- 1Department of Agricultural Insects and Pest Control, China Agricultural University, Beijing, China
- 2Department of Zoology, University of Cambridge, Cambridge, United Kingdom
- 3Plant Protection Institute, Shandong Provincial Engineering Technology Research Center on Biocontrol for Pests, Jinan, China
Introduction: Serotonin (5-hydroxytryptamine; 5-HT) and GABA (γ-aminobutyric acid) are involved in the regulation of behaviors in the central nervous system. However, it remains unclear whether they modulate olfaction in the peripheral nervous system, and how they modulate olfaction.
Methods and results: One 5-HT receptor sequence (Lmig5-HT2) and one GABA receptor sequence (LmigGABAb) were identified in locust antennae by transcriptome analysis and polymerase chain reaction experiments. In situ hybridization localized Lmig5-HT2 to accessory cells, while LmigGABAb was localized to olfactory receptor neurons (ORNs) in locust chemosensilla. Single-unit electrophysiological recordings combined with RNA interference (RNAi) experiments indicated ORNs of locusts with knockdown of Lmig5-HT2 (ds-Lmig5-HT2) and LmigGABAb (ds-LmigGABAb) to some odors had significantly higher responses than wild-type and control locusts in the dose-dependent responses. Moreover, the gaps between the responses of ORNs of RNAi ones and those of wild-type and ds-GFP enlarged with an increase in concentrations of odors.
Discussion: Taken together, our findings suggest that 5-HT, GABA, and their receptors exist in the insect peripheral nervous system and that they may function as negative feedback to ORNs and contribute to a fine-tuning mechanism for olfaction in the peripheral nervous system.
1. Introduction
Olfactory information processing involves a cascade of neural events that initiate at the level of peripheral olfactory organs and propagate through ascending pathways to the central nervous system (CNS). The information processed in olfactory streams is regulated by descending control systems, such as neuromodulatory systems; this process has been studied in the CNS in both vertebrates (McLean et al., 1993; Dugué and Mainen, 2009; Fletcher and Chen, 2010; Liu et al., 2012; Kapoor et al., 2016) and invertebrates, including insects (Gatellier et al., 2004; Dacks et al., 2008, 2009; Kloppenburg and Mercer, 2008). However, the role of neuromodulation in the processing of odor responses at the peripheral level remains poorly understood.
Antennae, the peripheral olfactory organs of insects, mainly function to acquire odor cues. In the peripheral olfactory process, odor molecules enter the lumen of hair-like organs called chemosensilla on insect antennae, where the molecules are bound and transported by odorant-binding proteins (OBPs). The odorants or odorant/OBP complexes, then, arrive at the dendrites of olfactory receptor neurons (ORNs), are bound at the membrane by odorant receptors (ORs) in chemosensilla, and evoke a membrane potential change, sending signals to the CNS (Hildebrand and Shepherd, 1997; Clyne et al., 1999; Dobritsa et al., 2003; Hallem and Carlson, 2006; Wang and Anderson, 2010; Leal, 2013).
Odorant receptors (ORs) either form ion channels directly with a co-receptor or couple with other proteins to perform metabotropic signal transduction (Sato et al., 2008; Wicher et al., 2008). In addition, OBPs are secreted by accessory cells surrounding ORNs and are commonly suggested to solubilize and transport non-soluble exogenous organic compounds to ORs (Pelosi et al., 2006; Laughlin et al., 2008). Investigating neuromodulation in antennae is crucial for understanding its role in the peripheral nervous system.
Serotonin (also known as 5-hydroxytryptamine; 5-HT) is a ubiquitous neuromodulator that is found throughout phylogeny and alters olfactory function in the CNS (Dierick and Greenspan, 2007; Ganesh et al., 2010; Johnson et al., 2011; Albin et al., 2015). It can directly suppress projection neuron responses to odors (Kloppenburg and Hildebrand, 1995; Petzold et al., 2009; Zhang and Gaudry, 2016; Gaudry, 2018). Moreover, 5-HT enhances the response of inhibitory local interneurons, GABAergic neurons, which indirectly results in reduced neurotransmitter release from ORN terminals via GABAb receptor-dependent presynaptic inhibition (Dacks et al., 2009; Dugué and Mainen, 2009; Petzold et al., 2009). Nevertheless, very few studies have focused on the role of 5-HT at the peripheral level (Dolzer et al., 2001). 5-HT is found in the hemolymph of insects (Lange et al., 1989; Siju et al., 2008; Zhukovskaya and Polyanovsky, 2017). In addition, 5-HT-immunoreactive fibers and putative 5-HT receptor genes have been identified in the antennae of mosquitoes (Siju et al., 2008; Pitts et al., 2011). These findings suggest the possibility that serotonergic modulation occurs in antennae.
To determine if there is a feedback mechanism in the olfactory peripheral nervous system of insects, immunohistochemistry was used to determine the localization of 5-HT and GABA in locust antennae. We, then, identified 5-HT and GABA receptors in locust antennae and characterized their expression patterns with dual-color fluorescence in situ hybridization experiments. We used a combination of single-unit electrophysiology and RNA interference (RNAi) techniques to reveal the functions of 5-HT and GABA receptors in ORNs. Our results suggest that, in the peripheral nervous system, 5-HT/GABA and their receptors may independently function to some extent as negative feedback in the response of ORNs to high concentrations of odors.
2. Materials and methods
2.1. Animals
Locusta migratoria (Orthoptera) was raised under crowded conditions in the Department of Entomology, China Agricultural University, with a relative humidity of 60% and a temperature of 28–30°C, under a photoperiod of 18:6 h (light:dark). Locusts were fed fresh wheat seedlings daily. Intact antennae were dissected using forceps and stored at −80°C until mRNA extraction, immunohistochemistry, and in situ hybridization.
2.2. Identification of 5-HT-/GABA-related genes in antennae
We used a collection of insect 5-HT-/GABA-related genes (Supplementary Tables S1–S3) to perform tblastx queries with a cutoff of 10−5 against our database using the L. migratoria antenna transcriptome. Identified hits, indicating candidate-related genes, were used to re-tblast against the National Center for Biotechnology Information database to verify gene identities. The phylogenetic analysis of 5-HT/GABA receptors was performed with multiple aligned sequences using the maximum likelihood distance method, with a bootstrap value of 1,000, in MEGA6 for Windows.
2.3. In situ hybridization
Templates of LmigOBP1, LmigORco, Lmig5-HT2, LmigGABAb, LmigTPH, and LmigAADC were generated by standard polymerase chain reaction (PCR) using gene-specific primer pairs (Supplementary Table S4). Digoxigenin (DIG)- or biotin (BIO)-labeled antisense probes were generated from linearized recombinant pGem-T Easy plasmids using the T7/SP6 RNA transcription system (Roche, Basel, Switzerland), following the recommended protocols. RNA in situ hybridization was performed according to previously reported procedures (Xu et al., 2017). In brief, antennae were dissected and embedded in a freezing medium (Tissue-Tek O.C.T. Compound; Sakura Finetek Europe). Sections (12 μm) were prepared in the same way as for immunohistochemistry. After a series of fixing and washing procedures, 100 μL of hybridization solution (Boster) containing RNA probes was applied to the tissue sections. After adding coverslips, slides were incubated in a humid box at 55°C overnight. After hybridization, slides were washed two times for 30 min in 0.1× saline-sodium citrate at 60°C, treated with 1% blocking reagent (Roche) in PBST for 30 min at room temperature, and then incubated for 30 min with an anti-DIG alkaline phosphatase-conjugated antibody (Roche). DIG-labeled probes were visualized using the anti-DIG alkaline phosphatase-conjugated antibody in combination with HNPP/Fast Red (Roche). For BIO-labeled probes, the TSA Kit (PerkinElmer, Waltham, MA, United States), including a streptavidin–horseradish peroxidase conjugate and fluorescein tyramide as a substrate, was used. Images were captured on an Olympus BX45 confocal microscope and analyzed using FV1000 software (Olympus). Images were not altered except for the uniform adjustment of brightness or contrast within a single figure.
2.4. RNAi
Double-stranded RNA (ds-RNA) was synthesized following the manufacturer's instructions. In brief, PCR products (for primer pairs see Supplementary Table S4) were amplified with T7 promoter conjugated primer and then purified using the Wizard® SV Gel and PCR Clean-Up System (Promega, Madison, WI, United States) as templates for in vitro transcription. ds-RNA was synthesized with T7 RiboMAXTM Express RNAi System (Promega), diluted to 1,000 ng/μL with ddH2O, and stored at −20°C. Target ds-RNA (10 μg) was delivered into each locust dorsal vessel through the inter-segmental membrane of nymphs on the first day of the fifth instar, using an IM-9B microinjector (Narishige, Tokyo, Japan) equipped with a glass capillary. ds-green fluorescent protein (GFP) was microinjected as a control group. The treated locusts were raised normally like wild-type insects. RNA silencing efficiency was checked on post-injection day 3. The number of biological replicates was at least 4, and the sample number in each replicate was at least 2. All RNAi-treated insects that were used for single sensillum recordings (SSRs) were also checked after recording to confirm the silencing efficiency.
2.5. Semi-quantitative real-time (RT)-PCR
Semi-quantitative RT-PCR was used to check the expression of target genes and investigate the silencing efficiency of gene-specific RNAi. The biological replicate was 3, and there were at least two samples in each replicate. In brief, 1 μg total RNA from various RNAi treatments and control tissues were transcribed into cDNA. For each independent PCR, gene-specific primer pairs were designed (see Supplementary Table S4), and the equivalent amount of cDNA was used as a template for amplification (TaKaRa Ex Taq, TaKaRa, Shiga, Japan). The following PCR cycling parameters were used: initial denaturation at 94°C for 5 min; 35 cycles of 94°C, 30 s; 50–60°C, 30 s (different genes had different annealing temperatures, see Supplementary Table S3); and 72°C, 1 min; followed by a final extension at 72°C for 10 min.
2.6. qPCR
The template of real-time quantitative PCR (QPCR) was a transcript from the same RNA samples of transcriptome sequencing using M-MLV reverse transcriptase (Promega, United States), according to the manufacturer's instructions. We designed the QPCR primers with 100–250 bp product primers from the unigene sequences. The primers were checked with normal PCR (TaKaRa, Dalian, Liaoning, China) and sequencing to verify product correction and no primer dimers. The 2−ΔΔCT method was used to qualify the relative expression levels of each gene. The expression level of genes was normalized by reference genes Rp49. The 20 μl reaction volume (including 10 μl SuperReal PreMix, Tiangen, Beijing, China) for qPCR was performed on an ABI QuantStudio 6 Flex (United States), with the following PCR program: 15 min at 95 °C, 40 cycles of 10 s at 95 °C, 30 s at 60 °C, and at last 60 °C to 95 °C to perform melting curve analysis and evaluate the specificity of the real-time PCR products. Each sample was performed with three technical replicates.
2.7. Single-unit electrophysiological experiments
Our in situ hybridization experiments showed that ORNs and accessory cells of sensilla on the distal sixth segment of antennae expressed LmigORco, LmigOBP1, Lmig5-HT2, and LmigGABAb. Moreover, scanning electron microscopy indicated that this sensillum was a basiconic sensillum; we, therefore, named it Ba6. Ba6 was used for the following SSR experiments. SSRs and chemical stimulations were performed on the antennae of fifth instar nymphs. Each locust or isolated antenna was mounted with tape and plasticine on a glass slide, and one of its antennae was immobilized with tape and plasticine on a piece of coverslip. Tungsten electrodes were sharpened electronically with 10% NaNO2 under a microscope. The recording electrode was inserted into the base of the basiconic sensillum using a motorized micromanipulator (CFT-8301D, C.M.D.T., China), and a ground electrode was inserted into the head. The stimulation duration was 1 s. The recording electrode was connected to a 10× universal AC/DC amplifier (Syntech, Venlo, the Netherlands). The recording signals were collected on an Intelligent Data Acquisition Controller (IDAC-4, Syntech) and were viewed on a personal computer. AUTOSPIKE (Syntech) software was used to quantify the neuronal responses recorded for 1 s before and after each stimulation, and the duration between the maximum point of frequency after stimulation to a 50% reduction of the response.
2.8. Chemicals and preparation
We chose benzaldehyde, phenylacetonitrile, and guaiacol to investigate during the SSR experiments. All three of these chemicals have been reported in the body odors of gregarious nymphs in migratory locusts, and they have been suggested to have important roles in the density-dependent phase change in locusts (Wei et al., 2017). Chemicals with the highest purity were used for SSRs; these are presented in Supplementary Table S5. The working solutions were prepared using mineral oil as the solvent.
2.9. Statistical analyses
Data from extracellular recordings were analyzed using the one-way analysis of variance (ANOVA) and Tukey's honest significant difference (HSD) post-hoc test in GraphPad Prism 7 (GraphPad Software, San Diego, CA, United States).
3. Results
3.1. 5-HT and its receptors exist under the olfactory sensilla of antenna
The genes encoding the putative key enzymes involved in the 5-HT synthesis, tryptophan hydroxylase (LmigTPH), and aromatic L-amino acid decarboxylase (LmigAADC, MN531682) were searched from the transcriptome database of locust antennae using BLAST. A phylogenetic tree was constructed (Supplementary Figure S1A), and PCR results revealed that the transcripts of these two genes existed in locust antennae (Supplementary Figure S1B). This finding indicates that 5-HT might be able to be biosynthesized in antennae. Furthermore, we synthesized LmigTPH or LmigAADC RNA antisense probes with DIG (red) to conduct dual-color fluorescence in situ hybridization experiments with LmigORco using a BIO-labeled probe (green). The OR co-expression receptor (ORco) is expressed in every ORN; thus, cells labeled by the LmigORco antisense probe can be taken to be ORNs. The antisense probes against LmigTPH or LmigAADC (red) and antisense probes against LmigORco (green) did not overlap fully, but they occurred in close proximity to sparse cell clusters (Figures 1A–F). Taken together, our results indicate that 5-HT exists around, but not in, ORNs in locust antennae.
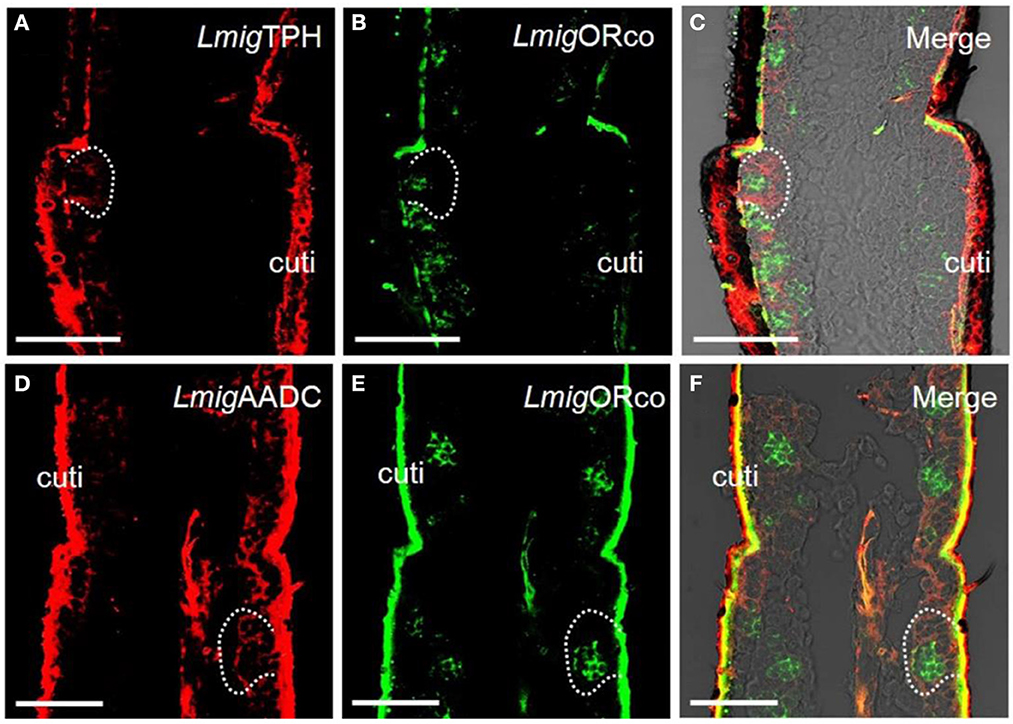
Figure 1. Localization of 5-HT using antiserum against 5-HT and localization of two key enzymes for 5-HT biosynthesis by dual-color fluorescence in situ hybridization in locust antennae. (A) The enzyme LmigTPH was stained by an antisense probe against LmigTPH RNA with digoxigenin (DIG), red. (B) Odorant receptor co-expressed protein (LmigORco) was stained by an antisense probe against LmigORco RNA with biotin (BIO), green. (C) A and B merged with the brightfield image. (D) The enzyme LmigAADC was stained by an antisense probe against LmigAADC RNA with DIG, red. (E) LmigORco was stained by an antisense probe against LmigORco RNA with BIO, green. (F) D and E merged with the brightfield image. Ba, basiconic sensilla; Tr, trichoid sensilla; Co, coeloconic sensilla; cuti, cuticle; tra, trachea. Scale bar: 100 μm.
Because 5-HT was observed in the antennae, we hypothesized that there may also be 5-HT receptors in the antennae to bind with the 5-HT. To examine whether there are 5-HT receptors in locust antennae, we first searched for the putative receptor genes in the transcriptome database of locust antennae using BLAST. One partial gene sequence was considered a putative 5-HT receptor gene. The phylogenetic tree indicated that the partial sequence belonged to one insect 5-HT receptor (Supplementary Figure S4A), namely, Lmig5-HT2 (MN531679). We, then, cloned the putative gene from locust antennae using PCR (Supplementary Figure S4B), thus indicating the existence of these three genes in locust antennae.
3.2. Accessory cells, but not ORNs, express 5-HT2 receptors in chemosensilla
The preliminary analysis of single-color fluorescent signals of locust 5-HT2 receptors (Lmig5-HT2) demonstrated that a subset of distinct antennal cells was present in each section (Figure 2A). Cell clusters labeled by the antisense probes were observed within all types of olfactory sensilla such as basiconic, trichoid, and coeloconic when checked in the same sections with a plain lens (Figure 2B). In negative controls (Figures 2C, D), autofluorescence in locust cuticle was observed as in many previous studies (Xu et al., 2013; Zhang et al., 2017).
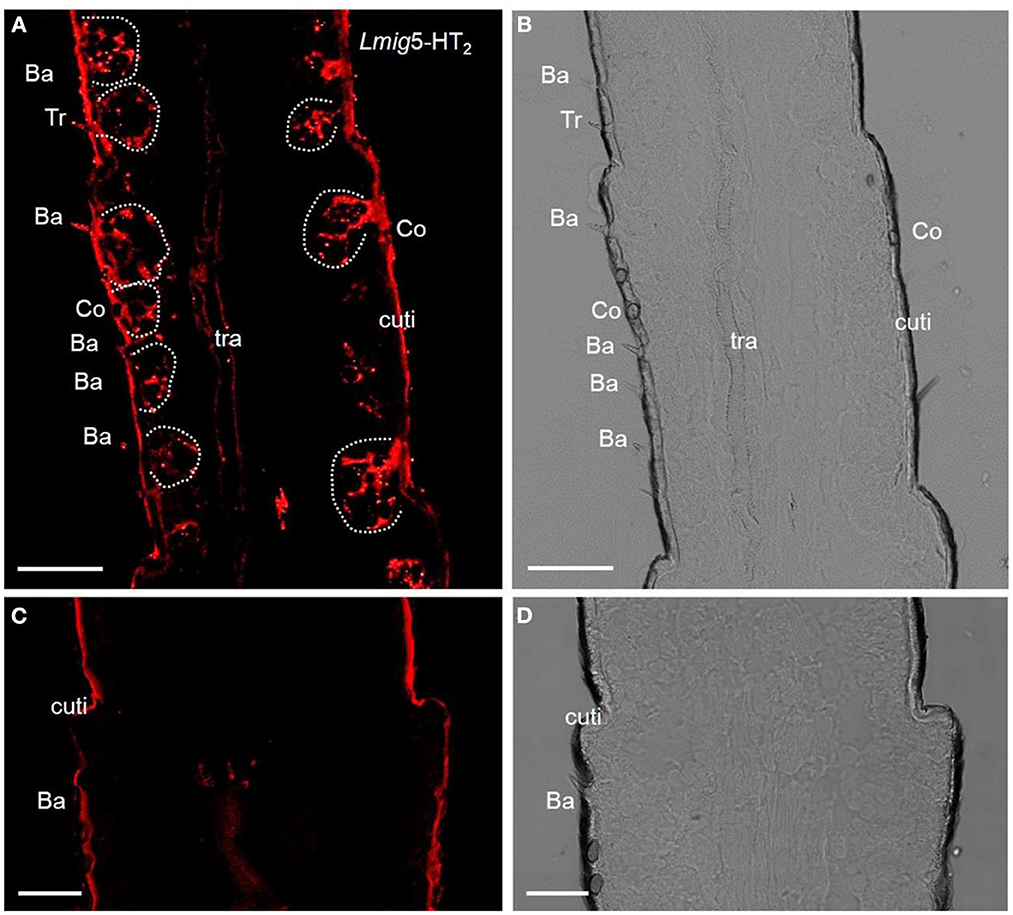
Figure 2. 5-HT receptors in locust antennae. (A) Single-color fluorescence in situ hybridization on the fifth to seventh segments with an antisense probe against Lmig5-HT2 RNA with DIG, red. (B) Blank control. Dotted circles indicate cell clusters. (C, D) Negative control without an antisense probe against Lmig5-HT2 RNA with DIG, red. Ba, basiconica sensilla; Tr, trichoid sensilla; cuti, cuticle. Scale bar: 100 μm.
Odorant-binding proteins (OBPs) and ORs in the olfactory sensilla are two protein families which are involved in peripheral odorant detection. OBPs are secreted by accessory cells, and LmigOBP1 is widely expressed in accessory cells of antennal olfactory sensilla (Jin et al., 2005), whereas Orco is expressed in apparently all ORNs expressing ORs but not ionotropic receptors (IRs) (Yang et al., 2011). Here, LmigOrco can be employed as a marker for ORNs and LmigOBP1 as an indicator of accessory cells. Next, we used LmigOBP and LmigOrco antisense probes to detect Lmig5-HT2 in accessory cells or ORNs. To distinguish the cell type(s) that expressed Lmig5-HT2, we carried out dual-color fluorescence in situ hybridization experiments, using the 5-HT2 RNA antisense probe with DIG (red color) and LmigOrco or LmigOBP1 with BIO (green color). The antisense probes against Lmig5-HT2 (Figure 3A, red color) and LmigOrco (Figure 3B, green color) did not overlap fully but were observed in close proximity to sparse cell clusters (Figure 3C). Conversely, Lmig5-HT2 (Figure 3D, red color) and LmigOBP1 (Figure 3E, green color) overlapped fully with one another (Figure 3F). In addition, the cell cluster circled by the dashed line was in a basiconic sensillum situated in the sixth segment, known as Ba6 (Supplementary Figure S3A, red square). Together, these observations indicate that Lmig5-HT2 is not co-expressed with LmigOrco in ORNs but it is co-expressed with LmigOBP1 in accessory cells neighboring ORNs.
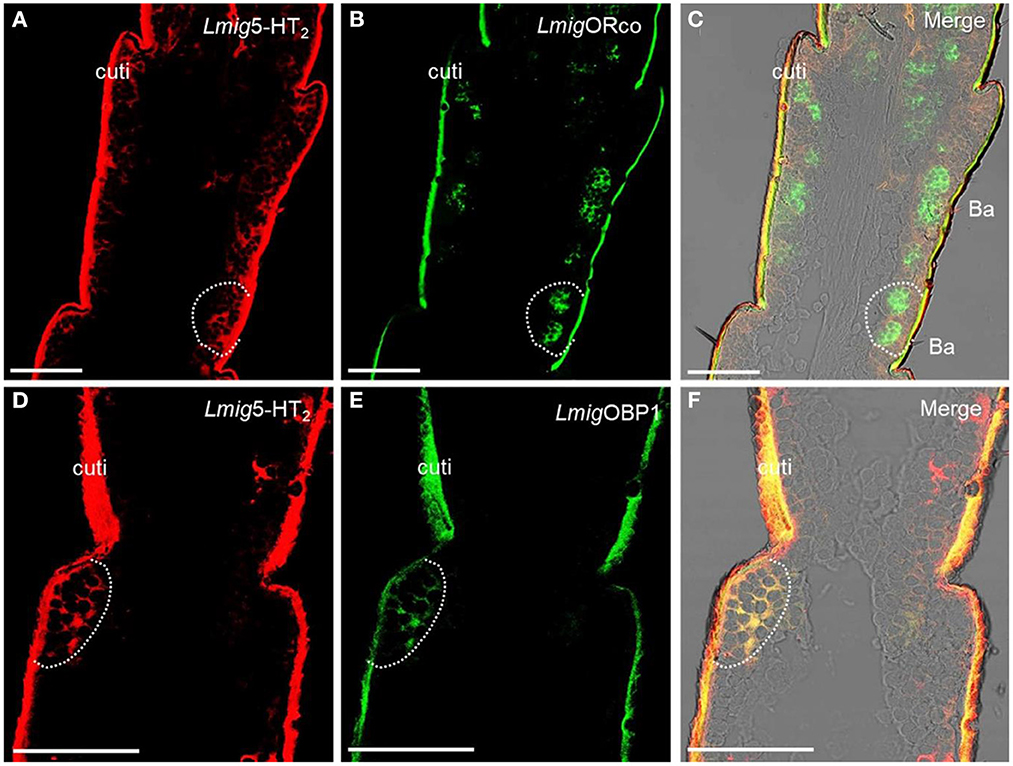
Figure 3. Localization of Lmig5-HT2 by dual-color fluorescence in situ hybridization experiments in locust antennae. (A) Antennal cryosection stained by an antisense probe against 5-HT2 RNA with DIG, red. (B) Antennal cryosection stained by an antisense probe against LmigOrco RNA with BIO, green. (C) A and B merged with the brightfield image. (D) Antennal cryosection stained by an antisense probe against 5-HT2 RNA with DIG, red. (E) Antennal cryosection stained by an antisense probe against LmigOBP1 RNA with BIO, green. (F) D and E merged WITH the brightfield image. Cuti, cuticle. Scale bars: 100 μm.
3.3. GABA and its receptor LmigGABAb exist in locust antennae
Serotonergic modulation in the CNS leads to inhibition via GABAb receptors (Dacks et al., 2009; Petzold et al., 2009). In the present study, we wanted to investigate whether GABA receptors exist in the peripheral nervous system. We analyzed the antennal transcriptome and identified a putative GABA receptor gene. The phylogenetic tree (Supplementary Figure S2A, Supplementary Table S2) indicated that this gene belonged to the clade of the GABAb receptor, named LmigGABAb (MN531681). Using PCR, we revealed that this gene existed in locust antennae (Supplementary Figure S2B).
Dual-color fluorescence in situ hybridization experiments demonstrated that the cells labeled by antisense probes against LmigGABAb with DIG (red color) were also labeled by antisense probes against LmigOrco with BIO (green color) (Figures 4A–C). The findings suggest that LmigGABAb is expressed in ORNs. Moreover, dual-color fluorescence in situ hybridization to explore the localization relationship between LmigGABAb and Lmig5-HT2 showed that LmigGABAb-expressing cells (green color) were surrounded by cells labeled by Lmig5-HT2 (red color) in sensilla basiconica in the antennae (Figures 4D–F). The cell cluster circled by the dashed line in Figure 4 is found in a basiconic sensillum named as Ba6, which is located closest to the end of the fifth segment of the antennae. This cell cluster can be marked jointly by Lmig5-HT2, LmigGABAb, and LmigOrco probes pairwise (Figures 3A–C, 4A–F). In addition, dual-color fluorescence in situ hybridization experiments had shown that Lmig5-HT2 and LmigOBP1 overlapped fully in the same sensillium' cell cluster (Figures 3D–F). In summary, these observations indicate that Lmig5-HT2, LmigGABAb, LmigOrco, and LmigOBP1 express in the same sensillum. Among them, Lmig5-HT2 and LmigOBP1 are expressed in the same cells (accessory cells), and LmigGABAb and LmigOrco are expressed in common ORNs.
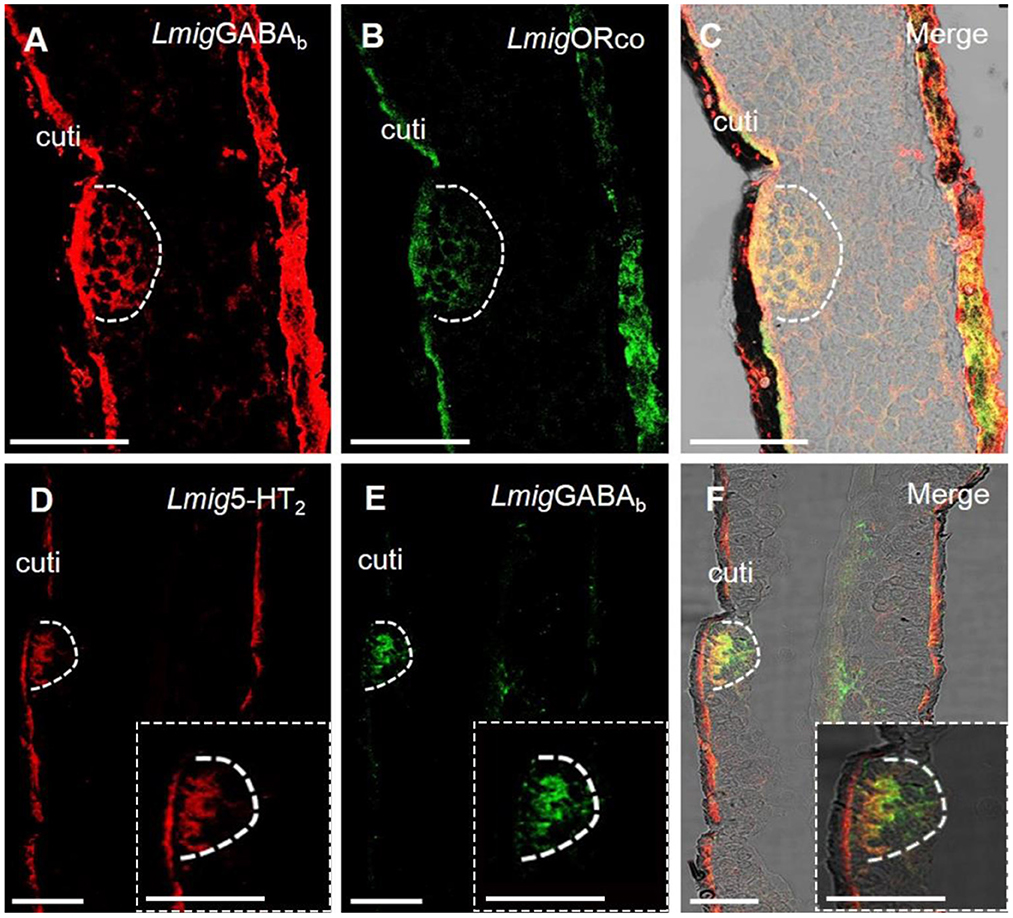
Figure 4. Localization of LmigGABAb by dual-color fluorescence in situ hybridization experiments in locust antennae. (A) Antennal cryosection stained by an antisense probe against LmigGABAb RNA with DIG, red. (B) Antennal cryosection stained by an antisense probe against LmigOrco RNA with BIO, green. (C) A and B merged with the brightfield image. (D) Antennal cryosection stained by an antisense probe against 5-HT2 RNA with DIG, red. (E) Antennal cryosection stained by an antisense probe against LmigGABAb RNA with BIO, green. (F) D and E merged with the brightfield image. Cuti, cuticle. Scale bars: 100 μm. The dashed box in the lower right corner of (D–F) shows the enlarged staining result.
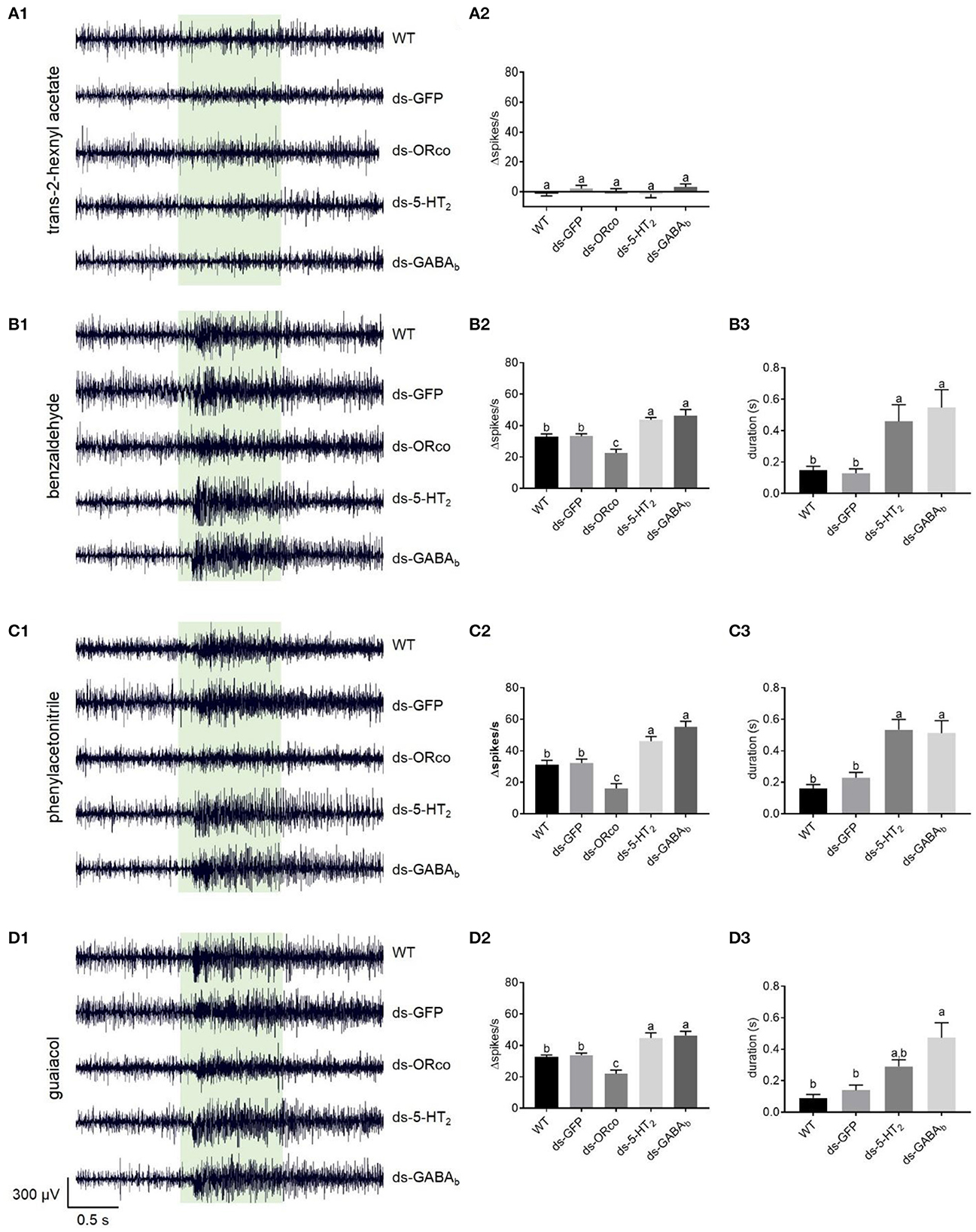
Figure 5. Responses to odorants at 1% (v/v) concentration of ORNs in the antennal basiconic sensilla of wild-type locusts and locusts microinjected with ds-GFP, ds-LmigOrco, ds-Lmig5-HT2, or ds-LmigGABAb. (A1–D1) Response traces of Ba6 neurons to trans-2-hexenyl acetate, benzaldehyde, phenylacetonitrile, and guaiacol. (A2–D2) Quantification of the mean changes of all spikes (Δspikes/s) in the 1 s before and after the stimulus of the Ba6 neurons to odorants in wild-type locusts and locusts microinjected with ds-GFP, ds-LmigOrco, ds-Lmig5-HT2, or ds-LmigGABAb. (B3–D3) Quantification of the duration from the maximum point of frequency after simulation to a 50% reduction of the response to odorants in the treated locusts. The green box indicates the stimulus duration (1 s). WT, wild-type locusts; ds-GFP, locusts injected with ds-GFP; ds-Orco, locusts injected with ds-LmigOrco; ds-5-HT2, locusts injected with ds-Lmig5-HT2; ds-GABAb, locusts injected with ds-LmigGABAb. Values are the mean ± SEM, n = 5–8 per group. For variables with the same letter above the columns, the difference was not statistically significant. For variables with different letters, the difference was statistically significant (p < 0.05, one-way ANOVA with Tukey's post-hoc tests).
3.4. ORNs respond robustly to odorants by knockdown of Lmig5-HT2 or LmigGABAb in antennae
We concluded that Lmig5-HT2, LmigGABAb, LmigOrco, and LmigOBP1 express in the Ba6, which we then chose as the target sensillum to conduct a single-unit electrophysiological experiment (SSR), to elucidate the roles of Lmig5-HT2 and LmigGABAb in olfaction in antennae. We performed microinjections of ds-RNA of target genes to knock down the expression levels of Lmig5-HT2 or LmigGABAb, thus creating locusts with knockdown of Lmig5-HT2 and LmigGABAb genes, which we named ds-Lmig5-HT2 and ds-LmigGABAb, respectively. We used locusts that were injected with ds-RNA of GFP as the negative control and locusts injected with LmigOrco as the positive control. Compared with the antennae of wild-type locusts (wt), PCR experiments revealed specific and significant decreases in the levels of Lmig5-HT2 mRNA in ds-5-HT2 locusts, LmigGABAb mRNA in ds-GABAb locusts, or LmigOrco mRNA in ds-Orco locusts (Supplementary Figures S3B, S3C). Conversely, there were no changes in the expression levels of these three target genes in the ds-GFP locusts.
The responses of Ba6 neurons to odorants in the ds-Lmig5-HT2, ds-LmigGABAb, ds-LmigOrco, ds-GFP, and wild-type locusts were examined using SSR. The ORNs in Ba6 were not activated by trans-2-hexenyl acetate at a concentration of 1% in wild-type locusts (Supplementary Figure 5A1). Similarly, the targeted neurons in the ds-Lmig5-HT2, ds-LmigGABAb, ds-LmigOrco, and ds-GFP locusts showed no response to 1% trans-2-hexenyl acetate. As expected, the mean changes of all spikes (Δspikes/s) in the 1 s before and after stimulation were not significantly different among the different types of locusts (Supplementary Figure 5A2).
Conversely, benzaldehyde (Supplementary Figure 5B1), phenylacetonitrile (Supplementary Figure 5C1), and guaiacol (Supplementary Figure 5D1) at concentrations of 1% evoked distinct excitement in the Ba6 neurons of wild-type locusts. These three odorants are important body volatiles of gregarious nymphs in migratory locusts. The changed spike number in the Ba6 neurons of wild-type locusts was not significantly different from that of ds-GFP locusts in response to these three odorants at 1% concentration. However, it was significantly lower than those in the Ba6 neurons of ds-Lmig5-HT2 and ds-LmigGABAb locusts, which were not significantly different from one another. Additionally, the Δspikes/s of Ba6 neurons in ds-Lmig5-HT2, ds-LmigGABAb, ds-GFP, and wild-type locusts were significantly greater than those in the ds-LmigOrco locusts in response to these three odorants (Supplementary Figures 5B2–D2). Moreover, we defined the duration from the maximum point of frequency after stimulation to a 50% reduction of the response as the response duration of Ba6 neurons. The response durations of Ba6 neurons in wild-type and ds-GFP locusts were significantly shorter than those in ds-Lmig5-HT2 and ds-LmigGABAb locusts in response to benzaldehyde and phenylacetonitrile (Supplementary Figures 5B3–D3), although guaiacol evoked a significantly longer response of Ba6 neurons between the ds-GFP and ds-LmigGABAb locusts but not the ds-Lmig5-HT2 locusts. These findings suggest that the response duration of ORNs may be prolonged when LmigGABAb is knocked down.
To check if the effect originated inside the antenna, we used isolated antenna to repeat the SSR experiments combined with RNAi. We found that the changed spike number in the Ba6 neurons of ds-GFP locusts was significantly different from that of ds-GABAb locusts in response to benzaldehyde, phenylacetonitrile, and guaiacol at 1% concentration (Figure 6). In addition, there was no significant difference between ds-5-HT2 and ds-GABAb locusts in response to the chemical. Although the difference is not significant, there was also a trend that the response evoked by benzaldehyde in the Ba6 neurons of ds-5-HT2 locusts is higher than the ds-GFP locusts (p = 0.055). The above results suggest that 5-HT2 and GABAb receptors at least play partially the role of negative feedback to ORNs of antennae to odors independently.
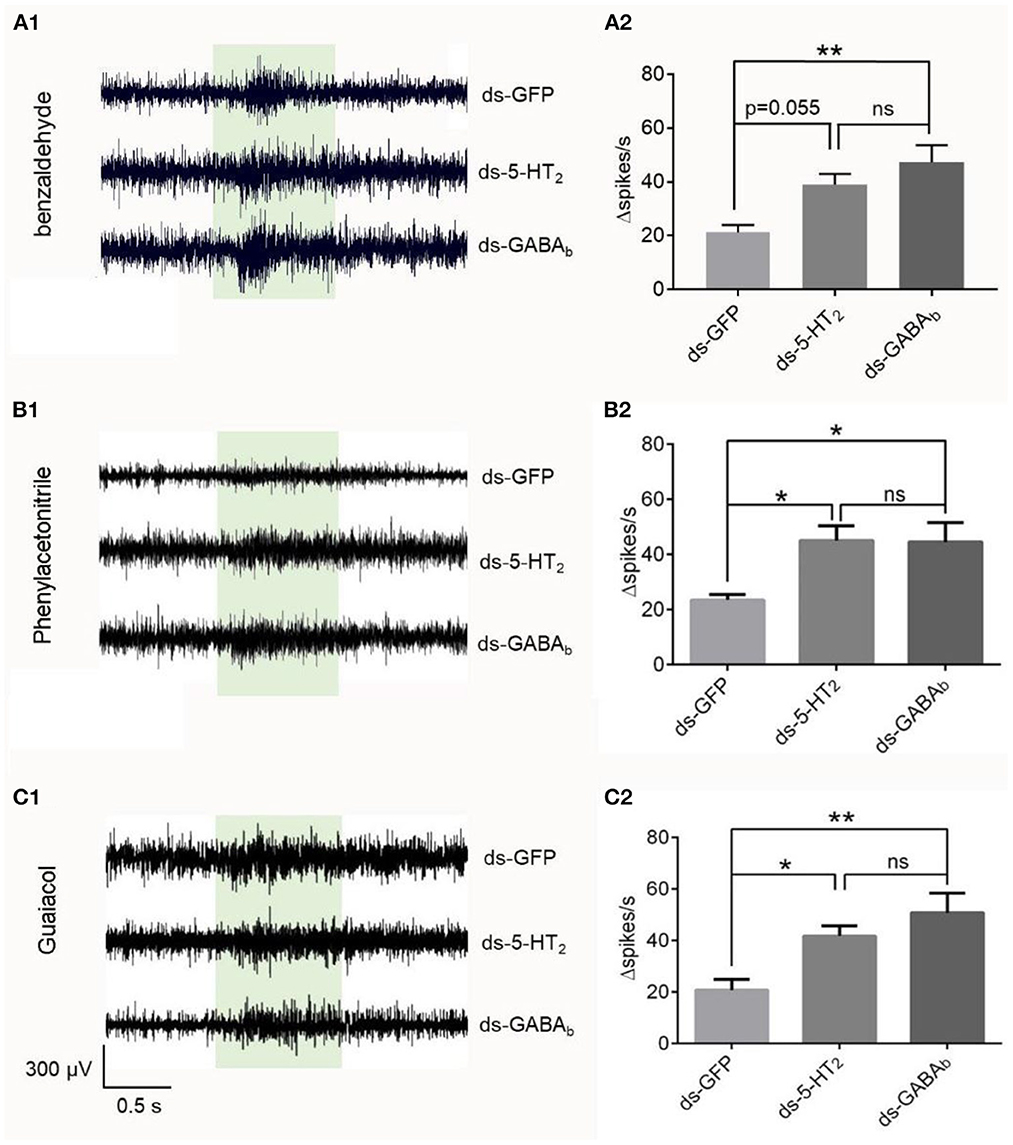
Figure 6. Responses to odorants at 1% (v/v) concentration of ORNs in isolated antennal basiconic sensilla of locusts microinjected with ds-GFP, ds-Lmig5-HT2, or ds-LmigGABAb. (A1–C1) Response traces of Ba6 neurons to benzaldehyde, phenylacetonitrile, and guaiacol. (A2–C2) Quantification of the mean changes of all spikes (Δspikes/s) in the 1 s before and after the stimulus of the Ba6 neurons to odorants in locusts microinjected with ds-GFP, ds-Lmig5-HT2, or ds-LmigGABAb. *p < 0.05, **p < 0.01. ns, no significant difference.
Therefore, the downregulation of Lmig5-HT2 or LmigGABAb resulted in a significant amplification of odor-evoked responses, as represented by Δspikes/s and the response duration of ORNs. This suggests that Lmig5-HT2 and LmigGABAb may provide negative feedback in local ORNs when they respond to odorants.
3.5. Downregulation Lmig5-HT2 or LmigGABAb ORNs respond dose-dependently to odorants
We, next, examined the responses of Ba6 neurons in ds-Lmig5HT2, ds-LmigGABAb, ds-GFP, and wild-type locusts to benzaldehyde (Figure 7A), guaiacol (Figure 7B), and phenylacetonitrile (Figure 7C) at concentrations (v/v) of 0.1%, 0.5%, 1%, 5%, and 10%.
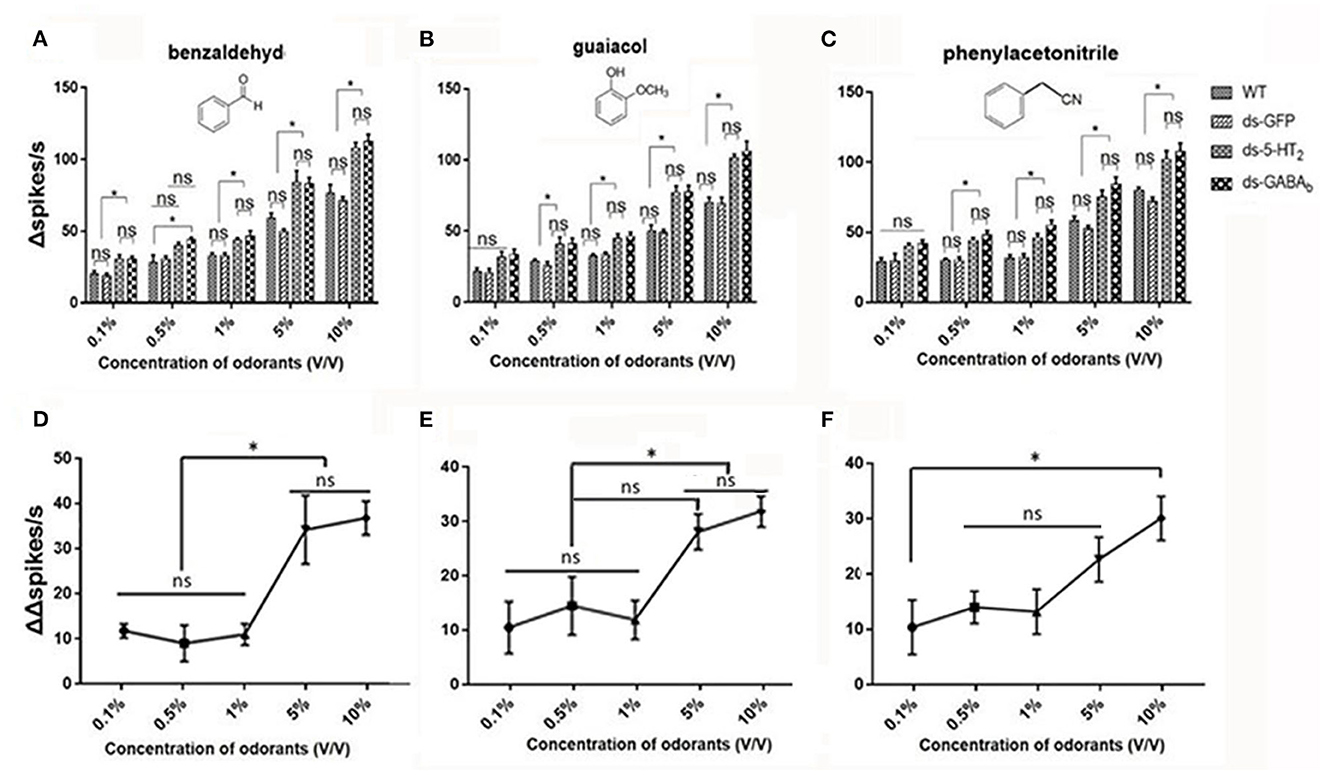
Figure 7. ORNs in the antennal basiconic sensilla of wild-type, ds-GFP, ds-5-HT2, and ds-GABAb locusts have dose-dependent responses to odorants. (A) The Δspikes/s numbers of Ba6 ORNs of locusts (WT, wild-type locusts; ds-GFP, locusts injected with ds-GFP; ds-5-HT2, locusts injected with ds-Lmig5-HT2; ds-GABAb, locusts injected with ds-LmigGABAb) in response to benzaldehyde. (B) The Δspikes/s numbers of Ba6 ORNs of WT, ds-GFP, ds-5-HT2, and ds-GABAb locusts in response to guaiacol. (C) The Δspikes/s numbers of Ba6 ORNs of WT, ds-GFP, ds-5-HT2, and ds-GABAb locusts in response to phenylacetonitrile. (D–F) The change of Δspikes/s numbers (represented by (ΔΔspikes/s) of Ba6 ORNs) in response to the three odorants between ds-5-HT2 or ds-GABAb and WT or ds-GFP. Values are the mean ± SEM, n = 5 per group. *p < 0.05, one-way ANOVA with Tukey's post-hoc tests. ns, no significant difference.
In Figures 7A–C, when using 0.1% of the concentration of the three odorants to stimulate, only benzaldehyde cause a significantly higher response (number of Δspikes/s) of RNAi of 5-HT2 receptor and GABAb genes (ds-5-HT2 and ds-GABAb) than those of WT and ds-GFP. Nevertheless, at 0.5%, 1%, 5%, and 10% concentrations, all responses of ds-5-HT2 and ds-GABAb exhibited significantly higher responses to all three odorants. This indicated that 5-HT2 and GABAb receptors do not function as inhibition when stimulation of odorant at low concentration, and there may be a threshold of odor concentration that they function.
Furthermore, we want to know whether there is a threshold odor concentration that affects the negative feedback provided by 5HT and GABA. In other words, does the strength of this negative feedback vary depending on the concentration of the stimulus odor? Therefore, we calculated the different values (represented by ΔΔspikes/s) between the ORNs' Δspikes in ds-5-HT2 and that in ds-GFP locusts using the same odor stimulus as a measure of the intensity of the 5HT negative feedback. We found that at low concentrations (0.1% to 1%) of benzaldehyde and guaiacol, ΔΔspikes/s kept stable, but as the concentrations increased, ΔΔspikes/s sharply increased to produce significant differences to low concentrations (Figures 7D, E). However, when phenylacetonitrile was used as the stimulus, ΔΔspikes/s increases with increasing concentration, with a significant difference in ΔΔspikes/s only between 0.1% and 10% concentrations (Figure 7F). The ΔΔspikes/s between ds-GABAb and dsGFP locusts as a variety of compounds' concentration was compared, and the trend was similar to that of ds-5-HT2 (Supplementary Figure S5). This finding indicates that 5-HT/GABA has a clear inhibitory or negative modulatory role in neuronal responses elicited by concentrations of odors, which means that the higher the concentration of odors, the stronger the inhibition of 5-HT/GABA to neuronal responses in the antenna of locust.
4. Discussion
Our experiment demonstrated that 5-HT and GABA exist in the antenna because two key synthetic enzyme genes for 5-HT were localized in the antenna, and these two genes were detected by PCR. However, the two synthetic enzyme genes for 5-HT were localized in cells surrounding ORNs in the antenna, indicating that 5-HT may be synthesized in cells surrounding ORNs, but not ORNs. Furthermore, our experiment on the localization of the 5-HT receptor in the antenna showed that the receptor was expressed in accessory cells surrounding ORNs, whereas the receptor was expressed in the GABAb receptor gene in ORNs. Depression of the 5-HT receptor gene in isolated antennae caused similar response patterns of antennal ORNs in locusts. Therefore, 5-HT, GABA, and their receptors exist in the peripheral nervous system and are at least partially involved in the local modulation of olfaction in insect antennae. Among them, the results of the 5HT receptor's localization were consistent with the previous studies on mosquitoes that 5-HT-immunoreactive fibers were observed in the antenna (Siju et al., 2008; Pitts et al., 2011).
A majority of previous studies of 5-HT function were focused on the CNS (Hurley et al., 2004; Becnel et al., 2011; Brunert et al., 2016; Sizemore and Dacks, 2016) or throughout the nervous system (Anstey et al., 2009; Guo et al., 2013; Tanaka and Nishide, 2013). For instance, 5-HT has been proposed to regulate gregarious behavior in the desert locust, Schistocerca gregaria (Anstey et al., 2009). Another study suggested that 5-HT enhances solitariness in the phase transition of locusts (Guo et al., 2013). Reciprocally, Tanaka et al. have suggested that 5-HT has no effect on the attraction/avoidance behavior, which may be the most important factor in the process of gregarious behavior of desert locusts (Tanaka and Nishide, 2013). Nonetheless, these studies on 5HT function do not distinguish between the central nervous system and the peripheral nervous system. There need to be further studies on 5-HT function in the peripheral nervous system of insects because of a lack of studies on its receptors. At least nine types of 5-HT receptors have been identified throughout the various layers of the mammalian olfactory bulb (Pazos et al., 1985; Shen et al., 1993; Tecott et al., 1993; Grailhe et al., 1999; Yuan et al., 2003; Ganesh et al., 2010; Klein et al., 2012; Oba et al., 2013; Suwa et al., 2014), and five receptor classes are broadly distributed throughout multiple cell types in the insect antennal lobe (Witz et al., 1990; Saudou et al., 1992; Colas et al., 1995; Gasque et al., 2013; Sizemore and Dacks, 2016). In our study, only one 5-HT receptor gene, Lmig5-HT2, was reported. However, there might be more other putative 5-HT receptor genes (series No. MN531678, MN531680) in locust antenna when we searched by tBlast and PCR (Supplementary Figure S4), and their function is open to be studied.
Little is known about the expression of 5-HT receptors in the peripheral nervous system, particularly their relationship with ORNs and accessory cells in sensilla of the antenna. Our dual-color fluorescence in situ hybridization experiments showed that Lmig5-HT2 localized in accessory cells adjacent to ORNs, but not in ORNs. This is similar to its expression in mammals that 5-HT2C receptor expressed in some juxtaglomerular cells in the olfactory bulb, not in ORNs (Petzold et al., 2009). In addition, our study showed that the GABAb receptor was identified and localized in ORNs of antenna, which is similar to a few previous studies that solely reported GABAb receptors in ORNs (Root et al., 2008; Pregitzer et al., 2013). Our localization experiment on 5-HT2 and GABAb receptors revealed the spatial relationship between these two receptors in insect antennae, and this provides a link to understanding the pathway for 5-HT/GABA and their receptors' functions considering that 5-HT/GABA receptors were often demonstrated in the cascade in the modulation of the nervous process (Jacobs and Azmitia, 1992; Dacks et al., 2008; Kloppenburg and Mercer, 2008).
By measuring the responses of ORNs after suppressing the expression of corresponding genes, we demonstrated that the Lmig5-HT2 receptor plays an inhibitory role in the response of ORNs to odorants, which results in the regulation of the signal output. Previous studies in moths suggested that 5-HT2 in the sensillum, lymph might regulate neuronal activity by altering the transepithelial potential, thus changing the threshold of firing without influencing the spontaneous action potential activity of ORNs (Dolzer et al., 2001). Because ORNs performed in very similar patterns when locusts had knockdown of GABAb, we suggest that regulation by the Lmig5-HT2 receptor may indirectly mediate olfaction through the activation of the LmigGABAb receptor in the ORNs of antennae. These results indirectly supported the pathway for 5-HT/GABA and their receptors' function in a cascade too.
Our findings indicate that the depression of the 5-HT2 and GABAb receptors increased sensory responses to odors, and in other words, the 5-HT2 and GABAb attenuated sensory response. In addition, the depression of 5-HT2 and GABAb receptors caused a dose-dependent response, indicating that serotonergic/GABAnergic modulation of odor input in the peripheral nervous system may be dose-dependent, and the modulation starts when the concentration of odorants over the thresholds. Another interesting result was that at higher odor concentrations, the inhibition by serotonin/GABA became stronger. Such dose-dependent modulation of ORNs during olfaction can dynamically control olfactory input. This process is similar to that of the olfactory bulbs of mammals or the antennal lobes of insects (Dacks et al., 2009; Petzold et al., 2009). However, it is the opposite of a study on moths that serotonin increased the amplitude of odor-evoked neuronal responses in the antenna (Dacks et al., 2008). It is interesting that our experiment on the isolated antenna (the antenna was cut from the body of locusts) for the first time showed that the depression of 5-HT and GABA receptors elicited an increase in responses of ORNs, indicating that the serotonergic/GABAergic modulation of odor input in the peripheral nervous system may be independent to some extent from the brain in insects.
The odorants pass through the pores on the sensillar wall and are then bound by OBPs that are secreted by accessory cells to form the odorant/OBP complex, which is transported onto ORs and OR co-receptors on the membranes of ORN dendrites, thus evoking action potentials in ORNs (Laughlin et al., 2008). Studies have shown that in the antennae of male Manduca sexta moths, 5-HT affects the transepithelial potential, generated by accessory cells in the olfactory sensillum and creates a driving force for the receptor current (Dolzer et al., 2001; Grosmaitre et al., 2001), whereas it has no direct effect on the activity of spontaneous action potential of olfactory receptor neurons (Dolzer et al., 2001). This is consistent with our results that the 5-HT receptor is expressed in the accessory cells but not on ORNs, which suggests that 5-HT acts directly on accessory cells to affect the ORNs when acting in the peripheral nervous system, rather than directly on ORNs. Response suppression about 5-HT might also be an important mechanism by which the brain uses 5-HT to gate out sensory information that might otherwise compete for attention or other cognitive resources (Saudou et al., 1992).
Data availability statement
The data presented in the study are deposited in the NCBI repository, accession number PRJNA950534. The deposition data has been successfully published, linked below. https://www.ncbi.nlm.nih.gov/bioproject/PRJNA950534/; https://doi.org/10.6084/m9.figshare.22216951.v1.
Author contributions
XZ proposed the idea. XZ, ML, and LZ designed the experiments. XX, ML, and BY conducted the experiments. XZ, ML, XX, and LZ wrote the manuscript. All authors contributed to the article and approved the submitted version.
Funding
This study was supported by the National Nature Science Foundation of China [Grant number: 31872968], the National Key R&D Program of China [2022YFD1400504], the Agricultural Scientific and Technological Innovation Project of Shandong Academy of Agricultural Sciences [CXGC2022E04], and the foundations from University of Cambridge for XZ.
Acknowledgments
We thank Dr. Liwei Zhang for the discussion and help in gene sequence analysis.
Conflict of interest
The authors declare that the research was conducted in the absence of any commercial or financial relationships that could be construed as a potential conflict of interest.
Publisher's note
All claims expressed in this article are solely those of the authors and do not necessarily represent those of their affiliated organizations, or those of the publisher, the editors and the reviewers. Any product that may be evaluated in this article, or claim that may be made by its manufacturer, is not guaranteed or endorsed by the publisher.
Supplementary material
The Supplementary Material for this article can be found online at: https://www.frontiersin.org/articles/10.3389/fncel.2023.1156144/full#supplementary-material
Abbreviations
CNS, central nervous system; PNS, peripheral nervous system; 5-HT, 5-hydroxytryptamine; OR, olfactory receptor; Orco, co-olfactory receptor; OBP, odorant-binding protein; ORN, olfactory receptor neuron; AC, accessory cell; SSR, single sensillum recording; PN, projection neuron; OB, olfactory bulb; AL, antennal lobe; TPH, tryptophan hydroxylase; AADC, aromatic L-amino acid decarboxylase; ds-RNA, double-stranded RNA.
References
Albin, S. D., Kaun, K. R., Knapp, J. M., Chung, P., Heberlein, U., and Simpson, J. H. (2015). A subset of serotonergic neurons evokes hunger in adult Drosophila. Curr. Biol. 25, 2435–2440. doi: 10.1016/j.cub.2015.08.005
Anstey, M. L., Rogers, S. M., Ott, S. R., Burrows, M., and Simpson, S. J. (2009). Serotonin mediates behavioral gregarization underlying swarm formation in desert locusts. Science. 323, 627–630. doi: 10.1126/science.1165939
Becnel, J., Johnson, O., Nässel, D. R., and Nichols, C. D. (2011). The serotonin 5-HT7Dro receptor is expressed in the brain of Drosophila, and is essential for normal courtship and mating. PLoS ONE. 6, e20800. doi: 10.1371/journal.pone.0020800
Brunert, D., Tsuno, Y., Rothermel, M., Shipley, M. T., and Wachowiask, M. (2016). Cell-type-specific modulation of sensory responses in olfactory bulb circuits by serotonergic projections from the raphe nuclei. J. Neurosci. 36, 6820–6835. doi: 10.1523/JNEUROSCI.3667-15.2016
Clyne, P. J., Warr, C. G., Freeman, M. R., Lessing, D., Kim, J., and Carlson, J. R. (1999). A novel family of divergent seven-transmembrane proteins: candidate odorant receptors in Drosophila. Neuron. 22, 327–338. doi: 10.1016/S0896-6273(00)81093-4
Colas, J. F., Launay, J. M., Kellermann, O., Rosay, P., and Maroteaux, L. (1995). Drosophila 5-HT2 serotonin receptor: Coexpression with fushi-tarazu during segmentation. Proc. Natl. Acad. Sci. U. S. A. 92, 5441–5445. doi: 10.1073/pnas.92.12.5441
Dacks, A. M., Christensen, T. A., and Hildebrand, J. G. (2008). Modulation of olfactory information processing in the antennal lobe of Manduca sexta by serotonin. J. Neurophysiol. 99, 2077–2085. doi: 10.1152/jn.01372.2007
Dacks, J. G., Green, D. S., Root, C. M., Nighorn, A. J., and Wang, J. W. (2009). Serotonin modulates olfactory processing in the antennal lobe of Drosophila. J. Neurogenet. 23, 366–377. doi: 10.3109/01677060903085722
Dierick, H. A., and Greenspan, R. J. (2007). Serotonin and neuropeptide F have opposite modulatory effects on fly aggression. Nat. Genet. 39, 678–682. doi: 10.1038/ng2029
Dobritsa, A. A., Van Der Goes Van Naters, W., Warr, C. G., Steinbrecht, R. A., and Carlson, J. R. (2003). Integrating the molecular and cellular basis of odor coding in the Drosophila antenna. Neuron 37, 827–841. doi: 10.1016/S0896-6273(03)00094-1
Dolzer, J., Krannich, S., Fischer, K., and Stengl, M. (2001). Oscillations of the transepithelial potential of moth olfactory sensilla are influenced by octopamine and serotonin. J. Exp. Biol. 204, 2781–2794. doi: 10.1242/jeb.204.16.2781
Dugué, G. P., and Mainen, Z. F. (2009). How serotonin gates olfactory information flow. Nat. Neurosci. 12, 673–675. doi: 10.1038/nn0609-673
Fletcher, M. L., and Chen, W. R. (2010). Neural correlates of olfactory learning: critical role of centrifugal neuromodulation. Learn Mem. 17, 561–570. doi: 10.1101/lm.941510
Ganesh, A., Bogdanowicz, W., Haupt, M., Marimuthu, G., and Rajan, K. E. (2010). Role of olfactory bulb serotonin in olfactory learning in the greater short-nosed fruit bat, Cynopterus sphinx (Chiroptera: Pteropodidae). Brain Res. 1352, 108–117. doi: 10.1016/j.brainres.2010.06.058
Gasque, G., Conway, S., Huang, J., Rao, Y., and Vosshall, L. B. (2013). Small molecule drug screening in Drosophila identifies the 5HT2A receptor as a feeding modulation target. Sci. Rep. 3, 1–8. doi: 10.1038/srep02120
Gatellier, L., Nagao, T., and Kanzaki, R. (2004). Serotonin modifies the sensitivity of the male silkmoth to pheromone. J. Exp. Biol. 207, 2487–2496. doi: 10.1242/jeb.01035
Gaudry, Q. (2018). Serotonergic modulation of olfaction in rodents and insects. Yale J. Biol. Med. 91, 23–32.
Grailhe, R., Waeber, C., Dulawa, S. C., Hornung, J. P., Zhuang, X., Brunner, D., et al. (1999). Increased exploratory activity and altered response to LSD in Mice lacking the 5-HT5A receptor. Neuron. 22, 581–591. doi: 10.1016/S0896-6273(00)80712-6
Grosmaitre, X., Marion-Poll, F., and Renou, M. (2001). Biogenic amines modulate olfactory neurons firing activity in Mamestra brassicae. Chem. Senses. 26, 653–661. doi: 10.1093/chemse/26.6.653
Guo, X., Ma, Z., and Kang, L. (2013). Serotonin enhances solitariness in phase transition of the migratory locust. Front. Behav. Neurosci. 7, 1–12. doi: 10.3389/fnbeh.2013.00129
Hallem, E. A., and Carlson, J. R. (2006). Coding of odors by a receptor repertoire. Cell. 125, 143–160. doi: 10.1016/j.cell.2006.01.050
Hildebrand, J. G., and Shepherd, G. M. (1997). Mechanisms if olfactory discrimination: Converging evidence for common principles across phyla. Annu. Rev. Neurosci. 20, 595–631. doi: 10.1146/annurev.neuro.20.1.595
Hurley, L. M., Devilbiss, D. M., and Waterhouse, B. D. (2004). A matter of focus: Monoaminergic modulation of stimulus coding in mammalian sensory networks. Curr. Opin. Neurobiol. 14, 488–495. doi: 10.1016/j.conb.2004.06.007
Jacobs, B. L., and Azmitia, E. C. (1992). Structure and function of the brain serotonin system. Physiol. Rev. 72, 165–229.
Jin, X., Brandazza, A., Navarrini, A., Ban, L., Zhang, S., Steinbrecht, R. A., et al. (2005). Expression and immunolocalisation of odorant-binding and chemosensory proteins in locusts. Cell. Mol. Life Sci. 62, 1156–1166. doi: 10.3390/ijms24065595
Johnson, O., Becnel, J., and Nichols, C. D. (2011). Serotonin receptor activity is necessary for olfactory learning and memory in Drosophila melanogaster. Neuroscience. 192, 372–381. doi: 10.1016/j.neuroscience.2011.06.058
Kapoor, V., Provost, A. C., Agarwal, P., and Murthy, V. N. (2016). Activation of raphe nuclei triggers rapid and distinct effects on parallel olfactory bulb output channels. Nat. Neurosci. 19, 271–282. doi: 10.1038/nn.4219
Klein, M. T., Teitler, M., and Pharmacol, J. (2012). Distribution of 5-ht 1E receptors in the mammalian brain and cerebral vasculature: an immunohistochemical and pharmacological study. Br. J. Pharmacol. 166, 1290–1302. doi: 10.1038/nrn2789
Kloppenburg, P., and Hildebrand, J. G. (1995). Neuromodulation by 5-hydroxytryptamine in the antennal lobe of the sphinx moth Manduca sexta. J. Exp. Biol. 198, 606–611.
Kloppenburg, P., and Mercer, A. R. (2008). Serotonin modulation of moth central olfactory neurons. Annu. Rev. Entomol. 53, 179–190. doi: 10.1146/annurev.ento.53.103106.093408
Lange, A. B., Orchard, I., and Barrett, F. M. (1989). Changes in haemolymph serotonin levels associated with feeding in the blood-sucking bug, Rhodnius prolixus. J. Insect Physiol. 35, 393–399. doi: 10.1002/arch.20010
Laughlin, J. D., Ha, T. S., Jones, D. N. M., and Smith, D. P. (2008). Activation of pheromone-sensitive neurons is mediated by conformational activation of pheromone-binding protein. Cell 133, 1255–1265. doi: 10.1016/j.cell.2008.04.046
Leal, W. S. (2013). Odorant reception in insects: Roles of receptors, binding proteins, and degrading enzymes. Annu. Rev. Entomol. 58, 373–391. doi: 10.1146/annurev-ento-120811-153635
Liu, S., Aungst, J. L., Puche, A. C., and Shipley, M. T. (2012). Serotonin modulates the population activity profile of olfactory bulb external tufted cells. J. Neurophysiol. 107, 473–483. doi: 10.1152/jn.00741.2011
McLean, J. H., Darby-King, A., and Sullivan, R. M. (1993). Serotonergic influence on olfactory learning in the neonate rat. Behav. Neural. Biol. 60, 152–162. doi: 10.1016/j.neuron.2005.10.031
Oba, A., Nakagawasai, O., Onogi, H., Nemoto, W., Yaoita, F., Arai, Y., et al. (2013). Chronic fluvoxamine treatment changes 5-HT2A/2C receptor-mediated behavior in olfactory bulbectomized mice. Life Sci. 92, 119–124. doi: 10.1016/j.lfs.2012.11.005
Pazos, A., Cortés, R., and Palacios, J. M. (1985). Quantitative autoradiographic mapping of serotonin receptors in the rat brain. II. Serotonin-2 receptors. Brain Res. 346, 231–249. doi: 10.1016/0006-8993(85)90857-1
Pelosi, P., Zhou, J. J., Ban, L. P., and Calvello, M. (2006). Soluble proteins in insect chemical communication. Cell. Mol. Life Sci. 63, 1658–1676. doi: 10.1007/s00018-005-5607-0
Petzold, G. C., Hagiwara, A., and Murthy, V. N. (2009). Serotonergic modulation of odor input to the mammalian olfactory bulb. Nat. Neurosci. 12, 784–791. doi: 10.1038/nn.2335
Pitts, R. J., Rinker, D. C., Jones, P. L., Rokas, A., and Zwiebel, L. J. (2011). Transcriptome profiling of chemosensory appendages in the malaria vector Anopheles gambiae reveals tissue-and sex-specific signatures of odor coding. BMC Genomics. 12, 271. doi: 10.1186/1471-2164-12-271
Pregitzer, P., Schultze, A., Raming, K., Breer, H., and Krieger, J. (2013). Expression of a GABAB - receptor in olfactory sensory neurons of sensilla trichodea on the male antenna of the moth, Heliothis virescens. Int. J. Biol. Sci. 9, 707–715. doi: 10.7150/ijbs.6674
Root, C. M., Masuyama, K., Green, D. S., Enell, L. E., Nässel, D. R., Lee, C. H., et al. (2008). A presynaptic gain control mechanism fine-tunes olfactory behavior. Neuron. 59, 311–321. doi: 10.1016/j.neuron.2008.07.003
Sato, K., Pellegrino, M., Nakagawa, T., Nakagawa, T., Vosshall, L. B., and Touhara, K. (2008). Insect olfactory receptors are heteromeric ligand-gated ion channels. Nature. 452, 1002–1006. doi: 10.1038/nature06850
Saudou, F., Boschert, U., Amlaiky, N., Plassat, J. L., and Hen, R. (1992). A family of Drosophila serotonin receptors with distinct intracellular signalling properties and expression patterns. EMBO J. 11, 7–17. doi: 10.1002/j.1460-2075.1992.tb05021.x
Shen, Y., Monsma, F. J., Metcalf, M. A., Jose, P. A., Hamblin, M. W., and Sibley, D. R. (1993). Molecular cloning and expression of a 5-hydroxytryptamine7 serotonin receptor subtype. J. Biol. Chem. 268, 18200–18204.
Siju, K. P., Hansson, B. S., and Ignell, R. (2008). Immunocytochemical localization of serotonin in the central and peripheral chemosensory system of mosquitoes. Arthropod Struct. Dev. 37, 248–259. doi: 10.1016/j.asd.2007.12.001
Sizemore, T. R., and Dacks, A. M. (2016). Serotonergic modulation differentially targets distinct network elements within the antennal lobe of Drosophila melanogaster. Sci. Rep. 2, 1–14. doi: 10.1038/srep37119
Suwa, B., Bock, N., Preusse, S., Rothenberger, A., and Manzke, T. (2014). Distribution of serotonin 4(a) receptors in the juvenile rat brain and spinal cord. J. Chem. Neuroanat. 55, 67–77. doi: 10.1016/j.jchemneu.2013.12.004
Tanaka, S., and Nishide, Y. (2013). Behavioral phase shift in nymphs of the desert locust, Schistocerca gregaria: Special attention to attraction/avoidance behaviors and the role of serotonin. J. Insect Physiol. 59, 101–112. doi: 10.1016/j.jinsphys.2012.10.018
Tecott, L. H., Maricq, A. V., and Juliust, D. (1993). Nervous system distribution of the serotonin 5-HT3 receptor mRNA. Proc. Nati. Acad. Sci. U. S. A. 90, 1430–1434. doi: 10.1073/pnas.90.4.1430
Wang, L., and Anderson, D. J. (2010). Identification of an aggression-promoting pheromone and its receptor neurons in Drosophila. Nature 463, 227–231. doi: 10.1038/nature08678
Wei, J., Shao, W., Wang, X., Ge, J., Chen, X., Yu, D., et al. (2017). Composition and emission dynamics of migratory locust volatiles in response to changes in developmental stages and population density. Insect Sci. 24, 60–72. doi: 10.1111/1744-7917.12396
Wicher, D., Schäfer, R., Bauernfeind, R., Stensmyr, M. C., Heller, R., Heinemann, S. H., et al. (2008). Drosophila odorant receptors are both ligand-gated and cyclic-nucleotide- activated cation channels. Nature 452, 1007–1011. doi: 10.1038/nature06861
Witz, P., Amlaiky, N., Plassat, J. L., Maroteaux, L., Borrelli, E., and Hen, R. (1990). Cloning and characterization of a Drosophila serotonin receptor that activates adenylate cyclase. Proc. Natl. Acad. Sci. U. S. A. 87, 8940–8944. doi: 10.1073/pnas.87.22.8940
Xu, H., Guo, M., Yang, Y., You, Y., and Zhang, L. (2013). Differential expression of two novel odorant receptors in the locust (Locusta migratoria). BMC Neurosci. 14, 50. doi: 10.1186/1471-2202-14-50
Xu, X., You, Y., and Zhang, L. (2017). Localization of odorant receptor genes in locust antennae by RNA in situ hybridization. J. Vis. Exp. 125, e55924. doi: 10.3791/55924
Yang, Y., Krieger, J., Zhang, L., and Breer, H. (2011). The olfactory co-receptor Orco from the migratory locust (Locusta migratoria) and the desert locust (Schistocerca gregaria): Identification and expression pattern. Int. J. Biol. Sci. 8, 159–170. doi: 10.7150/ijbs.8.159
Yuan, Q., Harley, C. W., and McLean, J. H. (2003). Mitral cell β1 and 5-HT2A receptor colocalization and cAMP coregulation: a new model of norepinephrine-induced learning in the olfactory bulb. Learn Mem. 10, 5–15. doi: 10.1101/lm.54803
Zhang, L., Li, H., and Zhang, L. (2017). Two olfactory pathways to detect aldehydes on locust mouthpart. Int. J. Biol. Sci. 13, 759–771. doi: 10.7150/ijbs.19820
Zhang, X., and Gaudry, Q. (2016). Functional integration of a serotonergic neuron in the Drosophila antennal lobe. eLife. 5, e16836. doi: 10.7554/eLife.16836
Keywords: modulation, 5-HT receptor, GABA receptor, olfaction, olfactory receptor neurons, peripheral nervous system
Citation: Lv M, Xu X, Zhang X, Yuwen B and Zhang L (2023) Serotonin/GABA receptors modulate odor input to olfactory receptor neuron in locusts. Front. Cell. Neurosci. 17:1156144. doi: 10.3389/fncel.2023.1156144
Received: 01 February 2023; Accepted: 27 March 2023;
Published: 28 April 2023.
Edited by:
Xin-Cheng Zhao, Henan Agricultural University, ChinaReviewed by:
Monika Stengl, University of Kassel, GermanyShuang-Lin Dong, Nanjing Agricultural University, China
Copyright © 2023 Lv, Xu, Zhang, Yuwen and Zhang. This is an open-access article distributed under the terms of the Creative Commons Attribution License (CC BY). The use, distribution or reproduction in other forums is permitted, provided the original author(s) and the copyright owner(s) are credited and that the original publication in this journal is cited, in accordance with accepted academic practice. No use, distribution or reproduction is permitted which does not comply with these terms.
*Correspondence: Xinyang Zhang, eHozMzQmI3gwMDA0MDtjYW0uYWMudWs=; Long Zhang, bG9jdXN0JiN4MDAwNDA7Y2F1LmVkdS5jbg==
†These authors have contributed equally to this work