- 1State Key Laboratory of Oral Diseases, National Clinical Research Center for Oral Diseases, National Center for Stomatology, West China School of Stomatology, Sichuan University, Chengdu, China
- 2Department of Prosthodontics, West China Hospital of Stomatology, Sichuan University, Chengdu, China
Peripheral and central sensitizations of the trigeminal nervous system are the main mechanisms to promote the development and maintenance of chronic orofacial pain characterized by allodynia, hyperalgesia, and ectopic pain after trigeminal nerve injury or inflammation. Although the pathomechanisms of chronic orofacial pain are complex and not well known, sufficient clinical and preclinical evidence supports the contribution of the N-methyl-D-aspartate receptors (NMDARs, a subclass of ionotropic glutamate receptors) to the trigeminal nociceptive signal processing pathway under various pathological conditions. NMDARs not only have been implicated as a potential mediator of pain-related neuroplasticity in the peripheral nervous system (PNS) but also mediate excitatory synaptic transmission and synaptic plasticity in the central nervous system (CNS). In this review, we focus on the pivotal roles and mechanisms of NMDARs in the trigeminal nervous system under orofacial neuropathic and inflammatory pain. In particular, we summarize the types, components, and distribution of NMDARs in the trigeminal nervous system. Besides, we discuss the regulatory roles of neuron-nonneuronal cell/neuron-neuron communication mediated by NMDARs in the peripheral mechanisms of chronic orofacial pain following neuropathic injury and inflammation. Furthermore, we review the functional roles and mechanisms of NMDARs in the ascending and descending circuits under orofacial neuropathic and inflammatory pain conditions, which contribute to the central sensitization. These findings are not only relevant to understanding the underlying mechanisms, but also shed new light on the targeted therapy of chronic orofacial pain.
Introduction
Orofacial pain comprises multiple pain conditions of dentoalveolar, myofascial, temporomandibular joint (TMJ), musculoskeletal, neurovascular, and neuropathic origin affecting the oral, face, head, and neck areas (Anonymous, 2020). Patients with orofacial pain have been diagnosed with temporomandibular joint disorders (TMD), neuralgias, migraine, trauma, nerve injuries, inflammation, cancer, as well as various neurological/muscle disorders (Sessle, 2021). It markedly impacts the life quality of patients and brings a huge social and economic burden to medicine and society. Acute pain lasting less than 3 months is a protective mechanism that alerts the body to tissue damage and can be well controlled (Kuner and Kuner, 2021). while chronic pain present for more than 3 months is difficult to diagnose and treat because of its complex etiology and pathogenesis. Chronic orofacial pain, typically characterized by the spontaneous nature, allodynia, hyperalgesia as well as pain extra-territorial spread and referral, is often induced by nerve injury, orofacial tissue inflammation, and TMD (Dahlstrom and Carlsson, 2010; Shinoda et al., 2019; Sessle, 2021). Besides, it is also often accompanied by a variety of comorbidities, including depression, anxiety, stress, and sleep disorders (Sessle, 2021). Peripheral and central sensitization is considered to be involved in the above characteristics of chronic orofacial pain (Shinoda et al., 2019). Although lots of signal molecules, receptors, ion channels, and intracellular signal pathways involved in the activation or sensitization of the nociceptive afferents have been reported, the knowledge of the mechanisms underlying the initiation and maintenance of chronic orofacial pain is still limited, and more sufficiently and consistently effective treatments are needed to be found.
N-methyl-D-aspartate receptors (NMDARs, a subclass of ionotropic glutamate receptors) have been proved to be associated with abnormalities in the peripheral nervous system (PNS) and central nervous system (CNS), resulting in neuronal excitation and chronic pain manifestations (Petrenko et al., 2003; Hansen et al., 2018). Recently, increasing evidence has also investigated the role and mechanisms of NMDARs in the peripheral and central sensitization of chronic orofacial pain. The findings demonstrate that the up-regulation and activation of NMDARs in the trigeminal nervous system initiate intracellular cascades through calcium influx and activation of protein kinases, which in turn modulates cell membrane excitability and enhances nociceptive transmission, resulting in chronic orofacial inflammatory and neuropathic pain (Kimura et al., 2022; Zhang et al., 2022). Therefore, NMDAR has been proposed as a promising target for the treatment of chronic orofacial pain. Current studies have found that a variety of NMDAR antagonists and modulators have an analgesic effect on patients with pain, including ketamine, memantine, dextromethorphan, magnesium, etc. (Zhou et al., 2011; Shiiba et al., 2021). While there is still ambiguity about the efficacy of NMDAR antagonists on chronic pain because positive as well as negative effects of NMDAR antagonists on pain relief are found in clinical trials (Collins et al., 2010). In addition, because NMDARs exist widely in the nervous system and the physiological activity of NMDARs is essential to maintain the normal function of neurons, systemic administration of NMDAR antagonists may lead to a series of adverse events, including loss of consciousness, sedation, hallucinations, hearing and postural disorders, insomnia and so on (McCartney et al., 2004; Alviar et al., 2016). In order to provide a better understanding of the role and mechanisms of NMDARs in the regulation of chronic orofacial pain, and to facilitate further research on therapeutic strategies and new drugs targeting NMDARs, the types, components, and distribution of NMDARs in the trigeminal nervous system were discussed in this topical review. Besides, we discussed the regulatory roles and mechanisms of NMDARs in the peripheral and central sensitization in the ascending and descending circuits for chronic orofacial pain following nerve injury and inflammation.NMDARs are also involved in the regulation of acute pain which participates in the transition from acute to chronic pain and NMDAR antagonists can provide effective control of acute postoperative pain (Corder et al., 2013; Tognoli et al., 2020), while it is not the main point of this topical review.
PubMed, MEDLINE, Google Scholar, Scopus, and Embase were searched for the studies with English restriction focusing on the role of NMDARs in the peripheral and central nervous systems that contribute to chronic orofacial pain from the inception of the databases to Aug 9, 2022. The search strategy followed was: (NMDA receptor OR N-Methyl-D-aspartate receptor) AND (chronic pain OR neuropathic pain OR chronic inflammatory pain) AND (orofacial OR trigeminal) in Title/Abstract.
Neural Circuits for The Signal Transmission of Orofacial Pain
Peripheral orofacial noxious information is transmitted ultimately to the cortex and subcortical nuclei via three-order neurons (Figure 1A). The primary neurons are trigeminal ganglion neurons (TGNs), which are pseudounipolar neurons with peripheral and central processes. Their peripheral processes innervate peripherally to facial skin, oral mucosa, jaw, teeth, and deep tissues such as masseter to receive nociceptive, mechanical, and thermal sensations. Besides, their central processes project centrally to the spinal trigeminal nucleus (SpV) subdivided into oralis (Vo), interpolaris (Vi), and caudalis (Vc) located in the medullar oblongata and to the upper cervical spinal cord (C1/C2) to synapse with the secondary neurons (Olszewski, 1950; Fernandez-Montoya et al., 2017). Then, the secondary neurons in the SpV and C1/C2 decussate to the contralateral side and ascend to make synapses with the third-order neurons located in the higher nucleus in the thalamus and brain. Retrograde tract-tracing and anatomical studies demonstrate that the secondary neurons in Vi/c, Vc, and C1/C2 mainly project upward to the parabrachial nucleus (PBN) located in the pons and ventral posteromedial nucleus of the thalamus (VPM; Ren and Dubner, 2011; Li X. et al., 2017; Zhang et al., 2018). According to previous studies, it has been confirmed that the Vc-thalamic pathway and Vc-PBN pathway are two important ascending projection pathways that mediate orofacial pain (Nash et al., 2009; Saito et al., 2017). It is generally believed that the Vc-thalamic pathway is related to the sensory discrimination of pain, such as the intensity, nature, and location, while the Vc-PBN pathway is involved in pain-related emotions (Gauriau and Bernard, 2002; Bushnell et al., 2013; Chiang et al., 2019). Nociceptive information from the thalamus is further sent to the cortex (Castro et al., 2017). Neuroelectrical and neurochemical methods have confirmed that the brain regions related to pain perception included the anterior cingulate cortex (ACC), insular cortex (IC), the primary somatosensory cortex (S1), secondary somatosensory cortex (S2), and prefrontal cortex (PFC; Apkarian et al., 2005). Neurons in the PBN project upwards to the central amygdala (CeA), hypothalamus, the periaqueductal gray (PAG), and ventrolateral medulla (VPM), which are thought to be involved in processing the emotional components of pain (Missig et al., 2017; Liu et al., 2019; Wilson et al., 2019; Deng et al., 2020; Jiang et al., 2021). These findings on the anatomical and functional organization between the orofacial input and central termination explain chronic orofacial pain can be emotional and interact with autonomic and hormonal functions.
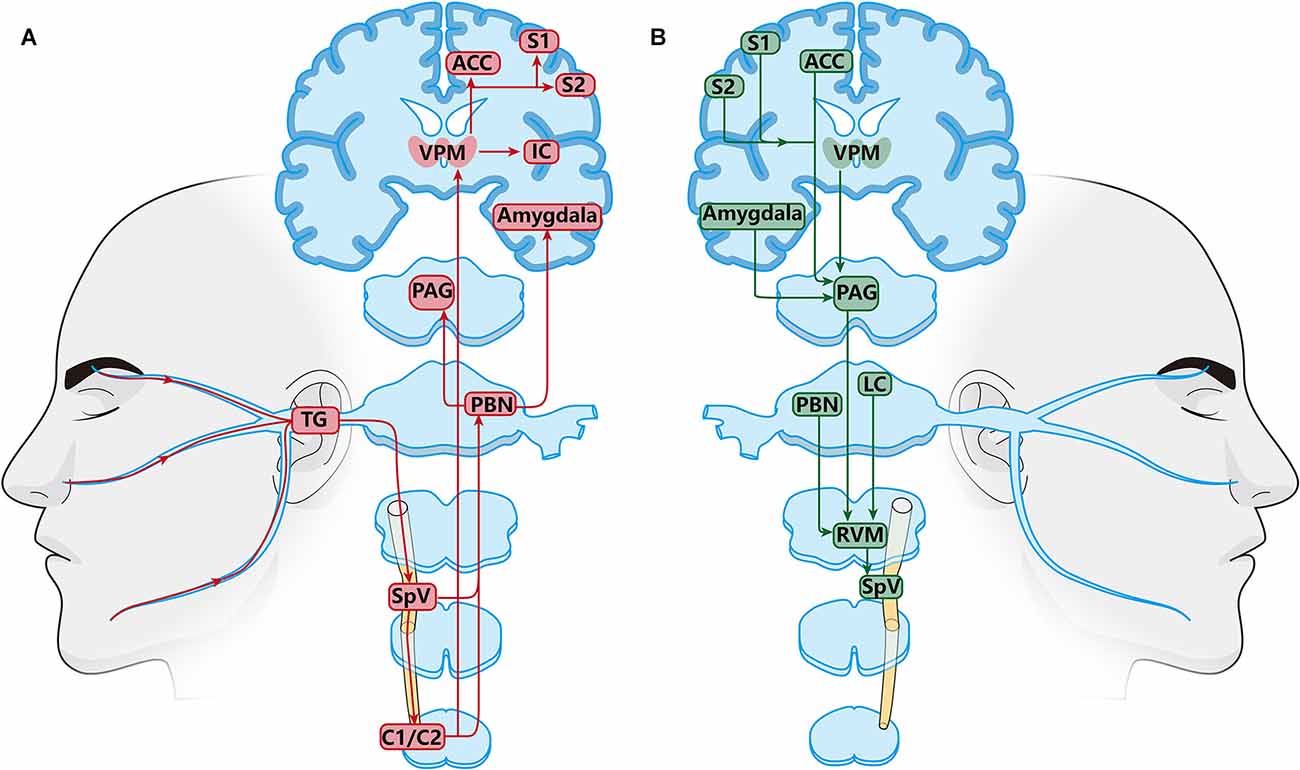
Figure 1. Schematic diagram of the orofacial pain modulation circuits. (A) Ascending nociceptive modulatory pathway. Neurons in the posteromedial thalamic nucleus (PBN) and thalamus (VPM) are key relays in ascending transmitting pathway. PBN and VPM receive nociceptive information from the SpVi/c, Vc, C1/C2 and project upward to the amygdala, anterior cingulate cortex (ACC), insular cortex (IC), the primary somatosensory cortex (S1), and secondary somatosensory cortex (S2). (B) Descending pain modulatory pathways. Periaqueductal gray (PAG), and ventrolateral medulla (VPM) are the main relays in the descending pain regulation pathway. The PAG receives regulatory information from the amygdala, VPM, ACC, S1, and S2, and finally transmits it down to the SpV through the RVM. TheRVM receives direct inputs from the PAG, thalamus, parabrachial region, and locus coeruleus (LC).
Nociceptive stimulation from orofacial areas not only activates the ascending nociceptive pathway but also triggers the descending pain regulation system (Figure 1B; Ren and Dubner, 2011). The descending regulation system is biphasic, including descending inhibition and descending facilitation system (Finnerup et al., 2021). The key integration centers of the descending circuit are the PAG and the rostral ventromedial medulla (RVM; Heinricher, 2016; Guo et al., 2022). The PAG receives descending nociceptive information from the somatosensory cortex, anterior cingulate cortex, hypothalamus, amygdala, and the dorsal medial prefrontal cortex, and then projects to the RVM (Floyd et al., 2000; Li and Sheets, 2018; Liang et al., 2020; Yin et al., 2020). In addition to PAG, the RVM plays a major role in the endogenous modulation of nociceptive transmission in the trigeminal nociceptive pathways in the ascending and descending nervous system. The RVM receives descending projections from several higher brain centers such as the PAG, PBN, locus coeruleus (LC), and hypothalamus (Behbehani and Fields, 1979; Willis, 1985; Fields et al., 1995; Ren and Dubner, 2011; Chichorro et al., 2017; Chen and Heinricher, 2019; Tang et al., 2021). The RVM can play a two-way role in pain regulation, including pain inhibition, and promotion. Earlier studies have found that electrical stimulation of RVM promotes nociceptive response at a lower intensity, while higher intensity stimulation has an antinociceptive effect (Zhuo and Gebhart, 1992; Sivanesan et al., 2019).
The joint regulation of ascending and descending pathways together forms the nociceptive sensation. Under physiological conditions, the ascending pathway is in balance with the endogenous descending pathway (Mills et al., 2020). In addition, nerve injury and inflammation trigger changes in molecules, receptors, ion channels, and neuroplasticity in the neural circuits of the trigeminal nervous system, leading to the imbalance between ascending and descending pain regulation system, which may be the basis of pathological pain (Ren and Dubner, 2011). Among the various potential targets, NMDARs have been reported to play a crucial role in the peripheral and central sensitization contributing to chronic orofacial pain (Wang et al., 2006; Ren and Dubner, 2011; Zhang et al., 2022).
Characteristics of NMDARs
NMDAR, a type of ionotropic glutamate receptor, consists of four subunits [two NR1 subunits and two NR2 (NR2A-D) or NR3 (NR3A-B) subunits], which together form a central ion channel pore (Sanz-Clemente et al., 2013a; Hansen et al., 2018). Seven genes encode the NMDAR subunits: a single GRIN1 gene encodes the NR1 subunit including eight NR1 distinct isoforms four GRIN2 genes encode NR2A-D, and two GRIN3 genes encode NR3A-B (Hardingham and Bading, 2010; Sanz-Clemente et al., 2013a). The two NR1 subunits, the binding site of glycine orD-serine, are obligatory in all functional NMDARs (Fernandez-Montoya et al., 2017; Hansen et al., 2018; Wu and Tymianski, 2018). The functional consequences of the gating reaction mechanism of NMDARs are strongly dependent on the identity of the four types of NR2 subunits (NR2A-D), the binding site of glutamate (Monyer et al., 1992; Wu and Tymianski, 2018). However, subunit assembly and physiological roles of the glycine-binding NR3 subunits remain elusive (Cavara and Hollmann, 2008; Henson et al., 2010). The detailed structure of NMDAR subunits has been described in a previous review (Hansen et al., 2018). The NR1 subunits combined with either NR2A or NR2B are the most widely expressed NMDARs in the nervous system (Wu and Tymianski, 2018).
Notably, NMDARs have several unique properties, including a voltage-dependent block by Mg2+, high permeability to Ca2+, and the requirement for binding of two coagonists (glutamate and glycine/D-serine) for channel activation, which can distinguish them from other glutamate ionotropic receptors (Traynelis et al., 2010). It is well-known that excitatory synaptic transmission is mainly regulated by the pre- and post-synaptic NMDARs. NMDARs are permeable to Na+, K+, and Ca2+ to regulate the membrane potential (Sanz-Clemente et al., 2013a; Hansen et al., 2018). NMDAR pore is strongly blocked by extracellular Mg2+ at the resting potential state, which can be released by neural depolarization or corresponding NMDAR agonists, resulting in a massive influx of Ca2+ (Traynelis et al., 2010). Then, the Ca2+ influx through NMDARs serves as a trigger for intracellular cascade signal activation such as the activation of Ca/calmodulin-dependent protein kinases II (CaMKII; Lee et al., 2012), which dramatically modifies neuronal functional properties and synaptic plasticity including short-term or long-term changes in synaptic strength (Hansen et al., 2018). Under physiological conditions, presynaptic NMDARs are not functionally active in regulating neurotransmitter release. However, in opioid-induced hyperalgesia and chronic neuropathic pain conditions, presynaptic NMDARs become tonically active and are stimulated by endogenous glutamate (Deng M. et al., 2019).
NMDAR subtypes confer different gating and permeation properties to the channel. One important property of NR1 is reduced agonist potency (Traynelis et al., 1998). In addition, NR1 can alleviate the inhibition of NMDAR function by NR2B-selective antagonist (Durand et al., 1992; Yi et al., 2018). These findings demonstrate the interactions between NR1 and NR2 subunits. NR2A and NR2B have unique characteristics, NR2A-containing receptors have faster activation/inactivation kinetics and a higher channel opening probability than the receptors containing NR2B subunits (Erreger et al., 2005; Sanz-Clemente et al., 2013a). NR2C-containing receptors show relatively unique channel properties, including low conductance, open probability, and sensitivity to Mg2+ (Farrant et al., 1994). NR2D is characterized by its expression early in development and its extremely slow decay time (Deng M. C. et al., 2019). Unlike NR2 subunits, NR3 binds to glycine and not to glutamate (Henson et al., 2010). The NR3 subunits function mainly as modulatory subunits that can reduce the vulnerability to Mg2+ block and attenuate the Ca2+ permeability of NMDARs (Cavara et al., 2010). NMDARs containing exclusively NR1/NR3 subunits can act as excitatory glycine receptors, which are impermeable to calcium.
Interestingly, recent studies have confirmed that NMDAR is mechanically sensitive (Casado and Ascher, 1998; Belin et al., 2022). In the model of stretch-induced neuronal injury, the Mg2+ blockage of the NMDAR channel significantly decreased and the NMDAR current increased (Zhang et al., 1996). Subsequent studies found that artificial lipid bilayer stretching can lead to the same phenomenon, indicating that mechanical energy is sufficient to regulate the activity of the NMDAR channel and is related to the decrease of Mg2+ blockage (Kloda et al., 2007). In addition to mechanical sensitivity, the latest study reported that NMDAR-mediated calcium influx in the absence of glutamate by applying shear force in cultured astrocytes in vitro, which indicated that mechanical stimulation itself can activate NMDAR currents (Maneshi et al., 2017). The oral and maxillofacial region receives abundant mechanical stimulation, and the NMDAR located in the peripheral nerve fibers may be more or less exposed to changing mechanical forces, therefore, understanding the regulation of mechanical sensitivity on NMDARs may be helpful to provide improved methods for chronic orofacial pain treatment.
The Changes in The Expression and Activation of NMDARs in The Neural Circuits Are Associated with Orofacial Pain Conditions
NMDARs are widely expressed in the PNS and CNS, such as the TG, dorsal root ganglion (DRG), spinal cord, brainstem, hippocampus, cerebellum, cortex, etc. (Dedek and Hildebrand, 2022). Research on the expression of NMDARs in the TG has found that about one-third of TG neurons express functional NMDARs, and the main subunits include GluN1, GluN2A, and GluN2B subunits (Fernandez-Montoya et al., 2016). And 99% of ganglion cells express GluN1, about 30% of TG neurons contain GluN2B subunits, and GluN2A subunits are expressed between 16% and 80% in different studies (Guerrero-Toro et al., 2022). The changes in the expression of NMDARs in the neural circuits of PNS and CNS are related to chronic orofacial pain including orofacial neuropathic pain, craniofacial musculoskeletal pain, and inflammatory pain induced by nerve injury and inflammation of the facial area, TMJ, and masseter (Li Y.-L. et al., 2021; Kimura et al., 2022; Zhang et al., 2022). Evidence supports that NMDARs exist in the peripheral terminals of small and large diameter primary afferent fibers in orofacial tissues such as masseter muscle, face, and TMJ; activation of NMDARs on the peripheral nerve endings can contribute to inflammatory pain (Yu et al., 1996; Cairns et al., 2003; Ro, 2003; Park et al., 2011). Although the expression of NMDARs in the periphery is elevated during inflammation both in animals and humans, studies on the relationship between chronic orofacial neuropathic pain and changes in the expression of NMDARs in the peripheral terminals are still lacking. Recently, several previous studies have focused on the role of NMDARs in the TG in the development of chronic orofacial neuropathic and inflammatory pain. For example, the expression of NR1 in the TG (expressed in the TGNs and SGCs) is upregulated under the condition of orofacial ectopic pain induced by inferior alveolar nerve transection (IANX; Fu et al., 2021; Li Y.-L. et al., 2021). Besides, p-NR2B is significantly upregulated in the TG of mice under the inflammatory orofacial pain condition induced by complete Freund’s adjuvant (CFA) injection into the whisker pad and orofacial neuropathic pain condition induced by INAX (Zhang et al., 2022). Moreover, conditional knockout NR2B in the TG alleviates the mechanical allodynia induced by inflammation and IANX (Zhang et al., 2022).
In the CNS, the expression of NR1, IL-6, and NF-κB are elevated following TMJ inflammation (Wang et al., 2009). Intracisternal injection of an IL-6 antiserum or NF-κB inhibitor (PDTC) can prevent both the upregulation of NR1 in the ipsilateral SpVc and pain behavior (Wang et al., 2009). Moreover, intracisternal IL-6 administration in naïve rats induces the NR1 upregulation and pain behavior similar to that after TMJ inflammation (Wang et al., 2009). These results indicate that the upregulation of IL-6 and NF-κB after inflammation of the unilateral TMJ region is a critical regulatory mechanism for the expression of NR1 in the ipsilateral SpVc, which contributed to the development of TMJ pain behavior in rats. p-NR2B is also upregulated in the SpVc after IANX and CFA-induced facial inflammation (Zhang et al., 2022). Moreover, TMJ arthritis can increase the expression of mRNA for all NMDAR subunits and p-NR1 in the SpVc, which is involved in the activation of astrocytes, resulting in chronic orofacial pain (Wang et al., 2012a; Cavalcante et al., 2013).
NMDARs are also expressed in astrocytes. Studies of the last two decades have provided evidence for the expression of all major NMDAR subunitsin astrocytes and the subunit composition of the receptor showing variation with tissue preparation and physiological context (Schipke et al., 2001; Dzamba et al., 2013; Jimenez-Blasco et al., 2015). The biophysical and pharmacological properties of the astrocytic NMDARs strongly suggest that they have AN atri-heteromeric structure composed of GluN1, GluN2C/D, and GluN3 subunits (Palygin et al., 2011; Hogan-Cann and Anderson, 2016). Accordingly, these NMDARs have distinct functional characteristics, including weak susceptibility to channel Mg2+ blockade, even at negative restingmembrane potentials, and reduced Ca2+ permeability (Hogan-Cann and Anderson, 2016). Astrocytic NMDARs may also be involved in neuroinflammatory processes (Hogan-Cann and Anderson, 2016). Astrocytic NMDARs may contribute to morphological transformations characteristic of reactive astrogliosis (Ting et al., 2009), and may mediatet he release of proinflammatory cytokines (Gerard and Hansson, 2012). Compared to neuronal NMDARs, astroglial NMDARs have different pharmacological properties with higher affinity to UBP141, memantine, and D-AP5, and lower sensitivity to Mg2+ and ifenprodil which may be due to their incorporation of GluN2C or GluN2D subunits (Palygin et al., 2011). The other important finding of this study is the difference in the affinity of glial and neuronal NMDARs to memantine at physiological concentrations of Mg2+ (Palygin et al., 2011). These findings above may be veryuseful for elucidating the mechanisms of neuron-glia communications and the development of novel therapeutic agents specifically targeting glial signaling.
However, there is still little known about the changes in the expression of NMDARs in the higher central nucleus such as PAG and RVM under chronic orofacial pain. According to the studies about chronic pain occurring in the other region such as the arms, legs, and lumbar region, NR2B in ACC is upregulated (Wu et al., 2005). Notably, there is a study that has reported that NR2A and NR2D mRNAs in the contralateral thalamus show an increase in dental pulp inflammation and it can be reduced by MK-801 (an NMDAR antagonist) application (Kaneko et al., 2011). It is reasonable to speculate that the NMDARs in the ascending and descending nervous system of the CNS also play a vital role in chronic orofacial pain. In conclusion, the above findings demonstrate that NMDARs are an important mediator involved in the peripheral and central mechanisms of chronic orofacial pain.
The Regulation in The Expression and Activation of NMDARs
The functional status of NMDARs is closely related to pain. Current studies have found that NMDARs are directly or indirectly regulated by a variety of ions, amino acid neurotransmitters, protein kinases, and scaffold proteins (Figure 2; Bereiter et al., 2000; Li X. et al., 2017; Zhang et al., 2018; Deng et al., 2020). These intermolecular interactions regulate the activity, membrane transport, localization, and signal transduction of NMDARs, thereby affecting neuronal excitability and pain.
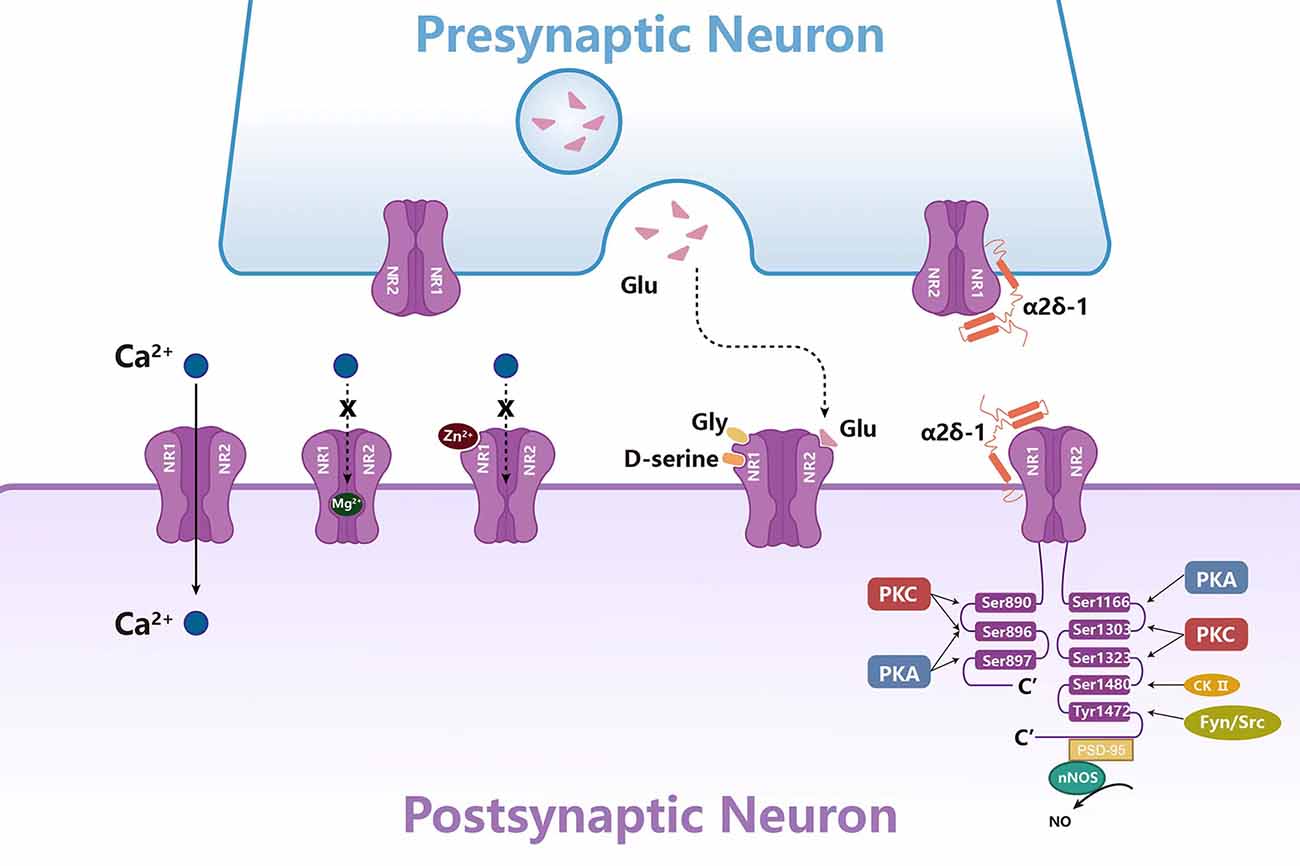
Figure 2. Molecules in NMDAR regulation in the synaptic transmission. The activity of NMDARs is regulated by a variety of ions, amino acid neurotransmitters, protein kinases, and scaffold proteins. Mg2+ and Zn2+ play an antagonistic role by blocking the channel of NMDARs. Gly, Glu, and D-serine can activate NMDAR together. Protein kinases such as PKC, PKA, CK-II, Fyn, and Src can phosphorylate NR1 or NR2. Scaffold proteins α2δ-1 and PSD-95 regulate NMDAR activity by directly binding to NMDARs.
Regulation of NMDAR function by extracellular ions
The functional state of NMDARs in the central nervous system is mainly regulated by Mg2+. As a physiological antagonist of NMDARs, Mg2+ can block the calcium influx of the channel and maintain the resting membrane potential (Kulik et al., 2021). The depolarization process removes the Mg2+-induced blockade of NMDARs in a voltage-dependent manner, resulting in hyperexcitability and consequently a sustained state of depolarization and increased synaptic strength (Johnson and Ascher, 1990). And oral administration of MgCl2 dissolved in mineral water inhibits expression of NR2B mRNA and p-NR1 and increases expression of NR3 mRNA in the SpVc, while Mg2+ deficiency increases expression of both NR1 and NR3 mRNAs and p-NR1 in the SpVc, contributing to the orofacial pain with TMJ arthritis (Cavalcante et al., 2013). Similarly, Mg2+ deficient rats develop mechanical hyperalgesia, which is reversed by NMDAR antagonists (Begon et al., 2001). Preclinical studies have found that the combination of Mg2+ and opioids can relieve neuropathic pain in diabetic rats by reducing the level of PKA in the PAG and increasing opioid receptor activity, indicating that Mg2+ can be used as a supplement for the treatment of pain (Rondon et al., 2010; Kulik et al., 2021). In addition to Mg2+, in vitro studies on mouse hippocampal neurons have confirmed that Zn2+ can selectively block NMDAR-mediated central neuron excitation (Westbrook and Mayer, 1987; Choi and Lipton, 1999; Morabito et al., 2022). Zn2+ can inhibit NMDAR by high affinity, voltage-independent, and low affinity, voltage-dependent (Fayyazuddin et al., 2000; Rachline et al., 2005). Nozaki et al. have found that the blocking effect of Zn2+ disappeared in the mice with NR2A knockout in hippocampus and spinal cord, resulting in thermal hyperalgesia (Nozaki et al., 2011). In addition, the analgesic effect of exogenous Zn2+ on inflammatory and pathological pain disappeared in NR2A knockout mice. Therefore, the interaction of Zn2+-NR2A may be one of the molecular bases for the occurrence and development of pain (SafiehGarabedian et al., 1996; Liu et al., 1999; Abdelrahman and Hackshaw, 2021).
Amino acids and NMDAR activation
NMDARs require co-activation of glutamate and glycine/D-serine: glutamate binds to the NR2 subunit and glycine/D-serine binds to the NR1 subunit to activate this channel (Chou et al., 2020). Glutamate and glycine can trigger conformational changes in the NMDAR ligand-binding domain, resulting in the opening of NMDAR channel pores, allowing calcium influx and depolarizing membrane potential, and mediating neuronal excitation (Yu and Lau, 2018). Besides, serine is also considered to be the endogenous ligand of the glycine site of the NMDAR. It is found that activated astrocytes may release endogenous D-serine to activate NMDAR, resulting in pain hypersensitivity (Miraucourt et al., 2011). Exogenous D-serine increases the phosphorylation of spinal cord PKC-dependent NR1 in Ser896 and promotes NMDAR-mediated mechanical hyperalgesia after nerve injury, which was alleviated by pretreatment with PKC inhibitors (Choi et al., 2017). In addition to directly regulating pain, further studies have confirmed that endogenous D-serine in rat rostral anterior cingulate cortex can also regulate pain-related negative emotion mediated by NMDAR (Ren et al., 2006). Therefore, reducing D-serine release or selectively inhibiting the glycine site of NMDAR may prevent emotional disturbance caused by chronic pain.
Phosphorylation of NMDARs
It has been reported that NMDAR phosphorylation is observed in both neuropathic and inflammatory pain (Pan et al., 2022; Zhang et al., 2022). NMDARs can be phosphorylated at different sites by a variety of kinases. It has been confirmed that the NR1 subunits can be phosphorylated by PKC at Ser890 and Ser896, and by PKA at Ser896 or Ser897 site (Tingley et al., 1993). In addition to the NR1 subunits, NR2 subunits can also be phosphorylated. The C-terminals of NR2A and NR2B are considered to be the main target of protein-protein interaction and phosphorylation, which contains multiple amino acid sites and can be phosphorylated by PKA, PKC, casein kinase II (CK2), Src, and Fyn tyrosine kinases (Tang et al., 2001; Cull-Candy and Leszkiewicz, 2004; Groc et al., 2009; Zhang et al., 2022). PKA can phosphorylate NR2B at Ser1166, which affects Ca2+ permeability in the spinal cord (Murphy et al., 2014). Notably, it is reported that subcutaneous administration of IL-1β in the vibrissa pad of rats induces orofacial mechanical allodynia by sensitizing peripheral NMDARs through PKA-mediated signaling in the large diameter primary afferent nerve fibers (Kim M. J. et al., 2014). On the other hand, activation of PKC can phosphorylate Ser1303 and Ser1323 at the C-terminal of NR2B (Liao et al., 2001), which potentiate the NMDAR currents by increasing the probability of channel openings and by reducing the voltage-dependent Mg2+ block of NMDAR channels in the isolated TGNs (Chen and Huang, 1992). CK2 is a serine/threonine protein kinase, which can mediate the phosphorylation of NR2B at Ser1480, drive NR2B endocytosis, reduce synaptic NR2B, and increase synaptic NR2A (Ye et al., 2012; Sanz-Clemente et al., 2013b; Chen et al., 2014). CK2 inhibitors can block the increase of NMDAR current in spinal dorsal horn neurons induced by nerve injury in rats, and inhibition of CK2 or CK2 gene knockout at the spinal cord level can significantly reverse the hyperalgesia caused by nerve injury (Chen et al., 2014). Src and Fyn can promote the phosphorylation of NR2B in the spinal dorsal horn at Tyr1472, resulting in up-regulation of NMDAR activity and membrane localization (Yang et al., 2011; Lai et al., 2016). Subsequent studies found that inhibition of Src activity can reduce Src-mediated NR2B phosphorylation, reduce postsynaptic density NR2B accumulation, and ultimately improve inflammatory pain (Guo et al., 2002; Suo et al., 2013). Similarly, after neuropathic injury and intrathecal activation of Fyn, the phosphorylation of NR2B in the spinal dorsal horn increased at Tyr1472 and led to hyperalgesia, which could be blocked by pre-inhibition of Fyn (Hildebrand et al., 2016; Nie et al., 2021). The membrane translocation of NMDARs is regulated by PKA and PKC phosphorylation. When PKA and PKC phosphorylate Ser897 and Ser896, respectively, the expression of NMDARs in the membrane increases (Scott et al., 2003). In summary, in the CNS, NMDAR phosphorylation strengthens the interaction between NMDAR and the postsynaptic density, which plays an essential role in the induction of long-term potentiation (LTP), a representative form of synaptic plasticity under chronic neuropathic pain conditions (Kronschlager et al., 2016).
Protein interaction and NMDAR activation
Recent studies have found that some intracellular proteins may be involved in NMDAR transport, synaptic localization, and activity regulation through interaction with NMDARs. Postsynaptic density protein-95 (PSD-95) is a scaffold protein that can cause a series of downstream molecular events that can cause pain by interacting with NMDARs (Chen et al., 2021a,b). It is generally believed that PSD-95 couples nNOS to NMDARs through the PDZ domain to form an NMDAR-PSD95-nNOS complex, which produces NO and leads to central sensitization (Lee et al., 2015; Li J. et al., 2021). NOS1 adaptor protein (NOS1AP) can bind to the N-terminal region of nNOS containing PDZ (Jaffrey et al., 1998). Current studies have found that NOS1AP mediates the inhibition or activation of NMDAR-driven nNOS (Li et al., 2013; Weber et al., 2014). The activation of NMDARs increases the connection between nNOS and NOS1AP, while the destruction of nNOS-NOS1AP interaction can inhibit the behavioral hypersensitivity in the model of neuropathic pain induced by chemotherapeutic drugs and trauma (Lee et al., 2018). Several experiments have confirmed that TAT-NR2B9c, IC87201, and ZL006 can block the central sensitization triggered by NMDARs by disrupting the interaction between NMDARs and PSD95, which can produce antinociceptive effects in both inflammatory and neuropathic pain models (Pedersen and Gjerstad, 2008; Chen et al., 2014; Peltoniemi et al., 2016; Li S. et al., 2017). α2δ-1, a subunit of voltage-activated calcium channel, is another powerful regulator of NMDARs. It promotes neuropathic pain by forming a complex with NMDARs and promoting the synaptic transport of NMDARs (Chen et al., 2018). And gabapentin can relieve pain hypersensitivity by inhibiting the synaptic transmission of the α2δ-1-NMDAR complex (Huang et al., 2020; Fu et al., 2021). It has been proved that gabapentin can be used to treat neuropathic pain and epilepsy (Moore et al., 2014). Similarly, NR1 can also physically and functionally interact with α2δ-1 in the TGNs and activated SGCs after trigeminal nerve injury, inhibition of the formation of α2δ-1-NR1 complex can alleviate mechanical allodynia in the whisker pad of rats treated with IANX (Fu et al., 2021). Furthermore, NR1 and NR2B form protein-protein complexes with TRPV1 in the TG, contributing to the mechanical hyperalgesia induced by the injection of NMDA into the masseter muscle via CaMKII and PKC signaling cascades (Lee et al., 2012). NMDAR-protein interaction is essential for central sensitization and the development and maintenance of pain, thus, interference with the protein-protein interaction downstream of NMDARs provides a potential target for the development of anti-allodynic drugs. Several experiments have confirmed that interfering peptides such as TAT-NR2B9c and TAT-GESV can produce anti-pain effects by disrupting the interaction between PSD95-nNOS and nNOS-NOS1AP, respectively, without causing dyskinesia or changing the normal nociceptive sensation (Pedersen and Gjerstad, 2008; Chen et al., 2014; Peltoniemi et al., 2016; Li S. et al., 2017). Some small molecular compounds such as IC87201 and ZL006 can destroy the interaction between NMDARs and PSD95 but have no significant effect on the interaction between PSD95 and other proteins (Tochio et al., 2000; Florio et al., 2009). Similarly, gabapentin and α2δ-1 TAT peptide can relieve pain allergy by blocking the binding of α2δ-1 to NMDARs (Moore et al., 2014; Huang et al., 2020; Fu et al., 2021). Blocking the protein-protein interaction downstream of NMDARs is a valuable therapeutic strategy, which can inhibit neuropathic pain without affecting the normal function of NMDARs (Zhou et al., 2010).
The Functional Role and Mechanisms of NMDARs in Regulating Peripheral Sensitization of Chronic Orofacial Pain
Evidence indicates subcutaneous injection of glutamate mechanically sensitizes rat facial cutaneous mechanoreceptors through activation of peripheral NMDARs (Gazerani et al., 2010). The subcutaneous injection of PPPA, a competitive NR2A antagonist, and Ro 25-6981, a selective NR2B antagonist, can inhibit the nociceptive scratching behavior produced by the injection of formalin (Park et al., 2011). These results suggest NR2 subunits participate in the transmission of nociceptive information in peripheral tissues, indicating peripheral NMDAR antagonists may be considered for chronic orofacial pain. Besides, it is reported that stimulation of peripheral NMDARs also causes the release of the neuropeptides, such as substance P (SP) and calcitonin gene-related peptide (CGRP), from peripheral nerve terminals (McRoberts et al., 2001). These neuropeptides further contribute to the initiation and development of chronic orofacial pain induced by nerve injury and inflammation. In addition, masseter injection of MK801, an NMDA receptor antagonist, attenuates nocifensive behaviors in lightly anesthetized rats and decreases c-fos expression in the SpVc induced by the masseteric injection of mustard oil (Ro, 2003; Ro et al., 2004), which demonstrates that the activation of NMDARs on the peripheral terminals is involved in the neuroplastic changes in the synaptic transmission in the SpVc under orofacial inflammatory conditions. Taken together, these results support the suggestion that the orofacial allodynia or hyperalgesia due to increased peripheral terminal NMDARs mediates the release of neuropeptides under the condition of nerve injury and inflammation.
Peripheral sensitization in the TG is a key mechanism underlying chronic orofacial pain induced by trigeminal nerve injury or orofacial inflammation. NMDARs have been reported to be involved in the peripheral sensitization in the TG by triggering the activation of intracellular signaling pathways and the communication between neuronal and SGCs (Figure 3; Fu et al., 2021; Li Y.-L. et al., 2021; Zhang et al., 2022). Following a chronic constriction injury of the inferior orbital nerve (CCI-ION), glutamate expression increases in the TG (Kung et al., 2013), further activating the NMDARs. In addition, glutamate increase occurs in the somata of neurons with injured axons as well as in the somata of neighboring uninjured neurons (Kung et al., 2013), indicating a cross-talk among TGNs. Besides, the p-NR2B expression in the TG is elevated following IANX or orofacial inflammation, indicating NR2B-containing NMDARs are activated under chronic orofacial pain conditions (Zhang et al., 2022). Activated NR2B can trigger the activation of CaMKII through the Ca2+ influx via autophosphorylation of the inhibitory domain in Thr286 to facilitate the activation of the cAMP-ERK-CREB signal pathway, resulting in the upregulation of transcriptional regulators and/or pain-related proteins, thus contributing to the development and maintenance of orofacial allodynia (Zhang et al., 2022). Besides, NR1 subunits also contribute to orofacial ectopic pain induced by IANX through activation of Src (a nonreceptor-type protein tyrosine kinase), further resulting in the upregulated expression of pannexin1 (Panx1), a subtype of pannexin family that is permeable to molecules smaller than 1 kDa, such as ions and neurotransmitters, for example, the algogenic ATP molecules (Fu et al., 2021; Li Y.-L. et al., 2021). Moreover, inhibition of Panx1 can in turn suppress the protein expression of NR1 due to ATP release through the Panx1 channel, which demonstrates a positive feedback loop on the expression of NR1 after trigeminal nerve injury (Li Y.-L. et al., 2021). Under masseter inflammation conditions, activation of NMDARs in the TG results in the phosphorylation of TRPV1, primarily at serine residues through the activation of PKC and CaMKII but not PKA pathways in rat TGNs (Lee et al., 2012). Meanwhile, NMDARs and TRPV1 interactions are essential for the development of mechanical hyperalgesia in the masseter muscle (Lee et al., 2012).
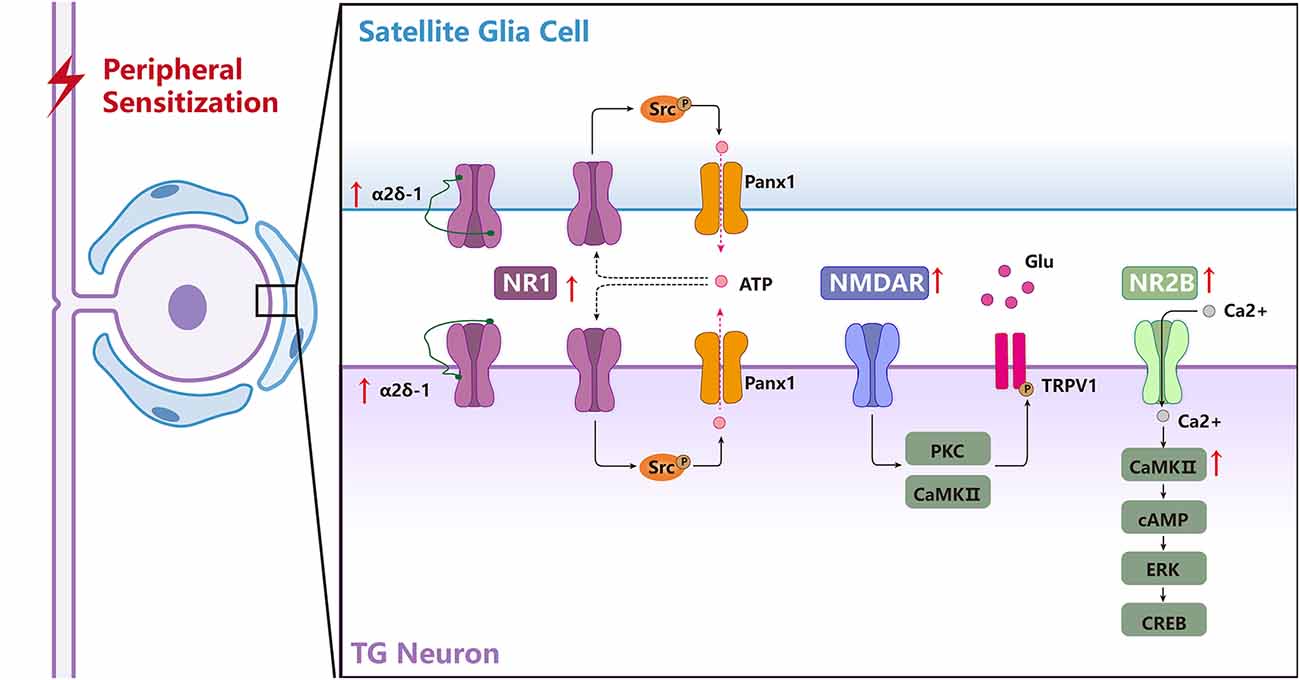
Figure 3. The biological mechanisms of NMDARs in the TG underlying regulating peripheral sensitization of orofacial pain. NMDARs are involved in the activation of chronic orofacial pain-related intracellular signaling pathways and the communication among the neuronal and satellite glial cells (SGCs) in the TG. NR1 forms a complex with α2δ-1 in the TGNeurons (TGNs) and activates SGCs, and the α2δ-1-NR1 complex is upregulated after trigeminal nerve injury, α2δ-1 may regulate NR1 expression through PKC. NR1-Src-Panx1 signal pathway distributes in the TGNs and SGCs contributes to orofacial neuropathic pain following trigeminal nerve injury. Activation of NMDARs results in phosphorylation of TRPV1, primarily at serine residues through the activation of PKC and CaMKII pathways in rat trigeminal sensory neurons, and NMDAR and TRPV1 interactions are essential for the development of mechanical hyperalgesia in the masseter muscle after inflammation. NR2B/CaMKII acts as an upstream cascade to facilitate cAMP production and ERK-CREB activation in the TG after inflammatory and nerve injury.
The Functional Role and Mechanisms of NMDARs in Central Sensitization
Nerve signals arising from sites of tissue or nerve injury lead to long-term increases in excitability and plasticity in the central nervous system often referred to as central sensitization (Ren and Dubner, 2011). NMDARs are well-known to be required for triggering central sensitization under chronic pain conditions by removal of the voltage-dependent magnesium block and phosphorylation (Ren and Dubner, 2011). In addition, it is well-known that the excitatory synaptic transmission is mainly regulated by the pre and postsynaptic NMDARs after being activated by glutamate. In the CNS, activation of NMDARs initiates intracellular cascades through calcium influx and activation of protein kinases, which in turn modulates cell membrane excitability, synaptic hyperexcitability, long-term plasticity, and enhances nociceptive transmission (Figure 4; Woolf and Mannion, 1999).
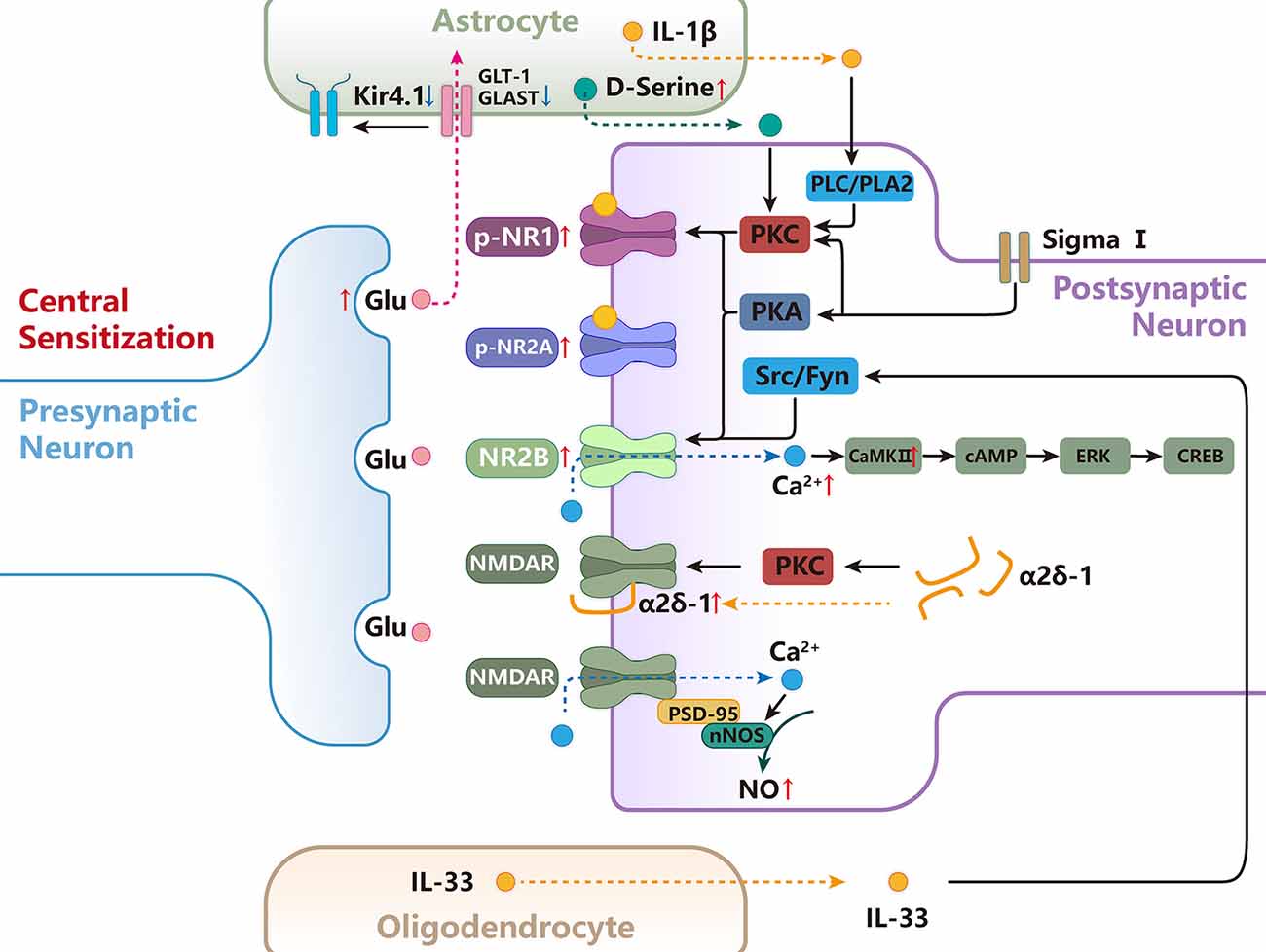
Figure 4. The functional role and mechanisms of NMDARs in regulating central sensitization of orofacial pain. D-Serine released from astrocytes increases PKC-dependent NR1 phosphorylation, contributing to central sensitization. Activation of the sigma-1 receptor enhances NMDA-induced pain via PKC- and PKA-dependent phosphorylation of the NR1 subunit. Src and Fyn can promote the phosphorylation of GluN2B at Tyr1472, resulting in up-regulation of NMDAR activity and membrane localization.NR2B/CaMKII acts as an upstream cascade to facilitate cAMP production and ERK-CREB activation after inflammatory and nerve injury in the SpVc. NMDAR forms a heteromeric complex with α2δ-1 in the spinal cord, and the α2δ-1-NMDAR interaction promotes surface trafficking and synaptic targeting of NMDAR. α2δ-1 can also indirectly regulate NMDAR through PKC.PSD-95 couples nNOS to NMDAR to form an NMDAR-PSD95-nNOS complex, which produces NO and leads to central sensitization. Astrocytes are responsible for the majority of glutamate uptake, which occurs mainly through two transporters, GLT-1 and GLAST, and Kir4.1 functions in the facilitation of astrocytic glutamate uptake. IL-33 act on the postsynaptic neuron to promote the phosphorylation of NR2B through activation of Fyn. the intracellular signal pathway of PLC, PLA2, and subsequent PKC activation is involved in the astrocyte-derived IL-1β-induced NR1 phosphorylation.
It is reported that presynaptic NMDAR activation can regulate the release of downstream neurotransmitters, thereby affecting hyperalgesia (Bardoni et al., 2004). Presynaptic NMDARs are known to facilitate the release of glutamate, SP, and BDNF from C-type primary nociceptive fibers (Larsson and Broman, 2011; Bardoni, 2013). Liu et al. (1997) found that NMDAR activation can lead to persistent pain by promoting the release of SP and glutamate. The changes in ions and neurotransmitters induced by NMDAR activation can change the excitability and synaptic plasticity of neurons (Ikeda et al., 2003). Repeated stimulation of peripheral C fibers can induce a gradually increased response of WDR neurons, resulting in overexcitation, a phenomenon known as windup (Mendell and Wall, 1965; Woda et al., 2004). Studies have found that windup actually depends on the activation of NMDARs (Woda et al., 2004; Dedek and Hildebrand, 2022). Under pathological conditions, the dysfunction of NMDAR-dependent windup inhibition may contribute to the central sensitization of the pain system (Mendell, 2022). Because of some characteristics that windup can induce central sensitization, it has been used as an experimental model to explore the mechanism of central sensitization (Coste et al., 2008). Long-term potentiation (LTP) was another important form of synaptic plasticity and it was considered a potential mechanism of hyperalgesia (Li X.-H. et al., 2021). Activation of NMDARs can further mediate LTP in neurons and induce hyperalgesia. It is reported that NMDAR-dependent LTP is widely found in synapses of the spinal dorsal horn and cortex (Zhuo, 2014; Li et al., 2019). Animal studies have confirmed that the induction of LTP in vivo requires the activation of NMDAR (Liu and Sandkühler, 1995; Kim et al., 2015). Besides, inhibition of NMDAR-dependent LTP has an analgesic effect (Benrath et al., 2005; Li and Baccei, 2016).
The central sensitization involved NMDARs in the spinal trigeminal nucleus
NMDARs have been found to play a role in regulating pain in several key relays in the ascending pain regulation pathway. Currently, the role and mechanisms of NMDARs related to chronic orofacial pain are mainly focused on the Vi/Vc transition zone (SpVi/c) and SpVc. The intracisternal administration of NR2A or NR2B subunit antagonists inhibits the nociceptive behavior produced by the injection of formalin into the vibrissa pads of rats (Yang et al., 2010). Similar to the role of NMDARs in the peripheral sensitization in the TG, it is also reported that NR2B/CaMKII act as an upstream cascade to facilitate cAMP production and ERK-CREB activation in the SpVc after inflammatory and nerve injury which increase neuronal excitability, contributing to the development and maintenance of central sensitization (Zhang et al., 2022). It has been reported that oligodendrocyte-derived IL-33 can act on the receptor suppression of tumorigenicity 2 on the postsynaptic neuron to promote the phosphorylation of synaptosomal NR2B through activation of Fyn and potentiate NR2B-containing NMDAR-mediated synaptic currents in the SpVc, which facilitates orofacial neuropathic pain induced by ION injury (Kimura et al., 2022). These findings demonstrate that NMDARs are involved in the interaction between oligodendrocyte-neuron interactions, resulting in the development of orofacial neuropathic pain. In addition, D-serine, an endogenous and mandatory co-agonist for the NMDAR glycine site in mammalian brains, is predominantly expressed in astrocytes and increases in the SpVc after CCI-ION (Wolosker, 2006; Dieb and Hafidi, 2013). These findings demonstrate that increased astrocyte-derived D-serine is involved in the modulation of orofacial posttraumatic neuropathic pain via acting on the NMDARs in the SpVc (Dieb and Hafidi, 2013).
Besides the SpVc, the NMDARs in the SpVi/c are also involved in the central sensitization related to chronic orofacial pain. Previous studies also have demonstrated that astroglial activation within the SpVi/c occurred following masseter or tooth pulp inflammation, which modulates the NMDAR phosphorylation via IL-1β derived from astroglial cells (Guo et al., 2007). It is reported that injection of the NMDAR antagonist into the SpVi/c attenuates masseter inflammatory hyperalgesia (Wang et al., 2006). Shimizu et al. (2009a) have reported that microinjection of IL-1β into the SpVi/c produces NMDAR-dependent orofacial hyperalgesia in rats. Guo et al. (2007) have reported that incubation of IL-1β in the medullary slices induced a significant and dose-dependent increase in P-NR1 levels in theSpVi/c. Microinjection of IL-1β into the SpVi/c in vivo also produces an increase in P-ser896-NR1 levels, which can be blocked by chelerythrine, a PKC inhibitor (Guo et al., 2007). Moreover, 2APB, a membrane permeable IP3 receptor antagonist also blocks IL-1β-induced NR1 phosphorylation. In addition, the PLC inhibitor U73122 and PLA2 inhibitor AACOCF3 can block the effect of IL-1β on NMDAR phosphorylation in SpVi/c (Guo et al., 2007). IL-1R colocalizes with the NR1 subunit in SpVi/c neurons, and the IL-1β signaling facilitates NMDAR activity in neurons (Guo et al., 2007). These findings demonstrate that the intracellular signal pathway of PLC, PLA2, and subsequent PKC activation is involved in the IL-1β-induced NR1 phosphorylation in the SpVi/c following peripheral nerve injury and inflammation, contributing to orofacial hyperalgesia.
The central sensitization involved NMDARs in the brain
NMDAR-mediated pain is also found in advanced relays such as the ACC, forebrain, and medial prefrontal cortex (Wei et al., 2001; Medeiros et al., 2019). Wu et al. (2005) have demonstrated that the upregulation of NMDAR subunit NR2B in the ACC is related to inflammation-related persistent pain, and the local injection of antagonist in the ACC can significantly relieve pain hypersensitivity. Wei et al. (2001) found that mice with overexpression of NR2B in the forebrain showed stronger inflammation-related pain, the genetic modification of NMDAR in the forebrain can affect pain perception. In the experimental model of chronic neuropathic pain, Medeiros et al. (2019) have shown that blocking NMDAR in the prelimbic region of the medial prefrontal cortex can reduce chronic neuropathic pain while activating NMDAR can enhance neuropathic pain.
The cerebellar cortex consists of a molecular layer, Purkinje cell (PC) layer, and granule cell layer, which are mainly involved in molecular layer interneurons (MLIs), PC, granule cell (GC), and Golgi cell (Palay and Chan-Palay, 1974; Llinás, 2011). The various information coming from outside is transferred into the cerebellar cortex through the mossy fibers (MF)-GC-parallel fiber (PF) pathway and the climbing fibers (CF) pathway, which is involved in the control of sensory perception (Eccles et al., 1971; Palay and Chan-Palay, 1974; Bower and Woolston, 1983; Llinás, 2011). NMDARs are found on the membrane of GCs and MLIs, the terminal of PFs, and the postsynaptic membrane of CF-PC synapses (Glitsch and Marty, 1999; Casado et al., 2000). Electrophysiological recording in vivo demonstrates that pharmacologically blocking NMDARs abolish the facial stimulation evoked by GABAergic inhibitory components in the cerebellar cortical molecular layer (Xu et al., 2017). NR2A containing NMDARs contribute to the facial stimulation-evoked MF-GC synaptic transmission, suggesting that the NMDARs play an important role during the lateral sensory information synaptic transmission in the cerebellar granular layer in vivo in mice (Zhang et al., 2020). These findings suggest NMDARs in the cerebellar cortex may contribute to the initiation and development of chronic orofacial pain through mediating the synaptic transmission in the MF-GC-PF pathway and CF pathway, which are needed more preclinical studies to prove.
The role of NMDARs in the descending nervous system
Activation of descending pathways is also involved in hyperalgesia in animal models of inflammatory and neuropathic pain (Porreca et al., 2002; Vanegas and Schaible, 2004; Kim Y. S. et al., 2014). Ferrari et al. (2014) have found that pretreatment of PAG with NMDAR antagonists can block the analgesic effect of electrical stimulation of the primary motor cortex, suggesting that the descending inhibition pathway depends on the function of NMDAR in the PAG (Porreca et al., 2002; Wang et al., 2019). Excitotoxic lesions of the RVM lead to significant attenuation of behavioral hyperalgesia after masseter inflammation, suggesting that modulatory inputs from the RVM enhance the hyperalgesia/allodynia after masseter inflammation (Sugiyo et al., 2005). Fields et al. (1995) have found that there are three types of neurons in RVM, namely, ON cell, OFF cell, and neutral cells, in which ON cells promote nociceptive sensation, while OFF cells inhibit nociceptive sensation. Therefore, RVM can produce two-way regulation by activating ON cells and OFF cells respectively. All three types of RVM cells expressed excitatory amino acid receptors and responded to exogenous glutamate (Heinricher and Roychowdhury, 1997). Current studies have found that NMDAR can produce two opposite effects in RVM. Heinricher et al. (2001) have found that the injection of NMDAR antagonist AP-5 into RVM attenuates the activation of OFF cells and the antinociceptive effect of morphine, so systemic administration of morphine could activate off cells in RVM through an NMDA-mediated excitation process, resulting in analgesic effect. Subsequent studies by Xu et al. (2007) have confirmed that secondary hyperalgesia induced by mustard oil is caused by continuous activation of ON cells mediated by NMDA.
NMDARs Are Involved in The Chronic Orofacial Pain Associated with Psychological Stress and Depression
As psychological stress is closely related to the initiation and development of chronic orofacial pain (Huang et al., 2011; Okamoto et al., 2012), several studies focus on the role and mechanism of NDMARs in the masseter hyperalgesia induced by stress (Lin et al., 2019; Li et al., 2020). It is reported that activated astrocytes in the SpVc evoked by chronic restraint stress can release excessive IL-1β, followed by IL-1β binding to its specific receptor and promoting NMDAR phosphorylation in the neurons, ultimately contributing to masseter hyperalgesia (Zhao et al., 2015). Besides, activation of NR2B containing NMDARs also plays a key role in the masseter hyperalgesia induced by psychological stress though promoting phosphorylation of astrocytic JNK in the SpVc (Lin et al., 2019). These findings show that NMDARs are involved in the crosstalk between neurons and astrocytes, contributing to the pain hypersensitivity in the masseter induced by stress. Moreover, NMDAR-dependent central sensitization in the spinal dorsal horn and 5-HT-dependent descending facilitation also contribute to stress-induced spreading hyperalgesia in rats with orofacial inflammation (Li et al., 2020). Notably, increased NR1 receptors in the spinal dorsal horn mediate 5-HT-dependent descending pain facilitation from the RVM by regulating the expression of spinal 5-HT3 receptors, which contribute to behavioral hypersensitivity via a reciprocal neuron-glial signaling cascade (Guo et al., 2014). Therefore, increased NMDARs in the SpVc may also regulate 5-HT-dependent descending facilitation from RVM through regulating 5-HT3 receptors and contribute to chronic orofacial pain via cross-talk between neuron-glial cells. In addition, there is a reciprocal relationship between depressive behavior and TMJ inflammation-induced mechanical hyperalgesia. The expression of the MT1 receptor (melatonin receptor) is downregulated, whereas the expression of the NR1 subunit is upregulated in the SpVc of WKY rats with genetically predisposed depressive behavior. Intracisternal administration of 6-chloromelatonin (a melatonin analog) improves depressive behavior as well as mechanical hyperalgesia in WKY rats by preventing the upregulation of NR1 expression (Wang et al., 2012b). In summary, NMDARs play a vital role in the chronic orofacial pain associated with psychological stress and depression.
The Sex-Related Difference in The Role and Mechanisms of NMDARs in Males and Females Under Chronic Orofacial Pain
It is widely known that there are sex differences in pain perception. For example, women with TMD reported lower experimental pain threshold and heat-pain tolerance limits along with greater severity of orofacial pain than their male counterparts (Ohrbach et al., 2011). However, it is still not fully clear, at present, what mechanisms may account for the sex-related difference in chronic orofacial pain. Some studies also have reported the effects of sex on the role and mechanism of NMDARs in chronic orofacial pain. There is a sex-related difference in the sensitivity of cutaneous mechanoreceptors to glutamate, a 4.5-fold lower EC50 for glutamate-induced mechanical sensitization in females as compared to male rats (Gazerani et al., 2010). Glutamate-induced cutaneous afferent fiber mechanical sensitization is significantly attenuated by co-administration of the NMDAR antagonist APV, which indicates that this effect is mediated, in part, through the activation of peripheral NMDARs (Gazerani et al., 2010). The NMDA-evoked afferent discharge is significantly greater in female than in male rats, which is resulted from an estrogen-mediated increase in the expression of peripheral NMDARs by masseter ganglion neurons in female rats (Dong et al., 2007). In addition, it is reported that estrogen can increase NMDAR-mediated neuronal responses through an effect on the NR2B subunit (Woolley et al., 1997; Foy et al., 1999). The sex-related difference in NMDAR expression and neuronal response in the masseter afferent fibers contribute to the greater sensitivity of NGF-induced mechanical sensitization to peripheral NMDAR antagonism in females than in male rats (Dong et al., 2007; Wong et al., 2014). However, no significant sex-related difference in the number of facial skin afferent fibers that expressed the NR2B subunit of the NMDAR was noted in the present study. The sex-related difference is also found in the mechanical sensitization induced by intramuscular injections of NGF into the masseter muscle in women and men. The mechanical sensitization is more pronounced in women than in men following injection of NGF into the masseter muscle (Svensson et al., 2003, 2008). Therefore, when we study the role and mechanisms of NMDARs related to chronic orofacial pain, sex differences should be taken into account. Furthermore, more studies are needed to investigate the mechanisms underlying the sex differences in the role of NMDARs in chronic orofacial pain.
Effects of NMDAR Antagonists in The Management of Pain
As activation of NMDARs plays an important role in the initiation and development of chronic pain, especially neuropathic pain, NMDAR antagonists have garnered increasing attention over recent years as an adjunctive/alternative pain treatment modality (Collins et al., 2010; Kamp et al., 2019; Kreutzwiser and Tawfic, 2019). According to its action mode, NMDAR antagonists mainly include competitive antagonists, channel blockers, allosteric regulators, and uncoupling agents (Traynelis et al., 2010; Song et al., 2018; Hansen et al., 2021). Competitive antagonists inhibit the response of NMDARs to all agonists by competing with agonists or ligands that activate receptors. Typical competitive antagonists include (R)-AP5, CGP-78608, 7-CKA, and 5-dichlorouric acid (DCKA), etc. (Davies et al., 1981, 1982; Cai, 2006). However, due to the high conservation of GluN2 binding sites, competitive antagonists lack subunit selectivity (Furukawa et al., 2005; Kinarsky et al., 2005). Channel blockers are macromolecules that can combine with the channels of ion channels to block ion flow, such as ketamine, memantine, phencyclidine, and MK-801 (Song et al., 2018). Because the channel pores of NMDAR are relatively conservative, channel blockers only show moderate subunit selectivity for GluN2 subtypes (Dravid et al., 2007). However, partial capture blockers (trapping blockers) such as MK-801 and ketamine can preferentially inhibit the high activity of NMDAR, which will be beneficial to treatment, because the physiological level of NMDAR activity will not be affected (Chen and Lipton, 2005, 2006). Compared with antagonists and channel blockers, allosteric regulators have many advantages. First of all, allosteric regulators have two-way regulation, including positive and negative allosteric regulators (Yang and Svensson, 2008). Some allosteric regulators can bind to conservative low NMDAR sites, which is beneficial to the development of drugs with subunit selectivity (Luessen and Conn, 2022). Typical compounds include GluN2A selective negative allosteric regulator STCN-201, GluN1/2B receptor regulator isopropylphenidil, GluN2C/D selective regulator QNZ-46, and so on (Zhu et al., 2020). In addition, allosteric regulators do not affect the binding of orthomorphic ligands, allowing them to have synergistic or antagonistic effects with ligands, resulting in a variety of effects (Zhu and Paoletti, 2015). Unlike the previously mentioned strategy of directly acting on NMDARs, uncouplers regulate the signal cascade that mediates pain by interfering with proteins interacting downstream of NMDARs (Courtney et al., 2014; Sun et al., 2021). At present, many studies have confirmed that the NMDAR-PSD95-nNOS complex downstream of NMDARs is one of the important pathways that mediate pain sensitization (Chen et al., 2022; Huang et al., 2022). The formation of the PSD95-nNOS complex can be blocked by interfering peptides such as TAT-NR2B9c and TAT-GESV (Lai et al., 2014; Lee et al., 2018). Since NMDAR activity is not directly blocked, interfering peptides can inhibit hypersensitivity in pathological pain without changing the normal nociceptive sensation and avoid the side effects related to NMDAR antagonism (Aarts et al., 2002). However, compared with conventional chemical drugs, interfering peptides still have some problems, such as limited administration route, high manufacturing cost, and immunogenicity (Lai and Wang, 2010). At present, it has been found that small molecular compounds ZL006 and IC87201 can effectively destroy the effect of PSD95-nNOS interaction to simulate interfering peptides (Tochio et al., 2000; Lee et al., 2015). In addition, ZL006 can easily cross the blood-brain barrier during intravenous administration, making it a promising drug (Zhou et al., 2010; Chen et al., 2013).
Traditionally, NMDAR antagonists, such as ketamine, memantine, dextromethorphan, Mg2+, etc. have been used in the management of pain. Kreutzwiser and Tawfic (2019) have reviewed the pharmacotherapeutic profiles of four NMDAR antagonists, including ketamine, memantine, dextromethorphan, and Mg2+, and concluded that ketamine is the most studied NMDAR antagonist used to treat pain and possesses the most clinical utility (Kamp et al., 2019). Despite animal data suggesting some NMDAR antagonists are promising drugs for alleviating the development of chronic pain, we focused on their applications in humans and here we provide an overview of the NMDAR antagonists and the evidence for their use in various pain management settings (Table 1). Kamp et al. (2019) have updated the pharmacokinetic and pharmacodynamic considerations for ketamine in the treatment of chronic neuropathic pain in recent literature, which also supports that ketamine is a viable alternative in refractory neuropathic pain [e.g., post-herpetic neuralgia, complex regional pain syndrome (CRPS), central neuropathic pain, traumatic peripheral nerve pain, trigeminal neuropathic pain, spinal cord injury, phantom limb pain, painful limb ischemia, sickle cell pain, temporomandibular pain, fibromyalgia, migraine, and whiplash pain; Kamp et al., 2019; Kreutzwiser and Tawfic, 2019; Shiiba et al., 2021]. Moreover, Collins et al. (2010) have reviewed NMDAR antagonists (including ketamine, memantine, amantadine, dextromethorphan, MgSO4, MgCl2, Riluzole, GV 196771, and CNS5161 HCl ) for the treatment of neuropathic pain in clinical trials by meta-analysis. Their results demonstrate that no conclusions can yet be made about the efficacy of NMDAR antagonists on neuropathic pain, as positive and negative outcomes on pain relief are found. In addition, adverse events (including sedation, headache, dizziness, etc.) are also reported in the clinical trials for suppressing the physiological functions of NMDARs (Collins et al., 2010; Alviar et al., 2016). As the clinical trials reported in the available literature about the efficiency of NMDAR antagonists in chronic orofacial pain management is still limited, further large high-quality RCTs in clearly defined pain populations with chronic orofacial pain are required to support the firm conclusions on the role and safety of NMDAR antagonists in the management of chronic orofacial pain before widespread use.
In the aspect of new potential alternatives to NMDAR antagonists, a recent study has found botulinum toxin promotes orofacial antinociception by modulating TRPV1 and NMDARs in adult zebrafish (Rocha Barreto et al., 2022). Besides, the dietary constituent, resveratrol also has been reported to alleviate pain hypersensitivity by suppressing glutamatergic neurotransmission of the SpVcwide dynamic range neuron hyperexcitability via NMDARs under inflammatory and neuropathic conditions (Shimizu et al., 2009b; Wang et al., 2009; Takehana et al., 2017). These findings support botulinum toxin and resveratrol may be used as alternative medicines for the treatment of chronic orofacial pain. Notably, intravenous injection of bone marrow stromal cells (BMSCs) has shown potential to treat chronic pain including chronic orofacial pain (Huh et al., 2017; Guo et al., 2018). Application of BMSCs can attenuate pain hypersensitivity in the CCI-ION model (Guo et al., 2011), and reduce the amplitude and frequency of spontaneous excitatory postsynaptic currents in the SpVc of CCI-ION mice (Guo et al., 2016), suggesting BMSCs inhibit trigeminal neuronal hyperexcitability and primary afferent input by inhibiting the activation of NMDARs. In addition, NR2A tyrosine phosphorylation and PKCγ immunoreactivity in the RVM can be suppressed after BMSC application (Guo et al., 2016). Taken together, these findings indicate that BMSCs may be a new alternative used to treat chronic orofacial pain by reducing the NMDAR activities in the ascending and descending nervous system, which needs further studies to investigate the detailed mechanisms and evaluate the efficiency and safety.
Glutamate sensitizes sensory neurons to nociceptive stimulation in a manner dependent on NMDARs (Coderre and Melzack, 1992), The SGCs play an important role in this process by expressing glutamate transporters that regulate glutamate neurotransmission (Castillo et al., 2013), but SGCs also express Ca2+-permeable, Mg2+-insensitive NMDARs which are required for sensitization of neurons (Ferrari et al., 2014). Although the involvement of SGC NMDARs sensitization remains to be demonstrated with conditional silencing approaches in vivo, these findings highlight the possibility that glial NMDARs are important nociceptive intermediates. Indeed, it is extremely difficult to design drugs that target only glial cells without affecting neurons. Furthermore, the elimination of glial cells with glia-selective toxins may cause detrimental effects, given the supportive and protective roles of glia (Ji et al., 2013). While it is reported that administration of glial toxin to the DRG has been shown to reduce neuropathic pain (Liu et al., 2012). Besides, targeting SGC activation might prolong acute opioid analgesia (Berta et al., 2012). However, research about only targeting NMDAR in SGCs to treat chronic orofacial pain is rarely reported.
Conclusions and Perspectives
In this review, we reviewed and elucidated the regulatory mechanisms of NMDAR and its role in the initiation, development, and treatment of chronic orofacial pain. NMDARs widely exist in the peripheral and central nervous system and participate in the ascending or descending regulation of chronic orofacial pain through a variety of molecules such as ions, amino acids, enzymes, and proteins, which provides many potential therapeutic targets for the strategy of chronic orofacial pain management through NMDARs. Therapeutic interventions on NMDARs have succeeded in alleviating orofacial inflammatory or neuropathic pain conditions, but we still need to search for more effective interventions. Because, at present, the effect of NMDAR antagonists is still controversial, and there are a series of side effects, its clinical application is limited. Meanwhile, different NMDAR subunits have different physiological functions in chronic orofacial pain. Studying the role of various NMDAR subunits in chronic orofacial pain may be beneficial to understand the pathogenesis of chronicorofacial pain. Therefore, further research on the regulatory mechanism of NMDARs after nerve injury or inflammation is helpful to develop new drugs to accurately regulate the pathological activities of NMDARs while ensuring their normal physiological function and reducing the side effects of drugs.
Moreover, the current studies about the role of NMDARs in the nociceptive circuits in the higher nervous centers are mostly based on spinal cord injury models, there are few studies on the role of NMDARs in the projection circuits based on orofacial pain models. Thus, more studies are needed to investigate the role and mechanism of NMDARs in the higher central nociceptive circuits in both ascending and descending nervous systems which regulate the nociceptive information from peripheral tissues of the trigeminal nervous system. In addition to the objective changes of tissue injury, inflammation, expression of acting factors, and pathway activation, the role of NMDARs in pain perception is also affected by psychological stress and depression, which suggests that there may be non-drug treatment strategies for chronic orofacial pain. Evidence indicated that there are sex-difference in the role of NMDARs in chronic orofacial pain, however, the detailed mechanisms underlying the regulation ofNMDARs on chronic orofacial pain are still not clear, which should be an important scientific topic in the future.
The limitations of this topical review are that even though we intend to focus on the role and regulatory mechanisms of NMDARs in the trigeminal nervous system in the development and maintenance of chronic orofacial pain including orofacial neuropathic and chronic orofacial inflammatory pain, about which there are still not many studies in the literature. Therefore, some summaries and discussions were based on the evidence found in the studies of the regulatory roles and mechanisms of NMDARs in the spinal nervous system in chronic pain.
In summary, there are many ways to regulate NMDARs, but the regulatory mechanism of NMDARs in the onset and development of chronic orofacial pain is not fully understood. Therefore, targeting upstream and downstream signal pathways of NMDARs may be a new approach to inhibit orofacial allodynia, hyperalgesia, and ectopic pain. This type of treatment is yet to be discovered and explored.
Author Contributions
Y-JL and Y-LL contributed to the literature search, writing—original draft. Z-HF and H-LL drew schematic diagrams. Y-YZ and JL revised the manuscript. FL and J-FS contributed to conceptualization, supervision, revision, and resources. All authors contributed to the article and approved the submitted version.
Funding
The study was funded by the National Natural Science Foundation of China (Grant Number: 81870800, 82071149), the Department of Science and Technology of Sichuan Province (Grant Number: 2021JDRC0166, 2020YJ0224), and the China Postdoctoral Science Foundation (Grant Number: 2020M683329).
Conflict of Interest
The authors declare that the research was conducted in the absence of any commercial or financial relationships that could be construed as a potential conflict of interest.
Publisher’s Note
All claims expressed in this article are solely those of the authors and do not necessarily represent those of their affiliated organizations, or those of the publisher, the editors and the reviewers. Any product that may be evaluated in this article, or claim that may be made by its manufacturer, is not guaranteed or endorsed by the publisher.
References
Aarts, M., Liu, Y., Liu, L., Besshoh, S., Arundine, M., Gurd, J. W., et al. (2002). Treatment of ischemic brain damage by perturbing NMDA receptor- PSD-95 protein interactions. Science 298, 846–850. doi: 10.1126/science.1072873
Abdelrahman, K. M., and Hackshaw, K. V. (2021). Nutritional supplements for the treatment of neuropathic pain. Biomedicines 9:674. doi: 10.3390/biomedicines9060674
Aiyer, R., Mehta, N., Gungor, S., and Gulati, A. (2018). A systematic review of NMDA receptor antagonists for treatment of neuropathic pain in clinical practice. Clin. J. Pain 34, 450–467. doi: 10.1097/AJP.0000000000000547
Alviar, M. J., Hale, T., and Dungca, M. (2016). Pharmacologic interventions for treating phantom limb pain. Cochrane Database Syst. Rev. 10:Cd006380. doi: 10.1002/14651858.CD006380.pub3
Anonymous (2020). International classification of orofacial pain, 1st edition (ICOP). Cephalagia 40, 129–221. doi: 10.1177/0333102419893823
Apkarian, A. V., Bushnell, M. C., Treede, R. D., and Zubieta, J. K. (2005). Human brain mechanisms of pain perception and regulation in health and disease. Eur. J. Pain 9, 463–484. doi: 10.1016/j.ejpain.2004.11.001
Bardoni, R. (2013). Role of presynaptic glutamate receptors in pain transmission at the spinal cord level. Curr. Neuropharmacol. 11, 477–483. doi: 10.2174/1570159X11311050002
Bardoni, R., Torsney, C., Tong, C., Prandini, M., and MacDermott, A. (2004). Presynaptic NMDA receptors modulate glutamate release from primary sensory neurons in rat spinal cord dorsal horn. J. Neurosci. 24, 2774–2781. doi: 10.1523/JNEUROSCI.4637-03.2004
Begon, S., Pickering, G., Eschalier, A., Mazur, A., Rayssiguier, Y., and Dubray, C. (2001). Role of spinal NMDA receptors, protein kinase C and nitric oxide synthase in the hyperalgesia induced by magnesium deficiency in rats. Br. J. Pharmacol. 134, 1227–1236. doi: 10.1038/sj.bjp.0704354
Behbehani, M. M., and Fields, H. L. (1979). Evidence that an excitatory connection between the periaqueductal gray and nucleus raphe magnus mediates stimulation produced analgesia. Brain Res. 170, 85–93. doi: 10.1016/0006-8993(79)90942-9
Belin, S., Maki, B. A., Catlin, J., Rein, B. A., and Popescu, G. K. (2022). Membrane stretch gates NMDA receptors. J. Neurosci. 42, 5672–5680. doi: 10.1523/JNEUROSCI.0350-22.2022
Benrath, J., Brechtel, C., Stark, J., and Sandkühler, J. (2005). Low dose of S+-ketamine prevents long-term potentiation in pain pathways under strong opioid analgesia in the rat spinal cord in vivo. Br. J. Anaesth. 95, 518–523. doi: 10.1093/bja/aei215
Bereiter, D. A., Hirata, H., and Hu, J. W. (2000). Trigeminal subnucleus caudalis: beyond homologies with the spinal dorsal horn. Pain 88, 221–224. doi: 10.1016/S0304-3959(00)00434-6
Berta, T., Liu, T., Liu, Y. C., Xu, Z. Z., and Ji, R. R. (2012). Acute morphine activates satellite glial cells and up-regulates IL-1β in dorsal root ganglia in mice via matrix metalloprotease-9. Mol. Pain 8:18. doi: 10.1186/1744-8069-8-18
Bower, J. M., and Woolston, D. C. (1983). Congruence of spatial organization of tactile projections to granule cell and Purkinje cell layers of cerebellar hemispheres of the albino rat: vertical organization of cerebellar cortex. J. Neurophysiol. 49, 745–766. doi: 10.1152/jn.1983.49.3.745
Bushnell, M. C., Ceko, M., and Low, L. A. (2013). Cognitive and emotional control of pain and its disruption in chronic pain. Nat. Rev. Neurosci. 14, 502–511. doi: 10.1038/nrn3516
Cai, S. X. (2006). Glycine/NMDA receptor antagonists as potential CNS therapeutic agents: ACEA-1021 and related compounds. Curr. Top. Med. Chem. 6, 651–662. doi: 10.2174/156802606776894465
Cairns, B. E., Svensson, P., Wang, K., Hupfeld, S., Graven-Nielsen, T., Sessle, B. J., et al. (2003). Activation of peripheral NMDA receptors contributes to human pain and rat afferent discharges evoked by injection of glutamate into the masseter muscle. J. Neurophysiol. 90, 2098–2105. doi: 10.1152/jn.00353.2003
Carlsson, K. C., Hoem, N. O., Moberg, E. R., and Mathisen, L. C. (2004). Analgesic effect of dextromethorphan in neuropathic pain. Acta Anaesthesiol. Scand. 48, 328–336. doi: 10.1111/j.0001-5172.2004.0325.x
Casado, M., and Ascher, P. (1998). Opposite modulation of NMDA receptors by lysophospholipids and arachidonic acid: common features with mechanosensitivity. J. Physiol. 513, 317–330. doi: 10.1111/j.1469-7793.1998.317bb.x
Casado, M., Dieudonné, S., and Ascher, P. (2000). Presynaptic N-methyl-D-aspartate receptors at the parallel fiber-Purkinje cell synapse. Proc. Natl. Acad. Sci. U S A 97, 11593–11597. doi: 10.1073/pnas.200354297
Castillo, C., Norcini, M., Hernandez, L. A. M., Correa, G., Blanck, T. J. J., and Recio-Pinto, E. (2013). Satellite glia cells in dorsal root ganglia express functional NMDA receptors. Neuroscience 240, 135–146. doi: 10.1016/j.neuroscience.2013.02.031
Castro, A., Raver, C., Li, Y., Uddin, O., Rubin, D., Ji, Y. D., et al. (2017). Cortical regulation of nociception of the trigeminal nucleus caudalis. J. Neurosci. 37, 11431–11440. doi: 10.1523/JNEUROSCI.3897-16.2017
Cavalcante, A. L., Siqueira, R. M., Araujo, J. C., Gondim, D. V., Ribeiro, R. A., Quetz, J. S., et al. (2013). Role of NMDA receptors in the trigeminal pathway and the modulatory effect of magnesium in a model of rat temporomandibular joint arthritis. Eur. J. Oral Sci. 121, 573–583. doi: 10.1111/eos.12093
Cavara, N. A., and Hollmann, M. (2008). Shuffling the deck anew: how NR3 tweaks NMDA receptor function. Mol. Neurobiol. 38, 16–26. doi: 10.1007/s12035-008-8029-9
Cavara, N. A., Orth, A., Hicking, G., Seebohm, G., and Hollmann, M. (2010). Residues at the tip of the pore loop of NR3B-containing NMDA receptors determine Ca2+ permeability and Mg2+ block. BMC Neurosci. 11:133. doi: 10.1186/1471-2202-11-133
Chen, Q. L., and Heinricher, M. M. (2019). Plasticity in the link between pain-transmitting and pain-modulating systems in acute and persistent inflammation. J. Neurosci. 39, 2065–2079. doi: 10.1523/JNEUROSCI.2552-18.2019
Chen, L., and Huang, L. Y. (1992). Protein kinase C reduces Mg2+ block of NMDA-receptor channels as a mechanism of modulation. Nature 356, 521–523. doi: 10.1038/356521a0
Chen, J., Li, L., Chen, S. R., Chen, H., Xie, J. D., Sirrieh, R. E., et al. (2022). The α2δ-1-NMDA receptor complex is critically involved in neuropathic pain development and gabapentin therapeutic actions. Cell Rep. 38:110308. doi: 10.1016/j.celrep.2022.110308
Chen, J., Li, L., Chen, S., Chen, H., Xie, J., Sirrieh, R., et al. (2018). The α2δ-1-NMDA receptor complex is critically involved in neuropathic pain development and gabapentin therapeutic actions. Cell Rep. 22, 2307–2321. doi: 10.1016/j.celrep.2018.02.021
Chen, H. S., and Lipton, S. A. (2005). Pharmacological implications of two distinct mechanisms of interaction of memantine with N-methyl-D-aspartate-gated channels. J. Pharmacol. Exp. Ther. 314, 961–971. doi: 10.1124/jpet.105.085142
Chen, H. S., and Lipton, S. A. (2006). The chemical biology of clinically tolerated NMDA receptor antagonists. J. Neurochem. 97, 1611–1626. doi: 10.1111/j.1471-4159.2006.03991.x
Chen, Q., Li, X., Lu, J., Liu, Y., Lee, J., Chen, Y., et al. (2021a). NMDA GluN2C/2D receptors contribute to synaptic regulation and plasticity in the anterior cingulate cortex of adult mice. Mol. Brain 14:60. doi: 10.1186/s13041-021-00744-3
Chen, Q., Li, X., and Zhuo, M. (2021b). NMDA receptors and synaptic plasticity in the anterior cingulate cortex. Neuropharmacology 197:108749. doi: 10.1016/j.neuropharm.2021.108749
Chen, K. B., Wei, V. C., Yen, L. F., Poon, K. S., Liu, Y. C., Cheng, K. S., et al. (2013). Intravenous mannitol does not increase blood-brain barrier permeability to inert dyes in the adult rat forebrain. Neuroreport 24, 303–307. doi: 10.1097/WNR.0b013e32835f8acb
Chen, S., Zhou, H., Byun, H., Chen, H., and Pan, H. (2014). Casein kinase II regulates N-methyl-D-aspartate receptor activity in spinal cords and pain hypersensitivity induced by nerve injury. J. Pharmacol. Exp. Ther. 350, 301–312. doi: 10.1124/jpet.114.215855
Chiang, M. C., Bowen, A., Schier, L. A., Tupone, D., Uddin, O., and Heinricher, M. M. (2019). Parabrachial complex: a hub for pain and aversion. J. Neurosci. 39, 8225–8230. doi: 10.1523/JNEUROSCI.1162-19.2019
Chichorro, J. G., Porreca, F., and Sessle, B. (2017). Mechanisms of craniofacial pain. Cephalalgia 37, 613–626. doi: 10.1177/0333102417704187
Choi, Y. B., and Lipton, S. A. (1999). Identification and mechanism of action of two histidine residues underlying high-affinity Zn2+ inhibition of the NMDA receptor. Neuron 23, 171–180. doi: 10.1016/s0896-6273(00)80763-1
Choi, S. R., Moon, J. Y., Roh, D. H., Yoon, S. Y., Kwon, S. G., Choi, H. S., et al. (2017). Spinal D-serine increases PKC-dependent GIuN1 phosphorylation contributing to the sigma-1 receptor-induced development of mechanical allodynia in a mouse model of neuropathic pain. J. Pain 18, 415–427. doi: 10.1016/j.jpain.2016.12.002
Chou, T. H., Tajima, N., Romero-Hernandez, A., and Furukawa, H. (2020). Structural basis of functional transitions in mammalian NMDA receptors. Cell 182, 357–371.e13. doi: 10.1016/j.cell.2020.05.052
Church, J., Lodge, D., and Berry, S. (1985). Differential effects of dextrorphan and levorphanol on the excitation of rat spinal neurons by amino acids. Eur. J. Pharmacol. 111, 185–190. doi: 10.1016/0014-2999(85)90755-1
Coderre, T. J., and Melzack, R. (1992). The role of nmda receptor operated calcium channels in persistent nociception after formalin-induced tissue-injury. J. Neurosci. 12, 3671–3675. doi: 10.1523/JNEUROSCI.12-09-03671.1992
Collins, S., Sigtermans, M. J., Dahan, A., Zuurmond, W. W., and Perez, R. S. (2010). NMDA receptor antagonists for the treatment of neuropathic pain. Pain Med. 11, 1726–1742. doi: 10.1111/j.1526-4637.2010.00981.x
Corder, G., Doolen, S., Donahue, R. R., Winter, M. K., Jutras, B. L., He, Y., et al. (2013). Constitutive mu-opioid receptor activity leads to long-term endogenous analgesia and dependence. Science 341, 1394–1399. doi: 10.1126/science.1239403
Coste, J., Voisin, D. L., Luccarini, P., and Dallel, R. (2008). A role for wind-up in trigeminal sensory processing: intensity coding of nociceptive stimuli in the rat. Cephalalgia 28, 631–639. doi: 10.1111/j.1468-2982.2008.01568.x
Courtney, M. J., Li, L. L., and Lai, Y. Y. (2014). Mechanisms of NOS1AP action on NMDA receptor-nNOS signaling. Front. Cell. Neurosci. 8:252. doi: 10.3389/fncel.2014.00252
Cull-Candy, S.G., and Leszkiewicz, D. N. (2004). Role of distinct NMDA receptor subtypes at central synapses. Sci. STKE 2004:re16. doi: 10.1126/stke.2552004re16
Dahlstrom, L., and Carlsson, G. E. (2010). Temporomandibular disorders and oral health-related quality of life. A systematic review. Acta Odontol. Scand. 68, 80–85. doi: 10.3109/00016350903431118
Davies, J., Evans, R. H., Jones, A. W., Smith, D. A., and Watkins, J. C. (1982). Differential activation and blockade of excitatory amino acid receptors in the mammalian and amphibian central nervous systems. Comp. Biochem. Physiol. C Comp. Pharmacol. 72, 211–224. doi: 10.1016/0306-4492(82)90086-7
Davies, J., Francis, A. A., Jones, A. W., and Watkins, J. C. (1981). 2-Amino-5-phosphonovalerate (2APV), a potent and selective antagonist of amino acid-induced and synaptic excitation. Neurosci. Lett. 21, 77–81. doi: 10.1016/0304-3940(81)90061-6
Dedek, A., and Hildebrand, M. E. (2022). Advances and barriers in understanding presynaptic N-methyl-D-aspartate receptors in spinal pain processing. Front. Mol. Neurosci. 15:864502. doi: 10.3389/fnmol.2022.864502
Deng, M., Chen, S. R., Chen, H., Luo, Y., Dong, Y., and Pan, H. L. (2019). Mitogen-activated protein kinase signaling mediates opioid-induced presynaptic NMDA receptor activation and analgesic tolerance. J. Neurochem. 148, 275–290. doi: 10.1111/jnc.14628
Deng, M. C., Chen, S. R., and Pan, H. L. (2019). Presynaptic NMDA receptors control nociceptive transmission at the spinal cord level in neuropathic pain. Cell. Mol. Life Sci. 76, 1889–1899. doi: 10.1007/s00018-019-03047-y
Deng, J., Zhou, H., Lin, J. K., Shen, Z. X., Chen, W. Z., Wang, L. H., et al. (2020). The parabrachial nucleus directly channels spinal nociceptive signals to the intralaminar thalamic nuclei, but not the amygdala. Neuron 107, 909–923.e6. doi: 10.1016/j.neuron.2020.06.017
Dieb, W., and Hafidi, A. (2013). Astrocytes are involved in trigeminal dynamic mechanical allodynia: potential role of D-serine. J. Dent. Res. 92, 808–813. doi: 10.1177/0022034513498898
Dinis-Oliveira, R. J. (2017). Metabolism and metabolomics of ketamine: a toxicological approach. Forensic Sci. Res. 2, 2–10. doi: 10.1080/20961790.2017.1285219
Dong, X. D., Mann, M. K., Kumar, U., Svensson, P., Arendt-Nielsen, L., Hu, J. W., et al. (2007). Sex-related differences in NMDA-evoked rat masseter muscle afferent discharge result from estrogen-mediated modulation of peripheral NMDA receptor activity. Neuroscience 146, 822–832. doi: 10.1016/j.neuroscience.2007.01.051
Dravid, S. M., Erreger, K., Yuan, H., Nicholson, K., Le, P., Lyuboslavsky, P., et al. (2007). Subunit-specific mechanisms and proton sensitivity of NMDA receptor channel block. J. Physiol. 581, 107–128. doi: 10.1113/jphysiol.2006.124958
Durand, G. M., Gregor, P., Zheng, X., Bennett, M. V., Uhl, G. R., and Zukin, R. S. (1992). Cloning of an apparent splice variant of the rat N-methyl-D-aspartate receptor NMDAR1 with altered sensitivity to polyamines and activators of protein kinase C. Proc. Natl. Acad. Sci. U S A 89, 9359–9363. doi: 10.1073/pnas.89.19.9359
Dzamba, D., Honsa, P., and Anderova, M. (2013). NMDA receptors in glial cells: pending questions. Curr. Neuropharmacol. 11, 250–262. doi: 10.2174/1570159X11311030002
Eccles, J. C., Faber, D. S., Murphy, J. T., Sabah, N. H., and Táboríková, H. (1971). Investigations on integration of mossy fiber inputs to Purkyns cells in the anterior lobe. Exp. Brain Res. 13, 54–77.
Erreger, K., Dravid, S. M., Banke, T. G., Wyllie, D. J. A., and Traynelis, S. F. (2005). Subunit-specific gating controls rat NRl/NR2A and NR1/NR2B NMDA channel kinetics and synaptic signalling profiles. J. Physiol. 563, 345–358. doi: 10.1113/jphysiol.2004.080028
Farrant, M., Feldmeyer, D., Takahashi, T., and Cullcandy, S. G. (1994). NMDA-receptor channel diversity in the developing cerebellum. Nature 368, 335–339. doi: 10.1038/368335a0
Fayyazuddin, A., Villarroel, A., Le Goff, A., Lerma, J., and Neyton, J. (2000). Four residues of the extracellular N-terminal domain of the NR2A subunit control high-affinity Zn2+ binding to NMDA receptors. Neuron 25, 683–694. doi: 10.1016/s0896-6273(00)81070-3
Felsby, S., Nielsen, J., ArendtNielsen, L., and Jensen, T. S. (1996). NMDA receptor blockade in chronic neuropathic pain: a comparison of ketamine and magnesium chloride. Pain 64, 283–291. doi: 10.1016/0304-3959(95)00113-1
Fernandez-Montoya, J., Avendano, C., and Negredo, P. (2017). The glutamatergic system in primary somatosensory neurons and its involvement in sensory input-dependent plasticity. Int. J. Mol. Sci. 19:69. doi: 10.3390/ijms19010069
Fernandez-Montoya, J., Buendia, I., Martin, Y. B., Egea, J., Negredo, P., and Avendano, C. (2016). Sensory input-dependent changes in glutamatergic neurotransmission- related genes and proteins in the adult rat trigeminal ganglion. Front. Mol. Neurosci. 9:132. doi: 10.3389/fnmol.2016.00132
Ferrari, L. F., Lotufo, C. M., Araldi, D., Rodrigues, M. A., Macedo, L. P., Ferreira, S. H., et al. (2014). Inflammatory sensitization of nociceptors depends on activation of NMDA receptors in DRG satellite cells. Proc. Natl. Acad. Sci. U S A 111, 18363–18368. doi: 10.1073/pnas.1420601111
Fields, H. L., Malick, A., and Burstein, R. (1995). Dorsal horn projection targets of ON and OFF cells in the rostral ventromedial medulla. J. Neurophysiol. 74, 1742–1759. doi: 10.1152/jn.1995.74.4.1742
Finnerup, N., Kuner, R., and Jensen, T. (2021). Neuropathic pain: from mechanisms to treatment. J. Physiol. Rev. 101, 259–301. doi: 10.1152/physrev.00045.2019
Florio, S. K., Loh, C., Huang, S. M., Iwamaye, A. E., Kitto, K. F., Fowler, K. W., et al. (2009). Disruption of nNOS-PSD95 protein-protein interaction inhibits acute thermal hyperalgesia and chronic mechanical allodynia in rodents. Br. J. Pharmacol. 158, 494–506. doi: 10.1111/j.1476-5381.2009.00300.x
Floyd, N. S., Price, J. L., Ferry, A. T., Keay, K. A., and Bandler, R. (2000). Orbitomedial prefrontal cortical projections to distinct longitudinal columns of the periaqueductal gray in the rat. J. Comp. Neurol. 422, 556–578. doi: 10.1002/1096-9861(20000710)422:4<556::aid-cne6>3.0.co;2-u
Foy, M. R., Xu, J., Xie, X., Brinton, R. D., Thompson, R. F., and Berger, T. W. (1999). 17β-estradiol enhances NMDA receptor-mediated EPSPs and long-term potentiation. J. Neurophysiol. 81, 925–929. doi: 10.1152/jn.1999.81.2.925
Fu, M., Liu, F., Zhang, Y. Y., Lin, J., Huang, C. L., Li, Y. L., et al. (2021). The α2δ-1-NMDAR1 interaction in the trigeminal ganglion contributes to orofacial ectopic pain following inferior alveolar nerve injury. Brain Res. Bull. 171, 162–171. doi: 10.1016/j.brainresbull.2021.03.019
Furukawa, H., Singh, S. K., Mancusso, R., and Gouaux, E. (2005). Subunit arrangement and function in NMDA receptors. Nature 438, 185–192. doi: 10.1038/nature04089
Gao, M., Rejaei, D., and Liu, H. (2016). Ketamine use in current clinical practice. Acta Pharmacol. Sin. 37, 865–872. doi: 10.1038/aps.2016.5
Gauriau, C., and Bernard, J. F. (2002). Pain pathways and parabrachial circuits in the rat. Exp. Physiol. 87, 251–258. doi: 10.1113/eph8702357
Gazerani, P., Dong, X., Wang, M., Kumar, U., and Cairns, B. E. (2010). Sensitization of rat facial cutaneous mechanoreceptors by activation of peripheral N-methyl-d-aspartate receptors. Brain Res. 1319, 70–82. doi: 10.1016/j.brainres.2010.01.018
Gerard, F., and Hansson, E. (2012). Inflammatory activation enhances NMDA-triggered Ca2+ signalling and IL-1 β secretion in primary cultures of rat astrocytes. Brain Res. 1473, 1–8. doi: 10.1016/j.brainres.2012.07.032
Gilron, I., Booher, S. L., Rowan, J. S., Smoller, B., and Max, M. B. (2000). A randomized, controlled trial of high-dose dextromethorphan in facial neuralgias. Neurology 55, 964–971. doi: 10.1212/wnl.55.7.964
Glitsch, M., and Marty, A. (1999). Presynaptic effects of NMDA in cerebellar Purkinje cells and interneurons. J. Neurosci. 19, 511–519. doi: 10.1523/JNEUROSCI.19-02-00511.1999
Gottrup, H., Bach, F., Juhl, G., and Jensen, T. (2006). Differential effect of ketamine and lidocaine on spontaneous and mechanical evoked pain in patients with nerve injury pain. Anesthesiology 104, 527–536. doi: 10.1097/00000542-200603000-00021
Groc, L., Bard, L., and Choquet, D. (2009). Surface trafficking of N-Methyl-D-aspartate receptors: physiological and pathological perspectives. Neuroscience 158, 4–18. doi: 10.1016/j.neuroscience.2008.05.029
Guerrero-Toro, C., Koroleva, K., Ermakova, E., Gafurov, O., Abushik, P., Tavi, P., et al. (2022). Testing the role of glutamate NMDA receptors in peripheral trigeminal nociception implicated in migraine pain. Int. J. Mol. Sci. 23:1529. doi: 10.3390/ijms23031529
Guo, W., Chu, Y. X., Imai, S., Yang, J. L., Zou, S., Mohammad, Z., et al. (2016). Further observations on the behavioral and neural effects of bone marrow stromal cells in rodent pain models. Mol. Pain 12:1744806916658043. doi: 10.1177/1744806916658043
Guo, F., Du, Y., Qu, F. H., Lin, S. D., Chen, Z., and Zhang, S. H. (2022). Dissecting the neural circuitry for pain modulation and chronic pain: insights from optogenetics. Neurosci. Bull. 38, 440–452. doi: 10.1007/s12264-022-00835-8
Guo, W., Imai, S., Yang, J. L., Zou, S., Li, H., Xu, H., et al. (2018). NF-κB pathway is involved in bone marrow stromal cell-produced pain relief. Front. Integr. Neurosci. 12:49. doi: 10.3389/fnint.2018.00049
Guo, W., Miyoshi, K., Dubner, R., Gu, M., Li, M., Liu, J., et al. (2014). Spinal 5-HT3 receptors mediate descending facilitation and contribute to behavioral hypersensitivity via a reciprocal neuron-glial signaling cascade. Mol. Pain 10:35. doi: 10.1186/1744-8069-10-35
Guo, W., Wang, H., Watanabe, M., Shimizu, K., Zou, S., LaGraize, S. C., et al. (2007). Glial-cytokine-neuronal interactions underlying the mechanisms of persistent pain. J. Neurosci. 27, 6006–6018. doi: 10.1523/JNEUROSCI.0176-07.2007
Guo, W., Wang, H., Zou, S., Gu, M., Watanabe, M., Wei, F., et al. (2011). Bone marrow stromal cells produce long-term pain relief in rat models of persistent pain. Stem Cells 29, 1294–1303. doi: 10.1002/stem.667
Guo, W., Zou, S., Guan, Y., Ikeda, T., Tal, M., Dubner, R., et al. (2002). Tyrosine phosphorylation of the NR2B subunit of the NMDA receptor in the spinal cord during the development and maintenance of inflammatory hyperalgesia. J. Neurosci. 22, 6208–6217. doi: 10.1523/jneurosci.22-14-06208.2002
Hansen, K. B., Wollmuth, L. P., Bowie, D., Furukawa, H., Menniti, F. S., Sobolevsky, A. I., et al. (2021). Structure, function and pharmacology of glutamate receptor ion channels. Pharmacol. Rev. 73, 298–487. doi: 10.1124/pharmrev.120.000131
Hansen, K. B., Yi, F., Perszyk, R. E., Furukawa, H., Wollmuth, L. P., Gibb, A. J., et al. (2018). Structure, function and allosteric modulation of NMDA receptors. J. Gen. Physiol. 150, 1081–1105. doi: 10.1085/jgp.201812032
Hardingham, G. E., and Bading, H. (2010). Synaptic versus extrasynaptic NMDA receptor signalling: implications for neurodegenerative disorders. Nat. Rev. Neurosci. 11, 682–696. doi: 10.1038/nrn2911
Heinricher, M. M. (2016). “Pain modulation and the transition from acute to chronic pain,” in Translational Research in Pain and Itch, eds C. Ma and Y. Huang (Dordrecht: Springer), 105–115. doi: 10.1007/978-94-017-7537-3_8
Heinricher, M., and Roychowdhury, S. (1997). Reflex-related activation of putative pain facilitating neurons in rostral ventromedial medulla requires excitatory amino acid transmission. Neuroscience 78, 1159–1165. doi: 10.1016/s0306-4522(96)00683-5
Heinricher, M., Schouten, J., and Jobst, E. (2001). Activation of brainstem N-methyl-D-aspartate receptors is required for the analgesic actions of morphine given systemically. Pain 92, 129–138. doi: 10.1016/s0304-3959(00)00480-2
Heiskanen, T., Hartel, B., Dahl, M. L., Seppala, T., and Kalso, E. (2002). Analgesic effects of dextromethorphan and morphine in patients with chronic pain. Pain 96, 261–267. doi: 10.1016/S0304-3959(01)00455-9
Henson, M. A., Roberts, A. C., Perez-Otano, I., and Philpot, B. D. (2010). Influence of the NR3A subunit on NMDA receptor functions. Prog. Neurobiol. 91, 23–37. doi: 10.1016/j.pneurobio.2010.01.004
Hildebrand, M., Xu, J., Dedek, A., Li, Y., Sengar, A., Beggs, S., et al. (2016). Potentiation of synaptic GluN2B NMDAR currents by fyn kinase is gated through BDNF-mediated disinhibition in spinal pain processing. Cell Rep. 17, 2753–2765. doi: 10.1016/j.celrep.2016.11.024
Hogan-Cann, A. D., and Anderson, C. M. (2016). Physiological roles of non-neuronal NMDA receptors. Trends Pharmacol. Sci. 37, 750–767. doi: 10.1016/j.tips.2016.05.012
Huang, Y., Chen, S., Chen, H., Luo, Y., and Pan, H.-L. (2020). Calcineurin inhibition causes α2δ-1-mediated tonic activation of synaptic NMDA receptors and pain hypersensitivity. J. Neurosci. 40, 3707–3719. doi: 10.1523/JNEUROSCI.0282-20.2020
Huang, Y., Chen, S. R., Chen, H., Zhou, J. J., Jin, D., and Pan, H. L. (2022). Theta-burst stimulation of primary afferents drives long-term potentiation in the spinal cord and persistent pain via α2δ-1-bound NMDA receptors. J. Neurosci. 42, 513–527. doi: 10.1523/JNEUROSCI.1968-21.2021
Huang, F., Zhang, M., Chen, Y. J., Li, Q., and Wu, A. Z. (2011). Psychological stress induces temporary masticatory muscle mechanical sensitivity in rats. J. Biomed. Biotechnol. 2011:720603. doi: 10.1155/2011/720603
Huh, Y., Ji, R. R., and Chen, G. (2017). Neuroinflammation, bone marrow stem cells and chronic pain. Front. Immunol. 8:1014. doi: 10.3389/fimmu.2017.01014
Ikeda, H., Heinke, B., Ruscheweyh, R., and Sandkühler, J. (2003). Synaptic plasticity in spinal lamina I projection neurons that mediate hyperalgesia. Science 299, 1237–1240. doi: 10.1126/science.1080659
Jaffrey, S. R., Snowman, A. M., Eliasson, M. J., Cohen, N. A., and Snyder, S. H. (1998). CAPON: a protein associated with neuronal nitric oxide synthase that regulates its interactions with PSD95. Neuron 20, 115–124. doi: 10.1016/s0896-6273(00)80439-0
Ji, R. R., Berta, T., and Nedergaard, M. (2013). Glia and pain: is chronic pain a gliopathy? Pain 154, S10–S28. doi: 10.1016/j.pain.2013.06.022
Jiang, H., Liu, J. P., Xi, K., Liu, L. Y., Kong, L. Y., Cai, J., et al. (2021). Contribution of AMPA receptor-mediated LTD in LA/BLA-CeA pathway to comorbid aversive and depressive symptoms in neuropathic pain. J. Neurosci. 41, 7278–7299. doi: 10.1523/JNEUROSCI.2678-20.2021
Jimenez-Blasco, D., Santofimia-Castano, P., Gonzalez, A., Almeida, A., and Bolanos, J. P. (2015). Astrocyte NMDA receptors’ activity sustains neuronal survival through a Cdk5-Nrf2 pathway. Cell Death Differ. 22, 1877–1889. doi: 10.1038/cdd.2015.49
Johnson, J. W., and Ascher, P. (1990). Voltage-dependent block by intracellular Mg2+ of N-methyl-D-aspartate-activated channels. Biophys. J. 57, 1085–1090. doi: 10.1016/S0006-3495(90)82626-6
Kamp, J., Van Velzen, M., Olofsen, E., Boon, M., Dahan, A., and Niesters, M. (2019). Pharmacokinetic and pharmacodynamic considerations for NMDA-receptor antagonist ketamine in the treatment of chronic neuropathic pain: an update of the most recent literature. Expert. Opin. Drug Metab. Toxicol. 15, 1033–1041. doi: 10.1080/17425255.2019.1689958
Kaneko, M., Kaneko, T., Kaneko, R., Chokechanachaisakul, U., Kawamura, J., Sunakawa, M., et al. (2011). The role of N-methyl-D-aspartate receptor subunits in the rat thalamic mediodorsal nucleus during central sensitization. Brain Res. 1371, 16–22. doi: 10.1016/j.brainres.2010.11.054
Kim, Y. S., Chu, Y., Han, L., Li, M., Li, Z., LaVinka, P. C., et al. (2014). Central terminal sensitization of TRPV1 by descending serotonergic facilitation modulates chronic pain. Neuron 81, 873–887. doi: 10.1016/j.neuron.2013.12.011
Kim, H., Jun, J., Wang, J., Bittar, A., Chung, K., and Chung, J. (2015). Induction of long-term potentiation and long-term depression is cell-type specific in the spinal cord. Pain 156, 618–625. doi: 10.1097/01.j.pain.0000460354.09622.ec
Kim, M. J., Lee, S. Y., Yang, K. Y., Nam, S. H., Kim, H. J., Kim, Y. J., et al. (2014). Differential regulation of peripheral IL-1β-induced mechanical allodynia and thermal hyperalgesia in rats. Pain 155, 723–732. doi: 10.1016/j.pain.2013.12.030
Kimura, Y., Hayashi, Y., Hitomi, S., Ikutame, D., Urata, K., Shibuta, I., et al. (2022). IL-33 induces orofacial neuropathic pain through Fyn-dependent phosphorylation of GluN2B in the trigeminal spinal subnucleus caudalis. Brain Behav. Immun. 99, 266–280. doi: 10.1016/j.bbi.2021.10.013
Kinarsky, L., Feng, B., Skifter, D. A., Morley, R. M., Sherman, S., Jane, D. E., et al. (2005). Identification of subunit- and antagonist-specific amino acid residues in the N-Methyl-D-aspartate receptor glutamate-binding pocket. J. Pharmacol. Exp. Ther. 313, 1066–1074. doi: 10.1124/jpet.104.082990
Kloda, A., Lua, L., Hall, R., Adams, D. J., and Martinac, B. (2007). Liposome reconstitution and modulation of recombinant N-methyl-D-aspartate receptor channels by membrane stretch. Proc. Natl. Acad. Sci. U S A 104, 1540–1545. doi: 10.1073/pnas.0609649104
Kreutzwiser, D., and Tawfic, Q. A. (2019). Expanding role of NMDA receptor antagonists in the management of pain. CNS Drugs 33, 347–374. doi: 10.1007/s40263-019-00618-2
Kronschlager, M. T., Drdla-Schutting, R., Gassner, M., Honsek, S. D., Teuchmann, H. L., and Sandkuhler, J. (2016). Gliogenic LTP spreads widely in nociceptive pathways. Science 354, 1144–1148. doi: 10.1126/science.aah5715
Kulik, K., Zyzynska-Granica, B., Kowalczyk, A., Kurowski, P., Gajewska, M., and Bujalska-Zadrozny, M. (2021). Magnesium and morphine in the treatment of chronic neuropathic pain-A biomedical mechanism of action. Int. J. Mol. Sci. 22:13599. doi: 10.3390/ijms222413599
Kuner, R., and Kuner, T. (2021). Cellular circuits in the brain and their modulation in acute and chronic pain. Physiol. Rev. 101, 213–258. doi: 10.1152/physrev.00040.2019
Kung, L. H., Gong, K., Adedoyin, M., Ng, J., Bhargava, A., Ohara, P. T., et al. (2013). Evidence for glutamate as a neuroglial transmitter within sensory ganglia. PLoS One 8:e68312. doi: 10.1371/journal.pone.0068312
Kvarnström, A., Karlsten, R., Quiding, H., and Gordh, T. (2004). The analgesic effect of intravenous ketamine and lidocaine on pain after spinal cord injury. Acta Anaesthesiol. Scand. 48, 498–506. doi: 10.1111/j.1399-6576.2003.00330.x
Kvarnström, A., Karlsten, R., Quiding, H., Emanuelsson, B., and Gordh, T. (2003). The effectiveness of intravenous ketamine and lidocaine on peripheral neuropathic pain. Acta Anaesthesiol. Scand. 47, 868–877. doi: 10.1034/j.1399-6576.2003.00187.x
Lai, C., Lin, T., Hsieh, M., Chen, G., and Peng, H. (2016). SIRPα1-SHP2 interaction regulates complete freund adjuvant-induced inflammatory pain via Src-dependent GluN2B phosphorylation in rats. Anesth. Analg. 122, 871–881. doi: 10.1213/ANE.0000000000001116
Lai, T. W., and Wang, Y. T. (2010). Fashioning drugs for stroke. Nat. Med. 16, 1376–1378. doi: 10.1038/nm1210-1376
Lai, T. W., Zhang, S., and Wang, Y. T. (2014). Excitotoxicity and stroke: identifying novel targets for neuroprotection. Prog. Neurobiol. 115, 157–188. doi: 10.1016/j.pneurobio.2013.11.006
Larsson, M., and Broman, J. (2011). Synaptic plasticity and pain: role of ionotropic glutamate receptors. Neuroscientist 17, 256–273. doi: 10.1177/1073858409349913
Lee, W. H., Li, L. L., Chawla, A., Hudmon, A., Lai, Y. Y., Courtney, M. J., et al. (2018). Disruption of nNOS-NOS1AP protein-protein interactions suppresses neuropathic pain in mice. Pain 159, 849–863. doi: 10.1097/j.pain.0000000000001152
Lee, J., Saloman, J. L., Weiland, G., Auh, Q. S., Chung, M. K., and Ro, J. Y. (2012). Functional interactions between NMDA receptors and TRPV1 in trigeminal sensory neurons mediate mechanical hyperalgesia in the rat masseter muscle. Pain 153, 1514–1524. doi: 10.1016/j.pain.2012.04.015
Lee, W. H., Xu, Z., Ashpole, N. M., Hudmon, A., Kulkarni, P. M., Thakur, G. A., et al. (2015). Small molecule inhibitors of PSD95-nNOS protein-protein interactions as novel analgesics. Neuropharmacology 97, 464–475. doi: 10.1016/j.neuropharm.2015.05.038
Leung, A., Wallace, M., Ridgeway, B., and Yaksh, T. (2001). Concentration-effect relationship of intravenous alfentanil and ketamine on peripheral neurosensory thresholds, allodynia and hyperalgesia of neuropathic pain. Pain 91, 177–187. doi: 10.1016/s0304-3959(00)00433-4
Li, J., and Baccei, M. L. (2016). Neonatal tissue damage promotes spike timing-dependent synaptic long-term potentiation in adult spinal projection neurons. J. Neurosci. 36, 5405–5416. doi: 10.1523/JNEUROSCI.3547-15.2016
Li, S., Cai, J., Feng, Z., Jin, Z., Liu, B., Zhao, H., et al. (2017). BDNF contributes to spinal long-term potentiation and mechanical hypersensitivity via fyn-mediated phosphorylation of NMDA receptor GluN2B subunit at tyrosine 1472 in rats following spinal nerve ligation. Neurochem. Res. 42, 2712–2729. doi: 10.1007/s11064-017-2274-0
Li, X., Ge, S. N., Li, Y., and Wang, H. T. (2017). Neurokinin-1 receptor-immunopositive neurons in the medullary dorsal horn provide collateral axons to both the thalamus and parabrachial nucleus in rats. Neurochem. Res. 42, 375–388. doi: 10.1007/s11064-016-2080-0
Li, L. L., Ginet, V., Liu, X., Vergun, O., Tuittila, M., Mathieu, M., et al. (2013). The nNOS-p38MAPK pathway is mediated by NOS1AP during neuronal death. J. Neurosci. 33, 8185–8201. doi: 10.1523/JNEUROSCI.4578-12.2013
Li, Y.-L., Liu, F., Zhang, Y. Y., Lin, J., Huang, C. L., Fu, M., et al. (2021). NMDAR1-Src-pannexin1 signal pathway in the trigeminal ganglion contributed to orofacial ectopic pain following inferior alveolar nerve transection. Neuroscience 466, 77–86. doi: 10.1016/j.neuroscience.2021.04.032
Li, X.-H., Matsuura, T., Xue, M., Chen, Q., Liu, R., Lu, J., et al. (2021). Oxytocin in the anterior cingulate cortex attenuates neuropathic pain and emotional anxiety by inhibiting presynaptic long-term potentiation. Cell Rep. 36:109411. doi: 10.1016/j.celrep.2021.109411
Li, X., Miao, H., and Zhuo, M. (2019). NMDA receptor dependent long-term potentiation in chronic pain. Neurochem. Res. 44, 531–538. doi: 10.1007/s11064-018-2614-8
Li, J. N., and Sheets, P. L. (2018). The central amygdala to periaqueductal gray pathway comprises intrinsically distinct neurons differentially affected in a model of inflammatory pain. J. Physiol. 596, 6289–6305. doi: 10.1113/JP276935
Li, J. H., Yang, J. L., Wei, S. Q., Li, Z. L., Collins, A. A., Zou, M., et al. (2020). Contribution of central sensitization to stress-induced spreading hyperalgesia in rats with orofacial inflammation. Mol. Brain 13:106. doi: 10.1186/s13041-020-00645-x
Li, J., Zhang, L., Xu, C., Shen, Y., Lin, Y., Zhang, Y., et al. (2021). A pain killer without analgesic tolerance designed by co-targeting PSD-95-nNOS interaction and α2-containning GABARs. Theranostics 11, 5970–5985. doi: 10.7150/thno.58364
Liang, S. H., Zhao, W. J., Yin, J. B., Chen, Y. B., Li, J. N., Feng, B., et al. (2020). A neural circuit from thalamic paraventricular nucleus to central amygdala for the facilitation of neuropathic pain. J. Neurosci. 40, 7837–7854. doi: 10.1523/JNEUROSCI.2487-19.2020
Liao, G. Y., Wagner, D. A., Hsu, M. H., and Leonard, J. P. (2001). Evidence for direct protein kinase-C mediated modulation of N-methyl-D-aspartate receptor current. Mol. Pharmacol. 59, 960–964. doi: 10.1124/mol.59.5.960
Lin, W., Zhao, Y., Cheng, B., Zhao, H., Miao, L., Li, Q., et al. (2019). NMDAR and JNK activation in the spinal trigeminal nucleus caudalis contributes to masseter hyperalgesia induced by stress. Front. Cell. Neurosci. 13:495. doi: 10.3389/fncel.2019.00495
Liu, H., Mantyh, P., and Basbaum, A. (1997). NMDA-receptor regulation of substance P release from primary afferent nociceptors. Nature 386, 721–724. doi: 10.1038/386721a0
Liu, X. G., and Sandkühler, J. (1995). Long-term potentiation of C-fiber-evoked potentials in the rat spinal dorsal horn is prevented by spinal N-methyl-D-aspartic acid receptor blockage. Neurosci. Lett. 191, 43–46. doi: 10.1016/0304-3940(95)11553-0
Liu, F. Y., Sun, Y. N., Wang, F. T., Li, Q., Su, L., Zhao, Z. F., et al. (2012). Activation of satellite glial cells in lumbar dorsal root ganglia contributes to neuropathic pain after spinal nerve ligation. Brain Res. 1427, 65–77. doi: 10.1016/j.brainres.2011.10.016
Liu, T., Walker, J. S., and Tracey, D. J. (1999). Zinc alleviates thermal hyperalgesia due to partial nerve injury. Neuroreport 10, 645–649. doi: 10.1097/00001756-199902250-00037
Liu, L. Y., Zhang, R. L., Chen, L., Zhao, H. Y., Cai, J., Wang, J. K., et al. (2019). Chronic stress increases pain sensitivity via activation of the rACC-BLA pathway in rats. Exp. Neurol. 313, 109–123. doi: 10.1016/j.expneurol.2018.12.009
Llinás, R. (2011). Electrophysiology of the cerebellar networks. Comp. Physiol. 2011, 831–876. doi: 10.1002/cphy.cp010217
Luessen, D. J., and Conn, P. J. (2022). Allosteric modulators of metabotropic glutamate receptors as novel therapeutics for neuropsychiatric disease. Pharmacol. Rev. 74, 630–661. doi: 10.1124/pharmrev.121.000540
Maneshi, M. M., Maki, B., Gnanasambandam, R., Belin, S., Popescu, G. K., Sachs, F., et al. (2017). Mechanical stress activates NMDA receptors in the absence of agonists. Sci. Rep. 7:39610. doi: 10.1038/srep39610
McCartney, C. J. L., Sinha, A., and Katz, J. (2004). A qualitative systematic review of the role of N-methyl-D-aspartate receptor antagonists in preventive analgesia. Anesth. Analg. 98, 1385–1400. doi: 10.1213/01.ane.0000108501.57073.38
McQuay, H. J., Carroll, D., Jadad, A. R., Glynn, C. J., Jack, T., Moore, R. A., et al. (1994). Dextromethorphan for the treatment of neuropathic pain - a double-blind randomized controlled crossover trial with integral N-of-1 design. Pain 59, 127–133. doi: 10.1016/0304-3959(94)90056-6
McRoberts, J. A., Coutinho, S. V., Marvizón, J. C., Grady, E. F., Tognetto, M., Sengupta, J. N., et al. (2001). Role of peripheral N-methyl-D-aspartate (NMDA) receptors in visceral nociception in rats. Gastroenterology 120, 1737–1748. doi: 10.1053/gast.2001.24848
Medeiros, P., Negrini-Ferrari, S., Palazzo, E., Maione, S., Ferreira, S., de Freitas, R., et al. (2019). N-methyl-D-aspartate Receptors in the prelimbic cortex are critical for the maintenance of neuropathic pain. Neurochem. Res. 44, 2068–2080. doi: 10.1007/s11064-019-02843-z
Medrik-Goldberg, T., Lifschitz, D., Pud, D., Adler, R., and Eisenberg, E. (1999). Intravenous lidocaine, amantadine and placebo in the treatment of sciatica: a double-blind, randomized, controlled study. Reg. Anesth. Pain Med. 24, 534–540. doi: 10.1016/s1098-7339(99)90045-7
Mendell, L. M. (2022). The path to discovery of windup and central sensitization. Front. Pain Res. (Lausanne) 3:833104. doi: 10.3389/fpain.2022.833104
Mendell, L. M., and Wall, P. D. (1965). Responses of single dorsal cord cells to peripheral cutaneous unmyelinated fibres. Nature 206, 97–99. doi: 10.1038/206097a0
Mills, E. P., Alshelh, Z., Kosanovic, D., Di Pietro, F., Vickers, E. R., Macey, P. M., et al. (2020). Altered brainstem pain-modulation circuitry connectivity during spontaneous pain intensity fluctuations. J. Pain Res. 13, 2223–2235. doi: 10.2147/JPR.S252594
Miraucourt, L. S., Peirs, C., Dallel, R., and Voisin, D. L. (2011). Glycine inhibitory dysfunction turns touch into pain through astrocyte-derived D-serine. Pain 152, 1340–1348. doi: 10.1016/j.pain.2011.02.021
Missig, G., Mei, L. D., Vizzard, M. A., Braas, K. M., Waschek, J. A., Ressler, K. J., et al. (2017). Parabrachial pituitary adenylate cyclaseactivating polypeptide activation of amygdala endosomal extracellular signal-regulated kinase signaling regulates the emotional component of pain. Biol. Psychiatry 81, 671–682. doi: 10.1016/j.biopsych.2016.08.025
Monyer, H., Sprengel, R., Schoepfer, R., Herb, A., Higuchi, M., Lomeli, H., et al. (1992). Heteromeric NMDA receptors: molecular and functional distinction of subtypes. Science 256, 1217–1221. doi: 10.1126/science.256.5060.1217
Moore, R., Wiffen, P., Derry, S., Toelle, T., and Rice, A. (2014). Gabapentin for chronic neuropathic pain and fibromyalgia in adults. Cochrane Database Syst. Rev. 2014:CD007938. doi: 10.1002/14651858.CD007938.pub3
Morabito, A., Zerlaut, Y., Serraz, B., Sala, R., Paoletti, P., and Rebola, N. (2022). Activity-dependent modulation of NMDA receptors by endogenous zinc shapes dendritic function in cortical neurons. Cell Rep. 38:110415. doi: 10.1016/j.celrep.2022.110415
Morley, J. S., Bridson, J., Nash, T., Miles, J., White, S., and Makin, M. (2003). Low-dose methadone has an analgesic effect in neuropathic pain: a double-blind randomized controlled crossover trial. Palliat. Med. 17, 576–587. doi: 10.1191/0269216303pm815oa
Murphy, J. A., Stein, I. S., Lau, C. G., Peixoto, R. T., Aman, T. K., Kaneko, N., et al. (2014). Phosphorylation of Ser1166 on GluN2B by PKA is critical to synaptic NMDA receptor function and Ca2+ signaling in spines. J. Neurosci. 34, 869–879. doi: 10.1523/JNEUROSCI.4538-13.2014
Nash, P. G., Macefield, V. G., Klineberg, I. J., Murray, G. M., and Henderson, L. A. (2009). Differential activation of the human trigeminal nuclear complex by noxious and non-noxious orofacial stimulation. Hum. Brain Mapp. 30, 3772–3782. doi: 10.1002/hbm.20805
Nie, L., Ye, W., Chen, S., Chirchiglia, D., and Wang, M. (2021). Src family kinases in the central nervous system: their emerging role in pathophysiology of migraine and neuropathic pain. Curr. Neuropharmacol. 19, 665–678. doi: 10.2174/1570159X18666200814180218
Niesters, M., Aarts, L., Sarton, E., and Dahan, A. (2013). Influence of ketamine and morphine on descending pain modulation in chronic pain patients: a randomized placebo-controlled cross-over proof-of-concept study. Br. J. Anaesth. 110, 1010–1016. doi: 10.1093/bja/aes578
Nikolajsen, L., Gottrup, H., Kristensen, A., and Jensen, T. (2000). Memantine (a N-methyl-D-aspartate receptor antagonist) in the treatment of neuropathic pain after amputation or surgery: a randomized, double-blinded, cross-over study. Anesth. Analg. 91, 960–966. doi: 10.1097/00000539-200010000-00036
Nozaki, C., Vergnano, A. M., Filliol, D., Ouagazzal, A. M., Le Goff, A., Carvalho, S., et al. (2011). Zinc alleviates pain through high-affinity binding to the NMDA receptor NR2A subunit. Nat. Neurosci. 14, 1017–1022. doi: 10.1038/nn.2844
Ohrbach, R., Fillingim, R. B., Mulkey, F., Gonzalez, Y., Gordon, S., Gremillion, H., et al. (2011). Clinical findings and pain symptoms as potential risk factors for chronic TMD: descriptive data and empirically identified domains from the OPPERA case-control study. J. Pain 12, T27–T45. doi: 10.1016/j.jpain.2011.09.001
Okamoto, K., Tashiro, A., Chang, Z., Thompson, R., and Bereiter, D. A. (2012). Temporomandibular joint-evoked responses by spinomedullary neurons and masseter muscle are enhanced after repeated psychophysical stress. Eur. J. Neurosci. 36, 2025–2034. doi: 10.1111/j.1460-9568.2012.08100.x
Olszewski, J. (1950). On the anatomical and functional organization of the spinal trigeminal nucleus. J. Comp. Neurol. 92, 401–413. doi: 10.1002/cne.900920305
Ossola, B., Schendzielorz, N., Chen, S., Bird, G., Tuominen, R., Männistö, P., et al. (2011). Amantadine protects dopamine neurons by a dual action: reducing activation of microglia and inducing expression of GDNF in astroglia [corrected]. Neuropharmacology 61, 574–582. doi: 10.1016/j.neuropharm.2011.04.030
Palay, S. L., and Chan-Palay, V. (1974). Cerebellar Cortex: Cytology and Organization. Berlin, Heidelberg: Springer.
Palygin, O., Lalo, U., and Pankratov, Y. (2011). Distinct pharmacological and functional properties of NMDA receptors in mouse cortical astrocytes. Br. J. Pharmacol. 163, 1755–1766. doi: 10.1111/j.1476-5381.2011.01374.x
Pan, L., Li, T., Wang, R., Deng, W., Pu, H., and Deng, M. (2022). Roles of phosphorylation of N-methyl-D-aspartate receptor in chronic pain. Cell. Mol. Neurobiol. . [Online ahead of print]. doi: 10.1007/s10571-022-01188-6
Park, M. K., Lee, J. H., Yang, G. Y., Won, K. A., Kim, M. J., Park, Y. Y., et al. (2011). Peripheral administration of NR2 antagonists attenuates orofacial formalin-induced nociceptive behavior in rats. Prog. Neuropsychopharmacol. Biol. Psychiatry 35, 982–986. doi: 10.1016/j.pnpbp.2011.01.018
Pedersen, L. M., and Gjerstad, J. (2008). Spinal cord long-term potentiation is attenuated by the NMDA-2B receptor antagonist Ro 25-6981. Acta Physiol. (Oxf) 192, 421–427. doi: 10.1111/j.1748-1716.2007.01756.x
Peltoniemi, M. A., Hagelberg, N. M., Olkkola, K. T., and Saari, T. I. (2016). Ketamine: a review of clinical pharmacokinetics and pharmacodynamics in anesthesia and pain therapy. Clin. Pharmacokinet. 55, 1059–1077. doi: 10.1007/s40262-016-0383-6
Petrenko, A. B., Yamakura, T., Baba, H., and Shimoji, K. (2003). The role of N-methyl-D-aspartate (NMDA) receptors in pain: a review. Anesth. Analg. 97, 1108–1116. doi: 10.1213/01.ANE.0000081061.12235.55
Pickering, G., Morel, V., Simen, E., Cardot, J. M., Moustafa, F., Delage, N., et al. (2011). Oral magnesium treatment in patients with neuropathic pain: a randomized clinical trial. Magnes. Res. 24, 28–35. doi: 10.1684/mrh.2011.0282
Porreca, F., Ossipov, M. H., and Gebhart, G. F. (2002). Chronic pain and medullary descending facilitation. Trends Neurosci. 25, 319–325. doi: 10.1016/s0166-2236(02)02157-4
Rachline, J., Perin-Dureau, F., Le Goff, A., Neyton, J., and Paoletti, P. (2005). The micromolar zinc-binding domain on the NMDA receptor subunit NR2B. J. Neurosci. 25, 308–317. doi: 10.1523/JNEUROSCI.3967-04.2005
Ren, K., and Dubner, R. (2011). The role of trigeminal interpolaris-caudalis transition zone in persistent orofacial pain. Int. Rev. Neurobiol. 97, 207–225. doi: 10.1016/B978-0-12-385198-7.00008-4
Ren, W. H., Guo, J. D., Cao, H., Wang, H., Wang, P. F., Sha, H., et al. (2006). Is endogenous D-serine in the rostral anterior cingulate cortex necessary for pain-related negative affect? J. Neurochem. 96, 1636–1647. doi: 10.1111/j.1471-4159.2006.03677.x
Rigo, F. K., Trevisan, G., Godoy, M., Rossato, M., Dalmolin, G., Silva, M., et al. (2017). Management of neuropathic chronic pain with methadone combined with ketamine: a randomized, double blind, active-controlled clinical trial. Pain Physician 20, 207–215.
Ro, J. Y. (2003). Contribution of peripheral NMDA receptors in craniofacial muscle nociception and edema formation. Brain Res. 979, 78–84. doi: 10.1016/s0006-8993(03)02873-7
Ro, J. Y., Capra, N. F., and Masri, R. (2004). Contribution of peripheral N-methyl-D-aspartate receptors to c-fos expression in the trigeminal spinal nucleus following acute masseteric inflammation. Neuroscience 123, 213–219. doi: 10.1016/s0306-4522(03)00465-2
Rocha Barreto, R., Lima Veras, P. J., de Oliveira Leite, G., Vieira-Neto, A. E., Sessle, B. J., Villaca Zogheib, L., et al. (2022). Botulinum toxin promotes orofacial antinociception by modulating TRPV1 and NMDA receptors in adult zebrafish. Toxicon 210, 158–166. doi: 10.1016/j.toxicon.2022.02.005
Rondon, L. J., Privat, A. M., Daulhac, L., Davin, N., Mazur, A., Fialip, J., et al. (2010). Magnesium attenuates chronic hypersensitivity and spinal cord NMDA receptor phosphorylation in a rat model of diabetic neuropathic pain. J. Physiol. 588, 4205–4215. doi: 10.1113/jphysiol.2010.197004
SafiehGarabedian, B., Poole, S., Allchorne, A., Kanaan, S., Saade, N., and Woolf, C. J. (1996). Zinc reduces the hyperalgesia and upregulation of NGF and IL-1 β produced by peripheral inflammation in the rat. Neuropharmacology 35, 599–603. doi: 10.1016/0028-3908(96)84630-2
Saito, H., Katagiri, A., Okada, S., Mikuzuki, L., Kubo, A., Suzuki, T., et al. (2017). Ascending projections of nociceptive neurons from trigeminal subnucleus caudalis: a population approach. Exp. Neurol. 293, 124–136. doi: 10.1016/j.expneurol.2017.03.024
Sanz-Clemente, A., Nicoll, R. A., and Roche, K. W. (2013a). Diversity in NMDA receptor composition: many regulators, many consequences. Neuroscientist 19, 62–75. doi: 10.1177/1073858411435129
Sanz-Clemente, A., Gray, J. A., Ogilvie, K. A., Nicoll, R. A., and Roche, K. W. (2013b). Activated CaMKII couples GluN2B and casein kinase 2 to control synaptic NMDA receptors. Cell Rep. 3, 607–614. doi: 10.1016/j.celrep.2013.02.011
Schipke, C. G., Ohlemeyer, C., Matyash, M., Nolte, C., Kettenmann, H., and Kirchhoff, F. (2001). Astrocytes of the mouse neocortex express functional N-methyl-D-aspartate receptors. FASEB J. 15, 1270–1272. doi: 10.1096/fj.00-0439fje
Schmid, R., Sandler, A., and Katz, J. (1999). Use and efficacy of low-dose ketamine in the management of acute postoperative pain: a review of current techniques and outcomes. Pain 82, 111–125. doi: 10.1016/S0304-3959(99)00044-5
Scott, D. B., Blanpied, T. A., and Ehlers, M. D. (2003). Coordinated PKA and PKC phosphorylation suppresses RXR-mediated ER retention and regulates the surface delivery of NMDA receptors. Neuropharmacology 45, 755–767. doi: 10.1016/s0028-3908(03)00250-8
Sessle, B. J. (2021). Chronic orofacial pain: models, mechanisms and genetic and related environmental influences. Int. J. Mol. Sci. 22:7112. doi: 10.3390/ijms22137112
Shiiba, S., Sago, T., and Kawabata, K. (2021). Pain relief in short-lasting unilateral neuralgiform headache with conjunctival injection and tearing syndrome with intravenous ketamine: a case report. Acta Neurol. Taiwan 30, 35–38.
Shimizu, K., Chai, B., Lagraize, S. C., Wei, F., Dubner, R., and Ren, K. (2009a). Microinjection of IL-1β into the trigeminal transition zone produces bilateral NMDA receptor-dependent orofacial hyperalgesia involving descending circuitry. Open Pain J. 2, 76–83. doi: 10.2174/1876386300902010076
Shimizu, K., Guo, W., Wang, H., Zou, S., LaGraize, S. C., Iwata, K., et al. (2009b). Differential involvement of trigeminal transition zone and laminated subnucleus caudalis in orofacial deep and cutaneous hyperalgesia: the effects of interleukin-10 and glial inhibitors. Mol. Pain 5:75. doi: 10.1186/1744-8069-5-75
Shinoda, M., Kubo, A., Hayashi, Y., and Iwata, K. (2019). Peripheral and central mechanisms of persistent orofacial pain. Front. Neurosci. 13:1227. doi: 10.3389/fnins.2019.01227
Sivanesan, E., Maher, D. P., Raja, S. N., Linderoth, B., and Guan, Y. (2019). Supraspinal mechanisms of spinal cord stimulation for modulation of pain five decades of research and prospects for the future. Anesthesiology 130, 651–665. doi: 10.1097/ALN.0000000000002353
Song, X., Jensen, M. O., Jogini, V., Stein, R. A., Lee, C. H., McHaourab, H. S., et al. (2018). Mechanism of NMDA receptor channel block by MK-801 and memantine. Nature 556, 515–519. doi: 10.1038/s41586-018-0039-9
Srebro, D., Vuckovic, S., Milovanovic, A., Kosutic, J., Vujovic, K. S., and Prostran, M. (2017). Magnesium in pain research: state of the art. Curr. Med. Chem. 24, 424–434. doi: 10.2174/0929867323666161213101744
Sugiyo, S., Takemura, M., Dubner, R., and Ren, K. (2005). Trigeminal transition zone/rostral ventromedial medulla connections and facilitation of orofacial hyperalgesia after masseter inflammation in rats. J. Comp. Neurol. 493, 510–523. doi: 10.1002/cne.20797
Sun, Z., Meng, P., Su, C., Ji, S., Gao, Y., Wang, H., et al. (2021). PCC-0105002, a novel small molecule inhibitor of PSD95-nNOS protein-protein interactions, attenuates neuropathic pain and corrects motor disorder associated with neuropathic pain model. Toxicol. Appl. Pharmacol. 429:115698. doi: 10.1016/j.taap.2021.115698
Suo, Z.-W., Yang, X., Li, L., Liu, Y., Shi, L., and Hu, X. (2013). Inhibition of protein tyrosine phosphatases in spinal dorsal horn attenuated inflammatory pain by repressing Src signaling. Neuropharmacology 70, 122–130. doi: 10.1016/j.neuropharm.2013.01.015
Svensson, P., Cairns, B. E., Wang, K., Hu, J. W., Graven-Nielsen, T., Arendt-Nielsen, L., et al. (2003). Glutamate-evoked pain and mechanical allodynia in the human masseter muscle. Pain 101, 221–227. doi: 10.1016/S0304-3959(02)00079-9
Svensson, P., Castrillon, E., and Cairns, B. E. (2008). Nerve growth factor-evoked masseter muscle sensitization and perturbation of jaw motor function in healthy women. J. Orofac. Pain 22, 340–348.
Takehana, S., Kubota, Y., Uotsu, N., Yui, K., Iwata, K., Shimazu, Y., et al. (2017). The dietary constituent resveratrol suppresses nociceptive neurotransmission via the NMDA receptor. Mol. Pain 13:1744806917697010. doi: 10.1177/1744806917697010
Tang, J. S., Chiang, C. Y., Dostrovsky, J. O., Yao, D., and Sessle, B. J. (2021). Responses of neurons in rostral ventromedial medulla to nociceptive stimulation of craniofacial region and tail in rats. Brain Res. 1767:147539. doi: 10.1016/j.brainres.2021.147539
Tang, F. R., Yeo, J. F., and Leong, S. K. (2001). Qualitative light and electron microscope study of glutamate receptors in the caudal spinal trigeminal nucleus of the rat. J. Dent. Res. 80, 1736–1741. doi: 10.1177/00220345010800081101
Ting, K. K., Brew, B. J., and Guillemin, G. J. (2009). Effect of quinolinic acid on human astrocytes morphology and functions: implications in Alzheimer’s disease. J. Neuroinflammation 6:36. doi: 10.1186/1742-2094-6-36
Tingley, W. G., Roche, K. W., Thompson, A. K., and Huganir, R. L. (1993). Regulation of NMDA receptor phosphorylation by alternative splicing of the C-terminal domain. Nature 364, 70–73. doi: 10.1038/364070a0
Tochio, H., Mok, Y. K., Zhang, Q., Kan, H. M., Bredt, D. S., and Zhang, M. (2000). Formation of nNOS/PSD-95 PDZ dimer requires a preformed β-finger structure from the nNOS PDZ domain. J. Mol. Biol. 303, 359–370. doi: 10.1006/jmbi.2000.4148
Tognoli, E., Proto, P. L., Motta, G., Galeone, C., Mariani, L., and Valenza, F. (2020). Methadone for postoperative analgesia: contribution of N-methyl-d-aspartate receptor antagonism A randomised controlled trial. Eur. J. Anaesthesiol. 37, 934–943. doi: 10.1097/EJA.0000000000001217
Traynelis, S. F., Burgess, M. F., Zheng, F., Lyuboslavsky, P., and Powers, J. L. (1998). Control of voltage-independent zinc inhibition of NMDA receptors by the NR1 subunit. J. Neurosci. 18, 6163–6175. doi: 10.1523/JNEUROSCI.18-16-06163.1998
Traynelis, S. F., Wollmuth, L. P., McBain, C. J., Menniti, F. S., Vance, K. M., Ogden, K. K., et al. (2010). Glutamate receptor ion channels: structure, regulation and function. Pharmacol. Rev. 62, 405–496. doi: 10.1124/pr.109.002451
Vanegas, H., and Schaible, H. G. (2004). Descending control of persistent pain: inhibitory or facilitatory? Brain Res. Brain Res. Rev. 46, 295–309. doi: 10.1016/j.brainresrev.2004.07.004
Wang, S., Lim, G., Mao, J., Sung, B., and Mao, J. (2009). Regulation of the trigeminal NR1 subunit expression induced by inflammation of the temporomandibular joint region in rats. Pain 141, 97–103. doi: 10.1016/j.pain.2008.10.021
Wang, S., Song, L., Tan, Y., Ma, Y., Tian, Y., Jin, X., et al. (2012a). A functional relationship between trigeminal astroglial activation and NR1 expression in a rat model of temporomandibular joint inflammation. Pain Med. 13, 1590–1600. doi: 10.1111/j.1526-4637.2012.01511.x
Wang, S., Tian, Y., Song, L., Lim, G., Tan, Y., You, Z., et al. (2012b). Exacerbated mechanical hyperalgesia in rats with genetically predisposed depressive behavior: role of melatonin and NMDA receptors. Pain 153, 2448–2457. doi: 10.1016/j.pain.2012.08.016
Wang, H., Wei, F., Dubner, R., and Ren, K. (2006). Selective distribution and function of primary afferent nociceptive inputs from deep muscle tissue to the brainstem trigeminal transition zone. J. Comp. Neurol. 498, 390–402. doi: 10.1002/cne.21062
Wang, W., Zhong, X. X., Li, Y. Y., Guo, R. X., Du, S. J., Wen, L. L., et al. (2019). Rostral ventromedial medulla-mediated descending facilitation following P2X7 receptor activation is involved in the development of chronic post-operative pain. J. Neurochem. 149, 760–780. doi: 10.1111/jnc.14650
Weber, H., Klamer, D., Freudenberg, F., Kittel-Schneider, S., Rivero, O., Scholz, C. J., et al. (2014). The genetic contribution of the NO system at the glutamatergic post-synapse to schizophrenia: further evidence and meta-analysis. Eur. Neuropsychopharmacol. 24, 65–85. doi: 10.1016/j.euroneuro.2013.09.005
Wei, F., Wang, G., Kerchner, G., Kim, S., Xu, H., Chen, Z., et al. (2001). Genetic enhancement of inflammatory pain by forebrain NR2B overexpression. Nat. Neurosci. 4, 164–169. doi: 10.1038/83993
Westbrook, G. L., and Mayer, M. L. (1987). Micromolar concentrations of ZN2+ antagonize nmda and gaba responses of hippocampal-neurons. Nature 328, 640–643. doi: 10.1038/328640a0
Willis, W. D., Jr. (1985). Central nervous system mechanisms for pain modulation. Appl. Neurophysiol. 48, 153–165. doi: 10.1159/000101121
Wilson, T. D., Valdivia, S., Khan, A., Ahn, H. S., Adke, A. P., Gonzalez, S. M., et al. (2019). Dual and opposing functions of the central amygdala in the modulation of pain. Cell Rep. 29, 332–346.e5. doi: 10.1016/j.celrep.2019.09.011
Witt, A., Macdonald, N., and Kirkpatrick, P. (2004). Memantine hydrochloride. Nat. Rev. Drug Discov. 3, 109–110. doi: 10.1038/nrd1311
Woda, A., Blanc, O., Voisin, D. L., Coste, J., Molat, J. L., and Luccarini, P. (2004). Bidirectional modulation of windup by NMDA receptors in the rat spinal trigeminal nucleus. Eur. J. Neurosci. 19, 2009–2016. doi: 10.1111/j.0953-816X.2004.03328.x
Wolosker, H. (2006). D-serine regulation of NMDA receptor activity. Sci. STKE 2006:pe41. doi: 10.1126/stke.3562006pe41
Wong, H., Kang, I., Dong, X. D., Christidis, N., Ernberg, M., Svensson, P., et al. (2014). NGF-induced mechanical sensitization of the masseter muscle is mediated through peripheral NMDA receptors. Neuroscience 269, 232–244. doi: 10.1016/j.neuroscience.2014.03.054
Woolf, C. J., and Mannion, R. J. (1999). Neuropathic pain: aetiology, symptoms, mechanisms and management. Lancet 353, 1959–1964. doi: 10.1016/S0140-6736(99)01307-0
Woolley, C. S., Weiland, N. G., McEwen, B. S., and Schwartzkroin, P. A. (1997). Estradiol increases the sensitivity of hippocampal CA1 pyramidal cells to NMDA receptor-mediated synaptic input: correlation with dendritic spine density. J. Neurosci. 17, 1848–1859. doi: 10.1523/JNEUROSCI.17-05-01848.1997
Wu, L. J., Toyoda, H., Zhao, M. G., Lee, Y. S., Tang, J. R., Ko, S. W., et al. (2005). Upregulation of forebrain NMDA NR2B receptors contributes to behavioral sensitization after inflammation. J. Neurosci. 25, 11107–11116. doi: 10.1523/JNEUROSCI.1678-05.2005
Wu, Q. J., and Tymianski, M. (2018). Targeting NMDA receptors in stroke: new hope in neuroprotection. Mol. Brain 11:15. doi: 10.1186/s13041-018-0357-8
Xu, M., Kim, C., Neubert, M., and Heinricher, M. (2007). NMDA receptor-mediated activation of medullary pro-nociceptive neurons is required for secondary thermal hyperalgesia. Pain 127, 253–262. doi: 10.1016/j.pain.2006.08.020
Xu, Y. H., Zhang, G. J., Zhao, J. T., Chu, C. P., Li, Y. Z., and Qiu, D. L. (2017). Roles of N-methyl-d-aspartate receptors during the sensory stimulation-evoked field potential responses in mouse cerebellar cortical molecular layer. Neurosci. Lett. 660, 135–139. doi: 10.1016/j.neulet.2017.09.030
Yang, C. R., and Svensson, K. A. (2008). Allosteric modulation of NMDA receptor via elevation of brain glycine and D-serine: the therapeutic potentials for schizophrenia. Pharmacol. Ther. 120, 317–332. doi: 10.1016/j.pharmthera.2008.08.004
Yang, G. Y., Woo, Y. W., Park, M. K., Bae, Y. C., Ahn, D. K., and Bonfa, E. (2010). Intracisternal administration of NR2 antagonists attenuates facial formalin-induced nociceptive behavior in rats. J. Orofac. Pain 24, 203–211. doi: 10.1002/9780470640425.ch15
Yang, H.-B., Yang, X., Cao, J., Li, S., Liu, Y., Suo, Z., et al. (2011). cAMP-dependent protein kinase activated Fyn in spinal dorsal horn to regulate NMDA receptor function during inflammatory pain. J. Neurochem. 116, 93–104. doi: 10.1111/j.1471-4159.2010.07088.x
Ye, Z. Y., Li, L., Li, D. P., and Pan, H. L. (2012). Casein kinase 2-mediated synaptic GluN2A up-regulation increases N-methyl-D-aspartate receptor activity and excitability of hypothalamic neurons in hypertension. J. Biol. Chem. 287, 17438–17446. doi: 10.1074/jbc.M111.331165
Yi, F., Zachariassen, L. G., Dorsett, K. N., and Hansen, K. B. (2018). Properties of triheteromeric N-methyl-d-aspartate receptors containing two distinct GluN1 isoforms. Mol. Pharmacol. 93, 453–467. doi: 10.1124/mol.117.111427
Yin, J. B., Liang, S. H., Li, F., Zhao, W. J., Bai, Y., Sun, Y., et al. (2020). dmPFC-vlPAG projection neurons contribute to pain threshold maintenance and antianxiety behaviors. J. Clin. Investig. 130, 6555–6570. doi: 10.1172/JCI127607
Yousef, A. A., and Al-deeb, A. E. (2013). A double-blinded randomised controlled study of the value of sequential intravenous and oral magnesium therapy in patients with chronic low back pain with a neuropathic component. Anaesthesia 68, 260–266. doi: 10.1111/anae.12107
Yu, A., and Lau, A. Y. (2018). Glutamate and glycine binding to the NMDA receptor. Structure 26, 1035–1043.e2. doi: 10.1016/j.str.2018.05.004
Yu, X., Sessle, J. B., Haas, A. D., Izzo, A., Vernon, H., and Hu, W. J. (1996). Involvement of NMDA receptor mechanisms in jaw electromyographic activity and plasma extravasation induced by inflammatory irritant application to temporomandibular joint region of rats. Pain 68, 169–178. doi: 10.1016/S0304-3959(96)03181-8
Zhang, C. K., Li, Z. H., Qiao, Y., Zhang, T., Lu, Y. C., Chen, T., et al. (2018). VGLUT1 or VGLUT2 mRNA-positive neurons in spinal trigeminal nucleus provide collateral projections to both the thalamus and the parabrachial nucleus in rats. Mol. Brain 11:22. doi: 10.1186/s13041-018-0362-y
Zhang, Y. Y., Liu, F., Lin, J., Li, Y. L., Fang, Z. H., Zhou, C., et al. (2022). Activation of the N-methyl-D-aspartate receptor contributes to orofacial neuropathic and inflammatory allodynia by facilitating calcium-calmodulin-dependent protein kinase II phosphorylation in mice. Brain Res. Bull. 185, 174–192. doi: 10.1016/j.brainresbull.2022.05.003
Zhang, L., Rzigalinski, B. A., Ellis, E. F., and Satin, L. S. (1996). Reduction of voltage-dependent Mg2+ blockade of NMDA current in mechanically injured neurons. Science 274, 1921–1923. doi: 10.1126/science.274.5294.1921
Zhang, X. Y., Zhang, G. J., Li, B. X., Bing, Y. H., Cui, B. R., Cui, L. N., et al. (2020). NMDARs contribute to the facial stimuli-evoked mossy fiber-granule cell synaptic transmission in vivo in mice. Neurosci. Lett. 736:135285. doi: 10.1016/j.neulet.2020.135285
Zhao, Y. J., Liu, Y., Li, Q., Zhao, Y. H., Wang, J., Zhang, M., et al. (2015). Involvement of trigeminal astrocyte activation in masseter hyperalgesia under stress. Physiol. Behav. 142, 57–65. doi: 10.1016/j.physbeh.2015.02.005
Zhou, H.-Y., Chen, S.-R., and Pan, H.-L. (2011). Targeting N-methyl-D-aspartate receptors for treatment of neuropathic pain. Expert. Rev. Clin. Pharmacol. 4, 379–388. doi: 10.1586/ecp.11.17
Zhou, L., Li, F., Xu, H. B., Luo, C. X., Wu, H. Y., Zhu, M. M., et al. (2010). Treatment of cerebral ischemia by disrupting ischemia-induced interaction of nNOS with PSD-95. Nat. Med. 16, 1439–1443. doi: 10.1038/nm.2245
Zhu, S., and Paoletti, P. (2015). Allosteric modulators of NMDA receptors: multiple sites and mechanisms. Curr. Opin. Pharmacol. 20, 14–23. doi: 10.1016/j.coph.2014.10.009
Zhu, Z., Yi, F., Epplin, M. P., Liu, D., Summer, S. L., Mizu, R., et al. (2020). Negative allosteric modulation of GluN1/GluN3 NMDA receptors. Neuropharmacology 176:108117. doi: 10.1016/j.neuropharm.2020.108117
Zhuo, M. (2014). Long-term potentiation in the anterior cingulate cortex and chronic pain. Philos. Trans. R. Soc. Lond. B Biol. Sci. 369:20130146. doi: 10.1098/rstb.2013.0146
Keywords: N-methyl-D-aspartate receptor, chronic orofacial pain, peripheral sensitization, central sensitization, ascending systems, descending system, sex differences
Citation: Liu Y-J, Li Y-L, Fang Z-H, Liao H-L, Zhang Y-Y, Lin J, Liu F and Shen J-F (2022) NMDARs mediate peripheral and central sensitization contributing to chronic orofacial pain. Front. Cell. Neurosci. 16:999509. doi: 10.3389/fncel.2022.999509
Received: 21 July 2022; Accepted: 22 August 2022;
Published: 27 September 2022.
Edited by:
Daniela Pietrobon, University of Padua, ItalyReviewed by:
Rashid Giniatullin, University of Eastern Finland, FinlandParisa Gazerani, Oslo Metropolitan University, Norway
Copyright © 2022 Liu, Li, Fang, Liao, Zhang, Lin, Liu and Shen. This is an open-access article distributed under the terms of the Creative Commons Attribution License (CC BY). The use, distribution or reproduction in other forums is permitted, provided the original author(s) and the copyright owner(s) are credited and that the original publication in this journal is cited, in accordance with accepted academic practice. No use, distribution or reproduction is permitted which does not comply with these terms.
*Correspondence: Jie-Fei Shen, c2hlbmppZWZlaUBzY3UuZWR1LmNu; Fei Liu bGl1ZmVpLmh4a3FAc2N1LmVkdS5jbg==
† These authors have contributed equally to this work and share the first authorship