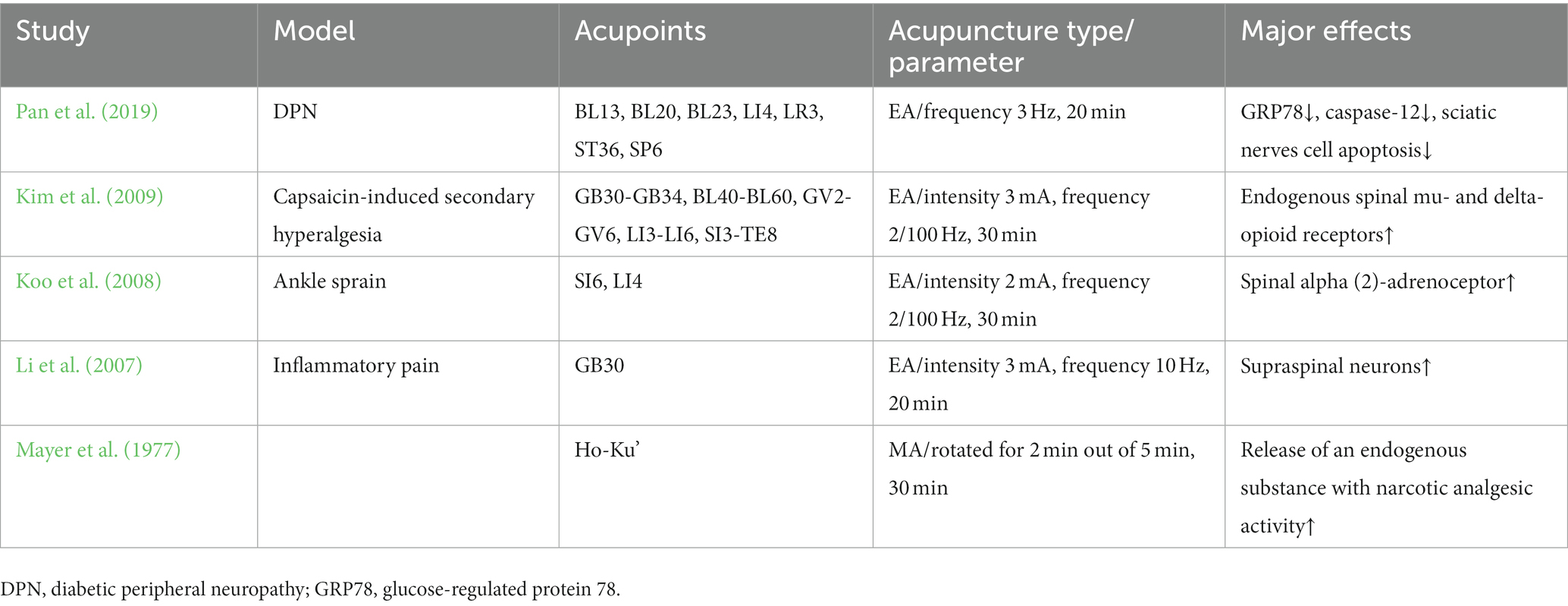
Table 2. Mechanism of acupuncture on the repair of spinal cord regulation in peripheral nerve injury.
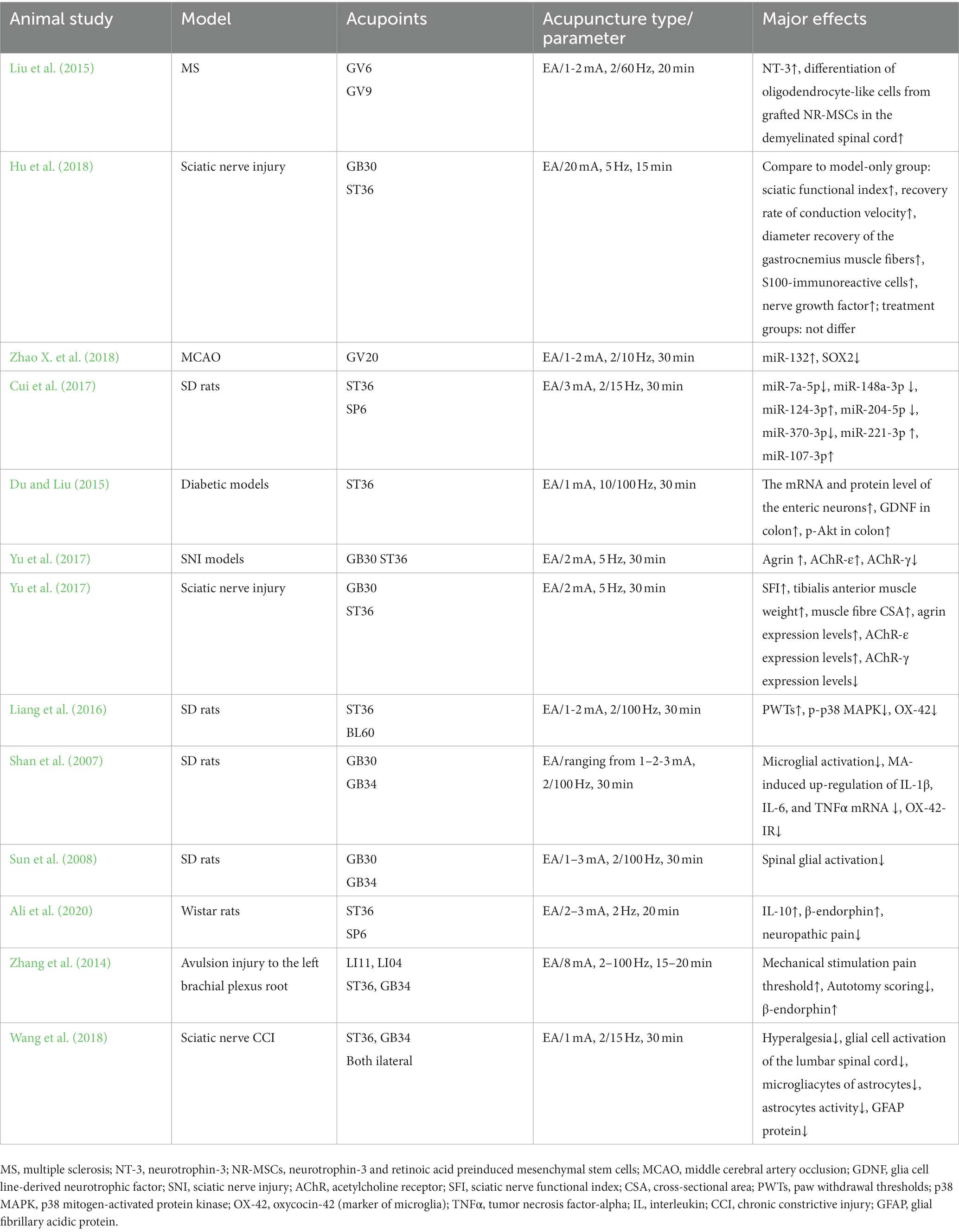
Table 4. Mechanism of acupuncture on the regulation of factors associated with neuronal response in peripheral nerve injury.
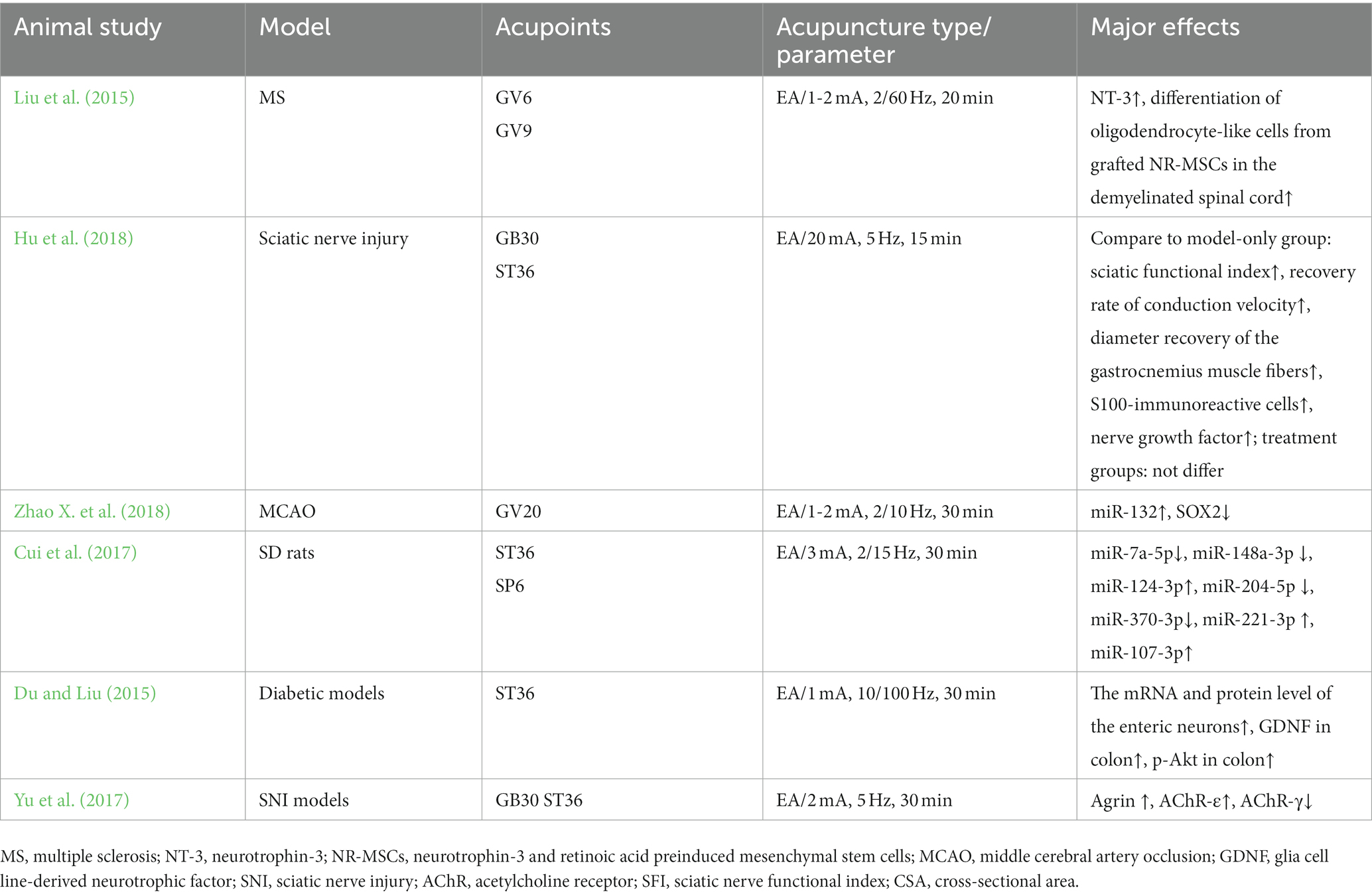
Table 5. Mechanism of acupuncture on the regulation of factors associated with neuronal response in peripheral nerve injury.
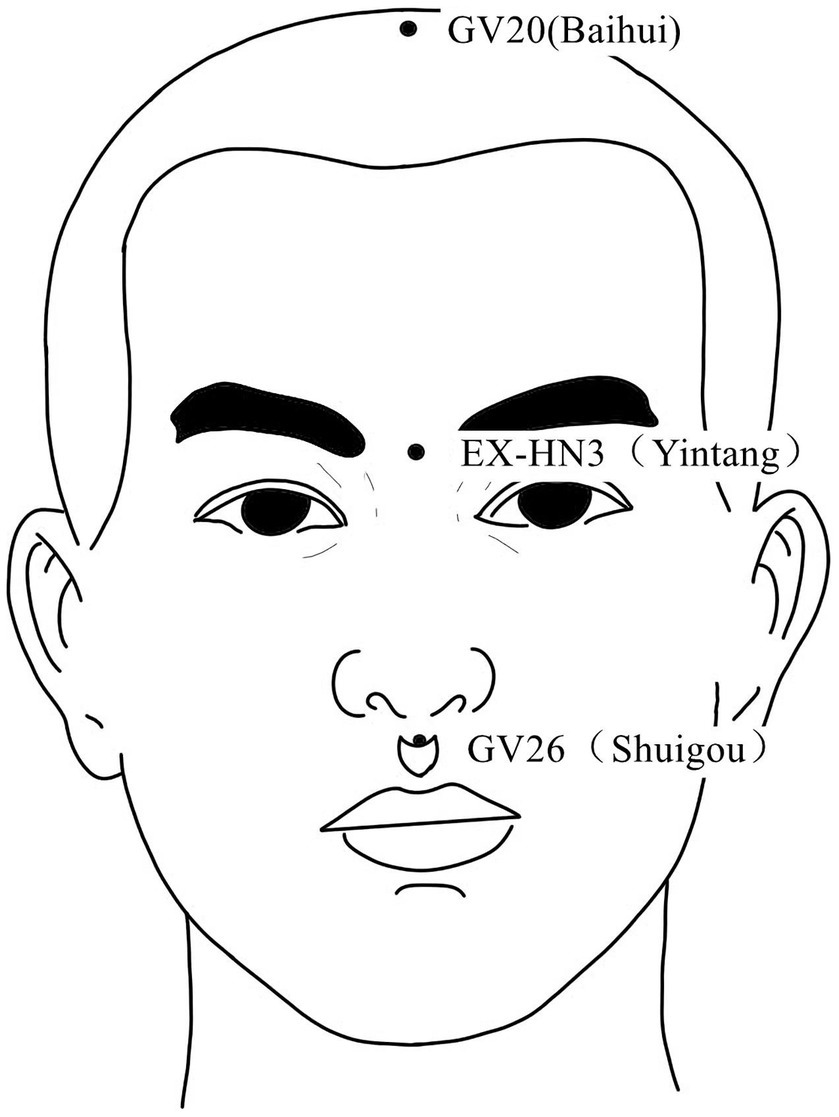
Figure 3. Acupoints on the head and face According to the newest National Standard of the People’s Republic of China GB/T 12346–2021 Nomenclature and Location of Meridia Points, Yintang belongs to Governor Vein (GV), in order to be consistent with the literature cited in this paper, Yintang is still labeled EX-HN3.
3. Nerve system remodeling-related mechanisms of acupuncture or electroacupuncture for the treatment of peripheral nerve injury
3.1. Peripheral nerve fiber regeneration and repair
The primary obstacles to saving neurological function following damage are repair, regeneration, and neuroprotection (Ribeiro et al., 2016; Tallon and Farah, 2017). The potential for regeneration after nerve fiber injury is reflected by the de novo expression or upregulation of a massive variety of molecules in the distal nerve fiber tracts (Gordon and Borschel, 2017). So, it is vital to maintain the integrity of the nerve fibers. Electrical stimulation can facilitate peripheral nerve regeneration and target reinnervation (Wenjin et al., 2011; Liu et al., 2013; Zhao et al., 2013). Investigators believe that a weak electric field may affect the regeneration of mammalian peripheral nerves (Schmidt et al., 1997), and extracellular electric fields could influence the orientation of neurite development (Patel and Poo, 1982). Under the influence of a weak electrical field, neurite outgrowth grew more quickly in the direction of the negative pole, but the growth in the direction of the positive pole was suppressed (Song et al., 2004). Studies have discovered that needles used for direct electrical stimulation of the damaged ulnar nerve together with rehabilitation expedite nerve regeneration (Tang et al., 2016). Additionally, electro-needling could increase the number of blood vessels and axon densities in rat peripheral nerves regenerated within a silicone rubber tube (Cheng et al., 2001), after nerve transection, EA could promote the recovery of transected median nerve morphology and function (Ho et al., 2013). According to the experimental results, electroacupuncture can upregulate β-endorphin expression, which reduces neuropathic pain and aid in the healing of damaged brachial plexus nerves (Zhang et al., 2014). According to some research, acupuncture therapy could enhance motor and sensory nerve conduction, scores for the sciatic nerve function index, compound muscle action potential, and motor nerve conduction velocity as well as better promote sciatic nerve regeneration and reduce muscle atrophy while causing less mechanical damage to nerve trunk (Yu et al., 2019). EA was reported to effectively improve functional outcomes following PNI (Zhi et al., 2017). After nerve reconstruction, the regeneration of the motion axis takes a long time (Pagnussat et al., 2012; Sturma et al., 2018). Therefore, EA can both serve as a foundation for nerve regeneration and has the potential to speed up the healing process for damaged nerves.
3.2. Mechanism of spinal cord regulation in peripheral nerve injury
3.2.1. Central sensitization
Central sensitization, where the input of noxious stimuli enhances the perceptual response of the central nervous system to pain, is caused by tissue inflammation or peripheral nerve damage and plays an important role in persistent pain (Van Griensven et al., 2020). Previous research has shown that a local cutaneous injury can produce primary hyperalgesia within the injured area and secondary hyperalgesia in the normal surrounding skin, for example, an intradermal injection of capsaicin in humans causes intense pain and hyperalgesia to heat and mechanical stimuli in the surrounding skin. Psychophysical studies in humans supported the conclusions that hyperalgesia was predominantly the secondary type and depended on one set of neurons sensitizing another (“neurogenic hyperalgesia”) and that the latter set of neurons is located in the central and not the peripheral nervous system (Baumann et al., 1991). Central sensitization is accountable for spreading pain and hyperalgesia to uninjured tissue, the process is involved in pain-transmission neurons in the central nervous system (CNS), often in the dorsal horn of the spinal cord (Kim et al., 2009). Second-level sensory neurons, or the relay stations of the conduction of the sensory system, are represented by the neurons of the spinal dorsal horn. The ascending fiber tracts are created by the spinal dorsal horn neurons’ axons as they enter the ipsilateral or contralateral white matter. These ascending fiber tracts then transmit the dorsal root afferents’ nerve impulses to the third-level neurons in the ventroposterolateral thalamic nucleus of the brain, which then transmits them to the cerebral cortex. Some results suggested that acupuncture may simultaneously modulate the resting state functional connectivity (rsFC) of key regions in the descending pain modulation (periaqueductal gray, PAG) and reward systems (ventral tegmental area, VTA), and the amygdala may be a key node linking the two systems to produce antinociceptive effects (Yu et al., 2020). This makes sense because it is widely known that ipsilateral acupuncture has an impact on numerous descending inhibitory networks from the amygdala, periaqueductal gray (PAG), and rostroventral medulla (RVM) which primarily modulate nociceptive signals entering the ipsilateral spinal cord and are influenced by ipsilateral acupuncture (Levine et al., 1991; Van Bockstaele et al., 1991; Manning, 1998; Urban et al., 1999). Acupuncture is demonstrated to activate the ascending sensory pathways, such as the spinal dorsal horn and thalamus, or the descending pain inhibitory mechanisms, such as opioid, adrenergic, and serotonergic pathways, however, the precise areas where EA affects pain are not fully established (Skrabanek, 1984; Tian et al., 2006; Li et al., 2007; Koo et al., 2008).
3.2.2. Neuronal apoptosis
According to previous findings, PNI not only results in localized damage but also trigger the apoptosis of certain spinal cord neurons in corresponding spinal cord segments (Inquimbert et al., 2018). The survival of central neurons plays a key role in determining the effectiveness of peripheral nerve regeneration (Kim and Choi, 2016). After PNI, the corresponding neuron cell bodies will undergo apoptosis because of neurotrophin transmission disorders. And we discovered that glial cell-derived neurotrophic factors and brain-derived neurotrophic factors could alleviate PNI in animal models, and decrease apoptosis and autophagy in vitro cell and cell injury model studies (Chou et al., 2014; Kingham et al., 2014). PNI causes apoptosis in spinal motor neurons and sensory spinal ganglion neurons, but it is not extensive and does not affect the entire spinal cord, it is associated with neurons that are connected to the injured peripheral nerves (Ahmad et al., 2015). According to a previous study, acupuncture can ensure and enhance the continuity between peripheral and central nerves, speeding up the process of repairing injured nerves, laying the groundwork for regeneration, and effectively restoring motor conduction (He et al., 2015), and electroacupuncture could dramatically boost facial nerve regeneration by increasing the expression of GDNF and N-cadherin in neurons, preventing neuronal apoptosis (Fei et al., 2019). Some results indicate that EA suppresses spinal nerve ligation (SNL)-induced neuropathic pain by improving neuronal plasticity via upregulating the adenosine A2A receptor (A2AR) and the cyclic adenosine monophosphate (cAMP)/protein kinase A (PKA) signaling pathway (Wu et al., 2021a), but more research is still needed to understand the underlying mechanism. Table 2 summarizes the evidence of the mechanism of acupuncture on the repair of spinal cord regulation in peripheral nerve injury.
3.3. Synaptic remodeling
The place where neurons join and where information is transmitted is called a synapse. Synapses, which are mostly found on dendritic spines and/or soma, serve a crucial role in the functional connections between neurons and are essential parts of information transmission. The synaptic region contains a variety of enzymes that break down neurotransmitters. Neurotransmitters transmit more slowly and are more degraded when there is a larger synaptic distance. As a result, the structural basis for the remodeling of synaptic transmission function may be the increase in the number of synapses, synaptic vesicle density, and the narrowing of synaptic space. A prior study suggested that the analgesic effect of EA might be connected to the suppression of inflammatory factors and dendritic spine/synaptic remodeling (Wu et al., 2021b).
Microglial cells, the smallest glial cells in the nervous system with polysynaptic and plasticity, are the innate immunological effector cells in the central nervous system. Previous research has shown that microglial cells are a crucial target for analgesia by EA (Shan et al., 2007; Sun et al., 2008), EA can reduce neuropathic pain by promoting the production of IL-10 in spinal microglial cells (Ali et al., 2020). EA-induced anti-hyperalgesia may be partially associated with the reduced expression of Phospho-p38 MAPK (p-p38 MAPK) on microglia, and subsequently reducing the activation of oxytocin-42 (OX-42, marker of microglia) in neuropathic pain (Liang et al., 2016). P2X7R overexpression in neuropathic pain models can result in microglia activation and overexpression of TNF-a and IL-1b. Long-term potentiation is avoided and the pain threshold is raised by downregulating P2X7R with P2X7-specific siRNA (Chu et al., 2010; Vadivelu et al., 2015; Shen et al., 2018). Following chronic constriction injury, there was an increase in P2X7R expression in the spinal cord and a corresponding drop in the rat pain threshold (Lin et al., 2018). Therefore, EA may reduce aberrant dendritic spine/synaptic remodeling, and inflammation by downregulating P2X7R to improve neuropathic pain and promote nerve repair (Figure 6; Wu et al., 2021b). Table 3 summarizes the evidence of the mechanism of acupuncture on the regulation of synaptic in peripheral nerve injury.
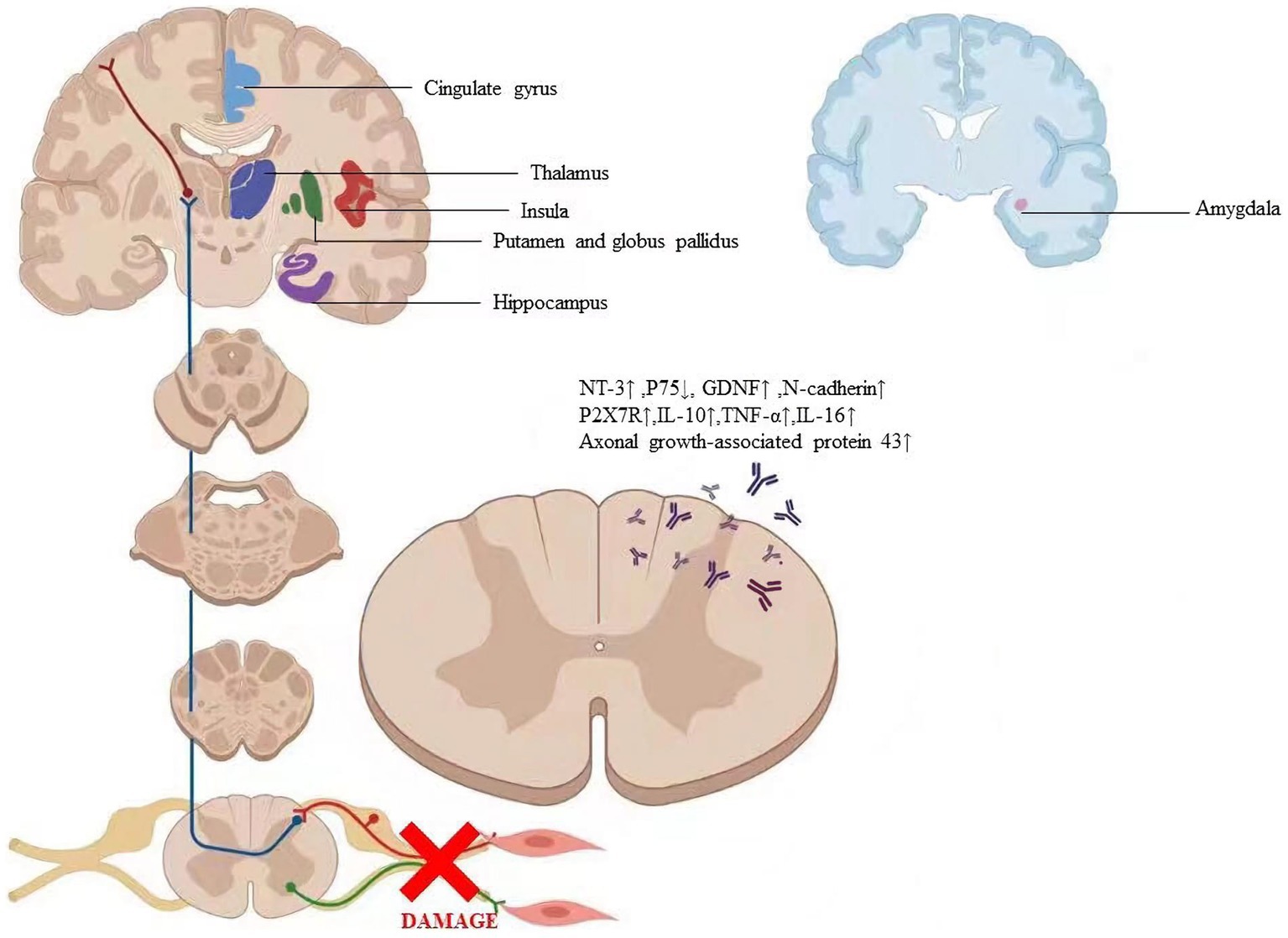
Figure 6. The labeled regions revealed where the particular alterations in the brain are caused by acupuncture. The factors regulated by acupuncture include axonal growth-associated protein 431, NT-31, P751, GDNF1, N-cadherin, P2X7R1, IL-101, TNF-at, and IL-161. Abbreviation: NT-3: Neurotrophins-3, GDNF: Glial cell line-derived neurotrophic factor, P2X7R: P2X7 receptor, IL- 10: Interleukin 10, TNF-α: Tumor necrosis factor-alpha, IL-16: Interleukin 16.
3.4. Regulation of brain regions in peripheral nerve injury
Plasticity following peripheral nerve transection has been demonstrated throughout the neuroaxis in animal models of nerve injury (Fawcett and Keynes, 1990). However, little research has demonstrated the brain changes that occur following peripheral nerve transection and surgical repair in humans. Furthermore, the extent to which peripheral nerve regeneration influences functional and structural brain changes has not been characterized (Taylor et al., 2009). Numerous studies on neural imaging extensively revealed the particular alterations in the brain caused by acupuncture (Figure 6; Kong et al., 2009; Napadow et al., 2009; Lee et al., 2013). Due to the tight relationship between behavioral performance and cortical plasticity, acupuncture may have an impact on cortical plasticity (Klingner et al., 2014; Jung et al., 2015; Zhang et al., 2016). Therefore, we asked whether acupuncture treatment of peripheral nerve injury is accompanied by gray and/or white matter structural changes and whether these changes relate to nerve system remodeling.
3.4.1. The endogenous opioid peptide system network
Numerous brain regions and nuclei, including the caudate nucleus, septal area, arcuate nucleus, periaqueductal gray, and nucleus raphe magnus, all of which contain opioid peptides, and μ, δ, and κ receptors, are involved in the transmission of acupuncture signals (Zhao, 2008). The hypothalamic arcuate nucleus is a significant structure in the endogenous opioid peptide system and a crucial region that mediates low-frequency electroanalgesia (Takeshige et al., 1991). Researchers found that acupuncture at HT7 points attenuates behavioral manifestation of alcohol dependence by activating endorphinergic input to the nucleus accumbens (NAc) from the arcuate nucleus(ARC) (Chang et al., 2019). The arcuate nucleus may control electroanalgesia based on the fact that its axons branch out to the adjacent nucleus accumbens, septum, periaqueductal gray, and locus coeruleus. In praxiology and electrophysiology, activating the arcuate nucleus will boost analgesic effects, increased the activity of neurons in the dorsal raphe nucleus and decreased the activity of neurons in the locus coeruleus (Kwon et al., 2004; Yoon et al., 2013). According to studies, more genes were differentially regulated by low-frequency EA than high-frequency EA (154 vs. 66 regulated genes/ESTs) in Arc, especially those related to neurogenesis (Wang et al., 2012). These findings suggest that the opioid-system network that includes the dorsal horn, periaqueductal gray, nucleus raphe magnus, and the arcuate nucleus is crucial for electroanalgesia (Mayer et al., 1977; Han et al., 1991).
3.4.2. Cortical remodeling
3.4.2.1. Remodeling pattern of areas of sensorimotor integration
Maintaining synergy and appropriate sensory and motor function depends on the sensorimotor circuit’s integrity (Yao et al., 2022). PNI disrupts the sensorimotor circuit’s integrity and results in the loss of sensory and motor feedback, which may result in changes to the sensory-motor network’s intrinsic activity (Taylor et al., 2009). However, the adult brain is capable of profound plasticity (Li C. et al., 2021). The fMRI study showed that insertion of the acupuncture needle at acupoint ST 36 significantly affected the proprioceptive brain activation by decreasing blood oxygenation level-dependent signal intensity in basal ganglia, limbic system, and cerebellum (Lee et al., 2013), and EA could reduce chronic pain, so it can be used to treat the paresthesia brought on by PNI persisted (Lu et al., 2021). Current research showed that acupuncture at local versus distal sites may improve median nerve function at the wrist by somatotopically distinct neuroplasticity in the primary somatosensory cortex following therapy (Maeda et al., 2017). Some studies found that after sciatic nerve injury, local synaptic activity increased in the ipsilateral somatosensory cortex and decreased in the contralateral somatosensory cortex (Zuo et al., 2010; Nugent et al., 2015), and the plasticity changes following PNI were situated in homologous regions of the ipsilateral hemisphere in addition to the contralateral hemisphere (Fornander et al., 2016). Acupuncture at GB34 may increase motor-cognition connectivity meanwhile decrease compensation of unaffected motor cortex and homolateral synkinesis (Chen et al., 2015). EA at ST 36 and LI 11 could enhance the neural activity of motor function-related brain regions, including the motor cortex, dorsal thalamus, and striatum in rats (Liang et al., 2017). The results of resting-state functional MRI connectivity show that acupuncture induces significant connectivity changes in the primary somatosensory region of recovery patients with Bell’s palsy (He et al., 2014). Acupuncture increased sensorimotor network (SMN) connectivity with pain-related brain regions (ACC, cerebellum) (Dhond et al., 2008). Nervous damage causes the sensorimotor circuit to lose its integrity, which may prompt the brain to reorganize itself to make up for the damaged sensorimotor circuit. However, these alterations in the brain could be beneficial or harmful (Mohan and Vanneste, 2017). Although EA treatment might reverse the maladaptive cortical plasticity, the status of the brain has not recovered to a level consistent with normalcy (Wu et al., 2018a).
3.4.2.2. Pain network of brain region
It has been demonstrated in both a human and a rat model that nerve transection in PNI typically causes paresthesia or pain before reinnervation (Campbell and Meyer, 2006; Gu et al., 2016; Peng et al., 2016; Lim et al., 2017). Researchers found that the activity pattern of the region associated with pain was remarkably coordinated (Lefaucheur et al., 2009). The changes in regions of the brain, such as the thalamus, amygdala, and cingulate, involved in pain modulation were observed under EA stimulation. For Carpal Tunnel Syndrome patients responding to acupuncture, functional connectivity was found between the hypothalamus and amygdala--the less deactivation in the amygdala, the greater the activation in the hypothalamus, and vice versa. Furthermore, hypothalamic response correlated positively with the degree of maladaptive cortical plasticity in Carpal Tunnel Syndrome patients (inter-digit separation distance; Napadow et al., 2007a). The thalamus, which relays sensory data to the cerebral cortex and is the most significant sensory integration region, has a strong correlation with pain (Çetin et al., 2014; Zitnik et al., 2014). It is well known that the amygdala, hippocampus, and parahippocampus are crucial for regulating emotions, motivation, memory, and the affective aspect of pain (LeDoux, 2000; Zubieta et al., 2001; Mears and Pollard, 2016). Some findings demonstrate the additive effect of acupuncture on antidepressant treatment and suggest that this effect may be achieved through the amygdala and the anterior cingulate cortex (ACC) (Wang et al., 2016). Researchers found higher centrality in the parahippocampal gyrus and middle temporal gyrus after ST36 stimulation. These regions are positively correlated to major hubs of the default mode network, which might be the primary network affected by chronic pain (Long et al., 2016). Studies showed that conventional methods to enhance the acupuncture dose induce different DMN modulatory effects. Conventional methods of enhancing the acupuncture dose could potentially be applied as a means of modulating brain activity (Lin et al., 2016).
3.4.2.3. Limbic-paralimbic system
A group of brain regions known as the limbic system have been linked to emotion, memory, and sensation. Numerous earlier research showed that acupuncture not only stimulated sensorimotor regions of the brain but also deactivated extensive regions of the brain, including the limbic-paralimbic system (Fang et al., 2009; Napadow et al., 2009; Chae et al., 2013). The “Deqi” sensation has been described as a phenomenon of concerted attenuation of signal strength in the para-limbic regions through fMRI investigations. Additionally, acupuncture’s “Deqi” effects may be mediated by modulation of the limbic-paralimbic-neocortical network (Hui et al., 2005; Shi et al., 2016). Acupuncture’s effects on depression, schizophrenia, ischemic stroke, and Alzheimer’s disease may be brought on by the limbic system, according to some animal and clinical investigations (Lu et al., 2014; Bosch et al., 2015; Li et al., 2015; Wang et al., 2016). Researchers discovered that, while treating PNI following surgical repair, the brain plasticity generated by longitudinal EA intervention also occurred in limbic/paralimbic areas. The fMRI experiments of manual acupuncture at LI4, ST36, and LV3 showed the weakness of signal in several limbic, paralimbic, and neocortical areas (Kong et al., 2002; Hui et al., 2005; Napadow et al., 2005; Yan et al., 2005). Some hypothesize that voluntary slow deep breathing functionally resets the autonomic nervous system through stretch-induced inhibitory signals and hyperpolarization currents propagated through both neural and non-neural tissue which synchronizes neural elements in the heart, lungs, limbic system, and cortex (Jerath et al., 2006). The anterior cingulate cortex and nearby medial prefrontal cortex, insula, hippocampus, amygdala, and midcingulate cortices were consistently linked to peripheral markers of autonomic nervous system activity, according to recent meta-analyzes and other reviews (Thayer et al., 2012; Beissner et al., 2013; Gianaros and Wager, 2015; Myers, 2017). According to the hypothesis, the limbic system’s fluctuating behavior was caused by a conflict between the up-regulating effects of paresthesia or pain and the down-regulating effects of EA intervention (Beissner and Henke, 2011). Previous research suggested that acupuncture could promote the regeneration of peripheral nerves (He et al., 2015). It demonstrated the changes in the brain caused by the long-term therapeutic effects of EA, which were manifested as a synchronized pattern of activation in the somatosensory and pain-related areas, as well as a fluctuating pattern in the limbic/paralimbic system (Wu et al., 2018b).
3.4.3. White matter remodeling
According to some studies, corticospinal tract(CST) and spinothalamic tract (STT) fibers are similarly altered when peripheral nerve injury occurs (Zhang et al., 2019). Previous research demonstrated that neural pathway injuries, such as brain and spinal cord injuries, caused STT fibers to adapt or be maladapt (Wang and Thompson, 2008; Wasner et al., 2008). According to findings from a rat study, chronic pain stimuli of the sciatic nerve could lead to the hyperexcitability of ventral posterior thalamus neurons, which were the major termination of STT fibers and responsible for the signal relay (Miki et al., 2000). Neuronal hyperexcitability could be an inappropriate reaction to a reduction or absence of sensory input. Although there was little evidence that acupuncture improved sensory function, some researchers thought that the plasticity alteration in STT may also be predicted to restore sensory function. Previous studies found that acupuncture treatment has a complex effect on the corticospinal system for a variety of disorders (Chen et al., 2015; Yang et al., 2017). It is demonstrated that based on routine rehabilitation treatment, scalp acupuncture plus low-frequency rTMS can promote white matter tracts repair better than scalp acupuncture alone for stroke hemiplegic patients (Zhao N. et al., 2018). Prior research changed the focus of plasticity from gray matter, which includes the motor cortex, to white matter, which includes the CST. Cortical alterations were anticipated to lead to modifications in efferent neural pathways (Matsumoto-Miyazaki et al., 2016). Acupuncture also aided in the recovery process and ameliorated the efferent neural pathway’s longitudinal prognosis (Zou et al., 2019). Table 4 summarizes the evidence of the mechanism of acupuncture on the regulation of brain regions in peripheral nerve injury.
3.5. Regulation of neurotrophic factor associated with PNI by acupuncture
Peripheral nerves in mammals can regenerate after being damaged (Menorca et al., 2013; Scheib and Höke, 2013). Schwann cell proliferation is frequently thought to be related to the mechanism behind peripheral nerve regeneration. In particular, neurotrophic substances released by Schwann cells are crucial for the regeneration of peripheral nerves (Jessen et al., 2015). According to research, neurotrophic factors secreted by Schwann cells as well as extracellular matrix and cell adhesion molecules can induce, stimulate, and modulate axon regeneration and the development of myelin sheaths (Madduri and Gander, 2010). Based on this discovery, a proposal was put forth that the distal portions of the nerve guided the regeneration of peripheral nerves, helping to extend neurites and restore innervation through identification and communication between neurons and neurogliocytes. After the sciatic nerve cut off, some researchers found that Schwann cells produced S100, suggesting central nervous system damage. For peripheral nerve injury, low-frequency EA (5 Hz) had the better effect, and the results were most pronounced in the early stages (Cheng et al., 2014; Liao et al., 2017). Neurotrophic factors, cell adhesion molecules (including L1, NCAM, and N-cadherin), transcription factors, growth-stimulating agents, extracellular matrix components, intracellular signaling enzymes, and proteins regulating cell-surface cytoskeletal interactions are related to neuronal response (Figure 6).
3.5.1. Neurotrophic factors
Nerve growth factor (NGF) is the earliest discovered neurotrophic factor and can nourish neurons and promote neurite sprouting (Yu et al., 2014).In response to nerve damage, NGF expression was upregulated, creating the ideal conditions for axon regeneration, the restoration of the connection between axons and Schwann cells, and the reinnervation of target locations to enhance nerve growth (Tang et al., 2013). After peripheral nerve damage, EA may strengthen new axonal connections, which would ultimately accelerate the transfer of NGF. Additionally, EA was able to promote the growth of Schwann cells, which greatly increased the amount of NGF released (Hu et al., 2018).
In the peripheral nervous system, Schwann cells (SCs) are the predominant glial cells. Following PNI, SC migration and proliferation result in the release of a variety of neurotrophic factors (NTFs), which are beneficial for preserving the survival of injured neurons, encouraging nerve fiber regeneration, and fostering the formation of new synapses (Dai et al., 2013). It has been demonstrated that EA may promote the recovery of neuroplasticity in rats subjected to spinal cord transection. This could be attributed to the systematic regulation of NTFs and their receptors after EA.EA stimulation at ST36 in rats increases the expression of axonal growth-associated protein 43 in neurons of the dorsal root ganglia, promoting axonal development (Wang et al., 2017). EA may alleviate tibialis anterior muscle atrophy induced by sciatic nerve injection injury by upregulating agrin and AChR-ε and downregulating AChR-γ (Yu et al., 2017). Some studies found that EA increased NT-3 levels and promoted differentiation of oligodendrocyte-like cells from grafted NR-MSCs in the demyelinated spinal cord and induced MSC transplantation combined with EA treatment not only increased MSC differentiation into oligodendrocyte-like cells forming myelin sheaths but also promoted remyelination and functional improvement of nerve conduction in the demyelinated spinal cord (Liu et al., 2015). Some researchers indicate that electroacupuncture and moxibustion promoted nerve regeneration and functional recovery, which mechanism might be associated with the enhancement of Schwann cell proliferation and upregulation of nerve growth factor (Hu et al., 2018).
As one of the most significant NTFs, persistent exogenous BDNF release in the damaged peripheral nerve may be a helpful tool to boost axonal density (Lopes et al., 2017). In the recovery process after peripheral nerve injury in rats, electrical muscle stimulation increases intramuscular BDNF levels (Willand et al., 2016). Studies have shown that BDNF actively contributes to the regeneration of motor neurons and the restoration of motor function. The amount of the protein BDNF, which has been identified as a crucial regulator of axon regeneration, was significantly enhanced in the spinal cord and DRG following PNI (Santos et al., 2016; Sanna et al., 2017; McGregor and English, 2018).
Previous research demonstrated that glial cell-derived neurotrophic factor (GDNF) was the most effective survival factor described for motoneurons in vitro (Höke et al., 2002). Recent investigations have proven GDNF’s function in neuronal protection and axonal regeneration in vivo (Allen et al., 2013; Kopra et al., 2015). Researchers discovered that GDNF mRNA is expressed in the cytoplasm membrane, notably in the neuromuscular junctions, and less in the axons and Schwann cells (Suzuki et al., 1998). To protect neurons, GDNF binds to N-cadherin and initiates the intracellular PI3K/Akt signaling pathway (Wang et al., 2014). Researchers found that EA with high-frequency and long-term stimuli at acupoint ST-36 can induce regeneration of lost enteric neurons in diabetic rats, and GDNF and PI3K/Akt signal pathway may play an important role in EA-induced regeneration of impaired enteric neurons (Du and Liu, 2015).
3.5.2. N-cadherin
Cadherins tend to form dimers or multimers, and the extracellular peptide chain partially folds to form five or six repeat domains (Stevens et al., 2023). Calcium ions are bound between the repeat domains, thus imparting rigidity and strength to the cadherin molecule (Oroz et al., 2011). The more calcium ions are bound, the more rigid the cadherin is, so when calcium ions are removed, the rigidity of the extracellular part of the cadherin is lost. Researchers revealed that electroacupuncture may encourage nerve regeneration through an increase in calcium concentration, which activates neuron outgrowth and repair (McCaig et al., 2002). Similarly to NCAM and integrin 1, N-cadherin is a calcium-dependent neuronal cell surface protein that facilitates adhesion and signal transduction (Neugebauer et al., 1988). Ret has a cadherin-like domain in its extracellular region that interacts with the GDNF/GDNF family receptor α1 (Oppenheim et al., 1995; Kjaer and Ibáñez, 2003). Some studies have confirmed that electroacupuncture promotes regeneration of peripheral facial nerve injury in rabbits, inhibits neuronal apoptosis, and reduces peripheral inflammatory response, resulting in the recovery of facial muscle function, which is achieved by up-regulating the expression of GDNF and N-cadherin in central facial neurons (Fei et al., 2019). GDNF binds to N-cadherin, a transmembrane cell adhesion molecule, to exercise its neuroprotective impact on dopaminergic neurons (Cao et al., 2010; Patil et al., 2011; Zuo et al., 2013).
3.5.3. MicroRNAs
MicroRNAs (miRNAs), which comprise between 2 and 3 percent of all human genes and primarily function to silence genes by binding to people’s targets’ 3′ untranslated regions (3′ UTR), are a vital and significant component of the regulation over a variety of signaling pathways and biological functions in the restoration of PNI (Wanke et al., 2018; Treiber et al., 2019), so MiRNAs have gained growing interest in the field of peripheral nerve regeneration (Yu et al., 2015). Numerous studies have shown that miRNAs play a key role in the recovery process after PNI. For instance, miRNA expression in rat dorsal root ganglia (DRG) tissues was drastically altered following sciatic nerve damage (Li et al., 2012). To increase rat DRG neurons’ survival and prevent apoptosis following sciatic nerve damage, miR142-3p can target CDKN1B and TIMP3. MiR-7 influences repair following PNI by targeting cdc42 and preventing neural stem cell migration and proliferation. By targeting GF1-A-binding protein 1, miR-221-3p may prevent SCs from maturing into myelin sheaths when they are cocultured with DRG neurons (Nab1) (Zhao L. et al., 2018; Zhou et al., 2018). Researchers have demonstrated miR-1-targeting BDNF in the regulation of SC proliferation and migration following nerve injury; in addition, miR-1 modulated chronic neuropathic pain in rats by targeting cx43 and BDNF, and miR-206 can target BDNF to the regulation of the MERK-ERK signaling pathway to influence neuro stress pain (Neumann et al., 2015; Yi et al., 2016; Sun et al., 2017). Liu et al. (2018) have discovered that miR-1b overexpression inhibited RSC96’s migration and proliferation while boosting cell apoptosis. Similar targets and expression profiles are used to identify miRNAs that belong to the same family. Numerous miRNAs, including miR-129 and miR-195, had altered expression following PNI, according to studies (Shi et al., 2013; Zhu et al., 2018). Using miR-132 to target SOX2-mediated axonal regeneration, it has been found that EA improved neurobehavioral functional recovery after ischemic stroke (Zhao X. et al., 2018). According to another study, let-7b-5p, miR-148a-3p, miR-124-3p, miR-107-3p, and miR-370-3p were confirmed to participate in EA tolerance probably through the functional categories related to nerve impulse transmission, receptor signal pathways, and gene expression regulation, as well as pathways related to MAPK, neurotrophin, fatty acid metabolism, lysosome, and the degradation of valine, leucine, and isoleucine (Cui et al., 2017). MiR-1b expression was initially found to be highly downregulated in the local nerve following EA in a prior investigation, and EA may promote the proliferation, migration of SC, and nerve repair after PNI by regulating miR-1b, which targets BDNF (Liu et al., 2020). Table 5 summarizes the evidence of the mechanism of acupuncture on the regulation of factors associated with neuronal response in peripheral nerve injury.
4. Conclusion and consideration
With in-depth research on the mechanism underlying the effects of acupuncture in treating PNI, we now fully appreciate the importance of nerve system remodeling in this process. we have found that after peripheral nerve injury, not only is the injury limited to the local area of the nerve, but the entire nervous system is altered accordingly. Acupuncture not only reverses the poor remodeling of the nervous system but also activates the central opioid system and the innate immunological effector cells in the nervous system to ameliorate pain. Acupuncture can promote the proliferation and migration of Schwann cells and the release of neurotrophic substances such as nerve growth factors, accelerating the regeneration and repair of nerve fibers. Based on the view that acupuncture affects the remodeling of the nervous system, it is believed that in the treatment of peripheral nerve injury, acupuncture should not only be applied to the local acupoint of peripheral nerve injury but also be combined with scalp acupuncture and Jiaji acupoints. At present, there are many studies on the mechanism of acupuncture affecting nerve remodeling, but there are still many limitations. First, the reduction of inflammatory pain by acupuncture may be related to the interaction between glial cells and neurons in the spinal cord, but the mechanisms of action of microglia and astrocytes after acupuncture are different. It is not known which factors influence their activation timing and molecular signaling within the cell. Secondly, in the process of acupuncture treatment of peripheral nerve injury, the related neural circuit, functional area, and network connection at the central nervous system level are still not clear. Thirdly, it is known that neurotrophic factors are involved in the repair process of peripheral nerve injury, but the production site, production time, and transport route of neurotrophic factors during acupuncture treatment need to be further improved. Finally, current animal models have drawbacks because they show an acute inflammatory response and short-lived hyperalgesia after PNI, both of which become attenuated over time. Most studies have only examined the protective effect of acupuncture in the initial phase of PNI. Better models or clinical trials are needed to explore the effectiveness of acupuncture in the process of chronic nerve repair. Overall, this review of studies provides strong evidence for the usefulness of acupuncture in the treatment of PNI. The elucidation of the mechanisms underlying the effects of acupuncture in the treatment of PNI from the perspective of nervous system remodeling will open a variety of opportunities for further applications of acupuncture and a combination of acupuncture and drugs for treating, managing, and accelerating nerve repair. Therefore, the continuation of research on this topic is extremely important.
Author contributions
YY: Conceptualization, Data curation, Formal analysis, Methodology, Writing – original draft, Writing – review & editing. CR: Writing – review & editing. TY: Writing – review & editing. SW: Writing – review & editing. HS: Conceptualization, Methodology, Writing – review & editing. XY: Methodology, Writing – review & editing. LZ: Funding acquisition, Writing – review & editing. XM: Writing – review & editing. WG: Writing – review & editing. YD: Writing – review & editing. FH: Funding acquisition, Supervision, Validation, Writing – review & editing.
Funding
This work was supported by the National Natural Science Foundation of China, No. 82205257.
Conflict of interest
The authors declare that the research was conducted in the absence of any commercial or financial relationships that could be construed as a potential conflict of interest.
Publisher’s note
All claims expressed in this article are solely those of the authors and do not necessarily represent those of their affiliated organizations, or those of the publisher, the editors and the reviewers. Any product that may be evaluated in this article, or claim that may be made by its manufacturer, is not guaranteed or endorsed by the publisher.
References
Ahmad, I., Fernando, A., Gurgel, R., Jason Clark, J., Xu, L., and Hansen, M. R. (2015). Merlin status regulates p75(NTR) expression and apoptotic signaling in Schwann cells following nerve injury. Neurobiol. Dis. 82, 114–122. doi: 10.1016/j.nbd.2015.05.021
Ali, U., Apryani, E., Wu, H. Y., Mao, X. F., Liu, H., and Wang, Y. X. (2020). Low frequency electroacupuncture alleviates neuropathic pain by activation of spinal microglial IL-10/β-endorphin pathway. Biomed. Pharmacother. 125:109898. doi: 10.1016/j.biopha.2020.109898
Allen, S. J., Watson, J. J., Shoemark, D. K., Barua, N. U., and Patel, N. K. (2013). GDNF, NGF and BDNF as therapeutic options for neurodegeneration. Pharmacol. Ther. 138, 155–175. doi: 10.1016/j.pharmthera.2013.01.004
Asplund, M., Nilsson, M., Jacobsson, A., and Von Holst, H. (2009). Incidence of traumatic peripheral nerve injuries and amputations in Sweden between 1998 and 2006. Neuroepidemiology 32, 217–228. doi: 10.1159/000197900
Balogun, J. A., Biasci, S., and Han, L. (1998). The effects of acupuncture, electroneedling and transcutaneous electrical stimulation therapies on peripheral haemodynamic functioning. Disabil. Rehabil. 20, 41–48. doi: 10.3109/09638289809166052
Baumann, T. K., Simone, D. A., Shain, C. N., and LaMotte, R. H. (1991). Neurogenic hyperalgesia: the search for the primary cutaneous afferent fibers that contribute to capsaicin-induced pain and hyperalgesia. J. Neurophysiol. 66, 212–227. doi: 10.1152/jn.1991.66.1.212
Beissner, F., and Henke, C. (2011). Methodological problems in FMRI studies on acupuncture: a critical review with special emphasis on visual and auditory cortex activations. Evid. Based Complement. Alternat. Med. 2011:607637. doi: 10.1093/ecam/nep154
Beissner, F., Meissner, K., Bär, K. J., and Napadow, V. (2013). The autonomic brain: an activation likelihood estimation meta-analysis for central processing of autonomic function. J. Neurosci. 33, 10503–10511. doi: 10.1523/JNEUROSCI.1103-13.2013
Bosch, P., van den Noort, M., Staudte, H., and Lim, S. (2015). Schizophrenia and depression: a systematic review of the effectiveness and the working mechanisms behind acupuncture. Explore (N.Y.) 11, 281–291. doi: 10.1016/j.explore.2015.04.004
Bruyns, C. N., Jaquet, J. B., Schreuders, T. A., Kalmijn, S., Kuypers, P. D., and Hovius, S. E. (2003). Predictors for return to work in patients with median and ulnar nerve injuries. J. Hand Surg. Am. 28, 28–34. doi: 10.1053/jhsu.2003.50026
Campbell, J. N., and Meyer, R. A. (2006). Mechanisms of neuropathic pain. Neuron 52, 77–92. doi: 10.1016/j.neuron.2006.09.021
Cao, J. P., Li, F. Z., Zhu, Y. Y., Yuan, H. H., Yu, Z. Q., and Gao, D. S. (2010). Expressions and possible roles of GDNF receptors in the developing dopaminergic neurons. Brain Res. Bull. 83, 321–330. doi: 10.1016/j.brainresbull.2010.09.009
Çetin, M. S., Christensen, F., Abbott, C. C., Stephen, J. M., Mayer, A. R., Cañive, J. M., et al. (2014). Thalamus and posterior temporal lobe show greater inter-network connectivity at rest and across sensory paradigms in schizophrenia. NeuroImage 97, 117–126. doi: 10.1016/j.neuroimage.2014.04.009
Chae, Y., Chang, D. S., Lee, S. H., Jung, W. M., Lee, I. S., Jackson, S., et al. (2013). Inserting needles into the body: a meta-analysis of brain activity associated with acupuncture needle stimulation. J. Pain 14, 215–222. doi: 10.1016/j.jpain.2012.11.011
Chang, S., Kim, D. H., Jang, E. Y., Yoon, S. S., Gwak, Y. S., Yi, Y. J., et al. (2019). Acupuncture attenuates alcohol dependence through activation of endorphinergic input to the nucleus accumbens from the arcuate nucleus. Sci. Adv. 5:eaax1342. doi: 10.1126/sciadv.aax1342
Chen, C. Y., Ke, M. D., Kuo, C. D., Huang, C. H., Hsueh, Y. H., and Chen, J. R. (2013). The influence of electro-acupuncture stimulation to female constipation patients. Am. J. Chin. Med. 41, 301–313. doi: 10.1142/S0192415X13500225
Chen, X., Zhang, H., and Zou, Y. (2015). A functional magnetic resonance imaging study on the effect of acupuncture at GB34 (Yanglingquan) on motor-related network in hemiplegic patients. Brain Res. 1601, 64–72. doi: 10.1016/j.brainres.2015.01.011
Cheng, Y. S., Cheng, W. C., Yao, C. H., Hsieh, C. L., Lin, J. G., Lai, T. Y., et al. (2001). Effects of buyang huanwu decoction on peripheral nerve regeneration using silicone rubber chambers. Am. J. Chin. Med. 29, 423–432. doi: 10.1142/S0192415X01000447
Cheng, C. Y., Lin, J. G., Tang, N. Y., Kao, S. T., and Hsieh, C. L. (2014). Electroacupuncture-like stimulation at the Baihui (GV20) and Dazhui (GV14) acupoints protects rats against subacute-phase cerebral ischemia-reperfusion injuries by reducing S100B-mediated neurotoxicity. PLoS One 9:e91426. doi: 10.1371/journal.pone.0091426
Chou, A. K., Yang, M. C., Tsai, H. P., Chai, C. Y., Tai, M. H., Kwan, A. L., et al. (2014). Adenoviral-mediated glial cell line-derived neurotrophic factor gene transfer has a protective effect on sciatic nerve following constriction-induced spinal cord injury. PLoS One 9:e92264. doi: 10.1371/journal.pone.0092264
Chu, Y. X., Zhang, Y., Zhang, Y. Q., and Zhao, Z. Q. (2010). Involvement of microglial P2X7 receptors and downstream signaling pathways in long-term potentiation of spinal nociceptive responses. Brain Behav. Immun. 24, 1176–1189. doi: 10.1016/j.bbi.2010.06.001
Cui, L., Ding, Y., Feng, Y., Chen, S., Xu, Y., Li, M., et al. (2017). MiRNAs are involved in chronic electroacupuncture tolerance in the rat hypothalamus. Mol. Neurobiol. 54, 1429–1439. doi: 10.1007/s12035-016-9759-8
Dai, L. G., Huang, G. S., and Hsu, S. H. (2013). Sciatic nerve regeneration by cocultured Schwann cells and stem cells on microporous nerve conduits. Cell Transplant. 22, 2029–2039. doi: 10.3727/096368912X658953
Dhond, R. P., Yeh, C., Park, K., Kettner, N., and Napadow, V. (2008). Acupuncture modulates resting state connectivity in default and sensorimotor brain networks. Pain 136, 407–418. doi: 10.1016/j.pain.2008.01.011
Dimitrova, A., Murchison, C., and Oken, B. (2017). Acupuncture for the treatment of peripheral neuropathy: a systematic review and Meta-analysis. J. Altern. Complement. Med. 23, 164–179. doi: 10.1089/acm.2016.0155
Du, F., and Liu, S. (2015). Electroacupuncture with high frequency at acupoint ST-36 induces regeneration of lost enteric neurons in diabetic rats via GDNF and PI3K/AKT signal pathway. Am. J. Physiol. Regul. Integr. Comp. Physiol. 309, R109–R118. doi: 10.1152/ajpregu.00396.2014
Fan, Y., and Wu, Y. (2015). Effect of electroacupuncture on muscle state and infrared thermogram changes in patients with acute lumbar muscle sprain. J. Tradit. Chin. Med. 35, 499–506. doi: 10.1016/s0254-6272(15)30131-x
Fang, J., Jin, Z., Wang, Y., Li, K., Kong, J., Nixon, E. E., et al. (2009). The salient characteristics of the central effects of acupuncture needling: limbic-paralimbic-neocortical network modulation. Hum. Brain Mapp. 30, 1196–1206. doi: 10.1002/hbm.20583
Fawcett, J. W., and Keynes, R. J. (1990). Peripheral nerve regeneration. Annu. Rev. Neurosci. 13, 43–60. doi: 10.1146/annurev.ne.13.030190.000355
Fei, J., Gao, L., Li, H. H., Yuan, Q. L., and Li, L. J. (2019). Electroacupuncture promotes peripheral nerve regeneration after facial nerve crush injury and upregulates the expression of glial cell-derived neurotrophic factor. Neural Regen. Res. 14, 673–682. doi: 10.4103/1673-5374.247471
Fornander, L., Nyman, T., Hansson, T., Brismar, T., and Engström, M. (2016). Inter-hemispheric plasticity in patients with median nerve injury. Neurosci. Lett. 628, 59–66. doi: 10.1016/j.neulet.2016.06.018
Gianaros, P. J., and Wager, T. D. (2015). Brain-body pathways linking psychological stress and physical health. Curr. Dir. Psychol. Sci. 24, 313–321. doi: 10.1177/0963721415581476
Gordon, T., and Borschel, G. H. (2017). The use of the rat as a model for studying peripheral nerve regeneration and sprouting after complete and partial nerve injuries. Exp. Neurol. 287, 331–347. doi: 10.1016/j.expneurol.2016.01.014
Gu, N., Peng, J., Murugan, M., Wang, X., Eyo, U. B., Sun, D., et al. (2016). Spinal microgliosis due to resident microglial proliferation is required for pain hypersensitivity after peripheral nerve injury. Cell Rep. 16, 605–614. doi: 10.1016/j.celrep.2016.06.018
Han, J. S., Chen, X. H., Sun, S. L., Xu, X. J., Yuan, Y., Yan, S. C., et al. (1991). Effect of low- and high-frequency TENS on met-enkephalin-Arg-Phe and dynorphin a immunoreactivity in human lumbar CSF. Pain 47, 295–298. doi: 10.1016/0304-3959(91)90218-M
Hao, J., Zhao, C., Cao, S., and Yang, S. (1995). Electric acupuncture treatment of peripheral nerve injury. J. Tradit. Chin. Med. 15, 114–117.
He, G. H., Ruan, J. W., Zeng, Y. S., Zhou, X., Ding, Y., and Zhou, G. H. (2015). Improvement in acupoint selection for acupuncture of nerves surrounding the injury site: electro-acupuncture with governor vessel with local meridian acupoints. Neural Regen. Res. 10, 128–135. doi: 10.4103/1673-5374.150720
He, X., Zhu, Y., Li, C., Park, K., Mohamed, A. Z., Wu, H., et al. (2014). Acupuncture-induced changes in functional connectivity of the primary somatosensory cortex varied with pathological stages of Bell’s palsy. Neuroreport 25, 1162–1168. doi: 10.1097/WNR.0000000000000246
Ho, C. Y., Lin, H. C., Lee, Y. C., Chou, L. W., Kuo, T. W., Chang, H. W., et al. (2014). Clinical effectiveness of acupuncture for carpal tunnel syndrome. Am. J. Chin. Med. 42, 303–314. doi: 10.1142/S0192415X14500207
Ho, C. Y., Yao, C. H., Chen, W. C., Shen, W. C., and Bau, D. T. (2013). Electroacupuncture and acupuncture promote the Rat's transected median nerve regeneration. Evid. Based Complement. Alternat. Med. 2013:514610. doi: 10.1155/2013/514610
Höke, A., Gordon, T., Zochodne, D. W., and Sulaiman, O. A. (2002). A decline in glial cell-line-derived neurotrophic factor expression is associated with impaired regeneration after long-term Schwann cell denervation. Exp. Neurol. 173, 77–85. doi: 10.1006/exnr.2001.7826
Hu, L. N., Tian, J. X., Gao, W., Zhu, J., Mou, F. F., Ye, X. C., et al. (2018). Electroacupuncture and moxibustion promote regeneration of injured sciatic nerve through Schwann cell proliferation and nerve growth factor secretion. Neural Regen. Res. 13, 477–483. doi: 10.4103/1673-5374.228731
Hoang, N. S., Sar, C., Valmier, J., and Sieso, V. (2012). Scamps, Electro-acupuncture on functional peripheral nerve regeneration in mice: a behavioural study. BMC Complement Altern Med. 12: 141.
Hui, K. K., Liu, J., Makris, N., Gollub, R. L., Chen, A. J., Moore, C. I., et al. (2000). Acupuncture modulates the limbic system and subcortical gray structures of the human brain: evidence from fMRI studies in normal subjects. Hum Brain Mapp. 9, 13–25.
Hui, K. K., Liu, J., Marina, O., Napadow, V., Haselgrove, C., Kwong, K. K., et al. (2005). The integrated response of the human cerebro-cerebellar and limbic systems to acupuncture stimulation at ST 36 as evidenced by fMRI. NeuroImage 27, 479–496. doi: 10.1016/j.neuroimage.2005.04.037
Inoue, M., Hojo, T., Yano, T., and Katsumi, Y. (2003). The effects of electroacupuncture on peripheral nerve regeneration in rats. Acupunct. Med. 21, 9–17. doi: 10.1136/aim.21.1-2.9
Inoue, M., Katsumi, Y., Itoi, M., Hojo, T., Nakajima, M., Ohashi, S., et al. (2011). Direct current electrical stimulation of acupuncture needles for peripheral nerve regeneration: an exploratory case series. Acupunct. Med. 29, 88–93. doi: 10.1136/aim.2010.003046
Inquimbert, P., Moll, M., Latremoliere, A., Tong, C. K., Whang, J., Sheehan, G. F., et al. (2018). NMDA receptor activation underlies the loss of spinal dorsal horn neurons and the transition to persistent pain after peripheral nerve injury. Cell Rep. 23, 2678–2689. doi: 10.1016/j.celrep.2018.04.107
Jerath, R., Edry, J. W., Barnes, V. A., and Jerath, V. (2006). Physiology of long pranayamic breathing: neural respiratory elements may provide a mechanism that explains how slow deep breathing shifts the autonomic nervous system. Med. Hypotheses 67, 566–571. doi: 10.1016/j.mehy.2006.02.042
Jessen, K. R., Mirsky, R., and Lloyd, A. C. (2015). Schwann cells: development and role in nerve repair. Cold Spring Harb. Perspect. Biol. 7:a020487. doi: 10.1101/cshperspect.a020487
Jung, W. M., Lee, I. S., Wallraven, C., Ryu, Y. H., Park, H. J., and Chae, Y. (2015). Cortical activation patterns of bodily attention triggered by acupuncture stimulation. Sci. Rep. 5:12455. doi: 10.1038/srep12455
Kim, J., and Choi, J. Y. (2016). The effect of subthreshold continuous electrical stimulation on the facial function of patients with Bell’s palsy. Acta Otolaryngol. 136, 100–105. doi: 10.3109/00016489.2015.1083121
Kim, H. Y., Wang, J., Lee, I., Kim, H. K., Chung, K., and Chung, J. M. (2009). Electroacupuncture suppresses capsaicin-induced secondary hyperalgesia through an endogenous spinal opioid mechanism. Pain 145, 332–340. doi: 10.1016/j.pain.2009.06.035
Kingham, P. J., Kolar, M. K., Novikova, L. N., Novikov, L. N., and Wiberg, M. (2014). Stimulating the neurotrophic and angiogenic properties of human adipose-derived stem cells enhances nerve repair. Stem Cells Dev. 23, 741–754. doi: 10.1089/scd.2013.0396
Kjaer, S., and Ibáñez, C. F. (2003). Identification of a surface for binding to the GDNF-GFR alpha 1 complex in the first cadherin-like domain of RET. J. Biol. Chem. 278, 47898–47904. doi: 10.1074/jbc.M309772200
Klingner, C. M., Volk, G. F., Brodoehl, S., Witte, O. W., and Guntinas-Lichius, O. (2014). The effects of deefferentation without deafferentation on functional connectivity in patients with facial palsy. Neuroimage Clin. 6, 26–31. doi: 10.1016/j.nicl.2014.08.011
Kong, J., Kaptchuk, T. J., Polich, G., Kirsch, I., Vangel, M., Zyloney, C., et al. (2009). An fMRI study on the interaction and dissociation between expectation of pain relief and acupuncture treatment. NeuroImage 47, 1066–1076. doi: 10.1016/j.neuroimage.2009.05.087
Kong, J., Ma, L., Gollub, R. L., Wei, J., Yang, X., Li, D., et al. (2002). A pilot study of functional magnetic resonance imaging of the brain during manual and electroacupuncture stimulation of acupuncture point (LI-4 Hegu) in normal subjects reveals differential brain activation between methods. J. Altern. Complement. Med. 8, 411–419. doi: 10.1089/107555302760253603
Koo, S. T., Lim, K. S., Chung, K., Ju, H., and Chung, J. M. (2008). Electroacupuncture-induced analgesia in a rat model of ankle sprain pain is mediated by spinal alpha-adrenoceptors. Pain 135, 11–19. doi: 10.1016/j.pain.2007.04.034
Kopra, J., Vilenius, C., Grealish, S., Härma, M. A., Varendi, K., Lindholm, J., et al. (2015). GDNF is not required for catecholaminergic neuron survival in vivo. Nat. Neurosci. 18, 319–322. doi: 10.1038/nn.3941
Kwon, Y. B., Han, H. J., Beitz, A. J., and Lee, J. H. (2004). Bee venom acupoint stimulation increases Fos expression in catecholaminergic neurons in the rat brain. Mol. Cells 17, 329–333. doi: 10.1016/j.jmb.2004.02.047
LeDoux, J. E. (2000). Emotion circuits in the brain. Annu. Rev. Neurosci. 23, 155–184. doi: 10.1146/annurev.neuro.23.1.155
Lee, S. H., Jahng, G. H., Choe, I. H., Choi, C. B., Kim, D. H., and Kim, H. Y. (2013). Neural pathway interference by retained acupuncture: a functional MRI study of a dog model of Parkinson's disease. CNS Neurosci. Ther. 19, 585–595. doi: 10.1111/cns.12108
Lefaucheur, J. P., Drouot, X., Cunin, P., Bruckert, R., Lepetit, H., Créange, A., et al. (2009). Motor cortex stimulation for the treatment of refractory peripheral neuropathic pain. Brain 132, 1463–1471. doi: 10.1093/brain/awp035
Levine, R., Morgan, M. M., Cannon, J. T., and Liebeskind, J. C. (1991). Stimulation of the periaqueductal gray matter of the rat produces a preferential ipsilateral antinociception. Brain Res. 567, 140–144. doi: 10.1016/0006-8993(91)91446-8
Li, M. K., Li, Y. J., Zhang, G. F., Chen, J. Q., Zhang, J. P., Qi, J., et al. (2015). Acupuncture for ischemic stroke: cerebellar activation may be a central mechanism following Deqi. Neural Regen. Res. 10, 1997–2003. doi: 10.4103/1673-5374.172318
Li, C., Liu, S. Y., Pi, W., and Zhang, P. X. (2021). Cortical plasticity and nerve regeneration after peripheral nerve injury. Neural Regen. Res. 16, 1518–1523. doi: 10.4103/1673-5374.303008
Li, A., Wang, Y., Xin, J., Lao, L., Ren, K., Berman, B. M., et al. (2007). Electroacupuncture suppresses hyperalgesia and spinal Fos expression by activating the descending inhibitory system. Brain Res. 1186, 171–179. doi: 10.1016/j.brainres.2007.10.022
Li, S., Yu, B., Wang, S., Gu, Y., Yao, D., Wang, Y., et al. (2012). Identification and functional analysis of novel micro-RNAs in rat dorsal root ganglia after sciatic nerve resection. J. Neurosci. Res. 90, 791–801. doi: 10.1002/jnr.22814
Li, H., Yu, L., Ye, D., Chang, L., Zhao, F., Wang, H., et al. (2021). Rehabilitation training combined with Jiaji Electroacupuncture can promote the recovery of muscle group function and improve the quality of life in patients with upper limb peripheral nerve injury. J. Healthc. Eng. 2021:3621568. doi: 10.1155/2021/3621568
Liang, Y., Du, J. Y., Qiu, Y. J., Fang, J. F., Liu, J., and Fang, J. Q. (2016). Electroacupuncture attenuates spinal nerve ligation-induced microglial activation mediated by p38 mitogen-activated protein kinase. Chin. J. Integr. Med. 22, 704–713. doi: 10.1007/s11655-015-2045-1
Liang, S., Lin, Y., Lin, B., Li, J., Liu, W., Chen, L., et al. (2017). Resting-state functional magnetic resonance imaging analysis of brain functional activity in rats with ischemic stroke treated by electro-acupuncture. J. Stroke Cerebrovasc. Dis. 26, 1953–1959. doi: 10.1016/j.jstrokecerebrovasdis.2017.06.018
Liao, H. Y., Hsieh, C. L., Huang, C. P., and Lin, Y. W. (2017). Electroacupuncture attenuates CFA-induced inflammatory pain by suppressing Nav1.8 through S100B, TRPV1, opioid, and adenosine pathways in mice. Sci. Rep. 7:42531. doi: 10.1038/srep42531
Lim, H., Lee, H., Noh, K., and Lee, S. J. (2017). IKK/NF-κB-dependent satellite glia activation induces spinal cord microglia activation and neuropathic pain after nerve injury. Pain 158, 1666–1677. doi: 10.1097/j.pain.0000000000000959
Lin, J. P., Chen, C. Q., Huang, L. E., Li, N. N., Yang, Y., Zhu, S. M., et al. (2018). Dexmedetomidine attenuates neuropathic pain by inhibiting P2X7R expression and ERK phosphorylation in rats. Exp. Neurobiol. 27, 267–276. doi: 10.5607/en.2018.27.4.267
Lin, Y. J., Kung, Y. Y., Kuo, W. J., Niddam, D. M., Chou, C. C., Cheng, C. M., et al. (2016). Effect of acupuncture 'dose' on modulation of the default mode network of the brain. Acupunct. Med. 34, 425–432. doi: 10.1136/acupmed-2016-011071
Liu, Y., Grumbles, R. M., and Thomas, C. K. (2013). Electrical stimulation of embryonic neurons for 1 hour improves axon regeneration and the number of reinnervated muscles that function. J. Neuropathol. Exp. Neurol. 72, 697–707. doi: 10.1097/NEN.0b013e318299d376
Liu, Z., He, B., Zhang, R. Y., Zhang, K., Ding, Y., Ruan, J. W., et al. (2015). Electroacupuncture promotes the differentiation of transplanted bone marrow mesenchymal stem cells Preinduced with Neurotrophin-3 and retinoic acid into oligodendrocyte-like cells in demyelinated spinal cord of rats. Cell Transplant. 24, 1265–1281. doi: 10.3727/096368914X682099
Liu, Y. P., Luo, Z. R., Wang, C., Cai, H., Zhao, T. T., Li, H., et al. (2020). Electroacupuncture promoted nerve repair after peripheral nerve injury by regulating miR-1b and its target brain-derived neurotrophic factor. Front. Neurosci. 14:525144. doi: 10.3389/fnins.2020.525144
Liu, S., Wang, Z., Su, Y., Qi, L., Yang, W., Fu, M., et al. (2021). A neuroanatomical basis for electroacupuncture to drive the vagal-adrenal axis. Nature 598, 641–645. doi: 10.1038/s41586-021-04001-4
Liu, Y. P., Xu, P., Guo, C. X., Luo, Z. R., Zhu, J., Mou, F. F., et al. (2018). miR-1b overexpression suppressed proliferation and migration of RSC96 and increased cell apoptosis. Neurosci. Lett. 687, 137–145. doi: 10.1016/j.neulet.2018.09.041
Long, X., Huang, W., Napadow, V., Liang, F., Pleger, B., Villringer, A., et al. (2016). Sustained effects of acupuncture stimulation investigated with centrality mapping analysis. Front. Hum. Neurosci. 10:510. doi: 10.3389/fnhum.2016.00510
Lopes, C. D. F., Gonçalves, N. P., Gomes, C. P., Saraiva, M. J., and Pêgo, A. P. (2017). BDNF gene delivery mediated by neuron-targeted nanoparticles is neuroprotective in peripheral nerve injury. Biomaterials 121, 83–96. doi: 10.1016/j.biomaterials.2016.12.025
Lu, M. C., Ho, C. Y., Hsu, S. F., Lee, H. C., Lin, J. H., Yao, C. H., et al. (2008). Effects of electrical stimulation at different frequencies on regeneration of transected peripheral nerve. Neurorehabil. Neural Repair 22, 367–373. doi: 10.1177/1545968307313507
Lu, Y., Huang, Y., Tang, C., Shan, B., Cui, S., Yang, J., et al. (2014). Brain areas involved in the acupuncture treatment of AD model rats: a PET study. BMC Complement. Altern. Med. 14:178. doi: 10.1186/1472-6882-14-178
Lu, Z., Wang, Q., Sun, X., Zhang, W., Min, S., Zhang, J., et al. (2021). Transcutaneous electrical acupoint stimulation before surgery reduces chronic pain after mastectomy: a randomized clinical trial. J. Clin. Anesth. 74:110453. doi: 10.1016/j.jclinane.2021.110453
Madduri, S., and Gander, B. (2010). Schwann cell delivery of neurotrophic factors for peripheral nerve regeneration. J. Peripher. Nerv. Syst. 15, 93–103. doi: 10.1111/j.1529-8027.2010.00257.x
Maeda, Y., Kim, H., Kettner, N., Kim, J., Cina, S., Malatesta, C., et al. (2017). Rewiring the primary somatosensory cortex in carpal tunnel syndrome with acupuncture. Brain 140, 914–927. doi: 10.1093/brain/awx015
Magistroni, E., Parodi, G., Fop, F., Battiston, B., and Dahlin, L. B. (2020). Cold intolerance and neuropathic pain after peripheral nerve injury in upper extremity. J. Peripher. Nerv. Syst. 25, 184–190. doi: 10.1111/jns.12376
Manning, B. H. (1998). A lateralized deficit in morphine antinociception after unilateral inactivation of the central amygdala. J. Neurosci. 18, 9453–9470. doi: 10.1523/JNEUROSCI.18-22-09453.1998
Mathiowetz, V., Kashman, N., Volland, G., Weber, K., Dowe, M., and Rogers, S. (1985). Grip and pinch strength: normative data for adults. Arch. Phys. Med. Rehabil. 66, 69–74.
Matsumoto-Miyazaki, J., Asano, Y., Yonezawa, S., Nomura, Y., Ikegame, Y., Aki, T., et al. (2016). Acupuncture increases the excitability of the Cortico-spinal system in patients with chronic disorders of consciousness following traumatic brain injury. J. Altern. Complement. Med. 22, 887–894. doi: 10.1089/acm.2014.0356
Mayer, D. J., Price, D. D., and Rafii, A. (1977). Antagonism of acupuncture analgesia in man by the narcotic antagonist naloxone. Brain Res. 121, 368–372. doi: 10.1016/0006-8993(77)90161-5
McCaig, C. D., Rajnicek, A. M., Song, B., and Zhao, M. (2002). Has electrical growth cone guidance found its potential? Trends Neurosci. 25, 354–359. doi: 10.1016/S0166-2236(02)02174-4
McGregor, C. E., and English, A. W. (2018). The role of BDNF in peripheral nerve regeneration: activity-dependent treatments and Val66Met. Front. Cell. Neurosci. 12:522. doi: 10.3389/fncel.2018.00522
Mears, D., and Pollard, H. B. (2016). Network science and the human brain: using graph theory to understand the brain and one of its hubs, the amygdala, in health and disease. J. Neurosci. Res. 94, 590–605. doi: 10.1002/jnr.23705
Menorca, R. M., Fussell, T. S., and Elfar, J. C. (2013). Nerve physiology: mechanisms of injury and recovery. Hand Clin. 29, 317–330. doi: 10.1016/j.hcl.2013.04.002
Miki, K., Iwata, K., Tsuboi, Y., Morimoto, T., Kondo, E., Dai, Y., et al. (2000). Dorsal column-thalamic pathway is involved in thalamic hyperexcitability following peripheral nerve injury: a lesion study in rats with experimental mononeuropathy. Pain 85, 263–271. doi: 10.1016/s0304-3959(99)00279-1
Mohan, A., and Vanneste, S. (2017). Adaptive and maladaptive neural compensatory consequences of sensory deprivation-from a phantom percept perspective. Prog. Neurobiol. 153, 1–17. doi: 10.1016/j.pneurobio.2017.03.010
Myers, B. (2017). Corticolimbic regulation of cardiovascular responses to stress. Physiol. Behav. 172, 49–59. doi: 10.1016/j.physbeh.2016.10.015
Napadow, V., Dhond, R. P., Kim, J., LaCount, L., Vangel, M., Harris, R. E., et al. (2009). Brain encoding of acupuncture sensation--coupling on-line rating with fMRI. NeuroImage 47, 1055–1065. doi: 10.1016/j.neuroimage.2009.05.079
Napadow, V., Kettner, N., Liu, J., Li, M., Kwong, K. K., Vangel, M., et al. (2007a). Hypothalamus and amygdala response to acupuncture stimuli in carpal tunnel syndrome. Pain 130, 254–266. doi: 10.1016/j.pain.2006.12.003
Napadow, V., Liu, J., Li, M., Kettner, N., Ryan, A., Kwong, K. K., et al. (2007b). Somatosensory cortical plasticity in carpal tunnel syndrome treated by acupuncture. Hum. Brain Mapp. 28, 159–171. doi: 10.1002/hbm.20261
Napadow, V., Makris, N., Liu, J., Kettner, N. W., Kwong, K. K., and Hui, K. K. (2005). Effects of electroacupuncture versus manual acupuncture on the human brain as measured by fMRI. Hum. Brain Mapp. 24, 193–205. doi: 10.1002/hbm.20081
Neugebauer, K. M., Tomaselli, K. J., Lilien, J., and Reichardt, L. F. (1988). N-cadherin, NCAM, and integrins promote retinal neurite outgrowth on astrocytes in vitro. J. Cell Biol. 107, 1177–1187. doi: 10.1083/jcb.107.3.1177
Neumann, E., Hermanns, H., Barthel, F., Werdehausen, R., and Brandenburger, T. (2015). Expression changes of microRNA-1 and its targets Connexin 43 and brain-derived neurotrophic factor in the peripheral nervous system of chronic neuropathic rats. Mol. Pain 11:39. doi: 10.1186/s12990-015-0045-y
Nugent, A. C., Martinez, A., D'Alfonso, A., Zarate, C. A., and Theodore, W. H. (2015). The relationship between glucose metabolism, resting-state fMRI BOLD signal, and GABAA-binding potential: a preliminary study in healthy subjects and those with temporal lobe epilepsy. J. Cereb. Blood Flow Metab. 35, 583–591. doi: 10.1038/jcbfm.2014.228
Oppenheim, R. W., Houenou, L. J., Johnson, J. E., Lin, L. F., Li, L., Lo, A. C., et al. (1995). Developing motor neurons rescued from programmed and axotomy-induced cell death by GDNF. Nature 373, 344–346. doi: 10.1038/373344a0
Oroz, J., Valbuena, A., Vera, A. M., Mendieta, J., Gómez-Puertas, P., and Carrión-Vázquez, M. (2011). Nanomechanics of the cadherin ectodomain: "canalization" by Ca2+ binding results in a new mechanical element. J. Biol. Chem. 286, 9405–9418. doi: 10.1074/jbc.M110.170399
Pagnussat, A. S., Michaelsen, S. M., Achaval, M., Ilha, J., Hermel, E. E., Back, F. P., et al. (2012). Effect of skilled and unskilled training on nerve regeneration and functional recovery. Braz. J. Med. Biol. Res. 45, 753–762. doi: 10.1590/S0100-879X2012007500084
Pan, H., Huang, H., Zhang, L., Ma, S., Yang, H., and Wang, H. (2019). "adjusting internal organs and dredging channel" electroacupuncture treatment prevents the development of diabetic peripheral neuropathy by downregulating glucose-related protein 78 (GRP78) and caspase-12 in streptozotocin-diabetic rats. J. Diabetes 11, 928–937. doi: 10.1111/1753-0407.12916
Patel, N., and Poo, M. M. (1982). Orientation of neurite growth by extracellular electric fields. J. Neurosci. 2, 483–496. doi: 10.1523/JNEUROSCI.02-04-00483.1982
Patil, S. B., Brock, J. H., Colman, D. R., and Huntley, G. W. (2011). Neuropathic pain- and glial derived neurotrophic factor-associated regulation of cadherins in spinal circuits of the dorsal horn. Pain 152, 924–935. doi: 10.1016/j.pain.2011.01.017
Peng, J., Gu, N., Zhou, L., Murugan, B. E. U., Gan, W. B., and Wu, L. J. (2016). Microglia and monocytes synergistically promote the transition from acute to chronic pain after nerve injury. Nat. Commun. 7:12029. doi: 10.1038/ncomms12029
Ren, L., Zhang, W. A., Fang, N. Y., and Wang, J. X. (2008). The influence of electro-acupuncture on neural plasticity in acute cerebral infarction. Neurol Res 30, 985–9
Ribeiro, F. F., Xapelli, S., Miranda-Lourenço, C., Tanqueiro, S. R., Fonseca-Gomes, J., Diógenes, M. J., et al. (2016). Purine nucleosides in neuroregeneration and neuroprotection. Neuropharmacology 104, 226–242. doi: 10.1016/j.neuropharm.2015.11.006
Sanna, M. D., Ghelardini, C., and Galeotti, N. (2017). HuD-mediated distinct BDNF regulatory pathways promote regeneration after nerve injury. Brain Res. 1659, 55–63. doi: 10.1016/j.brainres.2017.01.019
Santos, D., González-Pérez, F., Giudetti, G., Micera, S., Udina, E., Del Valle, J., et al. (2016). Preferential enhancement of sensory and motor axon regeneration by combining extracellular matrix components with neurotrophic factors. Int. J. Mol. Sci. 18:65. doi: 10.3390/ijms18010065
Scheib, J., and Höke, A. (2013). Advances in peripheral nerve regeneration. Nat. Rev. Neurol. 9, 668–676. doi: 10.1038/nrneurol.2013.227
Schmidt, C. E., Shastri, V. R., Vacanti, J. P., and Langer, R. (1997). Stimulation of neurite outgrowth using an electrically conducting polymer. Proc. Natl. Acad. Sci. U. S. A. 94, 8948–8953. doi: 10.1073/pnas.94.17.8948
Shan, S., Qi-Liang, M. Y., Hong, C., Tingting, L., Mei, H., Haili, P., et al. (2007). Is functional state of spinal microglia involved in the anti-allodynic and anti-hyperalgesic effects of electroacupuncture in rat model of monoarthritis? Neurobiol. Dis. 26, 558–568. doi: 10.1016/j.nbd.2007.02.007
Shen, Y., Guan, S., Ge, H., Xiong, W., He, L., Liu, L., et al. (2018). Effects of palmatine on rats with comorbidity of diabetic neuropathic pain and depression. Brain Res. Bull. 139, 56–66. doi: 10.1016/j.brainresbull.2018.02.005
Shi, G., Shi, J., Liu, K., Liu, N., Wang, Y., Fu, Z., et al. (2013). Increased miR-195 aggravates neuropathic pain by inhibiting autophagy following peripheral nerve injury. Glia 61, 504–512. doi: 10.1002/glia.22451
Shi, Y., Zhang, S., Li, Q., Liu, Z., Guo, S., Yang, J., et al. (2016). A study of the brain functional network of Deqi via acupuncturing stimulation at BL40 by rs-fMRI. Complement. Ther. Med. 25, 71–77. doi: 10.1016/j.ctim.2016.01.004
Song, B., Zhao, M., Forrester, J., and McCaig, C. (2004). Nerve regeneration and wound healing are stimulated and directed by an endogenous electrical field in vivo. J. Cell Sci. 117, 4681–4690. doi: 10.1242/jcs.01341
Stevens, A. J., Harris, A. R., Gerdts, J., Kim, K. H., Trentesaux, C., Ramirez, J. T., et al. (2023). Programming multicellular assembly with synthetic cell adhesion molecules. Nature 614, 144–152. doi: 10.1038/s41586-022-05622-z
Sturma, A., Hruby, L. A., Prahm, C., Mayer, J. A., and Aszmann, O. C. (2018). Rehabilitation of upper extremity nerve injuries using surface EMG biofeedback: protocols for clinical application. Front. Neurosci. 12:906. doi: 10.3389/fnins.2018.00906
Sullivan, R., Dailey, T., Duncan, K., Abel, N., and Borlongan, C. V. (2016). Peripheral nerve injury: stem cell therapy and peripheral nerve transfer. Int. J. Mol. Sci. 17:22101. doi: 10.3390/ijms17122101
Sun, S., Cao, H., Han, M., Li, T. T., Zhao, Z. Q., and Zhang, Y. Q. (2008). Evidence for suppression of electroacupuncture on spinal glial activation and behavioral hypersensitivity in a rat model of monoarthritis. Brain Res. Bull. 75, 83–93. doi: 10.1016/j.brainresbull.2007.07.027
Sun, W., Zhang, L., and Li, R. (2017). Overexpression of miR-206 ameliorates chronic constriction injury-induced neuropathic pain in rats via the MEK/ERK pathway by targeting brain-derived neurotrophic factor. Neurosci. Lett. 646, 68–74. doi: 10.1016/j.neulet.2016.12.047
Suzuki, H., Hase, A., Miyata, Y., Arahata, K., and Akazawa, C. (1998). Prominent expression of glial cell line-derived neurotrophic factor in human skeletal muscle. J. Comp. Neurol. 402, 303–312. doi: 10.1002/(SICI)1096-9861(19981221)402:3<303::AID-CNE2>3.0.CO;2-I
Takeshige, C., Tsuchiya, M., Guo, S. Y., and Sato, T. (1991). Dopaminergic transmission in the hypothalamic arcuate nucleus to produce acupuncture analgesia in correlation with the pituitary gland. Brain Res. Bull. 26, 113–122. doi: 10.1016/0361-9230(91)90195-P
Tallon, C., and Farah, M. H. (2017). Beta secretase activity in peripheral nerve regeneration. Neural Regen. Res. 12, 1565–1574. doi: 10.4103/1673-5374.217319
Tang, Y. J., Wu, M. H., and Tai, C. J. (2016). Direct electrical stimulation on the injured ulnar nerve using acupuncture needles combined with rehabilitation accelerates nerve regeneration and functional recovery-a case report. Complement. Ther. Med. 24, 103–107. doi: 10.1016/j.ctim.2015.12.003
Tang, S., Zhu, J., Xu, Y., Xiang, A. P., Jiang, M. H., and Quan, D. (2013). The effects of gradients of nerve growth factor immobilized PCLA scaffolds on neurite outgrowth in vitro and peripheral nerve regeneration in rats. Biomaterials 34, 7086–7096. doi: 10.1016/j.biomaterials.2013.05.080
Taylor, K. S., Anastakis, D. J., and Davis, K. D. (2009). Cutting your nerve changes your brain. Brain 132, 3122–3133. doi: 10.1093/brain/awp231
Thayer, J. F., Ahs, F., Fredrikson, M., Sollers, J. J. 3rd, and Wager, T. D. (2012). A meta-analysis of heart rate variability and neuroimaging studies: implications for heart rate variability as a marker of stress and health. Neurosci. Biobehav. Rev. 36, 747–756. doi: 10.1016/j.neubiorev.2011.11.009
Tian, X. Y., Bian, Z. X., Hu, X. G., Zhang, X. J., Liu, L., and Zhang, H. (2006). Electro-acupuncture attenuates stress-induced defecation in rats with chronic visceral hypersensitivity via serotonergic pathway. Brain Res. 1088, 101–108. doi: 10.1016/j.brainres.2006.03.014
Treiber, T., Treiber, N., and Meister, G. (2019). Regulation of microRNA biogenesis and its crosstalk with other cellular pathways. Nat. Rev. Mol. Cell Biol. 20, 5–20. doi: 10.1038/s41580-018-0059-1
Urban, M. O., Zahn, P. K., and Gebhart, G. F. (1999). Descending facilitatory influences from the rostral medial medulla mediate secondary, but not primary hyperalgesia in the rat. Neuroscience 90, 349–352. doi: 10.1016/S0306-4522(99)00002-0
Vadivelu, N., Kai, A., Maslin, B., Kodumudi, G., Legler, A., and Berger, J. M. (2015). Tapentadol extended release in the management of peripheral diabetic neuropathic pain. Ther. Clin. Risk Manag. 11, 95–105. doi: 10.2147/TCRM.S32193
Van Bockstaele, E. J., Aston-Jones, G., Pieribone, V. A., Ennis, M., and Shipley, M. T. (1991). Subregions of the periaqueductal gray topographically innervate the rostral ventral medulla in the rat. J. Comp. Neurol. 309, 305–327. doi: 10.1002/cne.903090303
Van Griensven, H., Schmid, A., Trendafilova, T., and Low, M. (2020). Central sensitization in musculoskeletal pain: lost in translation? J. Orthop. Sports Phys. Ther. 50, 592–596. doi: 10.2519/jospt.2020.0610
Wang, X., Chan, S. T., Fang, J., Nixon, E. E., Liu, J., Kwong, K. K., et al. (2013). Neural encoding of acupuncture needling sensations: evidence from a FMRI study, Evidence-based complementary and alternative medicine. eCAM, 483105.
Wang, J. Y., Gao, Y. H., Qiao, L. N., Zhang, J. L., Duan-Mu, C. L., Yan, Y. X., et al. (2018). Repeated electroacupuncture treatment attenuated hyperalgesia through suppression of spinal glial activation in chronic neuropathic pain rats. BMC Complement Altern Med, 18:74.
Wang, X., Ju, S., Chen, S., Gao, W., Ding, J., Wang, G., et al. (2017). Effect of electro-acupuncture on neuroplasticity of spinal cord-transected rats. Med. Sci. Monit. 23, 4241–4251. doi: 10.12659/MSM.903056
Wang, G., and Thompson, S. M. (2008). Maladaptive homeostatic plasticity in a rodent model of central pain syndrome: thalamic hyperexcitability after spinothalamic tract lesions. J. Neurosci. 28, 11959–11969. doi: 10.1523/JNEUROSCI.3296-08.2008
Wang, X., Wang, Z., Liu, J., Chen, J., Liu, X., Nie, G., et al. (2016). Repeated acupuncture treatments modulate amygdala resting state functional connectivity of depressive patients. Neuroimage Clin. 12, 746–752. doi: 10.1016/j.nicl.2016.07.011
Wang, C., Wang, H., Pang, J., Li, L., Zhang, S., Song, G., et al. (2014). Glial cell-derived neurotrophic factor attenuates neuropathic pain in a mouse model of chronic constriction injury: possible involvement of E-cadherin/p120ctn signaling. J. Mol. Neurosci. 54, 156–163. doi: 10.1007/s12031-014-0266-y
Wang, K., Zhang, R., He, F., Lin, L. B., Xiang, X. H., Ping, X. J., et al. (2012). Electroacupuncture frequency-related transcriptional response in rat arcuate nucleus revealed region-distinctive changes in response to low- and high-frequency electroacupuncture. J. Neurosci. Res. 90, 1464–1473. doi: 10.1002/jnr.23028
Wanke, K. A., Devanna, P., and Vernes, S. C. (2018). Understanding neurodevelopmental disorders: the promise of regulatory variation in the 3'UTRome. Biol. Psychiatry 83, 548–557. doi: 10.1016/j.biopsych.2017.11.006
Wasner, G., Lee, B. B., Engel, S., and McLachlan, E. (2008). Residual spinothalamic tract pathways predict development of central pain after spinal cord injury. Brain 131, 2387–2400. doi: 10.1093/brain/awn169
Wenjin, W., Wenchao, L., Hao, Z., Feng, L., Yan, W., Wodong, S., et al. (2011). Electrical stimulation promotes BDNF expression in spinal cord neurons through ca(2+)- and Erk-dependent signaling pathways. Cell. Mol. Neurobiol. 31, 459–467. doi: 10.1007/s10571-010-9639-0
Willand, M. P., Rosa, E., Michalski, B., Zhang, J. J., Gordon, T., Fahnestock, M., et al. (2016). Electrical muscle stimulation elevates intramuscular BDNF and GDNF mRNA following peripheral nerve injury and repair in rats. Neuroscience 334, 93–104. doi: 10.1016/j.neuroscience.2016.07.040
Wu, Q., Chen, J., Yue, J., Ying, X., Zhou, Y., Chen, X., et al. (2021a). Electroacupuncture improves neuronal plasticity through the A2AR/cAMP/PKA signaling pathway in SNL rats. Neurochem. Int. 145:104983. doi: 10.1016/j.neuint.2021.104983
Wu, J. J., Lu, Y. C., Hua, X. Y., Ma, S. J., Shan, C. L., and Xu, J. G. (2018a). Cortical remodeling after electroacupuncture therapy in peripheral nerve repairing model. Brain Res. 1690, 61–73. doi: 10.1016/j.brainres.2018.04.009
Wu, J. J., Lu, Y. C., Hua, X. Y., Ma, S. J., and Xu, J. G. (2018b). A longitudinal mapping study on cortical plasticity of peripheral nerve injury treated by direct anastomosis and Electroacupuncture in rats. World Neurosurg. 114, e267–e282. doi: 10.1016/j.wneu.2018.02.173
Wu, Q., Yue, J., Lin, L., Yu, X., Zhou, Y., Ying, X., et al. (2021b). Electroacupuncture may alleviate neuropathic pain via suppressing P2X7R expression. Mol. Pain 17:7654. doi: 10.1177/1744806921997654
Xiao, L. Y., Wang, X. R., Yang, Y., Yang, J. W., Cao, Y., Ma, S. M., et al. (2018). Applications of acupuncture therapy in modulating plasticity of central nervous system. Neuromodulation 21, 762–776. doi: 10.1111/ner.12724
Yan, B., Li, K., Xu, J., Wang, W., Li, K., Liu, H., et al. (2005). Acupoint-specific fMRI patterns in human brain. Neurosci. Lett. 383, 236–240. doi: 10.1016/j.neulet.2005.04.021
Yang, Y., Eisner, I., Chen, S., Wang, S., Zhang, F., and Wang, L. (2017). Neuroplasticity changes on human motor cortex induced by acupuncture therapy: a preliminary study. Neural Plast. 2017:4716792. doi: 10.1155/2017/4716792
Yang, C., Liu, J., Wang, J., Yin, A., Jiang, Z., Ye, S., et al. (2021). Activation of astroglial CB1R mediates cerebral ischemic tolerance induced by electroacupuncture. J. Cereb. Blood Flow Metab. 41, 2295–2310. doi: 10.1177/0271678X21994395
Yang, C. P., Wang, N. H., Li, T. C., Hsieh, C. L., Chang, H. H., Hwang, K. L., et al. (2011). A randomized clinical trial of acupuncture versus oral steroids for carpal tunnel syndrome: a long-term follow-up. J. Pain 12, 272–279. doi: 10.1016/j.jpain.2010.09.001
Yao, M., Fang, J., Li, J., Ng, A. C. K., Liu, J., Leung, G. K. K., et al. (2022). Modulation of the proteoglycan receptor PTPσ promotes white matter integrity and functional recovery after intracerebral hemorrhage stroke in mice. J. Neuroinflammation 19:207. doi: 10.1186/s12974-022-02561-4
Yi, S., Yuan, Y., Chen, Q., Wang, X., Gong, L., Liu, J., et al. (2016). Regulation of Schwann cell proliferation and migration by miR-1 targeting brain-derived neurotrophic factor after peripheral nerve injury. Sci. Rep. 6:29121. doi: 10.1038/srep29121
Yoon, Y. S., Lee, J. S., and Lee, H. S. (2013). Retrograde study of CART- or NPY-neuronal projection from the hypothalamic arcuate nucleus to the dorsal raphe and/or the locus coeruleus in the rat. Brain Res. 1519, 40–52. doi: 10.1016/j.brainres.2013.04.039
Yu, H., Liu, J., Ma, J., and Xiang, L. (2014). Local delivery of controlled released nerve growth factor promotes sciatic nerve regeneration after crush injury. Neurosci. Lett. 566, 177–181. doi: 10.1016/j.neulet.2014.02.065
Yu, S., Ortiz, A., Gollub, R. L., Wilson, G., Gerber, J., Park, J., et al. (2020). Acupuncture treatment modulates the connectivity of key regions of the descending pain modulation and reward Systems in Patients with chronic Low Back pain. J. Clin. Med. 9:1719. doi: 10.3390/jcm9061719
Yu, A. P., Shen, Y. J., Qiu, Y. Q., Li, J., Shen, Y. D., Wang, X. M., et al. (2019). Comparative effects of implanted electrodes with differing contact patterns on peripheral nerve regeneration and functional recovery. Neurosci. Res. 145, 22–29. doi: 10.1016/j.neures.2018.08.007
Yu, J., Wang, M., Liu, J., Zhang, X., and Yang, S. (2017). Effect of electroacupuncture on the expression of agrin and acetylcholine receptor subtypes in rats with tibialis anterior muscular atrophy induced by sciatic nerve injection injury. Acupunct. Med. 35, 268–275. doi: 10.1136/acupmed-2015-011005
Yu, B., Zhou, S., Yi, S., and Gu, X. (2015). The regulatory roles of non-coding RNAs in nerve injury and regeneration. Prog. Neurobiol. 134, 122–139. doi: 10.1016/j.pneurobio.2015.09.006
Zhang, C. H., Ma, Z. Z., Huo, B. B., Lu, Y. C., Wu, J. J., Hua, X. Y., et al. (2019). Diffusional plasticity induced by electroacupuncture intervention in rat model of peripheral nerve injury. J. Clin. Neurosci. 69, 250–256. doi: 10.1016/j.jocn.2019.08.088
Zhang, S., Tang, H., Zhou, J., and Gu, Y. (2014). Electroacupuncture attenuates neuropathic pain after brachial plexus injury. Neural Regen. Res. 9, 1365–1370. doi: 10.4103/1673-5374.137589
Zhang, M., Zhang, Y., Bian, Y., Fu, H., Xu, Y., and Guo, Y. (2018). Effect of long-term electroacupuncture stimulation on recovery of sensorimotor function after peripheral nerve anastomosis. Acupunct. Med. 36, 170–175. doi: 10.1136/acupmed-2017-011367
Zhang, J., Zheng, Y., Wang, Y., Qu, S., Zhang, S., Wu, C., et al. (2016). Evidence of a synergistic effect of Acupoint combination: a resting-state functional magnetic resonance imaging study. J. Altern. Complement. Med. 22, 800–809. doi: 10.1089/acm.2016.0016
Zhao, Z. Q. (2008). Neural mechanism underlying acupuncture analgesia. Prog. Neurobiol. 85, 355–375. doi: 10.1016/j.pneurobio.2008.05.004
Zhao, X., Bai, F., Zhang, E., Zhou, D., Jiang, T., Zhou, H., et al. (2018). Electroacupuncture improves neurobehavioral function through targeting of SOX2-mediated axonal regeneration by MicroRNA-132 after ischemic stroke. Front. Mol. Neurosci. 11:471. doi: 10.3389/fnmol.2018.00471
Zhao, F., He, W., Zhang, Y., Tian, D., Zhao, H., Yu, K., et al. (2013). Electric stimulation and decimeter wave therapy improve the recovery of injured sciatic nerves. Neural Regen. Res. 8, 1974–1984. doi: 10.3969/j.issn.1673-5374.2013.21.006
Zhao, L., Yuan, Y., Li, P., Pan, J., Qin, J., Liu, Y., et al. (2018). miR-221-3p inhibits Schwann cell myelination. Neuroscience 379, 239–245. doi: 10.1016/j.neuroscience.2018.03.019
Zhao, N., Zhang, J., Qiu, M., Wang, C., Xiang, Y., Wang, H., et al. (2018). Scalp acupuncture plus low-frequency rTMS promotes repair of brain white matter tracts in stroke patients: a DTI study. J. Integr. Neurosci. 17, 61–69. doi: 10.31083/JIN-170043
Zheng, Y., Pan, L., He, J., Yan, J., Xia, Y., Lin, C., et al. (2023). Electroacupuncture-modulated extracellular ATP levels in prefrontal cortex ameliorated depressive-like behavior of maternal separation rats. Behav. Brain Res. 452:114548. doi: 10.1016/j.bbr.2023.114548
Zhi, M. J., Liu, K., Zheng, Z. L., He, X., Li, T., Sun, G., et al. (2017). Application of the chronic constriction injury of the partial sciatic nerve model to assess acupuncture analgesia. J. Pain Res. 10, 2271–2280. doi: 10.2147/JPR.S139324
Zhou, N., Hao, S., Huang, Z., Wang, W., Yan, P., Zhou, W., et al. (2018). MiR-7 inhibited peripheral nerve injury repair by affecting neural stem cells migration and proliferation through cdc42. Mol. Pain 14:1744806918766793. doi: 10.1177/1744806918766793
Zhu, H., Xue, C., Yao, M., Wang, H., Zhang, P., Qian, T., et al. (2018). miR-129 controls axonal regeneration via regulating insulin-like growth factor-1 in peripheral nerve injury. Cell Death Dis. 9:720. doi: 10.1038/s41419-018-0760-1
Zitnik, G. A., Clark, B. D., and Waterhouse, B. D. (2014). Effects of intracerebroventricular corticotropin releasing factor on sensory-evoked responses in the rat visual thalamus. Brain Res. 1561, 35–47. doi: 10.1016/j.brainres.2014.02.048
Zou, Y., Tang, W., Li, X., Xu, M., and Li, J. (2019). Acupuncture reversible effects on altered default mode network of chronic migraine accompanied with clinical symptom relief. Neural Plast. 2019:5047463. doi: 10.1155/2019/5047463
Zubieta, J. K., Smith, Y. R., Bueller, J. A., Xu, Y., Kilbourn, M. R., Jewett, D. M., et al. (2001). Regional mu opioid receptor regulation of sensory and affective dimensions of pain. Science 293, 311–315. doi: 10.1126/science.1060952
Zuo, X. N., Di Martino, A., Kelly, C., Shehzad, Z. E., Gee, D. G., Klein, D. F., et al. (2010). The oscillating brain: complex and reliable. NeuroImage 49, 1432–1445. doi: 10.1016/j.neuroimage.2009.09.037
Keywords: acupuncture, peripheral nerve injury, mechanism, nerve system remodeling, nerve repair
Citation: Yang Y, Rao C, Yin T, Wang S, Shi H, Yan X, Zhang L, Meng X, Gu W, Du Y and Hong F (2023) Application and underlying mechanism of acupuncture for the nerve repair after peripheral nerve injury: remodeling of nerve system. Front. Cell. Neurosci. 17:1253438. doi: 10.3389/fncel.2023.1253438
Edited by:
Jian Shen, Zhejiang University, ChinaReviewed by:
Susu Mao, Nantong University, ChinaLuis R. Hernandez-Miranda, Charité University Medicine Berlin, Germany
Copyright © 2023 Yang, Rao, Yin, Wang, Shi, Yan, Zhang, Meng, Gu, Du and Hong. This is an open-access article distributed under the terms of the Creative Commons Attribution License (CC BY). The use, distribution or reproduction in other forums is permitted, provided the original author(s) and the copyright owner(s) are credited and that the original publication in this journal is cited, in accordance with accepted academic practice. No use, distribution or reproduction is permitted which does not comply with these terms.
*Correspondence: Feng Hong, 18322110670@163.com