- 1Beijing International Science and Technology Cooperation Base for Antiviral Drugs, Faculty of Environment and Life, Beijing University of Technology, Beijing, China
- 2Department of Rehabilitation, Beijing Rehabilitation Hospital, Capital Medical University, Beijing, China
N6-methyladenosine (m6A) is the most abundant post-transcription modification, widely occurring in eukaryotic mRNA and non-coding RNA. m6A modification is highly enriched in the mammalian brain and is associated with neurological diseases like Alzheimer’s disease (AD) and Parkinson’s disease (PD). Ischemic stroke (IS) was discovered to alter the cerebral m6A epi-transcriptome, which might have functional implications in post-stroke pathophysiology. Moreover, it is observed that m6A modification could regulate microglia’s pro-inflammatory and anti-inflammatory responses. Given the critical regulatory role of microglia in the inflammatory processes in the central nervous system (CNS), we speculate that m6A modification could modulate the post-stroke microglial inflammatory responses. This review summarizes the vital regulatory roles of m6A modification in microglia-mediated inflammation and IS. Stroke is associated with a high recurrence rate, understanding the relationship between m6A modification and stroke may help stroke rehabilitation and develop novel therapies in the future.
Introduction
A stroke occurs when the blood supply to any portion of the brain stops, and it could happen due to blockage or injury to the blood vessels that carry blood to the brain. It is one of the leading causes of death and long-term impairment worldwide (Lo et al., 2003). Hemorrhagic and IS are the two main types of strokes. Hemorrhagic stroke is caused by a rupture of a blood vessel inside the brain, and ischemic stroke (IS) results due to the blockage of an artery in the brain, but both could lead to local hypoxia and damage to brain tissue (Barthels and Das, 2020). Among both types of strokes, IS account for roughly 80% of all stroke cases (Zheng et al., 2020). Various risk factors, including high blood pressure, obesity, and previous transient ischemic attack, could lead to an IS which could cause ischemic neuronal injuries such as glutamate excitotoxicity, oxidative stress, apoptosis, and neuroinflammation (Hankey, 2017; Dichgans et al., 2019).
Microglia/macrophages (MMs)-mediated neuroinflammatory responses could contribute to subsequent secondary damage following stroke (Liu et al., 2019b). MMs play a vital role in maintaining brain homeostasis, and these are the first line of defense against any damage to the central nervous system (CNS). In response to the variations in the cerebral microenvironment, microglia are activated and undergo morphological and functional changes. During the initial phase of IS, microglia are polarized to a protective anti-inflammatory phenotype and then gradually shifted to a toxic pro-inflammatory phenotype (Hu et al., 2012). It has been revealed that pro-inflammatory microglia trigger brain damage, hinder neurogenesis, and interfere with the recovery and repair of neurological function following stroke. On the contrary, the anti-inflammatory microglia could repair damaged tissues through various mechanisms, including neurogenesis, axonal remodeling, and remyelination (Bassett et al., 2021; Gaire, 2021). In vivo and in vitro studies showed that neuroprotection could be achieved by inhibiting the pro-inflammatory microglia and stimulating the activities of anti-inflammatory microglia (Liu et al., 2019b).
Many drugs have been reported to protect against brain injury by regulating microglia polarization and inflammatory responses in ischemia or hypoperfusion models (Liu et al., 2017, 2019b; Ran et al., 2021). Various physiological factors, such as hypothermia, could alter the microglia phenotype and provide neuroprotection in the state of stroke (Liu et al., 2018). Exploring the approaches that could shift microglia phenotype from pro-inflammatory to anti-inflammatory may provide the opportunity to develop novel therapeutic strategies for IS.
It is necessary to understand the occurrence and development of IS and determine its biological characteristics to improve its treatment efficiency. In recent years, it was reported that epigenetic modifications might dynamically regulate the changes in MM’s phenotype, which provided new clues for treating IS. Epigenetic modifications such as DNA methylation, histone acetylation, and non-coding RNA regulation have been identified for their close association with microglial polarization (Guo et al., 2016; Rigillo et al., 2018; Li et al., 2020). RNA methylation refers to the selective addition of methyl groups at different positions on RNA. N6-methyladenosine (m6A) and C5-methylcytidine (m5C) are the most common types of RNA methylation (Wang et al., 2015; Han et al., 2021). m6A RNA methylation occurs at nitrogen number six of the adenine base, and it is the most abundant chemical modification in eukaryotic messenger RNA (mRNA) and long-chain non-coding RNA (Filippova et al., 2019; Muller et al., 2019; van Tran et al., 2019; Ontiveros et al., 2020; Wen et al., 2020). It is known to modulate a variety of post-transcriptional modification processes such as RNA translation, splicing, and stability. Despite recent advances in m6A modification research, it is still worthwhile to discover new m6A sites and their functional capabilities. Reports showed that m6A modification plays a crucial role in the regulation of critical biological processes and pathogenesis of a variety of neurological diseases, such as Alzheimer’s disease (AD) (Han et al., 2020; Shafik et al., 2021), Parkinson’s disease (PD) (Chen et al., 2019; Qin et al., 2020; Qiu et al., 2020) and gliomas (Xu et al., 2020b; Zhang et al., 2020b). Recently, it has been reported that transient focal ischemia could alter the cerebral m6A epitranscriptome, which indicates the involvement of m6A modification in the onset of stroke (Chokkalla et al., 2019). Furthermore, studies have identified a distinct m6A epi-transcriptome in microglia. Microglia are critical regulators of inflammatory processes in the CNS. m6A modification could affect microglia-mediated inflammatory responses following stroke (Li et al., 2021).
This review summarizes the recent studies describing the role of m6A modification in IS and discusses the functions of m6A modification in microglia-mediated inflammation. Moreover, we also discuss the vital role of m6A modification in regulating the classical activation of microglia/macrophages (M1) and alternative activation of microglia/macrophages (M2). It has been noted that m6A modification could potentially play a role in inhibiting microglia-mediated inflammation following stroke. These findings may provide worthwhile insight into identifying m6A-related molecular biomarkers and therapeutic targets in stroke.
Reversible/dynamic m6A RNA methylation in neurological diseases
Reversible/dynamic regulation and physiological function of m6A RNA methylation
m6A is the most prevalent modification in eukaryotic RNAs (Meyer and Jaffrey, 2017). On average, every transcript is estimated to contain two or three m6A-modified sites (Wang et al., 2020b). m6A RNA methylation is reversible, and dynamically regulated by methyltransferases (Writers) and demethylases (Erasers) (Figure 1A; Zhao et al., 2020). m6A methyltransferases are generally referred to as m6A methylase complex. It contains methyltransferase-like 3 (METTL3), methyltransferase-like 14 (METTL14), Wilm’s tumor-associated protein (WTAP), RNA-binding motif protein 15 (RBM15 and RBM15B), and KIAA1429 or VIRMA (vir-like m6A methyltransferase associated) (Zaccara et al., 2019; Zhao et al., 2020). S-adenosyl methionine (SAM) is a universal methyl-group donor in DNA, RNA, and histone methylation. METTL3 could combine with SAM and mediate RNA methylation in the nucleus. METTL14 acts as an RNA-binding platform and facilitates methyltransferase activity by forming a heterodimer with METTL3 (Zhang, 2018). WTAP is an essential adaptor protein that stabilizes the METTL3-METTL14 complex, and RBM15/15B and KIAA1429 help recruit the m6A methylase complex (He et al., 2019; Chen and Wong, 2020; Chen et al., 2020). At present, fat mass and obesity-associated protein (FTO) and AlkBHomolog5 (ALKBH5) are the only two demethylases that have been identified to remove the m6A modification of nuclear RNA (Deng et al., 2018; Wei et al., 2018; Zhang et al., 2020b).
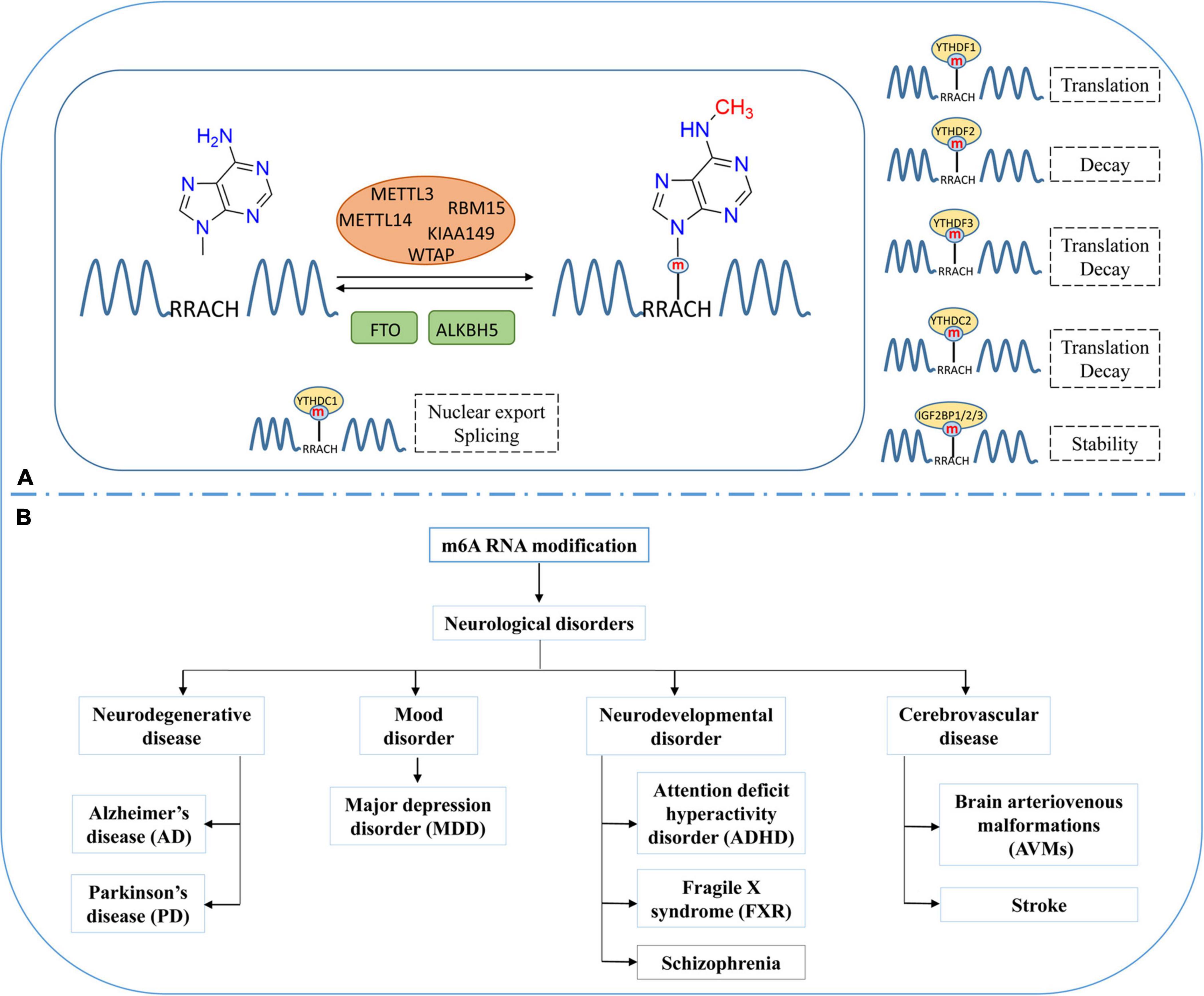
Figure 1. Dynamic and reversible regulation of m6A RNA modification and its role in neurological disorders. (A) Regulation of m6A modification by methyltransferases (METTL3, METTL14), demethylases (FTO and ALKBH5) and the effects of m6A modification on the processing, splicing, stability, and translation of mRNA. (B) Various neurological disorders regulated by m6A RNA modification.
m6A RNA methylation occurs in RRACH conserved sequences (R = A or G; and H = A, C, or U), frequently found near stop codons or 3′untranslated regions (3′UTRs). The function of m6A are regulated by m6A binding proteins (Readers), which can recognize and bind to the m6A modification sites at RNA and play an essential role in different biological functions (Lee et al., 2020). The YTH domain family, including YTH domain family protein 1–3 (YTHDF1/YTHDF2/YTHDF3) and YTH domain containing 1–2 (YTHDC1/YTHDC2), have m6A-binding domains that could bind to m6A modified RNA (Dai et al., 2021). YTHDF1 and YTHDF3 improve the translation efficiency of RNA by combining with translation initiation factor and ribosome (Liu et al., 2020; Shi et al., 2021). YTHDF2 is one of the most widely studied m6A binding proteins, which recognizes and combines with m6A modified mRNA to regulate its stability (Mapperley et al., 2021; Wang and Lu, 2021). Heterogeneous nuclear ribonucleoproteins (hnRNPs) are another kind of m6A binding protein. YTHDC1 and hnRNPs can recruit splicing factors to regulate pre-mRNA splicing (Martinez-Contreras et al., 2007; Kasowitz et al., 2018), whereas YTHDC2 accelerates the degradation of modified mRNA and enhances the translation of corresponding proteins via binding to targeted m6A modified RNA (Meyer and Jaffrey, 2017; Ma et al., 2019). Insulin-like growth factor-2 mRNA-binding proteins (IGF2BPs), including IGF2BP1/2/3, can identify m6A modification and promote mRNA stability and translation by protecting m6A-containing mRNA from degradation (Huang et al., 2018; Hu et al., 2020; Yang et al., 2020).
m6A methylation occurs after RNA transcription. In the nucleus, m6A modified RNA can be recognized by specific “Readers” that facilitate their splicing or nuclear export. After exporting to the cytoplasm, the cytoplasmic “reader” proteins could affect the translation, stability, and degradation of m6A modified mRNA (Jiang et al., 2021). Studies have shown that the m6A modification in RNA influences the regulation of many biological processes, including cell differentiation and proliferation, CNS and reproductive system development, and aging (Zhang et al., 2020a).
m6A RNA methylation in neurological diseases
RNA methylation occurs in abundance in the mammalian brain and involves in the regulation of synaptic plasticity, learning, memory, and neurogenesis (Mathoux et al., 2021). The imbalance of m6A modification could develop various neurological disorders such as neurodegenerative disorders, mood disorders, neurodevelopmental disorders, and cerebrovascular disease (Figure 1B; Dermentzaki and Lotti, 2020). Neurodegenerative disorders like AD and PD are characterized by progressive neuronal loss in the brain. As reported in AD and related dementias, the FTO gene showed a greater risk than the other m6A-associated genes. Reitz et al. (2012) found a significantly lower level of FTO in cortex and amygdala tissue obtained from AD patients than in controls individuals. Recently, Han et al. (2020) observed increased m6A modification in the AD mice models. This finding indicates that m6A modification could play a vital role in developing AD (Han et al., 2020). Moreover, it is observed that the increased expression of METTL3 and the decreased expression of FTO could increase the m6A modification rate in AD models (Han et al., 2020). In YTHDF1-knockout mice, ectopic expression of YTHDF1 could alleviate AD-related symptoms, including learning defects and weak memory (Dermentzaki and Lotti, 2020). PD is another common neurodegenerative disease. Recent studies have revealed the potential relationship between m6A and the pathogenesis of PD (Rasheed et al., 2021). Anti-apoptotic effects following FTO knockdown have been observed in PD cell models (Chen et al., 2019). Moreover, increased FTO expression promoted m6A demethylation and induced apoptosis. These findings indicate that m6A modification and its regulatory proteins can control the survival of dopaminergic neurons and influence the incidence of PD (Selberg et al., 2021).
Major depression disorder (MDD) is one of the common mood disorders, and literature shows that ALKHB5 activity could increase the risk of MDD (Du et al., 2015). Neurodevelopmental disorders are a group of disabilities that appear during early development, such as attention deficit hyperactivity disorder (ADHD), fragile X syndrome (FXR), and schizophrenia. Studies have shown the association of m6A modification or m6A-related proteins with these neurodevelopmental disorders (Choudhry et al., 2013; Yoon et al., 2017; Edens et al., 2019). In cerebrovascular disease, brain arteriovenous malformations (AVMs) are congenital vascular abnormality. Wang et al. (2020a) found that the expression of METTL3 and WTAP decreased in the larger pathological tissues of AVMs. Stroke affects the entire brain and its network properties and is considered a network neurological disease. Recently, many studies have shown that stroke is regulated by RNA methylation. Therefore, we summarize the recent studies describing the role of the m6A modification in stroke and microglia-mediated inflammation.
m6A RNA methylation in ischemic stroke
Epigenetic modifications, including DNA methylation, histone modification, and non-coding RNAs, could play a role in the pathogenesis of IS. The role of m6A modification in ischemic brain damage remained unclear. Recently, several studies showed that m6A modification could regulate the expression of inflammation-related genes during the stroke (Figure 2 and Table 1). Analyzing the genes and regulatory mechanisms related to m6A modification in IS may be of great significance for an in-depth understanding of the pathogenesis and rehabilitation of stroke.
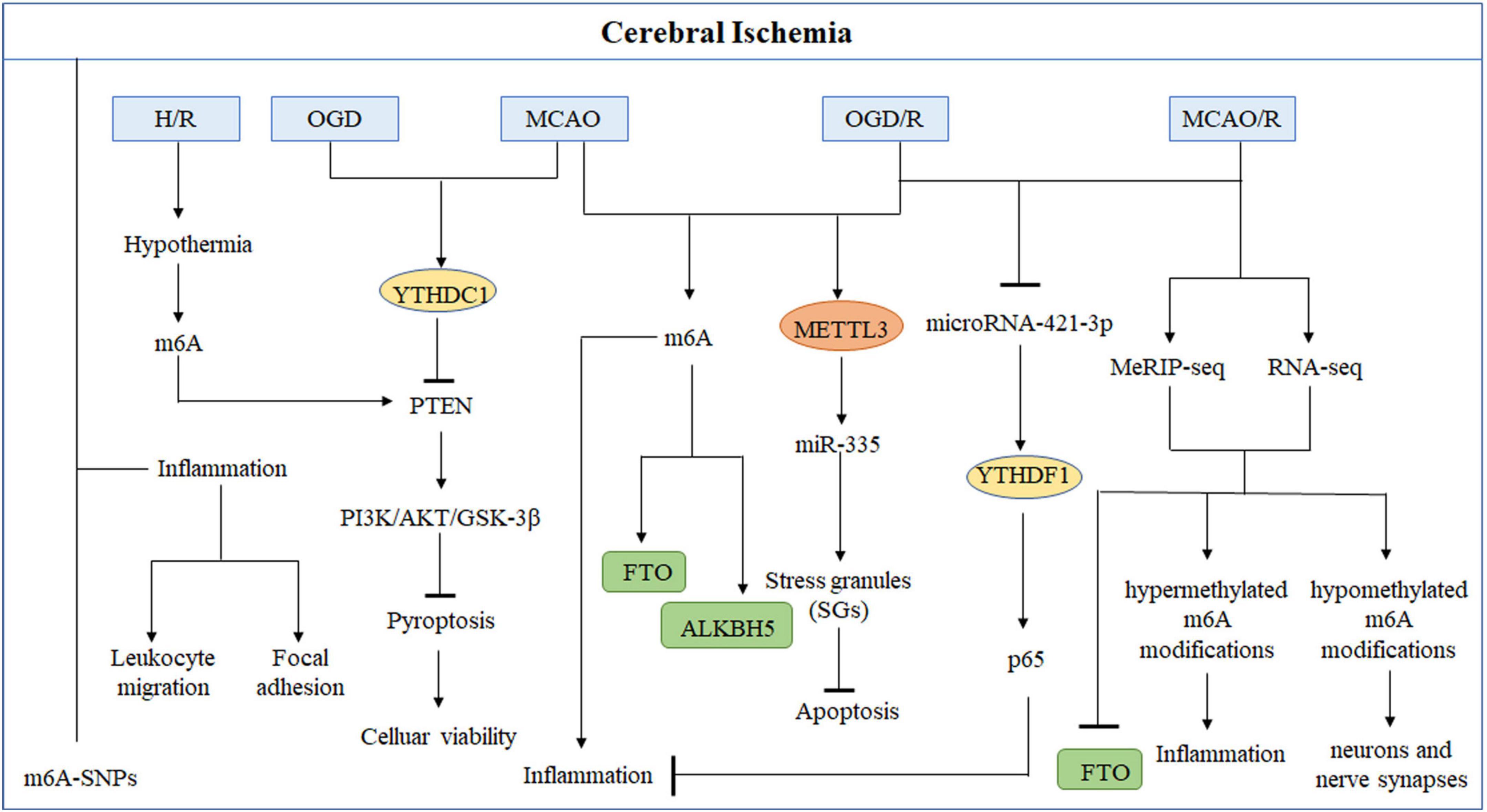
Figure 2. Role of m6A modifications in cerebral ischemia. Methyltransferases or demethylases regulated modulations in the levels of m6A modification and the effects of m6A binding proteins in the occurrence and development of stroke in different types of cerebral ischemia models.
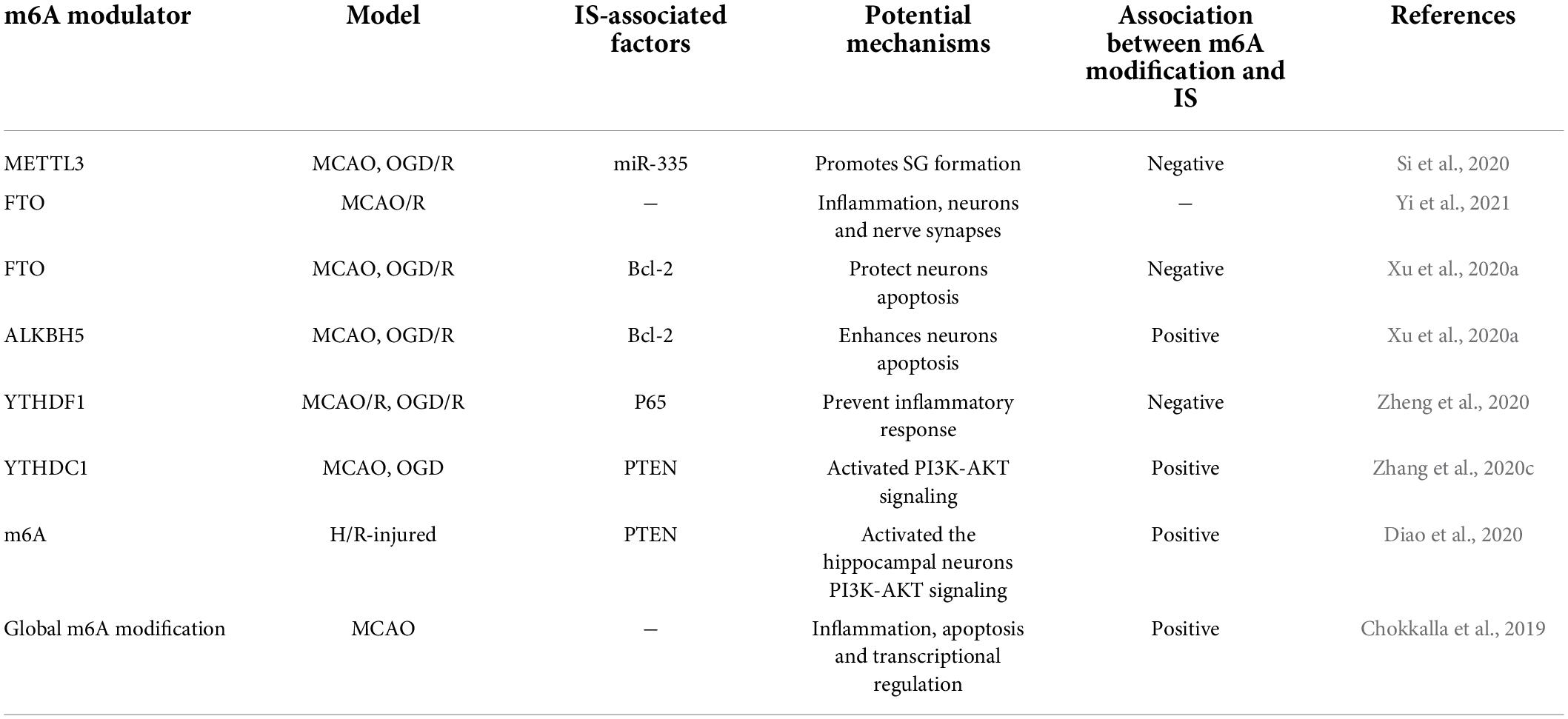
Table 1. The regulation of m6A RNA modification and its potential mechanism in ischemic stroke (IS).
Changes of m6A modification after ischemic stroke
It is observed that m6A modification increases in conditions like oxygen-glucose deprivation/reoxygenation (OGD/R) or middle cerebral artery occlusion and reperfusion (MCAO/R) (Chokkalla et al., 2019). Researchers found that the demethylases (ALKBH5 and FTO) primarily contribute to causing abnormal m6A modification in IS (Xu et al., 2020a; Yi et al., 2021). Yi et al. (2021) conducted a study in cerebral ischemia-reperfusion injury (CIRI) rat model to discover the variations in the m6A modifications and identified 1,160 differentially expressed genes having hypermethylated or hypomethylated m6A modifications. The genes that showed differential expression due to hypermethylation were found to participate in inflammation-related processes, while genes with hypomethylation modification were found to be associated with the functioning of neurons and nerve synapses. FTO, a demethylase, was explicitly present in neurons. Its downregulation was observed following MCAO/R, which caused elevation in the m6A level (Yi et al., 2021). Similarly, Xu et al. (2020a) found that in rat models, m6A levels increased with ALKBH5 expression in the cerebral cortex and primary neurons following MCAO and OGD/R, respectively. Moreover, FTO mRNA and protein levels were observed to be decreased in these rat models, which indicates that ALKBH5 and FTO work together to regulate m6A modification levels. ALKBH5 and FTO could selectively cause demethylation of Bcl-2 transcript; as a result, Bcl-2 expression is increased, which contributes to reduce neuronal damage. In the early stage of stroke, ischemia or exposure of neurons to hypoxia can temporarily halt the translation process, thereby reducing the development of cerebral ischemia and preventing additional damage. The translation arrest of proteins is essential for producing stress granules (SGs), which can immediately and temporarily halt mRNA translation. At an early stage of acute ischemic stroke (AIS), SGs will shield necessary mRNA and proteins from the potentially damaging environment, increasing cells’ survival (Aramburu-Nunez et al., 2022). Si et al. (2020) described that METTL3 promotes the maturation of miR-335, enhancing SG formation and reducing neuronal apoptosis. It showed that METTL3-mediated m6A methylation could alleviate AIS.
In these investigations, the m6A modification regulated by demethylases or methyltransferases was shown to play an essential role in IS. The incidence of stroke could increase intracellular m6A RNA methylation levels, which further regulates the expression of the stroke-related proteins and influences the activities of the corresponding signaling pathways. Therefore, m6A RNA methylation may bring new insights or prospective therapeutic options for IS in the future.
Changes of m6A modification- related inflammation after cerebral apoplexy
In IS, a lack of oxygen and energy might set off a chain reaction of physiological and pathological processes, such as an inflammatory response, glutamate excitotoxicity, oxidative stress, and apoptosis, which would ultimately lead to severe brain damage (Zhao et al., 2017). Following the stroke, inflammatory reactions start due to reduced blood flow, activation of intravascular immune cells, and the production of pro-inflammatory molecules by the damaged endothelium and brain parenchyma. Inflammation could contribute to worsening the ischemia-related damage. Blood vessel endothelium and platelets experience stress due to reduced blood flow, leading to intravascular congestion and ischemia (De Meyer et al., 2016). Clogging contributes to the coagulation cascades’ activation, which further generates inflammatory cues (Lambertsen et al., 2019). Coagulation cascades lead to intravascular inflammation, monocytes, and complement system activation through a series of downstream events. Intravascular inflammation could disrupt blood-brain barrier (BBB) (Szeplaki et al., 2009; Alawieh et al., 2015). A damaged BBB could allow the passage of activated complement proteins and immune cells to the brain parenchyma. As a result, inflammatory reactions occur in the brain parenchyma, which causes damage to neurons. Injured and dying neurons produce danger-/damage-associated molecular patterns (DAMP) (Garcia-Bonilla and Iadecola, 2012; Benakis et al., 2014; Petrovic-Djergovic et al., 2016; Singh et al., 2016).
Within hours of initiating ischemia, microglia respond to the changing environment (Zhang et al., 2011). Activated microglia penetrate the peri-infarcted and infarcted sites. Resident and invading cells regulate the inflammatory responses following stroke by interacting with one another and damaged neurons via signaling molecules, such as cytokines. At various phases following IS, inflammatory responses can be harmful and beneficial. Although it can contribute to increasing infarcts, it is also crucial for infarct clearance and influences remodeling and healing. Several preclinical and clinical investigations have demonstrated the efficacy of pharmaceutical therapies that target inflammation following IS. Targeting specific inflammatory molecules, such as interleukins (IL-1, IL-6, IL-10) and tumor necrosis factors, has shown significant therapeutic potential in preclinical settings (Lambertsen et al., 2019).
Neuroinflammation can eliminate cell injury and remove debris in the process of cerebral ischemia, and initiate tissue repairment. Therefore, it can be used as a therapeutic target for cerebral ischemia (Skaper et al., 2018). More and more studies have shown that different inflammation-related cells play an important role in different stages of cerebral ischemia. As inflammatory response occurs accompanied with cerebral ischemia, neuroinflammation may lead to a secondary neuronal damage, which will aggravate ischemia injury and impede the chronic recovery of brain function (Jurcau and Simion, 2021).
Despite recent advances in stroke research there is only a little research on m6A modification in pathological conditions, including IS and vascular inflammation, but what is known so far shows that it plays a crucial role in stroke and warrants more investigation. Zhu et al. (2021) examined the link between m6A single-nucleotide polymorphisms (m6A-SNPs) and the risk of IS using integrative analysis of genome-wide association studies (GWAS) and a list of single-nucleotide polymorphisms (SNPs) from the m6AVar database. More than 80 local genes (containing 87 m6A-SNPs) were discovered to be differentially expressed in IS patients in many biological processes, such as leukocyte migration and focal adhesion. Because m6A-SNPs are always adjacent to the methylation site, changes in m6A modification may impact the underlying biological process (Zheng et al., 2018). The earliest indicators of vascular inflammation include leukocyte migration and focal adhesion. The enrichment analysis revealed that m6A modification might have a role in the progression of IS by controlling vascular inflammation.
In recent years, neuronal inflammatory response and pyroptosis have been implicated in the etiology of CIRI and may provide a therapeutic target in the future. Chokkalla et al. (2019) established a transient ischemia-reperfusion (I/R) mouse model to investigate the transcriptome-wide m6A modification in CIRI. They found that mRNAs with various m6A modification were shown to be more prevalent in biological processes such as inflammation, apoptosis, and transcriptional regulation. Furthermore, the key inflammatory pathways that exhibited enhanced m6A RNA modification were cytokine, tumor necrosis factor (TNF), Toll-like receptor (TLR), and nuclear factor-kappa B (NF-κB) signaling. These are all known to affect the inflammation that occurs after a stroke. Diao et al. (2020) demonstrated that hypothermia can protect neurons from CIRI-induced proptosis via m6A-mediated activation of phosphatase and tensin homologous protein (PTEN). Furthermore, hypothermia can reduce inflammatory damage by lowering the expression levels of the NLRP3 (NLR pyrin domain containing 3) inflammasome and its related cytokines. In addition, the critical genes that were downregulated were mostly implicated in the cAMP signaling pathway, neuroactive ligand-receptor interaction, and the dopaminergic synapse (Yi et al., 2021). These findings indicate that the m6A modification could reduce the damage to neurons and synapses following CIRI, which provides new insight into the strategies for safeguarding neurons after CIRI. High-throughput sequencing and low-throughput investigations demonstrated that stroke can alter the levels of m6A in the brain and that demethylases FTO and ALKBH5 are involved in regulation, in addition to alterations in m6A recognition proteins, such as the YTH family. Zheng et al. (2020) found that microRNA-421-3p prevents inflammatory response in CIRI by targeting YTHDF1 to inhibit p65 mRNA translation. Moreover, Zhang et al. (2020c) confirmed that knockdown of YTHDC1 could exacerbate ischemic brain injury. Mechanistically, YTHDC1 facilitated neuronal survival following ischemia by promoting the degradation of PTEN mRNA, which led to an increase in Akt phosphorylation. In addition, it was found that m6A modifications in inflammation-related genes altered in stroke, which led to the activation of inflammation-related pathways (Zhang et al., 2020c).
In a nutshell, elucidating the function of m6A modification in the inflammatory response may yield valuable evidence for molecular targeting research, which may improve the treatment of inflammation-mediated cerebral apoplexy.
Role of microglia-mediated inflammation in ischemic stroke
Inflammation is one of the primary causes of IS, induced by developing reactive oxygen species (ROS), necrotic cells, or damaged tissues following cerebral ischemia (Khoshnam et al., 2017). Inflammation could cause neuronal apoptosis and brain injury in the acute phase of an IS through excitotoxicity, cytolysis, oxidative stress, and thrombotic inflammation (Shi et al., 2019). In IS models, microglia activation varies and is proportional to the severity of ischemia at various phases (Yu et al., 2021). Similarly, clinical trials demonstrated that microglial activation could occur in the acute, subacute, and chronic stages of IS (Qin et al., 2019). Numerous studies have shown that neuroinflammation caused by cerebral ischemia can influence the progression of cerebral ischemia in its later stages (Jayaraj et al., 2019). Therefore, neuroinflammation could be one of the therapeutic targets of cerebral ischemia that develops in the brain following ischemia.
MMs are the primary immune cells defending against CNS damage and are crucial in regulating brain homeostasis (Liu et al., 2019b; Qiu et al., 2021). After cerebral ischemia, local microglia and peripheral macrophages are quickly activated and regulate neuropathological processes to carry out functional recovery (Zhao et al., 2017; Li and Barres, 2018). Activated MMs play an important role in the pathological process of ischemic brain injury. To accomplish their diverse roles, MMs exhibit remarkable plasticity and can exhibit distinct active phenotypes depending on the microenvironment. There are two main phenotypes of activated MMs: pro-inflammatory (M1) and anti-inflammatory (M2) phenotypes. M1-like MMs have been shown to breach the BBB and aggravate neurological impairments by producing inflammatory cytokines like TNF, IL-1β, and inducible nitric oxide synthase (iNOS). In contrast, M2-like MMs support tissue repair by clearing cell debris, promoting neurogenesis, angiogenesis, axon regeneration, and releasing anti-inflammatory cytokines such as arginase-1 (Arg-1) and IL-10, and neurotrophic factors (Prinz et al., 2021; Qiu et al., 2021). Therefore, most of the current studies focus on the ways to inhibit M1 and/or enhance M2 phenotype to control the activation of microglia, to limit inflammatory injury and promote tissue repair to treat IS and improve neuronal survival.
m6A RNA methylation in microglia/macrophage-mediated inflammation
Neuroinflammation is the inflammatory response occurs in CNS, and is closely related to the occurrence of neurological diseases (Hickman et al., 2018). In the pathological process of AD, the level of inflammatory markers were increased and aggravates the brain damage, which indicated that neuroinflammation has a prominent role in the pathogenesis of AD (Leng and Edison, 2021). Suppression of microglial activity in AD mice models improved neurogenesis and enhanced cognitive functions (Biscaro et al., 2012). Moreover, Growing evidence indicates that microglia-mediated inflammation also participates in the process of PD (Zhang et al., 2017; Saha et al., 2021). The findings suggest that microglia activation-mediated modulations in immune responses could play a significant role in progression of neurodegenerative disorders. During stroke occurrence and development, cerebral inflammation plays important roles and was seem as a breakthrough in treatment of stroke (Lyu et al., 2021). Recently, it is showed that epigenetics could regulate the inflammatory response, which suggests novel understanding in inflammation research (Qiu et al., 2021). To better understand the role of m6A modification in the inflammatory response in the brain, we then focus on the microglia/macrophages that generate an inflammatory response in the brain to gain new insights into neuroprotection following cerebral ischemia.
m6A regulates the inflammatory response of microglia
Microglia act as vital communicators between CNS and immune systems. Li et al. (2021) created primary rat microglia models differentiated into M1, M2, and M0 (resting and unstimulated)-like phenotypes (M1-L, M2-L, M0-L) and compared their m6A modification profile of mRNA and lncRNA to demonstrate the regulatory role of m6A modification in microglia polarization. The findings revealed that 87 m6A-associated lncRNAs were hyper-methylated in M1-L/M0-L and hypo-methylated in M2-L/M1-L, with just three hypo-methylated lncRNAs in M1-L/M0-L and hyper-methylated in M2-L/M1-L. Moreover, pro-inflammatory M1-L exhibited the most remarkable shift in m6A RNA modification. KEGG analysis showed that nine overly expressed mRNAs with hyper-methylation in M1-L/M0-L were involved in 10 significantly upregulated pathways, such as signal transduction, immune system processing, and protein degradation. These pathways may represent diverse pro-inflammatory processes that can occur during microglial activation. These findings suggest that the lncRNA and mRNA with variable methylation can regulate the inflammatory responses in microglia by modulating immune system functioning and signal transduction pathways.
Inflammation, a vital component of the immune response, helps the body recover from damage or infection and maintains tissue homeostasis in potentially dangerous circumstances. On the other hand, if it continues uncontrollably, it could cause more tissue damage, chronic inflammatory, and autoimmune illnesses, and ultimately organ loss. Studies have demonstrated that microglia are the predominant cells responsible for the inflammatory response in the brain in the majority of neurodegenerative disorders (Smith et al., 2012; Tang and Le, 2016). Lipopolysaccharide (LPS), an early inflammation trigger, can be identified explicitly by Toll-like receptor 4 (TLR4) to activate intracellular signal transduction. Hence immunological and inflammatory responses are triggered. It has been reported that following LPS exposure, microglia activate and subsequently produce a large number of inflammatory agents and other cytotoxic chemicals, which leads to the death of dopaminergic neurons and significant damage to CNS (Zhou and Spittau, 2018). In microglial inflammation induced by LPS, the expression of METTL3 and the inflammatory cytokines were observed to be elevated (Ran et al., 2021; Wen et al., 2022). The tumor necrosis factor receptor-associated factor (TRAF) is a crucial binding protein in the TNF and Toll/IL-1 receptor (TIR) families. TRAF6, as a signal transduction factor, is also vital in the initiation of numerous signal cascades. NF-κB is a well-known pro-inflammatory signaling pathway and is considered an essential component in the regulation of both immunity and inflammation. Recent studies have stated that the TRAF6-NF-κB signaling mechanism significantly affects a variety of inflammatory responses (Allen et al., 2011). Wen et al. (2022) discovered that the level of METTL3 had a positive correlation with TRAF6, and METTL3 overexpression had the potential to activate the TRAF6-NF-kB pathway in an m6A-dependent manner, which would ultimately lead to microglial inflammation. Therefore, m6A modification could be a significant regulator during the immunological response of microglia.
m6A participates in macrophage polarization and inflammation
The polarization of macrophages is the driving force behind various inflammatory disorders, particularly those associated with an M1/M2 imbalance (Atri et al., 2018; Figure 3). Several transcription factors have been identified as essential regulators of macrophage polarization, including hypoxia-inducible factor-1 alpha (HIF-1α) and peroxisome proliferator activator receptor-γ (PPAR-γ), signal transducer and activator of transcription 1 (STAT1) and STAT6 (Funes et al., 2018). There is evidence that interferon-gamma (IFN-γ) could activate Jak-STAT1 signaling and promotes STAT1 phosphorylation, which leads to the polarization of M1-like macrophages. STAT6 signaling could trigger the expression of ligand-dependent PPAR-γ and promotes M2 polarization by promoting the expression of several gene subsets associated with anti-inflammatory function. The polarization of M1/M2 macrophages is driven by m6A modification. Due to a significant regulator of macrophage polarization, m6A modification may be utilized as an anti-inflammatory target. Gu et al. (2020) found that FTO silencing significantly inhibited the polarization of M1 and M2. FTO knockdown was observed to cause a significant decrease in the expression of STAT1 in M1 polarized macrophages and the expression of STAT6 and PPAR-γ in M2 polarized macrophages. Similarly, FTO knockdown caused the inhibition of the NF-κB signaling pathway and reduced the stability of STAT1 mRNA and PPAR-γ mRNA by activating YTHDF2, thus hindering the activation of macrophages (Gu et al., 2020). Liu et al. (2019a) found that METTL3 could cause M1 macrophage polarization through direct methylation of STAT1 mRNA. METTL3 knockdown significantly inhibited M1 polarization but enhanced M2 polarization. In addition, METTL3-mediated STAT1 mRNA methylation significantly increased the stability of mRNA and subsequently upregulated the expression of STAT1. Wang et al. (2021) observed that the polarization of M1 was enhanced by the IGF2BP2 knockdown in macrophages. Meanwhile, IGF2BP2 tended to transform the M1 phenotype into the M2 phenotype in an m6A-dependent manner. Moreover, it was also discovered that the IL-4-Jak-STAT6 axis could carry out the differentiation of M2 macrophages. Huangfu et al. (2020) found that RNA-binding motif 4 (RBM4) could interact with YTHDF2 to degrade STAT1 mRNA, thereby inhibiting the polarization of M1 macrophages. These findings prove that m6A modification plays a significant role in the polarization of macrophages.
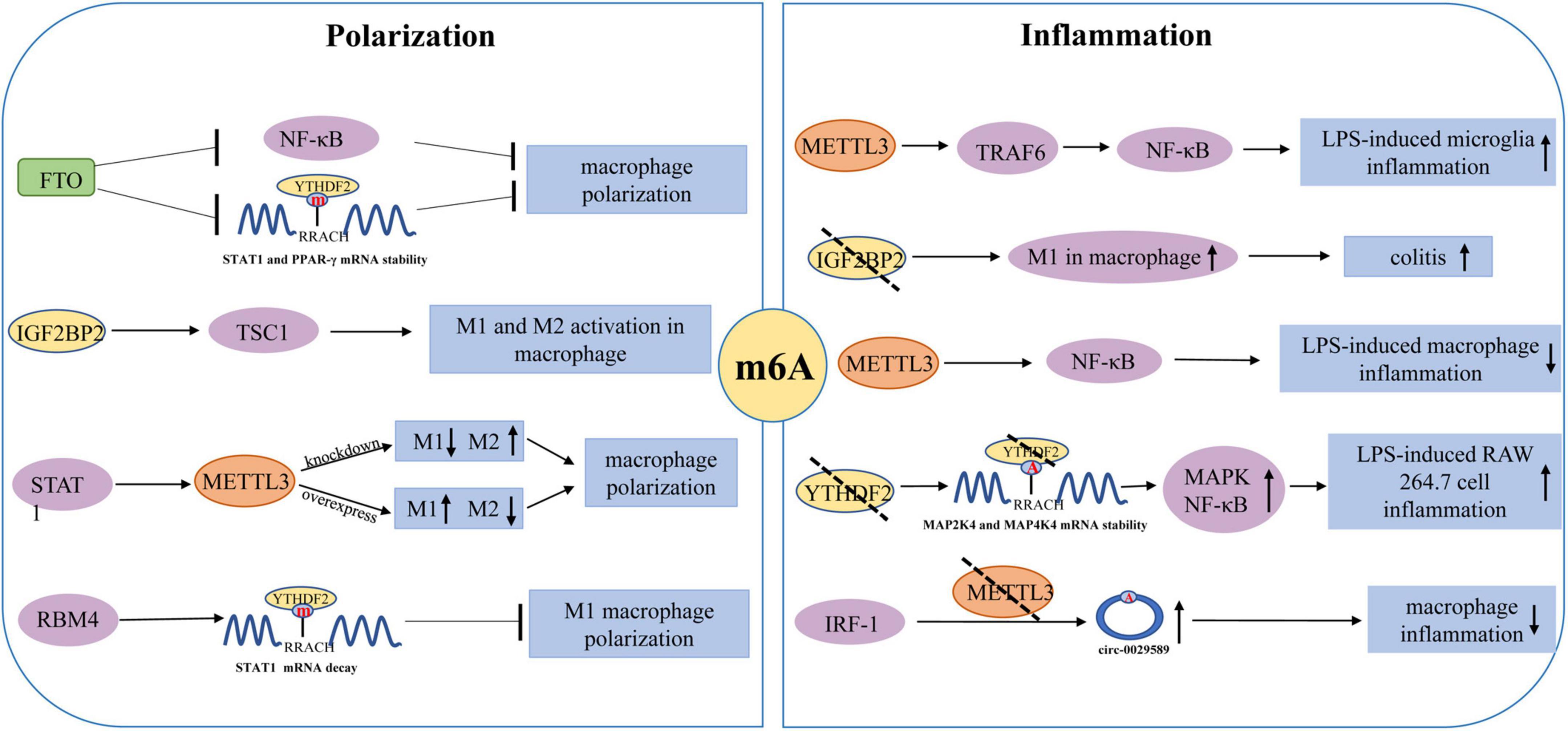
Figure 3. Regulatory role of m6A modification in the polarization of macrophages and macrophages/microglia mediated inflammation.
Moreover, an in vitro study showed that LPS could promote the expression and biological activity of METTL3 in macrophages (Wang et al., 2019b). However, the overexpression of METTL3 can attenuate LPS-induced inflammation through the NF-κB signaling pathway. Yu et al. (2019) reported that YTHDF2 knockdown could increase the expression of MAP2K4, which results in the activation of MAPK and NF-κB signaling pathways. This activation leads to the enhanced expression of pro-inflammatory cytokines and aggravates the inflammatory responses of the cells. Pyroptosis, a kind of programmed cell death, occurs due to the release of many pro-inflammatory factors in response to the inflammatory reactions (Robinson et al., 2019). Guo et al. (2020) demonstrated that m6A modification in circRNA (hsa_circ_0029589) could enable IFN Regulatory Factor-1 (IRF-1) to induce macrophage pyroptosis in patients with the acute coronary syndrome (ACS). High levels of m6A modification in circRNA (hsa_circ_0029589) caused a decrease in circRNA (hsa_circ_0029589) expression and an increase in METTL3 expression in macrophages of ACS patients. On the contrary, METTL3 inhibition significantly increased the expression of circRNA (hsa_circ_0029589) and alleviated the pyroptosis of macrophages. However, additional research is required to investigate the underlying processes of m6A modification in macrophage pyroptosis-mediated inflammation.
Therapeutic potential
m6A RNA modification is the most abundant type of epigenetic change in eukaryotic RNA. The function of m6A modification and its regulation in human disorders have undergone significant development over the past decade. It is notably true in neurological diseases caused by the enrichment of m6A in mammalian brains. Chokkalla et al. (2019) demonstrated for the first time that the global m6A levels in the whole cerebral transcriptome were altered after stroke. Many studies have reported that IS could occur due to imbalanced m6A RNA modification (Table 1). Recently, several studies exhibited that IS models have high levels of increase in m6A methylation, and m6A is found to reduce stroke-related damage. The regulation of m6A “Writers,” “Erasers,” and “Readers” may slow the progression of IS, and these findings may offer new insights into potential biomarkers or treatment options for stroke diagnosis and management. Many studies indicated that the up-regulated m6A level in IS is closely associated with the decreased expression of the demethylase FTO (Yi et al., 2021). Therefore, modulations in m6A levels and expression of FTO may be considered a bio-marker of IS development. Different studies reported the dual relationship, positive or negative, between m6A and IS, which may be due to the utilization of dissimilar IS models or targeting different m6A modified RNA. The level of m6A can affect the survival of neurons in the OGD/R model. Knockout of ALKBH5 could promote neuronal apoptosis, while the overexpression of FTO could promote the survival of neurons, indicating the neuroprotective role of FTO. At present, m6A modification regulatory drugs may be utilized for IS management. Recently it has been reported that cycloleucine (CL) is one kind of m6A inhibitor that can reduce nerve necrosis by inhibiting m6A modification (Xu et al., 2020a). Moreover, as a methyl donor, betaine can significantly increase the global m6A level and enhance the I/R brain injury by increasing m6A modification. Given the IS-regulatory role of m6A, using m6A inhibitory drugs may aid in managing IS.
The full transcriptome map of m6A in mRNA may help classify m6A targets and help understand the potential epigenetic mechanisms. Some studies have explained the effect of m6A-SNP on IS and identified the biological processes related to the pathogenesis of IS, such as inflammatory response. MeRIP-seq in the stroke model is also an important method for studying the pathogenesis of IS. Zhu et al. (2021) discovered that the primary biological processes that regulate post-stroke m6A differential methylation are inflammation, apoptosis, and transcriptional regulation. Other studies identified that m6A-influenced differential gene expression following stroke could affect inflammation, neuronal activity, and synaptic processes. In our study, we primarily focused on the regulatory role of m6A modification in the inflammatory process and its effects on inhibition or occurrence and progression of IS. The m6A-SNPs or MeRIP-seq mediated identification of the regulatory role of m6A-associated genes or signaling pathways in IS may help find potential biomarkers and therapeutic targets for IS.
Neuroinflammation is a complex pathophysiological process that includes microglial activation and the release of inflammatory cytokines. It is a significant contributor to neuronal damage and the pathogenesis of IS. Recent studies have shown that m6A modifications can promote neuroinflammation after a stroke and reduce the severity of the harm by controlling the inflammatory response. m6A modification plays a significant role in several inflammatory diseases via regulating various pathways, but the understanding of the inflammatory response in the disorders of the nervous system is insufficient. One of the most significant advances in treating stroke injury is identifying a secondary inflammatory reaction that occurs after a stroke. The investigation of m6A modification in inflammatory response could enable the development of effective drugs with particular targets. Curcumin is a yellow polyphenolic pigment extracted from turmeric. It is reported that curcumin has a protective effect on LPS-induced liver injury and lipid metabolism disorder (Lu et al., 2018). Dietary curcumin could control the mRNA expression of METTL3, METTL14, ALKBH5, FTO, and YTHDF2. It can also enhance the level of m6A modification and protect the liver from damage caused by inflammation and metabolic disorders. In addition, the natural product Rhein has been identified as an inhibitor of FTO. It could competitively bind to FTO active sites. It has been found that Rhein inhibited the ATP-induced inflammation in fibroblast-like synoviocytes in rheumatoid rat models and improved experimental colitis (Hu et al., 2019). Rhein treatment was also discovered to inhibit inflammatory lung injury caused by the human respiratory syncytial virus. A literature review showed that few studies investigated the regulatory function of m6A methylation in neuro-inflammation and its potential role in stroke treatment. In this review, we discussed some critical m6A regulatory factors which can change the phenotype and function of microglia/macrophages. There is still a need to conduct in-depth investigations of the regulatory role of m6A methylation in post-stroke neuro-inflammation. It could help us devise m6A modification-related novel therapeutic approaches to alleviate post-stroke inflammatory responses mediated damages.
In addition, aberrant m6A modification is involved in additional ischemia cascade activities, such as oxidative stress and apoptosis. In cerebral ischemia, plenty of reactive ROS are produced and cause oxidative stress and tissue damage. A study reported that METTL3-mediated m6A modification positively affects miR-873-5p, which regulates the Keap1-Nrf2 pathway and reduces oxidative stress (Wang et al., 2019a). It suggests that METTL3 may protect neurons from oxidative stress-induced damage, and it can be utilized as a treatment option to overcome oxidative stress produced by stroke. Excess glutamate synthesis after stroke promotes Ca2+ influx, which could cause excitotoxicity and neuronal damage. However, the role of m6A modification in glutamate-mediated excitotoxicity is not yet fully elucidated and requires further investigation.
m6A modification and its precise regulation in stroke remain mostly unclear and requires additional studies. Current investigations suggest that m6A modification may enable the identification of prospective targets for the treatment of stroke, leading to the development of promising m6A inhibitors or agonists for clinical use in the future. Furthermore, inhibition of post-stroke inflammation by targeting m6A methylation could help improve stroke-related injuries. We believe that m6A methylation may provide new insights into the molecular mechanism of IS and may serve as a potential therapeutic target for IS therapy.
Conclusion
The dynamic and reversible m6A RNA methylation proved to have a significant regulatory role in crucial biological processes in eukaryotes. Recent studies have revealed that the dysregulated m6A methylation process or abnormal m6A levels are closely related to the pathogenesis of IS. Moreover, m6A modification can facilitate the activation and polarization of microglia/macrophages and could also play a regulatory role in microglia-induced inflammatory responses following stroke. The interaction between m6A methylation and IS could be helpful in a better understanding of the pathogenesis of IS and discovering novel therapeutic targets for IS treatment.
Author contributions
XC raised the idea for the article, performed literature search, and revised the manuscript. FZ, YR, and MT wrote the first draft of the manuscript. ZL and JW revised the work. All authors contributed to the article and approved the submitted version.
Funding
This work was supported by the Beijing Municipal Natural Science Foundation (No. 7202001), the Scientific Research Project of Beijing Educational Committee (No. KM202010005022), and the National Natural Science Foundation of China (No. 81400935).
Conflict of interest
The authors declare that the research was conducted in the absence of any commercial or financial relationships that could be construed as a potential conflict of interest.
Publisher’s note
All claims expressed in this article are solely those of the authors and do not necessarily represent those of their affiliated organizations, or those of the publisher, the editors and the reviewers. Any product that may be evaluated in this article, or claim that may be made by its manufacturer, is not guaranteed or endorsed by the publisher.
References
Alawieh, A., Elvington, A., and Tomlinson, S. (2015). Complement in the homeostatic and ischemic brain. Front. Immunol. 6:417. doi: 10.3389/fimmu.2015.00417
Allen, I. C., Moore, C. B., Schneider, M., Lei, Y., Davis, B. K., Scull, M. A., et al. (2011). NLRX1 protein attenuates inflammatory responses to infection by interfering with the RIG-I-MAVS and TRAF6-NF-kappaB signaling pathways. Immunity 34, 854–865. doi: 10.1016/j.immuni.2011.03.026
Aramburu-Nunez, M., Custodia, A., Perez-Mato, M., Iglesias-Rey, R., Campos, F., Castillo, J., et al. (2022). Stress granules and acute ischemic stroke: beyond mRNA translation. Int. J. Mol. Sci. 23:3747. doi: 10.3390/ijms23073747
Atri, C., Guerfali, F. Z., and Laouini, D. (2018). Role of human macrophage polarization in inflammation during infectious diseases. Int. J. Mol. Sci. 19:1801. doi: 10.3390/ijms19061801
Barthels, D., and Das, H. (2020). Current advances in ischemic stroke research and therapies. Biochim. Biophys. Acta Mol. Basis Dis. 1866:165260. doi: 10.1016/j.bbadis.2018.09.012
Bassett, B., Subramaniyam, S., Fan, Y., Varney, S., Pan, H., Carneiro, A. M. D., et al. (2021). Minocycline alleviates depression-like symptoms by rescuing decrease in neurogenesis in dorsal hippocampus via blocking microglia activation/phagocytosis. Brain Behav. Immun. 91, 519–530. doi: 10.1016/j.bbi.2020.11.009
Benakis, C., Garcia-Bonilla, L., Iadecola, C., and Anrather, J. (2014). The role of microglia and myeloid immune cells in acute cerebral ischemia. Front. Cell Neurosci. 8:461. doi: 10.3389/fncel.2014.00461
Biscaro, B., Lindvall, O., Tesco, G., Ekdahl, C. T., and Nitsch, R. M. (2012). Inhibition of microglial activation protects hippocampal neurogenesis and improves cognitive deficits in a transgenic mouse model for Alzheimer’s disease. Neurodegener. Dis. 9, 187–198. doi: 10.1159/000330363
Chen, M., and Wong, C. M. (2020). The emerging roles of N6-methyladenosine (m6A) deregulation in liver carcinogenesis. Mol. Cancer 19:44. doi: 10.1186/s12943-020-01172-y
Chen, X., Yu, C., Guo, M., Zheng, X., Ali, S., Huang, H., et al. (2019). Down-Regulation of m6A mRNA methylation is involved in dopaminergic neuronal death. ACS Chem. Neurosci. 10, 2355–2363. doi: 10.1021/acschemneuro.8b00657
Chen, Y., Lin, Y., Shu, Y., He, J., and Gao, W. (2020). Interaction between N(6)-methyladenosine (m(6)A) modification and noncoding RNAs in cancer. Mol. Cancer 19:94. doi: 10.1186/s12943-020-01207-1204
Chokkalla, A. K., Mehta, S. L., Kim, T., Chelluboina, B., Kim, J., and Vemuganti, R. (2019). Transient focal ischemia significantly alters the m(6)A epitranscriptomic tagging of RNAs in the brain. Stroke 50, 2912–2921. doi: 10.1161/STROKEAHA.119.026433
Choudhry, Z., Sengupta, S. M., Grizenko, N., Thakur, G. A., Fortier, M. E., Schmitz, N., et al. (2013). Association between obesity-related gene FTO and ADHD. Obesity 21, E738–E744. doi: 10.1002/oby.20444
Dai, X. Y., Shi, L., Li, Z., Yang, H. Y., Wei, J. F., and Ding, Q. (2021). Main N6-Methyladenosine readers: YTH family proteins in cancers. Front. Oncol. 11:635329. doi: 10.3389/fonc.2021.635329
De Meyer, S. F., Denorme, F., Langhauser, F., Geuss, E., Fluri, F., and Kleinschnitz, C. (2016). Thromboinflammation in stroke brain damage. Stroke 47, 1165–1172. doi: 10.1161/STROKEAHA.115.011238
Deng, X., Su, R., Weng, H., Huang, H., Li, Z., and Chen, J. (2018). RNA N(6)-methyladenosine modification in cancers: current status and perspectives. Cell Res. 28, 507–517. doi: 10.1038/s41422-018-0034-36
Dermentzaki, G., and Lotti, F. (2020). New insights on the role of N (6)-methyladenosine RNA methylation in the physiology and pathology of the nervous system. Front. Mol. Biosci. 7:555372. doi: 10.3389/fmolb.2020.555372
Diao, M. Y., Zhu, Y., Yang, J., Xi, S. S., Wen, X., Gu, Q., et al. (2020). Hypothermia protects neurons against ischemia/reperfusion-induced pyroptosis via m6A-mediated activation of PTEN and the PI3K/Akt/GSK-3beta signaling pathway. Brain Res. Bull. 159, 25–31. doi: 10.1016/j.brainresbull.2020.03.011
Dichgans, M., Pulit, S. L., and Rosand, J. (2019). Stroke genetics: discovery, biology, and clinical applications. Lancet Neurol. 18, 587–599. doi: 10.1016/s1474-4422(19)30043-30042
Du, T., Rao, S., Wu, L., Ye, N., Liu, Z., Hu, H., et al. (2015). An association study of the m6A genes with major depressive disorder in Chinese Han population. J. Affect. Disord. 183, 279–286. doi: 10.1016/j.jad.2015.05.025
Edens, B. M., Vissers, C., Su, J., Arumugam, S., Xu, Z., Shi, H., et al. (2019). FMRP modulates neural differentiation through m(6)A-dependent mRNA nuclear export. Cell Rep. 28, 845–854.e5. doi: 10.1016/j.celrep.2019.06.072
Filippova, J. A., Matveeva, A. M., Zhuravlev, E. S., Balakhonova, E. A., Prokhorova, D. V., Malanin, S. J., et al. (2019). Are small nucleolar RNAs “CRISPRable”? a report on box C/D small nucleolar RNA editing in human cells. Front. Pharmacol. 10:1246. doi: 10.3389/fphar.2019.01246
Funes, S. C., Rios, M., Escobar-Vera, J., and Kalergis, A. M. (2018). Implications of macrophage polarization in autoimmunity. Immunology 154, 186–195. doi: 10.1111/imm.12910
Gaire, B. P. (2021). Microglia as the critical regulators of neuroprotection and functional recovery in cerebral ischemia. Cell Mol. Neurobiol. 92, 293–315. doi: 10.1007/s10571-021-01145-1149
Garcia-Bonilla, L., and Iadecola, C. (2012). Peroxiredoxin sets the brain on fire after stroke. Nat. Med. 18, 858–859. doi: 10.1038/nm.2797
Gu, X., Zhang, Y., Li, D., Cai, H., Cai, L., and Xu, Q. (2020). N6-methyladenosine demethylase FTO promotes M1 and M2 macrophage activation. Cell. Signal. 69:109553. doi: 10.1016/j.cellsig.2020.109553
Guo, M., Yan, R., Ji, Q., Yao, H., Sun, M., Duan, L., et al. (2020). IFN regulatory Factor-1 induced macrophage pyroptosis by modulating m6A modification of circ_0029589 in patients with acute coronary syndrome. Int. Immunopharmacol. 86:106800. doi: 10.1016/j.intimp.2020.106800
Guo, M. L., Periyasamy, P., Liao, K., Kook, Y. H., Niu, F., Callen, S. E., et al. (2016). Cocaine-mediated downregulation of microglial miR-124 expression involves promoter DNA methylation. Epigenetics 11, 819–830. doi: 10.1080/15592294.2016.1232233
Han, M., Liu, Z., Xu, Y., Liu, X., Wang, D., Li, F., et al. (2020). Abnormality of m6A mRNA methylation is involved in Alzheimer’s disease. Front. Neurosci. 14:98. doi: 10.3389/fnins.2020.00098
Han, X., Wang, M., Zhao, Y. L., Yang, Y., and Yang, Y. G. (2021). RNA methylations in human cancers. Semin. Cancer Biol. 75, 97–115. doi: 10.1016/j.semcancer.2020.11.007
He, L., Li, H., Wu, A., Peng, Y., Shu, G., and Yin, G. (2019). Functions of N6-methyladenosine and its role in cancer. Mol. Cancer 18:176. doi: 10.1186/s12943-019-1109-1109
Hickman, S., Izzy, S., Sen, P., Morsett, L., and El Khoury, J. (2018). Microglia in neurodegeneration. Nat. Neurosci. 21, 1359–1369. doi: 10.1038/s41593-018-0242-x
Hu, F., Zhu, D., Pei, W., Lee, I., Zhang, X., Pan, L., et al. (2019). Rhein inhibits ATP-triggered inflammatory responses in rheumatoid rat fibroblast-like synoviocytes. Int. Immunopharmacol. 75:105780. doi: 10.1016/j.intimp.2019.105780
Hu, X., Li, P., Guo, Y., Wang, H., Leak, R. K., Chen, S., et al. (2012). Microglia/macrophage polarization dynamics reveal novel mechanism of injury expansion after focal cerebral ischemia. Stroke 43, 3063–3070. doi: 10.1161/STROKEAHA.112.659656
Hu, X., Peng, W. X., Zhou, H., Jiang, J., Zhou, X., Huang, D., et al. (2020). IGF2BP2 regulates DANCR by serving as an N6-methyladenosine reader. Cell Death. Differ. 27, 1782–1794. doi: 10.1038/s41418-019-0461-z
Huang, H., Weng, H., Sun, W., Qin, X., Shi, H., Wu, H., et al. (2018). Recognition of RNA N(6)-methyladenosine by IGF2BP proteins enhances mRNA stability and translation. Nat. Cell Biol. 20, 285–295. doi: 10.1038/s41556-018-0045-z
Huangfu, N., Zheng, W., Xu, Z., Wang, S., Wang, Y., Cheng, J., et al. (2020). RBM4 regulates M1 macrophages polarization through targeting STAT1-mediated glycolysis. Int. Immunopharmacol. 83:106432. doi: 10.1016/j.intimp.2020.106432
Jayaraj, R. L., Azimullah, S., Beiram, R., Jalal, F. Y., and Rosenberg, G. A. (2019). Neuroinflammation: friend and foe for ischemic stroke. J. Neuroinflamm. 16:142. doi: 10.1186/s12974-019-1516-1512
Jiang, X., Liu, B., Nie, Z., Duan, L., Xiong, Q., Jin, Z., et al. (2021). The role of m6A modification in the biological functions and diseases. Signal Transduct. Target Ther. 6:74. doi: 10.1038/s41392-020-00450-x
Jurcau, A., and Simion, A. (2021). Neuroinflammation in cerebral ischemia and Ischemia/Reperfusion injuries: from pathophysiology to therapeutic strategies. Int. J. Mol. Sci. 23:14. doi: 10.3390/ijms23010014
Kasowitz, S. D., Ma, J., Anderson, S. J., Leu, N. A., Xu, Y., Gregory, B. D., et al. (2018). Nuclear m6A reader YTHDC1 regulates alternative polyadenylation and splicing during mouse oocyte development. PLoS Genet 14:e1007412. doi: 10.1371/journal.pgen.1007412
Khoshnam, S. E., Winlow, W., Farzaneh, M., Farbood, Y., and Moghaddam, H. F. (2017). Pathogenic mechanisms following ischemic stroke. Neurol. Sci. 38, 1167–1186. doi: 10.1007/s10072-017-2938-2931
Lambertsen, K. L., Finsen, B., and Clausen, B. H. (2019). Post-stroke inflammation-target or tool for therapy? Acta Neuropathol. 137, 693–714. doi: 10.1007/s00401-018-1930-z
Lee, Y., Choe, J., Park, O. H., and Kim, Y. K. (2020). Molecular mechanisms driving mRNA degradation by m(6)A modification. Trends Genet. 36, 177–188. doi: 10.1016/j.tig.2019.12.007
Leng, F., and Edison, P. (2021). Neuroinflammation and microglial activation in Alzheimer disease: where do we go from here? Nat. Rev. Neurol. 17, 157–172. doi: 10.1038/s41582-020-00435-y
Li, Q., and Barres, B. A. (2018). Microglia and macrophages in brain homeostasis and disease. Nat. Rev. Immunol. 18, 225–242. doi: 10.1038/nri.2017.125
Li, Q., Wen, S., Ye, W., Zhao, S., and Liu, X. (2021). The potential roles of m6A modification in regulating the inflammatory response in microglia. J. Neuroinflamm. 18:149. doi: 10.1186/s12974-021-02205-z
Li, T., Luo, Y., Zhang, P., Guo, S., Sun, H., Yan, D., et al. (2020). LncRNA MEG3 regulates microglial polarization through KLF4 to affect cerebral ischemia-reperfusion injury. J. Appl. Physiol. 129, 1460–1467. doi: 10.1152/japplphysiol.00433.2020
Liu, L. Q., Liu, X. R., Zhao, J. Y., Yan, F., Wang, R. L., Wen, S. H., et al. (2018). Brain-selective mild hypothermia promotes long-term white matter integrity after ischemic stroke in mice. CNS Neurosci. Ther. 24, 1275–1285. doi: 10.1111/cns.13061
Liu, T., Wei, Q., Jin, J., Luo, Q., Liu, Y., Yang, Y., et al. (2020). The m6A reader YTHDF1 promotes ovarian cancer progression via augmenting EIF3C translation. Nucleic Acids Res. 48, 3816–3831. doi: 10.1093/nar/gkaa048
Liu, Y., Liu, Z., Tang, H., Shen, Y., Gong, Z., Xie, N., et al. (2019a). The N(6)-methyladenosine (m(6)A)-forming enzyme METTL3 facilitates M1 macrophage polarization through the methylation of STAT1 mRNA. Am. J. Physiol. Cell Physiol. 317, C762–C775. doi: 10.1152/ajpcell.00212.2019
Liu, Z. J., Ran, Y. Y., Qie, S. Y., Gong, W. J., Gao, F. H., Ding, Z. T., et al. (2019b). Melatonin protects against ischemic stroke by modulating microglia/macrophage polarization toward anti-inflammatory phenotype through STAT3 pathway. CNS Neurosci. Therapeut. 25, 1353–1362. doi: 10.1111/cns.13261
Liu, Z., Ran, Y., Huang, S., Wen, S., Zhang, W., Liu, X., et al. (2017). Curcumin protects against ischemic stroke by titrating microglia/macrophage polarization. Front. Aging Neurosci. 9:233. doi: 10.3389/fnagi.2017.00233
Lo, E. H., Dalkara, T., and Moskowitz, M. A. (2003). Mechanisms, challenges and opportunities in stroke. Nat. Rev. Neurosci. 4, 399–415. doi: 10.1038/nrn1106
Lu, N., Li, X., Yu, J., Li, Y., Wang, C., Zhang, L., et al. (2018). Curcumin attenuates lipopolysaccharide-induced hepatic lipid metabolism disorder by modification of m(6) A RNA methylation in piglets. Lipids 53, 53–63. doi: 10.1002/lipd.12023
Lyu, J., Xie, D., Bhatia, T. N., Leak, R. K., Hu, X., and Jiang, X. (2021). Microglial/Macrophage polarization and function in brain injury and repair after stroke. CNS Neurosci. Ther. 27, 515–527. doi: 10.1111/cns.13620
Ma, C., Liao, S., and Zhu, Z. (2019). Crystal structure of human YTHDC2 YTH domain. Biochem. Biophys. Res. Commun. 518, 678–684. doi: 10.1016/j.bbrc.2019.08.107
Mapperley, C., van de Lagemaat, L. N., Lawson, H., Tavosanis, A., Paris, J., Campos, J., et al. (2021). The mRNA m6A reader YTHDF2 suppresses proinflammatory pathways and sustains hematopoietic stem cell function. J. Exp. Med. 218:e20200929. doi: 10.1084/jem.20200829
Martinez-Contreras, R., Cloutier, P., Shkreta, L., Fisette, J. F., Revil, T., and Chabot, B. (2007). hnRNP proteins and splicing control. Adv. Exp. Med. Biol. 623, 123–147. doi: 10.1007/978-0-387-77374-2_8
Mathoux, J., Henshall, D. C., and Brennan, G. P. (2021). Regulatory mechanisms of the RNA modification m(6)A and significance in brain function in health and disease. Front. Cell Neurosci. 15:671932. doi: 10.3389/fncel.2021.671932
Meyer, K. D., and Jaffrey, S. R. (2017). Rethinking m(6)A readers. writers, and erasers. Annu. Rev. Cell Dev. Biol. 33, 319–342. doi: 10.1146/annurev-cellbio-100616-160758
Muller, S., Glass, M., Singh, A. K., Haase, J., Bley, N., Fuchs, T., et al. (2019). IGF2BP1 promotes SRF-dependent transcription in cancer in a m6A- and miRNA-dependent manner. Nucleic Acids Res. 47, 375–390. doi: 10.1093/nar/gky1012
Ontiveros, R. J., Shen, H., Stoute, J., Yanas, A., Cui, Y., Zhang, Y., et al. (2020). Coordination of mRNA and tRNA methylations by TRMT10A. Proc. Natl. Acad. Sci. U S A. 117, 7782–7791. doi: 10.1073/pnas.1913448117
Petrovic-Djergovic, D., Goonewardena, S. N., and Pinsky, D. J. (2016). Inflammatory disequilibrium in stroke. Circ. Res. 119, 142–158. doi: 10.1161/CIRCRESAHA.116.308022
Prinz, M., Masuda, T., Wheeler, M. A., and Quintana, F. J. (2021). Microglia and central nervous system-associated macrophages-from origin to disease modulation. Annu. Rev. Immunol. 39, 251–277. doi: 10.1146/annurev-immunol-093019-110159
Qin, C., Zhou, L. Q., Ma, X. T., Hu, Z. W., Yang, S., Chen, M., et al. (2019). Dual functions of microglia in ischemic stroke. Neurosci. Bull. 35, 921–933. doi: 10.1007/s12264-019-00388-383
Qin, L., Min, S., Shu, L., Pan, H., Zhong, J., Guo, J., et al. (2020). Genetic analysis of N6-methyladenosine modification genes in Parkinson’s disease. Neurobiol. Aging 93, 143.e9–143.e13. doi: 10.1016/j.neurobiolaging.2020.03.018
Qiu, M., Xu, E., and Zhan, L. (2021). Epigenetic regulations of microglia/macrophage polarization in ischemic stroke. Front. Mol. Neurosci. 14:697416. doi: 10.3389/fnmol.2021.697416
Qiu, X., He, H., Huang, Y., Wang, J., and Xiao, Y. (2020). Genome-wide identification of m(6)A-associated single-nucleotide polymorphisms in Parkinson’s disease. Neurosci. Lett. 737:135315. doi: 10.1016/j.neulet.2020.135315
Ran, Y., Su, W., Gao, F., Ding, Z., Yang, S., Ye, L., et al. (2021). Curcumin ameliorates white matter injury after ischemic stroke by inhibiting microglia/macrophage pyroptosis through NF-κB suppression and NLRP3 inflammasome inhibition. Oxid. Med. Cell. Long. 2021, 1–25. doi: 10.1155/2021/1552127
Rasheed, M., Liang, J., Wang, C., Deng, Y., and Chen, Z. (2021). Epigenetic regulation of neuroinflammation in Parkinson’s disease. Int. J. Mol. Sci. 22:4956. doi: 10.3390/ijms22094956
Reitz, C., Tosto, G., Mayeux, R., and Luchsinger, J. A., NIA-Load/NCRAD Family Study Group, and Alzheimer’s Disease Neuroimaging Initiative. (2012). Genetic variants in the Fat and Obesity Associated (FTO) gene and risk of Alzheimer’s disease. PLoS One 7:e50354. doi: 10.1371/journal.pone.0050354
Rigillo, G., Vilella, A., Benatti, C., Schaeffer, L., Brunello, N., Blom, J. M. C., et al. (2018). LPS-induced histone H3 phospho(Ser10)-acetylation(Lys14) regulates neuronal and microglial neuroinflammatory response. Brain Behav. Immun. 74, 277–290. doi: 10.1016/j.bbi.2018.09.019
Robinson, N., Ganesan, R., Hegedus, C., Kovacs, K., Kufer, T. A., and Virag, L. (2019). Programmed necrotic cell death of macrophages: focus on pyroptosis, necroptosis, and parthanatos. Redox. Biol. 26:101239. doi: 10.1016/j.redox.2019.101239
Saha, S., Buttari, B., Profumo, E., Tucci, P., and Saso, L. (2021). A perspective on Nrf2 signaling pathway for neuroinflammation: a potential therapeutic target in Alzheimer’s and Parkinson’s diseases. Front. Cell Neurosci. 15:787258. doi: 10.3389/fncel.2021.787258
Selberg, S., Yu, L. Y., Bondarenko, O., Kankuri, E., Seli, N., Kovaleva, V., et al. (2021). Small-Molecule inhibitors of the RNA M6A demethylases FTO potently support the survival of dopamine neurons. Int. J. Mol. Sci. 22:4537. doi: 10.3390/ijms22094537
Shafik, A. M., Zhang, F., Guo, Z., Dai, Q., Pajdzik, K., Li, Y., et al. (2021). N6-methyladenosine dynamics in neurodevelopment and aging, and its potential role in Alzheimer’s disease. Genome Biol. 22:17. doi: 10.1186/s13059-020-02249-z
Shi, K., Tian, D.-C., Li, Z.-G., Ducruet, A. F., Lawton, M. T., and Shi, F.-D. (2019). Global brain inflammation in stroke. Lancet Neurol. 18, 1058–1066. doi: 10.1016/s1474-4422(19)30078-x
Shi, R., Ying, S., Li, Y., Zhu, L., Wang, X., and Jin, H. (2021). Linking the YTH domain to cancer: the importance of YTH family proteins in epigenetics. Cell Death Dis. 12:346. doi: 10.1038/s41419-021-03625-3628
Si, W., Li, Y., Ye, S., Li, Z., Liu, Y., Kuang, W., et al. (2020). Methyltransferase 3 mediated miRNA m6A methylation promotes stress granule formation in the early stage of acute ischemic stroke. Front. Mol. Neurosci. 13:103. doi: 10.3389/fnmol.2020.00103
Singh, V., Roth, S., Veltkamp, R., and Liesz, A. (2016). HMGB1 as a key mediator of immune mechanisms in ischemic stroke. Antioxid. Redox. Signal. 24, 635–651. doi: 10.1089/ars.2015.6397
Skaper, S. D., Facci, L., Zusso, M., and Giusti, P. (2018). An inflammation-centric view of neurological disease: beyond the neuron. Front. Cell Neurosci. 12:72. doi: 10.3389/fncel.2018.00072
Smith, J. A., Das, A., Ray, S. K., and Banik, N. L. (2012). Role of pro-inflammatory cytokines released from microglia in neurodegenerative diseases. Brain Res. Bull. 87, 10–20. doi: 10.1016/j.brainresbull.2011.10.004
Szeplaki, G., Szegedi, R., Hirschberg, K., Gombos, T., Varga, L., Karadi, I., et al. (2009). Strong complement activation after acute ischemic stroke is associated with unfavorable outcomes. Atherosclerosis 204, 315–320. doi: 10.1016/j.atherosclerosis.2008.07.044
Tang, Y., and Le, W. (2016). Differential roles of M1 and M2 microglia in neurodegenerative diseases. Mol. Neurobiol. 53, 1181–1194. doi: 10.1007/s12035-014-9070-9075
van Tran, N., Ernst, F. G. M., Hawley, B. R., Zorbas, C., Ulryck, N., Hackert, P., et al. (2019). The human 18S rRNA m6A methyltransferase METTL5 is stabilized by TRMT112. Nucleic Acids Res. 47, 7719–7733. doi: 10.1093/nar/gkz619
Wang, J., Ishfaq, M., Xu, L., Xia, C., Chen, C., and Li, J. (2019a). METTL3/m(6)A/miRNA-873-5p attenuated oxidative stress and apoptosis in colistin-induced kidney injury by modulating Keap1/Nrf2 pathway. Front. Pharmacol. 10:517. doi: 10.3389/fphar.2019.00517
Wang, J., Yan, S., Lu, H., Wang, S., and Xu, D. (2019b). METTL3 attenuates LPS-Induced inflammatory response in macrophages via NF-kappaB signaling pathway. Mediators Inflamm. 2019:3120391. doi: 10.1155/2019/3120391
Wang, J. Y., and Lu, A. Q. (2021). The biological function of m6A reader YTHDF2 and its role in human disease. Cancer Cell Int. 21:109. doi: 10.1186/s12935-021-01807-1800
Wang, L. J., Xue, Y., Li, H., Huo, R., Yan, Z., Wang, J., et al. (2020a). Wilms’ tumour 1-associating protein inhibits endothelial cell angiogenesis by m6A-dependent epigenetic silencing of desmoplakin in brain arteriovenous malformation. J. Cell Mol. Med. 24, 4981–4991. doi: 10.1111/jcmm.15101
Wang, T., Kong, S., Tao, M., and Ju, S. (2020b). The potential role of RNA N6-methyladenosine in Cancer progression. Mol. Cancer 19:88. doi: 10.1186/s12943-020-01204-1207
Wang, X., Ji, Y., Feng, P., Liu, R., Li, G., Zheng, J., et al. (2021). The m6A reader IGF2BP2 regulates macrophage phenotypic activation and inflammatory diseases by stabilizing TSC1 and PPARgamma. Adv. Sci. 8:2100209. doi: 10.1002/advs.202100209
Wang, X., Zhao, B. S., Roundtree, I. A., Lu, Z., Han, D., Ma, H., et al. (2015). N(6)-methyladenosine modulates messenger RNA translation efficiency. Cell 161, 1388–1399. doi: 10.1016/j.cell.2015.05.014
Wei, J., Liu, F., Lu, Z., Fei, Q., Ai, Y., He, P. C., et al. (2018). Differential m(6)A, m(6)Am, and m(1)A demethylation mediated by FTO in the Cell nucleus and cytoplasm. Mol. Cell. 71, 973–985.e5. doi: 10.1016/j.molcel.2018.08.011
Wen, L., Sun, W., Xia, D., Wang, Y., Li, J., and Yang, S. (2022). The m6A methyltransferase METTL3 promotes LPS-induced microglia inflammation through TRAF6/NF-kappaB pathway. Neuroreport 33, 243–251. doi: 10.1097/WNR.0000000000001550
Wen, S., Wei, Y., Zen, C., Xiong, W., Niu, Y., and Zhao, Y. (2020). Long non-coding RNA NEAT1 promotes bone metastasis of prostate cancer through N6-methyladenosine. Mol. Cancer 19:171. doi: 10.1186/s12943-020-01293-1294
Xu, K., Mo, Y., Li, D., Yu, Q., Wang, L., Lin, F., et al. (2020a). N(6)-methyladenosine demethylases Alkbh5/Fto regulate cerebral ischemia-reperfusion injury. Ther. Adv. Chronic. Dis. 11:2040622320916024. doi: 10.1177/2040622320916024
Xu, S., Tang, L., Dai, G., Luo, C., and Liu, Z. (2020b). Expression of m6A regulators correlated with immune microenvironment predicts therapeutic efficacy and prognosis in gliomas. Front. Cell Dev. Biol. 8:594112. doi: 10.3389/fcell.2020.594112
Yang, Z., Wang, T., Wu, D., Min, Z., Tan, J., and Yu, B. (2020). RNA N6-methyladenosine reader IGF2BP3 regulates cell cycle and angiogenesis in colon cancer. J. Exp. Clin. Cancer Res. 39:203. doi: 10.1186/s13046-020-01714-1718
Yi, D., Wang, Q., Zhao, Y., Song, Y., You, H., Wang, J., et al. (2021). Alteration of N (6) -Methyladenosine mRNA methylation in a rat model of cerebral ischemia-reperfusion injury. Front. Neurosci. 15:605654. doi: 10.3389/fnins.2021.605654
Yoon, K. J., Ringeling, F. R., Vissers, C., Jacob, F., Pokrass, M., Jimenez-Cyrus, D., et al. (2017). Temporal control of mammalian cortical neurogenesis by m(6)A methylation. Cell 171, 877–889.e17. doi: 10.1016/j.cell.2017.09.003
Yu, F., Huang, T., Ran, Y., Li, D., Ye, L., Tian, G., et al. (2021). New insights into the roles of microglial regulation in brain plasticity-dependent stroke recovery. Front. Cell. Neurosci. 15:727899. doi: 10.3389/fncel.2021.727899
Yu, R., Li, Q., Feng, Z., Cai, L., and Xu, Q. (2019). m6A reader YTHDF2 regulates LPS-Induced inflammatory response. Int. J. Mol. Sci. 20:1323. doi: 10.3390/ijms20061323
Zaccara, S., Ries, R. J., and Jaffrey, S. R. (2019). Reading, writing and erasing mRNA methylation. Nat. Rev. Mol. Cell Biol. 20, 608–624. doi: 10.1038/s41580-019-0168-165
Zhang, H., Shi, X., Huang, T., Zhao, X., Chen, W., Gu, N., et al. (2020a). Dynamic landscape and evolution of m6A methylation in human. Nucleic Acids Res. 48, 6251–6264. doi: 10.1093/nar/gkaa347
Zhang, Y., Geng, X., Li, Q., Xu, J., Tan, Y., Xiao, M., et al. (2020b). m6A modification in RNA: biogenesis, functions and roles in gliomas. J. Exp. Clin. Cancer Res. 39:192. doi: 10.1186/s13046-020-01706-1708
Zhang, Z., Wang, Q., Zhao, X., Shao, L., Liu, G., Zheng, X., et al. (2020c). YTHDC1 mitigates ischemic stroke by promoting Akt phosphorylation through destabilizing PTEN mRNA. Cell Death Dis. 11:977. doi: 10.1038/s41419-020-03186-3182
Zhang, Q. S., Heng, Y., Yuan, Y. H., and Chen, N. H. (2017). Pathological alpha-synuclein exacerbates the progression of Parkinson’s disease through microglial activation. Toxicol. Lett. 265, 30–37. doi: 10.1016/j.toxlet.2016.11.002
Zhang, S. (2018). Mechanism of N(6)-methyladenosine modification and its emerging role in cancer. Pharmacol. Ther. 189, 173–183. doi: 10.1016/j.pharmthera.2018.04.011
Zhang, S., Wang, X. J., Tian, L. P., Pan, J., Lu, G. Q., Zhang, Y. J., et al. (2011). CD200-CD200R dysfunction exacerbates microglial activation and dopaminergic neurodegeneration in a rat model of Parkinson’s disease. J. Neuroinflamm. 8:154. doi: 10.1186/1742-2094-8-154
Zhao, S. C., Ma, L. S., Chu, Z. H., Xu, H., Wu, W. Q., and Liu, F. (2017). Regulation of microglial activation in stroke. Acta Pharmacol. Sin. 38, 445–458. doi: 10.1038/aps.2016.162
Zhao, Y., Shi, Y., Shen, H., and Xie, W. (2020). m(6)A-binding proteins: the emerging crucial performers in epigenetics. J. Hematol. Oncol. 13:35. doi: 10.1186/s13045-020-00872-8
Zheng, L., Tang, X., Lu, M., Sun, S., Xie, S., Cai, J., et al. (2020). microRNA-421-3p prevents inflammatory response in cerebral ischemia/reperfusion injury through targeting m6A Reader YTHDF1 to inhibit p65 mRNA translation. Int. Immunopharmacol. 88:106937. doi: 10.1016/j.intimp.2020.106937
Zheng, Y., Nie, P., Peng, D., He, Z., Liu, M., Xie, Y., et al. (2018). m6AVar: a database of functional variants involved in m6A modification. Nucleic Acids Res. 46, D139–D145. doi: 10.1093/nar/gkx895
Zhou, X., and Spittau, B. (2018). Lipopolysaccharide-Induced microglia activation promotes the survival of midbrain dopaminergic neurons in vitro. Neurotox. Res. 33, 856–867. doi: 10.1007/s12640-017-9842-6
Keywords: RNA methylation, ischemic stroke, microglia, neuroinflammation, polarization
Citation: Zhang F, Ran Y, Tahir M, Li Z, Wang J and Chen X (2022) Regulation of N6-methyladenosine (m6A) RNA methylation in microglia-mediated inflammation and ischemic stroke. Front. Cell. Neurosci. 16:955222. doi: 10.3389/fncel.2022.955222
Received: 28 May 2022; Accepted: 12 July 2022;
Published: 04 August 2022.
Edited by:
Guodong Cao, University of Pittsburgh, United StatesReviewed by:
Xiaoxing Xiong, Renmin Hospital of Wuhan University, ChinaYuan Wang, Xuanwu Hospital, Capital Medical University, China
Zhongfang Weng, University of Pittsburgh, United States
Copyright © 2022 Zhang, Ran, Tahir, Li, Wang and Chen. This is an open-access article distributed under the terms of the Creative Commons Attribution License (CC BY). The use, distribution or reproduction in other forums is permitted, provided the original author(s) and the copyright owner(s) are credited and that the original publication in this journal is cited, in accordance with accepted academic practice. No use, distribution or reproduction is permitted which does not comply with these terms.
*Correspondence: Xuechai Chen, Y2hlbnh1ZWNoYWlAYmp1dC5lZHUuY24=
†These authors have contributed equally to this work