- 1Department of Otorhinolaryngology-Head and Neck Surgery, Zhongnan Hospital of Wuhan University, Wuhan, China
- 2Department of Otorhinolaryngology, Dawu County People's Hospital, Xiaogan, China
- 3Department of Biliary Pancreatic Surgery, Tongji Hospital, Tongji Medical College, Huazhong University of Science and Technology, Wuhan, China
- 4Division of Applied Physical Chemistry, Analytical Chemistry, Department of Chemistry, School of Engineering Sciences in Chemistry, Biotechnology and Health, Kungliga Tekniska Högskolan (KTH) Royal Institute of Technology, Stockholm, Sweden
- 5Key Laboratory of Applied Surface and Colloid Chemistry, Ministry of Education, School of Chemistry and Chemical Engineering, Shaanxi Normal University, Xi'an, China
Hearing loss is a common disease due to sensory loss caused by the diseases in the inner ear. The development of delivery systems for inner ear disease therapy is important to achieve high efficiency and reduce side effects. Currently, traditional drug delivery systems exhibit the potential to be used for inner ear disease therapy, but there are still some drawbacks. As nanotechnology is developing these years, one of the solutions is to develop nanoparticle-based delivery systems for inner ear disease therapy. Various nanoparticles, such as soft material and inorganic-based nanoparticles, have been designed, tested, and showed controlled delivery of drugs, improved targeting property to specific cells, and reduced systemic side effects. In this review, we summarized recent progress in nanocarriers for inner ear disease therapy. This review provides useful information on developing promising nanocarriers for the efficient treatment of inner ear diseases and for further clinical applications for inner ear disease therapy.
Introduction
Hearing loss is a common disease due to sensory loss that affects human health and life. According to World Health Organization (WHO) data, about 250 million patients suffered from hearing loss in 2005. By 2050, over 5% of the people in the world will suffer from hearing loss (World Health Organization). The production of hearing begins with the collection of sound waves by the outer ear. Then, the sound is transmitted to the hair cells of the inner ear through the middle ear. The inner ear of mammals consists of the vestibule, the semicircular canals, and the cochlea, which is responsible for hearing (Figure 1). The environmental factors, such as excessive acoustic stimulation, aging, infection, autoimmune inner ear diseases, and application of ototoxic drugs, will cause hearing disfunction in the inner ear, directly or indirectly resulting in damage to the cochlear sensory cells and/or related peripheral neurons (Staecker et al., 2001; Ross et al., 2016; Schilder et al., 2019).
The ways to deliver drugs into the inner ear include systemic circulation, from which drugs enter the inner ear through the labyrinth artery, and the round window membrane (RWM). However, current administering drugs have drawbacks, such as disorders, limited labyrinth artery supply, and difficulty in accessing RWM. For example, anti-inflammatory drugs are widely used for inner ear disease therapy; however, the short half-time of drugs in the cochlea that causes rapid elimination is the main problem. Scientists have tried different delivery systems, such as systemic drug delivery systems, intratympanic drug delivery systems, and hydrogel delivery systems, to deliver drugs into the inner ear to treat various inner ear diseases, such as Meniere's disease, autoimmune inner ear disease, and sudden sensorineural hearing loss (SHL) (Havia et al., 2002; Salt, 2005; Nakashima et al., 2016; Rathnam et al., 2019). However, there are drawbacks for each system. It is urgent to develop a new delivery system for high efficiency of inner ear disease therapy, high stability of the drugs before they reach the target cells (outer hair cells as OHC and inner hair cells as IHC) in the inner ear, and the ability to target delivery to the inner ear. Very recently, nanoparticle-based drug delivery systems appeared and attracted the attention of many scientists. Although these systems provide opportunities to solve current problems, there are many things that are unclear and need further investigation. Therefore, a review to summarize recent developments and drawbacks of current nanocarriers for inner ear disease therapy is needed.
In this review, we will summarize the nanocarrier systems for inner ear disease therapy, such as systemic systems, intratympanic systems, hydrogel systems, and nanocarrier systems. Then, we will summarize the soft materials and inorganic nanoparticles that can be used for inner ear disease therapy. Finally, we will conclude the advantages and current challenges of nanocarriers for inner ear disease therapy. This review will provide useful information on nanocarrier drug delivery systems for inner ear disease therapy, and these drug delivery systems could also be further used for other diseases.
Current Drug Delivery Systems For Inner Ear Disease Therapy
Delivery systems, including systemic drug delivery, intratympanic injection, hydrogel drug delivery, and nanocarrier drug delivery systems, have been used for inner ear disease therapy (Li et al., 2017; Kayyali et al., 2018; Hao and Li, 2019; Mittal et al., 2019; Rathnam et al., 2019; Gheorghe et al., 2021; Jaudoin et al., 2021). Among all current inner ear therapies, the intratympanic injection of liquid drugs is most widely used. Other delivery systems, such as hydrogel delivery systems and nanocarrier delivery systems, are also available. At present, we still need to overcome some barriers.
Systemic Drug Delivery
Inner ear diseases have been treated by systemic drug delivery systems via the oral route, intramuscular, or intravenous (Ruckenstein, 2004; Alexander et al., 2009; Buniel et al., 2009; McCall et al., 2010; Li et al., 2017). For example, corticosteroids are widely used to treat sudden SHL and have been found to be efficient with a recovery rate of 61%, which is much higher than using a placebo (recovery rate of 32%) demonstrated by Li and Ding (2020). Recently, it was reported that the recovery rates of SHL could reach up to 57–66% with oral corticosteroid (Filipo et al., 2014; Chen et al., 2015). But the limitations of these studies are the small number of patients and the relatively short term for investigation. A long-term course was found when treating autoimmune inner ear disease occurring over weeks to months (Buniel et al., 2009). Nevertheless, when using systemic administration, subtherapeutic local concentrations occur due to the limited blood supply in the inner ear and poor ability to cross the inner ear barrier. However, large doses lead to severe toxicities and undesirable side effects. For example, when aminoglycosides were used, they caused vestibulotoxicity and damage of cochlear, and SHL occurred due to high doses (Graham et al., 1984; Erol, 2007).
Intratympanic Drug Delivery
Intratympanic systems have also been widely used for inner ear disease therapy. The tympanic membrane is a thin membrane between the external and middle ear. For most of the substances, it is difficult to permeate the tympanic membrane, which is considered a barrier. However, the tympanic membrane is easy to be broken during injection of drugs into the middle ear. Taking steroids as an example, inner ear disease therapy was first treated by intratympanic delivery of steroids in the 1990s (Itoh and Sakata, 1991). At present, intratympanic delivery systems are used for the treatment of sudden sensorineural hearing loss (SHL), Meniere's disease, and vertigo (Chandrasekhar, 2001; Doyle et al., 2004). By using intratympanic delivery systems, the bony structure of the tympanic membrane could be prevented. Moreover, physical barriers, such as the round window membrane, and cellular barriers need to be overcome, when delivering drugs into the inner ear. Small molecules enter the inner ear through the RWM by passive diffusion, which will lead to different concentrations related to the location of the cochlea (Salt and Plontke, 2009). In addition, treatment efficacy during intratympanic injections for inner ear disease therapy will be strongly affected by the parameters, such as cone angle and depth of the tympanic membrane of the patients, and various biological, anatomical, and protocol effects (Volandri et al., 2011).
Hydrogel Delivery System
To overcome some drawbacks in intratympanic drug delivery systems, such as short residence time and difficulty in sustained release of drugs, hydrogel delivery systems, such as hydrophilic polymeric networks, have been developed for inner ear disease therapy. Chitosan-glycerophosphate hydrogel was developed for the first time to achieve sustained drug release and reduce the variation (Paulson et al., 2008). Chitosan-glycerophosphate hydrogel was a porous matrix and could be degraded by lysozymes to achieve the sustained release of drugs into the inner ear. This hydrogel delivery system showed a low risk of hearing loss and longer vestibular suppression (Xu et al., 2010). In hydrogel delivery systems, drugs are loaded in the hydrogel and located in a certain region, where the drugs can diffuse across the RWM and are released at meaningful concentrations (El Kechai et al., 2015). In contrast, hydrogels exhibit high viscosity and enable a higher residence time of drugs to reach equilibrium in the inner ear. Hydrogel systems could be designed by controlling the chemical composition of monomers and formed by both natural and synthetic products (Li and Mooney, 2016). Therefore, hydrogel delivery systems are potential for inner ear disease therapy due to good biocompatibility, easy functionality, high drug loading, and easy degradation (Ahmed, 2015).
Nanoparticle-Based Drug Delivery Systems For Inner Ear Disease Therapy
Although the delivery systems mentioned earlier could deliver regulated drugs into the inner ear, they face problems for the delivery of new types of drugs, such as biomolecules. Compared to the regulated drugs, these new types of drugs are less stable within the extracellular compartments. Therefore, it is difficult to deliver new types of drugs to the targeted locations or cells in the inner ear using the delivery systems discussed earlier. Novel drug delivery systems that could overcome these drawbacks are highly demanded in the treatment of inner ear diseases. Recently, various types of nanoparticles, such as nanosized polymer, peptides, silicas, and metal-organic frameworks (MOFs), have been widely employed as drug delivery systems for different kinds of therapies, such as anticancer therapies, anti-inflammatory, and antibacterial therapies. These nanocarriers have a particle size in the nano range, and the particle size could be controlled during the synthesis. For efficient drug delivery, a particle size of <300 nm is usually required to avoid opsonization and elimination (Ulbrich et al., 2016). They also possess a tunable surface with modifiable physicochemical properties for different applications. The nanoparticle-based drug delivery systems could increase the solubility of the drugs, protect the drugs from degradation, prolong the half-life of the drugs during circulation, and allow low passage of the loaded drugs across physiological barriers. Furthermore, these nanocarriers could protect drug properties from degradation, increase the solubility of drugs, difficulty in crossing physiological barriers. Nanoparticle-based delivery systems could also deliver a sustained release of drugs and provide targeted delivery to certain cells. Therefore, nanoparticle-based delivery systems have a great potential for inner ear disease therapy. Nanocarriers for inner ear disease therapy will be discussed in detail in the following section.
Soft Material Nanoparticle-Based Delivery System
Among different nanocarrier delivery systems that can be used for inner ear disease therapy, soft materials, such as polymeric, liposome, micelles, and lipid nanoparticle-based delivery systems, are widely used to load different kinds of drugs (Figure 2; Lu et al., 2011). These soft material nanoparticle-based delivery systems could increase the half-life of drugs and achieve sustained or targeted release of drugs. As demonstrated by Food and Drug Administration (FDA), poly(lactic-co-glycolic acid) (PLGA) was a biodegradable polymer and can be easily functionalized for target delivery. When being conjugated with rhodamine, the rhodamine-conjugated PLGA nanoparticles were observed in the cochlea of guinea pigs and long-term residence in the liver due to tissue-specific barriers and fast degradation of PLGA nanoparticles (Figure 3; Palao-Suay et al., 2015; Cai et al., 2017; Szeto et al., 2019). Then, scientists tried to decrease the particle size of the nanocarriers to the range of 150–300 nm, which would enhance the entry of nanoparticles into the inner ear. Further functionalization of the surface with pluronic F127 (PEO106-PPO70-PEO106) increased the accumulation of particles (Zou et al., 2010; Leso et al., 2019). PEGylated polymers could increase the half-life, biocompatibility, and solubility of the loaded drugs and decrease the side effects, as well as the immune reactions, to drugs (Veronese and Mero, 2008). The selected location of nanoparticles in the inner ear was also achieved by modifying the nanoparticles with chitosan, which changed the surface charge and hydrophilicity of the nanoparticles. These modifications could help nanoparticles reach the inner ear before endocytosis. Other types of polymeric nanoparticles, such as dendriplexes and chitosan-based nanoparticles, poly(L-lactic acid) (PLLA), PLLA-PEG, polycaprolactone (PCL), and polyethylene glycol (PEG), have also been used for inner ear disease therapy to encapsulate drugs via electrostatic interaction or hydrophobic-hydrophobic interaction (Dash et al., 2011; Wang et al., 2011; El Kechai et al., 2015; Lajud et al., 2015; Vigani et al., 2019).
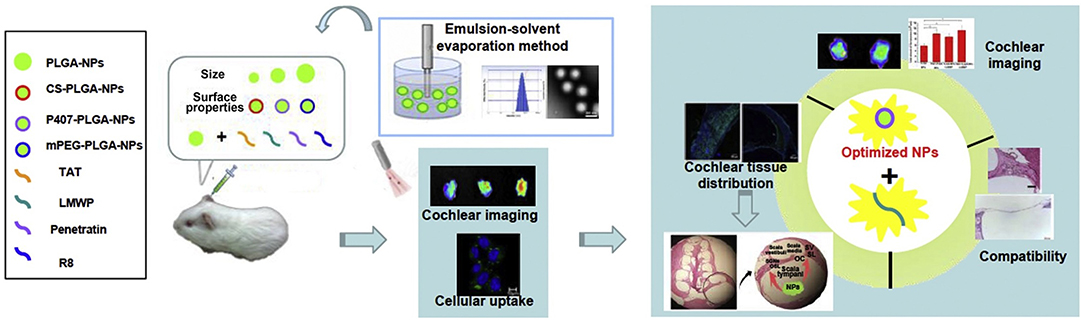
Figure 3. Poly(lactic-co-glycolic acid) (PLGA) nano-based systems with cell-penetrating peptides for cochlear drug delivery. Reprinted with permission from Cai et al. (2017). Copyright 2017 Published by Elsevier B.V.
Micelles and liposomes are formed by molecules with both hydrophobic and hydrophilic parts. Micelles have a hydrophobic environment inside micelles and enable the loading and delivery of hydrophobic drugs encapsulated inside, while the hydrophilic property of the outer surface of micelles increases their solubilization in aqueous solutions. Furthermore, micelle-based delivery systems could also protect unstable drugs from biological attacks during circulation. When liposome nanoparticles are used as delivery systems for inner ear disease therapy, both the external and internal surface of the liposome are hydrophilic with the same structure as the phospholipid bilayer (Panahi et al., 2017; Zylberberg et al., 2017). Amphiphilic liposomes can carry them across the RWM and deliver them into the cells (Uri et al., 2003; Meyer et al., 2012). Liposomes degrade readily in cells, resulting in low toxicity of liposomal drugs. Multifunctional liposome nanoparticles could be prepared by modifying the surface with polyethylene glycol, carbohydrates, and folic acid.
There are many other soft materials of nanometer size that could also be used for inner ear disease therapy. Cubosomes formed by a lipid core with a single lipid bilayer and a polymeric shell were very efficient for loading drugs (Barriga et al., 2019). Solid lipid nanoparticles and lipid nanocapsules have also been reported (Gao et al., 2015; Yang et al., 2018). Protein nanoparticles are another option to increase the delivery to the tissue and reduce the toxicity of compounds (Lohcharoenkal et al., 2014).
Inorganic Nanoparticle-Based Delivery System
Compared to soft materials, inorganic nanoparticle-based delivery systems are still in the developing stage. Inorganic nanoparticle-based delivery systems could provide unique properties, such as antimicrobial and magnetic properties (Figure 4). Although inorganic nanoparticles provide opportunities for inner ear disease therapy due to some useful qualities, there are many drawbacks, such as high price and limited biological stability.
Superparamagnetic Fe3O4 nanoparticles are a kind of inorganic material. These materials could pass the round window by magnetic force and arrive at the inner ear (Guigou et al., 2021). Superparamagnetic iron oxide nanoparticles are easy to synthesize and exhibit low toxicity and intrinsic antimicrobial activity for effective delivery systems (Rodrigues et al., 2019). Superparamagnetic iron oxide nanoparticles have no pores to encapsulate drug molecules, while in combination with polymers, such as PLGA and chitosan, superparamagnetic iron oxide nanoparticles could adsorb drugs via the electrostatic/hydrophobic interaction for inner ear drug delivery (Grumezescu et al., 2013; Sangaiya and Jayaprakash, 2018). Other metal-oxide-based nanoparticles have shown effective inner ear disease therapy-related infectious diseases. For example, TiO2 nanoparticles are photosensitive and show activity against fungi and bacteria (Luksiene, 2017). Zinc oxide, copper oxide, calcium oxide, silver oxide, aluminum oxide, and zirconium oxide have also been widely investigated as carriers for biomedical applications (Narayanan et al., 2012; Karimiyan et al., 2015; Swaminathan and Sharma, 2019). However, the particle size of these metal oxides is still difficult to control, and the target delivery systems using these metal oxides are needed to be designed to specifically locate nanoparticles in the inner ear.
Porous nanoparticles, such as mesoporous silica nanoparticles, and MOF nanoparticles possess pores for the encapsulation of both hydrophobic and hydrophilic drugs. These materials are usually easy to prepare, have large pore volumes, and can be easily functionalized. Mesoporous silica nanoparticles are biocompatible and could slowly degrade under physiological conditions (Zheng et al., 2011, 2013; Bernardos et al., 2019). These materials can be used to construct “smart” delivery systems, which are sensitive to changes in the environment, including changes to the pH, thermal, and magnetic fields. Recently, mesoporous silica nanoparticles could be used to control the encapsulation and release of antibiotics (Selvarajan et al., 2020). Furthermore, the surface of porous silica nanoparticles could be functionalized to target the designed tissues and used for inner ear disease therapy (Tang et al., 2012). When loaded with a brain-derived neurotrophic factor, porous silica nanoparticles could target the spiral ganglion neurons and release drugs for a long time period (Schmidt et al., 2018). More recently, MOFs have been designed and studied for many applications, including biomedical applications (Zheng et al., 2016; Kaneti et al., 2017; Wu and Yang, 2017; Lu et al., 2018). Recently, Xu et al. (2020) encapsulated methylprednisolone (MP) in ZIF-90 nanoparticles for the treatment of inner ear disease for the first time. ZIF-90 prevents the degradation of drugs during circulation after intraperitoneal injection and delivers MP into the inner ear. These MOF nanoparticles exhibit good protection from noise, low damage to the inner ear structure, and low nephrotoxicity during therapy.
Metallic and metal oxide nanoparticles are one group of the most promising inorganic materials for inner ear disease therapy (Paladini et al., 2015). Silver (Ag) nanoparticles could interact with the surface of bacterial cells and break the cell membranes to achieve permeability (Hamad et al., 2020). Ag nanoparticles could enter the inner ear after intraperitoneal injection and break pathogens with antibiotic formulations, which could overcome the drawbacks and achieve high efficacy in the ear therapy (Muhsin and Hachim, 2014; Zou et al., 2015). Similar situation happens to gold (Au) nanoparticles, which are used for loading drugs and imaging applications. Au nanoparticles have shown potential to be used as inner ear contrast agents and are located in cochlear cells (Kayyali et al., 2017). However, at present, Ag and Au nanoparticles have not yet been used for inner ear disease therapy. Quantum dots, such as semiconductor nanocrystals, show unique optical properties and are considered another option (Liu et al., 2010). Due to their advantages of good biocompatibility, biodistribution, stability, and long half-life, metallic and metal oxide nanoparticles and their combinations with polymers and/or proteins are highly potential for inner ear disease therapy in the near future.
Targeting Modification of Nanoparticle-Based Delivery System
Nanoparticles could increase circulation time and prevent the degradation of drugs. Moreover, a specific surface modification could further enable the nanoparticle-based drug delivery systems with target properties to reach a specific type of cell, which is ideal for inner ear therapies to deliver the drugs to a specific type of inner ear cell. Ligands have a specific interaction with certain cells and, thus, could be used for cell-specific targeting of nanoparticles for inner ear disease therapy (Frutos et al., 2018; Valero et al., 2018). For example, in an in vitro model prepared from the mouse cochleae, peptide-based nanoparticles could interact with spiral ganglion cells through tyrosine kinase and p75 neurotrophin receptors (Roy et al., 2010). Cy3-labeled silica nanoparticles were demonstrated to be located within the inner ear of RWM of mice compared to the control group of mice (Praetorius et al., 2007). Cy3-labeled silica nanoparticles could also reach central auditory nuclei and superior olive through retrograde axon transport. To target OHCs, prestin was connected to peptides and coupled to nanoparticles. The cellular uptake of these nanoparticles in the OHCs of rat cochleae was achieved (Surovtseva et al., 2012). Using a similar method, nanoparticles were found to be taken up by OHCs in vivo (Wang et al., 2018). Using this method, the nanoparticles were located into designed cells, and the uptake efficiency could also be improved.
Moreover, a deep understanding of a suitable ligand for targeting delivery is needed. The interaction between ligand-loaded nanoparticles, and the receptor in the inner ear should be further investigated. The targeting delivery system is also interesting and with high potential to be developed for inner ear disease therapy (Li et al., 2017).
Conclusion And Prospects
In this review, we summarized the currently used systems for inner ear disease therapy, including the most widely used delivery systems (i.e., systemic, intratympanic, and hydrogel delivery systems) and nanotechnology-based systems. To overcome the barriers of these systems in overcoming barriers in the inner ear, nanoparticle-based drug delivery systems, which have shown many advantages in the treatment of various diseases, have gained increasing attention in inner ear diseases during the past years. Several soft materials and inorganic-based nanoparticles have been investigated and shown to improve the efficiency of drugs, enhance antimicrobial performance, and reduce the side effects of inner ear disease therapy. Nanoparticles could cross the barrier in the inner ear, deliver drugs into the inner ear with low side effects, and remain harmless for healthy tissues. Furthermore, we discussed the targeting drug delivery systems using nanoparticles by modifying the surface with ligands, proteins, and so on. Developing multifunctional nanoparticles that could target specific cells and release drugs in a controlled manner is the way for the future.
Although nanocarriers have been used for inner ear disease therapy, there are still many works that need to be carried out in the future. First, the information on the interaction between nanoparticles and ear toxicity, such as effects on organs, is still unclear. The long-term investigation of health effects could be studied in the future. Second, the critical physicochemical characteristics that affect their biodistribution and the way to overcome physical and cellular barriers are not well-defined. The rational design of nanoparticles to achieve target delivery to the inner ear needs more effort. In summary, nanoparticle-based delivery systems have brought potential solutions and paved a novel way for inner ear disease therapy, but it still has a long way to go for real clinical applications.
Author Contributions
XX, JZ, YH, KL, SL, YZha, PS, YZho, and XC prepared this manuscript. YZho and XC guided all aspects of this work. All authors contributed to the article and approved the submitted version.
Funding
This work was supported by Swedish Research Council (2021-00295).
Conflict of Interest
The authors declare that the research was conducted in the absence of any commercial or financial relationships that could be construed as a potential conflict of interest.
Publisher's Note
All claims expressed in this article are solely those of the authors and do not necessarily represent those of their affiliated organizations, or those of the publisher, the editors and the reviewers. Any product that may be evaluated in this article, or claim that may be made by its manufacturer, is not guaranteed or endorsed by the publisher.
References
Ahmed, E. M. (2015). Hydrogel: preparation, characterization, and applications: a review. J. Adv. Res. 6, 105–121. doi: 10.1016/j.jare.2013.07.006
Alexander, T. H., Weisman, M. H., Derebery, J. M., Espeland, M. A., Gantz, B. J., Gulya, A. J., et al. (2009). Safety of high-dose corticosteroids for the treatment of autoimmune inner ear disease. Otol. Neurotol. 30, 443–448. doi: 10.1097/MAO.0b013e3181a52773
Barriga, H. M. G., Holme, M. N., and Stevens, M. M. (2019). Cubosomes: the next generation of smart lipid nanoparticles? Angew. Chem. Int. Ed. 58, 2958–2978. doi: 10.1002/anie.201804067
Bernardos, A., Piacenza, E., Sancenón, F., Hamidi, M., Maleki, A., Turner, R. J., et al. (2019). Mesoporous silica-based materials with bactericidal properties. Small 15:1900669. doi: 10.1002/smll.201900669
Buniel, M. C., Geelan-Hansen, K., Weber, P. C., and Tuohy, V. K. (2009). Immunosuppressive therapy for autoimmune inner ear disease. Immunotherapy 1, 425–434. doi: 10.2217/imt.09.12
Cai, H., Liang, Z., Huang, W., Wen, L., and Chen, G. (2017). Engineering PLGA nano-based systems through understanding the influence of nanoparticle properties and cell-penetrating peptides for cochlear drug delivery. Int. J. Pharm. 532, 55–65. doi: 10.1016/j.ijpharm.2017.08.084
Chandrasekhar, S. S. (2001). Intratympanic dexamethasone for sudden sensorineural hearing loss: clinical and laboratory evaluation. Otol. Neurotol. 22, 18–23. doi: 10.1097/00129492-200101000-00005
Chen, W. T., Lee, J. W., Yuan, C. H., and Chen, R. F. (2015). Oral steroid treatment for idiopathic sudden sensorineural hearing loss. Saudi Med. J. 36, 291–296. doi: 10.15537/smj.2015.3.9940
Dash, M., Chiellini, F., Ottenbrite, R. M., and Chiellini, E. (2011). Chitosan—a versatile semi-synthetic polymer in biomedical applications. Prog. Polym. Sci. 36, 981–1014. doi: 10.1016/j.progpolymsci.2011.02.001
Doyle, K. J., Bauch, C., Battista, R., Beatty, C., Hughes, G. B., Mason, J., et al. (2004). Intratympanic steroid treatment: a review. Otol. Neurotol. 25, 1034–1039. doi: 10.1097/00129492-200411000-00031
El Kechai, N., Agnely, F., Mamelle, E., Nguyen, Y., Ferrary, E., and Bochot, A. (2015). Recent advances in local drug delivery to the inner ear. Int. J. Pharm. 494, 83–101. doi: 10.1016/j.ijpharm.2015.08.015
Erol, S. (2007). Aminoglycoside-induced ototoxicity. Curr. Pharm. Des. 13, 119–126. doi: 10.2174/138161207779313731
Filipo, R., Attanasio, G., Russo, F. Y., Cartocci, G., Musacchio, A., De Carlo, A., et al. (2014). Oral versus short-term intratympanic prednisolone therapy for idiopathic sudden hearing loss. Audiol. Neurotol. 19, 225–233. doi: 10.1159/000360069
Frutos, S., Hernández, J. L., Otero, A., Calvis, C., Adan, J., Mitjans, F., et al. (2018). Site-specific antibody drug conjugates using streamlined expressed protein ligation. Bioconjugate Chem. 29, 3503–3508. doi: 10.1021/acs.bioconjchem.8b00630
Gao, G., Liu, Y., Zhou, C.-H., Jiang, P., and Sun, J.-J. (2015). Solid lipid nanoparticles loaded with edaravone for inner ear protection after noise exposure. Chin. Med. J. 128, 203–209. doi: 10.4103/0366-6999.149202
Gheorghe, D. C., Niculescu, A.-G., Bîrcă, A. C., and Grumezescu, A. M. (2021). Nanoparticles for the treatment of inner ear infections. Nanomaterials 11:1311. doi: 10.3390/nano11051311
Graham, M. D., Sataloff, R. T., and Kemink, J. L. (1984). Titration streptomycin therapy for bilateral Meniere's disease: a preliminary report. Otolaryngol. Head Neck Surg. 92, 440–447. doi: 10.1177/019459988409200413
Grumezescu, A. M., Andronescu, E., Holban, A. M., Ficai, A., Ficai, D., Voicu, G., et al. (2013). Water dispersible cross-linked magnetic chitosan beads for increasing the antimicrobial efficiency of aminoglycoside antibiotics. Int. J. Pharm. 454, 233–240. doi: 10.1016/j.ijpharm.2013.06.054
Guigou, C., Lalande, A., Millot, N., Belharet, K., and Bozorg Grayeli, A. (2021). Use of super paramagnetic iron oxide nanoparticles as drug carriers in brain and ear: state of the art and challenges. Brain Sci. 11:358. doi: 10.3390/brainsci11030358
Hamad, A., Khashan, K. S., and Hadi, A. (2020). Silver nanoparticles and silver ions as potential antibacterial agents. J. Inorg. Organomet. Polym. Mater. 30, 4811–4828. doi: 10.1007/s10904-020-01744-x
Hao, J., and Li, S. K. (2019). Inner ear drug delivery: recent advances, challenges, and perspective. Eur. J. Pharm. Sci. 126, 82–92. doi: 10.1016/j.ejps.2018.05.020
Havia, M., Kentala, E., and Pyykkö, I. (2002). Hearing loss and tinnitus in Meniere's disease. Auris Nasus Larynx 29, 115–119. doi: 10.1016/S0385-8146(01)00142-0
Itoh, A., and Sakata, E. (1991). Treatment of vestibular disorders. Acta Otolaryngol. 111, 617–623. doi: 10.3109/00016489109131486
Jaudoin, C., Agnely, F., Nguyen, Y., Ferrary, E., and Bochot, A. (2021). Nanocarriers for drug delivery to the inner ear: physicochemical key parameters, biodistribution, safety and efficacy. Int. J. Pharm. 592:120038. doi: 10.1016/j.ijpharm.2020.120038
Kaneti, Y. V., Dutta, S., Hossain, M. S. A., Shiddiky, M. J. A., Tung, K.-L., Shieh, F.-K., et al. (2017). Strategies for improving the functionality of zeolitic imidazolate frameworks: tailoring nanoarchitectures for functional applications. Adv. Mater. 29:1700213. doi: 10.1002/adma.201700213
Karimiyan, A., Najafzadeh, H., Ghorbanpour, M., and Hekmati-Moghaddam, S. H. (2015). Antifungal effect of magnesium oxide, zinc oxide, silicon oxide and copper oxide nanoparticles against Candida albicans. Zahedan J. Res. Med. Sci. 17:e2179. doi: 10.17795/zjrms-2179
Kayyali, M. N., Brake, L., Ramsey, A. J., Wright, A. C., O'Malley, B. W., and Li, D. D. (2017). A novel nano-approach for targeted inner ear imaging. J. Nanomed. Nanotechnol. 8:456. doi: 10.4172/2157-7439.1000456
Kayyali, M. N., Wooltorton, J. R. A., Ramsey, A. J., Lin, M., Chao, T. N., Tsourkas, A., et al. (2018). A novel nanoparticle delivery system for targeted therapy of noise-induced hearing loss. J. Controlled Release 279, 243–250. doi: 10.1016/j.jconrel.2018.04.028
Lajud, S. A., Nagda, D. A., Qiao, P., Tanaka, N., Civantos, A., Gu, R., et al. (2015). A novel chitosan-hydrogel-based nanoparticle delivery system for local inner ear application. Otol. Neurotol. 36, 341–347. doi: 10.1097/MAO.0000000000000445
Leso, V., Fontana, L., Ercolano, M. L., Romano, R., and Iavicoli, I. (2019). Opportunities and challenging issues of nanomaterials in otological fields: an occupational health perspective. Nanomedicine 14, 2613–2629. doi: 10.2217/nnm-2019-0114
Li, J., and Ding, L. (2020). Effectiveness of steroid treatment for sudden sensorineural hearing loss: a meta-analysis of randomized controlled trials. Ann. Pharmacother. 54, 949–957. doi: 10.1177/1060028020908067
Li, J., and Mooney, D. J. (2016). Designing hydrogels for controlled drug delivery. Nat. Rev. Mater. 1:16071. doi: 10.1038/natrevmats.2016.71
Li, L., Chao, T., Brant, J., O'Malley, B., Tsourkas, A., and Li, D. (2017). Advances in nano-based inner ear delivery systems for the treatment of sensorineural hearing loss. Adv. Drug Del. Rev. 108, 2–12. doi: 10.1016/j.addr.2016.01.004
Liu, J., Lau, S. K., Varma, V. A., Moffitt, R. A., Caldwell, M., Liu, T., et al. (2010). Molecular mapping of tumor heterogeneity on clinical tissue specimens with multiplexed quantum dots. ACS Nano 4, 2755–2765. doi: 10.1021/nn100213v
Lohcharoenkal, W., Wang, L., Chen, Y. C., and Rojanasakul, Y. (2014). Protein nanoparticles as drug delivery carriers for cancer therapy. Biomed Res. Int. 2014:180549. doi: 10.1155/2014/180549
Lu, K., Aung, T., Guo, N., Weichselbaum, R., and Lin, W. (2018). Nanoscale metal–organic frameworks for therapeutic, imaging, and sensing applications. Adv. Mater. 30:1707634. doi: 10.1002/adma.201707634
Lu, X.-Y., Wu, D.-C., Li, Z.-J., and Chen, G.-Q. (2011). “Chpater 7: Polymer nanoparticles,” in Progress in Molecular Biology and Translational Science, Vol. 104, ed Villaverde, A, . (Academic Press), 299–323. doi: 10.1016/B978-0-12-416020-0.00007-3
Luksiene, Z. (2017). “Chpater 16: Nanoparticles and their potential application as antimicrobials in the food industry,” in Food Preservation, ed Grumezescu, A. M. Grumezescu (Academic Press), 567–601. doi: 10.1016/B978-0-12-804303-5.00016-X
McCall, A. A., Swan, E. E. L., Borenstein, J. T., Sewell, W. F., Kujawa, S. G., and McKenna, M. J. (2010). Drug delivery for treatment of inner ear disease: current state of knowledge. Ear Hear. 31, 156–165. doi: 10.1097/AUD.0b013e3181c351f2
Meyer, H., Stöver, T., Fouchet, F., Bastiat, G., Saulnier, P., Bäumer, W., et al. (2012). Lipidic nanocapsule drug delivery: neuronal protection for cochlear implant optimization. Int. J. Nanomedicine 7, 2449–2464. doi: 10.2147/IJN.S29712
Mittal, R., Pena, S. A., Zhu, A., Eshraghi, N., Fesharaki, A., Horesh, E. J., et al. (2019). Nanoparticle-based drug delivery in the inner ear: current challenges, limitations and opportunities. Artif. Cell. Nanomed. B 47, 1312–1320. doi: 10.1080/21691401.2019.1573182
Muhsin, T. M., and Hachim, A. K. (2014). Mycosynthesis and characterization of silver nanoparticles and their activity against some human pathogenic bacteria. World J. Microbiol. Biotechnol. 30, 2081–2090. doi: 10.1007/s11274-014-1634-z
Nakashima, T., Pyykkö, I., Arroll, M. A., Casselbrant, M. L., Foster, C. A., Manzoor, N. F., et al. (2016). Meniere's disease. Nat. Rev. Dis. Primers 2:16028. doi: 10.1038/nrdp.2016.28
Narayanan, P. M., Wilson, W. S., Abraham, A. T., and Sevanan, M. (2012). Synthesis, characterization, and antimicrobial activity of zinc oxide nanoparticles against human pathogens. BioNanoScience 2, 329–335. doi: 10.1007/s12668-012-0061-6
Paladini, F., Pollini, M., Sannino, A., and Ambrosio, L. (2015). Metal-based antibacterial substrates for biomedical applications. Biomacromolecules 16, 1873–1885. doi: 10.1021/acs.biomac.5b00773
Palao-Suay, R., Aguilar, M. R., Parra-Ruiz, F. J., Fernández-Gutiérrez, M., Parra, J., Sánchez-Rodríguez, C., et al. (2015). Anticancer and antiangiogenic activity of surfactant-free nanoparticles based on self-assembled polymeric derivatives of vitamin E: structure–activity relationship. Biomacromolecules 16, 1566–1581. doi: 10.1021/acs.biomac.5b00130
Panahi, Y., Farshbaf, M., Mohammadhosseini, M., Mirahadi, M., Khalilov, R., Saghfi, S., et al. (2017). Recent advances on liposomal nanoparticles: synthesis, characterization and biomedical applications. Artif. Cell. Nanomed. B 45, 788–799. doi: 10.1080/21691401.2017.1282496
Paulson, D. P., Abuzeid, W., Jiang, H., Oe, T., O'Malley, B. W., and Li, D. (2008). A novel controlled local drug delivery system for inner ear disease. Laryngoscope 118, 706–711. doi: 10.1097/MLG.0b013e31815f8e41
Praetorius, M., Brunner, C., Lehnert, B., Klingmann, C., Schmidt, H., Staecker, H., et al. (2007). Transsynaptic delivery of nanoparticles to the central auditory nervous system. Acta Otolaryngol. 127, 486–490. doi: 10.1080/00016480600895102
Rathnam, C., Chueng, S.-T. D., Ying, Y.-L. M., Lee, K.-B., and Kwan, K. (2019). Developments in bio-inspired nanomaterials for therapeutic delivery to treat hearing loss. Front. Cell. Neurosci. 13:493. doi: 10.3389/fncel.2019.00493
Rodrigues, G. R., López-Abarrategui, C., de la Serna Gómez, I., Dias, S. C., Otero-González, A. J., and Franco, O. L. (2019). Antimicrobial magnetic nanoparticles based-therapies for controlling infectious diseases. Int. J. Pharm. 555, 356–367. doi: 10.1016/j.ijpharm.2018.11.043
Ross, A. M., Rahmani, S., Prieskorn, D. M., Dishman, A. F., Miller, J. M., Lahann, J., et al. (2016). Persistence, distribution, and impact of distinctly segmented microparticles on cochlear health following in vivo infusion. J. Biomed. Mater. Res. A 104, 1510–1522. doi: 10.1002/jbm.a.35675
Roy, S., Johnston, A. H., Newman, T. A., Glueckert, R., Dudas, J., Bitsche, M., et al. (2010). Cell-specific targeting in the mouse inner ear using nanoparticles conjugated with a neurotrophin-derived peptide ligand: potential tool for drug delivery. Int. J. Pharm. 390, 214–224. doi: 10.1016/j.ijpharm.2010.02.003
Ruckenstein, M. J.. (2004). Autoimmune inner ear disease. Curr. Opin. Otolaryngol. Head Neck Surg. 12, 426–430. doi: 10.1097/01.moo.0000136101.95662.aa
Salt, A. N., and Plontke, S. K. (2009). Principles of local drug delivery to the inner ear. Audiol. Neurootol. 14, 350–360. doi: 10.1159/000241892
Sangaiya, P., and Jayaprakash, R. (2018). A review on iron oxide nanoparticles and their biomedical applications. J. Supercond. Nov. Magn. 31, 3397–3413. doi: 10.1007/s10948-018-4841-2
Schilder, A. G. M., Su, M. P., Blackshaw, H., Lustig, L., Staecker, H., Lenarz, T., et al. (2019). Hearing protection, restoration, and regeneration: an overview of emerging therapeutics for inner ear and central hearing disorders. Otol. Neurotol. 40, 559–570. doi: 10.1097/MAO.0000000000002194
Schmidt, N., Schulze, J., Warwas, D. P., Ehlert, N., Lenarz, T., Warnecke, A., et al. (2018). Long-term delivery of brain-derived neurotrophic factor (BDNF) from nanoporous silica nanoparticles improves the survival of spiral ganglion neurons in vitro. PLoS ONE 13:e0194778. doi: 10.1371/journal.pone.0194778
Selvarajan, V., Obuobi, S., and Ee, P. L. R. (2020). Silica nanoparticles—a versatile tool for the treatment of bacterial infections. Front. Chem. 8:602. doi: 10.3389/fchem.2020.00602
Staecker, H., Li, D., O'Malley, B. W. Jr., and Van De Water, T. R. (2001). Gene expression in the mammalian cochlea: a study of multiple vector systems. Acta Otolaryngol. 121, 157–163. doi: 10.1080/000164801300043307
Surovtseva, E. V., Johnston, A. H., Zhang, W., Zhang, Y., Kim, A., Murakoshi, M., et al. (2012). Prestin binding peptides as ligands for targeted polymersome mediated drug delivery to outer hair cells in the inner ear. Int. J. Pharm. 424, 121–127. doi: 10.1016/j.ijpharm.2011.12.042
Swaminathan, M., and Sharma, N. K. (2019). “Antimicrobial activity of the engineered nanoparticles used as coating agents,” in Handbook of Ecomaterials, eds Martínez, L. M. T. Kharissova, O. V. and Kharisov, B. I. (Cham: Springer International Publishing), 549–563. doi: 10.1007/978-3-319-68255-6_1
Szeto, B., Chiang, H., Valentini, C., Yu, M., Kysar, J. W., and Lalwani, A. K. (2019). Inner ear delivery: challenges and opportunities. Laryngoscope Investig. Otolaryngol. 5, 122–131. doi: 10.1002/lio2.336
Tang, F., Li, L., and Chen, D. (2012). Mesoporous silica nanoparticles: synthesis, biocompatibility and drug delivery. Adv. Mater. 24, 1504–1534. doi: 10.1002/adma.201104763
Ulbrich, K., Holá, K., Šubr, V., Bakandritsos, A., Tuček, J., and Zboril, R. (2016). Targeted drug delivery with polymers and magnetic nanoparticles: covalent and noncovalent approaches, release control, and clinical studies. Chem. Rev. 116, 5338–5431. doi: 10.1021/acs.chemrev.5b00589
Uri, N., Doweck, I., Cohen-Kerem, R., and Greenberg, E. (2003). Acyclovir in the treatment of idiopathic sudden sensorineural hearing loss. Otolaryngol. Head Neck Surg. 128, 544–549. doi: 10.1016/S0194-5998(03)00004-4
Valero, T., Delgado-González, A., Unciti-Broceta, J. D., Cano-Cortés, V., Pérez-López, A. M., Unciti-Broceta, A., et al. (2018). Drug “clicking” on cell-penetrating fluorescent nanoparticles for in cellulo chemical proteomics. Bioconjugate Chem. 29, 3154–3160. doi: 10.1021/acs.bioconjchem.8b00481
Veronese, F. M., and Mero, A. (2008). The impact of PEGylation on biological therapies. Biodrugs 22, 315–329. doi: 10.2165/00063030-200822050-00004
Vigani, B., Rossi, S., Sandri, G., Bonferoni, M. C., Caramella, C. M., and Ferrari, F. (2019). Hyaluronic acid and chitosan-based nanosystems: a new dressing generation for wound care. Expert Opin. Drug Deliv. 16, 715–740. doi: 10.1080/17425247.2019.1634051
Volandri, G., Di Puccio, F., Forte, P., and Carmignani, C. (2011). Biomechanics of the tympanic membrane. J. Biomech. 44, 1219–1236. doi: 10.1016/j.jbiomech.2010.12.023
Wang, H., Shi, H.-B., and Yin, S.-K. (2011). Polyamidoamine dendrimers as gene delivery carriers in the inner ear: how to improve transfection efficiency. Exp. Ther. Med. 2, 777–781. doi: 10.3892/etm.2011.296
Wang, X., Chen, Y., Tao, Y., Gao, Y., Yu, D., and Wu, H. (2018). A666-conjugated nanoparticles target prestin of outer hair cells preventing cisplatin-induced hearing loss. Int. J. Nanomedicine 13, 7517–7531. doi: 10.2147/IJN.S170130
World Health Organization. Available online at: https://www.who.int/news-room/fact-sheets/detail/deafness-and-hearing-loss (accessed April 1 2021).
Wu, M.-X., and Yang, Y.-W. (2017). Metal–organic framework (MOF)-based drug/cargo delivery and cancer therapy. Adv. Mater. 29:1606134. doi: 10.1002/adma.201606134
Xu, L., Heldrich, J., Wang, H., Yamashita, T., Miyamoto, S., Li, A., et al. (2010). A controlled and sustained local gentamicin delivery system for inner ear applications. Otol. Neurotol. 31, 1115–1121. doi: 10.1097/MAO.0b013e3181eb32d1
Xu, X., Lin, K., Wang, Y., Xu, K., Sun, Y., Yang, X., et al. (2020). A metal–organic framework based inner ear delivery system for the treatment of noise-induced hearing loss. Nanoscale 12, 16359–16365. doi: 10.1039/D0NR04860G
Yang, K.-J., Son, J., Jung, S. Y., Yi, G., Yoo, J., Kim, D.-K., et al. (2018). Optimized phospholipid-based nanoparticles for inner ear drug delivery and therapy. Biomaterials 171, 133–143. doi: 10.1016/j.biomaterials.2018.04.038
Zheng, H., Gao, C., Peng, B., Shu, M., and Che, S. (2011). pH-responsive drug delivery system based on coordination bonding in a mesostructured surfactant/silica hybrid. J. Phys. Chem. C 115, 7230–7237. doi: 10.1021/jp110808f
Zheng, H., Xing, L., Cao, Y., and Che, S. (2013). Coordination bonding based pH-responsive drug delivery systems. Coord. Chem. Rev. 257, 1933–1944. doi: 10.1016/j.ccr.2013.03.007
Zheng, H., Zhang, Y., Liu, L., Wan, W., Guo, P., Nyström, A. M., et al. (2016). One-pot synthesis of metal–organic frameworks with encapsulated target molecules and their applications for controlled drug delivery. J. Am. Chem. Soc. 138, 962–968. doi: 10.1021/jacs.5b11720
Zou, J., Hannula, M., Misra, S., Feng, H., Labrador, R. H., Aula, A. S., et al. (2015). Micro CT visualization of silver nanoparticles in the middle and inner ear of rat and transportation pathway after transtympanic injection. J. Nanobiotechnol. 13:5. doi: 10.1186/s12951-015-0065-9
Zou, J., Zhang, W., Poe, D., Qin, J., Fornara, A., Zhang, Y., et al. (2010). MRI manifestation of novel superparamagnetic iron oxide nanoparticles in the rat inner ear. Nanomedicine 5, 739–754. doi: 10.2217/nnm.10.45
Keywords: nanocarrier, drug delivery system, inner ear disease therapy, soft material nanoparticle, inorganic nanoparticle
Citation: Xu X, Zheng J, He Y, Lin K, Li S, Zhang Y, Song P, Zhou Y and Chen X (2021) Nanocarriers for Inner Ear Disease Therapy. Front. Cell. Neurosci. 15:791573. doi: 10.3389/fncel.2021.791573
Received: 08 October 2021; Accepted: 28 October 2021;
Published: 03 December 2021.
Edited by:
Hai Huang, Tulane University, United StatesReviewed by:
Mingliang Tang, Southeast University, ChinaLei Xing, China Pharmaceutical University, China
Jieyu Qi, Southeast University, China
Copyright © 2021 Xu, Zheng, He, Lin, Li, Zhang, Song, Zhou and Chen. This is an open-access article distributed under the terms of the Creative Commons Attribution License (CC BY). The use, distribution or reproduction in other forums is permitted, provided the original author(s) and the copyright owner(s) are credited and that the original publication in this journal is cited, in accordance with accepted academic practice. No use, distribution or reproduction is permitted which does not comply with these terms.
*Correspondence: Yuye Zhou, eXV5ZUBrdGguc2U=; Xiong Chen, em5fY2hlbnhpb25nQDEyNi5jb20=
†These authors have contributed equally to this work