- 1Department of Otolaryngology-Head and Neck Surgery, Shandong Provincial ENT Hospital, Shandong University, Jinan, Shandong, China
- 2Shandong Provincial Vertigo and Dizziness Medical Center, Jinan, Shandong, China
- 3Laboratory of Vertigo Disease, Shandong Second Provincial General Hospital, Shandong Institute of Otorhinolaryngology, Jinan, Shandong, China
- 4Center of Clinical Laboratory, Shandong Second Provincial General Hospital, Jinan, Shandong, China
Introduction: Ménière’s disease (MD), a common disease in the inner ear, is characterized by an increase in endolymph in the cochlear duct and vestibular labyrinth. The pathophysiology of the condition appears to be the immune response. Studies have shown that basal levels of the IL-1β increased in some MD patients.
Methods: Here, we used a murine model of endolymphatic hydrops (EH) to study the effect of anakinra on auditory and vestibular function. Mice were intraperitoneal injected with anakinra or saline before LPS by postauricular injection. Weight and disease severity were measured, histologic changes in auditory were assessed, and inflammation state was evaluated.
Results: We found that anakinra therapy reduced LPS-induced EH, alleviated LPS-induced hearing loss and vestibular dysfunction, and inhibited the expression of the inflammatory cytokines and macrophage infiltration in the cochlea of mice. We further demonstrated that anakinra ameliorated the disorganization and degeneration of myelin sheath, and reduced the neuron damage in cochlea of EH mice.
Discussion: Consequently, anakinra contributes to a promising therapeutic approach to MD, by restricting EH, alleviating auditory and vestibular function, inhibiting inflammation of the inner ear and protecting the cochlear nerve. Further investigations are needed to assess the potential therapeutic benefits of anakinra in patients with MD.
1 Introduction
Ménière’s disease (MD), a complex peripheral vestibular illness, is characterized by episodic vertigo, fluctuating low-to-medium frequencies of sensorineural hearing loss, tinnitus, and/or aural fullness (Sajjadi and Paparella, 2008). Population-based studies report that MD affects about 3.5–513 people per 100,000 annually (Alexander and Harris, 2010). Endolymphatic hydrops (EH) underlies its classic pathological characteristics (Hallpike and Cairns, 1938; Okuno and Sando, 1987). Numerous underlying factors have been postulated to be involved in MD, including anatomical variations in the temporal bone, genetic heterogeneity, allergies, immune dysfunction, and cellular and molecular mechanisms (Derebery and Berliner, 2010; Gazquez et al., 2011; Greco et al., 2012; Requena et al., 2014; Nakashima et al., 2016; Na Zhang et al., 2022). Because the mechanisms underlying MD remain poorly understood, the clinical treatment strategy of MD is mainly to relieve the symptoms. Patients after treatment still suffer from recurrent vertigo and progressive hearing loss, and need hospital treatment frequently, which seriously affects the psychological status, quality of life and economic burden of patients (Tyrrell et al., 2016). Therefore, it is of great practical significance to study the pathogenesis and open up a more accurate treatment direction of MD.
Tissue-resident macrophages play critical roles in inflammation regulation for specific organ by releasing interferons, inflammatory cytokines, and chemokines. Studies have showed that there is a large population of macrophages in the inner ear, can be recruited from blood-borne monocytes to hair cells following damage induced by noise, ototoxic drugs, and aging (Liu et al., 2018), which may be important to initiate local inflammatory response in the cochlear and vestibular system (Zhang et al., 2013; O’Malley et al., 2016; Nakanishi et al., 2017).
Recent studies have shown that immunology is an important pathogenic factor of MD, although the molecular mechanisms remain poorly understood. It is estimated that a third of patients with MD may have immune dysfunction (Greco et al., 2012). Our previous study suggested that MD is in a state of inflammation manifested in elevated levels of Th2-relative cytokines such as interleukin (IL)-4, IL-5, IL-10, and IL-13 in serum, vestibular end organ (VEO) and endolymphatic sac (ES) (Zhang et al., 2022). IL-1β, exerts strong proinflammation, overproduction by macrophages causes autoinflammatory diseases (Gabay et al., 2010; Canna et al., 2014; Jesus and Goldbach-Mansky, 2014). A study reported that compared to healthy controls, the levels of pro-inflammatory cytokines such as IL-1β, IL1-receptor antagonist (IL-1RA), tumor necrosis factor alpha (TNFα), and IL-6 released by peripheral blood mononuclear cells are elevated in 24 of 113 patients (20%) with MD (Frejo et al., 2018). Additionally, polymorphisms in IL-1 are associated with MD (Furuta et al., 2011; Li et al., 2017; Kouhi et al., 2021). These studies suggest that IL-1β may play an important role in MD pathogenesis and IL-1β-targeted therapy may be a potential treatment for MD.
IL-1RA blocks the biological cascades and inhibits the biological effects of IL-1, acting as a natural decoy for IL-1β (Dinarello, 2018). Anakinra, a recombinant form of IL-1RA, exerts anti-inflammatory and immunomodulatory roles. In 2001, it was approved by the US Food and Drug Administration for the treatment of rheumatoid arthritis (Garces, 2001), and has shown significant therapeutic effects associated with an unparalleled record of safety on a broad spectrum autoimmune and inflammation diseases such as steroid-refractory immune effector cell-associated neurotoxicity syndrome (Wehrli et al., 2022), Coronavirus disease 2019 (Kooistra et al., 2020), and gout flares (Saag et al., 2021). Moreover, anakinra has been demonstrated to reverse or improve hearing loss and inflammatory of an atypical cryopyrin-associated periodic syndromes (Nakanishi et al., 2017). However, previous studies have neither revealed its role in MD development nor its regulatory mechanisms. Although MD is considered closely related to inflammation, whether anakinra have therapeutic effect on MD remains unexplored.
Animal models are an important tool for exploring the pathogenesis and treatment of diseases. At present, models of chronic EH have been established by surgical obstruction of the ES or systemic drugs such as aldosterone, vasopressin, or lipopolysaccharide (LPS) for cochlear and vestibular research (Seo and Brown, 2020). For example, LPS induces moderate to severe hearing loss and EH (Takumida et al., 2008; Brown et al., 2018), and contributes to a better understanding of the MD pathogenesis.
In this study, we investigated whether blockade of IL-1β signaling by anakinra could inhibit EH and audiovestibular symptoms in LPS-induced EH murine model. We further focused on the alterations of inflammatory response and cochlear nerve. Taken together, this points to a potential and promising therapeutic strategy for MD.
2 Materials and methods
2.1 Mice and LPS injection
Wild type C57BL/6 mice (purchased from the Animal Center of Shandong university) were housed in a temperature-controlled (20–22°C) room that allows for a 12/12 h light/dark cycle. Sex-matched mice had access to food and drinking water, weighed 17–25 g, were 8–10 weeks old. All study protocols were approved by the Animal Care Committee of Shandong university and conformed to the National institutes of Health Guide for the Care and Use of Laboratory Animals.
C57BL/6 mice were subjected to LPS-induced EH. Briefly, mice were challenged with LPS (2 mg/kg, Sigma) solvent in saline by bilateral postauricular injection (p.a.) (Li et al., 2013) once a day for 3 days, while control groups were p.a. injected with equivalent 0.9% normal saline (NS).
2.2 Anakinra injection
To evaluate the roles for anakinra in LPS-induced EH, mice were intraperitoneal injected (i.p.) with anakinra (10 mg/kg, Med Chem Express, Monmouth Junction, NJ, USA) or equivalent NS 30 min before LPS challenge. In all cases, hearing and vestibular function were tested on day 5 after first dose of LPS injection. After then, those mice were anesthetized by intraperitoneal injection with a mixture of xylazine (10 mg/kg) and ketamine (100 mg/kg) and inner ears were subjected to protein or RNA expression and EH analysis.
2.3 Frozen sections
The mouse cochleae were collected, fixed with 4% paraformaldehyde overnight at 4°C, continuously dehydrated in a sucrose phosphate-buffered saline mixture with sucrose concentrations increasing at 15, 20, and 30%, and ultimately encapsulated in an OCT compound (Tissue−Tek, Sakura Finetek, Torrance, USA). The embedded cochlea was sliced (5 μm thick) using a cryostat (Leica CM 1850, Nussloch, Germany) and the slice was stored at −80°C.
2.4 Histological and histomorphometric analyses
For hematoxylin-eosin (HE) staining, the cochlear sections were stained with hematoxylin (H8070, Solarbio life sciences, Beijing, China) for 3 min followed 2 min of staining with eosin (C0109, Beyotime, Shanghai, China) at room temperature.
For the immunofluorescence, the cochlear sections were permeabilized in 0.5% Triton X-100 for 30 min, and blocked with 10% donkey serum for 60 min at room temperature. The sections were incubated with the appropriate primary antibodies at 4°C overnight: anti-CD68 antibody (1:200; 97778, CST, Boston, USA), anti-Tuj1 (1:800; 5568, CST, Boston, USA), anti- Myelin Basic Protein (MBP, 1:200; 78896, CST, Boston, USA), and anti-Neurofilament 200 antibody (NF-200, 1:20; 2836, CST, Boston, USA). At last, the sections were incubated with the relevant fluorescently labeled secondary antibodies (1:1,000; Invitrogen, Carlsbad, CA, USA) and DAPI (1:1,000; D9542; Sigma-Aldrich, St. Louis, MO, USA) for 60 min in dark at room temperature. The sections were photographed under a laser scanning confocal microscope (Leica SP8; Leica, Wetzlar, Germany).
2.5 Rotarod test
The mice were placed on an electric rotating rod (ZH–600 B, Huaibei Zhenghua Biological Instruments Co., Ltd.). Gradually increased the speed to 30 rpm for 2 min. Each mouse was trained 2 times per day for 3 days and tested twice. Whereafter, the average time to fall off the rotarod in 2 trials was calculated for analysis.
2.6 Swim test
General vestibular function could be scored using swimming tests (Clopath et al., 2014). Mice were placed in a standard pool with a water level height of approximately 15 cm and a water temperature of around 25°C. Mice were scored for swimming posture, with mice swimming in the water (score 0), swimming irregularly (score 1), floating stationarily (score 2), or rolling underwater (score 3).
2.7 Vestibular evoked myogenic potentials (VEMPs)
Click-evoked VEMPs was recorded at the same time as electromyography potential after anesthesia. A custom-made holder previously reported (Sheykholeslami et al., 2009) was used to fix the mice with a suspension wire behind the anterior teeth, so that the mice hyperextended their necks and raised their heads with free legs, holding them in a prone position. For the test, a needle electrode was inserted into the cervical extensor muscle and a reference electrode was placed in the midline of the cervico-occipital region, with the ground electrode placed on the back. Each animal was tested for VEMPs with a stimulation intensity of 100 dB nHL. The response threshold is the minimum threshold at which the waveform occurred. Its repeatability was verified by running it continuously (>3 times). Eventually, the latencies and amplitudes of the negative and positive peaks were recorded.
2.8 Vestibular ocular reflexes (VOR)
VOR test was conducted as reported previously (Yang et al., 2019). Briefly, mice were placed with a non-invasive animal-immobility setup. Horizontal eye position signals were recorded using a binocular VOG-based VFT system provided by Prof. Fangyi Chen’s team (Southern University of Science and Technology). An infrared camera equipped with a zoom lens (MI®, China) was placed on translation stages at 45° angles to the anteroposterior axis of the mouse. Illumination of the video recording was achieved by two near-infrared light-emitting diode lamps (940 nm, Chuntaxin®, China) that were connected to the camera. The eye tracker tracked the region of interest (ROI) at a speed of 60 frames/s. ROI, which contains the pupil, in each frame was automatically selected following a template-matching method. Then, an ellipse fit was used to determine the pupil center, and to extract the horizontal component derived from the eye movements. The obtained translation distances were converted to eye rotation angel for calibration. To measure the VOR responses, horizontal rotations were delivered at 20°/s (0.2, 0.5, 0.8, 1.0, 1.6, and 3.2 Hz). Exported eye-position data underwent Fourier transformation using the MATLAB 2016b software to yield amplitude data for eye movement. The VOR gain were calculated as the amplitude ratio between response and stimulus.
2.9 Auditory function evaluation
The auditory brainstem response (ABR) were measured as previously described (Zhang et al., 2020). The sound level started at a 90-dB sound pressure level (SPL) and then decreased by 5-dB to the acoustic threshold. The ABR threshold was determined at each frequency, which refers to the minimal SPL resulting in a reliable ABR recording with one or more distinguishable waves clearly identified by visual inspection. Repeated the process for low SPLs near the threshold to ensure the waveform consistency. Mice were housed in a sound isolation chamber and stimulated at sound frequencies of 4, 8, 12, 16, 24, and 32 kHz, each frequency to be repeated 1,024 times, and ABR responses were measured using the TDT System 3 (Tucker-Davis Technologies, Alachua, FL, USA). Briefly, a total of 15 mice (n = 5 per group) were anesthetized by intraperitoneal injection with a mixture of xylazine (10 mg/kg) and ketamine (100 mg/kg). The speaker was positioned 10 cm away from each mouse. Needle electrodes are inserted into the subcutaneous tissue of the mouse. The recording electrode is inserted into the cranial vault, the reference electrode is inserted in the subauricular mastoid region of the ipsilateral ear, and the grounding electrode is inserted on the back. Starting from a SPL of 90 dB and decreasing by 5 dB to the hearing threshold. The ABR threshold determined at each frequency was the minimum SPL that produced a reliable ABR recording where the eye could clearly identify the waveform. This process was repeated for lower SPLs close to the threshold to ensure consistency of the waveform.
2.10 Extraction of mRNA and quantitative real-time PCR (qRT-PCR)
Total RNA of the mouse cochlea was obtained using the RNA extraction kit (RNeasy Mini QIAcube Kit, QIAGEN, Hilden, Germany). The relative expression levels of RNA were calculated by reverse transcription and assayed using qRT-PCR (Eppendorf AG 22331 PCR, Hamburg, Germany). The qRT-PCR reaction mixture comprised 2 × SYBR Green Premix EX Taq (RR42LR, Takara Biotechnology, Shiga, Japan), cDNA template, forward and reverse primer, and deionized water. The qRT-PCR parameters involved an initial denaturation step at 95°C for 3 min, then denaturing at 95°C for 50 s, 60°C with annealing for 45 s, and a further extension at 72°C for 50 s across 40 cycles. The specificity of each PCR reaction was identified by the melting curve analysis. The primers used in this experiment were listed in Table 1. Actin was used to standard the target mRNA expression. The fold change for each gene was measured with the comparative Ct method (Zhang et al., 2020).
2.11 Enzyme linked immunosorbent assay (ELISA)
Cytokine concentrations were determined using mouse IL-1β ELISA kit (ab197742; Abcam, Cambridge, MA, USA) depending on the manufacturer’s instructions. The signal was examined at 450 nm using a microplate reader (Bio-RAD).
2.12 Quantitative assessment of changes in endolymphatic space in cochlea
For quantitative assessment of the changes of EH in each cochlea, the length of the stretched Reissner’s membrane (L) and the ideal length of the Reissner’s membrane (L*) were measured as stated in the literature before (Katagiri et al., 2014). The increase ratio of the length change of the Reissner’s membrane (IR-L = L/L* × 100%) was calculated to evaluate the level of EH in upper and lower turns. Image J software was used for the above measurements.
2.13 Quantitative evaluation of immunofluorescence staining
All quantification in the study was performed in a blinded fashion. Three fields that covered a similar area were obtained for the mid-modiolar cochlear sections. Images were analyzed using ImageJ v1.51. The eight-bit blue channel image was corrected for background illumination using the eight-bit blue channel of a bright-field image (Zhang et al., 2022). A threshold was then applied to the image to measure the total expression identified through specific immunostaining. The integrated optical density (IOD)/pixel of the fields was used to indicate the CD68 load and the expression of MBP and FN-200.
2.14 Statistical analysis
SPSS 13.0 software (SPSS Inc., USA) was applied for statistical analysis. All data are shown as the mean ± standard deviation (SD). One-way ANOVA followed by a Dunnett multiple comparisons test was used to compare more than 2 groups. P-values < 0.05 were regarded as statistically significant differences.
3 Results
3.1 Anakinra reduced the severity of LPS-induced EH in mice
Based on previous studies, LPS was injected subcutaneous behind the ear to induce EH model (Brown et al., 2018) to determine whether anakinra effects the clinically relevant MD process. Anakinra (i.p.) and/or LPS (p.a.) was injected to age- and sex-matched C57BL/6 mice for 3 consecutive days, and then we assessed the features of relevant EH and audiovestibular symptoms thus induced (Figure 1A). Ranging from 1 to 5 days, the body weight of control mice increased slowly, while the body weight in the LPS group decreased, which was significantly different from that in the control group from the second day (Figure 1B). Compared with LPS group, anakinra treatment significantly decreased the weight loss induced by LPS (Figure 1B). Here, we quantified and evaluated the EH level. As shown in Figures 1C, D and the LPS-mice showed enlargement of scala media and more severe EH in all cochlear turns than control mice. Next, we analyzed whether anakinra reduced the outcomes of LPS-induced EH. Indeed, we found that LPS mice treated with anakinra result in alleviating of EH in cochlear upper-turns, not in lower-turns (Figures 1C, D). These data showed that anakinra decreased the severity of hydrops in LPS-induced EH mouse model.
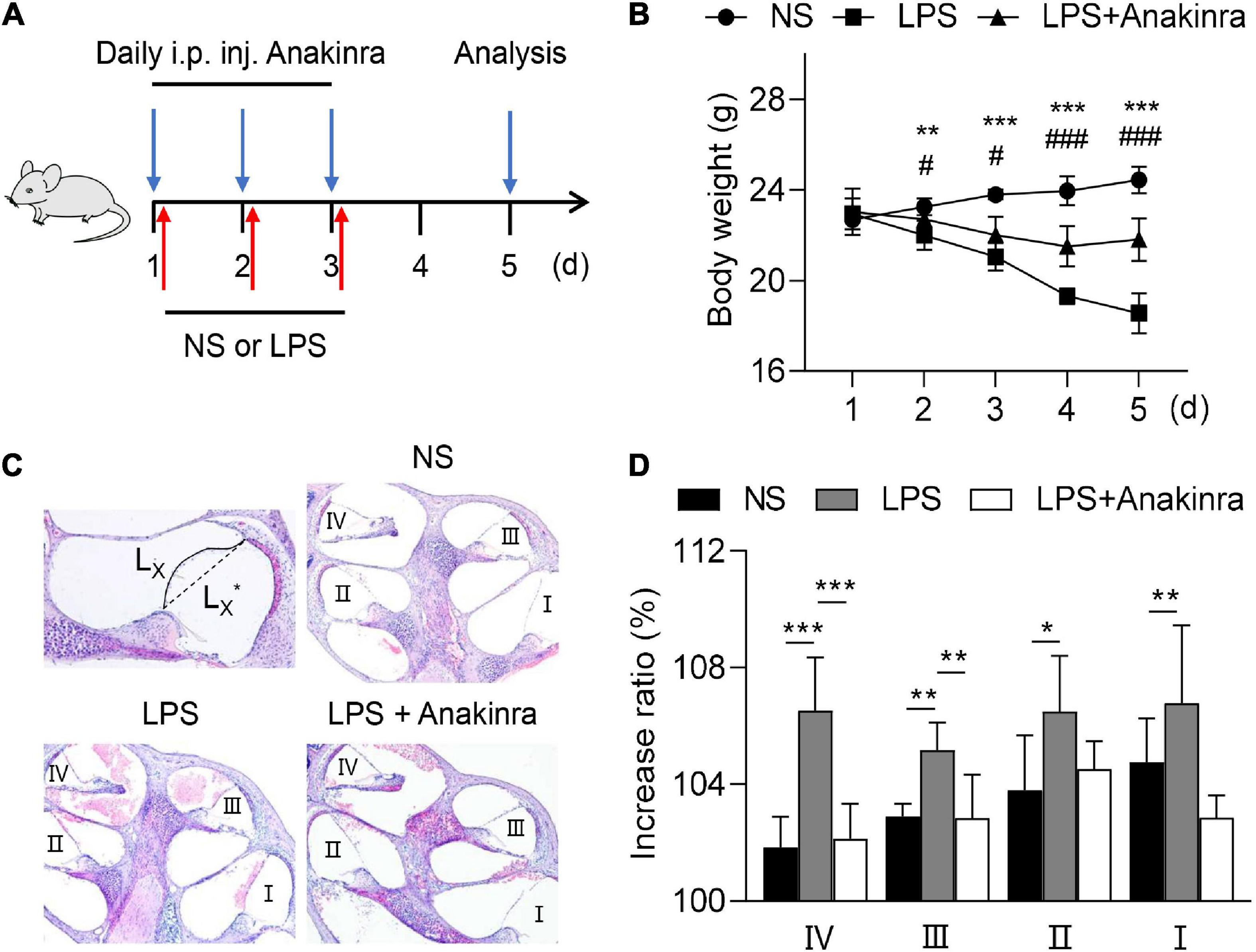
Figure 1. Anakinra reduced the severity of LPS-induced endolymphatic hydrops in mice. (A) Mice were untreated or pretreated treated with Anakinra (10 mg/kg) and challenged with LPS (10 mg/kg, i.p.) or equivalent saline for 3 consecutive days, then sacrificed at 5 days. (B) Changes of body weight in all groups (n = 5, *NS group compared to LPS group, #LPS group compared to LPS + AN group). (C) Representative images of mid-modiolar cochlear sections in all groups. (D) Measurements of the increase rations of the length of the Reissner’s membrane in cochlear half-turns I–IV (n = 5). Data are presented as mean ± SD. *or #p < 0.05, **< 0.01 ***or ###p < 0.001. NS, 0.9% normal saline; i.p. inj., intraperitoneal injected.
3.2 Anakinra alleviated LPS-induced vestibular dysfunction in mice
Vertigo caused by vestibular dysfunction is one of main clinical symptoms of MD, we next performed experiments to determine whether anakinra alleviated the vestibular symptoms of LPS-induced EH murine model. To evaluate the vestibular function, we performed behavioral tests and electrophysiological examination for mice (Nist-Lund et al., 2019). The mice were subjected to rotarod tests and the time remained on the rotating rod with increasing acceleration were recorded. After training, LPS-challenged mice performed shorter latency time to fall from accelerating rotarod than the control mice, while LPS mice treated with anakinra increased the residence time on the rotarod (Figure 2A and Supplementary Video 1). For swim test, the control group displayed normal swimming (score 0), while the scores were significantly raised for LPS-treated mice, and reversed after anakinra treatment (Figure 2B). The positive peak (P1, ↓) and negative peak (N1, ▲) latency in VEMPs in response to clicks presented at 100 dB nHL were performed and shown in Figure 3A. After administration of LPS, the mice showed increased threshold compared to those control mice, but no differences in the threshold or amplitudes between P1 and N1 or latency of P1 and N1 (Figures 3B, C). Indeed, anakinra treatment decreased the threshold and latency of P1 and N1 (Figures 3B, C). We further examined the function of horizontal semicircular canal using horizontal VOR test delivered at 20°/s (0.2, 0.5, 0.8, 1.0, 1.6, and 3.2 Hz), and the VOR gain and phase were calculated (Figure 3D). The LPS group showed a significant decrease of VOR gain from 0.2 to 3.2 Hz, and the increased phase was identified at 0.8, 1.0, and 3.2 Hz compared to NS group (Figure 3E). In contrast, LPS mice treated with anakinra resulted in reversing the gain decreases and phase increases (Figure 3E). Together, these data showed that anakinra alleviated damage to vestibular function in LPS-induced EH mouse model.
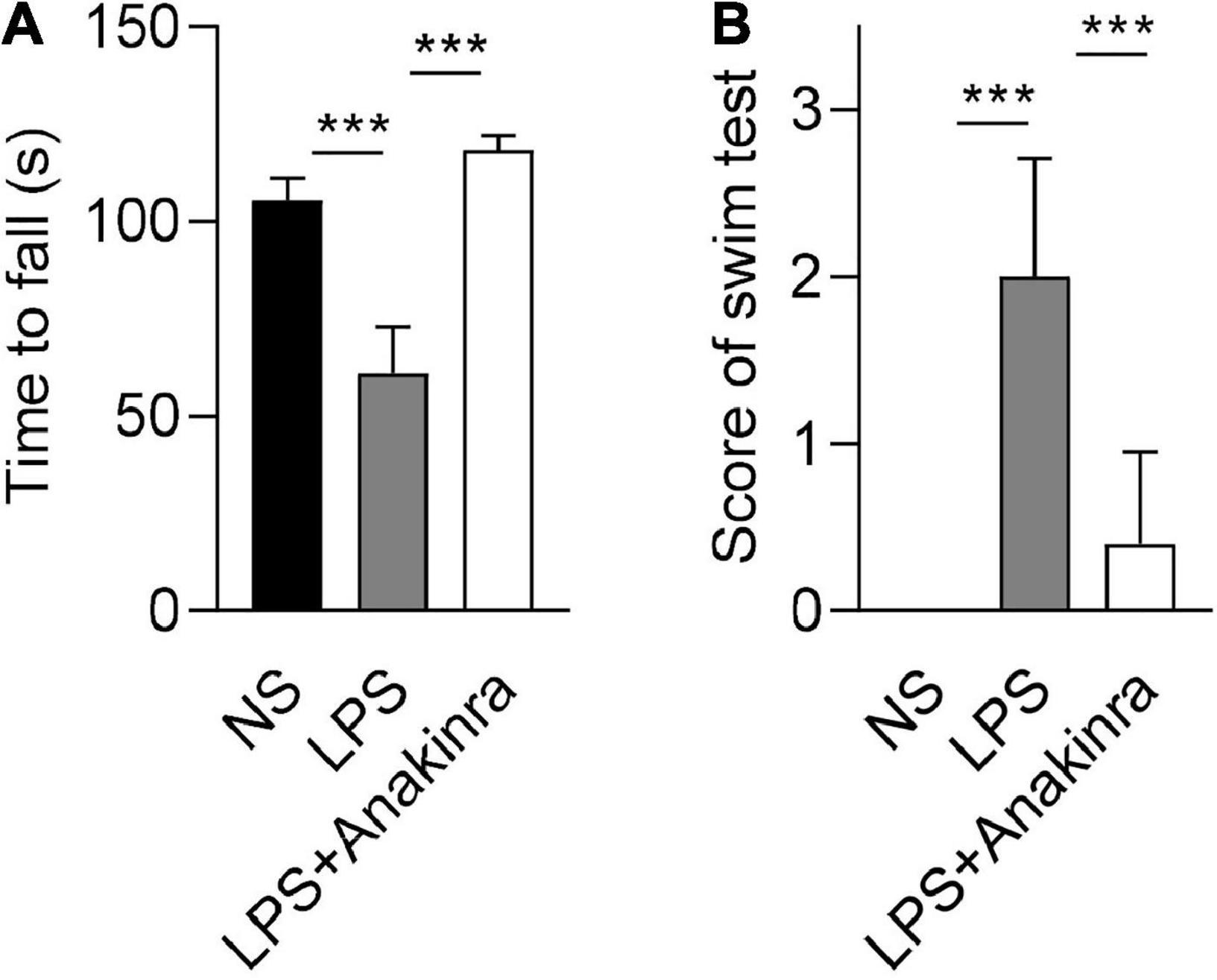
Figure 2. Anakinra alleviated LPS-induced vestibular dysfunction in mice. (A) Quantification of rotarod test (n = 5). (B) Quantification of swim tests (n = 5). Data are presented as mean ± SD. ***p < 0.001. NS, 0.9% normal saline.
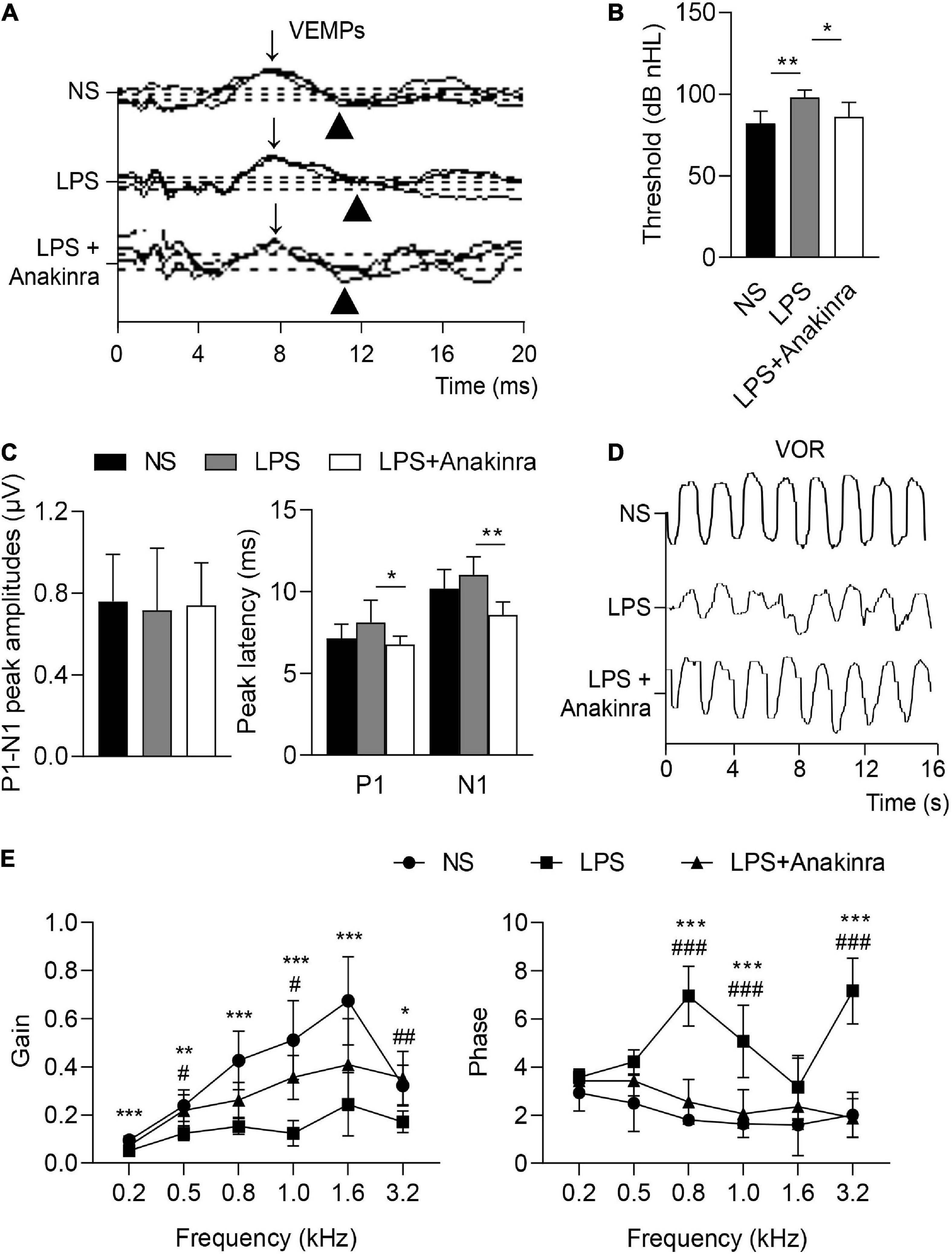
Figure 3. Anakinra alleviated LPS-induced vestibular dysfunction in mice. (A) Representative click-evoked VEMPs waves, P1(↓) and N1(▲). (B) Changes in VEMPs threshold (n = 5). (C) Changes in P1-N1 peak amplitudes, and the peak latencies of P1 and N1 at 100 dB nHL (n = 5). (D) Representative horizontal VOR waves of all groups. (E) The VOR gains and phases are plotted for all groups at a peak velocity (20°/s) and frequencies range from 0.2 to 3.2 kHz (n = 5, *NS group compared to LPS group, #LPS group compared to LPS + Anakinra group). Data are presented as mean ± SD. *or #p < 0.05, **or ##< 0.01 ***or ###p < 0.001. NS, 0.9% normal saline; VEMPs, vestibular-evoked myogenic potentials; P1, positive peak; N1, negative peak.
3.3 Anakinra ameliorated LPS-induced hearing loss in mice
To evaluate the auditory function, we measured click-evoked and tone burst-evoked (8–32 kHz) ABR. The results showed that click-induced ABR threshold were considerably higher in LPS mice compared to saline group, these effects were significantly reversed by anakinra therapy (Figures 4A, B). For tone burst-induced ABR, LPS induced the threshold and threshold shift increases in the frequency range from 8 to 24 kHz, not in 32 kHz (Figure 4C), which consistent with the clinical manifestation of low-to-medium frequencies of sensorineural hearing loss in patients with MD. Indeed, we found that mice treated with anakinra reversed threshold and threshold shift increases in the frequency range from 8 to 16 kHz caused by LPS (Figure 4C). Altogether, these data showed that anakinra ameliorated damage to auditory function especially in low-to-medium frequencies in LPS-induced EH mouse model.
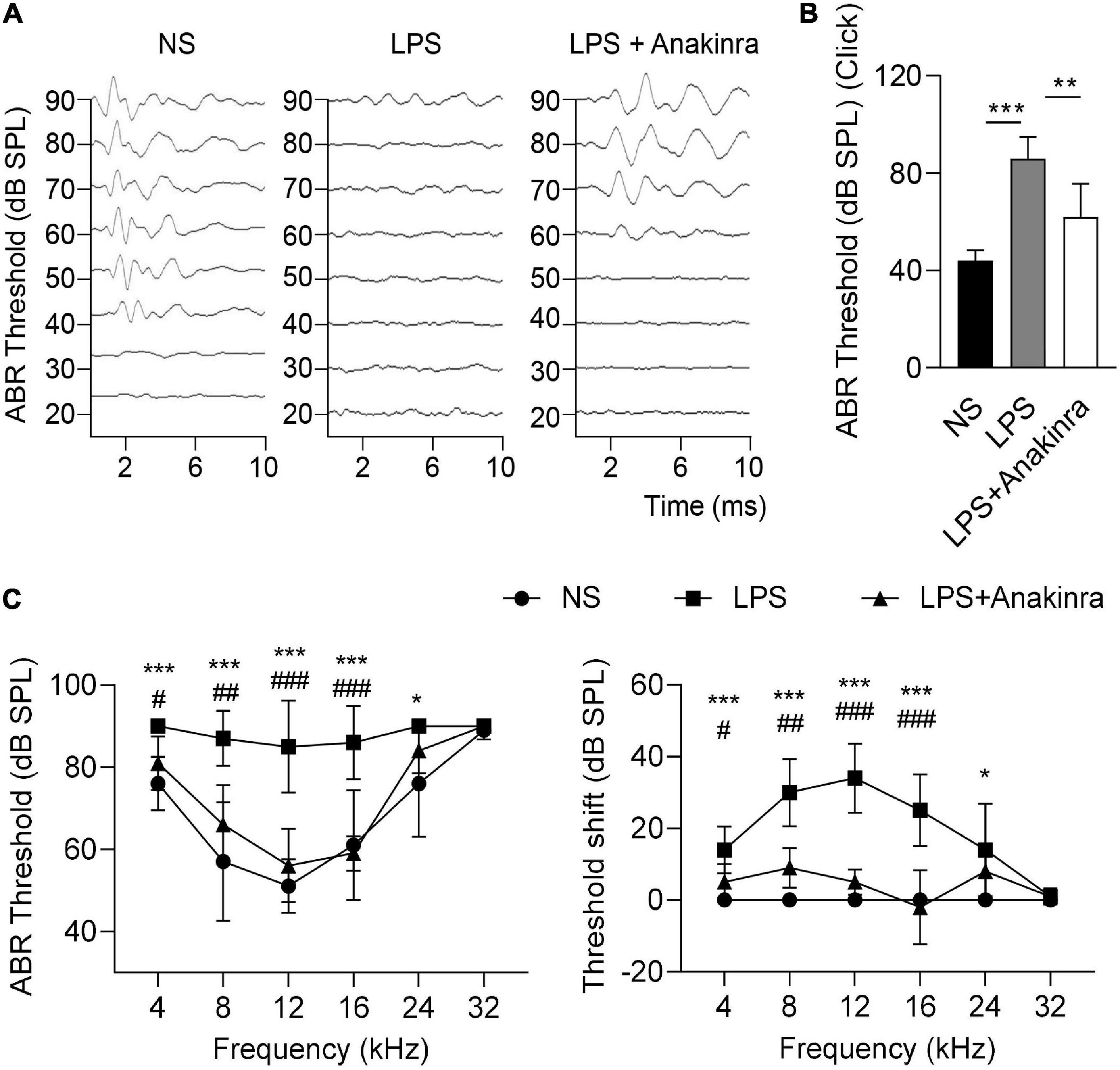
Figure 4. Anakinra ameliorated LPS-induced hearing loss in mice. (A) Representative serial ABR wave recordings in response to click sounds (n = 5). (B) ABR thresholds in response to click sounds (n = 5). (C) ABR thresholds and threshold shift in response to pure tone bursts across all frequencies tested (4, 8, 12, 16, 24, and 32 kHz) (n = 5). Data are presented as mean ± SD. *or #p < 0.05, **or ##< 0.01 ***or ###p < 0.001. NS, 0.9% normal saline; ABR, auditory brainstem response.
3.4 Anakinra inhibited LPS-induced inflammation and macrophage infiltration
As per our previous report (Zhang et al., 2022), VEO and ES of patients with MD were associated with elevated expression of inflammatory cytokines. Microarray analysis demonstrated the increased expression levels of inflammatory cytokines in the cochlea of mice treated with LPS (Lee et al., 2022). Consistent with these findings, mRNA expressions of inflammatory cytokines Il1b, Il1a, Il6, Tnf, Ifnb, Il4, Il5, and Il8 were elevated in the cochlea of LPS-treated mice (Figure 5A). Elevated matrix metalloproteinase-3 (MMP3) level affects hearing function (Nasution and Haryuna, 2019). Real-time PCR also revealed that the mRNA levels of Mmp3 and Mmp13 were upregulated in the cochlea of LPS-treated mice relative to control mice (Figure 5A). Intervention with anakinra decreased the mRNA expression levels of any of the above genes in LPS-treated mice (Figure 5A). In addition, our results showed that the serum IL-1β in LPS-treated mice was significantly increased, and effectively reduced when intervention with anakinra (Figure 5B).
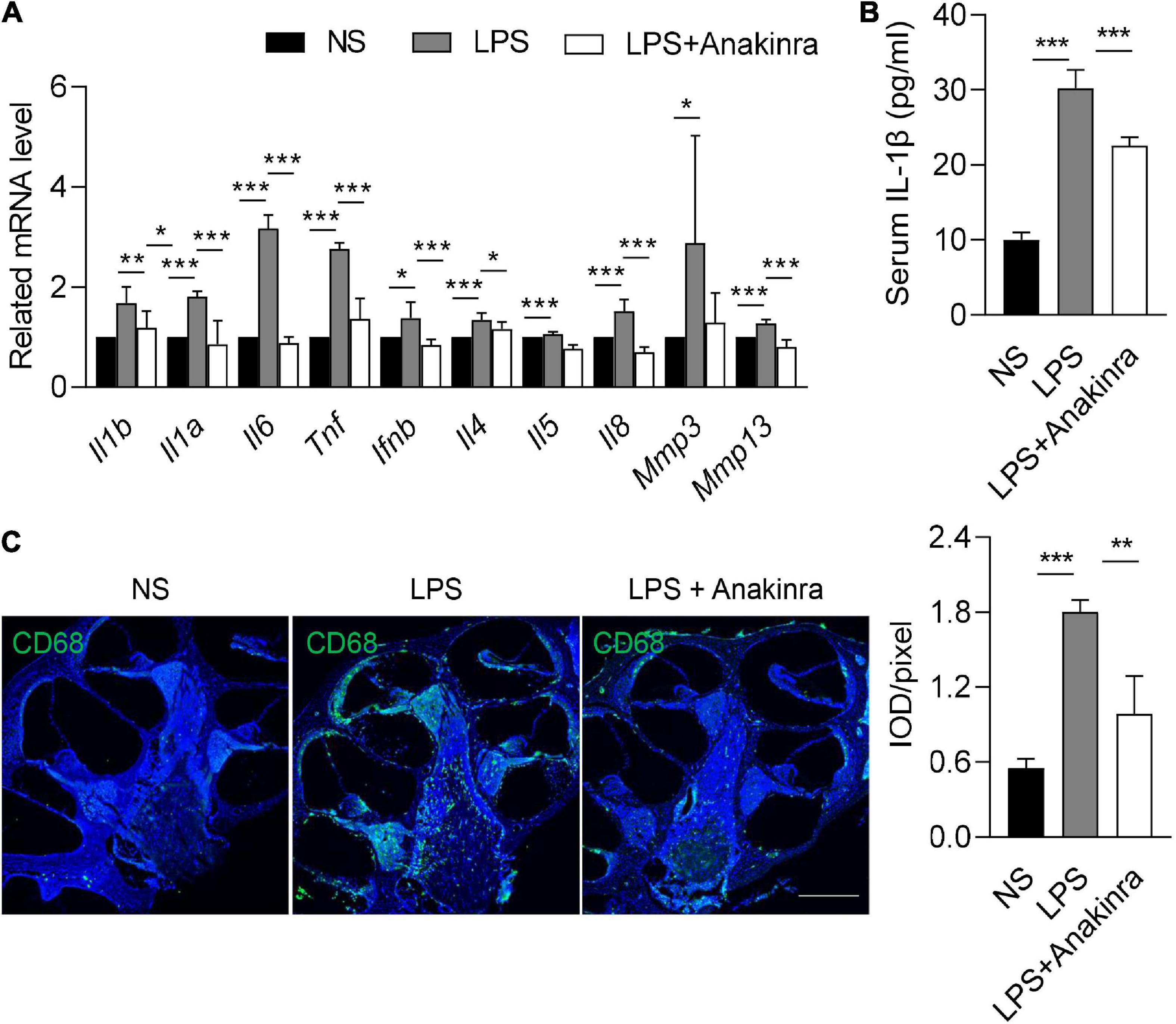
Figure 5. Anakinra inhibited LPS-induced inflammation and macrophage infiltration. (A) Related mRNA expression level in cochlea (n = 5). (B) ELISA for serum IL-1β levels (n = 5). (C) Immunofluorescence staining and quantification for CD68 (green) and DAPI (blue) in mid-modiolar cochlear sections. Analysis of images is shown at right, quantifying the load of CD68 per pixel (n = 3), scale bar = 500 μm. Data are presented as mean ± SD. *p < 0.05, **< 0.01 ***p < 0.001. NS, 0.9% normal saline.
Macrophage is the main group and considered to play an important role in the microenvironment of the cochlea (Kampfe Nordstrom et al., 2018). We next performed immunofluorescence to determine if anakinra similarly reduced macrophages numbers in the cochlea. Compared with control mice, LPS-induced EH was associated with elevated numbers of macrophages in the cochlea (Figure 5C). Indeed, LPS mice treated with anakinra attenuated the infiltration of macrophages (Figure 5C). Together, these data suggested that anakinra inhibited inflammation response and macrophage infiltration in LPS-induced EH mouse model.
3.5 Anakinra protected LPS-induced neurons and myelin sheath damage
MBP is one of the major myelin protein produced by oligodendrocytes and Schwann cells in the nervous system, and plays a critical role in maintaining the compact structure of the myelin sheath (Simons and Trotter, 2007). Class III β-tubulin (Tuj1) stains the microtubule components of type I spiral ganglion neurons (SGNs) (Liu et al., 2021b). To evaluate the effects of anakinra on cochlear nerve, we performed immunofluorescence staining to analyze myelination of SGN fibers and nerve fiber architecture using anti-MBP and anti-NF-200-antibodies, respectively. Focusing on the center of SGNs, we found that MBP surrounded SGNs and their processed (Figure 6A). In control mice, high-resolution confocal images revealed strong immunoreactivity of MBP was seen in the central myelinated processes of the cochlear nerve, and most SGNs were intact and enclosed by a MBP+ myelin sheath (Figures 6B–D). In the LPS-treated mice, MBP was expressed in the same locations as in control mice. However, abnormalities in the staining pattern and weaker immunostaining reaction for MBP were seen in the SGNs of LPS mice compared to that in NS control mice. Numerous disruptions were present in the MBP+ myelin sheath surrounding LPS SGNs in cochlear upper turn suggesting an excess of demyelinated axons and decreased myelin integrity in LPS-treated SGNs (Figure 6B). The MBP+ myelin sheaths, Tuj1+ terminations and axons in many neurons were discontinuous and in some cases appeared to be missing altogether (Figures 6C, D). In addition, LPS mice treated with anakinra upregulated the expression of MPB and reduced the loss of myelin sheath and SGNs. NF-200 is a neuronal structural protein (Chen et al., 2017). A marked decrease in immunostaining intensity for NF-200 was seen in SGNs of LPS group compared to NS group, while anakinra reversed the loss of NF-200 signal (Figure 6E). Together, these results demonstrated that anakinra protected structural damage of cochlear nerve in EH mouse cochlea.
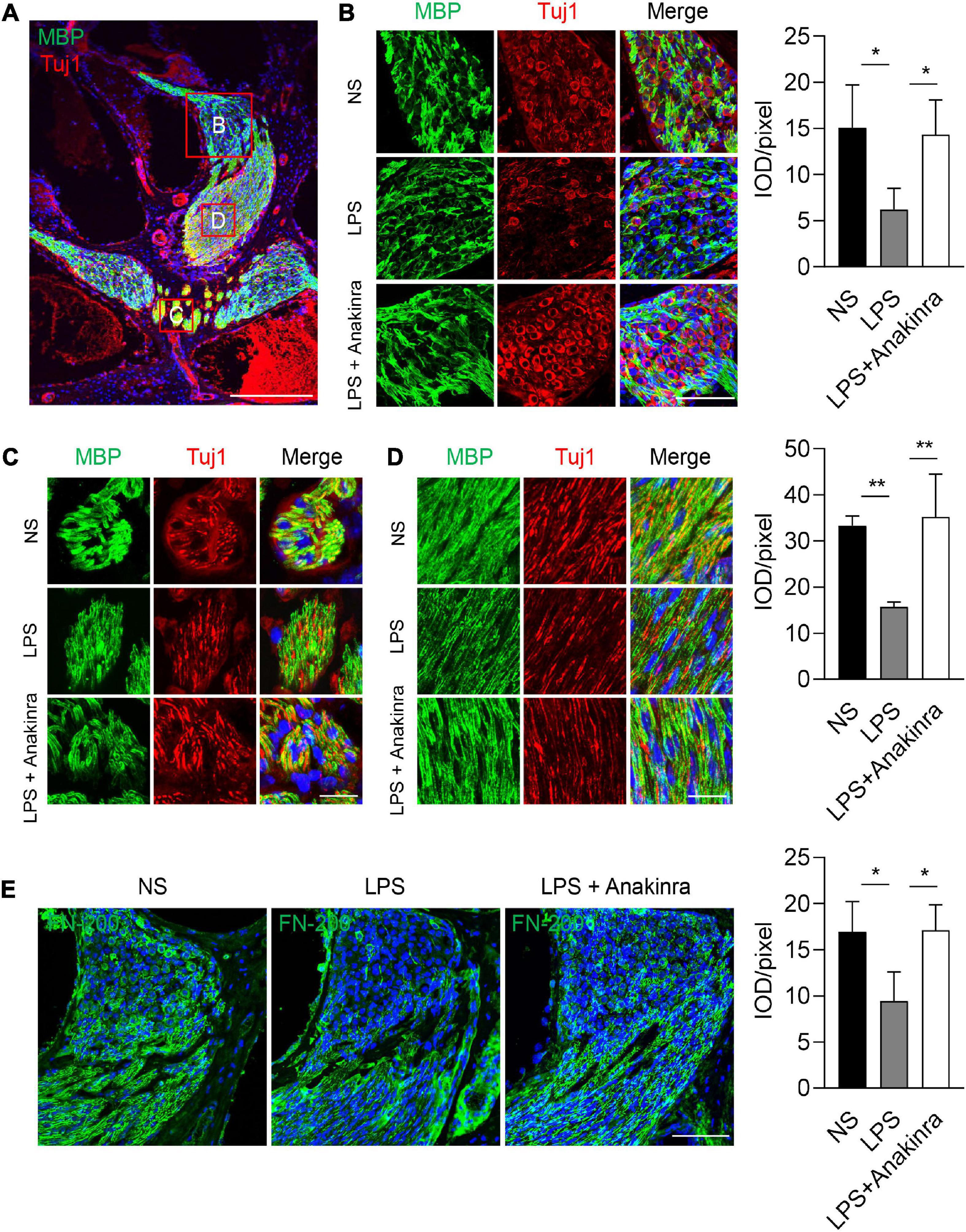
Figure 6. Anakinra protected LPS-induced neurons and myelin sheath damage. (A) Low-power view of mid-modiolar cochlear sections from LPS group illustrating auditory nerve fibers in different regions stained positively for MBP (green) and Tuj1 (red). Nuclei were counterstained with DAPI (blue), scale bar = 500μm. (B) Confocal images of SGNs for MBP (green), Tuj1 (red), and DAPI (blue) within the medial portion of Rosenthal’s canal in the upper turns of mice. Analysis of images is shown at right, quantifying the expression of MBP per pixel (n = 3), scale bar = 50 μm. (C, D) MBP+ nerve fibers are illustrated in central portions of the auditory nerve in the ears. Analysis of images is shown at right, quantifying the expression of MBP per pixel (n = 3), scale bar = 12.5 μm. (E) Immunofluorescence staining for FN-200 (green) and DAPI (bule) in cochlear axis. Analysis of images is shown at right, quantifying the expression of FN-200 per pixel (n = 3), scale bar = 50 μm. Tuj1, class III β -tubulin. Data are presented as mean ± SD. *p < 0.05, **< 0.01. NS, 0.9% normal saline.
4 Discussion
IL-1β is a strong pro-inflammatory cytokine which is mainly produced by activated macrophages and is widely regarded as a hallmark of inflammatory gene cascades (Weber et al., 2010). Consistent with high basal levels of IL-1β released by peripheral blood mononuclear cells in MD (Frejo et al., 2018), our study found that LPS induced abnormal increase of IL-1β and macrophage infiltration in EH murine model, accompanied by elevated serum IL-1β. Many immune-related diseases are accompanied by immune imbalance and increased pro-inflammatory cytokines (Smolen et al., 2005; Tian et al., 2020), which is also found in our MD-relevant murine model. The findings further suggest that IL-1β signal plays an important role in the pathogenesis of MD.
The abnormal IL-1β in the inner ear may be secreted by macrophage. Macrophage is the main group and is considered to play an important role in the innate and adaptive defense and tolerance in the inner ear (Kampfe Nordstrom et al., 2018; Liu et al., 2021a). Resident macrophages in inner ear proliferate slowly, and renewal is primarily by the infiltration and differentiation of circulating bone-marrow-derived cells (Shi, 2010), whether IL-1β in the inner ear is secreted by resident macrophages or by macrophages derived from bone marrow cells is remain unknown. Studies have shown that macrophages effect blood/labyrinth barrier integrity, and loss of macrophages is associated with tissue edema (Zhang et al., 2012). More importantly, LPS and ototoxic drugs activate macrophage and initiate local inflammatory response in the inner ear (Zhang et al., 2013, 2020; Nakanishi et al., 2017). Moreover, whether the local immune disorder leads to the systemic immune imbalance, or the local abnormality caused by the systemic imbalance remains to be elucidated, which need further study.
IL-1 receptor (IL-1R) promotes or inhibits inflammation and immune response, notably innate immune reactions, and the downstream signal transduction regulates the expression of various proinflammatory cytokines, such as IL-1, IL-6, IL-8, and TNF-a (Narayanan and Park, 2015). IL-1RA binds to IL-1R with a high affinity and competitively interferes with the binding of IL-1 to IL-1R as an inhibitory protein for IL-1 activity. Deficiency in IL-1RA due to mutation results in autoinflammatory disease, underlining the critical role of IL-1RA in regulating inflammation (Aksentijevich et al., 2009). Anakinra is a recombinant, non-glycosylated form of human IL-1RA, exerts anti-inflammatory and immunomodulatory roles by binding to IL-1R and blocking its activity. The beneficial effects of anakinra have been demonstrated in the treatment of a wide range of disorders, including auto-inflammatory disorders (Cavalli and Dinarello, 2018; Kuemmerle-Deschner et al., 2020), seizure (Lai et al., 2020), pericarditis (Khayata et al., 2020), and gout (Janssen et al., 2019), wherein the pathogenesis is associated with an excessive IL-1β expression. Study has demonstrated that anakinra improved hearing loss and inflammatory in patients of atypical cryopyrin-associated periodic syndromes (Nakanishi et al., 2017). Here, we found that anakinra reduced EH, protected auditory and vestibular function, inhibited never injury, and effectively inhibited the local and systemic inflammation in a murine model of EH, which providing a new direction for clinical treatment of MD. Given that in many diseases, anakinra plays a protective role by inhibiting IL-1β signal, we speculate that anakinra alleviates EH and audiovestibular symptoms and pathological damage by inhibiting IL-1β inflammation. However, the exact and molecular mechanisms remain unexplored.
Since its introduction in 2002, thousands of patients have received anakinra for numerous indications, with a remarkable record of safety and tolerance (Cavalli and Dinarello, 2018; Janssen et al., 2019; Khayata et al., 2020; Kuemmerle-Deschner et al., 2020; Lai et al., 2020). Our study also found that anakinra inhibited weight loss induced by LPS in mice, which further validates the well-tolerated of anakinra and provides theoretical basis for future clinical trials.
Although MD is a common disease in otology, its pathogenesis is still not clear. One of the limitations of the study is that the phenotypes of EH model currently existed do not fully mimic the clinical characteristics of MD. Previous studies directly injected LPS to the scala media, although it caused obvious hearing loss, but no vestibular dysfunction (Brown et al., 2018). Intraperitoneal injection or middle ear injection of LPS resulted in inflammation in the inner ear (Bae et al., 2021; Lee et al., 2022). In this study, postauricular injection of LPS not only induced EH and serious low-to-medium frequencies hearing loss, also caused vestibular dysfunction, which comprehensively imitated the clinical symptoms of MD. Therefore, this mode of administration provided a good animal model for MD, which is beneficial to the study of the pathological mechanism, prevention and treatment of MD. However, animal studies can never replace clinical experiments, but provide basis for clinical experiments, all animal studies need be verified in more rigorous clinical trial.
As per our previous report (Zhang et al., 2022), there are elevated cytokines in the inner ear in VEO and ES of patients with MD. In addition, early study has shown that administration of LPS activated many more cytokines and provoked an inflammatory response in mice and hair cells (Fantuzzi and Dinarello, 1996; He et al., 2020). Elevated MMP3 level also affected hearing function (Nasution and Haryuna, 2019). Our study found that except IL-1β, LPS increased expression of many cytokines (Il4, Il1a, Il6, Tnf, Ifnb, and Il8) and Mmp (Mmp3 and Mmp13). Interestingly, anakinra as an IL-1RA also inhibited the expression of the above genes. IL-1β upregulates the expression of many inflammatory cytokines, such as MMP3 and MMP13 (Fei et al., 2019; Singh et al., 2019). Studies show that anakinra effectively inhibited IL-8 and IL-6 secretion induced by IL-1β (Stoffels et al., 2014; Olivera et al., 2022), which is consistent with our results and suggest that anakinra plays a protective role by inhibiting these inflammatory mediators. Do these inflammatory mediators play a role in LPS-induced auditory and vestibular dysfunction? Case report has shown that Omalizumab by targeted immunoglobulin E completely resolved debilitating vertigo and nausea without any associated side effects in MD (Siebenhaar et al., 2007). Does targeted these inflammatory mediators (such as IL-6 receptor blockers sarilumab, anti-TNF agent infliximab) to regulate immune balance have a therapeutic effect on MD? The effectiveness of these immunotherapies will further verify that MD is an immune mediated disease.
In this study, we found that most of SGNs in the control group were enveloped by MBP+ myelin sheath. In contrast, in the LPS group, disorganization and degeneration of the myelin sheath in the cochlear nerve as well as the loss of SGNs were shown. In all complex nervous systems, neurons coexist with glial cells, which suggests that neuron-glial interaction is a main feature of neurological function. In the peripheral niveous nervous system, Schwann cells not only myelinate axons and neuron bodies, but also play an important role in maintaining the long-term functional integrity and survival of neuron (Nave and Trapp, 2008). Unlike in human, the somata of most mouse SGNs are myelinated (Xing et al., 2012). Importantly, loss of Schwann cells and intact myelin causes progressive axon degeneration and local inflammation, contributing to various peripheral neuropathies (Bourque et al., 2015). Thus, it is highly likely that the LPS-induced loss of myelin sheath integrity contributes to the degeneration of SGNs and declines in cochlear nerve function. Overload of IL-1β secretion have been shown to promote demyelination and deteriorate the severity of inflammatory neurodegenerative experimental autoimmune encephalomyelitis (Inoue et al., 2012; Huang et al., 2019). In our study, anakinra effectively alleviated cochlear nerve injury and reduced the loss of MBP by inhibiting IL-1β response, which further proves that LPS-induced neurodegeneration is at least partly mediated by IL-1β.
Cochlear nerve and vestibular nerve are very similar in terms of anatomy and development (Jeffery and Spoor, 2004). Both cochlear and vestibular nerve exhibited similar damage following exposure to ototoxic agent (Ye et al., 2019). Therefore, we only demonstrated the changes of the cochlear nerve, did not pay attention to the vestibular nerve. Whether vestibular dysfunction induced by LPS is caused by injury of vestibular myelin sheath and nerve fibers remains to be further investigated. Although no attempt was made to study the effect of hair cell in our study, study has shown that neuronal degeneration occurs independent of hair cell loss (Linthicum and Fayad, 2009). It is unclear whether LPS-induced myelin loss is a primary event which leads to secondary neurodegeneration, and vice versa. Further molecular and functional studies of the myelin protein are needed to better understand the causes of demyelination and axon degeneration.
In summary, our results demonstrated that anakinra attenuated EH, improved auditory and vestibular function, protected cochlear nerve in a murine model of EH. Furthermore, anakinra reduced the expressions of the inflammatory cytokines, macrophage infiltration and neurodegeneration in the cochlea of mice. These findings suggest that targeting IL-1 signaling may be a promising approach for treating MD.
Data availability statement
The original contributions presented in this study are included in the article/Supplementary material, further inquiries can be directed to the corresponding author/s.
Ethics statement
The animal study was reviewed and approved by Shandong Provincial ENT Hospital, Cheeloo College of Medicine, Shandong University Ethics Committee.
Author contributions
HW, DZ, and NL designed the study. NZ, JL, and YLiu performed experiments. NZ and NL drafted the manuscript. SW and JG analyzed the data. XL, YS, LK, and WX housed the mice. All authors revised and approved the final version of the manuscript.
Funding
This study was supported by the Major Program of National Natural Science Foundation of China (no. 82196821), the Major Fundamental Research Program of the Natural Science Foundation of Shandong Province, China (no. ZR2021ZD40), The Taishan Scholar Foundation of Shandong Province (no. ts20130913), the National Natural Science Foundation of China (nos. 82171150 and 82271172), and the Natural Science Foundation of Shandong Province (nos. ZR2020MH179 and ZR2021MH385).
Conflict of interest
The authors declare that the research was conducted in the absence of any commercial or financial relationships that could be construed as a potential conflict of interest.
Publisher’s note
All claims expressed in this article are solely those of the authors and do not necessarily represent those of their affiliated organizations, or those of the publisher, the editors and the reviewers. Any product that may be evaluated in this article, or claim that may be made by its manufacturer, is not guaranteed or endorsed by the publisher.
Supplementary material
The Supplementary Material for this article can be found online at: https://www.frontiersin.org/articles/10.3389/fncel.2022.1088099/full#supplementary-material
References
Aksentijevich, I., Masters, S., Ferguson, P., Dancey, P., Frenkel, J., van Royen-Kerkhoff, A., et al. (2009). An autoinflammatory disease with deficiency of the interleukin-1-receptor antagonist. N Engl J. Med. 360, 2426–2437. doi: 10.1056/NEJMoa0807865
Alexander, T., and Harris, J. (2010). Current epidemiology of Meniere’s syndrome. Otolaryngol. Clin. North Am. 43, 965–970. doi: 10.1016/j.otc.2010.05.001
Bae, S., Yoo, J., Choe, Y., Kwak, S., Choi, J., Jung, J., et al. (2021). Neutrophils infiltrate into the spiral ligament but not the stria vascularis in the cochlea during lipopolysaccharide-induced inflammation. Theranostics 11, 2522–2533. doi: 10.7150/thno.49121
Bourque, P., Chardon, J., and Massie, R. (2015). Autoimmune peripheral neuropathies. Clin. Chim. Acta. 449, 37–42. doi: 10.1016/j.cca.2015.02.039
Brown, D., Sokolic, L., Fung, A., and Pastras, C. (2018). Response of the inner ear to lipopolysaccharide introduced directly into scala media. Hear. Res. 370, 105–112. doi: 10.1016/j.heares.2018.10.007
Canna, S., de Jesus, A., Gouni, S., Brooks, S., Marrero, B., Liu, Y., et al. (2014). An activating NLRC4 inflammasome mutation causes autoinflammation with recurrent macrophage activation syndrome. Nat. Genet. 46, 1140–1146. doi: 10.1038/ng.3089
Cavalli, G., and Dinarello, C. (2018). Anakinra Therapy for Non-cancer Inflammatory Diseases. Front. Pharmacol. 9:1157. doi: 10.3389/fphar.2019.00148
Chen, G., Kim, Y., Li, H., Luo, H., Liu, D., Zhang, Z., et al. (2017). PD-L1 inhibits acute and chronic pain by suppressing nociceptive neuron activity via PD-1. Nat. Neurosci. 20, 917–926. doi: 10.1038/nn.4571
Clopath, C., Badura, A., De Zeeuw, C., and Brunel, N. (2014). A cerebellar learning model of vestibulo-ocular reflex adaptation in wild-type and mutant mice. J. Neurosci. 34, 7203–7215. doi: 10.1523/JNEUROSCI.2791-13.2014
Derebery, M., and Berliner, K. (2010). Allergy and its relation to Meniere’s disease. Otolaryngol. Clin. North Am. 43, 1047–1058. doi: 10.1016/j.otc.2010.05.004
Dinarello, C. (2018). Overview of the IL-1 family in innate inflammation and acquired immunity. Immunol. Rev. 281, 8–27. doi: 10.1111/imr.12621
Fantuzzi, G., and Dinarello, C. (1996). The inflammatory response in interleukin-1 beta-deficient mice: Comparison with other cytokine-related knock-out mice. J. Leukoc. Biol. 59, 489–493. doi: 10.1002/jlb.59.4.489
Fei, J., Liang, B., Jiang, C., Ni, H., and Wang, L. (2019). Luteolin inhibits IL-1beta-induced in fl ammation in rat chondrocytes and attenuates osteoarthritis progression in a rat model. Biomed Pharmacother. 109, 1586–1592. doi: 10.1016/j.biopha.2018.09.161
Frejo, L., Gallego-Martinez, A., Requena, T., Martin-Sanz, E., Amor-Dorado, J., Soto-Varela, A., et al. (2018). Proinflammatory cytokines and response to molds in mononuclear cells of patients with Meniere disease. Sci. Rep. 8:5974. doi: 10.1038/s41598-018-23911-4
Furuta, T., Teranishi, M., Uchida, Y., Nishio, N., Kato, K., Otake, H., et al. (2011). Association of interleukin-1 gene polymorphisms with sudden sensorineural hearing loss and Meniere’s disease. Int. J. Immunogenet. 38, 249–254. doi: 10.1111/j.1744-313X.2011.01004.x
Gabay, C., Lamacchia, C., and Palmer, G. (2010). IL-1 pathways in inflammation and human diseases. Nat. Rev. Rheumatol. 6, 232–241. doi: 10.1038/nrrheum.2010.4
Garces, K. (2001). Anakinra: Interleukin-1 receptor antagonist therapy for rheumatoid arthritis. Issues Emerg. Health Technol. 30, 365–380. doi: 10.1016/j.rdc.2004.01.005
Gazquez, I., Soto-Varela, A., Aran, I., Santos, S., Batuecas, A., Trinidad, G., et al. (2011). High prevalence of systemic autoimmune diseases in patients with Menière’s disease. PLoS One. 6:e26759. doi: 10.1371/journal.pone.0026759
Greco, A., Gallo, A., Fusconi, M., Marinelli, C., Macri, G., and de Vincentiis, M. (2012). Meniere’s disease might be an autoimmune condition? Autoimmun. Rev. 11, 731–738. doi: 10.1016/j.autrev.2012.01.004
Hallpike, C., and Cairns, H. (1938). Observations on the pathology of Meniere’s syndrome: (Section of Otology). Proc. R. Soc. Med. 31, 1317–1336. doi: 10.1177/003591573803101112
He, Z., Zou, S., Li, M., Liao, F., Wu, X., Sun, H., et al. (2020). The nuclear transcription factor FoxG1 affects the sensitivity of mimetic aging hair cells to inflammation by regulating autophagy pathways. Redox Biol. 28:101364. doi: 10.1016/j.redox.2019.101364
Huang, X., Feng, Z., Jiang, Y., Li, J., Xiang, Q., Guo, S., et al. (2019). VSIG4 mediates transcriptional inhibition of and β in macrophages. Sci. Adv. 5:eaau7426. doi: 10.1126/sciadv.aau7426
Inoue, M., Williams, K., Gunn, M., and Shinohara, M. (2012). NLRP3 inflammasome induces chemotactic immune cell migration to the CNS in experimental autoimmune encephalomyelitis. Proc. Natl. Acad. Sci. U.S.A. 109, 10480–10485. doi: 10.1073/pnas.1201836109
Janssen, C., Oude Voshaar, M., Vonkeman, H., Jansen, T., Janssen, M., Kok, M., et al. (2019). Anakinra for the treatment of acute gout flares: A randomized, double-blind, placebo-controlled, active-comparator, non-inferiority trial. Rheumatology. 58, 1344–1352. doi: 10.1093/rheumatology/key402
Jeffery, N., and Spoor, F. (2004). Prenatal growth and development of the modern human labyrinth. J. Anat. 204, 71–92. doi: 10.1111/j.1469-7580.2004.00250.x
Jesus, A., and Goldbach-Mansky, R. (2014). IL-1 blockade in autoinflammatory syndromes. Annu. Rev. Med. 65, 223–244. doi: 10.1146/annurev-med-061512-150641
Kampfe Nordstrom, C., Danckwardt-Lilliestrom, N., Laurell, G., Liu, W., and Rask-Andersen, H. (2018). The human endolymphatic sac and inner ear immunity: Macrophage interaction and molecular expression. Front. Immunol. 9:3181. doi: 10.3389/fimmu.2018.03181
Katagiri, Y., Takumida, M., Hirakawa, K., and Anniko, M. (2014). Long-term administration of vasopressin can cause Meniere’s disease in mice. Acta Otolaryngol. 134, 990–1004. doi: 10.3109/00016489.2014.902989
Khayata, M., Shah, N., Verma, B., Giugni, A., Alkharabsheh, S., Asher, C., et al. (2020). Usefulness of interleukin-1 receptor antagonists in patients with recurrent pericarditis. Am. J. Cardiol. 127, 184–190. doi: 10.1016/j.amjcard.2020.03.041
Kooistra, E., Waalders, N., Grondman, I., Janssen, N., de Nooijer, A., Netea, M., et al. (2020). Anakinra treatment in critically ill COVID-19 patients: A prospective cohort study. Crit. Care 24:688. doi: 10.1186/s13054-020-03364-w
Kouhi, A., Shakeri, S., Yazdani, N., Shababi, N., Mohseni, A., Mohseni, A., et al. (2021). Association of pro-inflammatory cytokine gene polymorphism with Meniere’s disease in an iranian sample. Iran. J. Allergy Asthma Immunol. 20, 734–739. doi: 10.18502/ijaai.v20i6.8024
Kuemmerle-Deschner, J., Gautam, R., George, A., Raza, S., Lomax, K., and Hur, P. (2020). Systematic literature review of efficacy/effectiveness and safety of current therapies for the treatment of cryopyrin-associated periodic syndrome, hyperimmunoglobulin D syndrome and tumour necrosis factor receptor-associated periodic syndrome. RMD Open 6:e001227. doi: 10.1136/rmdopen-2020-001227
Lai, Y., Muscal, E., Wells, E., Shukla, N., Eschbach, K., Hyeong Lee, K., et al. (2020). Anakinra usage in febrile infection related epilepsy syndrome: An international cohort. Ann. Clin. Transl. Neurol. 7, 2467–2474. doi: 10.1002/acn3.51229
Lee, S., Kim, S., Han, K., Woong Choi, J., Byung Chae, H., Yeon Choi, D., et al. (2022). Microarray analysis of lipopolysaccharide-induced endotoxemia in the cochlea. Gene. 823:146347. doi: 10.1016/j.gene.2022.146347
Li, J., Yu, L., Xia, R., Gao, F., Luo, W., and Jing, Y. (2013). Postauricular hypodermic injection to treat inner ear disorders: Experimental feasibility study using magnetic resonance imaging and pharmacokinetic comparison. J. Laryngol. Otol. 127, 239–245. doi: 10.1017/S0022215113000017
Li, L., Wang, Y., An, L., Kong, X., and Huang, T. (2017). A network-based method using a random walk with restart algorithm and screening tests to identify novel genes associated with Meniere’s disease. PLoS One. 12:e0182592. doi: 10.1371/journal.pone.0182592
Linthicum, F., and Fayad, J. (2009). Spiral ganglion cell loss is unrelated to segmental cochlear sensory system degeneration in humans. Otol. Neurotol. 30, 418–422. doi: 10.1097/MAO.0b013e31819a8827
Liu, W., Danckwardt-Lilliestrom, N., Schrott-Fischer, A., Glueckert, R., and Rask-Andersen, H. (2021b). Distribution of immune cells including macrophages in the human cochlea. Front. Neurol. 12:781702. doi: 10.3389/fneur.2021.781702
Liu, W., Molnar, M., Garnham, C., Benav, H., and Rask-Andersen, H. (2018). Macrophages in the human cochlea: Saviors or predators-a study using super-resolution immunohistochemistry. Front. Immunol. 9:223. doi: 10.3389/fimmu.2018.00223
Liu, W., Xu, L., Wang, X., Zhang, D., Sun, G., Wang, M., et al. (2021a). PRDX1 activates autophagy via the PTEN-AKT signaling pathway to protect against cisplatin-induced spiral ganglion neuron damage. Autophagy 17, 4159–4181. doi: 10.1080/15548627.2021.1905466
Nakanishi, H., Kawashima, Y., Kurima, K., Chae, J., Ross, A., Pinto-Patarroyo, G., et al. (2017a). NLRP3 mutation and cochlear autoinflammation cause syndromic and nonsyndromic hearing loss DFNA34 responsive to anakinra therapy. Proc. Natl. Acad. Sci. U.S.A. 114, E7766–E7775. doi: 10.1073/pnas.1702946114
Nakashima, T., Pyykko, I., Arroll, M., Casselbrant, M., Foster, C., Manzoor, N., et al. (2016). Meniere’s disease. Nat. Rev. Dis. Primers. 2:16028. doi: 10.1038/nrdp.2016.28
Narayanan, K., and Park, H. (2015). Toll/interleukin-1 receptor (TIR) domain-mediated cellular signaling pathways. Apoptosis 20, 196–209. doi: 10.1007/s10495-014-1073-1
Nasution, M., and Haryuna, T. (2019). Elevated matrix metalloproteinase-3 level may affect hearing function in patients with rheumatoid arthritis. J. Chin. Med. Assoc. 82, 272–276. doi: 10.1097/JCMA.0000000000000036
Nave, K., and Trapp, B. (2008). Axon-glial signaling and the glial support of axon function. Annu. Rev. Neurosci. 31, 535–561. doi: 10.1146/annurev.neuro.30.051606.094309
Nist-Lund, C., Pan, B., Patterson, A., Asai, Y., Chen, T., Zhou, W., et al. (2019). Improved TMC1 gene therapy restores hearing and balance in mice with genetic inner ear disorders. Nat. Commun. 10:236. doi: 10.1038/s41467-018-08264-w
Okuno, T., and Sando, I. (1987). Localization, frequency, and severity of endolymphatic hydrops and the pathology of the labyrinthine membrane in Meniere’s disease. Ann. Otol. Rhinol. Laryngol. 96, 438–445. doi: 10.1177/000348948709600418
Olivera, I., Sanz-Pamplona, R., Bolanos, E., Rodriguez, I., Etxeberria, I., Cirella, A., et al. (2022). A Therapeutically Actionable Protumoral Axis of Cytokines Involving IL-8. TNFalpha, and IL-1beta. Cancer Discov. 12, 2140–2157. doi: 10.1158/2159-8290.CD-21-1115
O’Malley, J., Nadol, J. Jr., and McKenna, M. (2016). Anti CD163+, Iba1+, and CD68+ Cells in the adult human inner ear: Normal distribution of an unappreciated class of macrophages/microglia and implications for inflammatory otopathology in humans. Otol. Neurotol. 37, 99–108. doi: 10.1097/MAO.0000000000000879
Requena, T., Espinosa-Sanchez, J., Cabrera, S., Trinidad, G., Soto-Varela, A., Santos-Perez, S., et al. (2014). Familial clustering and genetic heterogeneity in Meniere’s disease. Clin. Genet. 85, 245–252. doi: 10.1111/cge.12150
Saag, K., Khanna, P., Keenan, R., Ohlman, S., Osterling Koskinen, L., Sparve, E., et al. (2021). A Randomized, phase II study evaluating the efficacy and safety of anakinra in the treatment of gout flares. Arthritis Rheumatol. 73, 1533–1542. doi: 10.1002/art.41699
Sajjadi, H., and Paparella, M. (2008). Meniere’s disease. Lancet. 372, 406–414. doi: 10.1016/S0140-6736(08)61161-7
Seo, Y., and Brown, D. (2020). Experimental animal models for Meniere’s disease: A mini-review. J. Audiol. Otol. 24, 53–60. doi: 10.7874/jao.2020.00115
Sheykholeslami, K., Megerian, C., and Zheng, Q. (2009). Vestibular evoked myogenic potentials in normal mice and Phex mice with spontaneous endolymphatic hydrops. Otol. Neurotol. 30, 535–544. doi: 10.1097/MAO.0b013e31819bda13
Shi, X. (2010). Resident macrophages in the cochlear blood-labyrinth barrier and their renewal via migration of bone-marrow-derived cells. Cell Tissue Res. 342, 21–30. doi: 10.1007/s00441-010-1040-2
Siebenhaar, F., Kühn, W., Zuberbier, T., and Maurer, M. (2007). Successful treatment of cutaneous mastocytosis and Ménière disease with anti-IgE therapy. J. Allergy Clin. Immunol. 120, 213–215. doi: 10.1016/j.jaci.2007.05.011
Simons, M., and Trotter, J. (2007). Wrapping it up: The cell biology of myelination. Curr. Opin. Neurobiol. 17, 533–540. doi: 10.1016/j.conb.2007.08.003
Singh, A., Fechtner, S., Chourasia, M., Sicalo, J., and Ahmed, S. (2019). Critical role of IL-1alpha in IL-1beta-induced inflammatory responses: Cooperation with NF-kappaBp65 in transcriptional regulation. FASEB J. 33, 2526–2536. doi: 10.1096/fj.201801513R
Smolen, J., Redlich, K., Zwerina, J., Aletaha, D., Steiner, G., and Schett, G. (2005). Pro-inflammatory cytokines in rheumatoid arthritis: Pathogenetic and therapeutic aspects. Clin. Rev. Allergy Immunol. 28, 239–248. doi: 10.1385/CRIAI:28:3:239
Stoffels, B., Hupa, K., Snoek, S., van Bree, S., Stein, K., Schwandt, T., et al. (2014). Postoperative ileus involves interleukin-1 receptor signaling in enteric glia. Gastroenterology 146, 176–87e1. doi: 10.1053/j.gastro.2013.09.030
Takumida, M., Akagi, N., and Anniko, M. (2008). A new animal model for Meniere’s disease. Acta Otolaryngol. 128, 263–271. doi: 10.1080/00016480701497436
Tian, M., Liu, W., Zhang, Q., Huang, Y., Li, W., Wang, W., et al. (2020). MYSM1 represses innate immunity and autoimmunity through suppressing the cGAS-STING pathway. Cell Rep. 33:108297. doi: 10.1016/j.celrep.2020.108297
Tyrrell, J., Whinney, D., and Taylor, T. (2016). The cost of Meniere’s disease: A novel multisource approach. Ear Hear. 37, e202–e209. doi: 10.1097/AUD.0000000000000264
Weber, A., Wasiliew, P., and Kracht, M. (2010). Interleukin-1 (IL-1) pathway. Sci. Signal. 3:cm1. doi: 10.1126/scisignal.3105cm1
Wehrli, M., Gallagher, K., Chen, Y., Leick, M., McAfee, S., El-Jawahri, A., et al. (2022). Single-center experience using anakinra for steroid-refractory immune effector cell-associated neurotoxicity syndrome (ICANS). J. Immunother. Cancer 10:e003847. doi: 10.1136/jitc-2021-003847
Xing, Y., Samuvel, D., Stevens, S., Dubno, J., Schulte, B., and Lang, H. (2012). Age-related changes of myelin basic protein in mouse and human auditory nerve. PLoS One. 7:e34500. doi: 10.1371/journal.pone.0034500
Yang, X., Zhou, S., Wu, J., Liao, Q., Wang, C., Liu, M., et al. (2019). Surgery-free video-oculography in mouse models: Enabling quantitative and short-interval longitudinal assessment of vestibular function. Neurosci. Lett. 696, 212–218. doi: 10.1016/j.neulet.2018.12.036
Ye, H., Xing, Y., Zhang, L., Zhang, J., Jiang, H., Ding, D., et al. (2019). Bilirubin-induced neurotoxic and ototoxic effects in rat cochlear and vestibular organotypic cultures. Neurotoxicology 71, 75–86. doi: 10.1016/j.neuro.2018.12.004
Zhang, F., Zhang, J., Neng, L., and Shi, X. (2013). Characterization and inflammatory response of perivascular-resident macrophage-like melanocytes in the vestibular system. J. Assoc. Res. Otolaryngol. 14, 635–643. doi: 10.1007/s10162-013-0403-2
Zhang, N., Cai, J., Xu, L., Wang, H., and Liu, W. (2020). Cisplatin-induced stria vascularis damage is associated with inflammation and fibrosis. Neural Plasticity 2020, 1–13. doi: 10.1155/2020/8851525
Zhang, N., Lyu, Y., Guo, J., Liu, J., Song, Y., Fan, Z., et al. (2022). Bidirectional transport of IgE by CD23 in the inner ear of patients with Meniere’s disease. J. Immunol. 208, 827–838. doi: 10.4049/jimmunol.2100745
Zhang, W., Dai, M., Fridberger, A., Hassan, A., Degagne, J., Neng, L., et al. (2012). Perivascular-resident macrophage-like melanocytes in the inner ear are essential for the integrity of the intrastrial fluid-blood barrier. Proc. Natl. Acad. Sci. U.S.A. 109, 10388–10393. doi: 10.1073/pnas.1205210109
Keywords: anakinra, IL-1β, endolymphatic hydrops, Ménière’s disease, demyelination
Citation: Zhang N, Li N, Wang S, Xu W, Liu J, Lyu Y, Li X, Song Y, Kong L, Liu Y, Guo J, Fan Z, Zhang D and Wang H (2022) Protective effect of anakinra on audiovestibular function in a murine model of endolymphatic hydrops. Front. Cell. Neurosci. 16:1088099. doi: 10.3389/fncel.2022.1088099
Received: 03 November 2022; Accepted: 29 November 2022;
Published: 15 December 2022.
Edited by:
Chen Chen, Stanford University, United StatesReviewed by:
Qing Sun, PLA General Hospital, ChinaAngela Ballesteros, National Institute on Deafness and Other Communication Disorders (NIH), United States
Copyright © 2022 Zhang, Li, Wang, Xu, Liu, Lyu, Li, Song, Kong, Liu, Guo, Fan, Zhang and Wang. This is an open-access article distributed under the terms of the Creative Commons Attribution License (CC BY). The use, distribution or reproduction in other forums is permitted, provided the original author(s) and the copyright owner(s) are credited and that the original publication in this journal is cited, in accordance with accepted academic practice. No use, distribution or reproduction is permitted which does not comply with these terms.
*Correspondence: Daogong Zhang, zhangdaogong1978@163.com; Haibo Wang, whboto11@163.com
†These authors have contributed equally to this work and share first authorship