- 1Department of Cell Biology, Duke University School of Medicine, Durham, NC, United States
- 2Department of Anesthesiology, Duke University School of Medicine, Durham, NC, United States
- 3Center for Cell and Gene Therapy, Baylor College of Medicine, Houston, TX, United States
- 4Department of Neuroscience, Baylor College of Medicine, Houston, TX, United States
- 5Department of Neurosurgery, Baylor College of Medicine, Houston, TX, United States
Under normal conditions, neural stem cells (NSCs or B cells) in the adult subventricular zone (SVZ) give rise to amplifying neural progenitor cells (NPCs or C cells), which can produce neuroblasts (or A cells) that migrate to the olfactory bulb and differentiate into new neurons. However, following brain injury, these cells migrate toward the injury site where they differentiate into astrocytes and oligodendrocytes. In this review, we will focus on recent findings that chronicle how astrocytes and oligodendrocytes derived from SVZ-NSCs respond to different types of injury. We will also discuss molecular regulators of SVZ-NSC proliferation and their differentiation into astrocytes and oligodendrocytes. Overall, the goal of this review is to highlight how SVZ-NSCs respond to injury and to summarize the regulatory mechanisms that oversee their glial response. These molecular and cellular processes will provide critical insights needed to develop strategies to promote brain repair following injury using SVZ-NSCs.
Introduction
In recent years, research has begun to unravel complex biological cascades that follows injury to the brain: astroglial activation and proliferation (Pekny et al., 2016, 2019), parenchymal inflammation and infiltration of immune cells (Liesz et al., 2015; Gill and Veltkamp, 2016; Sofroniew, 2020), glial scar formation (Burda et al., 2016), revascularization/re-establishment of blood-brain barrier (Li et al., 2021), remodeling of connections between surviving neurons (Jones, 2017) and replenishment of oligodendrocytes following demyelination injury (Nait-Oumesmar et al., 2007; Butti et al., 2019).
Research in this area has revealed new methods to improve and augment these responses after injury. One potentially exciting strategy is to enhance NSC proliferation in the postnatal/adult brain. During embryogenesis these NSC populations are relatively abundant, however, in the adult they become restricted to specialized regions/niches in the brain. Specifically, NSCs reside in the SVZ along the lateral walls of lateral brain ventricles (Ihrie and Álvarez-Buylla, 2011) and the subgranular zone (SGZ) of the hippocampal dentate gyrus (Bonaguidi et al., 2012). In the SVZ, NSCs (B cells) are located in the walls of the ventricular-SVZ and give rise to transit amplifying cells (NPC or C cells), which can produce neuroblasts (A cells) (Altman, 1962). Under physiological conditions, neuroblasts migrate a long-distance (3–8 mm in mice) from the SVZ to the olfactory bulb (Lois and Alvarez-Buylla, 1994; Lois et al., 1996), where they differentiate into granule neurons (Grelat et al., 2018; Li et al., 2018). Following injury such as ischemic stroke, neuroblast migration can be redirected from the rostral migratory stream (RMS)-olfactory bulb toward the site of injury (for review see Chang et al., 2016), suggesting that endogenous injury responses can mobilize NSCs from the SVZ niche.
In addition to neurons, NSCs also make astrocytes and oligodendrocytes. Several groups reported that following stroke, cells coming from the SVZ are predominantly glial instead of neuronal (Givogri et al., 2006; Li et al., 2010) and that these cells play a major role in glial scar formation following injury (Benner et al., 2013). The SVZ also plays a role in myelin repair and oligodendrocyte formation. After white matter injury, the number of oligodendrocytes derived from SVZ increases in the corpus callosum (CC) (Nait-Oumesmar et al., 1999; Picard-Riera et al., 2002; Menn et al., 2006).
Neurogenesis is a cardinal feature of SVZ-NSCs under homeostatic conditions, however after injury the differentiation programs of these SVZ-NSCs can be redirected toward the generation of glial cells (astrocytes and oligodendrocytes) (Figure 1). Therefore, in this review we will describe SVZ-glia contribution following different forms of brain injury, and discuss the factors regulating their function with the goal of identifying areas of potential therapeutic interest.
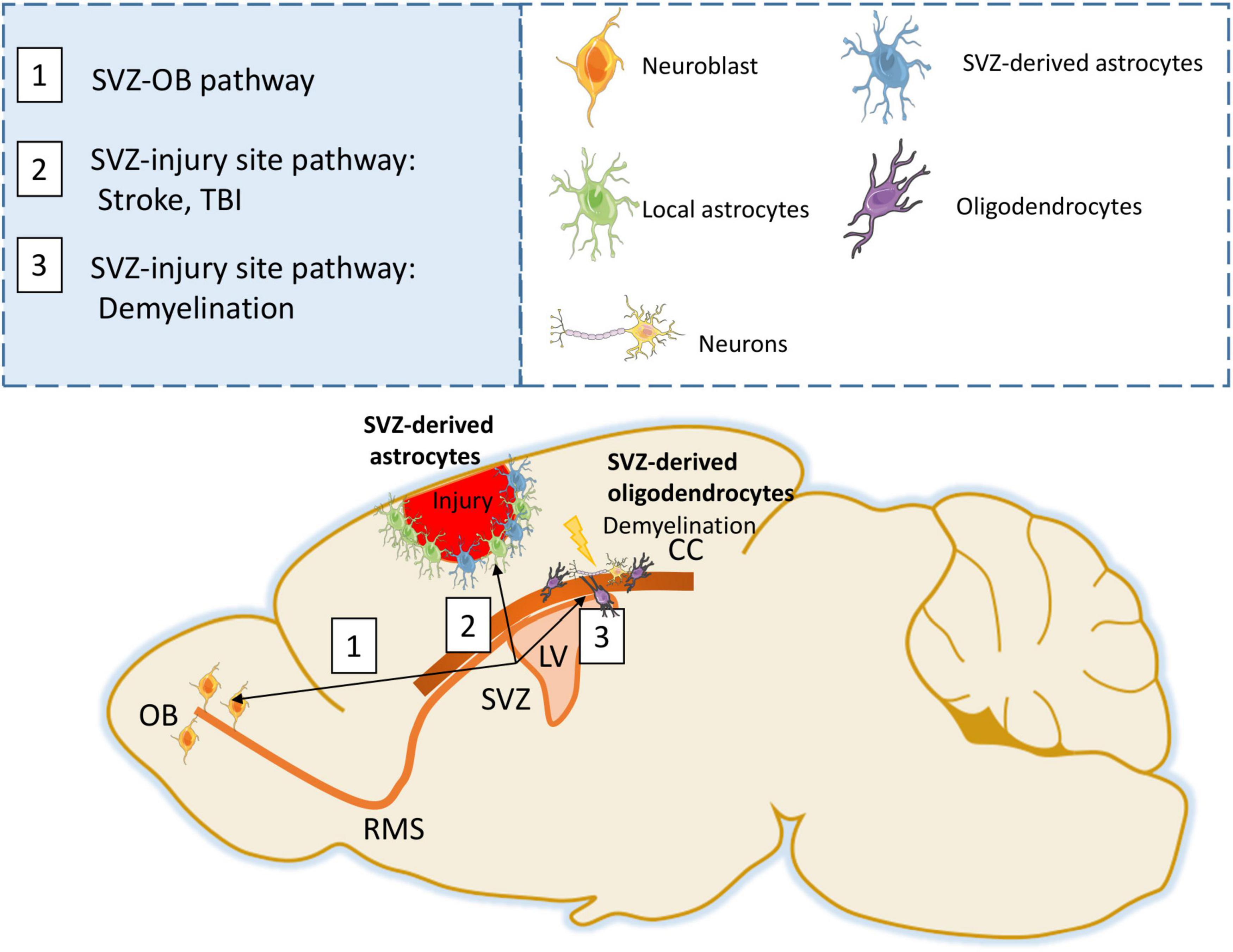
Figure 1. SVZ response following injury. Under physiological conditions, SVZ progenitors generate neuroblasts that migrate through the RMS to the Olfactory bulb (OB) (Path 1). Following stroke or TBI, SVZ progenitors are able to generate astrocytes that migrate to the injury site forming a glial scar (Path 2). Following demyelination, SVZ progenitors can differentiate into oligodendrocytes and participate in remyelination (Path 3).
Subventricular Zone-Derived Astrocytes Response to Injury
Under normal physiological conditions, the SVZ can generate mature astrocytes (Sohn et al., 2015) and following injury, the number of astrocytes produced by the SVZ drastically increases. Lineage tracing with the Nestin-CreERT2 promoter has been used to directly identify SVZ-derived progenitors migrating to the injury site, with the Nestin-CreERT2:R26R-YFP/RFP traced cells predominately expressing the astrocytic marker GFAP within the injured striatal parenchyma after middle cerebral artery occlusion (MCAO) (Li et al., 2010), in the cortex after injury (PBS filling brain cavity) (Wang et al., 2019), cortical stroke (Benner et al., 2013; Faiz et al., 2015) or stab-wound injury (Burns et al., 2009). These studies reveal that the SVZ produces a more predominant astrocytic component than previously appreciated.
These lineage tracing experiments not only provide strong evidence of SVZ-derived astrocytes migrating to sites of injury, but also demonstrate that several types of injury can elicit this response. Additional injuries that also elicit astrocyte differentiation responses from SVZ-NSCs include: motor cortex lesion (Saha et al., 2013), cortical stab wound injury (Givogri et al., 2006), aspiration of the left frontoparietal cerebral (Goings et al., 2004), cortical contusion injury (Radomski et al., 2013), controlled cortical impact injury (Kernie et al., 2001), and puncture wound (Mundim et al., 2019). Interestingly, clonal studies also revealed that astrocytes from the SVZ migrate to the injury site following fine needle injury (Martín-López et al., 2013).
In addition to the cortex, there is evidence that multiple types of SVZ cells migrate to the striatum after injury. SVZ cells migrate toward the striatum in the 6-hydroxydopamine model of Parkinson’s disease, illustrating their migration in neurodegenerative diseases as well as injury. Using Nestin-CreERT2, striatal TGFα infusion recruits a large population of SVZ-derived multipotent “C-like” cells to the DA-depleted striatum (De Chevigny et al., 2008). Transient migration of PSA-NCAM/Bromodeoxyuridine (BrdU)-positive cells from the SVZ to the striatum also has been described in the 6-hydroxydopamine implying that newborn cells are capable of migrating into the dopamine deprived striatum (Liu et al., 2006). Another study using BrdU reported that SVZ progenitors proliferate following 6-hydroxydopamine-lesioned striatum after intraventricular injection. Although they report that BrdU + cells co-expressing the astrocytic marker GFAP are widely distributed throughout the lesioned striatum, the authors did not attribute astrogenesis to SVZ progenitors (Aponso et al., 2008).
The type of cells migrating to the injury site can depend on timing post-injury (i.e., short- vs. long- term responses), the type of injury (mechanical vs. chemical), and brain location (proximal or distal from the SVZ). For instance, following stroke or traumatic brain injury (TBI), NPCs migrate toward the injury site to form astrocytes. After these injuries, newly formed neurons are less abundant and do not integrate well, suggesting that NPCs differentiation depends on local environment cues. Additional lineage tracing studies that accounts for timing, location, and cellular diversity (see below) are needed to further investigate how spatial-temporal factors influence SVZ-progenitor fate decisions responses to various forms of injuries. Interestingly, one in vitro study showed that murine astrocytes isolated from different brain regions (hippocampus, striatum and cortex) had differential sensitivity to injury (Xu et al., 2001).
It has become increasingly clear that CNS astrocytes represent a diverse cell population (Chai et al., 2017; John Lin et al., 2017; Morel et al., 2017) and it is possible that different classes of astrocytes have distinct functions during tissue recovery after injury. However, whether SVZ-generated astrocytes migrating to the injury site represent a distinct subset of astrocytes remains unclear, and determining if parenchymal astrocytes and SVZ-derived astrocytes have different functions in brain repair is of interest, as it remains poorly defined. Finally, understanding if modulation of these astrocyte populations following injury can stimulate repair may reveal therapeutic targets to improve outcomes after brain injury.
Molecular Regulators of Subventricular Zone-Derived Astrocytes
Recent studies have defined a molecular framework for developmental astrogenesis that includes the following signaling pathways and transcription factors: Notch, BMP, NFIA, STAT3, Sox9, and LIF (for review Molofsky et al., 2012; Sloan and Barres, 2014; Akdemir et al., 2020). Therefore, in this review, we will discuss the role of these developmental regulators in SVZ- astrogliogenesis with a focus on factors that positively regulate astrogliogenesis in the SVZ in the context of injury (Table 1).
Notch Pathway
Thrombospondin 4
Localized photothrombotic/ischemic cortical injury initiates a marked increase in Thrombospondin 4 high (Thbs4hi) astrocyte production from the postnatal SVZ and these cells home to the injured cortex. Thbs4 homozygous knockout mice (Thbs4KO/KO) demonstrated severe defects in cortical-injury-induced SVZ astrogenesis, resulting in abnormal glial scar formation (Benner et al., 2013). This robust post-injury astrogenic response requires SVZ Notch activation, modulated by Thbs4 via direct Notch1 receptor binding and endocytosis to activate downstream signals, including increased expression of the transcription factor NFIA, which is important for developmental gliogenesis (Deneen et al., 2006). In another report, Thbs4KO/KO animals exhibited impaired migration of newly formed neurons along the RMS, with several neurons migrating out of the RMS (Girard et al., 2014). Together, these studies suggest a role for Thbs4 associated with Notch and NFIA in SVZ-issued astrocytes migration following injury.
NFIA
NFIA plays a crucial role in the onset of gliogenesis, astrocyte differentiation, and maintaining morphological integrity of astrocytes in the adult hippocampus (Kang et al., 2012; Huang A. Y.-S. et al., 2020). NFIA is highly expressed in SVZ-NSCs and plays a general role in maintaining proliferative cell populations in the SVZ under homeostatic conditions (Laug et al., 2019). In addition, NFIA is required for SVZ proliferation in the uninjured brain and after cortical ischemia, suggesting that defects in reactive astrogenesis could be a result of these defects in the SVZ. Furthermore, absence of NFIA was associated with a decrease in cellular proliferation. After ischemic stroke, NFIA plays a role in the production of reactive astrocytes from the SVZ and its absence was associated with aberrant glial scar formation, highlighted by increased and prolonged blood serum leakage into the parenchyma. Mechanistically, NFIA directly regulates the expression of Thbs4 in the SVZ, revealing a key transcriptional node that contributes to reactive astrogenesis following cortical injuries (Laug et al., 2019).
Sox9
The transcription factor Sox9 regulates induction of NFIA and plays a crucial role in the onset of gliogenesis (Stolt et al., 2003; Kang et al., 2012), while activation of Notch1 during neuroectodermal differentiation has been shown to upregulate Sox9 expression (Martini et al., 2013). Furthermore, in adult astrocytes, Sox9-expression is required to maintain morphological integrity of astrocytes in the olfactory bulb (Ung et al., 2021). Overexpression of Sox9 in the adult SVZ suppresses production of neurons from NSCs, whereas Sox9 knockdown stimulates neurogenesis and inhibits gliogenesis (Cheng et al., 2009) indicating that Sox9 promotes astrogenesis in SVZ-NSC populations. The role of Sox9 in SVZ-NSC astrocyte production after injury remains poorly defined, however, Sox9 is expressed in reactive astrocytes after MCAO injury (Sun et al., 2017) suggesting that it may also have a role in injury associated SVZ-astrogenesis.
Notch/STAT3
During development, NPCs express Notch ligands and activate Notch signaling in neighboring NPCs, conferring astrocytic differentiation potential through the induction of NFIA. This Notch-NFIA pathway potentiates Stat3-activity and further reinforces the astrocytic differentiation program (Namihira et al., 2009). Following cortical stab wound or stroke, the astrogliogenic response of the SVZ to injury is accompanied by activation of the Notch pathway modulated by Thbs4 (Givogri et al., 2006; Benner et al., 2013). Therefore, NOTCH/STAT3 pathway appears to play a central role in SVZ-induced astrogliogenesis response following injury.
JAK/STAT Pathway
Leukemia Inhibitory Factor
Leukemia inhibitory factor (LIF) activates the JAK/STAT pathway, which plays an important role in NSC/NSP differentiation into glia. Indeed, the JAK/STAT pathway promotes astrocyte differentiation during development (Bonni et al., 1997) and is specifically activated in subsets of CNS lesions (Okada et al., 2006). Treatment of cultured embryonic SVZ-NPCs with LIF generates GFAP + cells that have the characteristics typical of adult SVZ and SGZ stem cells/astrocytes (Bonaguidi et al., 2005). In addition, LIF impairs neuroblast formation in the SVZ and stimulates the formation of SVZ astrocytes (Bauer and Patterson, 2006). Therefore, under homeostatic conditions LIF, via the JAK/STAT pathway, can promote SVZ-astrogliogenesis. However, because JAK/STAT signaling promotes astrogliogenesis, coupled with the fact that it is activated following CNS injury, suggests that it may also contribute to SVZ-astrogliogenesis following injury.
Endothelin-1
Astrocytic endothelin-1 overexpression in mice (GET-1) promotes NSC proliferation and astrocytic differentiation via the Jak2/Stat3 pathway after MCAO in the ipsilateral SVZ (Cheng et al., 2019). Therefore, JAK2/STAT3 pathway appears to play a central role in SVZ-induced astrogliogenesis response following injury.
Bone Morphogenetic Protein Pathway
Bone Morphogenetic Protein 4
Bone morphogenetic protein (BMP) signaling promotes the generation of astrocytes from the SVZ (Gross et al., 1996; Gomes et al., 2003). BMP receptor activation inhibits proliferation and suppresses SVZ neurogenesis while promoting gliogenesis (Lim et al., 2000). In the absence of injury, BMP4 promotes astrocytic differentiation of SVZ-NPCs both in vitro and in vivo (Gross et al., 1996; Bonaguidi et al., 2005). During demyelination BMP4 increased levels are associated with an increase in phosphorylated SMAD 1/5/8. Further, treatment with BMP4 or demyelination increased production of astrocytes within the SVZ compared to naïve mice indicating that BMP4 is sufficient to promote SVZ-produced astrocytes (Cate et al., 2010).
ID-3, P57kip2, and Agmantine
In addition, genetic depletion of the transcriptional regulator Id3 (BMP-2-induced transcriptional regulator) decreased the number of astrocytes generated from SVZ-derived adult NSCs/NSPs in the cortical lesion area after TBI (Bohrer et al., 2015). Deletion of P57kip2, an important upstream promoter of BMP4-mediated astrogliogenesis, abrogated astrogenesis from SVZ-NSCs, possibly through increased Noggin and chordin levels (Jadasz et al., 2012) comforting the role of the BMP pathway in SVZ-astrocytes production following injury. In SVZ-NSCs cultures, treatment with Agmatine, an endogenous primary amine, increased ERK1/2 expression and suppressed astrogenesis by decreasing expression of BMP 2,4 and SMAD 1,5,8 in SVZ-NSCs (Song et al., 2011) confirming the involvement of BMP and SMAD in SVZ-astrocytes response after injury.
Galectin-3
In another series of BMP-associated studies, galectin-3 (Gal-3) overexpression increased the percentage of striatal astrocytes generated by the SVZ, coupled with a concomitant decrease in the percentage of oligodendrocytes. Mechanistically, Gal-3 induced BMP signaling by binding to the BMP receptor one alpha (BMPR1α) and by increasing the phosphorylation of pSmad1/5/8, BMP signaling in turn suppressed Gal-3 expression. Indeed, Gal-3 mRNA levels were reduced 24 and 48 h after BMP4 treatment of SVZ cells, suggesting negative feedback of BMP on Gal-3 transcription. In the same study, in human following hypoxia/ischemia, Gal-3 immunoreactivity was increased in the perinatal human SVZ and striatum suggesting a role of Gal-3 in promoting SVZ-gliogenesis after injury (Al-Dalahmah et al., 2020). Of note, Gal-3 appears to play an important role in non-SVZ gliogenesis as well, as its deletion led to a reduction in the number of striatal glial populations, whereas its overexpression led to an increase in glial production.
Fibrinogen
In addition, fibrinogen which is enriched in the SVZ niche following cortical brain injury in mice, inhibited neuronal differentiation in SVZ and hippocampal NSPs/NSCs while promoting astrogenesis via activation of the BMP receptor signaling pathway. These results suggest that fibrinogen is a regulator of NSPC-derived astrogenesis from the SVZ niche via BMP receptor signaling pathway following injury (Pous et al., 2020). Collectively, these studies demonstrate that activation of BMP signaling promotes SVZ-astrogliogenesis under both homeostatic and injury conditions.
To conclude, several pathways can influence SVZ-astrogliogenesis. Taking into account the relationship between these factors, we propose a potential pathway between Thbs4, Notch, Sox9, NFIA and Stat 3 that drives SVZ-astrogenesis. It begins with Thbs4 physically interacting with Notch1 to activate downstream effectors of the Notch pathway (Givogri et al., 2006; Benner et al., 2013) and inducing NFIA expression in primary SVZ-NSCs (Benner et al., 2013). Next, Sox9 contributes to the induction of NFIA expression (Kang et al., 2012), while activation of Notch1 upregulates Sox9 expression, thus reinforcing the transcriptional apparatus that confers astrocyte identity (Martini et al., 2013). Subsequently, NFIA directly regulates Thbs4-expression (Laug et al., 2019) and Notch activates NFIA leading to STAT3-activating signals (Namihira et al., 2009) providing a feed-forward, self-propagating gliogenic signaling mechanism (Figure 2).
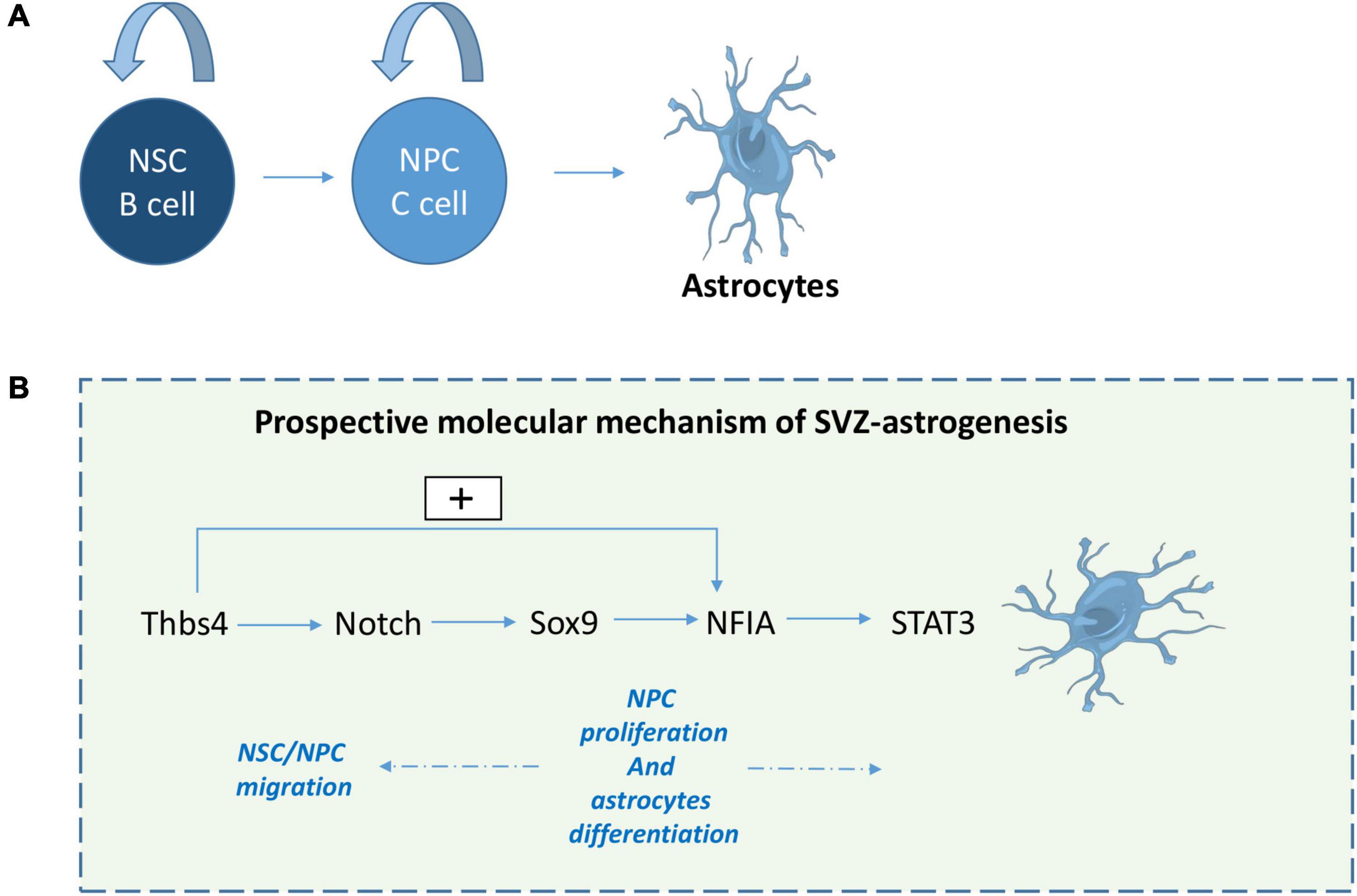
Figure 2. Prospective molecular mechanism of SVZ-derived astrogliogenesis. Following stroke or TBI, NPCs give rise to astrocytes (A). Here we suggest a prospective mechanism for SVZ astrogenesis (B): 1- Thbs4 activates downstream effectors of the Notch pathway and NFIA, 2- Sox9 regulates NFIA induction, while Notch activation upregulates Sox9 expression, 3- NFIA directly regulates Thbs4-expression and 4- Notch activates NFIA leading to STAT3-activating signal which play a role in NPC proliferation and astrocytes differentiation.
The Subventricular Zone Produces Myelinated Oligodendrocytes Following Demyelination
SVZ-NSCs are also able to produce oligodendrocytes as discussed above. Parenchymal oligodendrocyte precursor cells (OPCs) and SVZ-derived progenitors are the two main sources of progenitor cells that contribute to oligodendrogenesis (for review El Waly et al., 2014). A small fraction of SVZ-NSCs are also able to generate OPCs that migrate out of the SVZ into the overlying white matter and cortex (Menn et al., 2006).
The number of oligodendrocytes derived from NSCs increased fourfold after a demyelinating lesion in the CC, indicating that SVZ-NPCs participate in myelin repair in the adult brain following injury (Nait-Oumesmar et al., 1999; Picard-Riera et al., 2002; Menn et al., 2006). Examination of post-mortem human Multiple Sclerosis (MS) brains revealed the migration of SVZ-OPCs to periventricular lesions, where they could participate in remyelination (Nait-Oumesmar et al., 2007). In mice, SVZ-OPCs migrate during the remyelination phase after cuprizone-induced demyelination to the CC and are capable of forming new oligodendrocytes (Butti et al., 2019), while also producing thicker myelin sheets (Xing et al., 2014). Therefore, stimulating the regenerative potential of SVZ-NPCs could be a promising strategy for therapies for demyelinating diseases such as MS.
In the nestin-CreERT2-R26R-YFP mouse model, demyelination led to decreased neurogenesis in the adult brain (Luo et al., 2020) that was coupled with a massive recruitment of SVZ-NSCs to the demyelinated CC during the acute phases of injury response, where these recruited cells subsequently differentiated into myelinating oligodendrocytes (Brousse et al., 2015). In addition, ablation of SVZ-NSCs using Ganciclovir during cuprizone-induced demyelination resulted in reduced numbers of oligodendrocytes within the lesioned CC (Butti et al., 2019), while local irradiation of the SVZ preserves the capacity of NSCs to respond to a demyelinating lesion in the striatum and differentiate in oligodendrocytes (Capilla-Gonzalez et al., 2014).
Following experimental autoimmune encephalomyelitis (EAE) (Calzà et al., 1998; Picard-Riera et al., 2002), aspiration induced cortical lesions (Goings et al., 2004), or traumatic axonal injury (Sullivan et al., 2013), SVZ cells also migrate from the SVZ to the CC and differentiate into oligodendrocytes. Hypoxic-ischemic insult also stimulates SVZ-NSCs to generate new neurons and oligodendrocytes in vitro (Yang and Levison, 2006) or in vivo (Zaidi et al., 2004). OPC expression of NG2-/Olig2 in the SVZ occurs within the first few days after hypoxia (Jablonska et al., 2012), with one study reporting an increase in the number of Olig2 + cells in the posterior part of the SVZ, which subsequently migrated into the injured white matter (Kako et al., 2012).
In a model of demyelination following injection of lysolecythin in the anterior CC, SVZ-NPCs labeled by direct injection of cytomegalovirus (CMV)-GFP retrovirus revealed co-labeling with Olig2 and the proteoglycan NG2. These cells were predominately located within the CC. In addition, spontaneous excitatory postsynaptic currents significantly increased 1 week after the lesion, indicating that oligodendrocytes became synaptically connected (Etxeberria et al., 2010). An additional study used genetic fate mapping following acute demyelination by local injection of α-lysophosphatidylcholine (LPC) in the CC to demonstrate that local OPCs rapidly respond and expand in the lesion within 7 days, and produced oligodendrocytes within 2 weeks after injury. By contrast, NSC-derived NG2 cells did not significantly increase in the lesion until 4 weeks after demyelination and generated fewer oligodendrocytes than parenchymal OPCs.
These observations suggest that local OPCs may be the primary responders to repair acutely demyelinated lesion whereas SVZ-NSCs may contribute to repopulating OPCs following their depletion due to oligodendrocyte differentiation (Serwanski et al., 2018).
In contrast, another study reported that SVZ-derived cells of the oligodendroglial lineage that migrate either to the intact or the focally demyelinated CC have limited migratory and self-renewal capacity and fail to generate mature myelin (Kazanis et al., 2017). A further study proposed that SVZ-NSCs are dispensable for myelin repair but protect neurons from degeneration (Butti et al., 2019). Beyond their role replacing oligodendrocytes, SVZ-NSCs also display immunomodulatory properties, highlighting a new role for endogenous SVZ-NSC in myelin regeneration. Indeed, SVZ-NSC may minimize demyelination by modulating microglial activity and promoting myelin debris phagocytosis (Brousse et al., 2020).
Molecular Regulators of Subventricular Zone-Derived Oligodendrocytes
Recently, several studies have demonstrated that a range of factors such as LIF/CNTF, Endothelin-1, Notch, EGFR play a major role in oligodendrogenesis (for review Gonzalez-Perez and Alvarez-Buylla, 2011; Maki et al., 2013; El Waly et al., 2014; Adams et al., 2020). In this review, we will summarize the role of these factors in SVZ-oligodendrogenesis with a focus on factors positively regulating oligodendrogenesis in the SVZ in the context of injury and especially white matter injury (Table 2).
Notch Pathway
Endothelin-1
Endothelial cells produce endothelin (ET-1), which promotes oligodendrocyte differentiation in the SVZ. ET-1 stimulates a pro-migratory phenotype in cultured OPCs and SVZ explants, while selective ET receptor antagonists or anti-ET-1 antibodies inhibit OPC migration from the SVZ (Gadea et al., 2009). Another study revealed that ET-1 acts selectively through EDNRB on astrocytes, but not OPCs, to indirectly inhibit remyelination (Hammond et al., 2015). Loss of ET-1 signaling increases neurogenesis and reduces OPC in the developing SVZ. In addition, ET-1 acts mechanistically by promoting Notch activation in OPCs during remyelination through induction of Jagged1 expression in reactive astrocytes (Hammond et al., 2015; Adams et al., 2020). ET-1 also induces upregulation of Gsx1 and downregulation of S100b in SVZ OPCs, which acts to increase their proliferation as well (Adams et al., 2020). Together, these studies reinforce the notion that ET-1 is required for increased NPCs and OPC proliferation in the adult mouse SVZ following demyelination (Adams et al., 2020).
Jagged 1, TGF-β, F3/Contactin, Apotransferrin
Levels of Notch ligand Jagged1 increase in the CC and SVZ during the early phases of LPC-mediated demyelination in rats. This increase was found to induce Notch intracellular domain (NICD) release and Hes5 expression, which increased OPC proliferation (Aparicio et al., 2013). TGF-β also has pro-oligodendrogenic effects on adult SVZ progenitors in vitro and induced the expression of Jagged1 and downstream gene Hes1 (Pinto et al., 2018). In this context it appears that Notch activation is mediated by the expression of F3/contactin, which could then induce apotransferrin-mediated oligodendroglial maturation (Aparicio et al., 2013). Indeed, intranasal administration of apotransferrin enhanced OPC proliferation in the SVZ and CC and promoted OPC differentiation (Guardia Clausi et al., 2012). Therefore, these studies suggest a role for Notch involving apotransferrin in promoting OPC proliferation and oligodendrocytes differentiation.
Bone Morphogenetic Protein Pathway
Noggin
Noggin promotes the proliferation of SVZ-NSCs, and shifts the differentiation of NSCs from mature astrocytes to transit amplifying NPCs and OPCs without depleting the NSC population (Morell et al., 2015). Intraventricular infusion of Noggin, which is an endogenous antagonist of BMP4, reduced pSMAD1/5/8, decreased astrocyte numbers, and increased oligodendrocyte numbers in the SVZ during cuprizone-induced demyelination (Cate et al., 2010). Therefore, these results suggest that Noggin promotes oligodendrogenesis following demyelination injury.
JAK/STAT Pathway
Leukemia Inhibitory Factor/Ciliary Neurotrophic Factor
Ciliary neurotrophic factor (CNTF) and LIF are neurotrophic cytokine belonging to the interleukin-6 (IL6) family that activates the JAK/STAT pathway, which also plays an active role in astrogenesis.
LIF stimulates the self-renewal of adult NSCs in the SVZ, which may expand this population to facilitate repair (Bauer and Patterson, 2006). This finding has relevance for the repair of demyelination since NSCs can generate migratory OPCs that differentiate into oligodendrocytes and contribute to remyelination (Menn et al., 2006).
On the other hand, CNTF (Ciliary neurotrophic factor) controls the migration of SVZ-derived progenitors following HEK cells secreting CNTF graft into the CC and also controls OPCs toward the demyelinated CC both in vivo and in in vitro models (Vernerey et al., 2013) suggesting a role for both LIF and CNTF in oligodendrocytes migration/differentiation following demyelination.
WNT Pathway
Canonical Wnt
Shifts between non-canonical and canonical Wnt signaling activate quiescent NSCs during demyelination injury (Chavali et al., 2018). In vivo activation or inhibition of canonical Wnt signaling increased or decreased the number of Olig2 and PDGFR-α positive cells, respectively, suggesting that this pathway contributes to the fine tuning of oligodendrogliogenesis in the adult SVZ (Ortega et al., 2013).
SFRP1 and SFRP5
Evidence suggests that altered-clock-derived signals in the demyelinated lesion mediate communication with the SVZ to switch NSCs toward generation of oligodendrocyte lineage cells, which enhances remyelination. Astrocyte circadian clocks produce the Wnt inhibitors SFRP1 and SFRP5 that signal to the SVZ to reduce the circadian transcription factor BMAL1 (Huang S. et al., 2020). Together, these studies indicate that Wnt signaling promotes oligodendrogenesis issued from the SVZ in the demyelination lesion.
Growth Factors
Epidermal Growth Factor and Fibroblast Growth Factor Signaling
Epidermal growth factor (EGF) and fibroblast growth factor (FGF) signaling pathways play key roles in a spectrum of developmental and physiological processes, including SVZ-NSC responses. Increasing FGF receptor-3 activity in adult SVZ-NSCs cells in a Nestin-CreER mouse model transiently promoted differentiation from the neuronal to the oligodendroglial lineage following demyelination and improved myelin repair in the CC and in the lower cortical layers (Kang et al., 2019). In addition, anosmin-1 over-expression was shown to regulate OPCs proliferation, migration and myelin sheath thickness predominately through FGFR1 receptor (Murcia-Belmonte et al., 2016).
EGFR-dependent, N-cadherin signaling promotes migration of oligodendrocytes or oligodendrocyte progenitors into demyelinated lesions (Klingener et al., 2014). Additional studies also demonstrated that NG2+ cells responses in the SVZ and differentiation in CC after focal demyelination are dependent upon EGFR signaling (Aguirre and Gallo, 2007). EGFR overexpression in the SVZ and CC during early postnatal development expanded oligodendrocytes progenitors, which enhanced the generation of oligodendrocytes and subsequent axonal myelination in the lesion (Aguirre et al., 2007). Together, these studies indicate that EGF signaling plays a role in SVZ-NSC responses following demyelination injury by promoting oligodendrogenesis. Taken further, direct administration via intraperitoneal injection of EGF, FGF-2, and platelet-derived growth factor (PDGF) promoted the survival, migration, and differentiation of grafted SVZ cells into myelin-forming oligodendrocytes. This combination of growth factors expanded the constitutively proliferative PSA-NCAM + population in vivo and facilitated their differentiation toward the neuronal and oligodendroglial cell fates (Lachapelle et al., 2002). Furthermore, EGF infusion into the lateral ventricle (Gonzalez-Perez et al., 2009) or intranasal HB-EGF administration (Cantarella et al., 2008) promoted OPC recruitment from the SVZ to demyelinated lesions.
Importantly, endogenous EGF is upregulated in the white matter and SVZ after perinatal hypoxia and EGFR overexpression in the oligodendrocyte lineage enhances their regeneration and promotes functional recovery in white matter (Scafidi et al., 2014). In addition, in the SVZ, Notch regulates NSC identity and self-renewal, whereas EGFR specifically affects NPC proliferation and migration. Enhanced EGFR signaling resulted in the expansion of the NPC pool and reduced NSC number and self-renewal by inhibiting Notch signaling (Aguirre et al., 2010). Intranasal EGF was also shown to accelerate oligodendrocyte maturation in white matter after chronic neonatal hypoxia (Scafidi et al., 2014) suggesting a role of growth factors in promoting SVZ-issued oligodendrogenesis.
Insulin-Like Growth Factor I
It was also shown that administration of a related-growth factor, Insulin-like growth factor I (IGF-1), prevented immature oligodendrocyte death, enhanced myelination after hypoxia/ischemia and protected OPCs in the SVZ and white matter regions (Lin et al., 2005; Zhong et al., 2009) suggesting that additional IGF-1 associated pathway promote SVZ-issued oligodendrogenesis.
Thyroid Hormone (TH) Signaling—T3
EGFR+ oligodendrocyte progenitors, but not neuroblasts, express high levels of a T3-inactivating deiodinase, Dio3. T3 through its nuclear receptor, TRα1, favors progenitor commitment toward a neuroblast phenotype. However, a transient T3-free window increases OPCs numbers. Therefore T3 free window provides a favorable environment for SVZ-derived oligodendrocyte progenitor generation (Remaud et al., 2017). This finding indicates that T3 free window promotes remyelination and therefore, plays a role in SVZ-issued oligodendrocytes.
Sonic Hedgehog Pathway
SmoM2
Smoothened (Smo) is essential for Sonic hedgehog signaling. Genetic ablation of Smo in the dorsal SVZ resulted in a reduction of oligodendroglial cells in the CC. In contrast, expression of constitutively active SmoM2 significantly increased the number of oligodendrocytes (Tong et al., 2015). Overall, these results suggest that Smo increases SVZ-issued oligodendrocytes and suggest a potential role for Smo in demyelination injury, though these mechanisms have not been directly examined in this context.
Others Associated Pathways
CDK4, Prickle1, and Nfe2l3
Hypoxia-induced proliferation of NG2-/Olig2-expressing OPCs occurs in the SVZ within the first few days after insult and depends on activation of the Cdk4 pathway (Jablonska et al., 2012). Subsequent studies demonstrated that Prickle1 and Nfe2l3 are strongly associated with differentiation toward myelin-producing cells. Prickle1 dramatically affects OPCs maturation and differentiation to mature myelinating oligodendrocytes, while Nfe2l3 has a moderate effect on OPC maturation in the absence of injury (Zilkha-Falb et al., 2017). Overall, these studies suggest a role of Cdk4 and Prickle1 in SVZ-induced oligodendrogenesis with a potential role in the context of injury.
Asialo-Erythropoietin, Zfp488, FTY720
Long-term post-injury treatment with a non-erythropoietic derivative of EPO, asialo-erythropoietin, promoted the maturation of the posterior SVZ-derived OPCs and the recovery of neurological function, without affecting hematopoiesis (Kako et al., 2012). On the other hand, overexpression of oligodendrocyte-specific zinc finger transcription repressor (Zfp488) retrovirus in SVZ-NSCs following Cuprizone-induced demyelination in mice promoted differentiation into mature oligodendrocytes (Soundarapandian et al., 2011). In addition, FTY720, a Src-bcr-Abl tyrosine kinase inhibitor and S1P receptor agonist, increased postnatal SVZ-NSCs differentiation into both neurons and oligodendrocytes in vitro and partially increased proliferation and differentiation of OPC after kainic acid lesion in vivo (Cipriani et al., 2017).
These collective studies implicate a role for SVZ-NSC derived oligodendrocytes in myelin-associated injury responses and highlight several pathways and strategies that are able to promote SVZ-oligodendrogenesis and myelin repair following demyelination. It appears that Notch has a dual role in oligodendrogenesis being both inhibited by EGFR signaling which promotes OPC proliferation and oligodendrocytes differentiation (Aguirre et al., 2010; Scafidi et al., 2014) but also being activated by Endothelin-1 and Jagged 1 [also activated by TGF B (Pinto et al., 2018)] to promote OPC proliferation through NICD and Hes5 (Adams et al., 2020). Interestingly Endothelin-1 also plays a role on OPC proliferation by activation Gsx1 but also inhibits oligodendrocytes maturation through S100b inhibition (Adams et al., 2020; Figure 3). Taking this dual role of Notch, it would be of interest following demyelination to assess EGFR signaling and Endothelin-1 signaling relationship.
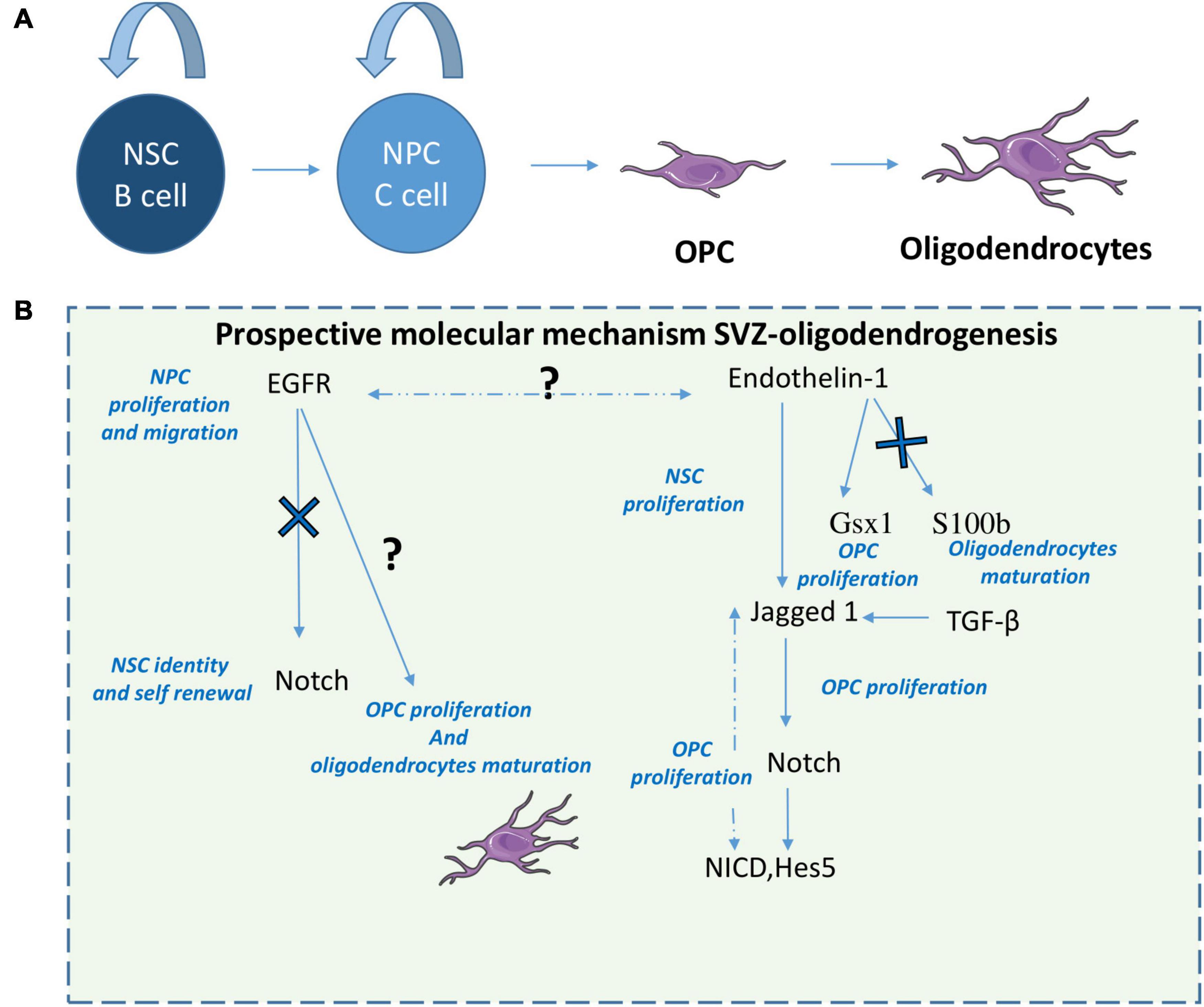
Figure 3. Prospective molecular mechanisms of SVZ-derived oligodendrogenesis. After demyelination or other white matter injuries, NPCs give rise to OPCs that will differentiate in oligodendrocytes (A). Here we suggest a prospective mechanism that modulates SVZ oligodendrogenesis (B). 1-EGFR signaling increases NPC proliferation and migration but also oligodendrocyte maturation by inhibiting Notch. 2-ET-1 promotes Notch activation in OPCs during remyelination through induction of Jagged1 and increases NPCs and OPC proliferation. 3- ET-1 also induces upregulation of Gsx1 and downregulation of S100b in SVZ OPCs, increasing their proliferation but blocking oligodendrocytes maturation. 4-TGF-β has pro-oligodendrogenic effects by increasing Jagged 1.
Conclusion
In conclusion, astrocytes and oligodendrocytes play pivotal roles in diverse injury responses throughout the CNS and the type of injury and location in the brain where the injury occurs dictates their generation by SVZ-NSCs. Indeed, ischemia and TBI induce the SVZ to produce astrocytes, whereas oligodendrocytes are produced after white matter injury and demyelinating events. Furthermore, the fate of SVZ-NSCs under physiological conditions is regulated by the combined actions of intrinsic and extrinsic factors, in addition of regional differences within the SVZ. Although several key features of these two cell types under normal physiological conditions and in response to injury have emerged in recent decades, additional studies combining new genetic tools, molecular studies, and behavioral tests are warranted to further decipher how these injury-specific responses regulate SVZ production of astrocytes or oligodendrocytes.
In addition, SVZ-issued astrocytes play a major role in glial scar formation. It is unclear whether SVZ-generated astrocytes migrating to the injury site, 1- represent a distinct subset of astrocytes compared to parenchymal astrocytes, 2- have different functions in brain repair and capacity to integrate to the existing brain circuitry. Furthermore, future studies should consider SVZ-astrocytes as a strategy to compensate for neuronal loss following injury. The adult brain cortex has limited ability to produce new neurons, therefore reprogramming astrocytes into neurons could be an ideal approach to replenish the lost cells and repair the damage. As it can be challenging to reprogram fully differentiated astrocytes and that NSCs make newly formed astrocytes contributing to the glial scar following injury, there is a potential for in vivo reprogramming of SVZ-issued astrocytes into neurons.
SVZ-issued oligodendrocytes also play a major role in re-myelination and their proliferation rate or subtype may also differ from parenchymal oligodendrocytes. SVZ-OPCs are recruited during the remyelination phase to the CC and are capable of forming new oligodendrocytes. Therefore, future studies should focus on promoting SVZ-OPCs proliferation and differentiation by endogenous or exogenous factors in order to promote myelin repair. It would also be of interest to establish therapies stimulating myelin repair to prevent neurodegeneration in pathology like MS.
Lastly, there are sub-regional differences of the SVZ niche with respect to embryonic origins and cell subtype generation (Young et al., 2007), where single-cell analysis revealed that SVZ lateral and septal wall astrocytes are primarily neurogenic and oligodendrogenic, respectively (Mizrak et al., 2019). This suggests functionally relevant spatial diversity in neurogenesis and oligodendrogenesis in the adult brain, while also revealing molecular correlates of adult NSC dormancy and lineage specialization (Mizrak et al., 2019). To our knowledge, single-cell analysis on SVZ subregions involved in astrogenesis has yet to be performed and could provide insight into the origins of diverse astrocyte responses. Therefore, future studies should assess astrogliogenesis modulation by specific factors in specific SVZ subregions in order to understand specific sub-SVZ regions implicated in SVZ-astrogliogenesis response following injury. Besides, the dorsal SVZ appears to be more oligodendrogliogenic than the lateral SVZ, consistent with the notion of a mosaic organization of the SVZ (Merkle et al., 2007; Cebrian-Silla et al., 2021; Delgado et al., 2021). Therefore, future studies assessing oligodendrogliogenic modulation by specific factors in specific SVZ subregions are necessary to increase our understanding of specific sub-SVZ regions implication in SVZ-oligodendrogenesis response following injury.
Author Contributions
All authors listed have made a substantial, direct, and intellectual contribution to the work, and approved it for publication.
Funding
This work was supported by the US National Institutes of Health grants (R01NS096096).
Conflict of Interest
The authors declare that the research was conducted in the absence of any commercial or financial relationships that could be construed as a potential conflict of interest.
Publisher’s Note
All claims expressed in this article are solely those of the authors and do not necessarily represent those of their affiliated organizations, or those of the publisher, the editors and the reviewers. Any product that may be evaluated in this article, or claim that may be made by its manufacturer, is not guaranteed or endorsed by the publisher.
Acknowledgments
We thank Catherine Gillepsie for editorial assistance and Moawiah Naffa’A, Kehali Woldemichael, and Rutesh Vyas for helpful discussions and input.
References
Adams, K. L., Riparini, G., Banerjee, P., Breur, M., Bugiani, M., and Gallo, V. (2020). Endothelin-1 signaling maintains glial progenitor proliferation in the postnatal subventricular zone. Nat. Commun. 11:2138. doi: 10.1038/s41467-020-16028-8
Aguirre, A., Dupree, J. L., Mangin, J. M., and Gallo, V. (2007). A functional role for EGFR signaling in myelination and remyelination. Nat. Neurosci. 10, 990–1002. doi: 10.1038/nn1938
Aguirre, A., and Gallo, V. (2007). Reduced EGFR signaling in adult progenitors of the subventricular zone attenuates oligodendrogenesis after demyelination. Neuron Glia Biol. 3, 209–220. doi: 10.1017/S1740925X08000082
Aguirre, A., Rubio, M. E., and Gallo, V. (2010). Notch and EGFR pathway interaction regulates neural stem cell number and self-renewal. Nature 467, 323–327. doi: 10.1038/nature09347
Akdemir, E. S., Huang, A. Y.-S., and Deneen, B. (2020). Astrocytogenesis: where, when, and how. F1000Research 9:F1000 Faculty Rev-233. doi: 10.12688/f1000research.22405.1
Al-Dalahmah, O., Campos Soares, L., Nicholson, J., Draijer, S., Mundim, M., Lu, V. M., et al. (2020). Galectin-3 modulates postnatal subventricular zone gliogenesis. Glia 68, 435–450. doi: 10.1002/glia.23730
Altman, J. (1962). Are new neurons formed in the brains of adult mammals? Science 135, 1127–1128. doi: 10.1126/science.135.3509.1127
Aparicio, E., Mathieu, P., Pereira Luppi, M., Almeira Gubiani, M. F., and Adamo, A. M. (2013). The Notch signaling pathway: its role in focal CNS demyelination and apotransferrin-induced remyelination. J. Neurochem. 127, 819–836. doi: 10.1111/jnc.12440
Aponso, P. M., Faull, R. L. M., and Connor, B. (2008). Increased progenitor cell proliferation and astrogenesis in the partial progressive 6-hydroxydopamine model of Parkinson’s disease. Neuroscience 151, 1142–1153. doi: 10.1016/j.neuroscience.2007.11.036
Bauer, S., and Patterson, P. H. (2006). Leukemia inhibitory factor promotes neural stem cell self-renewal in the adult brain. J. Neurosci. 26, 12089–12099. doi: 10.1523/JNEUROSCI.3047-06.2006
Benner, E. J., Luciano, D., Jo, R., Abdi, K., Paez-Gonzalez, P., Sheng, H., et al. (2013). Protective astrogenesis from the SVZ niche after injury is controlled by Notch modulator Thbs4. Nature 497, 369–373. doi: 10.1038/nature12069
Bohrer, C., Pfurr, S., Mammadzada, K., Schildge, S., Plappert, L., Hils, M., et al. (2015). The balance of Id3 and E47 determines neural stem/precursor cell differentiation into astrocytes. EMBO J. 34, 2804–2819. doi: 10.15252/embj.201591118
Bonaguidi, M. A., McGuire, T., Hu, M., Kan, L., Samanta, J., and Kessler, J. A. (2005). LIF and BMP signaling generate separate and discrete types of GFAP-expressing cells. Development 132, 5503–5514. doi: 10.1242/dev.02166
Bonaguidi, M. A., Song, J., Ming, G., and Song, H. (2012). A unifying hypothesis on mammalian neural stem cell properties in the adult hippocampus. Curr. Opin. Neurobiol. 22, 754–761. doi: 10.1016/j.conb.2012.03.013
Bonni, A., Sun, Y., Nadal-Vicens, M., Bhatt, A., Frank, D. A., Rozovsky, I., et al. (1997). Regulation of gliogenesis in the central nervous system by the JAK-STAT signaling pathway. Science 278, 477–483. doi: 10.1126/science.278.5337.477
Brousse, B., Magalon, K., Daian, F., Durbec, P., and Cayre, M. (2020). Endogenous neural stem cells modulate microglia and protect from demyelination. bioRxiv [Preprint]. doi: 10.1101/2020.06.18.158782
Brousse, B., Magalon, K., Durbec, P., and Cayre, M. (2015). Region and dynamic specificities of adult neural stem cells and oligodendrocyte precursors in myelin regeneration in the mouse brain. Biol. Open 4, 980–992. doi: 10.1242/bio.012773
Burda, J. E., Bernstein, A. M., and Sofroniew, M. V. (2016). Astrocyte roles in traumatic brain injury. Exp. Neurol. 275(Pt 3), 305–315. doi: 10.1016/j.expneurol.2015.03.020
Burns, K. A., Murphy, B., Danzer, S. C., and Kuan, C.-Y. (2009). Developmental and post-injury cortical gliogenesis: a genetic fate-mapping study with nestin-CreER mice. Glia 57, 1115–1129. doi: 10.1002/glia.20835
Butti, E., Bacigaluppi, M., Chaabane, L., Ruffini, F., Brambilla, E., Berera, G., et al. (2019). Neural stem cells of the subventricular zone contribute to neuroprotection of the corpus callosum after cuprizone-induced demyelination. J. Neurosci. Off. J. Soc. Neurosci. 39, 5481–5492. doi: 10.1523/JNEUROSCI.0227-18.2019
Calzà, L., Giardino, L., Pozza, M., Bettelli, C., Micera, A., and Aloe, L. (1998). Proliferation and phenotype regulation in the subventricular zone during experimental allergic encephalomyelitis: In vivo evidence of a role for nerve growth factor. Proc. Natl. Acad. Sci. U.S.A. 95, 3209–3214. doi: 10.1073/pnas.95.6.3209
Cantarella, C., Cayre, M., Magalon, K., and Durbec, P. (2008). Intranasal HB-EGF administration favors adult SVZ cell mobilization to demyelinated lesions in mouse corpus callosum. Dev. Neurobiol. 68, 223–236. doi: 10.1002/dneu.20588
Capilla-Gonzalez, V., Guerrero-Cazares, H., Bonsu, J. M., Gonzalez-Perez, O., Achanta, P., Wong, J., et al. (2014). The subventricular zone is able to respond to a demyelinating lesion after localized radiation. Stem Cells Dayt. Ohio 32, 59–69. doi: 10.1002/stem.1519
Cate, H. S., Sabo, J. K., Merlo, D., Kemper, D., Aumann, T. D., Robinson, J., et al. (2010). Modulation of bone morphogenic protein signalling alters numbers of astrocytes and oligodendroglia in the subventricular zone during cuprizone-induced demyelination. J. Neurochem. 115, 11–22. doi: 10.1111/j.1471-4159.2010.06660.x
Cebrian-Silla, A., Nascimento, M. A., Redmond, S. A., Mansky, B., Wu, D., Obernier, K., et al. (2021). Single-cell analysis of the ventricular-subventricular zone reveals signatures of dorsal and ventral adult neurogenesis. eLife 10:e67436. doi: 10.7554/eLife.67436
Chai, H., Diaz-Castro, B., Shigetomi, E., Monte, E., Octeau, J. C., Yu, X., et al. (2017). Neural circuit-specialized astrocytes: transcriptomic, proteomic, morphological, and functional evidence. Neuron 95, 531.e9–549.e9. doi: 10.1016/j.neuron.2017.06.029
Chang, E. H., Adorjan, I., Mundim, M. V., Sun, B., Dizon, M. L. V., and Szele, F. G. (2016). Traumatic brain injury activation of the adult subventricular zone neurogenic niche. Front. Neurosci. 10:332. doi: 10.3389/fnins.2016.00332
Chavali, M., Klingener, M., Kokkosis, A. G., Garkun, Y., Felong, S., Maffei, A., et al. (2018). Non-canonical Wnt signaling regulates neural stem cell quiescence during homeostasis and after demyelination. Nat. Commun. 9:36. doi: 10.1038/s41467-017-02440-0
Cheng, L.-C., Pastrana, E., Tavazoie, M., and Doetsch, F. (2009). miR-124 regulates adult neurogenesis in the SVZ stem cell niche. Nat. Neurosci. 12, 399–408. doi: 10.1038/nn.2294
Cheng, X., Yeung, P. K. K., Zhong, K., Zilundu, P. L. M., Zhou, L., and Chung, S. K. (2019). Astrocytic endothelin-1 overexpression promotes neural progenitor cells proliferation and differentiation into astrocytes via the Jak2/Stat3 pathway after stroke. J. Neuroinflammation 16:227. doi: 10.1186/s12974-019-1597-y
Cipriani, R., Chara, J. C., Rodríguez-Antigüedad, A., and Matute, C. (2017). Effects of FTY720 on brain neurogenic niches in vitro and after kainic acid-induced injury. J. Neuroinflammation 14:147. doi: 10.1186/s12974-017-0922-6
De Chevigny, A., Cooper, O., Vinuela, A., Reske-Nielsen, C., Lagace, D. C., Eisch, A. J., et al. (2008). Fate mapping and lineage analyses demonstrate the production of a large number of striatal neuroblasts after TGFα and noggin striatal infusions into the dopamine-depleted striatum. Stem Cells Dayt. Ohio 26, 2349–2360. doi: 10.1634/stemcells.2008-0080
Delgado, A. C., Maldonado-Soto, A. R., Silva-Vargas, V., Mizrak, D., von Känel, T., Tan, K. R., et al. (2021). Release of stem cells from quiescence reveals gliogenic domains in the adult mouse brain. Science 372, 1205–1209. doi: 10.1126/science.abg8467
Deneen, B., Ho, R., Lukaszewicz, A., Hochstim, C. J., Gronostajski, R. M., and Anderson, D. J. (2006). The transcription factor NFIA controls the onset of gliogenesis in the developing spinal cord. Neuron 52, 953–968. doi: 10.1016/j.neuron.2006.11.019
El Waly, B., Macchi, M., Cayre, M., and Durbec, P. (2014). Oligodendrogenesis in the normal and pathological central nervous system. Front. Neurosci. 8:145. doi: 10.3389/fnins.2014.00145
Etxeberria, A., Mangin, J.-M., Aguirre, A., and Gallo, V. (2010). Adult-born SVZ progenitors receive transient glutamatergic synapses during remyelination of the corpus callosum. Nat. Neurosci. 13, 287–289. doi: 10.1038/nn.2500
Faiz, M., Sachewsky, N., Gascón, S., Bang, K. W. A., Morshead, C. M., and Nagy, A. (2015). Adult neural stem cells from the subventricular zone give rise to reactive astrocytes in the cortex after stroke. Cell Stem Cell 17, 624–634. doi: 10.1016/j.stem.2015.08.002
Gadea, A., Aguirre, A., Haydar, T. F., and Gallo, V. (2009). Endothelin-1 regulates oligodendrocyte development. J. Neurosci. Off. J. Soc. Neurosci. 29, 10047–10062. doi: 10.1523/JNEUROSCI.0822-09.2009
Gill, D., and Veltkamp, R. (2016). Dynamics of T cell responses after stroke. Curr. Opin. Pharmacol. 26, 26–32. doi: 10.1016/j.coph.2015.09.009
Girard, F., Eichenberger, S., and Celio, M. R. (2014). Thrombospondin 4 deficiency in mouse impairs neuronal migration in the early postnatal and adult brain. Mol. Cell. Neurosci. 61, 176–186. doi: 10.1016/j.mcn.2014.06.010
Givogri, M. I., de Planell, M., Galbiati, F., Superchi, D., Gritti, A., Vescovi, A., et al. (2006). Notch signaling in astrocytes and neuroblasts of the adult subventricular zone in health and after cortical injury. Dev. Neurosci. 28, 81–91. doi: 10.1159/000090755
Goings, G. E., Sahni, V., and Szele, F. G. (2004). Migration patterns of subventricular zone cells in adult mice change after cerebral cortex injury. Brain Res. 996, 213–226. doi: 10.1016/j.brainres.2003.10.034
Gomes, W. A., Mehler, M. F., and Kessler, J. A. (2003). Transgenic overexpression of BMP4 increases astroglial and decreases oligodendroglial lineage commitment. Dev. Biol. 255, 164–177. doi: 10.1016/s0012-1606(02)00037-4
Gonzalez-Perez, O., and Alvarez-Buylla, A. (2011). Oligodendrogenesis in the subventricular zone and the role of epidermal growth factor. Brain Res. Rev. 67, 147–156. doi: 10.1016/j.brainresrev.2011.01.001
Gonzalez-Perez, O., Romero-Rodriguez, R., Soriano-Navarro, M., Garcia-Verdugo, J. M., and Alvarez-Buylla, A. (2009). Epidermal growth factor induces the progeny of subventricular zone type b cells to migrate and differentiate into oligodendrocytes. Stem Cells 27, 2032–2043. doi: 10.1002/stem.119
Grelat, A., Benoit, L., Wagner, S., Moigneu, C., Lledo, P.-M., and Alonso, M. (2018). Adult-born neurons boost odor–reward association. Proc. Natl. Acad. Sci. U.S.A. 115, 2514–2519. doi: 10.1073/pnas.1716400115
Gross, R. E., Mehler, M. F., Mabie, P. C., Zang, Z., Santschi, L., and Kessler, J. A. (1996). Bone morphogenetic proteins promote astroglial lineage commitment by mammalian subventricular zone progenitor cells. Neuron 17, 595–606. doi: 10.1016/S0896-6273(00)80193-2
Guardia Clausi, M., Paez, P. M., Campagnoni, A. T., Pasquini, L. A., and Pasquini, J. M. (2012). Intranasal administration of aTf protects and repairs the neonatal white matter after a cerebral hypoxic–ischemic event. Glia 60, 1540–1554. doi: 10.1002/glia.22374
Hammond, T. R., McEllin, B., Morton, P. D., Raymond, M., Dupree, J., and Gallo, V. (2015). Endothelin-B receptor activation in astrocytes regulates the rate of oligodendrocyte regeneration during remyelination. Cell Rep. 13, 2090–2097. doi: 10.1016/j.celrep.2015.11.002
Huang, A. Y.-S., Woo, J., Sardar, D., Lozzi, B., Bosquez Huerta, N. A., Lin, C.-C. J., et al. (2020). Region-specific transcriptional control of astrocyte function oversees local circuit activities. Neuron 106, 992.e9–1008.e9. doi: 10.1016/j.neuron.2020.03.025
Huang, S., Choi, M. H., Huang, H., Wang, X., Chang, Y. C., and Kim, J. Y. (2020). Demyelination regulates the circadian transcription factor BMAL1 to signal adult neural stem cells to initiate oligodendrogenesis. Cell Rep. 33:108394. doi: 10.1016/j.celrep.2020.108394
Ihrie, R. A., and Álvarez-Buylla, A. (2011). Lake front property: a unique germinal niche by the lateral ventricles of the adult brain. Neuron 70, 674–686. doi: 10.1016/j.neuron.2011.05.004
Jablonska, B., Scafidi, J., Aguirre, A., Vaccarino, F., Nguyen, V., Borok, E., et al. (2012). Oligodendrocyte regeneration after neonatal hypoxia requires FoxO1-mediated p27Kip1 expression. J. Neurosci. Off. J. Soc. Neurosci. 32, 14775–14793. doi: 10.1523/JNEUROSCI.2060-12.2012
Jadasz, J. J., Rivera, F. J., Taubert, A., Kandasamy, M., Sandner, B., Weidner, N., et al. (2012). p57kip2 regulates glial fate decision in adult neural stem cells. Dev. Camb. Engl. 139, 3306–3315. doi: 10.1242/dev.074518
John Lin, C.-C., Yu, K., Hatcher, A., Huang, T.-W., Lee, H. K., Carlson, J., et al. (2017). Identification of diverse astrocyte populations and their malignant analogs. Nat. Neurosci. 20, 396–405. doi: 10.1038/nn.4493
Jones, T. A. (2017). Motor compensation and its effects on neural reorganization after stroke. Nat. Rev. Neurosci. 18, 267–280. doi: 10.1038/nrn.2017.26
Kako, E., Kaneko, N., Aoyama, M., Hida, H., Takebayashi, H., Ikenaka, K., et al. (2012). Subventricular zone-derived oligodendrogenesis in injured neonatal white matter in mice enhanced by a nonerythropoietic erythropoietin derivative. Stem Cells 30, 2234–2247. doi: 10.1002/stem.1202
Kang, P., Lee, H. K., Glasgow, S. M., Finley, M., Donti, T., Gaber, Z. B., et al. (2012). Sox9 and NFIA coordinate a transcriptional regulatory cascade during the initiation of gliogenesis. Neuron 74, 79–94. doi: 10.1016/j.neuron.2012.01.024
Kang, W., Nguyen, K. C. Q., and Hébert, J. M. (2019). Transient redirection of SVZ stem cells to oligodendrogenesis by FGFR3 activation promotes remyelination. Stem Cell Rep. 12, 1223–1231. doi: 10.1016/j.stemcr.2019.05.006
Kazanis, I., Evans, K. A., Andreopoulou, E., Dimitriou, C., Koutsakis, C., Karadottir, R. T., et al. (2017). Subependymal zone-derived oligodendroblasts respond to focal demyelination but fail to generate myelin in young and aged mice. Stem Cell Rep. 8, 685–700. doi: 10.1016/j.stemcr.2017.01.007
Kernie, S. G., Erwin, T. M., and Parada, L. F. (2001). Brain remodeling due to neuronal and astrocytic proliferation after controlled cortical injury in mice. J. Neurosci. Res. 66, 317–326. doi: 10.1002/jnr.10013
Klingener, M., Chavali, M., Singh, J., McMillan, N., Coomes, A., Dempsey, P. J., et al. (2014). N-cadherin promotes recruitment and migration of neural progenitor cells from the SVZ neural stem cell niche into demyelinated lesions. J. Neurosci. Off. J. Soc. Neurosci. 34, 9590–9606. doi: 10.1523/JNEUROSCI.3699-13.2014
Lachapelle, F. Avellana-Adalid, V. Nait-Oumesmar, B.Baron-Van Evercooren, A. (2002). Fibroblast growth factor-2 (FGF-2) and platelet-derived growth factor AB (PDGF AB) promote adult SVZ-derived oligodendrogenesis in vivo. Mol. Cell. Neurosci. 20, 390–403. doi: 10.1006/mcne.2002.1124
Laug, D., Huang, T.-W., Huerta, N. A. B., Huang, A. Y.-S., Sardar, D., Ortiz-Guzman, J., et al. (2019). Nuclear factor I-A regulates diverse reactive astrocyte responses after CNS injury. J. Clin. Invest. 129, 4408–4418. doi: 10.1172/JCI127492
Li, L., Harms, K. M., Ventura, P. B., Lagace, D. C., Eisch, A. J., and Cunningham, L. A. (2010). Focal cerebral ischemia induces a multilineage cytogenic response from adult subventricular zone that is predominantly gliogenic. Glia 58, 1610–1619. doi: 10.1002/glia.21033
Li, W., Cao, F., Takase, H., Arai, K., Lo, E. H., and Lok, J. (2021). Blood–brain barrier mechanisms in stroke and trauma. Handb. Exp. Pharmacol. [Epub ahead of print]. doi: 10.1007/164_2020_426
Li, W. L., Chu, M. W., Wu, A., Suzuki, Y., Imayoshi, I., and Komiyama, T. (2018). Adult-born neurons facilitate olfactory bulb pattern separation during task engagement. eLife 7:e33006. doi: 10.7554/eLife.33006
Liesz, A., Dalpke, A., Mracsko, E., Roth, S., Zhou, W., Yang, H., et al. (2015). DAMP signaling is a key pathway inducing immune modulation after brain injury. J. Neurosci. 35, 583–598. doi: 10.1523/JNEUROSCI.2439-14.2015
Lim, D. A., Tramontin, A. D., Trevejo, J. M., Herrera, D. G., García-Verdugo, J. M., and Alvarez-Buylla, A. (2000). Noggin antagonizes BMP signaling to create a niche for adult neurogenesis. Neuron 28, 713–726. doi: 10.1016/s0896-6273(00)00148-3
Lin, S., Fan, L.-W., Pang, Y., Rhodes, P. G., Mitchell, H. J., and Cai, Z. (2005). IGF-1 protects oligodendrocyte progenitor cells and improves neurological functions following cerebral hypoxia-ischemia in the neonatal rat. Brain Res. 1063, 15–26. doi: 10.1016/j.brainres.2005.09.042
Liu, B. F., Gao, E. J., Zeng, X. Z., Ji, M., Cai, Q., Lu, Q., et al. (2006). Proliferation of neural precursors in the subventricular zone after chemical lesions of the nigrostriatal pathway in rat brain. Brain Res. 1106, 30–39. doi: 10.1016/j.brainres.2006.05.111
Lois, C., and Alvarez-Buylla, A. (1994). Long-distance neuronal migration in the adult mammalian brain. Science 264, 1145–1148. doi: 10.1126/science.8178174
Lois, C., García-Verdugo, J. M., and Alvarez-Buylla, A. (1996). Chain migration of neuronal precursors. Science 271, 978–981. doi: 10.1126/science.271.5251.978
Luo, F., Zhang, Z., Barnett, A., Bellinger, T. J., Turcato, F., Schmidt, K., et al. (2020). Cuprizone-induced demyelination under physiological and post-stroke condition leads to decreased neurogenesis response in adult mouse brain. Exp. Neurol. 326:113168. doi: 10.1016/j.expneurol.2019.113168
Maki, T., Liang, A., Miyamoto, N., Lo, E., and Arai, K. (2013). Mechanisms of oligodendrocyte regeneration from ventricular-subventricular zone-derived progenitor cells in white matter diseases. Front. Cell. Neurosci. 7:275. doi: 10.3389/fncel.2013.00275
Martini, S., Bernoth, K., Main, H., Ortega, G. D. C., Lendahl, U., Just, U., et al. (2013). A critical role for Sox9 in notch-induced astrogliogenesis and stem cell maintenance. Stem Cells 31, 741–751. doi: 10.1002/stem.1320
Martín-López, E., García-Marques, J., Núñez-Llaves, R., and López-Mascaraque, L. (2013). Clonal astrocytic response to cortical injury. PLoS One 8:e74039. doi: 10.1371/journal.pone.0074039
Menn, B., Garcia-Verdugo, J. M., Yaschine, C., Gonzalez-Perez, O., Rowitch, D., and Alvarez-Buylla, A. (2006). Origin of oligodendrocytes in the subventricular zone of the adult brain. J. Neurosci. 26, 7907–7918. doi: 10.1523/JNEUROSCI.1299-06.2006
Merkle, F. T., Mirzadeh, Z., and Alvarez-Buylla, A. (2007). Mosaic organization of neural stem cells in the adult brain. Science 317, 381–384. doi: 10.1126/science.1144914
Mizrak, D., Levitin, H. M., Delgado, A. C., Crotet, V., Yuan, J., Chaker, Z., et al. (2019). Single-cell analysis of regional differences in adult V-SVZ neural stem cell lineages. Cell Rep. 26, 394.e5–406.e5. doi: 10.1016/j.celrep.2018.12.044
Molofsky, A. V., Krenick, R., Ullian, E., Tsai, H., Deneen, B., Richardson, W. D., et al. (2012). Astrocytes and disease: a neurodevelopmental perspective. Genes Dev. 26, 891–907. doi: 10.1101/gad.188326.112
Morel, L., Chiang, M. S. R., Higashimori, H., Shoneye, T., Iyer, L. K., Yelick, J., et al. (2017). Molecular and functional properties of regional astrocytes in the adult brain. J. Neurosci. 37, 8706–8717. doi: 10.1523/JNEUROSCI.3956-16.2017
Morell, M., Tsan, Y., and O’Shea, K. S. (2015). Inducible expression of noggin selectively expands neural progenitors in the adult SVZ. Stem Cell Res. 14, 79–94. doi: 10.1016/j.scr.2014.11.001
Mundim, M. V., Zamproni, L. N., Pinto, A. A. S., Galindo, L. T., Xavier, A. M., Glezer, I., et al. (2019). A new function for Prokineticin 2: recruitment of SVZ-derived neuroblasts to the injured cortex in a mouse model of traumatic brain injury. Mol. Cell. Neurosci. 94, 1–10. doi: 10.1016/j.mcn.2018.10.004
Murcia-Belmonte, V., Esteban, P. F., Martínez-Hernández, J., Gruart, A., Luján, R., Delgado-García, J. M., et al. (2016). Anosmin-1 over-expression regulates oligodendrocyte precursor cell proliferation, migration and myelin sheath thickness. Brain Struct. Funct. 221, 1365–1385. doi: 10.1007/s00429-014-0977-4
Nait-Oumesmar, B., Decker, L., Lachapelle, F., Avellana-Adalid, V., Bachelin, C., and Baron-Van Evercooren, A. (1999). Progenitor cells of the adult mouse subventricular zone proliferate, migrate and differentiate into oligodendrocytes after demyelination. Eur. J. Neurosci. 11, 4357–4366. doi: 10.1046/j.1460-9568.1999.00873.x
Nait-Oumesmar, B., Picard-Riera, N., Kerninon, C., Decker, L., Seilhean, D., Höglinger, G. U., et al. (2007). Activation of the subventricular zone in multiple sclerosis: evidence for early glial progenitors. Proc. Natl. Acad. Sci. U.S.A. 104, 4694–4699. doi: 10.1073/pnas.0606835104
Namihira, M., Kohyama, J., Semi, K., Sanosaka, T., Deneen, B., Taga, T., et al. (2009). Committed neuronal precursors confer astrocytic potential on residual neural precursor cells. Dev. Cell 16, 245–255. doi: 10.1016/j.devcel.2008.12.014
Okada, S., Nakamura, M., Katoh, H., Miyao, T., Shimazaki, T., Ishii, K., et al. (2006). Conditional ablation of Stat3 or Socs3 discloses a dual role for reactive astrocytes after spinal cord injury. Nat. Med. 12, 829–834. doi: 10.1038/nm1425
Ortega, F., Gascón, S., Masserdotti, G., Deshpande, A., Simon, C., Fischer, J., et al. (2013). Oligodendrogliogenic and neurogenic adult subependymal zone neural stem cells constitute distinct lineages and exhibit differential responsiveness to Wnt signalling. Nat. Cell Biol. 15, 602–613. doi: 10.1038/ncb2736
Pekny, M., Pekna, M., Messing, A., Steinhäuser, C., Lee, J.-M., Parpura, V., et al. (2016). Astrocytes: a central element in neurological diseases. Acta Neuropathol. 131, 323–345. doi: 10.1007/s00401-015-1513-1
Pekny, M., Wilhelmsson, U., Tatlisumak, T., and Pekna, M. (2019). Astrocyte activation and reactive gliosis-A new target in stroke? Neurosci. Lett. 689, 45–55. doi: 10.1016/j.neulet.2018.07.021
Picard-Riera, N., Decker, L., Delarasse, C., Goude, K., Nait-Oumesmar, B., Liblau, R., et al. (2002). Experimental autoimmune encephalomyelitis mobilizes neural progenitors from the subventricular zone to undergo oligodendrogenesis in adult mice. Proc. Natl. Acad. Sci. U.S.A. 99, 13211–13216. doi: 10.1073/pnas.192314199
Pinto, L. I. G., Rodríguez, D., Adamo, A. M., and Mathieu, P. A. (2018). TGF-β pro-oligodendrogenic effects on adult SVZ progenitor cultures and its interaction with the Notch signaling pathway. Glia 66, 396–412. doi: 10.1002/glia.23253
Pous, L., Deshpande, S. S., Nath, S., Mezey, S., Malik, S. C., Schildge, S., et al. (2020). Fibrinogen induces neural stem cell differentiation into astrocytes in the subventricular zone via BMP signaling. Nat. Commun. 11:630. doi: 10.1038/s41467-020-14466-y
Radomski, K. L., Zhou, Q., Yi, K. J., and Doughty, M. L. (2013). Cortical contusion injury disrupts olfactory bulb neurogenesis in adult mice. BMC Neurosci. 14:142. doi: 10.1186/1471-2202-14-142
Remaud, S., Ortiz, F. C., Perret-Jeanneret, M., Aigrot, M.-S., Gothié, J.-D., Fekete, C., et al. (2017). Transient hypothyroidism favors oligodendrocyte generation providing functional remyelination in the adult mouse brain. eLife 6:e29996. doi: 10.7554/eLife.29996
Saha, B., Peron, S., Murray, K., Jaber, M., and Gaillard, A. (2013). Cortical lesion stimulates adult subventricular zone neural progenitor cell proliferation and migration to the site of injury. Stem Cell Res. 11, 965–977. doi: 10.1016/j.scr.2013.06.006
Scafidi, J., Hammond, T. R., Scafidi, S., Ritter, J., Jablonska, B., Roncal, M., et al. (2014). Intranasal epidermal growth factor treatment rescues neonatal brain injury. Nature 506, 230–234. doi: 10.1038/nature12880
Serwanski, D. R., Rasmussen, A. L., Brunquell, C. B., Perkins, S. S., and Nishiyama, A. (2018). Sequential contribution of parenchymal and neural stem cell-derived oligodendrocyte precursor cells toward remyelination. Neuroglia Basel Switz. 1, 91–105. doi: 10.3390/neuroglia1010008
Sloan, S. A., and Barres, B. A. (2014). Mechanisms of astrocyte development and their contributions to neurodevelopmental disorders. Curr. Opin. Neurobiol. 27, 75–81. doi: 10.1016/j.conb.2014.03.005
Sofroniew, M. V. (2020). Astrocyte reactivity: subtypes, states, and functions in CNS innate immunity. Trends Immunol. 41, 758–770. doi: 10.1016/j.it.2020.07.004
Sohn, J., Orosco, L., Guo, F., Chung, S.-H., Bannerman, P., and Mills, et al. (2015). The subventricular zone continues to generate corpus callosum and rostral migratory stream astroglia in normal adult mice. J. Neurosci. Off. J. Soc. Neurosci. 35, 3756–3763. doi: 10.1523/JNEUROSCI.3454-14.2015
Song, H. W., Kumar, B. K., Kim, S. H., Jeon, Y. H., Lee, Y. A., Lee, W. T., et al. (2011). Agmatine enhances neurogenesis by increasing ERK1/2 expression, and suppresses astrogenesis by decreasing BMP 2,4 and SMAD 1,5,8 expression in subventricular zone neural stem cells. Life Sci. 89, 439–449. doi: 10.1016/j.lfs.2011.07.003
Soundarapandian, M. M., Selvaraj, V., Lo, U.-G., Golub, M. S., Feldman, D. H., Pleasure, D. E., et al. (2011). Zfp488 promotes oligodendrocyte differentiation of neural progenitor cells in adult mice after demyelination. Sci. Rep. 1:2. doi: 10.1038/srep00002
Stolt, C. C., Lommes, P., Sock, E., Chaboissier, M.-C., Schedl, A., and Wegner, M. (2003). The Sox9 transcription factor determines glial fate choice in the developing spinal cord. Genes Dev. 17, 1677–1689. doi: 10.1101/gad.259003
Sullivan, G. M., Mierzwa, A. J., Kijpaisalratana, N., Tang, H., Wang, Y., Song, S.-K., et al. (2013). Oligodendrocyte lineage and subventricular zone response to traumatic axonal injury in the corpus callosum. J. Neuropathol. Exp. Neurol. 72, 1106–1125. doi: 10.1097/NEN.0000000000000009
Sun, W., Cornwell, A., Li, J., Peng, S., Osorio, M. J., Aalling, N., et al. (2017). SOX9 is an astrocyte-specific nuclear marker in the adult brain outside the neurogenic regions. J. Neurosci. 37, 4493–4507. doi: 10.1523/JNEUROSCI.3199-16.2017
Tong, C. K., Fuentealba, L. C., Shah, J. K., Lindquist, R. A., Ihrie, R. A., Guinto, C. D., et al. (2015). A dorsal SHH-dependent domain in the V-SVZ produces large numbers of oligodendroglial lineage cells in the postnatal brain. Stem Cell Rep. 5, 461–470. doi: 10.1016/j.stemcr.2015.08.013
Ung, K., Huang, T.-W., Lozzi, B., Woo, J., Hanson, E., Pekarek, B., et al. (2021). Olfactory bulb astrocytes mediate sensory circuit processing through Sox9 in the mouse brain. Nat. Commun. 12:5230. doi: 10.1038/s41467-021-25444-3
Vernerey, J., Macchi, M., Magalon, K., Cayre, M., and Durbec, P. (2013). Ciliary neurotrophic factor controls progenitor migration during remyelination in the adult rodent brain. J. Neurosci. 33, 3240–3250. doi: 10.1523/JNEUROSCI.2579-12.2013
Wang, Z., Zheng, Y., Zheng, M., Zhong, J., Ma, F., Zhou, B., et al. (2019). Neurogenic niche conversion strategy induces migration and functional neuronal differentiation of neural precursor cells following brain injury. Stem Cells Dev. 29, 235–248. doi: 10.1089/scd.2019.0147
Xing, Y. L., Röth, P. T., Stratton, J. A. S., Chuang, B. H. A., Danne, J., Ellis, S. L., et al. (2014). Adult neural precursor cells from the subventricular zone contribute significantly to oligodendrocyte regeneration and remyelination. J. Neurosci. Off. J. Soc. Neurosci. 34, 14128–14146. doi: 10.1523/JNEUROSCI.3491-13.2014
Xu, L., Sapolsky, R. M., and Giffard, R. G. (2001). Differential sensitivity of murine astrocytes and neurons from different brain regions to injury. Exp. Neurol. 169, 416–424. doi: 10.1006/exnr.2001.7678
Yang, Z., and Levison, S. W. (2006). Hypoxia/ischemia expands the regenerative capacity of progenitors in the perinatal subventricular zone. Neuroscience 139, 555–564. doi: 10.1016/j.neuroscience.2005.12.059
Young, K. M., Fogarty, M., Kessaris, N., and Richardson, W. D. (2007). Subventricular zone stem cells are heterogeneous with respect to their embryonic origins and neurogenic fates in the adult olfactory bulb. J. Neurosci. Off. J. Soc. Neurosci. 27, 8286–8296. doi: 10.1523/JNEUROSCI.0476-07.2007
Zaidi, A. U., Bessert, D. A., Ong, J. E., Xu, H., Barks, J. D. E., Silverstein, F. S., et al. (2004). New oligodendrocytes are generated after neonatal hypoxic-ischemic brain injury in rodents. Glia 46, 380–390. doi: 10.1002/glia.20013
Zhong, J., Zhao, L., Du, Y., Wei, G., Yao, W.-G., and Lee, W.-H. (2009). Delayed IGF-1 treatment reduced long-term hypoxia-ischemia-induced brain damage and improved behavior recovery of immature rats. Neurol. Res. 31, 483–489. doi: 10.1179/174313208X338133
Keywords: SVZ, neural stem cell, niche, astrocytes, oligodendrocytes, injury
Citation: David-Bercholz J, Kuo CT and Deneen B (2021) Astrocyte and Oligodendrocyte Responses From the Subventricular Zone After Injury. Front. Cell. Neurosci. 15:797553. doi: 10.3389/fncel.2021.797553
Received: 18 October 2021; Accepted: 15 November 2021;
Published: 24 December 2021.
Edited by:
Shinghua Ding, University of Missouri, United StatesReviewed by:
François D. Boussin, Université de Paris-Saclay, FranceShaoyu Ge, Stony Brook University, United States
Copyright © 2021 David-Bercholz, Kuo and Deneen. This is an open-access article distributed under the terms of the Creative Commons Attribution License (CC BY). The use, distribution or reproduction in other forums is permitted, provided the original author(s) and the copyright owner(s) are credited and that the original publication in this journal is cited, in accordance with accepted academic practice. No use, distribution or reproduction is permitted which does not comply with these terms.
*Correspondence: Benjamin Deneen, ZGVuZWVuQGJjbS5lZHU=