- 1Programa de Pós-Graduação em Ciências Biológicas: Bioquímica, Instituto de Ciências Básicas da Saúde, Universidade Federal do Rio Grande do Sul, Porto Alegre, RS, Brazil
- 2Programa de Pós-Graduação em Neurociências, Instituto de Ciências Básicas da Saúde, Universidade Federal do Rio Grande do Sul, Porto Alegre, RS, Brazil
We are living in a terrifying pandemic caused by Sars-CoV-2, in which patients with diabetes mellitus have, from the beginning, been identified as having a high risk of hospitalization and mortality. This viral disease is not limited to the respiratory system, but also affects, among other organs, the central nervous system. Furthermore, we already know that individuals with diabetes mellitus exhibit signs of astrocyte dysfunction and are more likely to develop cognitive deficits and even dementia. It is now being realized that COVID-19 incurs long-term effects and that those infected can develop several neurological and psychiatric manifestations. As this virus seriously compromises cell metabolism by triggering several mechanisms leading to the unfolded protein response (UPR), which involves endoplasmic reticulum Ca2+ depletion, we review here the basis involved in this response that are intimately associated with the development of neurodegenerative diseases. The discussion aims to highlight two aspects—the role of calcium-binding proteins and the role of astrocytes, glial cells that integrate energy metabolism with neurotransmission and with neuroinflammation. Among the proteins discussed are calpain, calcineurin, and sorcin. These proteins are emphasized as markers of the UPR and are potential therapeutic targets. Finally, we discuss the role of drugs widely prescribed to patients with diabetes mellitus, such as statins, metformin, and calcium channel blockers. The review assesses potential neuroprotection mechanisms, focusing on the UPR and the restoration of reticular Ca2+ homeostasis, based on both clinical and experimental data.
Introduction
We are still experiencing a terrible pandemic caused by severe acute respiratory syndrome coronavirus 2 (Sars-CoV-2), despite our collective attempt and abilities to deal with the situation. We quickly understood the virus’ mechanism of transmission, identified and learned how to mitigate the most severe cases of the coronavirus disease 2019 (COVID-19), and prepared vaccines in record time. Unfortunately, ideological resistance, fueled by ignorance and the pursuit of profits, has undermined and continues to undermine this collective effort. Furthermore, clinical and epidemiological data show that COVID-19 has long-term effects that lead to post-COVID-19 neuropsychiatric disorders, including neurodegenerative diseases such as Alzheimer’s disease (AD; Heneka et al., 2020; Robinson-Agramonte et al., 2021; Toniolo et al., 2021).
In this review, we aim to discuss the neurochemical bases of these disorders, focusing on the role of Ca2+-binding proteins (CBP) associated with endoplasmic reticulum (ER) stress, particularly in astrocytes, which can result in an event known as an unfolded protein response (UPR). We address the steps and markers of this event. Then conclude by discussing drugs with potential neuroprotective actions against COVID-19, addressing two questions. First, we know that patients with diabetes mellitus are at increased risk of hospitalization and mortality for COVID-19 (Zhou et al., 2021). Could drugs already used in this disease, such as metformin, statins, and calcium channel blockers (CCB), provide some neuroprotection against COVID-19? Secondly, could these drugs affect the UPR and consequently contribute to reducing post-COVID-19 neurodegenerative diseases and/or cognitive impairment? These issues and questions will be discussed briefly in this commentary/review in an attempt to emphasize the role of reticular Ca2+ and CBP in brain activity and to broaden our understanding of the impact of COVID-19 on the development of neurodegenerative diseases.
Astrocytes Are Integrative Players in Neuroinflammation
Cognitive decline features among the numerous and worrisome neurological and psychiatric manifestations associated with COVID-19. This manifestation results from many injurious factors, including direct viral aggression and systemic changes in the disease, such as coagulopathy, hypoxia, and cytokine storm, which all affect brain tissue, in addition to prolonged sedation in cases that require intubation and mechanical ventilation (Miners et al., 2020). However, to understand brain inflammation in COVID-19, whether by direct infection or due to ischemia or hypercytokinemia, we need to address the central regulatory role of astrocytes in the immune response (see Murta et al., 2020; Tremblay et al., 2020 for a review).
Astrocytes and microglia are the major cells that mount the inflammatory response in the central nervous system (CNS). However, unlike microglia, astrocytes are not immune cells, but when they receive molecular signs of injury they develop a complex machinery that makes them essential regulators of the adaptive and innate immune response (see Colombo and Farina, 2016; Sofroniew, 2020 for a review). Due to their heterogeneity and widespread location in the brain tissue, astrocytes facilitate communication with other cells as they coat the cerebral vascular endothelium, actively envelop the synapses (functioning as the third synaptic element), intimately contact the microglia and form a glial syncytial network through gap junctions, thereby functioning as integrating elements in the CNS. All this communication involves intense Ca2+ signaling from the ER (Verkhratsky and Parpura, 2014; Lia et al., 2021). The close functional relationship between neurons and astrocytes has supported the idea that astrocytic dysfunction accompanies or even precedes neuronal and cognitive damage in diseases such as diabetes mellitus and Alzheimer’s disease (González-Reyes et al., 2016).
Astrocytes respond to inflammatory cytokines, and produce cytokines in response to them and/or via the direct activation of receptors for damage-associated molecular patterns and pathogen-associated molecular patterns. In fact, they actively change under injury. For example, soluble tumor necrosis factor (sTNFα; molecular weight of 17 kDa) is recognized by receptor TNFR1 in astrocytes, which change and then start to express TNFR2, a typical receptor (but not exclusive to) on immune cells, which can recognize mTNFα (membrane-bound protein of 26 kDa) on visiting and neighboring cells (Fischer et al., 2014). These changes involve several transcription factors, such as nuclear factor kappa B (NF-kB), signal transducer and activator of transcription 3 (STAT3), and nuclear factor of activated T cells (NFAT), which in turn depend on Ca2+-mediated orchestration (Colombo and Farina, 2016; Schultz et al., 2021).
Significant infection and viral replication were observed in astrocytes of human brain cell cultures exposed to Sars-CoV-2, but minimal infection in other cell types was seen (Andrews et al., 2021). These data reinforce the astroglial commitment observed in brain postmortem studies of COVID-19 patients, where macrophage and T cell infiltration are observed, as well as vascular damage with fibrinogen leakage and extensive astrogliosis (Matschke et al., 2020). In fact, in postmortem studies, Sars-CoV-2 nucleocapsid was found in cortical astrocytes, as well as in neurons, microglia, and oligodendrocytes (Cama et al., 2021). Another very important aspect of COVID-19 is the greater vulnerability of elderly patients; possibly the aging of astrocytes makes these individuals more susceptible to Sars-CoV-2-induced dysfunction (Tremblay et al., 2020).
The plasma membrane enzyme angiotensin converting enzyme 2 (ACE-2), is involved in the anchoring and entry of Sars-CoV-2 in several cell types; however, in mature astrocytes, a role for this enzyme is debatable (Andrews et al., 2021). Nevertheless, the intracellular process of Sars-CoV-2 recognition apparently involves the activation of NOD-, LRR- and pyrin domain-containing protein 3 (NLRP3), a member of the pattern recognition receptors family (Heneka et al., 2020). NLRP3 has been associated with the development of degenerative diseases, such as diabetes mellitus (Volpe et al., 2016), and more recently associated with neurodegenerative diseases, possibly mediated by the UPR (discussed below; Guan and Han, 2020). This activation of NLRP3 by Sars-CoV-2 may support post-COVID-19 neurodegenerative diseases (Heneka et al., 2020).
In addition to the possibility of direct neuroinfection, the endothelial Sars-CoV-2-induced damage affects neural cells and can be triggered and/or aggravated by the ischemic inflammatory insult of COVID-19, which, in severe cases, progresses with atypical coagulation and hypoxia (Sashindranath and Nandurkar, 2021). In addition, hypercytokinemia (cytokine storm) in more severe cases of the disease can, through a mechanism involving astrocytes, mediate neurological damage, including cognitive deficit (Alnefeesi et al., 2021).
UPR Steps and Markers
Currently, the understanding of neurodegenerative diseases involves changes associated with UPR, which result from ER stress and are often triggered by an imbalance in intracellular Ca2+ (van Vliet and Agostinis, 2016). We will review the UPR, emphasizing the main steps, CBP as markers of this event, and, finally, we will discuss a potential role for neuroviruses, in particular Sars-CoV-2, in the UPR in order to justify the observed increased risk of neurodegenerative diseases.
UPR steps
The ER occupies a large structural and functional dimension in cell life. It possesses 60% of the membranous structure (in contrast to the plasma membrane, which has 5%) and is the major organelle responsible for the regulation of intracellular Ca2+ (Alberts et al., 2008). The ER is responsible for much of the synthesis, maturation, and folding of cellular proteins, including the transmembrane protein machinery involved in cellular secretion, emphasizing its importance for intercellular communication and the progression of diseases. Many physiological and pathological changes affect ER activity, such as ER-Ca2+ depletion, elevated protein synthesis and trafficking, glucose deprivation, inflammatory stimuli, hypoxia, and ROS, leading to ER stress (Bravo et al., 2013). Although the mechanisms are not all known, the changes mentioned are detected by ER stress sensors. There are three well-characterized transmembrane sensors; inositol requiring enzyme 1 (IRE1), protein kinase R-like ER kinase (PERK) and activating transcription factor 6 (ATF6). An additional sensor, functionally equivalent to ATF6, is found specifically in astrocytes, the old astrocyte specifically induced substance (OASIS); see Sims et al. (2022) for a review of these sensors in astrocytes. All these sensors, when activated, result in the restoration of reticular protein homeostasis (named proteostasis), i.e., they (a) reduce mRNA expression (e.g., via IRE1 activation) and protein synthesis (e.g., via PERK activation), contributing to lower protein traffic in the ER; (b) increase the synthesis of chaperones and other specific proteins that contribute to restoring the reticular function; and (c) increase the expression of proteins involved in the degradation of unfolded reticular proteins (via ATF6 activation), called ER-associated degradation (ERAD; Bahar et al., 2016; Martin-Jiménez et al., 2017).
These sensor proteins are inactive, due to contact with Ca2+-binding chaperone proteins. Calreticulin (or glucose-regulated protein 94—GRP94) and immunoglobulin heavy chain-binding protein (BiP or GRP78) are the most abundant Ca2+-binding chaperones in the ER (Bravo et al., 2013). When these proteins move to fold the misfolded proteins in an attempt to restore the proteostasis compromised by ER stress, they activate the sensors. Therefore, the displacement of these chaperones (which are CBP) signals the ER stress and stimulates their further expression as a result of the activation of sensor proteins, thereby acting as primary markers of ER stress (see Figure 1).
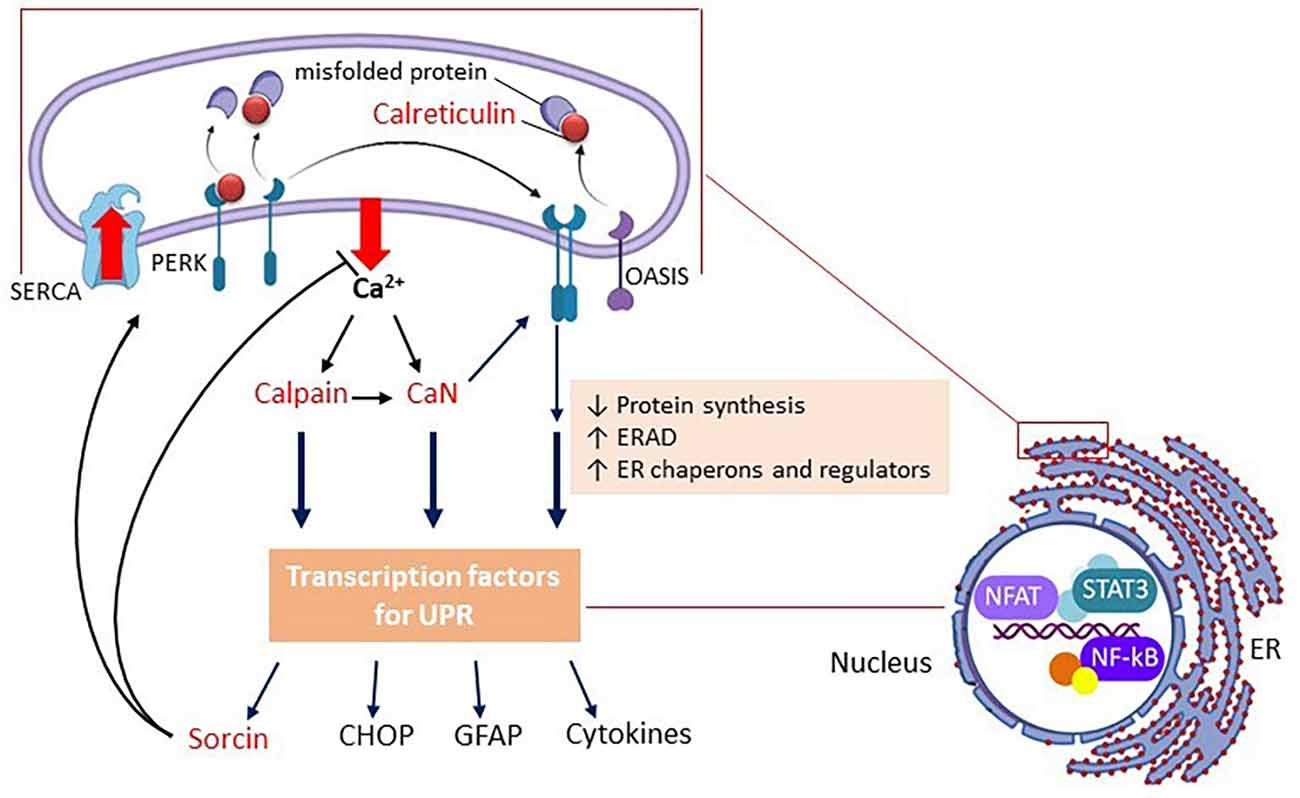
Figure 1. Schematic representation of the UPR in astrocytes. Only PERK and OASIS are represented as ER stress sensor proteins. Upon Ca2+ efflux, the chaperone CBPs (e.g., calreticulin) move from the sensor proteins (in the membrane) to the unfolded proteins (in the lumen), promoting the oligomerization of the sensor proteins, and triggering the UPR. In parallel, but not independently, the outgoing Ca2+ activates calpain and calcineurin (CaN). CBPs are all represented in red. Note that CaN (independent of enzyme activity) positively modulates PERK. Gene expression in the UPR, in general, is reduced, but some modulating proteins are upregulated, such as sorcin, CHOP, and GFAP, in addition to pro-inflammatory cytokines. Sorcin plays a role at the beginning of the UPR (negatively modulating Ca2+ efflux and CHOP expression). CHOP will play an important role in cell death if the triggering factor(s) of the UPR persist. It is important to mention that calpain and CaN modulate ER Ca2+-channels and SERCA, but in this scheme, only sorcin, which also plays this role, is highlighted. The image on the right emphasizes the structural relationship and bidirectional communication between the ER and the nucleus, where the main transcription factors of the astroglial inflammatory response (NF-kB, NFAT, and STAT) are highlighted. Abbreviations: CaN, calcineurin; CBP, calcium-binding protein; CHOP, CCAAT-enhancer-binding protein homologous protein; ERAD, ER-associated degradation; GFAP, glial fibrillary acidic protein; NF-kB, nuclear factor kappa B; NFAT, nuclear factor of activated T-cells; OASIS, old astrocyte specifically induced substance; PERK, protein kinase R-like ER kinase PERK; SERCA, sarco/endoplasmic reticulum Ca2+-ATPase; STAT, signal transducer and activator of transcription.
Extra-reticular UPR markers
In addition to the reticular chaperones, other proteins are synthesized to restore ER function. One of these, sorcin, has been shown more recently to play a multifunctional role in ER stress (Colotti et al., 2014). Soluble resistance-related calcium-binding protein (sorcin), like calpains, belongs to the penta-EF hand CBP family, which we will address later. Sorcin is a homodimer of 22 kDa that is highly expressed in the brain, heart, and several types of cancer cells, including glioma (Yokota et al., 2006). In fact, resistance in the name of this protein refers to its involvement in the resistance to chemotherapeutics in cancer cells. With regard to the UPR, this protein functions primarily by restoring reticular Ca2+ levels, inhibiting the Ca2+ efflux through ryanodine channels and stimulating entry through Ca2+-ATPase, as well as regulating L-type calcium channels and Na+-Ca2+ exchangers in the plasma membrane. Most data have been obtained in cardiomyocytes (Meyers et al., 1995; Matsumoto et al., 2005; Fowler et al., 2008) but the presence (Clemen et al., 2003) and co-localization of sorcin with ryanodine channels in astrocytes has been reported (Pickel et al., 1997). Taken together the data regarding brain sorcin indicate that this protein could be an early marker for neurodegenerative diseases (Genovese et al., 2020).
It should be pointed out that the UPR is an acute and adaptive response and, in situations in which triggering elements remain active, a new scenario arises, where UPR failure can lead to cell death, commonly mediated by the CCAAT-enhancer-binding protein homologous protein (CHOP; 29 kDa). Sorcin, in addition to regulating the flow of Ca2+, opposes ER stress, modulates the ATF6 sensor (Parks et al., 2021), likely promoting ERAD and inhibiting the synthesis of CHOP. However, all ER stress sensors potentially activate downstream signals for CHOP synthesis and prolonged reticular stress elevates CHOP, which in turn triggers cellular apoptosis involving several mechanisms (see Hu et al., 2019 for a review). These data emphasize the importance of the sorcin/CHOP ratio for characterizing the UPR phase and its association with degenerative diseases.
Calpains are also markers for the UPR. In fact, hypoxia and glucose deprivation of neurons cause UPR, associated with calpain elevation, which is able to induce apoptosis mediated by caspase activation (Wang et al., 2013; de la Cadena et al., 2014). In addition, exposure of APP/PS1 transgenic mice to hypoxia caused a calpain-mediated increment of beta amyloid deposition and tau phosphorylation (Wang et al., 2013). Calpains 1 and 2 are the best characterized calpains in brain tissue; they have a dimeric organization and weigh 80 kDa. The regulatory subunit is identical in calpains and both subunits have a domain containing penta-EF-hand calcium-binding motifs (Sorimachi and Ono, 2012), as observed in sorcin.
The role of calpains in astrocytes has been highlighted, particularly in neurodegenerative diseases such as AD (see Schultz et al., 2021 for a review). In fact, calpains are activated by reticular Ca2+, and many proteins involved in UPR that regulate the flow of this Ca2+ are targets of calpain (e.g., ryanodine channels). In addition to their direct role in Ca2+ signaling, these CBPs have many specific substrates that affect the cytoskeleton, gene expression, and protein degradation, which certainly reinforce their importance in UPR and cell fate.
Another key protein in UPR and a potential marker is calcineurin (CaN), which in turn is also a target of calpain (Mukherjee and Soto, 2011). CaN is the Ca2+-calmodulin protein phosphatase, which has numerous substrates and is a dimmer of 80 kDa, in which the catalytic subunit (CaN-A, 61 kDa) has two α and β isoforms in the brain tissue (Shah et al., 2017). This phosphatase is activated by the binding of calmodulin, a ubiquitous CBP, which displaces an auto-inhibitory domain, or by calpain, which cuts this domain (Wu et al., 2007). Other CBPs such as S100B (astrocyte-specific) and RCaN1 can also modulate CaN activity (Leal et al., 2004; Mitchell et al., 2007). Interestingly, induction of the UPR by Ca2+ depletion has been demonstrated in cultured neuronal cells, primarily due to an accumulation of gangliosides, caused a biphasic activation of the ER stress sensor PERK (Virgolini et al., 2019). An early (1 h) upregulation of CaN was observed and, under prolonged ER stress (48 h), an upregulation of CHOP was seen (where CaN expression was not different from controls), illustrating this mechanism discussed so far. This early increase in CaN was also observed in ER-Ca2+ depleted astrocytes in culture, where CaN (Aβ) was able to modulate PERK, promoting its oligomerization, independently of its phosphatase activity (Chen et al., 2016). This early protective effect of the astroglial CaN/PERK pathway was also identified in in vivo models of stroke and traumatic brain injury (Chen et al., 2016). This interaction of UPR-induced CaN (Aα) and PERK, as well as ER- Ca2+-ATPase, was previously described in cultured astrocytes (Bollo et al., 2010). Taken together, data indicate that CaN is a marker of UPR and has a protective role at the beginning of the response. However, prolonged astroglial CaN activation (involving calpain activity) may underly neuroinflammation and neurodegenerative diseases such as AD (Furman and Norris, 2014; Pleiss et al., 2016; Dos Santos et al., 2020).
It is not clear whether glial fibrillary acidic protein (GFAP), the classic marker of glial reactivity, can be measured to assess UPR in astrocytes. Data in the literature show that the increase in GFAP follows specific UPR markers, both in astrocyte cultures (Chen et al., 2016; Fan and He, 2016) and in in vivo injury models (Chen et al., 2016; Wang et al., 2019). GFAP has a complex gene regulation, where multiple transcription factors are potentially regulated by calpain and calcineurin. Apparently, all UPR sensors (Saito, 2014; Mehrbod et al., 2019) activation could affect GFAP gene expression.
UPR spreading and viral infection
At this point, it is important to draw attention to a phenomenon that amplifies the impact of UPR, the reticular transmissibility in which molecules, during the UPR, can signal to neighboring cells, modifying the functionality of the ER, either preparing them for adaptation or inducing them to a functional failure. This phenomenon was first described in tumor cells (Mahadevan et al., 2011). Experiments using conditioned media from neural cell cultures (Meares et al., 2014; Sprenkle et al., 2017, 2019) emphasize the role of astrocytes in the transmissibility of ER stress. Surprisingly, although inflammatory mediators (cytokines) are the result of astroglial UPR, they are not the messengers of this transmissibility, which also does not seem to involve exosomes or vesicular particles.
How can Sars-CoV-2 trigger UPR or contribute to its aggravation? Potentially, many viruses such as coronavirus, as well as arboviruses, influenza virus, and human immunodeficiency virus (HIV) are able to induce the UPR (Mehrbod et al., 2019). At the beginning of the pandemic, it was not known whether the virus could infect neural cells and whether the effects on the CNS observed were consequences of the systemic impact induced by the cytokine storm or by ischemia (due the coagulopathy, red blood cell agglutination and hypoxemia). We now know that Sars-CoV-2 affects the CNS through direct infection and through systemic effects (e.g., Murta et al., 2020). Figure 2 summarizes the routes and potential mechanisms that trigger UPR. In the case of Sars-CoV-2, it has been proposed that there is a huge demand for double membrane vesicles for viral replication (which come from ER) and increased protein traffic in the ER, due to the synthesis of structural transmembrane proteins of the virus, such as the S protein. This overloads ER activity and induces UPR (Prestes et al., 2021). Moreover, viral protein E is able to oligomerize and works as a viroporin, promoting Ca2+ efflux from the ER and consequently the UPR (Cao et al., 2021). In fact, confirming the occurrence of the UPR in coronavirus-infected cells, PERK activation and increases in GRP78 and GRP94 expression were detected (Chan et al., 2006; Palmeira et al., 2020). The commitment of astrocytes in patients with COVID-19 has been suggested by increases in blood serum GFAP, as well as neuronal damage from increased tau proteins and L neurofilaments (Pilotto et al., 2021; Virhammar et al., 2021). It is possible that the efflux of Ca2+, caused by protein E itself, could directly activate the NLRP3/inflammasome (Cao et al., 2021). Thus, reticular Ca2+ depletion leads to two possibilities; activation of the UPR (via the ER stress sensors) and/or inflammasome assembly, both of which are closely related mechanistically to the development of neurodegenerative diseases. Furthermore, it should be emphasized that, as mentioned, the UPR per se spreads, leading to what could be called a contagious UPR, without the need for the virus to be present.
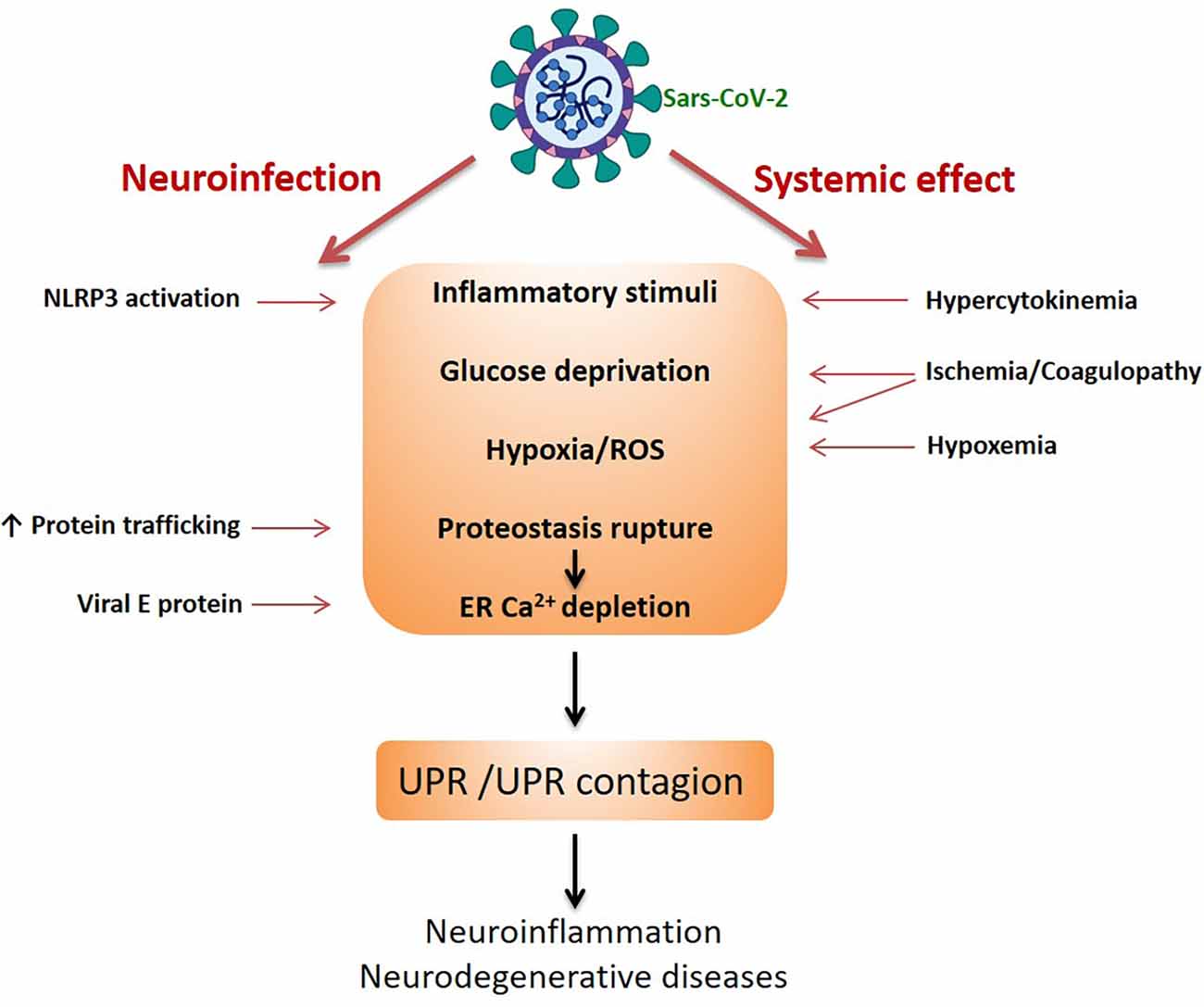
Figure 2. Sars-CoV-2-induced UPR in neural cells. The scheme highlights the two pathways of damage to the CNS by the coronavirus, indicating the possible mechanisms triggering UPR. The UPR and the intercellular spread of the UPR (UPR contagion) would be the basis of neurodegenerative diseases.
Putative Neuroprotective Drugs Against Post-COVID-19 Disorders
The first reports from COVID-19 showed a worse outcome in individuals with diabetes mellitus (Guo et al., 2020). These observations were accompanied by speculative attempts to explain the mediators involved, such as serum amyloid A protein (Gonçalves and Sesterheim, 2021) or arachidonic acid (Das, 2021). Furthermore, doubts persist as to whether medications used for the treatment of diabetes mellitus should be continued (Cheng et al., 2020; Fedson, 2020; Gao et al., 2020; Hariyanto and Kurniawan, 2020; Cariou et al., 2021; Kow et al., 2021). Some studies indicate benefits from the use of metformin, a blood glucose lowering agent, and statins, which inhibit the enzyme that regulates cholesterol synthesis. It is not our intention to discuss all the possible mechanisms of these drugs but to seek their associations with the UPR and, consequently, with their potential to attenuate or prevent the development of neurodegenerative diseases, associated with cognitive deficit and dementia. It should be noted that all of these drugs act beneficially on astrocytic functions in different models of injury; however, their specific role in the UPR of these cells is less explored (e.g., Déry and LeBlanc, 2017; Natrus et al., 2022). A summary of the data presented in this section about the effects of metformin, statins, and Ca2+ channel blockers (CCB) on UPR and neuroprotection is presented in Table 1.
People with diabetes mellitus (with poor glycemic control) are more prone to hospitalization and worse outcome following COVID-19 infection (Singh et al., 2020; Schlesinger et al., 2021). Since metformin is a blood glucose reducer, the benefit of this drug seems obvious. However, insulin does not provide the same protection in COVID-19 patients, suggesting that the effect does not depend exclusively on lowering blood glucose (Kastora et al., 2022). The effect of metformin is attributed to the activation of AMP-activated protein kinase (AMPK), a key enzyme in cell metabolism (see Varghese et al., 2021 for a detailed review). However, the primary action of metformin is the inhibition of mitochondrial complex 1, which alters the AMP/ATP ratio. This inhibition, in addition to increasing the levels of AMP, reduces the generation of mitochondrial ROS, which in turn, due to the structural and functional intimacy between the organelles, through the MAMs (mitochondria-associated endoplasmic reticulum membranes; Vance, 2014), reduces the efflux of reticular Ca2+ (Figure 3). Furthermore, metformin appears to inhibit store-operated calcium entry (SOCE; Soberanes et al., 2019), which we will address later.
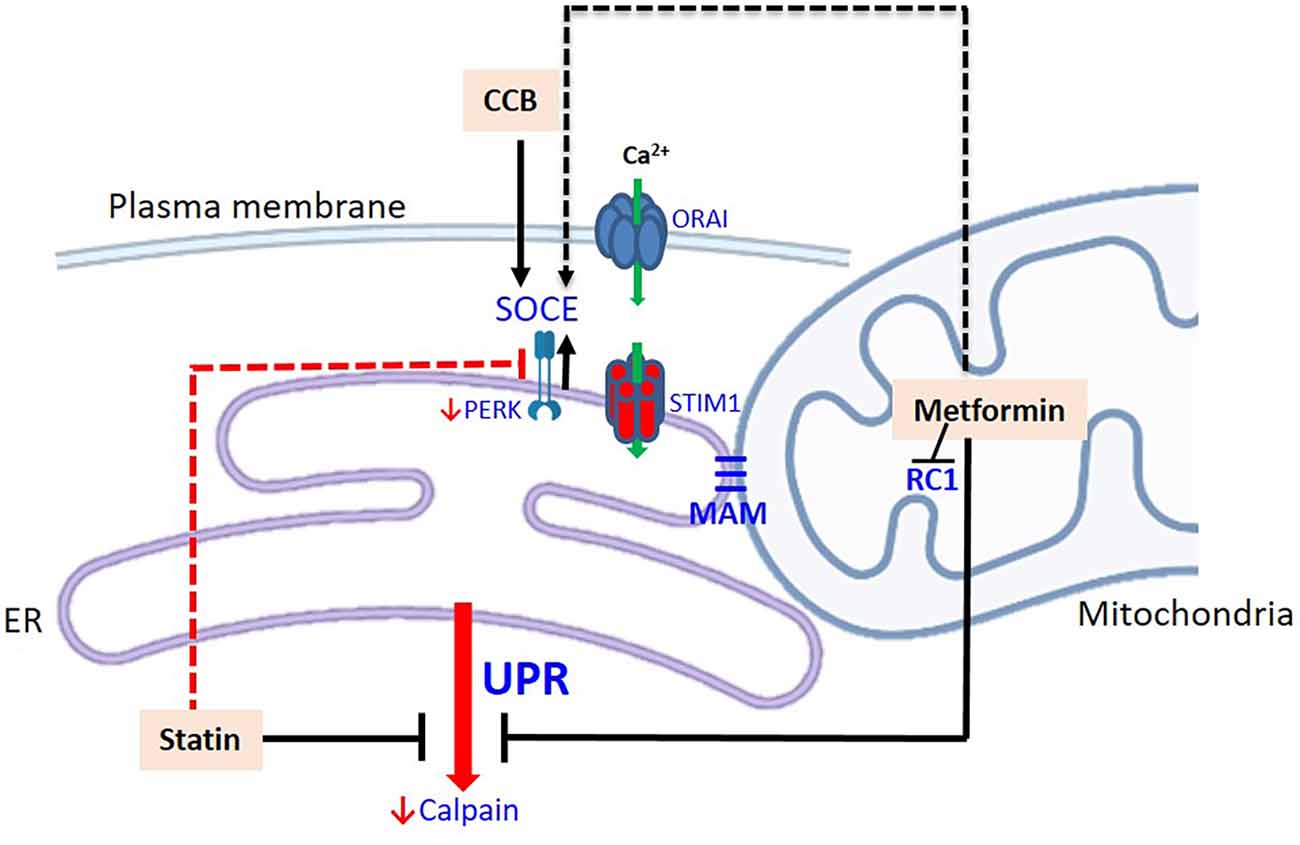
Figure 3. Modulation of the UPR by drugs prescribed for patients with diabetes mellitus. Metformin inhibits the respiratory chain complex 1 (decreasing ROS) and consequently the efflux of Ca2+ from the ER. This could potentially stimulate Ca2+ entry via SOCE. Statins down-regulate the expression of CBP chaperones of the ER, calpains, and PERK. Potentially, statins have a dual role, as downregulation of PERK can decrease Ca2+ entry into the ER, via SOCE. CCB stimulates STIM-1 and increase the input of Ca2+ to the ER. The dashed routes refer to potential pathways and their effects on neural cells, particularly astrocytes, need to be confirmed. Abbreviations: CCB, calcium channel blockers; MAM, mitochondria-associated ER membrane; RC1, respiratory chain complex 1; STIM-1, stromal-interacting molecule 1.
The ability of metformin to reduce the UPR (based on GRP94 and CHOP changes) was initially reported in renal tubular cell cultures, where the effect was independent of AMPK activation (Thériault et al., 2011). More recently, the abrogating effect of metformin against the UPR and its neuroprotection has been confirmed in STZ-induced diabetic mice (Docrat et al., 2020) and senescence-accelerated mice (Liu et al., 2020). Moreover, the protection of neural cells by metformin could involve the inhibition of Cdk5 activation by inhibiting calpain, a downstream UPR enzyme (Wang Y. et al., 2020). These findings indicate possible neurological benefits when maintaining metformin use in diabetic patients during COVID-19, although neuroprotection has not been properly evaluated (Ibrahim et al., 2021; Zangiabadian et al., 2021). In fact, benefits were observed in patients with a moderate cognitive deficit, who were properly assessed with the ADAS-Cog (AD assessment scale—cognitive subscale; Luchsinger et al., 2016). However, the use of metformin and its neuroprotective potential cannot be seen as a panacea for post-COVID-19 neurological disorders, as other pre-COVID-19 results contradict this neuroprotection (Ping et al., 2020).
Statins are cholesterol-lowering compounds and are the first drug of choice for the treatment of dyslipidemias in cardiovascular disease and diabetes mellitus patients (van Stee et al., 2018). Although the mechanism of action of statins involves the reduction of cholesterol synthesis by inhibiting the enzyme, HMG-CoA reductase, the effects of these compounds are potentially very wide, as they not only affect the synthesis of cholesterol and derivatives but also affect the prenylation of many proteins. This justifies, in part, the pleiotropic effect of statins, including their anti-inflammatory and neuroprotective effects (Barone et al., 2014; Oesterle and Liao, 2019). Clinical benefits of statins in COVID-19 patients were raised based on their anti-inflammatory effects, particularly on NF-kB and NLRP3 (e.g., Liu et al., 2021). In fact, a recent meta-analysis suggests that in-hospital statin use leads to a significant reduction in all-cause mortality in COVID-19 cases (Vahedian-Azimi et al., 2021). However, some results are questionable, indicating clinical benefits only in non-severe COVID-19 patients (Fedson, 2020; Chow et al., 2021; Hariyanto and Kurniawan, 2021).
The use of statins is currently widespread with the expectation of a reduction in cognitive deficits with age and a reduction in the risk of dementia (Schultz et al., 2018). Clinical trials with statins in cardiovascular disease (CVD) patients indicate benefits, but suggest that they do not modify the risk for dementia (Gurm and Hoogwerf, 2003; McGuinness et al., 2016). However, experimental data support this possibility since statins reduce UPR markers in different models of ER stress in brain tissue (Urban et al., 2009; Liu et al., 2018; Mounier et al., 2021), although the mechanism remains unknown (Mollazadeh et al., 2018). Calpains could be targets of statins, since their expressions are downregulated in neural and non-neural cells (Li et al., 2015; Han et al., 2019).
Interestingly, some calpain inhibitors have been proposed as antiviral compounds against Sars-CoV-2 infection (Rut et al., 2020; Sacco et al., 2020; Milligan et al., 2021). The viral target of calpain inhibitors is the main protease (Mpro or Nsp5), responsible for activating/release of many non-structural viral proteins (Nsp), such as the RNA-dependent RNA polymerase (Nsp12); inhibition of Mpro prevents viral replication. However, caution is needed with these potential antiviral compounds as they affect a key enzyme for host UPR, which in turn affects multiple substrates in various cellular activities (Sorimachi and Ono, 2012). Calpain inhibitors for AD are also in preclinical studies (Lon et al., 2019) and these investigations should take into account the cell-specific effects of calpain. Calpain appears to favor beta amyloid formation and accumulation in neurons, but it is possible that it is responsible for the beta amyloid clearance performed by astrocytes (Schultz et al., 2021).
In addition to calpains, the expressions of other UPR proteins are also downregulated by statins, such as GRP78 and GRP94, and the sensor protein PERK itself (Mollazadeh et al., 2018). Therefore, statins could reduce the UPR as a whole. It is noteworthy that PERK can play a modulating role in store-operated calcium entry (van Vliet and Agostinis, 2016). SOCE is the main reticular Ca2+ replacement mechanism. ER Ca2+ depletion is detected by the CBP chaperones, which detach from the ER stress sensor proteins, as already discussed (see Figure 1), and are detected by the transmembrane reticular protein, STIM1 (stromal-interacting molecule 1; Figure 2), which (like the ER stress sensor proteins) oligomerizes. STIM1 has its own EF-hand calcium-binding sites that “feel” Ca2+ depletion. Oligomeric STIMs connect to specific plasma membrane Ca2+ channels and allow Ca2+ to enter to replenish reticular Ca2+, working in coordination with ER Ca2+ ATPase. Activated PERK could modulate the connection of STIM with membrane channels, promoting Ca2+ replenishment (van Vliet and Agostinis, 2016).
Thus, statins may potentially have a dual role, as they are able to reduce the activation of the UPR, and also reduce the replenishment of reticular Ca2+ (by decreasing PERK expression). This duality may contribute to understanding the antitumor role of statins. In fact, inhibition of PERK by simvastatin favors the death of U87 glioblastoma cells (Dastghaib et al., 2020). The final result would depend on the machinery involved, varying in each cell type. Cultured astrocytes express reticular STIM1 and the plasma membrane Ca2+ channels, ORAI/CRAC 1–3, and TRPC1, which when coupled to STIM1 mediate the replacement of Ca2+ (Kwon et al., 2017).
Other potential modulators of the UPR, Ca2+ channel blockers (CCB), have also been widely used to treat hypertension in CVD and diabetes mellitus patients (Bergantin, 2019; Zhu et al., 2021). These drugs block L-type voltage-dependent Ca2+ channels (LVCC), reducing cardiac and vascular contractility. Several reports show the benefits of these drugs in COVID-19, where they reduce the need for intensive care and mortality (Zhang et al., 2020; Crespi and Alcock, 2021). Experimental data have reinforced the possible benefits of these drugs in COVID-19 cases (Manohar et al., 2021; Straus et al., 2021).
As we mentioned, SOCE is closely related to ER stress and, consequently, to neurodegenerative diseases (van Vliet and Agostinis, 2016; Secondo et al., 2018). In addition to these channels, it is well documented that blocking LVCC can also prevent ER stress (e.g., Wang et al., 2011). Although the mechanism is not completely understood, a positive modulation of STIM-1 by CCB in vascular smooth muscle cells has been suggested (Johnson et al., 2020). In agreement with the possibility of neuroprotection, the induction of hippocampal UPR by the infusion of beta amyloid peptides into the entorhinal cortex was blocked by CCB, which not only reversed the increases in markers (GRP78 and CHOP) but also improved the cognitive deficit in these animals (Ghanbari-Maman et al., 2019). In other words, these blockers may represent potential neuroprotective drugs in COVID-19, but broader clinical studies such as those performed with ACE inhibitors for the treatment of hypertension in COVID-19 patients, are still lacking (Xu et al., 2021). It is worth remembering that clinical studies did not convincingly show that antihypertensive drugs (including CCB) could reduce cognitive impairment or the risk of dementia (Cunningham et al., 2021). However, a trial with CBB nilvadipine was able to improve cognition (assessed by the ADAS-Cog) in a population with a moderate cognitive deficit (Lawlor et al., 2018).
It is important to raise some criticisms of the pharmacological therapeutic approach of this review and of how we have conducted this discussion. Metformin, statins and CCB potentially act by blocking UPR or activating SOCE as possible protective mechanisms. Parenthetically, recent results in cardiomyocytes indicate that metformin, in addition to blocking the UPR, could potentially stimulate SOCE, where it acts by blocking L-type channels (Wang H. et al., 2020; see Figure 3). However, we cannot take such a simplified view of intervention. In AD, a reduction of STIM-1 has been reported and, therefore inhibiting SOCE could aggravate the disease. Conversely, inhibition of SOCE seems to be neuroprotective in Huntington’s disease (Secondo et al., 2018). Similarly, SOCE blockade provided neuroprotection to cultured neurons submitted to hyperglycemia (Xu et al., 2016). Another aspect that deserves attention is the fact that neural cell heterogeneity is commonly reduced to the neuron. We often discuss neuroprotection as neuronal protection, and do not value the dysfunction of other cells, in particular astrocytes, which may precede neuronal dysfunction. Finally, it is worth emphasizing that we often discuss UPR and SOCE without taking into account the nature of the stimulus that is inducing the response; this can lead to undue generalizations. For example, cultured astrocytes in the presence of LPS respond with a decrease in SOCE, but respond to amyloid β42 with an increase in SOCE (Ronco et al., 2014).
Concluding Remarks
We would like to summarize some ideas, based on references presented throughout the text. A large quantity of material has been, and is still being, produced about the Sars-CoV-2 pandemic. We have addressed a part of these studies and, therefore, such ideas are possibly far from the conclusions that we will learn from this pandemic with regard to its impact on the CNS, especially in diabetic individuals. However, these ideas express, with many limitations, the result of our attempt to establish a link between UPR (aggravated by Sars-CoV-2) in neural cells and possible neurological outcomes. (i) We have advanced in understanding the efflux and influx of Ca2+ in the ER, which are structurally and functionally coupled to the plasma membrane and mitochondria. Depletion of reticular Ca2+ induces displacement of CBP chaperones and activation of sensor proteins, initiating the UPR. The long-lasting UPR is the trigger for many neurodegenerative diseases. (ii) Considering the role of the astrocyte network in neuronal communication, in immune defense and in the metabolic integration of the brain, we can suggest that the astroglial UPR (and the possibility of “reticular contagion” between cells) may trigger neuroinflammation and neurodegenerative diseases, and represent a target for therapeutic strategies. (iii) As astrocytes are targets of Sars-CoV-2 (and other infectious agents) we may predict the impact of the virus on the activity of brain cells in COVID-19 and post-COVID-19, which may aggravate the ongoing UPR or trigger neurodegenerative diseases. (iv) Our knowledge and therapeutic arsenal (metformin, statins, and CCB) against the UPR, although useful, is still very limited. The pandemic has put us on the spot, but now we have to advance further in understanding these and other neuroprotective drugs, going beyond assessing clinical severity and mortality. (v) We have to advance in the translational investigation of neuroprotection, with more detailed clinical assessment tools to evaluate cognition. All projections about neurodegenerative diseases, and in particular AD, that we made before the pandemic, taking into account the increase in life expectancy and the increase in non-communicable diseases (such as diabetes mellitus), may have aged.
Author Contributions
All authors contributed to the article (conception and elaboration) and approved the submitted version.
Funding
This research was supported by Conselho Nacional de Desenvolvimento Científico e Tecnológico (CNPq), Coordenação de Aperfeiçoamento de Pessoal de Nível Superior (CAPES), Fundação de Amparo à Pesquisa do Estado do Rio Grande do Sul (FAPERGS, PPSUS 21/2551-0000067-8) and Instituto Nacional de Ciência e Tecnologia para Excitotoxicidade e Neuroproteção (INCTEN 465671/2014-4).
Conflict of Interest
The authors declare that the research was conducted in the absence of any commercial or financial relationships that could be construed as a potential conflict of interest.
Publisher’s Note
All claims expressed in this article are solely those of the authors and do not necessarily represent those of their affiliated organizations, or those of the publisher, the editors and the reviewers. Any product that may be evaluated in this article, or claim that may be made by its manufacturer, is not guaranteed or endorsed by the publisher.
References
Alberts, B., Johnson, A., Lewis, J., Raff, M., Roberts, K., and Walter, P. (2008). Molecular Biology of the Cell. Fifth Edn. New York: Garland Science.
Alnefeesi, Y., Siegel, A., Lui, L. M. W., Teopiz, K. M., Ho, R. C. M., Lee, Y., et al. (2021). Impact of SARS-CoV-2 infection on cognitive function: a systematic review. Front. Psychiatry 11:621773. doi: 10.3389/fpsyt.2020.621773
Andrews, M. G., Mukhtar, T., Eze, U. C., Simoneau, C. R., Perez, Y., Mostajo-Radji, M. A., et al. (2021). Tropism of SARS-CoV-2 for developing human cortical astrocytes. bioRxiv [Preprint]. doi: 10.1101/2021.01.17.427024
Bahar, E., Kim, H., and Yoon, H. (2016). ER stress-mediated signaling: action potential and Ca2+ as key players. Int. J. Mol. Sci. 17:1558. doi: 10.3390/ijms17091558
Barone, E., Di Domenico, F., and Butterfield, D. A. (2014). Statins more than cholesterol lowering agents in Alzheimer disease: their pleiotropic functions as potential therapeutic targets. Biochem. Pharmacol. 88, 605–616. doi: 10.1016/j.bcp.2013.10.030
Bergantin, L. B. (2019). Hypertension, diabetes and neurodegenerative diseases: is there a clinical link through the Ca2+/cAMP signalling interaction? Curr. Hypertens. Rev. 15, 32–39. doi: 10.2174/1573402114666180817113242
Bollo, M., Paredes, R. M., Holstein, D., Zheleznova, N., Camacho, P., and Lechleiter, J. D. (2010). Calcineurin interacts with PERK and dephosphorylates calnexin to relieve ER stress in mammals and frogs. PLoS One 5:e11925. doi: 10.1371/journal.pone.0011925
Bravo, R., Parra, V., Gatica, D., Rodriguez, A. E., Torrealba, N., Paredes, F., et al. (2013). Endoplasmic reticulum and the unfolded protein response: dynamics and metabolic integration. Int. Rev. Cell Mol. Biol. 301, 215–290. doi: 10.1016/B978-0-12-407704-1.00005-1
Cama, V. F., Marín-Prida, J., Acosta-Rivero, N., Acosta, E. F., Díaz, L. O., Casadesús, A. V., et al. (2021). The microglial NLRP3 inflammasome is involved in human SARS-CoV-2 cerebral pathogenicity: a report of three post-mortem cases. J. Neuroimmunol. 361:577728. doi: 10.1016/j.jneuroim.2021.577728
Cao, Y., Yang, R., Lee, I., Zhang, W., Sun, J., Wang, W., et al. (2021). Characterization of the SARS-CoV-2 E protein: sequence, structure, viroporin and inhibitors. Protein Sci. 30, 1114–1130. doi: 10.1002/pro.4075
Cariou, B., Goronflot, T., Rimbert, A., Boullu, S., Le May, C., Moulin, P., et al. (2021). Routine use of statins and increased COVID-19 related mortality in patients with type 2 diabetes: results from the CORONADO study. Diabetes Metab. 47:101202. doi: 10.1016/j.diabet.2020.10.001
Chan, C.-P., Siu, K.-L., Chin, K.-T., Yuen, K.-Y., Zheng, B., and Jin, D.-Y. (2006). Modulation of the unfolded protein response by the severe acute respiratory syndrome coronavirus spike protein. J. Virol. 80, 9279–9287. doi: 10.1128/JVI.00659-06
Chen, Y., Holstein, D. M., Aime, S., Bollo, M., and Lechleiter, J. D. (2016). Calcineurin β protects brain after injury by activating the unfolded protein response. Neurobiol. Dis. 94, 139–156. doi: 10.1016/j.nbd.2016.06.011
Cheng, X., Liu, Y.-M., Li, H., Zhang, X., Lei, F., Qin, J.-J., et al. (2020). Metformin is associated with higher incidence of acidosis, but not mortality, in individuals with COVID-19 and pre-existing type 2 diabetes. Cell Metab. 32, 537–547.e3. doi: 10.1016/j.cmet.2020.08.013
Chow, R., Im, J., Chiu, N., Chiu, L., Aggarwal, R., Lee, J., et al. (2021). The protective association between statins use and adverse outcomes among COVID-19 patients: a systematic review and meta-analysis. PLoS One 16:e0253576. doi: 10.1371/journal.pone.0253576
Clemen, C. S., Herr, C., Hövelmeyer, N., and Noegel, A. A. (2003). The lack of annexin A7 affects functions of primary astrocytes. Exp. Cell Res. 291, 406–414. doi: 10.1016/j.yexcr.2003.07.012
Colombo, E., and Farina, C. (2016). Astrocytes: key regulators of neuroinflammation. Trends Immunol. 37, 608–620. doi: 10.1016/j.it.2016.06.006
Colotti, G., Poser, E., Fiorillo, A., Genovese, I., Chiarini, V., and Ilari, A. (2014). Sorcin, a calcium binding protein involved in the multidrug resistance mechanisms in cancer cells. Molecules 19, 13976–13989. doi: 10.3390/molecules190913976
Crespi, B., and Alcock, J. (2021). Conflicts over calcium and the treatment of COVID-19. Evol. Med. Public Health 9, 149–156. doi: 10.1093/emph/eoaa046
Cunningham, E. L., Todd, S. A., Passmore, P., Bullock, R., and McGuinness, B. (2021). Pharmacological treatment of hypertension in people without prior cerebrovascular disease for the prevention of cognitive impairment and dementia. Cochrane Database Syst. Rev. 5:CD004034. doi: 10.1002/14651858.CD004034.pub4
Das, U. N. (2021). Bioactive lipids in COVID-19-further evidence. Arch. Med. Res. 52, 107–120. doi: 10.1016/j.arcmed.2020.09.006
Dastghaib, S., Shojaei, S., Mostafavi-Pour, Z., Sharma, P., Patterson, J. B., Samali, A., et al. (2020). Simvastatin induces unfolded protein response and enhances temozolomide-induced cell death in glioblastoma cells. Cells 9:2339. doi: 10.3390/cells9112339
de la Cadena, S. G., Hernández-Fonseca, K., Camacho-Arroyo, I., and Massieu, L. (2014). Glucose deprivation induces reticulum stress by the PERK pathway and caspase-7- and calpain-mediated caspase-12 activation. Apoptosis 19, 414–427. doi: 10.1007/s10495-013-0930-7
Déry, M.-A., and LeBlanc, A. C. (2017). Luman contributes to brefeldin A-induced prion protein gene expression by interacting with the ERSE26 element. Sci. Rep. 7:42285. doi: 10.1038/srep42285
Docrat, T. F., Nagiah, S., Naicker, N., Baijnath, S., Singh, S., and Chuturgoon, A. A. (2020). The protective effect of metformin on mitochondrial dysfunction and endoplasmic reticulum stress in diabetic mice brain. Eur. J. Pharmacol. 875:173059. doi: 10.1016/j.ejphar.2020.173059
Dos Santos, J. P. A., Vizuete, A. F., and Gonçalves, C.-A. (2020). Calcineurin-mediated hippocampal inflammatory alterations in streptozotocin-induced model of dementia. Mol. Neurobiol. 57, 502–512. doi: 10.1007/s12035-019-01718-2
Fan, Y., and He, J. J. (2016). HIV-1 tat induces unfolded protein response and endoplasmic reticulum stress in astrocytes and causes neurotoxicity through glial fibrillary acidic protein (GFAP) activation and aggregation. J. Biol. Chem. 291, 22819–22829. doi: 10.1074/jbc.M116.731828
Fedson, D. S. (2020). Statin treatment of COVID-19. Am. J. Cardiol. 136, 171–173. doi: 10.1016/j.amjcard.2020.09.050
Fischer, R., Wajant, H., Kontermann, R., Pfizenmaier, K., and Maier, O. (2014). Astrocyte-specific activation of TNFR2 promotes oligodendrocyte maturation by secretion of leukemia inhibitory factor. Glia 62, 272–283. doi: 10.1002/glia.22605
Fowler, M. R., Colotti, G., Chiancone, E., Smith, G. L., and Fearon, I. M. (2008). Sorcin modulates cardiac L-type Ca2+ current by functional interaction with the alpha1C subunit in rabbits. Exp. Physiol. 93, 1233–1238. doi: 10.1113/expphysiol.2008.043497
Furman, J. L., and Norris, C. M. (2014). Calcineurin and glial signaling: neuroinflammation and beyond. J. Neuroinflammation 11:158. doi: 10.1186/s12974-014-0158-7
Gao, Y., Liu, T., Zhong, W., Liu, R., Zhou, H., Huang, W., et al. (2020). Risk of metformin in patients with type 2 diabetes with COVID-19: a preliminary retrospective report. Clin. Transl. Sci. 13, 1055–1059. doi: 10.1111/cts.12897
Genovese, I., Giamogante, F., Barazzuol, L., Battista, T., Fiorillo, A., Vicario, M., et al. (2020). Sorcin is an early marker of neurodegeneration, Ca2+ dysregulation and endoplasmic reticulum stress associated to neurodegenerative diseases. Cell Death Dis. 11:861. doi: 10.1038/s41419-020-03063-y
Ghanbari-Maman, A., Ghasemian-Roudsari, F., Aliakbari, S., Gholami Pourbadie, H., Khodagholi, F., Shaerzadeh, F., et al. (2019). Calcium channel blockade ameliorates endoplasmic reticulum stress in the hippocampus induced by amyloidopathy in the entorhinal cortex. Iran. J. Pharm. Res. 18, 1466–1476. doi: 10.22037/ijpr.2019.111532.13216
Gonçalves, C.-A., and Sesterheim, P. (2021). Serum amyloid A protein has been undervalued as a biomarker of COVID-19. Diabetes Metab. Res. Rev. 37:e3376. doi: 10.1002/dmrr.3376
González-Reyes, R. E., Aliev, G., Ávila-Rodrigues, M., and Barreto, G. E. (2016). Alterations in glucose metabolism on cognition: a possible link between diabetes and dementia. Curr. Pharm. Des. 22, 812–888. doi: 10.2174/1381612822666151209152013
Guan, Y., and Han, F. (2020). Key mechanisms and potential targets of the NLRP3 inflammasome in neurodegenerative diseases. Front. Integr. Neurosci. 14:37. doi: 10.3389/fnint.2020.00037
Guo, W., Li, M., Dong, Y., Zhou, H., Zhang, Z., Tian, C., et al. (2020). Diabetes is a risk factor for the progression and prognosis of COVID-19. Diabetes Metab. Res. Rev. 36:e3319. doi: 10.1002/dmrr.3319
Gurm, H. S., and Hoogwerf, B. (2003). The heart protection study: high-risk patients benefit from statins, regardless of LDL-C level. Cleve. Clin. J. Med. 70, 991–997. doi: 10.3949/ccjm.70.11.991
Han, Q., Liu, Q., Zhang, H., Lu, M., Wang, H., Tang, F., et al. (2019). Simvastatin improves cardiac hypertrophy in diabetic rats by attenuation of oxidative stress and inflammation induced by calpain-1-mediated activation of nuclear factor-κB (NF-κB). Med. Sci. Monit. 25, 1232–1241. doi: 10.12659/MSM.913244
Hariyanto, T. I., and Kurniawan, A. (2021). Statin and outcomes of coronavirus disease 2019 (COVID-19): a systematic review, meta-analysis and meta-regression. Nutr. Metab. Cardiovasc. Dis. 31, 1662–1670. doi: 10.1016/j.numecd.2021.02.020
Hariyanto, T. I., and Kurniawan, A. (2020). Statin therapy did not improve the in-hospital outcome of coronavirus disease 2019 (COVID-19) infection. Diabetes Metab. Syndr. 14, 1613–1615. doi: 10.1016/j.dsx.2020.08.023
Heneka, M. T., Golenbock, D., Latz, E., Morgan, D., and Brown, R. (2020). Immediate and long-term consequences of COVID-19 infections for the development of neurological disease. Alzheimers Res. Ther. 12:69. doi: 10.1186/s13195-020-00640-3
Hu, H., Tian, M., Ding, C., and Yu, S. (2019). The C/EBP homologous protein (CHOP) transcription factor functions in endoplasmic reticulum stress-induced apoptosis and microbial infection. Front. Immun. 9:3083. doi: 10.3389/fimmu.2018.03083
Ibrahim, S., Lowe, J. R., Bramante, C. T., Shah, S., Klatt, N. R., Sherwood, N., et al. (2021). Metformin and Covid-19: focused review of mechanisms and current literature suggesting benefit. Front. Endocrinol. (Lausanne) 12:587801. doi: 10.3389/fendo.2021.587801
Johnson, M. T., Gudlur, A., Zhang, X., Xin, P., Emrich, S. M., Yoast, R. E., et al. (2020). L-type Ca2+ channel blockers promote vascular remodeling through activation of STIM proteins. Proc. Natl. Acad. Sci. U S A 117, 17369–17380. doi: 10.1073/pnas.2007598117
Kastora, S., Patel, M., Carter, B., Delibegovic, M., and Myint, P. K. (2022). Impact of diabetes on COVID-19 mortality and hospital outcomes from a global perspective: an umbrella systematic review and meta-analysis. Endocrinol. Diabetes Metab. 5:e00338. doi: 10.1002/edm2.338
Kow, C. S., Ramachandram, D. S., and Hasan, S. S. (2021). Statin use in patients with diabetes amid COVID-19 pandemic. Diabetes Metab. 48:101298. doi: 10.1016/j.diabet.2021.101298
Kwon, J., An, H., Sa, M., Won, J., Shin, J. I., and Lee, C. J. (2017). Orai1 and Orai3 in combination with stim1 mediate the majority of store-operated calcium entry in astrocytes. Exp. Neurobiol. 26, 42–54. doi: 10.5607/en.2017.26.1.42
Lawlor, B., Segurado, R., Kennelly, S., Olde Rikkert, M. G. M., Howard, R., Pasquier, F., et al. (2018). Nilvadipine in mild to moderate Alzheimer disease: a randomised controlled trial. PLoS Med. 15:e1002660. doi: 10.1371/journal.pmed.1002660
Leal, R. B., Frizzo, J. K., Tramontina, F., Fieuw-Makaroff, S., Bobrovskaya, L., Dunkley, P. R., et al. (2004). S100B protein stimulates calcineurin activity. Neuroreport 15, 317–320. doi: 10.1097/00001756-200402090-00021
Li, M., Zhang, W., Chen, T., and Liu, L. (2015). [Protective effects of levetiracetam and simvastatin on pilocarpine-induced epilepsy in rat models]. Sichuan Da Xue Xue Bao. Yi Xue Ban 46, 201–204.
Lia, A., Henriques, V. J., Zonta, M., Chiavegato, A., Carmignoto, G., Gómez-Gonzalo, M., et al. (2021). Calcium signals in astrocyte microdomains, a decade of great advances. Front. Cell. Neurosci. 15:673433. doi: 10.3389/fncel.2021.673433
Liu, P., Gao, Q., Guan, L., Hu, Y., Jiang, J., Gao, T., et al. (2021). Atorvastatin attenuates surgery-induced BBB disruption and cognitive impairment partly by suppressing NF-κB pathway and NLRP3 inflammasome activation in aged mice. Acta Biochim. Biophys. Sin. (Shanghai) 53, 528–537. doi: 10.1093/abbs/gmab022
Liu, B., Huang, B., Liu, J., and Shi, J.-S. (2020). Dendrobium nobile Lindl alkaloid and metformin ameliorate cognitive dysfunction in senescence-accelerated mice via suppression of endoplasmic reticulum stress. Brain Res. 1741:146871. doi: 10.1016/j.brainres.2020.146871
Liu, W., Zhao, Y., Zhang, X., and Ji, J. (2018). Simvastatin ameliorates cognitive impairments via inhibition of oxidative stress-induced apoptosis of hippocampal cells through the ERK/AKT signaling pathway in a rat model of senile dementia. Mol. Med. Rep. 17, 1885–1892. doi: 10.3892/mmr.2017.8098
Lon, H.-K., Mendonca, N., Goss, S., Othman, A. A., Locke, C., Jin, Z., et al. (2019). Pharmacokinetics, safety, tolerability and pharmacodynamics of alicapistat, a selective inhibitor of human calpains 1 and 2 for the treatment of Alzheimer disease: an overview of phase 1 studies. Clin. Pharmacol. Drug Dev. 8, 290–303. doi: 10.1002/cpdd.598
Luchsinger, J. A., Perez, T., Chang, H., Mehta, P., Steffener, J., Pradabhan, G., et al. (2016). Metformin in amnestic mild cognitive impairment: results of a pilot randomized placebo controlled clinical trial. J. Alzheimers Dis. 51, 501–514. doi: 10.3233/JAD-150493
Mahadevan, N. R., Rodvold, J., Sepulveda, H., Rossi, S., Drew, A. F., and Zanetti, M. (2011). Transmission of endoplasmic reticulum stress and pro-inflammation from tumor cells to myeloid cells. Proc. Natl. Acad. Sci. U S A 108, 6561–6566. doi: 10.1073/pnas.1008942108
Manohar, K., Gupta, R. K., Gupta, P., Saha, D., Gare, S., Sarkar, R., et al. (2021). FDA approved L-type channel blocker Nifedipine reduces cell death in hypoxic A549 cells through modulation of mitochondrial calcium and superoxide generation. Free Radic. Biol. Med. 177, 189–200. doi: 10.1016/j.freeradbiomed.2021.08.245
Martin-Jiménez, C. A., García-Vega, Á., Cabezas, R., Aliev, G., Echeverria, V., González, J., et al. (2017). Astrocytes and endoplasmic reticulum stress: a bridge between obesity and neurodegenerative diseases. Prog. Neurobiol. 158, 45–68. doi: 10.1016/j.pneurobio.2017.08.001
Matschke, J., Lütgehetmann, M., Hagel, C., Sperhake, J. P., Schröder, A. S., Edler, C., et al. (2020). Neuropathology of patients with COVID-19 in Germany: a post-mortem case series. Lancet Neurol. 19, 919–929. doi: 10.1016/S1474-4422(20)30308-2
Matsumoto, T., Hisamatsu, Y., Ohkusa, T., Inoue, N., Sato, T., Suzuki, S., et al. (2005). Sorcin interacts with sarcoplasmic reticulum Ca2+-ATPase and modulates excitation-contraction coupling in the heart. Basic Res. Cardiol. 100, 250–262. doi: 10.1007/s00395-005-0518-7
McGuinness, B., Craig, D., Bullock, R., and Passmore, P. (2016). Statins for the prevention of dementia. Cochrane Database Syst. Rev. CD003160. doi: 10.1002/14651858.CD003160.pub3
Meares, G. P., Liu, Y., Rajbhandari, R., Qin, H., Nozell, S. E., Mobley, J. A., et al. (2014). PERK-dependent activation of JAK1 and STAT3 contributes to endoplasmic reticulum stress-induced inflammation. Mol. Cell. Biol. 34, 3911–3925. doi: 10.1128/MCB.00980-14
Mehrbod, P., Ande, S. R., Alizadeh, J., Rahimizadeh, S., Shariati, A., Malek, H., et al. (2019). The roles of apoptosis, autophagy and unfolded protein response in arbovirus, influenza virus and HIV infections. Virulence 10, 376–413. doi: 10.1080/21505594.2019.1605803
Meyers, M. B., Pickel, V. M., Sheu, S. S., Sharma, V. K., Scotto, K. W., and Fishman, G. I. (1995). Association of sorcin with the cardiac ryanodine receptor. J. Biol. Chem. 270, 26411–26418. doi: 10.1074/jbc.270.44.26411
Milligan, J. C., Zeisner, T. U., Papageorgiou, G., Joshi, D., Soudy, C., Ulferts, R., et al. (2021). Identifying SARS-CoV-2 antiviral compounds by screening for small molecule inhibitors of Nsp5 main protease. Biochem. J. 478, 2499–2515. doi: 10.1042/BCJ20210197
Miners, S., Kehoe, P. G., and Love, S. (2020). Cognitive impact of COVID-19: looking beyond the short term. Alzheimers Res. Ther. 12:170. doi: 10.1186/s13195-020-00744-w
Mitchell, A. N., Jayakumar, L., Koleilat, I., Qian, J., Sheehan, C., Bhoiwala, D., et al. (2007). Brain expression of the calcineurin inhibitor RCAN1 (Adapt78). Arch. Biochem. Biophys. 467, 185–192. doi: 10.1016/j.abb.2007.08.030
Mollazadeh, H., Atkin, S. L., Butler, A. E., Ruscica, M., Sirtori, C. R., and Sahebkar, A. (2018). The effect of statin therapy on endoplasmic reticulum stress. Pharmacol. Res. 137, 150–158. doi: 10.1016/j.phrs.2018.10.006
Mounier, N. M., Wahdan, S. A., Gad, A. M., and Azab, S. S. (2021). Role of inflammatory, oxidative and ER stress signaling in the neuroprotective effect of atorvastatin against doxorubicin-induced cognitive impairment in rats. Naunyn Schmiedebergs Arch. Pharmacol. 394, 1537–1551. doi: 10.1007/s00210-021-02081-7
Mukherjee, A., and Soto, C. (2011). Role of calcineurin in neurodegeneration produced by misfolded proteins and endoplasmic reticulum stress. Curr. Opin. Cell Biol. 23, 223–230. doi: 10.1016/j.ceb.2010.12.006
Murta, V., Villarreal, A., and Ramos, A. J. (2020). Severe acute respiratory syndrome coronavirus 2 impact on the central nervous system: are astrocytes and microglia main players or merely bystanders? ASN Neuro 12:1759091420954960. doi: 10.1177/1759091420954960
Natrus, L. V., Osadchuk, Y. S., Lisakovska, O. O., Labudzinskyi, D. O., Klys, Y. G., and Chaikovsky, Y. B. (2022). Effect of propionic acid on diabetes-induced impairment of unfolded protein response signaling and astrocyte/microglia crosstalk in rat ventromedial nucleus of the hypothalamus. Neural Plast. 2022:6404964. doi: 10.1155/2022/6404964
Oesterle, A., and Liao, J. K. (2019). The pleiotropic effects of statins - from coronary artery disease and stroke to atrial fibrillation and ventricular tachyarrhythmia. Curr. Vasc. Pharmacol. 17, 222–232. doi: 10.2174/1570161116666180817155058
Palmeira, A., Sousa, E., Köseler, A., Sabirli, R., Gören, T., Türkçüer, İ., et al. (2020). Preliminary virtual screening studies to identify grp78 inhibitors which may interfere with SARS-CoV-2 infection. Pharmaceuticals (Basel) 13:132. doi: 10.3390/ph13060132
Parks, S. Z., Gao, T., Jimenez Awuapura, N., Ayathamattam, J., Chabosseau, P. L., Kalvakolanu, D. V., et al. (2021). The Ca2+-binding protein sorcin stimulates transcriptional activity of the unfolded protein response mediator ATF6. FEBS Lett. 595, 1782–1796. doi: 10.1002/1873-3468.14101
Pickel, V. M., Clarke, C. L., and Meyers, M. B. (1997). Ultrastructural localization of sorcin, a 22 kDa calcium binding protein, in the rat caudate-putamen nucleus: association with ryanodine receptors and intracellular calcium release. J. Comp. Neurol. 386, 625–634. doi: 10.1002/(sici)1096-9861(19971006)386:4<625::aid-cne8>3.0.co;2-4
Pilotto, A., Masciocchi, S., Volonghi, I., De Giuli, V., Caprioli, F., Mariotto, S., et al. (2021). Severe acute respiratory syndrome coronavirus 2 (SARS-CoV-2) encephalitis is a cytokine release syndrome: evidences from cerebrospinal fluid analyses. Clin. Infect. Dis. 73, e3019–e3026. doi: 10.1093/cid/ciaa1933
Ping, F., Jiang, N., and Li, Y. (2020). Association between metformin and neurodegenerative diseases of observational studies: systematic review and meta-analysis. BMJ Open Diabetes Res. Care 8:e001370. doi: 10.1136/bmjdrc-2020-001370
Pleiss, M. M., Sompol, P., Kraner, S. D., Abdul, H. M., Furman, J. L., Guttmann, R. P., et al. (2016). Calcineurin proteolysis in astrocytes: implications for impaired synaptic function. Biochim. Biophys. Acta 1862, 1521–1532. doi: 10.1016/j.bbadis.2016.05.007
Prestes, E. B., Bruno, J. C. P., Travassos, L. H., and Carneiro, L. A. M. (2021). The unfolded protein response and autophagy on the crossroads of coronaviruses infections. Front. Cell. Infect. Microbiol. 11:668034. doi: 10.3389/fcimb.2021.668034
Robinson-Agramonte, M. A., Gonçalves, C.-A., Noris-García, E., Préndes Rivero, N., Brigida, A. L., Schultz, S., et al. (2021). Impact of SARS-CoV-2 on neuropsychiatric disorders. World J. Psychiatry 11, 347–354. doi: 10.5498/wjp.v11.i7.347
Ronco, V., Grolla, A. A., Glasnov, T. N., Canonico, P. L., Verkhratsky, A., Genazzani, A. A., et al. (2014). Differential deregulation of astrocytic calcium signalling by amyloid-β, TNFα, IL-1β and LPS. Cell Calcium 55, 219–229. doi: 10.1016/j.ceca.2014.02.016
Rut, W., Lv, Z., Zmudzinski, M., Patchett, S., Nayak, D., Snipas, S. J., et al. (2020). Activity profiling and crystal structures of inhibitor-bound SARS-CoV-2 papain-like protease: a framework for anti-COVID-19 drug design. Sci. Adv. 6:eabd4596. doi: 10.1126/sciadv.abd4596
Sacco, M. D., Ma, C., Lagarias, P., Gao, A., Townsend, J. A., Meng, X., et al. (2020). Structure and inhibition of the SARS-CoV-2 main protease reveals strategy for developing dual inhibitors against Mpro and cathepsin L. bioRxiv [Preprint]. doi: 10.1101/2020.07.27.223727.
Saito, A. (2014). Physiological functions of endoplasmic reticulum stress transducer OASIS in central nervous system. Anat. Sci. Int. 89, 11–20. doi: 10.1007/s12565-013-0214-x
Sashindranath, M., and Nandurkar, H. H. (2021). Endothelial dysfunction in the brain: setting the stage for stroke and other cerebrovascular complications of COVID-19. Stroke 52, 1895–1904. doi: 10.1161/STROKEAHA.120.032711
Schlesinger, S., Neuenschwander, M., Lang, A., Pafili, K., Kuss, O., Herder, C., et al. (2021). Risk phenotypes of diabetes and association with COVID-19 severity and death: a living systematic review and meta-analysis. Diabetologia 64, 1480–1491. doi: 10.1007/s00125-021-05458-8
Schultz, B. G., Patten, D. K., and Berlau, D. J. (2018). The role of statins in both cognitive impairment and protection against dementia: a tale of two mechanisms. Transl. Neurodegener. 7:5. doi: 10.1186/s40035-018-0110-3
Schultz, B., Taday, J., Menezes, L., Cigerce, A., Leite, M. C., and Gonçalves, C.-A. (2021). Calpain-mediated alterations in astrocytes before and during amyloid chaos in Alzheimer’s disease. J. Alzheimers Dis. 84, 1415–1430. doi: 10.3233/JAD-215182
Secondo, A., Bagetta, G., and Amantea, D. (2018). On the role of store-operated calcium entry in acute and chronic neurodegenerative diseases. Front. Mol. Neurosci. 11:87. doi: 10.3389/fnmol.2018.00087
Shah, S. Z. A., Hussain, T., Zhao, D., and Yang, L. (2017). A central role for calcineurin in protein misfolding neurodegenerative diseases. Cell. Mol. Life Sci. 74, 1061–1074. doi: 10.1007/s00018-016-2379-7
Sims, S. G., Cisney, R. N., Lipscomb, M. M., and Meares, G. P. (2022). The role of endoplasmic reticulum stress in astrocytes. Glia 70, 5–19. doi: 10.1002/glia.24082
Singh, A. K., Gupta, R., Ghosh, A., and Misra, A. (2020). Diabetes in COVID-19: prevalence, pathophysiology, prognosis and practical considerations. Diabetes Metab. Syndr. 14, 303–310. doi: 10.1016/j.dsx.2020.04.004
Soberanes, S., Misharin, A. V., Jairaman, A., Morales-Nebreda, L., McQuattie-Pimentel, A. C., Cho, T., et al. (2019). Metformin targets mitochondrial electron transport to reduce air-pollution-induced thrombosis. Cell Metab. 29, 335–347.e5. doi: 10.1016/j.cmet.2018.09.019
Sofroniew, M. V. (2020). Astrocyte reactivity: subtypes, states and functions in cns innate immunity. Trends Immunol. 41, 758–770. doi: 10.1016/j.it.2020.07.004
Sorimachi, H., and Ono, Y. (2012). Regulation and physiological roles of the calpain system in muscular disorders. Cardiovasc. Res. 96, 11–22. doi: 10.1093/cvr/cvs157
Sprenkle, N. T., Lahiri, A., Simpkins, J. W., and Meares, G. P. (2019). Endoplasmic reticulum stress is transmissible in vitro between cells of the central nervous system. J. Neurochem. 148, 516–530. doi: 10.1111/jnc.14642
Sprenkle, N. T., Sims, S. G., Sánchez, C. L., and Meares, G. P. (2017). Endoplasmic reticulum stress and inflammation in the central nervous system. Mol. Neurodegener. 12:42. doi: 10.1186/s13024-017-0183-y
Straus, M. R., Bidon, M. K., Tang, T., Jaimes, J. A., Whittaker, G. R., and Daniel, S. (2021). Inhibitors of L-type calcium channels show therapeutic potential for treating SARS-CoV-2 infections by preventing virus entry and spread. ACS Infect. Dis. 7, 2807–2815. doi: 10.1021/acsinfecdis.1c00023
Thériault, J. R., Palmer, H. J., and Pittman, D. D. (2011). Inhibition of the Unfolded Protein Response by metformin in renal proximal tubular epithelial cells. Biochem. Biophys. Res. Commun. 409, 500–505. doi: 10.1016/j.bbrc.2011.05.034
Toniolo, S., Scarioni, M., Di Lorenzo, F., Hort, J., Georges, J., Tomic, S., et al. (2021). Dementia and COVID-19, a bidirectional liaison: risk factors, biomarkers and optimal health care. J. Alzheimers Dis. 82, 883–898. doi: 10.3233/JAD-210335
Tremblay, M.-E., Madore, C., Bordeleau, M., Tian, L., and Verkhratsky, A. (2020). Neuropathobiology of COVID-19: the role for glia. Front. Cell. Neurosci. 14:592214. doi: 10.3389/fncel.2020.592214
Urban, P., Pavlíková, M., Sivonová, M., Kaplán, P., Tatarková, Z., Kaminska, B., et al. (2009). Molecular analysis of endoplasmic reticulum stress response after global forebrain ischemia/reperfusion in rats: effect of neuroprotectant simvastatin. Cell. Mol. Neurobiol. 29, 181–192. doi: 10.1007/s10571-008-9309-7
Vahedian-Azimi, A., Mohammadi, S. M., Banach, M., Beni, F. H., Guest, P. C., Al-Rasadi, K., et al. (2021). Improved COVID-19 outcomes following statin therapy: an updated systematic review and meta-analysis. Biomed Res. Int. 2021:1901772. doi: 10.1155/2021/1901772
van Stee, M. F., de Graaf, A. A., and Groen, A. K. (2018). Actions of metformin and statins on lipid and glucose metabolism and possible benefit of combination therapy. Cardiovasc. Diabetol. 17:94. doi: 10.1186/s12933-018-0738-4
van Vliet, A. R., and Agostinis, P. (2016). When under pressure, get closer: PERKing up membrane contact sites during ER stress. Biochem. Soc. Trans. 44, 499–504. doi: 10.1042/BST20150272
Vance, J. E. (2014). MAM (mitochondria-associated membranes) in mammalian cells: Lipids and beyond. Biochim. Biophys. Acta 1841, 595–609. doi: 10.1016/j.bbalip.2013.11.014
Varghese, E., Samuel, S. M., Liskova, A., Kubatka, P., and Büsselberg, D. (2021). Diabetes and coronavirus (SARS-CoV-2): molecular mechanism of Metformin intervention and the scientific basis of drug repurposing. PLoS Pathog. 17:e1009634. doi: 10.1371/journal.ppat.1009634
Verkhratsky, A., and Parpura, V. (2014). Calcium signalling and calcium channels: evolution and general principles. Eur. J. Pharmacol. 739, 1–3. doi: 10.1016/j.ejphar.2013.11.013
Virgolini, M. J., Feliziani, C., Cambiasso, M. J., Lopez, P. H., and Bollo, M. (2019). Neurite atrophy and apoptosis mediated by PERK signaling after accumulation of GM2-ganglioside. Biochim. Biohys. Acta Mol. Cell Res. 1866, 225–239. doi: 10.1016/j.bbamcr.2018.10.014
Virhammar, J., Nääs, A., Fällmar, D., Cunningham, J. L., Klang, A., Ashton, N. J., et al. (2021). Biomarkers for central nervous system injury in cerebrospinal fluid are elevated in COVID-19 and associated with neurological symptoms and disease severity. Eur. J. Neurol. 28, 3324–3331. doi: 10.1111/ene.14703
Volpe, C. M. O., Anjos, P. M. F., and Nogueira-Machado, J. A. (2016). Inflammasome as a new therapeutic target for diabetic complications. Recent Patents Endocr. Metab. Immune Drug Discov. 10, 56–62. doi: 10.2174/1872214810666160219163314
Wang, Z.-F., Gao, C., Chen, W., Gao, Y., Wang, H.-C., Meng, Y., et al. (2019). Salubrinal offers neuroprotection through suppressing endoplasmic reticulum stress, autophagy and apoptosis in a mouse traumatic brain injury model. Neurobiol. Learn. Mem. 161, 12–25. doi: 10.1016/j.nlm.2019.03.002
Wang, Y., Gao, L., Li, Y., Chen, H., and Sun, Z. (2011). Nifedipine protects INS-1 β-cell from high glucose-induced ER stress and apoptosis. Int. J. Mol. Sci. 12, 7569–7580. doi: 10.3390/ijms12117569
Wang, H., Wang, C., Lu, Y., Yan, Y., Leng, D., Tian, S., et al. (2020). Metformin shortens prolonged QT interval in diabetic mice by inhibiting L-type calcium current: a possible therapeutic approach. Front. Pharmacol. 11:614. doi: 10.3389/fphar.2020.00614
Wang, C.-Y., Xie, J.-W., Wang, T., Xu, Y., Cai, J.-H., Wang, X., et al. (2013). Hypoxia-triggered m-calpain activation evokes endoplasmic reticulum stress and neuropathogenesis in a transgenic mouse model of Alzheimer’s disease. CNS Neurosci. Ther. 19, 820–833. doi: 10.1111/cns.12151
Wang, Y., Zhao, J., Guo, F.-L., Gao, X., Xie, X., Liu, S., et al. (2020). Metformin ameliorates synaptic defects in a mouse model of AD by inhibiting Cdk5 activity. Front. Cell. Neurosci. 14:170. doi: 10.3389/fncel.2020.00170
Wu, H.-Y., Tomizawa, K., and Matsui, H. (2007). Calpain-calcineurin signaling in the pathogenesis of calcium-dependent disorder. Acta Med. Okayama 61, 123–137. doi: 10.18926/AMO/32905
Xu, J., Teng, Y., Shang, L., Gu, X., Fan, G., Chen, Y., et al. (2021). The effect of prior angiotensin-converting enzyme inhibitor and angiotensin receptor blocker treatment on coronavirus disease 2019 (COVID-19) susceptibility and outcome: a systematic review and meta-analysis. Clin. Infect. Dis. 72, e901–e913. doi: 10.1093/cid/ciaa1592
Xu, Z., Xu, W., Song, Y., Zhang, B., Li, F., and Liu, Y. (2016). Blockade of store-operated calcium entry alleviates high glucose-induced neurotoxicity via inhibiting apoptosis in rat neurons. Chem. Biol. Interact. 254, 63–72. doi: 10.1016/j.cbi.2016.05.025
Yokota, T., Kouno, J., Adachi, K., Takahashi, H., Teramoto, A., Matsumoto, K., et al. (2006). Identification of histological markers for malignant glioma by genome-wide expression analysis: dynein, alpha-PIX and sorcin. Acta Neuropathol. 111, 29–38. doi: 10.1007/s00401-005-1085-6
Zangiabadian, M., Nejadghaderi, S. A., Zahmatkesh, M. M., Hajikhani, B., Mirsaeidi, M., and Nasiri, M. J. (2021). The efficacy and potential mechanisms of metformin in the treatment of COVID-19 in the diabetics: a systematic review. Front. Endocrinol. (Lausanne) 12:645194. doi: 10.3389/fendo.2021.645194
Zhang, L.-K., Sun, Y., Zeng, H., Wang, Q., Jiang, X., Shang, W.-J., et al. (2020). Calcium channel blocker amlodipine besylate therapy is associated with reduced case fatality rate of COVID-19 patients with hypertension. Cell Discov. 6:96. doi: 10.1038/s41421-020-00235-0
Zhou, Y., Chi, J., Lv, W., and Wang, Y. (2021). Obesity and diabetes as high-risk factors for severe coronavirus disease 2019 (Covid-19). Diabetes Metab. Res. Rev. 37:e3377. doi: 10.1002/dmrr.3377
Keywords: astrocyte, calcium-binding proteins, COVID-19, diabetes mellitus, neuroprotection, UPR
Citation: Gonçalves C-A, Sesterheim P,Wartchow KM, Bobermin LD, Leipnitz G and Quincozes-Santos A (2022) Why antidiabetic drugs are potentially neuroprotective during the Sars-CoV-2 pandemic: The focus on astroglial UPR and calcium-binding proteins. Front. Cell. Neurosci. 16:905218. doi: 10.3389/fncel.2022.905218
Received: 26 March 2022; Accepted: 30 June 2022;
Published: 29 July 2022
Edited by:
Nektarios Barabutis, University of Louisiana at Monroe, United StatesReviewed by:
Yu-Feng Wang, Harbin Medical University, ChinaAlejandro Villarreal, University of Freiburg, Germany
Copyright © 2022 Gonçalves, Sesterheim, Wartchow, Bobermin, Leipnitz and Quincozes-Santos. This is an open-access article distributed under the terms of the Creative Commons Attribution License (CC BY). The use, distribution or reproduction in other forums is permitted, provided the original author(s) and the copyright owner(s) are credited and that the original publication in this journal is cited, in accordance with accepted academic practice. No use, distribution or reproduction is permitted which does not comply with these terms.
*Correspondence: Carlos-Alberto Gonçalves, casg@ufrgs.br