- 1Department of Anesthesiology and Perioperative Medicine, University of California, Los Angeles, Los Angeles, CA, United States
- 2Department of Psychiatry and Biobehavioral Sciences, Semel Institute for Neuroscience and Human Behavior, University of California, Los Angeles, Los Angeles, CA, United States
- 3Department of Radiological Sciences, University of California, Los Angeles, Los Angeles, CA, United States
- 4Department of Bioengineering, University of California, Los Angeles, Los Angeles, CA, United States
- 5David Geffen School of Medicine, Brain Research Institute, University of California, Los Angeles, Los Angeles, CA, United States
Background: Adults undergoing lung transplant, as a lifesaving treatment for end stage lung disease, exhibit high levels of peri-operative neurocognitive dysfunction in multiple domains, including delirium, cognition, and autonomic deficits. These complications impact healthcare costs, quality of life, and patient outcomes. Post-operative symptoms likely result from loss of brain tissue integrity in sites mediating such regulatory functions. Our aim in this study was to examine peri-operative neurocognitive dysfunction and brain tissue changes after lung transplant in adults.
Methods: We retrospectively examined the UCLA lung transplant database to identify 114 lung transplant patients with pre-operative clinical and neurocognitive data. Of 114 patients, 9 lung transplant patients had pre- and post-transplant brain magnetic resonance imaging. Clinical and neurocognitive data were summarized for all subjects, and brain tissue volume changes, using T1-weighted images, before and after transplant were examined. T1-weighted images were partitioned into gray matter (GM)-tissue type, normalized to a common space, smoothed, and the smoothed GM-volume maps were compared between pre- and post-transplant (paired t-tests; covariate, age; SPM12, p < 0.005).
Results: Increased comorbidities, including the diabetes mellitus (DM), hypertension, kidney disease, and sleep disordered breathing, as well as higher rates of neurocognitive dysfunction were observed in the lung transplant patients, with 41% experiencing post-operative delirium, 49% diagnosed with a mood disorder, and 25% of patients diagnosed with cognitive deficits, despite incomplete documentation. Similarly, high levels of delirium, cognitive dysfunction, and mood disorder were noted in a subset of patients used for brain MRI evaluation. Significantly decreased GM volumes emerged in multiple brain regions, including the frontal and prefrontal, parietal, temporal, bilateral anterior cingulate and insula, putamen, and cerebellar cortices.
Conclusion: Adults undergoing lung transplant often show significant pre-operative comorbidities, including diabetes mellitus, hypertension, and chronic kidney disease, as well as neurocognitive dysfunction. In addition, patients with lung transplant show significant brain tissue changes in regions that mediate cognition, autonomic, and mood functions. The findings indicate a brain structural basis for many enhanced post-operative symptoms and suggest a need for brain tissue protection in adults undergoing lung transplant to improve health outcomes.
Introduction
Chronic lung diseases affect over 35 million people in the United States each year, with escalating healthcare costs of over $50 billion per year (Yelin et al., 2002; European Respiratory Society, 2017). For majority of patients, lung transplant remains the only viable treatment option for severe end-stage lung disease (Maxwell et al., 2015; Keller et al., 2019). Over 2,000 adults each year undergo lung transplant and contribute several billion dollars more in post-surgery healthcare costs (Chambers et al., 2018; Keller et al., 2019). In addition to increased survival, lung transplant can dramatically improve quality of life (Smeritschnig et al., 2005; Cohen et al., 2014). However, many challenges exist for patients after lung transplant, and despite significant advancement in clinical management strategies, the post-transplant survival continues to be lower (over 6 years), with few factors adding significant predictors of long-term risks (van der Mark et al., 2020).
Several factors, including the development of perioperative neurocognitive dysfunction (PNCD) and pre-operative comorbidities can affect post-transplant outcomes (Smith et al., 2018a). Patients with significant preoperative cognitive dysfunction and dementia are disqualified for lung transplant at most centers. PNCD encompasses cognitive impairment existing preoperatively, postoperative delirium, delayed neurocognitive disorder, and postoperative neurocognitive disorder that likely stem from multifaceted interactions between patients’ underlying comorbid susceptibilities and surgical factors (Moller et al., 1998; Vacas et al., 2013; Vandiver and Vacas, 2020). However, many centers do not routinely utilize a thorough battery of cognitive testing preoperatively to assess cognitive dysfunction, and thus, limited data are available. In addition, several comorbidities, including the diabetes mellitus (DM), hypertension, kidney disease, and sleep disordered breathing are common in adults undergoing lung transplant. Such comorbidities may enhance post-operative neurocognitive dysfunction that occur in over 50% of cases (O’keeffe and Ni Chonchubhair, 1994; Dyer et al., 1995; Abildstrom et al., 2000; Lemstra et al., 2008; Whitlock et al., 2011; Hood et al., 2018; Altay et al., 2020), but the exact underlying mechanisms remain poorly understood (Cupples and Stilley, 2005; Cohen et al., 2014; Smith et al., 2018a; Sommerwerck et al., 2021).
End stage lung disease leads to chronic hypoxic states that result in brain tissues changes, which are known to occur in other conditions with chronic hypoxia (Kumar et al., 2008, 2011, 2012, 2014; Macey et al., 2008; Pike et al., 2021; Roy et al., 2021a). Multiple brain areas are included in cognition control, including the prefrontal cortices, caudate, and hippocampus, which are involved in cognitive deficits post injury (Rubin et al., 2014; Pike et al., 2018; Roy et al., 2021a). Although the role of particular brain sites in PNCD is unclear, recent invasive and non-invasive imaging studies show compromised global gray matter volume within the postoperative period in patients that develop cognitive deficits, without determining precise regions of damage (Forsberg et al., 2017; Kant et al., 2017). While multiple reports enumerate neurologic complications after lung transplant, there is a paucity of imaging studies investigating brain tissue changes following lung transplant. Such investigation may shed light upon the mechanisms underlying cognitive dysfunction post-transplant.
To this end, MRI based diffusion tensor imaging (DTI) has been utilized to examine non-invasively regional brain neuro-inflammatory-induced brain tissue changes (Benveniste et al., 1992; Pierpaoli et al., 1993, 1996a; Matsumoto et al., 1995; Ahlhelm et al., 2002). Using DTI, several quantitative indices, including mean diffusivity (MD), can be calculated, which is commonly used to evaluate brain tissue injury (Basser and Pierpaoli, 1998; Le Bihan et al., 2001). MD measures average water diffusion within tissue (Basser et al., 1994; Pierpaoli et al., 1996b), and detects tissue pathology (Matsumoto et al., 1995; Warach et al., 1996; Ahlhelm et al., 2002; Chan et al., 2009). MD of water within tissue is influenced by the presence of tissue barriers (Le Bihan et al., 1991), and extra-cellular/extra-axonal fluid. In acute neuro-inflammation and tissue changes, neuronal and axonal swelling increase tissue barriers, reduce extracellular volume, and escalate cytotoxic edema; all factors contributing to reduced MD values (Matsumoto et al., 1995; Warach et al., 1996; Ahlhelm et al., 2002; Chan et al., 2009), as shown in animal models accompanying hypoxia (Pierpaoli et al., 1993; Tao et al., 2012). Since adults undergoing lung transplant will accompany neuro-inflammation, MD procedures may help identify PNCD and postoperative cognitive regulatory brain changes in adults with chronic lung disease.
Our study aim was to retrospectively assess the presence of PNCD and other comorbidities in an adult population that had undergone lung transplantation. We hypothesized that these patients would exhibit high levels of comorbidities and patients would reveal structural brain changes, based on magnetic resonance imaging, in cognitive regulatory areas explaining clinical findings.
Materials and methods
Using the UCLA transplant database, we examined 114 adult patients admitted between Years 2009 and 2020 for inclusion in this study. We obtained clinical, demographic, and physiologic data from all patients. Of 114 patients, we identified 9 adult patients that had undergone lung transplant and had brain MRI scans both before and after lung transplant. The MRI data for 9 subjects were downloaded, as well as demographic, medical co-morbidities, Confusion Assessment method for the ICU (CAM-ICU), documented psychiatric or mood disorder, and any documentation of cognitive dysfunction following surgery were obtained. Demographic, physiologic, and primary lung pathology information are summarized in Table 1, and the presence of delirium, documented mood disorder, and any documentation of cognitive deficits after lung transplant are tabulated in Table 2.
Magnetic resonance imaging
Brain imaging data were collected using a 1.5- or 3.0-Tesla MRI scanner (Siemens or GE). T1-weighted image series were acquired using the following parameters: repetition-time = 250–2,000 ms; echo-time = 2.43–15 ms; number of averages = 1/2; flip angle = 8–90°; matrix size = 192–313 × 256–384; field-of-view = 178–256 mm2 × 220–256 mm2; echo train length = 0/1; bandwidth = 81–320 Hz; slice thickness = 0.9–5 mm. We visually assessed T1-weighted images of all subjects for any major pathology, such as cystic lesions, infarcts, or tumors to subsequently exclude subjects, if found with any abnormality. For any head-motion related or other imaging artifacts, we critically examined T1-weighted images, and excluded the subject if any motion-related or other artifacts were found. All 9 adult patients’ MRI data before and after lung transplant were free from motion artifacts or any serious brain pathology.
Data processing and analyses
The statistical parametric mapping package (SPM12),1 MRIcroN, and MATLAB-based (The MathWorks Inc., Natick, MA, USA) custom software were used for data processing and analyses. T1-weighted images were partitioned into gray matter, white matter, and cerebrospinal fluid tissue types. The Diffeomorphic Anatomical Registration Through Exponentiated Lie algebra algorithm (DARTEL) toolbox (Ashburner, 2007) was used to generate the flow field maps, which are non-linear deformations applied for warping all the gray matter images to match each other and template images that were implemented for normalization of gray matter maps to Montreal Neurological Institute (MNI) space (voxel size: 0.46 mm3 × 0.46 mm3 × 6 mm3). The modulated and normalized gray matter maps were smoothed using a Gaussian filter, and the smoothed gray matter (GM) maps were used for further statistical analyses.
Background image
The average T1-weighted images from one control subject were normalized to MNI space. The normalized images were used as background images for structural identification.
Region-of-interest analyses
Region-of-interest (ROI) analyses were performed to calculate regional gray matter volumes to determine magnitude differences between groups. The ROI values were extracted using the regional masks of specific brain regions that appeared in whole brain analyses and smoothed gray matter maps of all subjects.
Statistical analyses
Demographics, clinical, and neuropsychologic variables
The Statistical Package for the Social Sciences (IBM SPSS, v28.0, Armonk, NY, USA) was used for assessment of demographic, physiologic, mood, cognition, and clinical variables. A p < 0.05 was considered significant.
Regional brain gray matter volume changes after lung transplant
For assessment of regional brain GM volume changes, the smoothed whole-brain gray matter maps were compared between pre- and post- lung transplant using paired t-tests (SPM12; covariate, age; uncorrected p < 0.005). Brain clusters with significant GM volume differences between pre- and post-transplant were superimposed onto the background images for structural identification.
Regional brain gray matter volumes
Regional gray matter volumes, calculated from ROI analyses, were examined for significant differences between pre- and post- lung transplant using repeated measure ANOVA (SPSS; covariates, age). A p-value of <0.05 was chosen to establish statistical significance.
Results
Demographics, clinical, and neuropsychologic variables
Demographics and clinical data of lung transplant patients were summarized in Table 1. Mean age of adults undergoing transplant was 58 years, and 58% subjects were males. Also, mean body-mass-index (BMI) was 24.2 ± 4.7 kg/m2 with lower (8%) obesity rate. Of 114 adults, 42 patients (37%) had type 2 diabetes mellitus, 70 (61%) hypertension, 51 (45%) chronic kidney disease, and 17 (15%) were diagnosed with obstructive sleep apnea (OSA). In addition, majority (58.7%) of lung transplant patients have interstitial pulmonary fibrosis, with 9.6% having a primary diagnosis of chronic obstructive pulmonary disease (COPD)/Emphysema, 5.3% hypersensitivity pneumonitis, 11.4% connective tissue disease, 4.4% Alpha-1-Anti-Trypsin disease, 3.5% Bronchiolitis Obliterans, 3.5% pulmonary arterial hypertension, and 3.5% cystic fibrosis.
Perioperative neurocognitive dysfunction and mood disorders are summarized in Table 2. Of 114 patients, delirium emerged in 50 (41%) patients, cognitive deficits were diagnosed or were worse in 28 (25%) following transplantation, and mood disorders noted in 53 (49%) adults. Severity of mood disorder was not recorded for these patients in the medical record. Delirium was assessed utilizing CAM-ICU scores, and to assess for cognitive dysfunction, we investigated the electronic medical record (EMR) for any diagnoses from the patient’s primary care provider, advanced lung disease physician (pulmonologist), psychiatrist, or formal cognitive testing which included documentation of cognitive deficits. At least 21 (18%) of the subjects had documented autonomic dysfunction, such as gastroparesis, excessive sweating, bulbar dysfunction, orthostasis, or unexplained tachycardia, although much of the data for these diagnoses are lacking from patients prior to 2015. Mood disorders were investigated mainly with diagnoses of anxiety disorder and or depression by the patient’s primary care provider, advanced lung disease physician (pulmonologist), psychiatrist, or other consultant physician.
Demographic data from adults with pre- and post- lung transplant and MRI are summarized in Table 1. Mean age of adults is 65.1 years, with 56% subjects as male, BMI 24.3 ± 4.0 kg/m2 with an average of 3.9% reduction in BMI from pre- to post-op MRI. Of 9 adults, 2 (22%) patients have type 2 diabetes mellitus, 5 (56%) hypertension, 3 (33%) chronic kidney disease, and 1 (11%) diagnosed with OSA. EMR analysis revealed delirium in 4 (44%) patients with documented cognitive deficits post-operatively in 4 (44%), and autonomic dysfunction and mood disorder in 7 (78%) patients (Table 2).
Regional brain gray matter volume changes after lung transplant
Multiple brain areas showed reduced relative regional gray matter volume after lung transplant compared to pre- lung transplant (Figure 1), and those sites included the bilateral putamen (a), bilateral insular cortices, bilateral anterior cingulate (c, h), left frontal cortex (d), para-hippocampal gyrus (e), left temporal cortex (f), right cerebellum (i), left prefrontal cortex (j), and left parietal cortex (k). Only right temporal cortex showed a slightly increased gray matter volume in post lung-transplant compared to pre- lung transplant adults. ROI analysis was performed and is shown in Figure 2 and Table 3. This includes GMV changes in mm3 with associated p-values (all <0.02). These regions of interest show decreases in GMV between pre- and post-operative MRI with varying effect sizes based on region. Given recent publications showing that BMI can affect GMV in individuals (Fernández-Andújar et al., 2021; Hidese et al., 2022; Li et al., 2022b), we performed a supplemental analysis (Supplementary Figure 1), integrating BMI changes, age, and sex as covariates. Regions identified with GMV changes were mostly unaffected, with slight decreases or increases of clusters.
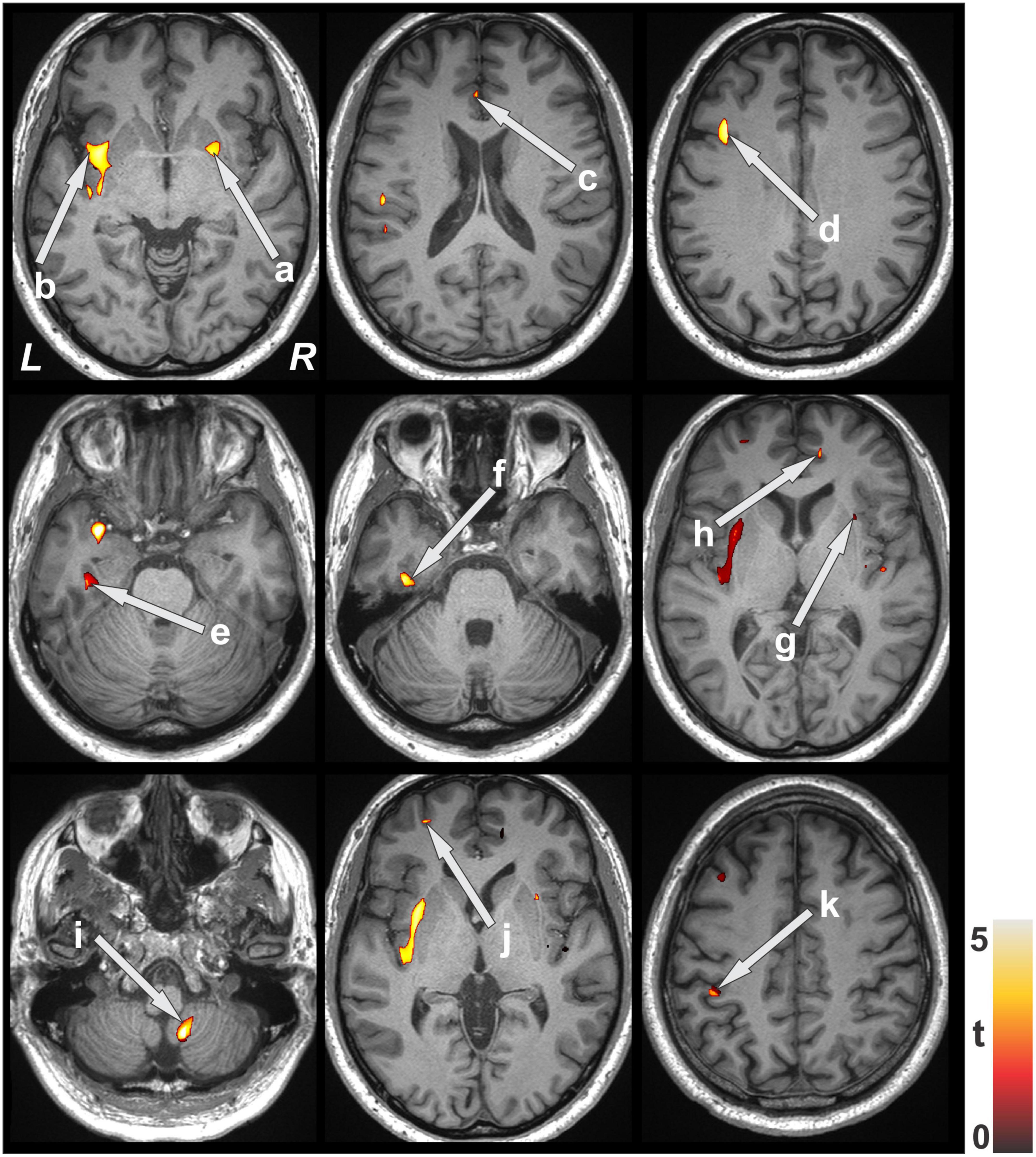
Figure 1. Brain regions with decreased gray matter volume after lung transplant over pre- lung transplant. Brain clusters with significant GM volume differences between pre- and post-transplant were superimposed onto the background image for structural identification. Sites pointed with arrows showing reduced gray matter volume included the putamen (a), insular cortices (b,g), anterior cingulate (c,h), frontal cortices (d), para-hippocampal gyrus (e), temporal cortices (f), cerebellum (i), prefrontal cortices (j) and parietal cortices (k). All images are in neurological convention (L, left; R, right). Color bar indicates t-statistic values.
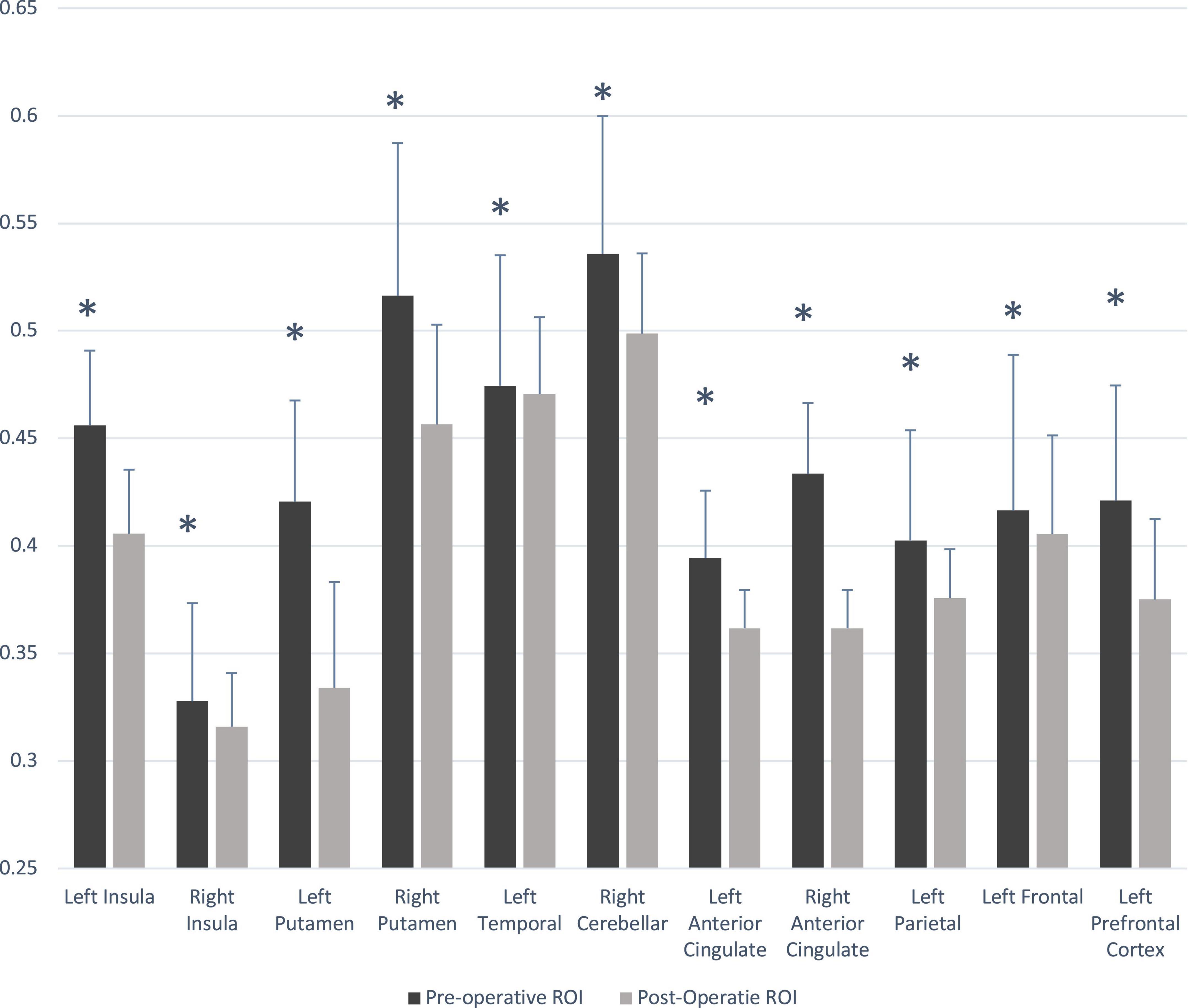
Figure 2. Gray matter volume changes in pre- and post- lung transplant patients. Significant difference between group (p < 0.05) is marked with asterisk (*). ROI, region of interest.
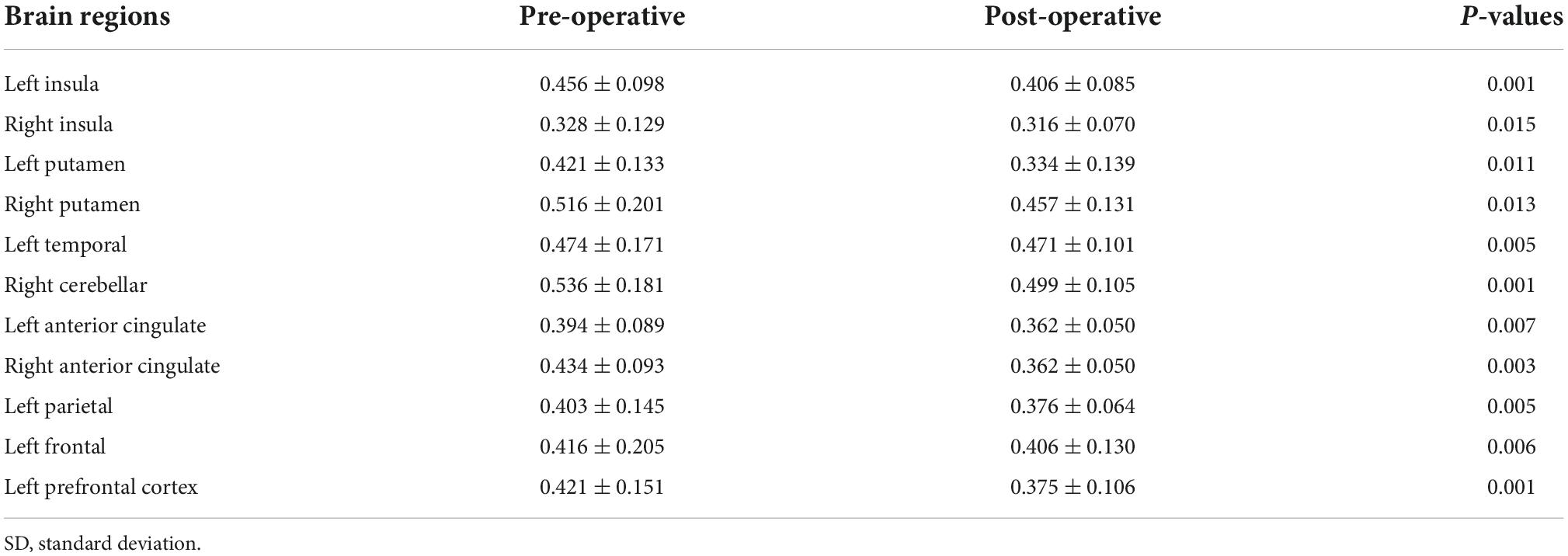
Table 3. Regional brain gray matter volume values (n = 8, mean ± SD, mm3) from pre- and post-lung transplant patients.
Discussion
In this study, preoperative lung transplant patients show multiple comorbidities, including type 2 diabetes mellitus, hypertension, chronic kidney disease, and OSA, with the primary lung pathology interstitial pulmonary fibrosis, COPD/Emphysema, hypersensitivity pneumonitis, connective tissue disease, Alpha-1-Anti-Trypsin Disease, Bronchiolitis Obliterans, pulmonary arterial hypertension, and cystic fibrosis. In addition, significant perioperative neurocognitive, mood, and autonomic dysfunction, including delirium, cognitive deficits following transplantation, and anxiety/depression were documented. Adults after lung transplant showed reduced regional gray matter volume in multiple brain areas, including the putamen, insula, anterior cingulate, frontal, parietal, and temporal cortices, para-hippocampal gyrus, and cerebellum, regions that mediate mood, autonomic, and cognitive functions, which may have contributed to postoperative functional deficits.
It should be noted that although voxel based analysis of gray matter volume changes have been used extensively to study a variety of neurologic disorders (Lavretsky et al., 2008; Popescu et al., 2015; Yankowitz et al., 2021; Li et al., 2022a; Long et al., 2022), the underlying mechanisms for these gray matter volume changes is unclear. The GMV changes have been correlated in multiple studies with cellular metrics in mice utilizing MRI, two-photon in vivo microscopy, miRNA and immunohistochemical analysis in neurodegenerative and mood disorder models of mice showing physical tissue volume, cellular number, nearest neighbor distance, nucleus volume, and a host of smaller contributors, such as spine density and plasticity (Khan et al., 2020; Asan et al., 2021). Additionally multiple studies have shown morphologic cellular changes in post mortem studies in mood and cognitive disorders (Rajkowska, 2000; Stockmeier et al., 2004; Van Otterloo et al., 2009; Rubinow et al., 2016; Rajkowska et al., 2017; Anderson et al., 2020). These studies show different patterns of cell loss, cellular atrophy (Rajkowska, 2000), length of axons (Rajkowska et al., 2017), and increased cell number in differing conditions and locations. Thus, neuronal loss secondary to hypoxia is only one hypothesis for GMV changes in this population. However, all of these possible contributors may affect cognition, and this demonstrates the need for future studies with biochemical components that may better tease out the underlying neurologic architecture changes, leading to worsening cognition. We still note, however, that GMV has been extensively associated with changes in many and disorders as described above and even in specific traits such as respect (Nakatani et al., 2020). Aging related changes in brain volume may confound studies utilizing MRI imaging (Asan et al., 2021), however, keeping the scan times close together may alleviate this potential confounder allowing for the contribution from surgery to be assessed more clearly. Additionally, other co-morbidities and even BMI (Fernández-Andújar et al., 2021; Hidese et al., 2022; Li et al., 2022b) will affect gray matter volume as we have demonstrated, which necessitates close control of patient selection and attention to changes in their overall health status before and after their lung transplant.
Post lung transplant adults are at a significantly elevated risk of cognitive dysfunction and a myriad of poor neurologic outcomes after their transplant. Previous observational studies have demonstrated deficits in cognition, including learning, memory, and executive function, all of which are vital to perform activities of daily living and the maintenance of wellness (Hoffman et al., 2012; Smith et al., 2018a,b). Several other diseases with respiratory compromise, such as cystic fibrosis, chronic obstructive pulmonary disease, obstructive sleep apnea, and asthma demonstrate altered cognition and mood (Crews et al., 2001; Dodd et al., 2010; Dodd, 2015; Roy et al., 2021b), and suggest that these disorders might be seen with even high frequency in the end-stage lung disease adults requiring lung transplant. Our study findings demonstrate a sizeable amount of PNCD in this lung transplant population, which are likely underdiagnosed, given the lack of standardized formal testing in the postoperative period. Our adults also exhibited large amounts of mood and anxiety symptoms (∼50%), as well as autonomic dysfunction. We also demonstrated that while OSA is generally similar in prevalence to the general adults (Senaratna et al., 2017), we note relatively high percentages of hypertension and chronic kidney disease, likely related to the events leading up to surgery and beyond. Although these conditions are relatively mild in the preoperative period, since severe comorbidities are disqualifying for acceptable transplantation status, the surgery induced stress, immunocompromising steroids and medications, and patients’ operative course likely contribute to new comorbidities or worsening conditions after surgery. In addition, these comorbidities also likely contribute to worse post-operative outcomes and may contribute to higher rates of PNCD, larger sample size and prospective studies would be required to identify each comorbidity contribution to overall increase in PNCD. Also, we report very low levels of obesity compared to the general population; however, this is most likely related to standard cut-offs for BMI in lung transplant and the disease process that often limits patients ability to gain weight with morbid obesity being a disqualifying factor for most transplant centers. The data suggest that this is a critical problem faced by post-lung transplant adults, as they navigate life after surgery and the desperate need to assess and address any cognitive deficits prior to their escalation to a problem easily identified by impact on daily life activities.
Here we report significantly reduced gray matter volumes in multiple brain areas after lung transplant adults, including the cerebellum, putamen, temporal, frontal, and prefrontal cortices, areas that are highly involved in cognition, mood, and autonomic functions. Injury in the prefrontal cortex has adverse implications in planning complex cognitive behavior, personality expression, decision making, and moderating social behavior (Frith and Dolan, 1996). The hippocampus, a crucial site involved in cognition, has extensive connections with the prefrontal cortex, including direct reciprocal connections between the medial prefrontal cortex and the medial temporal lobe (Rubin et al., 2014) and changes in this area have been associated with pathological states such as major depressive disorder (Stockmeier et al., 2004).
While neuronal damage and morphological changes are suggested as possible primary mechanisms in end-stage lung diseases, hypoxia and hypercapnia are also present in this patient population and likely contribute to poor outcomes through a variety of mechanisms as discussed above. While one might posit that many impaired functions, especially mood disturbances and cognitive deficits in this patient population may result from many outside aspects of a patient’s life in end-stage lung disease, our findings demonstrate a brain structural origin for their symptomatology. Multiple prior studies demonstrated that the prefrontal cortex, hippocampus, and amygdala comprise a network for mood regulation (Soares and Mann, 1997; Drevets, 2000), and in our study, part of these regions showed decreased regional gray matter volume. Anxiety and fear response are known to be affected by dopamine levels in the nucleus accumbens, a region which is regulated by the amygdala. It has been established that the insula and cingulate regions are involved in a network that regulates behavioral responses, including mood regulation, and both insula and cingulate areas are injured here.
Another region that we identified with reduced gray matter volume in adults after lung transplant was the cerebellum. Cerebellar climbing fibers are crucial for functional possesses, and tissue changes can lead to disruption in motor performance, as well as autonomic dysfunction (Miura and Reis, 1969; Hirano, 2013). In our patient population, over 40% adults showed some form of autonomic abnormality after lung transplant, which is not surprising given the impressive tissue changes post-transplant in those regulatory areas. In addition to motor and autonomic functions, the cerebellum also contributes to cognitive function via visuospatial processing and executive functioning. There are both feed-forward and feed-backward connectivity in this respect and further provides a foundation for the understanding of cognitive deficits in this patient population.
Altered gray matter regions that we discovered in our study of lung transplant adults likely play an important role in their cognition, mood, and general wellbeing. High levels of anxiety, depression, and impaired cognition can greatly affect patient management given that adherence to immunosuppression, follow-up at outpatient clinics, the ability to exercise, and general daily activities; all affect outcomes from life-saving lung transplant procedures. Disruptions in these key areas in adults will lead to increased hospitalizations, increases in healthcare costs, and significant impacts on the quality of life (Riekert et al., 2007; Smith et al., 2010; Yohannes et al., 2012; Snell et al., 2014). These findings even more strongly suggest the need for cognitive screening, and especially the prompt recognition and treatment of depression and anxiety in these adults. More thorough identification of cognitive and mood deficits will lead to improvements in outcomes in an already difficult adult patient population.
Several study limitations warrant further investigation with bigger MRI sample size before and after lung transplant data. One of the biggest limitations of our study is the relatively small sample size with MRI data, making it difficult to correct for multiple comparisons, which may affect the findings. However, an excellent mitigating factor is that the use of the same adults for pre- and post-transplant comparisons with extended clusters threshold that shows subtle and accurate tissue changes. In addition, MRI data were collected with different scanners and image resolutions, possibly also leading to type 1 error. However, all brain MRI images were bias corrected and down-sampled to one resolution before voxel-based comparisons that reduces variability. Additionally, white matter damage and small ischemic strokes can also be responsible for symptoms of depression, apathy, anxiety and executive dysfunction as a large body of literature has shown (Lavretsky et al., 2008; Mueller et al., 2010), and while many of these changes are likely reflected in structural brain changes, larger sample size and higher resolution would help in differentiating specific causes for loss of gray matter volume. We mitigated the contribution for ischemic infarcts by excluding patients with diagnosed stroke on MRI. Another significant limitation of this study includes the lack of CAM-ICU data for many participants, which were transplanted before routine documentation of CAM-ICU scores in the electronic medical record. The retrospective nature of this study also limits our ability to control for scan timing which may introduce age related changes as a confounder, although we address this within the statistical analysis it does not address the underlying mechanism for gray matter volume loss as no biochemical or pathologic sample data is available for these patients and future studies may help address this point by the addition of biomarkers of neuronal cell death or inflammation tied to brain pathology. In addition, the patient population’s lack of both universal screening and nuanced assessment of cognition in these adults likely underestimates the number of patients with cognitive deficits as we were unable to utilize Montreal cognitive assessment or other standard screening tool, given the nature of retrospective analysis. Thus more longitudinal assessments of cognition and mood evaluation would further enhance the validity of tying specific brain regions that mediate impaired functions.
Conclusion
Adults waiting for lung transplant show significantly increased comorbidities, including the diabetes mellitus, hypertension, kidney disease, OSA, and neurocognitive dysfunction before transplant. In addition, high levels of delirium, impaired cognition, and mood changes were observed in a subset of patients available for brain MRI evaluation. Adults after lung transplant showed significant brain structural changes, as evidenced by reduced gray matter volume, and these neuronal changes were localized in specific brain regions, including the frontal, prefrontal, parietal, and temporal cortices, anterior cingulate, insular, putamen, and cerebellar areas that mediate in cognition, autonomic, and mood regulation. The findings suggest a brain structural basis for neurocognitive dysfunction after lung transplant and demonstrate the need for early identification and treatment of deficits in these adults to improve outcomes.
Data availability statement
The data analyzed in this study is subject to the following licenses/restrictions: The datasets analyzed during the current study are not publicly available since it contains patient’s personal health information but are available from the corresponding author on reasonable request. Requests to access these datasets should be directed to RK, cmt1bWFyQG1lZG5ldC51Y2xhLmVkdQ==.
Ethics statement
The studies involving human participants were reviewed and approved by Institutional Review Board of University of California, Los Angeles. Written informed consent for participation was not required for this study in accordance with the national legislation and the institutional requirements.
Author contributions
MV: conception, data acquisition, analysis, interpretation of data, and drafted the work. BR: analysis, interpretation of data, and substantively revised the initial draft. FM: data acquisition and analysis. HL: interpretation of data and substantively revised it. RK: conception, data acquisition, interpretation of data, and substantively revised the initial draft. All authors read and approved the final manuscript.
Funding
MV was supported by UCLA Department of Anesthesiology Seed Grant. RK and BR were supported by the National Institutes of Health R21HD102544 and R01 NR017190.
Acknowledgments
We thank Stephanie Fraschiilla for her help in obtaining the UCLA Lung Transplant Database data and Harshika Chowdhary for her help in downloading MRI images for analysis.
Conflict of interest
The authors declare that the research was conducted in the absence of any commercial or financial relationships that could be construed as a potential conflict of interest.
Publisher’s note
All claims expressed in this article are solely those of the authors and do not necessarily represent those of their affiliated organizations, or those of the publisher, the editors and the reviewers. Any product that may be evaluated in this article, or claim that may be made by its manufacturer, is not guaranteed or endorsed by the publisher.
Supplementary material
The Supplementary Material for this article can be found online at: https://www.frontiersin.org/articles/10.3389/fncel.2022.1015568/full#supplementary-material
Abbreviations
BMI, body-mass-index; DM, diabetes mellitus; CAM-ICU, confusion assessment method for the ICU; COPD, chronic obstructive pulmonary disease; EMR, electronic medical record; MNI, Montreal Neurological Institute; PNCD, perioperative neurocognitive dysfunction; SPM12, statistical parametric mapping package 12; SPSS, statistical package for the social sciences; OSA, obstructive sleep apnea.
Footnotes
References
Abildstrom, H., Rasmussen, L. S., Rentowl, P., Hanning, C. D., Rasmussen, H., Kristensen, P. A., et al. (2000). Cognitive dysfunction 1-2 years after non-cardiac surgery in the elderly. ISPOCD group. International study of post-operative cognitive dysfunction. Acta Anaesthesiol. Scand. 44, 1246–1251. doi: 10.1034/j.1399-6576.2000.441010.x
Ahlhelm, F., Schneider, G., Backens, M., Reith, W., and Hagen, T. (2002). Time course of the apparent diffusion coefficient after cerebral infarction. Eur. Radiol. 12, 2322–2329.
Altay, S., Gurdogan, M., Kaya, C., Kardas, F., Zeybey, U., Cakir, B., et al. (2020). The etiology and age-related properties of patients with delirium in coronary intensive care unit and its effects on inhospital and follow up prognosis. Ideggyogy. Sz. 73, 189–197. doi: 10.18071/isz.73.0189
Anderson, K. M., Collins, M. A., Kong, R., Fang, K., Li, J., He, T., et al. (2020). Convergent molecular, cellular, and cortical neuroimaging signatures of major depressive disorder. Proc. Natl. Acad. Sci. U.S.A. 117, 25138–25149. doi: 10.1073/pnas.2008004117
Asan, L., Falfán-Melgoza, C., Beretta, C. A., Sack, M., Zheng, L., Weber-Fahr, W., et al. (2021). Cellular correlates of gray matter volume changes in magnetic resonance morphometry identified by two-photon microscopy. Sci. Rep. 11:4234. doi: 10.1038/s41598-021-83491-8
Basser, P. J., and Pierpaoli, C. (1998). A simplified method to measure the diffusion tensor from seven MR images. Magn. Reson. Med. 39, 928–934. doi: 10.1002/mrm.1910390610
Basser, P. J., Mattiello, J., and Lebihan, D. (1994). Estimation of the effective self-diffusion tensor from the NMR spin echo. J. Magn. Reson. B 103, 247–254. doi: 10.1006/jmrb.1994.1037
Benveniste, H., Hedlund, L. W., and Johnson, G. A. (1992). Mechanism of detection of acute cerebral ischemia in rats by diffusion-weighted magnetic resonance microscopy. Stroke 23, 746–754. doi: 10.1161/01.str.23.5.746
Chambers, D. C., Cherikh, W. S., Goldfarb, S. B., Hayes, D. Jr., Kucheryavaya, A. Y., Toll, A. E., et al. (2018). The international thoracic organ transplant registry of the international society for heart and lung transplantation: Thirty-fifth adult lung and heart-lung transplant report-2018; Focus theme: Multiorgan transplantation. J. Heart Lung Transplant. 37, 1169–1183.
Chan, K. C., Khong, P. L., Lau, H. F., Cheung, P. T., and Wu, E. X. (2009). Late measures of microstructural alterations in severe neonatal hypoxic-ischemic encephalopathy by MR diffusion tensor imaging. Int. J. Dev. Neurosci. 27, 607–615. doi: 10.1016/j.ijdevneu.2009.05.012
Cohen, D. G., Christie, J. D., Anderson, B. J., Diamond, J. M., Judy, R. P., Shah, R. J., et al. (2014). Cognitive function, mental health, and health-related quality of life after lung transplantation. Ann. Am. Thorac. Soc. 11, 522–530.
Crews, W. D., Jefferson, A. L., Bolduc, T., Elliott, J. B., Ferro, N. M., Broshek, D. K., et al. (2001). Neuropsychological dysfunction in patients suffering from end-stage chronic obstructive pulmonary disease. Arch. Clin. Neuropsychol. 16, 643–652.
Cupples, S. A., and Stilley, C. S. (2005). Cognitive function in adult cardiothoracic transplant candidates and recipients. J. Cardiovasc. Nurs. 20, S74–S87. doi: 10.1097/00005082-200509001-00009
Dodd, J. W. (2015). Lung disease as a determinant of cognitive decline and dementia. Alzheimers Res. Ther. 7:32.
Dodd, J. W., Getov, S. V., and Jones, P. W. (2010). Cognitive function in COPD. Eur. Respir. J. 35, 913–922.
Drevets, W. C. (2000). Functional anatomical abnormalities in limbic and prefrontal cortical structures in major depression. Prog. Brain Res. 126, 413–431.
Dyer, C. B., Ashton, C. M., and Teasdale, T. A. (1995). Postoperative delirium. A review of 80 primary data-collection studies. Arch. Intern. Med. 155, 461–465.
European Respiratory Society (2017). “The global impact of respiratory disease,” in Forum of international respiratory societies (Lausanne: European Respiratory Society).
Fernández-Andújar, M., Morales-García, E., and García-Casares, N. (2021). Obesity and gray matter volume assessed by neuroimaging: A systematic review. Brain Sci. 11:999.
Forsberg, A., Cervenka, S., Jonsson Fagerlund, M., Rasmussen, L. S., Zetterberg, H., Erlandsson Harris, H., et al. (2017). The immune response of the human brain to abdominal surgery. Ann. Neurol. 81, 572–582.
Frith, C., and Dolan, R. (1996). The role of the prefrontal cortex in higher cognitive functions. Brain Res. Cogn. Brain Res. 5, 175–181.
Hidese, S., Ota, M., Matsuo, J., Ishida, I., Yokota, Y., Hattori, K., et al. (2022). Association of body mass index and its classifications with gray matter volume in individuals with a wide range of body mass index group: A whole-brain magnetic resonance imaging study. Front. Hum. Neurosci. 16:926804. doi: 10.3389/fnhum.2022.926804
Hirano, T. (2013). Long-term depression and other synaptic plasticity in the cerebellum. Proc. Jpn. Acad. Ser. B Phys. Biol. Sci. 89, 183–195.
Hoffman, B. M., Blumenthal, J. A., Carney, R. C., O’hayer, C. V. F., Freedland, K., Smith, P. J., et al. (2012). Changes in neurocognitive functioning following lung transplantation. Am. J. Transplantat. 12, 2519–2525.
Hood, R., Budd, A., Sorond, F. A., and Hogue, C. W. (2018). Peri-operative neurological complications. Anaesthesia 73 Suppl 1, 67–75.
Kant, I. M. J., De Bresser, J., Van Montfort, S. J. T., Slooter, A. J. C., and Hendrikse, J. (2017). MRI markers of neurodegenerative and neurovascular changes in relation to postoperative delirium and postoperative cognitive decline. Am. J. Geriatr. Psychiatry 25, 1048–1061. doi: 10.1016/j.jagp.2017.06.016
Keller, C. A., Gonwa, T. A., White, L. J., Rucci, M. E., Visscher, S. L., Kennedy, C. C., et al. (2019). Utilization and cost analysis of lung transplantation and survival after 10 years of adapting the lung allocation score. Transplantation 103, 638–646.
Khan, A. R., Geiger, L., Wiborg, O., and Czéh, B. (2020). Stress-induced morphological, cellular and molecular changes in the brain-lessons learned from the chronic mild stress model of depression. Cells 9:1026. doi: 10.3390/cells9041026
Kumar, R., Chavez, A. S., Macey, P. M., Woo, M. A., Yan-Go, F. L., and Harper, R. M. (2012). Altered global and regional brain mean diffusivity in patients with obstructive sleep apnea. J. Neurosci. Res. 90, 2043–2052.
Kumar, R., Farahvar, S., Ogren, J. A., Macey, P. M., Thompson, P. M., Woo, M. A., et al. (2014). Brain putamen volume changes in newly-diagnosed patients with obstructive sleep apnea. Neuroimage Clin. 4, 383–391. doi: 10.1016/j.nicl.2014.01.009
Kumar, R., Macey, P. M., Woo, M. A., Alger, J. R., and Harper, R. M. (2008). Diffusion tensor imaging demonstrates brainstem and cerebellar abnormalities in congenital central hypoventilation syndrome. Pediatr. Res. 64, 275–280. doi: 10.1203/PDR.0b013e31817da10a
Kumar, R., Nguyen, H. D., Ogren, J. A., Macey, P. M., Thompson, P. M., Fonarow, G. C., et al. (2011). Global and regional putamen volume loss in patients with heart failure. Eur. J. Heart Fail 13, 651–655. doi: 10.1093/eurjhf/hfr012
Lavretsky, H., Zheng, L., Weiner, M. W., Mungas, D., Reed, B., Kramer, J. H., et al. (2008). The MRI brain correlates of depressed mood, anhedonia, apathy, and anergia in older adults with and without cognitive impairment or dementia. Int. J. Geriatr. Psychiatry 23, 1040–1050.
Le Bihan, D., Mangin, J. F., Poupon, C., Clark, C. A., Pappata, S., Molko, N., et al. (2001). Diffusion tensor imaging: Concepts and applications. J. Magn. Reson. Imaging 13, 534–546. doi: 10.1002/jmri.1076
Le Bihan, D., Moonen, C. T., Van Zijl, P. C., Pekar, J., and Despres, D. (1991). Measuring random microscopic motion of water in tissues with MR imaging: A cat brain study. J. Comput. Assist. Tomogr. 15, 19–25. doi: 10.1097/00004728-199101000-00002
Lemstra, A. W., Kalisvaart, K. J., Vreeswijk, R., Van Gool, W. A., and Eikelenboom, P. (2008). Pre-operative inflammatory markers and the risk of postoperative delirium in elderly patients. Int. J. Geriatr. Psychiatry 23, 943–948.
Li, L., Ji, B., Zhao, T., Cui, X., Chen, J., and Wang, Z. (2022a). The structural changes of gray matter in Parkinson disease patients with mild cognitive impairments. PLoS One 17:e0269787. doi: 10.1371/journal.pone.0269787
Li, L., Yu, H., Zhong, M., Liu, S., Wei, W., Meng, Y., et al. (2022b). Gray matter volume alterations in subjects with overweight and obesity: Evidence from a voxel-based meta-analysis. Front. Psychiatry 13:955741. doi: 10.3389/fpsyt.2022.955741
Long, Q., Lv, Z., Zhao, J., Shi, K., Li, C., Fan, B., et al. (2022). Cerebral gray matter volume changes in patients with anti-N-methyl-D-aspartate receptor encephalitis: A voxel-based morphometry study. Front. Neurol. 13:892242. doi: 10.3389/fneur.2022.892242
Macey, P. M., Kumar, R., Woo, M. A., Valladares, E. M., Yan-Go, F. L., and Harper, R. M. (2008). Brain structural changes in obstructive sleep apnea. Sleep 31, 967–977.
Matsumoto, K., Lo, E. H., Pierce, A. R., Wei, H., Garrido, L., and Kowall, N. W. (1995). Role of vasogenic edema and tissue cavitation in ischemic evolution on diffusion-weighted imaging: Comparison with multiparameter MR and immunohistochemistry. AJNR Am. J. Neuroradiol. 16, 1107–1115.
Maxwell, B. G., Mooney, J. J., Lee, P. H., Levitt, J. E., Chhatwani, L., Nicolls, M. R., et al. (2015). Increased resource use in lung transplant admissions in the lung allocation score era. Am. J. Respir. Crit. Care Med. 191, 302–308.
Miura, M., and Reis, D. J. (1969). Cerebellum: A pressor response elicited from the fastigial nucleus and its efferent pathway in brainstem. Brain Res. 13, 595–599. doi: 10.1016/0006-8993(69)90269-8
Moller, J. T., Cluitmans, P., Rasmussen, L. S., Houx, P., Rasmussen, H., Canet, J., et al. (1998). Long-term postoperative cognitive dysfunction in the elderly ISPOCD1 study. ISPOCD investigators. International study of post-operative cognitive dysfunction. Lancet 351, 857–861. doi: 10.1016/s0140-6736(97)07382-0
Mueller, S. G., Mack, W. J., Mungas, D., Kramer, J. H., Cardenas-Nicolson, V., Lavretsky, H., et al. (2010). Influences of lobar gray matter and white matter lesion load on cognition and mood. Psychiatry Res. 181, 90–96. doi: 10.1016/j.pscychresns.2009.08.002
Nakatani, H., Nonaka, Y., Muto, S., Asano, M., Fujimura, T., Nakai, T., et al. (2020). Trait respect is linked to reduced gray matter volume in the anterior temporal lobe. Front. Hum. Neurosci. 14:344. doi: 10.3389/fnhum.2020.00344
O’keeffe, S. T., and Ni Chonchubhair, A. (1994). Postoperative delirium in the elderly. Br. J. Anaesth. 73, 673–687. doi: 10.1093/bja/73.5.673
Pierpaoli, C., Alger, J. R., Righini, A., Mattiello, J., Dickerson, R., Des Pres, D., et al. (1996a). High temporal resolution diffusion MRI of global cerebral ischemia and reperfusion. J. Cereb. Blood Flow Metab. 16, 892–905. doi: 10.1097/00004647-199609000-00013
Pierpaoli, C., Jezzard, P., Basser, P. J., Barnett, A., and Di Chiro, G. (1996b). Diffusion tensor MR imaging of the human brain. Radiology 201, 637–648. doi: 10.1148/radiology.201.3.8939209
Pierpaoli, C., Righini, A., Linfante, I., Tao-Cheng, J. H., Alger, J. R., and Di Chiro, G. (1993). Histopathologic correlates of abnormal water diffusion in cerebral ischemia: Diffusion-weighted MR imaging and light and electron microscopic study. Radiology 189, 439–448. doi: 10.1148/radiology.189.2.8210373
Pike, N. A., Roy, B., Gupta, R., Singh, S., Woo, M. A., Halnon, N. J., et al. (2018). Brain abnormalities in cognition, anxiety, and depression regulatory regions in adolescents with single ventricle heart disease. J. Neurosci. Res. 96, 1104–1118. doi: 10.1002/jnr.24215
Pike, N. A., Roy, B., Moye, S., Cabrera-Mino, C., Woo, M. A., Halnon, N. J., et al. (2021). Reduced hippocampal volumes and memory deficits in adolescents with single ventricle heart disease. Brain Behav. 11:e01977. doi: 10.1002/brb3.1977
Popescu, V., Klaver, R., Voorn, P., Galis-De Graaf, Y., Knol, D. L., Twisk, J. W., et al. (2015). What drives MRI-measured cortical atrophy in multiple sclerosis? Mult. Scler. 21, 1280–1290. doi: 10.1177/1352458514562440
Rajkowska, G. (2000). Postmortem studies in mood disorders indicate altered numbers of neurons and glial cells. Biol. Psychiatry 48, 766–777. doi: 10.1016/s0006-3223(00)00950-1
Rajkowska, G., Mahajan, G., Legutko, B., Challagundla, L., Griswold, M., Albert, P. R., et al. (2017). Length of axons expressing the serotonin transporter in orbitofrontal cortex is lower with age in depression. Neuroscience 359, 30–39. doi: 10.1016/j.neuroscience.2017.07.006
Riekert, K. A., Bartlett, S. J., Boyle, M. P., Krishnan, J. A., and Rand, C. S. (2007). The association between depression, lung function, and health-related quality of life among adults with cystic fibrosis. Chest 132, 231–237. doi: 10.1378/chest.06-2474
Roy, B., Vacas, S., Ehlert, L., Mccloy, K., Saggar, R., and Kumar, R. (2021a). Brain structural changes in patients with pulmonary arterial hypertension. J. Neuroimaging 31, 524–531. doi: 10.1111/jon.12840
Roy, B., Woo, M. S., Vacas, S., Eshaghian, P., Rao, A. P., and Kumar, R. (2021b). Regional brain tissue changes in patients with cystic fibrosis. J. Transl. Med. 19, 419. doi: 10.1186/s12967-021-03092-x
Rubin, R. D., Watson, P. D., Duff, M. C., and Cohen, N. J. (2014). The role of the hippocampus in flexible cognition and social behavior. Front. Hum. Neurosci. 8:742. doi: 10.3389/fnhum.2014.00742
Rubinow, M. J., Mahajan, G., May, W., Overholser, J. C., Jurjus, G. J., Dieter, L., et al. (2016). Basolateral amygdala volume and cell numbers in major depressive disorder: A postmortem stereological study. Brain Struct. Funct. 221, 171–184. doi: 10.1007/s00429-014-0900-z
Senaratna, C. V., Perret, J. L., Lodge, C. J., Lowe, A. J., Campbell, B. E., Matheson, M. C., et al. (2017). Prevalence of obstructive sleep apnea in the general population: A systematic review. Sleep Med. Rev. 34, 70–81. doi: 10.1016/j.smrv.2016.07.002
Smeritschnig, B., Jaksch, P., Kocher, A., Seebacher, G., Aigner, C., Mazhar, S., et al. (2005). Quality of life after lung transplantation: A cross-sectional study. J. Heart Lung Transplant. 24, 474–480. doi: 10.1016/j.healun.2003.12.013
Smith, B. A., Modi, A. C., Quittner, A. L., and Wood, B. L. (2010). Depressive symptoms in children with cystic fibrosis and parents and its effects on adherence to airway clearance. Pediatr. Pulmonol. 45, 756–763. doi: 10.1002/ppul.21238
Smith, P. J., Blumenthal, J. A., Hoffman, B. M., Davis, R. D., and Palmer, S. M. (2018a). Postoperative cognitive dysfunction and mortality following lung transplantation. Am. J. Transplant. 18, 696–703. doi: 10.1111/ajt.14570
Smith, P. J., Stonerock, G. L., Ingle, K. K., Saulino, C. K., Hoffman, B., Wasserman, B., et al. (2018b). Neurological sequelae and clinical outcomes after lung transplantation. Transplant. Direct 4:e353. doi: 10.1097/TXD.0000000000000766
Snell, C., Fernandes, S., Bujoreanu, I. S., and Garcia, G. (2014). Depression, illness severity, and healthcare utilization in cystic fibrosis. Pediatr. Pulmonol. 49, 1177–1181. doi: 10.1002/ppul.22990
Soares, J. C., and Mann, J. J. (1997). The anatomy of mood disorders–review of structural neuroimaging studies. Biol. Psychiatry 41, 86–106. doi: 10.1016/s0006-3223(96)00006-6
Sommerwerck, U., Jokisch, D., Weinreich, G., Neurath, M., Heinze, C., Bessa, V., et al. (2021). Cognitive function after lung transplantation. Adv. Exp. Med. Biol. 1324, 91–101. doi: 10.1007/5584_2020_590
Stockmeier, C. A., Mahajan, G. J., Konick, L. C., Overholser, J. C., Jurjus, G. J., Meltzer, H. Y., et al. (2004). Cellular changes in the postmortem hippocampus in major depression. Biol. Psychiatry 56, 640–650. doi: 10.1016/j.biopsych.2004.08.022
Tao, J. D., Barnette, A. R., Griffith, J. L., Neil, J. J., and Inder, T. E. (2012). Histopathologic correlation with diffusion tensor imaging after chronic hypoxia in the immature ferret. Pediatr. Res. 71, 192–198. doi: 10.1038/pr.2011.32
Vacas, S., Degos, V., Feng, X., and Maze, M. (2013). The neuroinflammatory response of postoperative cognitive decline. Br. Med. Bull. 106, 161–178. doi: 10.1093/bmb/ldt006
van der Mark, S. C., Hoek, R. A. S., and Hellemons, M. E. (2020). Developments in lung transplantation over the past decade. Eur. Respir. Rev. 29:190132. doi: 10.1183/16000617.0132-2019
Van Otterloo, E., O’dwyer, G., Stockmeier, C. A., Steffens, D. C., Krishnan, R. R., and Rajkowska, G. (2009). Reductions in neuronal density in elderly depressed are region specific. Int. J. Geriatr. Psychiatry 24, 856–864. doi: 10.1002/gps.2281
Vandiver, M. S., and Vacas, S. (2020). Interventions to improve perioperative neurologic outcomes. Curr. Opin. Anaesthesiol. 33, 661–667. doi: 10.1097/ACO.0000000000000905
Warach, S., Mosley, M., Sorensen, A. G., and Koroshetz, W. (1996). Time course of diffusion imaging abnormalities in human stroke. Stroke 27, 1254–1256.
Whitlock, E. L., Vannucci, A., and Avidan, M. S. (2011). Postoperative delirium. Minerva Anestesiol. 77, 448–456.
Yankowitz, L. D., Yerys, B. E., Herrington, J. D., Pandey, J., and Schultz, R. T. (2021). Dissociating regional gray matter density and gray matter volume in autism spectrum condition. Neuroimage Clin. 32:102888. doi: 10.1016/j.nicl.2021.102888
Yelin, E., Trupin, L., Cisternas, M., Eisner, M., Katz, P., and Blanc, P. (2002). A national study of medical care expenditures for respiratory conditions. Eur. Respir. J. 19, 414–421. doi: 10.1183/09031936.02.00522001
Keywords: perioperative neurocognitive dysfunction, depression, anxiety, delirium, magnetic resonance imaging
Citation: Vandiver MS, Roy B, Mahmud F, Lavretsky H and Kumar R (2022) Functional comorbidities and brain tissue changes before and after lung transplant in adults. Front. Cell. Neurosci. 16:1015568. doi: 10.3389/fncel.2022.1015568
Received: 09 August 2022; Accepted: 17 November 2022;
Published: 02 December 2022.
Edited by:
Eleonora Napoli, University of California, Davis, United StatesReviewed by:
Phan Q. Duy, Yale University, United StatesMbemba Jabbi, The University of Texas at Austin, United States
Copyright © 2022 Vandiver, Roy, Mahmud, Lavretsky and Kumar. This is an open-access article distributed under the terms of the Creative Commons Attribution License (CC BY). The use, distribution or reproduction in other forums is permitted, provided the original author(s) and the copyright owner(s) are credited and that the original publication in this journal is cited, in accordance with accepted academic practice. No use, distribution or reproduction is permitted which does not comply with these terms.
*Correspondence: Rajesh Kumar, cmt1bWFyQG1lZG5ldC51Y2xhLmVkdQ==