- 1Department of Neurology, Huashan Hospital, Fudan University, Shanghai, China
- 2Department of Neurology, Pudong People’s Hospital, Shanghai, China
- 3State Key Laboratory of Medical Neurobiology and MOE Frontiers Center for Brain Science, Department of Neurology, Huashan Hospital, Fudan University, Shanghai, China
Chronic cerebral hypoperfusion (CCH) is a major cause of vascular cognitive impairment and dementia (VCID). Although the underlying mechanisms have not been fully elucidated, the emerging data suggest that blood–brain barrier (BBB) dysfunction is one of the pivotal pathological changes in CCH. BBB dysfunction appears early in CCH, contributing to the deterioration of white matter and the development of cognitive impairment. In this review, we summarize the latest experimental and clinical evidence implicating BBB disruption as a major cause of VCID. We discuss the mechanisms of BBB dysfunction in CCH, focusing on the cell interactions within the BBB, as well as the potential role of APOE genotype. In summary, we provide novel insights into the pathophysiological mechanisms underlying BBB dysfunction and the potential clinical benefits of therapeutic interventions targeting BBB in CCH.
Introduction
As the aging population grows, cognitive impairment, consisting of mild cognitive impairment and dementia, has become a prominent health challenge worldwide. Globally, the number of people afflicted with dementia more than doubled from 20.2 million in 1990 to 43.8 million in 2016, while this figure is estimated to more than triple in 2050 (152.8 million; GBD, 2019, 2022). In China, the prevalence of dementia was 5.60% in 2019 among individuals aged above 65 years (Jia et al., 2020). Vascular cognitive impairment and dementia (VCID), which refers to cognitive impairment and dementia attributed to vascular risks, is deemed as the second leading cause of dementia (van der Flier et al., 2018).
A growing amount of evidence from animal models and epidemiological investigations in humans suggests that chronic cerebral hypoperfusion (CCH) is one of the major pathophysiological hallmarks of cognitive decline (Washida et al., 2019; Zlokovic et al., 2020; Rundek et al., 2021). CCH, despite without a clear definition, is implicated in multiple morbid conditions like heart failure, hypotension, and carotid stenosis (Iturria-Medina et al., 2016; Ciacciarelli et al., 2020), whose relationships to cognitive function have been verified in previous research. For instance, the Whitehall II cohort study demonstrated that adults with atrial fibrillation were associated with accelerated cognitive decline and a higher risk of dementia (Singh-Manoux et al., 2017). Besides, a meta-analysis on the relationship between orthostatic hypotension and cognition also indicated that orthostatic hypotension was associated with an increased risk of dementia and cognitive dysfunction (Peters et al., 2018).
The white matter hyperintensity (WMH) is regarded as the direct manifestation of CCH (Prins and Scheltens, 2015; Prabhakaran, 2019). In a population with high vascular risks, WMH volume was negatively correlated to local perfusion (Jann et al., 2021). Cerebral blood flow (CBF) was lower in WMH (Stewart et al., 2021), and WMH was related to higher risks of cognitive impairment and dementia. Increasing WMH volume started 10.6 years before mild cognitive impairment (MCI) onset on average (Silbert et al., 2012). Moreover, based on a meta-analysis summarizing 23 cross-sectional and 14 longitudinal studies, WMHs exerted a global effect on cognition (Kloppenborg et al., 2014). Taken together, these studies substantiate the pivotal role of CCH in cognition.
The blood–brain barrier (BBB), mainly comprised of cerebral endothelial cells, astrocytes, pericytes, microglia, and basement membrane, is a selective and dynamic interface that separates the central nervous system from peripheral vascularization (Langen et al., 2019; Pandit et al., 2020). By regulating the exchange of substances and cells between neuronal tissues and circulating blood, the BBB maintains the local central nervous environment and homeostasis (Segarra et al., 2021). However, BBB permeability varies under pathological situations, leading to brain dysfunction (Ueno et al., 2016; Pan and Nicolazzo, 2018). For instance, BBB leakage seemed to appear before the onset of hippocampus dystrophy in the early stage of Alzheimer’s disease (AD; Montagne et al., 2019). Besides, BBB impairment and CCH are closely linked to cerebral small vessel disease (CSVD; Wong et al., 2019). Also, BBB failure could predict a poorer functional outcome in patients with CSVD (Wardlaw et al., 2013).
Normal appearing white matter (NAWM) or white matter penumbra, owing to its inclination to evolve into WMH (Maillard et al., 2011), refers to subtle white matter injuries that are undetectable by conventional magnetic resonance imaging (MRI) but are only visible using novel imaging techniques such as diffusion tensor imaging (DTI) and magnetization transfer imaging (MTI; Schmidt et al., 2011). Similar to WHM, lower CBF is also presented in NAWM: the closer to the WMH region, the lower the CBF (Promjunyakul et al., 2015; Wong et al., 2019). Using DCE-MRI, BBB leakage in NAWM was also confirmed in SVD cases (Zhang et al., 2017a). Precisely, leakage rate and area under the leakage curve in the NAWM were found to be proportional to the total CSVD burden in MRI (Li et al., 2018). Furthermore, studies revealed the correlation of NAWM to cognitive performance, which was even better than WMH. In a 2-year follow-up study, higher BBB leakage volume at baseline in NAWM was associated with an increased overall cognitive decline (Kerkhofs et al., 2021). Therefore, BBB disruption is an early change that holds significant prognostic value in CCH-induced cognitive impairment, offering a critical time window for therapeutic intervention.
In conclusion, the clinical evidence indicates that BBB dysfunction is a cardinal factor in CCH-induced VICD.
Experimental and Clinical Evidence of Blood–Brain Barrier Dysfunction in Chronic Cerebral Hypoperfusion
Experimental Evidence
Blood–Brain Barrier Integrity Detection Based on Structural Components in vitro
The intact components of BBB, both at the cell level and the molecular level, ensure its normal functions. Given the ultrastructure, such as the smallest capillaries in the rat brain that are about 4 μm in diameter (Wong et al., 2013), the electron microscope (EM) is applied to directly detect the constituents of BBB. For instance, attenuated capillary density, capillary lumen diameter, increased pericytic degeneration, and the number of endothelial mitochondria were observed under EM in rats that underwent bilateral occlusion of the common carotid arteries (BCAO), concluding that the breakdown of BBB leads to compromised spatial memory (de Wilde et al., 2002). Besides, EM was also used in the observation of large gaps between tight junctions (TJs) of endothelial cells in CCH rats with impaired behavioral performance in the Morris water maze test (Xu et al., 2020).
Furthermore, researchers also developed methods to gage the BBB integrity from a molecular angle. TJs that seal the endothelium preventing BBB leakage (Tietz and Engelhardt, 2015) are widely utilized as markers of BBB permeability. The commonly used TJ molecules include claudins, occludin, and TJ-associated proteins, ZO-1 and ZO-2 (Sweeney et al., 2019). For example, cognitive impairment in CCH rats is combined with BBB disruption in view of reduced expression of occludin and ZO-1 (Lee et al., 2017). Similarly, CCH mice exhibited lower endothelial marker CD31 expression in immunostaining compared to sham controls, suggesting more remarkable BBB damage, which partially explained cognitive decline (Lee et al., 2019). Other markers come from different cell types. CCH-induced mice memory deficits, partially owing to the loss of BBB integrity, were inferred from decreased co-localization of GFAP and AQP4 expression on astrocytic endfeet (Wang et al., 2016b).
Blood–Brain Barrier Integrity Detection Based on Functions in vitro
The major function of the BBB is to regulate the permeability of molecules and sustain the homeostasis of the brain. Only in cases when the BBB is damaged could make the exchange of large molecules that are supposed to be blocked possible, which is widely used to evaluate the BBB integrity.
After systemic administration, the presence of exogenous tracers within the brain could be used to reflect the BBB integrity qualitatively or quantitatively by investigating the distribution of markers under microscopy or spectrophotometric assays (Kaya and Ahishali, 2011). These compounds are divided into radioisotope and fluorescence-labeled or autofluorescence markers, such as 14C-sucrose, 14C-mannitol, horseradish peroxidase (HRP), sodium fluorescein, Evans Blue (EB), fluorescein isothiocyanate-dextran, and so forth (Okada et al., 2020; Sun et al., 2021). For instance, more EB extravasation was observed in spontaneously hypertensive rats with severe deficits in spatial memory performance, in contrast to normotensive controls (Choi et al., 2015). Additionally, diffuse leakage of HRP was observed in BCAO rats, judged by the dispersion of the HRP reaction product by microscopy from plasmalemmal vesicles in the endothelial cells to the cytoplasm in endothelial cells and glial cells (Ueno et al., 2002).
Clinical Evidence
Blood–Brain Barrier Integrity Detection Based on Biomarkers in Serum and Cerebral Spinal Fluid
In humans, instead of measuring these protein levels in situ, which is rare since brain biopsies are not easily accessible, clinicians turn to search for fluid biomarkers in serum and cerebral spinal fluid (CSF) that come from the degradation of BBB under pathological conditions, such as occludin, S100, claudin-4, ZO-1, fibronectin, matrix metalloproteinases (MMPs), and UCH-L1 (Okada et al., 2020). For example, the elevated level of MMP2 index (MMPCSF/albuminCSF)/(MMPblood/albuminblood) is closely aligned with the clinical diagnosis of Binswanger disease, one subtype of VCI (Rosenberg et al., 2015). Besides, S100β, primarily synthesized by the astrocytic endfeet process, was found to be upregulated in serum derived from patients with VICD and correlated to cognitive impairment (Wang et al., 2017). Additionally, upregulation of soluble platelet-derived growth factor receptor-β (sPDGFRβ) in CSF, mainly derived from damaged pericyte, indicated BBB leakage in individuals with AD (Miners et al., 2019), which implicated the potential value of sPDGFRβ as a biomarker of BBB breakdown, but it needs to be further evaluated in CCH.
Alternatively, some molecules in circulating blood can abnormally enter CNS through damaged BBB under morbid conditions, indicating the increased permeability of BBB. The most widely used molecules are albumin and IgG. For example, an increased CSF/serum albumin ratio, which was significantly associated with a higher composite vascular risk score, was identified in patients with MCI, suggesting the possible role of BBB dysfunction in inducing cognitive impairment (Lin et al., 2021). Also, patients diagnosed with Binswanger disease showed higher grading scores for IgG extravasation, namely, worse BBB breakdown, than those without neurological diseases, in both the periventricular and subcortical white matter (Akiguchi et al., 1998).
Clinical Imaging
A myriad of novel imaging techniques (Elschot et al., 2021) have been applied in assessing BBB integrity in CSVD, among which the most accepted one is DCE imaging, combined with computed tomography (CT), positron emission tomography (PET)/CT, MRI, or near-infrared spectroscopy (NIRS; Raja et al., 2018; Thrippleton et al., 2019; Sun et al., 2021). For instance, patients with MCI revealed a higher BBB permeability index (i.e., the ratio between late enhancement at the 4th to 5th min to the peak value at 50 s) than those in the control group as measured by DCE-MRI (Wang et al., 2006). Second, dynamic susceptibility contrast (DSC), traditionally considered a perfusion imaging method (Sourbron and Buckley, 2013), showed potential in BBB integrity evaluation by calculating parameters like relative recirculation (Liebner et al., 2018). Specifically, BBB leakage parameters in DSC, representing BBB failure, were significantly correlated with total WML volume even in asymptomatic individuals (Dewey et al., 2021). Besides, arterial spin labeling (ASL) MRI gauges arterially labeled blood spins that are drained into cerebral veins to further determine water extraction fraction (E) and permeability-surface-area product (PS) of BBB (Lin et al., 2018). For example, using water extraction with phase-contrast arterial spin tagging (WEPCAST) MRI, a higher PS value (leakier BBB to water) was implicated in MCI cases compared to normal counterparts (Lin et al., 2021).
Updated Evidence of Blood–Brain Barrier Dysfunction Mechanisms Involved in Chronic Cerebral Hypoperfusion
The BBB is not only comprised of a single layer of endothelial cells that form the interface between the CNS and the periphery entities but also refers to other components such as pericyte, astrocyte, and microglia (Figure 1). Here, we investigate how the BBB is damaged by CCH, focusing on cell interactions and gene hints.
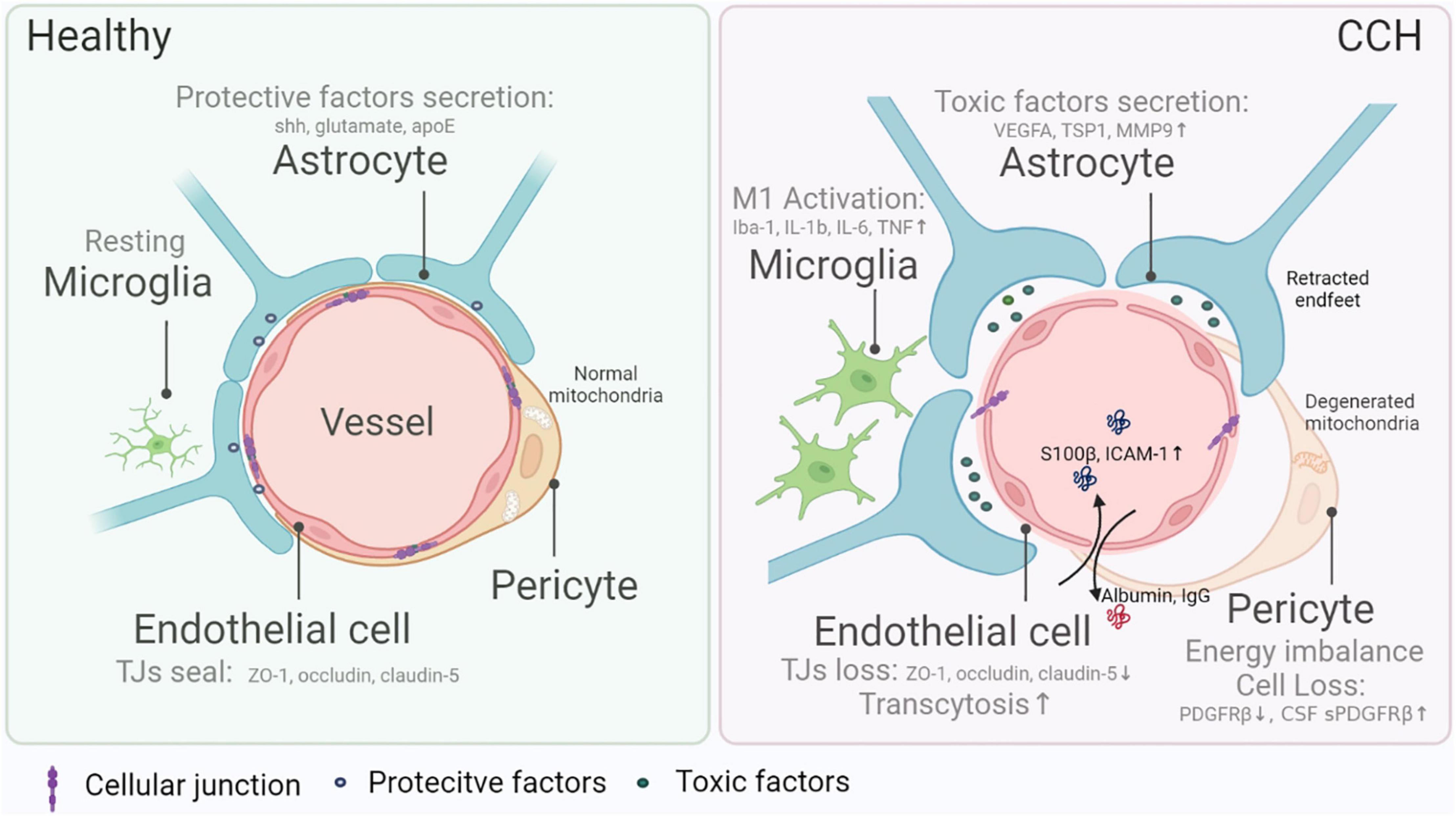
Figure 1. Blood–brain barrier (BBB) damage in chronic cerebral hypoperfusion (CCH). The composition of the BBB mainly includes endothelial cells, pericytes, astrocytes, and microglia, all of which are indispensable in the maintenance of normal BBB integrity and functions. Endothelial cells form a seamless barrier that confines solute exchange between the CNS and the systemic vasculature, attributed to intercellular junction molecules (e.g., ZO-1, occluding, claudin-5), while the loss of barrier in CCH gives rise to BBB leakage and leads to more frequent substance exchange like albumin and IgG entering CNS. Pericytes, wrapping most endothelial cells, protect BBB completeness via nurturing endothelial cells and controlling substance transportation. In CCH, diminishing and malfunction of pericytes cause BBB dysfunction. Reduced pericyte marker PDGFRβ in situ and elevated sPDGFRβ in the cerebral spinal fluid are hallmarks of CCH. The function or metabolism of the pericyte is also vital in regulating BBB damage. For example, energy imbalance resulting from mitochondria degeneration causes the malfunction of pericytes in CCH. Furthermore, astrocytes excrete cytokines that might either be protective (e.g., sonic hedgehog, glutamate, and apoE) or toxic (e.g., VEGFA, TSP1, and MMP9). The environmental changes in CCH trigger off harmful factors release and lead to increased BBB permeability. Also, microglia exert dual effects on BBB physiologically, while in CCH, in general, pro-inflammatory subtypes worsen BBB damage.
Endothelial Cell
The endothelial cells within BBB are endowed with unique characteristics that are differentiated from those in the peripheral vasculature (Langen et al., 2019). First, between the brain microvascular endothelial cells (BMEC), TJs physically fill the gap and form a seamless barrier. Next, the highly selective nature of BBB requires a highly arranged expression of transporters within the cell membrane. Third, the BBB physically prevents and regulates transcytosis of solutes through the endothelial cells in physiological milieus (Liebner et al., 2018). Additionally, low leukocyte adhesion molecule (LAM) expression on the endothelium limits the entrance of immune cells into the brain. In CCH, however, endothelial cells are dysregulated. An increase of serum antibodies against the NR2 subunit of the NMDA receptor (NR2ab), probably due to endothelial damage in the early stage of SVD, was confirmed in patients with subjective cognitive impairment (Dobrynina et al., 2021). In vitro studies also observed endothelial injury and BBB dysfunction in CCH rats with worse performance in the Morris water maze test (Ueno et al., 2009). The TJ-related protein, ZO-1, was found to be downregulated in CCH mice, targeted by TNFα–miR-501-3p axis (Toyama et al., 2018). Besides, downregulation of major facilitator superfamily domain-containing protein 2a (Mfsd2a) might cause cognitive impairment in CCH rats by promoting transcytosis in endothelial cells and exacerbating BBB breakdown (Qu et al., 2020).
Pericyte
Encircling a large proportion of endothelial cells in the brain (Armulik et al., 2011), pericytes contribute to the normal function of BBB in multiple ways: supporting endothelial cells (Cheng et al., 2018; Procter et al., 2021), participating in the exchange of substances between CNS and systemic circulation directly (Balabanov and Dore-Duffy, 1998; Ferland-McCollough et al., 2017), begetting neuroinflammation (Rustenhoven et al., 2017), etc. Thus, it is not surprising to monitor the alteration of pericyte number and function in BBB integrity. Around 35–45% decrease in pericyte number in the frontal white matter was confirmed in patients with vascular dementia (VaD) compared to healthy aging controls (Ding et al., 2020). In the postmortem samples of patients with AD, poorer precuneus perfusion was implicated to be associated with attenuated expression of platelet-derived growth factor receptor-β (PDGFRβ; a pericyte marker), indicating pericyte damage and BBB dysfunction (Miners et al., 2018). CSF sPDGFRβ was also found to be elevated in early cognitively impaired patients, independent of Aβ and tau (Nation et al., 2019). In CCH rats, mitochondria degeneration in pericytes was observed under electron microscopy 7 and 14 days after BCAO operation (Ueno et al., 2002), implying an energy imbalance of pericytes. Together, these findings emphasize the importance of pericytes in BBB dysfunction.
Astrocyte
As the most abundant glial cells in the brain, astrocytes cast a double-faced impact on the completeness of BBB. On the one hand, astrocytes excrete morphogens (sonic hedgehog and retinoic acid), trophic factors (VEGF), gliotransmitters (glutamate), and apolipoprotein E (apoE), among others, to protect BBB. On the other hand, in algetic conditions, the same protective factors could increase BBB permeability (VEGF and glutamate induce junctional damage), or different factors like MMP and nitric oxide, derived from reactive astrocytes, cause damage to BBB (Michinaga and Koyama, 2019; Spampinato et al., 2019). In CCH-induced VaD, lipocalin-2 (LCN2), a neutrophil gelatinase-associated lipocalin, could activate astrocytes to upregulate vascular endothelial growth factor A (VEGFA), which in turn elevated vascular permeability (Kim et al., 2017). Moreover, by manufacturing more exosomal TSP1 (a secretory glycoprotein that mediates cell-cell interactions), which was upregulated in VaD patients’ serum, astrocytes led to BBB disruption (Chen et al., 2020).
Microglia
Microglias have dual roles on the BBB as either protective or detrimental, reflecting the previously accepted dichotomy: M1, the pro-inflammatory subtype and M2, the anti-inflammatory subtype (Kang et al., 2020; Ronaldson and Davis, 2020). In CCH-induced cognitive impairment models, M1 microglia were found abundantly in the brain parenchyma and perivascular sites. For instance, in CCH rats, activated microglia with enlarged soma and thicker processes were observed in the hippocampus CA1 subarea, together with upregulated cytokines like IL-1b, IL-6, and TNF, which involved BBB breakdown (Hei et al., 2018). Moreover, pro-inflammatory microglia (CD68 positive) were detected at the site of BBB leakage (dextran positive) in angiotensin II (Ang-II) induced hypertension mice with impaired short-term memory (Kerkhofs et al., 2020). On the contrary, on being polarized to the M2 phenotype by activating nuclear factor-like 2 (Nrf2), microglia in the cortex could preserve BBB integrity and improve cognition in CCH rats (Mao et al., 2019), indicating the possibility of regulating the phenotype of microglia in protecting BBB.
Genetic Clues: APOE as a Promising Gene Target
In the CCH entities, the role of APOE in BBB breakdown has taken center stage recently. There are three APOE isoforms in humans, APOEε2, APOEε3, and APOEε4 (Serrano-Pozo et al., 2021). APOEε4, the most prevalent risk factor of sporadic AD (Michaelson, 2014), was recently revealed to be the top overlapping gene between AD and vascular pathology (Lin et al., 2019). APOEε4 presence could significantly predict the diagnosis of VaD (Stocker et al., 2020), making it one of the strongest risk genes for VaD (Ikram et al., 2017). Furthermore, reducing cerebrovascular reactivity might be an early pathological change in APOEε4 allele-induced cognitive impairment (Suri et al., 2015). Compared to normal aging, patients bearing APOEε4 demonstrated a more considerable cerebral blood flow drop (Zhang et al., 2017b). Also, APOEε4 mutant individuals had a greater decrease in fractional anisotropy in the genu and the splenium of the corpus callosum detected by DTI (Williams et al., 2019). Similarly, after BCAS operation, CBF decline was more evident in apoE-deficient mice than in wild-type controls, the former of which, not surprisingly, showed more severe BBB dysfunction (Lee et al., 2019). Concerning molecular mechanisms, research elucidated the direct impact of apoE on BBB via triggering the proinflammatory CypA-NFκB-MMP-9 pathway in pericytes (Bell et al., 2012). In a cohort study of 245 participants, APOEε4 carriers exhibited more extensive BBB leakage and worse cognitive impairment, independent of AD pathology, of which an activated CypA-MMP9 pathway in CSF was confirmed (Montagne et al., 2020). Thus, targeting APOEε4 was proved to be a promising therapeutic method in CCH-induced individuals with VICD.
Potent Therapeutic Interventions Targeting Blood–Brain Barrier in Chronic Cerebral Hypoperfusion
Blood–Brain Barrier Function Recovery
Given the complex composition of the BBB, potent therapies that target multiple cell components have been raised (summarized in Table 1). For instance, in pericytes, PDGFRβ was found to be elevated by NXP031 (a vitamin C/DNA aptamer complex that boosts vitamin C’s antioxidant efficacy) in VaD mice, which demonstrated better cognitive performance (Lee et al., 2021). Moreover, it was reported that dl-3-n-butylphthalide treatment suppressed astrocyte activation (given by repressed GFAP, TNF-α, and IL-6 expression) in CCH mice, which partially explained the attenuated spatial memory dysfunction (Han et al., 2019). Environmental enrichment (a multitarget rehabilitation method based on early lifestyle modification) partially improved the memory of VCI rats by reversing TJ downregulation caused by CCH (Xu et al., 2020). However, these treatments were all tested in animal models which require further validation in humans.
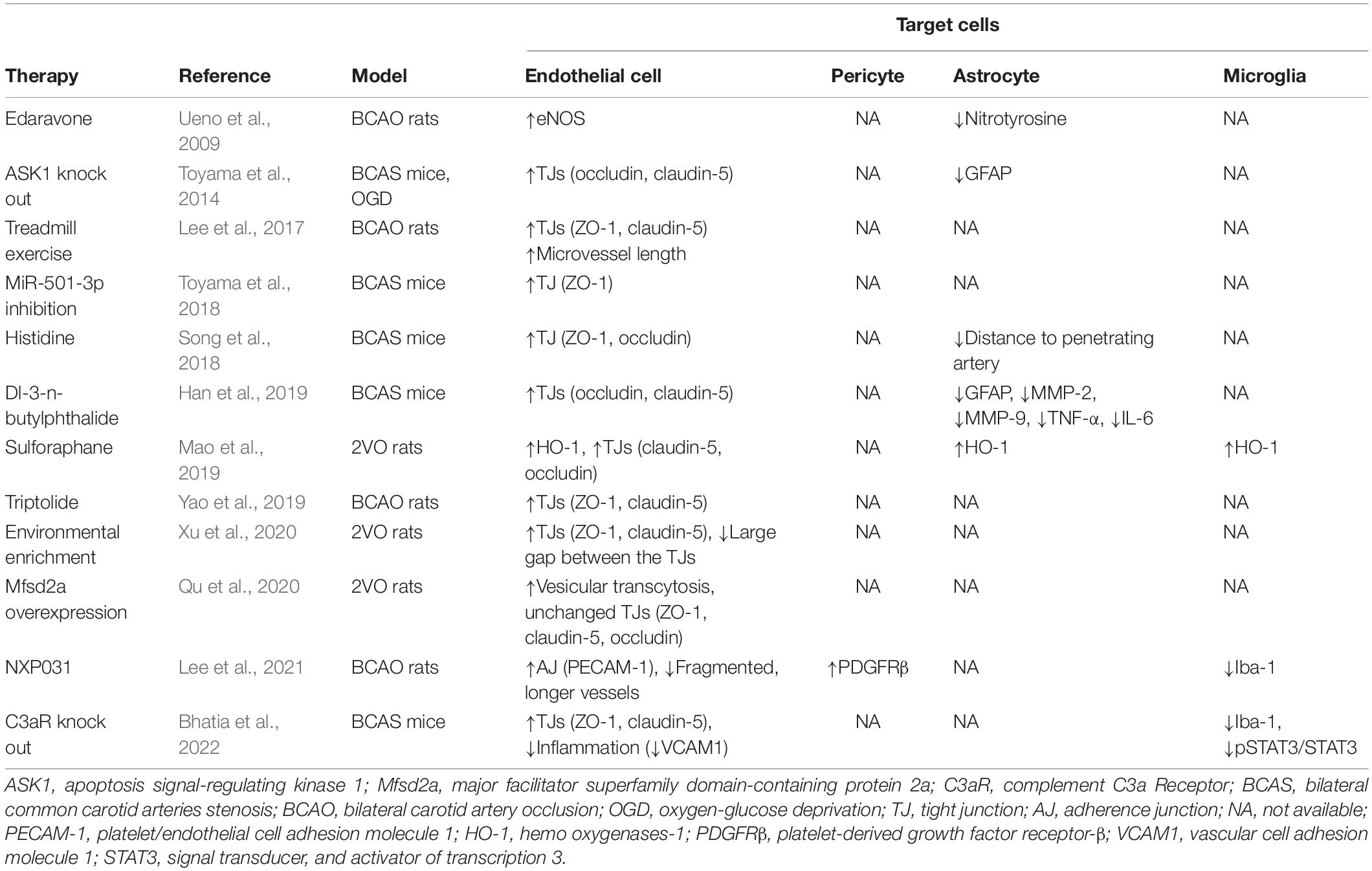
Table 1. Therapeutic interventions and the underlying mechanisms targeting blood–brain barrier in chronic cerebral hypoperfusion.
Cell Therapy
Beyond recovering BBB function, an alternate therapeutic intervention is to maintain BBB integrity by transplanting cells. Particularly, by transplanting endothelial progenitor cells (EPCs) into the hippocampus of APP/PS1 transgenic AD mice, Zhang et al. upregulated TJ expression and repaired BBB, ultimately improving spatial learning and reference memory in the Morris water maze test (Zhang et al., 2018). Similarly, Nakazaki et al. elicited functional recovery in CSVD rats via intravenous infusing mesenchymal stem cells (MSC), in which restoration of endothelial cells and pericytes was found in BBB (Nakazaki et al., 2019). Furthermore, preconditioning the CCH rat model with VEGF, a BBB breakdown inducer allowed bone marrow mononuclear cells (BMMNC) to migrate into the brain parenchyma, resulting in better learning capability and memory performance (Wang et al., 2016a). All in all, albeit immature, cell therapy demonstrates great potential in restoring the physiological function of the BBB and preventing or reducing cognitive decline.
Conclusion
In this review, we stressed the significance of BBB abruption in CCH-induced cognitive deterioration by expounding the findings from the latest research on BBB failure. These results raise awareness and encourage us to pay more attention to the BBB dysfunction in the early stage of CCH and provide us with a new avenue for developing therapies targeting BBB to improve cognitive functions in individuals with CCH.
Author Contributions
MG, MC, WX, and QB searched data for this review, wrote the manuscript, and contributed substantially to discussions of its content. QD helped with the revision, polishing, and editing of the manuscript before submission. All the authors read, revised, and approved the final manuscript.
Funding
This study was supported by the National Natural Science Foundation of China (81971013 to MC; 82071197 to QD; 82101336 to MG), the grant from the Shanghai Sailing Program (21YF1404300 to M.G), The Featured Clinical Discipline Project of Shanghai Pudong (PWYst2018-01), and the Key Discipline Group Construction Project of Shanghai Pudong (PWZxq2017-02).
Conflict of Interest
The authors declare that the research was conducted in the absence of any commercial or financial relationships that could be construed as a potential conflict of interest.
Publisher’s Note
All claims expressed in this article are solely those of the authors and do not necessarily represent those of their affiliated organizations, or those of the publisher, the editors and the reviewers. Any product that may be evaluated in this article, or claim that may be made by its manufacturer, is not guaranteed or endorsed by the publisher.
References
Akiguchi, I., Tomimoto, H., Suenaga, T., Wakita, H., and Budka, H. (1998). Blood-brain barrier dysfunction in Binswanger’s disease; an immunohistochemical study. Acta Neuropathol. 95, 78–84. doi: 10.1007/s004010050768
Armulik, A., Genové, G., and Betsholtz, C. (2011). Pericytes: developmental, physiological, and pathological perspectives, problems, and promises. Dev Cell 21, 193–215. doi: 10.1016/j.devcel.2011.07.001
Balabanov, R., and Dore-Duffy, P. (1998). Role of the CNS microvascular pericyte in the blood-brain barrier. J. Neurosci. Res. 53, 637–644. doi: 10.1002/(sici)1097-4547(19980915)53:6<637::Aid-jnr1<3.0.Co;2-6
Bell, R. D., Winkler, E. A., Singh, I., Sagare, A. P., Deane, R., Wu, Z., et al. (2012). Apolipoprotein E controls cerebrovascular integrity via cyclophilin A. Nature 485, 512–516. doi: 10.1038/nature11087
Bhatia, K., Kindelin, A., Nadeem, M., Khan, M. B., Yin, J., Fuentes, A., et al. (2022). Complement C3a Receptor (C3aR) Mediates Vascular Dysfunction, Hippocampal Pathology, and Cognitive Impairment in a Mouse Model of VCID. Transl. Stroke Res. [Epub ahead of print]. doi: 10.1007/s12975-022-00993-x
Chen, C. Y., Chao, Y. M., Lin, H. F., Chen, C. J., Chen, C. S., Yang, J. L., et al. (2020). miR-195 reduces age-related blood-brain barrier leakage caused by thrombospondin-1-mediated selective autophagy. Aging Cell 19:e13236. doi: 10.1111/acel.13236
Cheng, J., Korte, N., Nortley, R., Sethi, H., Tang, Y., and Attwell, D. (2018). Targeting pericytes for therapeutic approaches to neurological disorders. Acta Neuropathol. 136, 507–523. doi: 10.1007/s00401-018-1893-0
Choi, J., Cui, Y., and Kim, B. (2015). Interaction between hypertension and cerebral hypoperfusion in the development of cognitive dysfunction and white matter pathology in rats. Neuroscience 303, 115–125. doi: 10.1016/j.neuroscience.2015.06.056
Ciacciarelli, A., Sette, G., Giubilei, F., and Orzi, F. (2020). Chronic cerebral hypoperfusion: An undefined, relevant entity. J. Clin. Neurosci. 73, 8–12. doi: 10.1016/j.jocn.2020.01.026
de Wilde, M. C., Farkas, E., Gerrits, M., Kiliaan, A. J., and Luiten, P. G. (2002). The effect of n-3 polyunsaturated fatty acid-rich diets on cognitive and cerebrovascular parameters in chronic cerebral hypoperfusion. Brain Res. 947, 166–173. doi: 10.1016/s0006-8993(02)02695-1
Dewey, B. E., Xu, X., Knutsson, L., Jog, A., Prince, J. L., Barker, P. B., et al. (2021). MTT and Blood-Brain Barrier Disruption within Asymptomatic Vascular WM Lesions. AJNR Am. J. Neuroradiol. 42, 1396–1402. doi: 10.3174/ajnr.A7165
Ding, R., Hase, Y., Ameen-Ali, K. E., Ndung’u, M., Stevenson, W., Barsby, J., et al. (2020). Loss of capillary pericytes and the blood–brain barrier in white matter in poststroke and vascular dementias and Alzheimer’s disease. Brain Pathol. 30, 1087–1101. doi: 10.1111/bpa.12888
Dobrynina, L. A., Alexandrova, E. V., Zabitova, M. R., Kalashnikova, L. A., Krotenkova, M. V., and Akhmetzyanov, B. M. (2021). Anti-NR2 glutamate receptor antibodies as an early biomarker of cerebral small vessel disease. Clin. Biochem. 96, 26–32. doi: 10.1016/j.clinbiochem.2021.07.003
Elschot, E. P., Backes, W. H., Postma, A. A., van Oostenbrugge, R. J., Staals, J., Rouhl, R. P., et al. (2021). A Comprehensive View on MRI Techniques for Imaging Blood-Brain Barrier Integrity. Inves Radiol. 56, 10–19. doi: 10.1097/RLI.0000000000000723
Ferland-McCollough, D., Slater, S., Richard, J., Reni, C., and Mangialardi, G. (2017). Pericytes, an overlooked player in vascular pathobiology. Pharmacol. Therapeutics 171, 30–42. doi: 10.1016/j.pharmthera.2016.11.008
GBD (2019). Global, regional, and national burden of Alzheimer’s disease and other dementias, 1990-2016: a systematic analysis for the Global Burden of Disease Study 2016. Lancet Neurol. 18, 88–106. doi: 10.1016/s1474-4422(18)30403-4
GBD (2022). Estimation of the global prevalence of dementia in 2019 and forecasted prevalence in 2050: an analysis for the Global Burden of Disease Study 2019. Lancet Public Health 7, e105–e125. doi: 10.1016/S2468-2667(21)00249-8
Han, Q. Y., Zhang, H., Zhang, X., He, D. S., Wang, S. W., Cao, X., et al. (2019). dl-3-n-butylphthalide preserves white matter integrity and alleviates cognitive impairment in mice with chronic cerebral hypoperfusion. CNS Neurosci. Ther. 25, 1042–1053. doi: 10.1111/cns.13189
Hei, Y., Chen, R., Yi, X., Long, Q., Gao, D., and Liu, W. (2018). HMGB1 Neutralization Attenuates Hippocampal Neuronal Death and Cognitive Impairment in Rats with Chronic Cerebral Hypoperfusion via Suppressing Inflammatory Responses and Oxidative Stress. Neuroscience 383, 150–159. doi: 10.1016/j.neuroscience.2018.05.010
Ikram, M. A., Bersano, A., Manso-Calderón, R., Jia, J.-P., Schmidt, H., Middleton, L., et al. (2017). Genetics of vascular dementia–review from the ICVD working group. BMC Med. 15:48. doi: 10.1186/s12916-017-0813-9
Iturria-Medina, Y., Sotero, R. C., Toussaint, P. J., Mateos-Pérez, J. M., and Evans, A. C. (2016). Early role of vascular dysregulation on late-onset Alzheimer’s disease based on multifactorial data-driven analysis. Nat. Commun. 7:11934. doi: 10.1038/ncomms11934
Jann, K., Shao, X., Ma, S. J., Cen, S. Y., D’Orazio, L., Barisano, G., et al. (2021). Evaluation of Cerebral Blood Flow Measured by 3D PCASL as Biomarker of Vascular Cognitive Impairment and Dementia (VCID) in a Cohort of Elderly Latinx Subjects at Risk of Small Vessel Disease. Front. Neurosci. 15:35. doi: 10.3389/fnins.2021.627627
Jia, L., Quan, M., Fu, Y., Zhao, T., Li, Y., Wei, C., et al. (2020). Dementia in China: epidemiology, clinical management, and research advances. Lancet Neurol. 19, 81–92. doi: 10.1016/s1474-4422(19)30290-x
Kang, R., Gamdzyk, M., Lenahan, C., Tang, J., Tan, S., and Zhang, J. H. (2020). The Dual Role of Microglia in Blood-Brain Barrier Dysfunction after Stroke. Curr. Neuropharmacol. 18, 1237–1249. doi: 10.2174/1570159x18666200529150907
Kaya, M., and Ahishali, B. (2011). Assessment of permeability in barrier type of endothelium in brain using tracers: Evans blue, sodium fluorescein, and horseradish peroxidase. Methods Mol. Biol. 763, 369–382. doi: 10.1007/978-1-61779-191-8_25
Kerkhofs, D., van Hagen, B. T., Milanova, I. V., Schell, K. J., van Essen, H., Wijnands, E., et al. (2020). Pharmacological depletion of microglia and perivascular macrophages prevents Vascular Cognitive Impairment in Ang II-induced hypertension. Theranostics 10, 9512–9527. doi: 10.7150/thno.44394
Kerkhofs, D., Wong, S. M., Zhang, E., Uiterwijk, R., Hoff, E. I., Jansen, J. F. A., et al. (2021). Blood-brain barrier leakage at baseline and cognitive decline in cerebral small vessel disease: a 2-year follow-up study. Geroscience 43, 1643–1652. doi: 10.1007/s11357-021-00399-x
Kim, J. H., Ko, P. W., Lee, H. W., Jeong, J. Y., Lee, M. G., Kim, J. H., et al. (2017). Astrocyte-derived lipocalin-2 mediates hippocampal damage and cognitive deficits in experimental models of vascular dementia. Glia 65, 1471–1490. doi: 10.1002/glia.23174
Kloppenborg, R. P., Nederkoorn, P. J., Geerlings, M. I., and van den Berg, E. (2014). Presence and progression of white matter hyperintensities and cognition: a meta-analysis. Neurology 82, 2127–2138. doi: 10.1212/wnl.0000000000000505
Langen, U. H., Ayloo, S., and Gu, C. (2019). Development and Cell Biology of the Blood-Brain Barrier. Annu. Rev. Cell Dev. Biol. 35, 591–613. doi: 10.1146/annurev-cellbio-100617-062608
Lee, E.-S., Yoon, J.-H., Choi, J., Andika, F. R., Lee, T., and Jeong, Y. (2019). A mouse model of subcortical vascular dementia reflecting degeneration of cerebral white matter and microcirculation. J. Cereb. Blood Flow Metabol. 39, 44–57. doi: 10.1177/0271678X17736963
Lee, J.-M., Lee, J.-H., Song, M.-K., and Kim, Y.-J. (2021). NXP031 Improves Cognitive Impairment in a Chronic Cerebral Hypoperfusion-Induced Vascular Dementia Rat Model through Nrf2 Signaling. Int. J. Mol. Sci. 22:6285. doi: 10.3390/ijms22126285
Lee, J.-M., Park, J.-M., Song, M. K., Oh, Y. J., Kim, C.-J., and Kim, Y.-J. (2017). The ameliorative effects of exercise on cognitive impairment and white matter injury from blood-brain barrier disruption induced by chronic cerebral hypoperfusion in adolescent rats. Neurosci. Lett. 638, 83–89. doi: 10.1016/j.neulet.2016.12.018
Li, Y., Li, M., Zuo, L., Shi, Q., Qin, W., Yang, L., et al. (2018). Compromised Blood-Brain Barrier Integrity Is Associated With Total Magnetic Resonance Imaging Burden of Cerebral Small Vessel Disease. Front. Neurol. 9:221. doi: 10.3389/fneur.2018.00221
Liebner, S., Dijkhuizen, R. M., Reiss, Y., Plate, K. H., Agalliu, D., and Constantin, G. (2018). Functional morphology of the blood–brain barrier in health and disease. Acta Neuropathol. 135, 311–336. doi: 10.1007/s00401-018-1815-1
Lin, B. Z., Sur, S., Liu, P., Li, Y., Jiang, D., Hou, B. X., et al. (2021). Blood-brain barrier breakdown in relationship to Alzheimer and vascular disease. Ann. Neurol. 90, 227–238. doi: 10.1002/ana.26134
Lin, Y.-F., Smith, A. V., Aspelund, T., Betensky, R. A., Smoller, J. W., Gudnason, V., et al. (2019). Genetic overlap between vascular pathologies and Alzheimer’s dementia and potential causal mechanisms. Alzheimer’s Dementia 15, 65–75. doi: 10.1016/j.jalz.2018.08.002
Lin, Z., Li, Y., Su, P., Mao, D., Wei, Z., Pillai, J. J., et al. (2018). Non-contrast MR imaging of blood-brain barrier permeability to water. Magn. Reson. Med. 80, 1507–1520. doi: 10.1002/mrm.27141
Maillard, P., Fletcher, E., Harvey, D., Carmichael, O., Reed, B., Mungas, D., et al. (2011). White matter hyperintensity penumbra. Stroke 42, 1917–1922. doi: 10.1161/strokeaha.110.609768
Mao, L., Yang, T., Li, X., Lei, X., Sun, Y., Zhao, Y., et al. (2019). Protective effects of sulforaphane in experimental vascular cognitive impairment: Contribution of the Nrf2 pathway. J. Cereb. Blood Flow Metab. 39, 352–366. doi: 10.1177/0271678x18764083
Michaelson, D. M. (2014). APOE ε4: The most prevalent yet understudied risk factor for Alzheimer’s disease. Alzheimer’s Dementia 10, 861–868. doi: 10.1016/j.jalz.2014.06.015
Michinaga, S., and Koyama, Y. (2019). Dual Roles of Astrocyte-Derived Factors in Regulation of Blood-Brain Barrier Function after Brain Damage. Int. J. Mol. Sci. 20:571 doi: 10.3390/ijms20030571
Miners, J. S., Kehoe, P. G., Love, S., Zetterberg, H., and Blennow, K. (2019). CSF evidence of pericyte damage in Alzheimer’s disease is associated with markers of blood-brain barrier dysfunction and disease pathology. Alzheimers Res. Ther. 11:81. doi: 10.1186/s13195-019-0534-8
Miners, J. S., Schulz, I., and Love, S. (2018). Differing associations between Aβ accumulation, hypoperfusion, blood-brain barrier dysfunction and loss of PDGFRB pericyte marker in the precuneus and parietal white matter in Alzheimer’s disease. J. Cereb. Blood Flow Metab. 38, 103–115. doi: 10.1177/0271678x17690761
Montagne, A., Huuskonen, M. T., Rajagopal, G., Sweeney, M. D., Nation, D. A., Sepehrband, F., et al. (2019). Undetectable gadolinium brain retention in individuals with an age-dependent blood-brain barrier breakdown in the hippocampus and mild cognitive impairment. Alzheimers Dement 15, 1568–1575. doi: 10.1016/j.jalz.2019.07.012
Montagne, A., Nation, D. A., Sagare, A. P., Barisano, G., Sweeney, M. D., Chakhoyan, A., et al. (2020). APOE4 leads to blood–brain barrier dysfunction predicting cognitive decline. Nature 581, 71–76. doi: 10.1038/s41586-020-2247-3
Nakazaki, M., Sasaki, M., Kataoka-Sasaki, Y., Oka, S., Suzuki, J., Sasaki, Y., et al. (2019). Intravenous infusion of mesenchymal stem cells improves impaired cognitive function in a cerebral small vessel disease model. Neuroscience 408, 361–377. doi: 10.1016/j.neuroscience.2019.04.018
Nation, D. A., Sweeney, M. D., Montagne, A., Sagare, A. P., D’Orazio, L. M., Pachicano, M., et al. (2019). Blood-brain barrier breakdown is an early biomarker of human cognitive dysfunction. Nat. Med. 25, 270–276. doi: 10.1038/s41591-018-0297-y
Okada, T., Suzuki, H., Travis, Z. D., and Zhang, J. H. (2020). The stroke-induced blood-brain barrier disruption: current progress of inspection technique, mechanism, and therapeutic target. Curr. Neuropharmacol. 18, 1187–1212. doi: 10.2174/1570159X18666200528143301
Pan, Y., and Nicolazzo, J. A. (2018). Impact of aging, Alzheimer’s disease and Parkinson’s disease on the blood-brain barrier transport of therapeutics. Adv. Drug Deliv. Rev. 135, 62–74. doi: 10.1016/j.addr.2018.04.009
Pandit, R., Chen, L., and Götz, J. (2020). The blood-brain barrier: Physiology and strategies for drug delivery. Adv. Drug Deliv. Rev. 16, 1–14. doi: 10.1016/j.addr.2019.11.009
Peters, R., Anstey, K. J., Booth, A., Beckett, N., Warwick, J., Antikainen, R., et al. (2018). Orthostatic hypotension and symptomatic subclinical orthostatic hypotension increase risk of cognitive impairment: an integrated evidence review and analysis of a large older adult hypertensive cohort. Eur. Heart J. 39, 3135–3143. doi: 10.1093/eurheartj/ehy418
Prabhakaran, S. (2019). Blood Pressure, Brain Volume and White Matter Hyperintensities, and Dementia Risk. JAMA 322, 512–513. doi: 10.1001/jama.2019.10849
Prins, N. D., and Scheltens, P. (2015). White matter hyperintensities, cognitive impairment and dementia: an update. Nat. Rev. Neurol. 11, 157–165. doi: 10.1038/nrneurol.2015.10
Procter, T. V., Williams, A., and Montagne, A. (2021). Interplay between Brain Pericytes and Endothelial Cells in Dementia. Am. J. Pathol. 191, 1917–1931. doi: 10.1016/j.ajpath.2021.07.003
Promjunyakul, N., Lahna, D., Kaye, J. A., Dodge, H. H., Erten-Lyons, D., Rooney, W. D., et al. (2015). Characterizing the white matter hyperintensity penumbra with cerebral blood flow measures. Neuroimage Clin. 8, 224–229. doi: 10.1016/j.nicl.2015.04.012
Qu, C., Song, H., Shen, J., Xu, L., Li, Y., Qu, C., et al. (2020). Mfsd2a Reverses Spatial Learning and Memory Impairment Caused by Chronic Cerebral Hypoperfusion via Protection of the Blood–Brain Barrier. Front. Neurosci. 14:461. doi: 10.3389/fnins.2020.00461
Raja, R., Rosenberg, G. A., and Caprihan, A. (2018). MRI measurements of blood-brain barrier function in dementia: a review of recent studies. Neuropharmacology 134, 259–271. doi: 10.1016/j.neuropharm.2017.10.034
Ronaldson, P. T., and Davis, T. P. (2020). Regulation of blood-brain barrier integrity by microglia in health and disease: A therapeutic opportunity. J. Cereb. Blood Flow Metab. 40, S6–S24. doi: 10.1177/0271678x20951995
Rosenberg, G. A., Prestopnik, J., Adair, J. C., Huisa, B. N., Knoefel, J., Caprihan, A., et al. (2015). Validation of biomarkers in subcortical ischaemic vascular disease of the Binswanger type: approach to targeted treatment trials. J. Neurol. Neurosurg. Psychiatr. 86, 1324–1330. doi: 10.1136/jnnp-2014-309421
Rundek, T., Tolea, M., Ariko, T., Fagerli, E. A., and Camargo, C. J. (2021). Vascular Cognitive Impairment (VCI). Neurotherapeutics 19, 68–88. doi: 10.1007/s13311-021-01170-y
Rustenhoven, J., Jansson, D., Smyth, L. C., and Dragunow, M. (2017). Brain Pericytes As Mediators of Neuroinflammation. Trends Pharmacol. Sci. 38, 291–304. doi: 10.1016/j.tips.2016.12.001
Schmidt, R., Schmidt, H., Haybaeck, J., Loitfelder, M., Weis, S., Cavalieri, M., et al. (2011). Heterogeneity in age-related white matter changes. Acta Neuropathol. 122, 171–185. doi: 10.1007/s00401-011-0851-x
Segarra, M., Aburto, M. R., and Acker-Palmer, A. (2021). Blood-Brain Barrier Dynamics to Maintain Brain Homeostasis. Trends Neurosci. 44, 393–405. doi: 10.1016/j.tins.2020.12.002
Serrano-Pozo, A., Das, S., and Hyman, B. T. (2021). APOE and Alzheimer’s disease: advances in genetics, pathophysiology, and therapeutic approaches. Lancet Neurol. 20, 68–80. doi: 10.1016/s1474-4422(20)30412-9
Silbert, L. C., Dodge, H. H., Perkins, L. G., Sherbakov, L., Lahna, D., Erten-Lyons, D., et al. (2012). Trajectory of white matter hyperintensity burden preceding mild cognitive impairment. Neurology 79, 741–747. doi: 10.1212/WNL.0b013e3182661f2b
Singh-Manoux, A., Fayosse, A., Sabia, S., Canonico, M., Bobak, M., Elbaz, A., et al. (2017). Atrial fibrillation as a risk factor for cognitive decline and dementia. Eur. Heart J. 38, 2612–2618. doi: 10.1093/eurheartj/ehx208
Song, J., Yang, L., Nan, D., He, Q., Wan, Y., and Guo, H. (2018). Histidine Alleviates Impairments Induced by Chronic Cerebral Hypoperfusion in Mice. Front. Physiol. 9:662. doi: 10.3389/fphys.2018.00662
Sourbron, S. P., and Buckley, D. L. (2013). Classic models for dynamic contrast-enhanced MRI. NMR Biomed. 26, 1004–1027. doi: 10.1002/nbm.2940
Spampinato, S. F., Bortolotto, V., Canonico, P. L., Sortino, M. A., and Grilli, M. (2019). Astrocyte-Derived Paracrine Signals: Relevance for Neurogenic Niche Regulation and Blood-Brain Barrier Integrity. Front. Pharmacol. 10:1346. doi: 10.3389/fphar.2019.01346
Stewart, C. R., Stringer, M. S., Shi, Y., Thrippleton, M. J., and Wardlaw, J. M. (2021). Associations between white matter hyperintensity burden, cerebral blood flow and transit time in small vessel disease: an updated meta-analysis. Front. Neurol. 12:621. doi: 10.3389/fneur.2021.647848
Stocker, H., Perna, L., Weigl, K., Möllers, T., Schöttker, B., Thomsen, H., et al. (2020). Prediction of clinical diagnosis of Alzheimer’s disease, vascular, mixed, and all-cause dementia by a polygenic risk score and APOE status in a community-based cohort prospectively followed over 17 years. Mol. Psychiatr. 26, 5812–5822. doi: 10.1038/s41380-020-0764-y
Sun, H., Hu, H., Liu, C., Sun, N., and Duan, C. (2021). Methods used for the measurement of blood-brain barrier integrity. Metabolic Brain Dis. 36, 723–735. doi: 10.1007/s11011-021-00694-8
Suri, S., Mackay, C. E., Kelly, M. E., Germuska, M., Tunbridge, E. M., Frisoni, G. B., et al. (2015). Reduced cerebrovascular reactivity in young adults carrying the APOE ε4 allele. Alzheimer’s Dementia 11, 648.–657. doi: 10.1016/j.jalz.2014.05.1755
Sweeney, M. D., Zhao, Z., Montagne, A., Nelson, A. R., and Zlokovic, B. V. (2019). Blood-brain barrier: from physiology to disease and back. Physiol. Rev. 99, 21–78. doi: 10.1152/physrev.00050.2017
Thrippleton, M. J., Backes, W. H., Sourbron, S., Ingrisch, M., van Osch, M. J. P., Dichgans, M., et al. (2019). Quantifying blood-brain barrier leakage in small vessel disease: Review and consensus recommendations. Alzheimers Dement 15, 840–858. doi: 10.1016/j.jalz.2019.01.013
Tietz, S., and Engelhardt, B. (2015). Brain barriers: crosstalk between complex tight junctions and adherens junctions. J. Cell Biol. 209, 493–506. doi: 10.1083/jcb.201412147
Toyama, K., Koibuchi, N., Uekawa, K., Hasegawa, Y., Kataoka, K., Katayama, T., et al. (2014). Apoptosis signal-regulating kinase 1 is a novel target molecule for cognitive impairment induced by chronic cerebral hypoperfusion. Arterioscler Thromb. Vasc. Biol. 34, 616–625. doi: 10.1161/atvbaha.113.302440
Toyama, K., Spin, J. M., Deng, A. C., Huang, T.-T., Wei, K., Wagenhäuser, M. U., et al. (2018). MicroRNA-Mediated Therapy Modulating Blood–Brain Barrier Disruption Improves Vascular Cognitive Impairment. Arterioscler. Thromb.Vasc. Biol 38, 1392–1406. doi: 10.1161/ATVBAHA.118.310822
Ueno, M., Chiba, Y., Murakami, R., Matsumoto, K., Kawauchi, M., and Fujihara, R. (2016). Blood-brain barrier and blood-cerebrospinal fluid barrier in normal and pathological conditions. Brain Tumor Pathol. 33, 89–96. doi: 10.1007/s10014-016-0255-7
Ueno, M., Tomimoto, H., Akiguchi, I., Wakita, H., and Sakamoto, H. (2002). Blood-brain barrier disruption in white matter lesions in a rat model of chronic cerebral hypoperfusion. J. Cereb. Blood Flow Metab. 22, 97–104. doi: 10.1097/00004647-200201000-00012
Ueno, Y., Zhang, N., Miyamoto, N., Tanaka, R., Hattori, N., and Urabe, T. (2009). Edaravone attenuates white matter lesions through endothelial protection in a rat chronic hypoperfusion model. Neuroscience 162, 317–327. doi: 10.1016/j.neuroscience.2009.04.065
van der Flier, W. M., Skoog, I., Schneider, J. A., Pantoni, L., Mok, V., Chen, C. L. H., et al. (2018). Vascular cognitive impairment. Nat. Rev. Dis. Primers 4:18003. doi: 10.1038/nrdp.2018.3
Wang, F., Zou, Z. R., Yuan, D., Gong, Y., Zhang, L., Chen, X., et al. (2017). Correlation between serum S100β protein levels and cognitive dysfunction in patients with cerebral small vessel disease: a case-control study. Biosci. Rep. 37:BSR20160446. doi: 10.1042/bsr20160446
Wang, H., Golob, E. J., and Su, M. Y. (2006). Vascular volume and blood-brain barrier permeability measured by dynamic contrast enhanced MRI in hippocampus and cerebellum of patients with MCI and normal controls. J. Magn. Reson. Imaging 24, 695–700. doi: 10.1002/jmri.20669
Wang, J., Fu, X., Yu, L., Li, N., Wang, M., Liu, X., et al. (2016a). Preconditioning with VEGF enhances angiogenic and neuroprotective effects of bone marrow mononuclear cell transplantation in a rat model of chronic cerebral hypoperfusion. Mol. Neurobiol. 53, 6057–6068. doi: 10.1007/s12035-015-9512-8
Wang, L., Du, Y., Wang, K., Xu, G., Luo, S., and He, G. (2016b). Chronic cerebral hypoperfusion induces memory deficits and facilitates Aβ generation in C57BL/6J mice. Exper. Neurol. 283, 353–364. doi: 10.1016/j.expneurol.2016.07.006
Wardlaw, J. M., Doubal, F. N., Valdes-Hernandez, M., Wang, X., Chappell, F. M., Shuler, K., et al. (2013). Blood–brain barrier permeability and long-term clinical and imaging outcomes in cerebral small vessel disease. Stroke 44, 525–527. doi: 10.1161/STROKEAHA.112.669994
Washida, K., Hattori, Y., and Ihara, M. (2019). Animal Models of Chronic Cerebral Hypoperfusion: From Mouse to Primate. Int. J. Mol. Sci. 20:6176. doi: 10.3390/ijms20246176
Williams, O. A., An, Y., Beason-Held, L., Huo, Y., Ferrucci, L., Landman, B. A., et al. (2019). Vascular burden and APOE ε4 are associated with white matter microstructural decline in cognitively normal older adults. Neuroimage 188, 572–583. doi: 10.1016/j.neuroimage.2018.12.009
Wong, A., Ye, M., Levy, A., Rothstein, J., Bergles, D., and Searson, P. C. (2013). The blood-brain barrier: an engineering perspective. Front. Neuroeng. 6:7. doi: 10.3389/fneng.2013.00007
Wong, S. M., Jansen, J. F., Zhang, C. E., Hoff, E. I., Staals, J., van Oostenbrugge, R. J., et al. (2019). Blood-brain barrier impairment and hypoperfusion are linked in cerebral small vessel disease. Neurology 92, e1669–e1677. doi: 10.1212/wnl.0000000000007263
Xu, L., Qu, C., Qu, C., Shen, J., Song, H., Li, Y., et al. (2020). Improvement of autophagy dysfunction as a potential mechanism for environmental enrichment to protect blood-brain barrier in rats with vascular cognitive impairment. Neurosci. Lett. 739:135437. doi: 10.1016/j.neulet.2020.135437
Yao, P., Li, Y., Yang, Y., Yu, S., and Chen, Y. (2019). Triptolide Improves Cognitive Dysfunction in Rats with Vascular Dementia by Activating the SIRT1/PGC-1α Signaling Pathway. Neurochem. Res. 44, 1977–1985. doi: 10.1007/s11064-019-02831-3
Zhang, C. E., Wong, S. M., van de Haar, H. J., Staals, J., Jansen, J. F., Jeukens, C. R., et al. (2017a). Blood-brain barrier leakage is more widespread in patients with cerebral small vessel disease. Neurology 88, 426–432. doi: 10.1212/wnl.0000000000003556
Zhang, N., Gordon, M. L., and Goldberg, T. E. (2017b). Cerebral blood flow measured by arterial spin labeling MRI at resting state in normal aging and Alzheimer’s disease. Neurosci. Biobehav. Rev. 72, 168–175. doi: 10.1016/j.neubiorev.2016.11.023
Zhang, S., Zhi, Y., Li, F., Huang, S., Gao, H., Han, Z., et al. (2018). Transplantation of in vitro cultured endothelial progenitor cells repairs the blood-brain barrier and improves cognitive function of APP/PS1 transgenic AD mice. J.Neurol. Sci. 387, 6–15. doi: 10.1016/j.jns.2018.01.019
Zlokovic, B. V., Gottesman, R. F., Bernstein, K. E., Seshadri, S., McKee, A., Snyder, H., et al. (2020). Vascular contributions to cognitive impairment and dementia (VCID): A report from the 2018 National Heart, Lung, and Blood Institute and National Institute of Neurological Disorders and Stroke Workshop. Alzheimers Dement 16, 1714–1733. doi: 10.1002/alz.12157
Keywords: chronic cerebral hypoperfusion, blood–brain barrier, vascular cognitive impairment and dementia, white matter hyperintensity, normal appearing white matter
Citation: Xu W, Bai Q, Dong Q, Guo M and Cui M (2022) Blood–Brain Barrier Dysfunction and the Potential Mechanisms in Chronic Cerebral Hypoperfusion Induced Cognitive Impairment. Front. Cell. Neurosci. 16:870674. doi: 10.3389/fncel.2022.870674
Received: 07 February 2022; Accepted: 16 May 2022;
Published: 16 June 2022.
Edited by:
Huanxing Su, University of Macau, Macao SAR, ChinaReviewed by:
Scott Miners, University of Bristol, United KingdomCopyright © 2022 Xu, Bai, Dong, Guo and Cui. This is an open-access article distributed under the terms of the Creative Commons Attribution License (CC BY). The use, distribution or reproduction in other forums is permitted, provided the original author(s) and the copyright owner(s) are credited and that the original publication in this journal is cited, in accordance with accepted academic practice. No use, distribution or reproduction is permitted which does not comply with these terms.
*Correspondence: Mei Cui, cuimei@fudan.edu.cn; Min Guo, guo_min@fudan.edu.cn
†These authors have contributed equally to this work and share first authorship