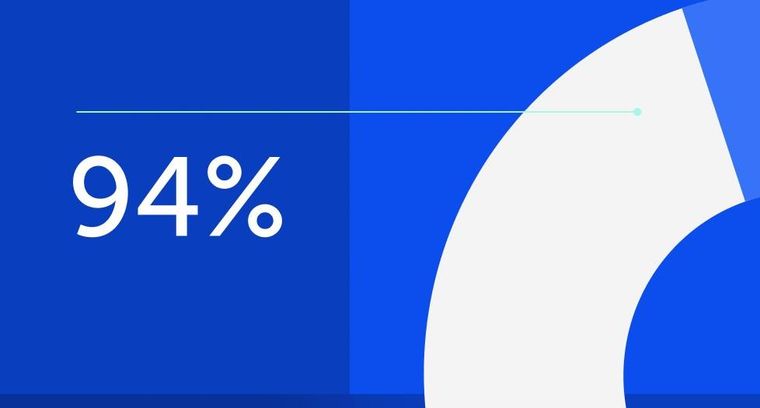
94% of researchers rate our articles as excellent or good
Learn more about the work of our research integrity team to safeguard the quality of each article we publish.
Find out more
REVIEW article
Front. Cell. Neurosci., 07 June 2021
Sec. Non-Neuronal Cells
Volume 15 - 2021 | https://doi.org/10.3389/fncel.2021.682888
This article is part of the Research TopicAstrocytes Ca2+ Signaling in the Modulation of Neural Networks Excitability and Synaptic TransmissionsView all 12 articles
Astroglia display a wide range of spontaneous and behavioral state-dependent Ca2+ dynamics. During heightened vigilance, noradrenergic signaling leads to quasi-synchronous Ca2+ elevations encompassing soma and processes across the brain-wide astroglia network. Distinct from this vigilance-associated global Ca2+ rise are apparently spontaneous fluctuations within spatially restricted microdomains. Over the years, several strategies have been pursued to shed light on the physiological impact of these signals including deletion of endogenous ion channels or receptors and reduction of intracellular Ca2+ through buffering, extrusion or inhibition of release. Some experiments that revealed the most compelling behavioral alterations employed chemogenetic and optogenetic manipulations to modify astroglia Ca2+ signaling. However, there is considerable contrast between these findings and the comparatively modest effects of inhibiting endogenous sources of Ca2+. In this review, we describe the underlying mechanisms of various forms of astroglia Ca2+ signaling as well as the functional consequences of their inhibition. We then discuss how the effects of exogenous astroglia Ca2+ modification combined with our knowledge of physiological mechanisms of astroglia Ca2+ activation could guide further refinement of behavioral paradigms that will help elucidate the natural Ca2+-dependent function of astroglia.
Astroglia are ubiquitous in the brain and exhibit a range of morphological structure adapted for each brain region. Star-shaped or protoplasmic astrocytes in gray matter possess minimal internal structure and are shaped by the surrounding neuropil, whereas fibrous astrocytes in white matter contain fibrils that assist integration within axon bundles (Köhler et al., 2019). Specialized astrocytes like retinal Müller cells and cerebellar Bergmann glia have distinct orientation that aligns with the stratification of nearby sensory cells and neurons, respectively (Siegel et al., 1991; Franze et al., 2007). Across all astroglia, their space-filling arrangement provides contact with a myriad of neural cell types including the vasculature (McCaslin et al., 2011). Astroglia perform dual functions of both isolating and modulating individual synaptic contacts, conceptualized in the tripartite synapse model (Araque et al., 1999), and also maintaining extensive intercellular communication through gap junctions producing an interconnected composition within the brain (Giaume and McCarthy, 1996; Theis and Giaume, 2012). Expression of numerous neurotransmitter receptors, ion channels, and transporters (Verkhratsky and Nedergaard, 2018) demonstrates that both anatomically and functionally, astroglia are primed for a role in sensing and dynamically modulating brain-wide neural networks. Astroglia do not fire action potentials and rather display a large and selective permeability to potassium, conferring the exceptionally negative resting membrane potential of −85 to −90 mV (Kuffler, 1967; Ransom and Goldring, 1973). High potassium conductance underlies their ability to buffer extracellular ions and allows modulation of neuronal activity (Wang et al., 2015; Weiss et al., 2019).
Aside from a relatively simple electrical constitution, astroglia abundantly express receptors and molecular components that produce a range of dynamic fluctuations in intracellular Ca2+. A list of many but not all membrane proteins enabling rise in astroglia intracellular Ca2+ is shown in Table 1. The following organizing principles were used: dependence on inositol-triphosphate receptor type 2 (IP3R2 protein or ITPR2 gene) – whether demonstrated or expected; in vivo characterization – a crucial factor given that even in the most conservative ex vivo preparation that is acute brain slice preparation, alterations in astroglia protein expression, morphology and Ca2+ dynamics can occur within less than 2 h of preparation (Takano et al., 2014); use of unanesthetized animal model – since general anesthesia strongly suppresses global astroglia Ca2+ elevations (Nimmerjahn et al., 2009; Thrane et al., 2012); and trigger of Ca2+ signal – to describe whether or not astroglia signaling pathways contributing to Ca2+ dynamics have been studied within their physiological dynamic range. Since in most cases it is very difficult if even possible to quantify the physiological dynamic range, for the tables we take a conservative approach and consider as “physiological” only astroglia Ca2+ dynamics that occur spontaneously or are driven by sensory stimulation or behavioral state, which we distinguish from electrical stimulation of an axonal fiber tract, application of a receptor agonist, or activation of an exogenously expressed receptor or channel. Various exogenous proteins for manipulation of astroglia intracellular Ca2+ are further explored in Table 2. We follow these concepts in order to emphasize the importance of using in vivo experimental paradigms that preserve or at least mimic the natural condition in order to understand which properties among the many purported abilities of astrocytes are physiologically employed. For each experiment, if concepts two or three are met, they are represented in green, and if not, they are represented in orange or without color. The more concepts are represented in green, we consider the respective finding to demonstrate a realized astroglia function. Conversely, the fewer concepts are represented in green, we consider that the respective finding may indicate a potential astroglia function.
We intentionally do not categorize astroglia Ca2+ dynamics by somatic or process location since, as we will discuss, a considerable portion of process Ca2+ elevations share the underlying mechanism with somatic Ca2+ elevations. Also, it is noted that astroglia Ca2+ signaling has been tightly associated with the concept of gliotransmission, which is the Ca2+-dependent vesicular release of signaling molecules from astroglia (Araque et al., 2014). The existence and/or significance of this concept still faces controversy (Fiacco and McCarthy, 2018; Savtchouk and Volterra, 2018). It is beyond the reach of this review to resolve this controversy. Instead, we take an agnostic glial neuromodulation view at what has been found about astroglia Ca2+ dynamics in living animals and how it is or may be linked to neuronal activity and behavior.
In this review, we describe the various forms of astroglia Ca2+ signaling distinguishing between (i) whole-cell, behavior-associated signals as well as (ii) spatially localized microdomain activity, and (iii) the functional consequences of their inhibition. We then discuss (iv) what effects of exogenous astroglia Ca2+ activation have been identified that could guide further refinement of behavioral paradigms to study the natural function of astroglia.
Relating mammalian astroglia Ca2+ dynamics directly to behavioral state became possible with the introduction of an approach that allows study of cellular brain activity in head-fixed awake mice on a spherical treadmill using two-photon microscopy (Dombeck et al., 2007). It was found that protoplasmic astrocytes in the somatosensory cortex produce Ca2+ transients that correlate with bouts of motor activity. The head-fixation two-photon microscopy paradigm was soon adopted to study cerebellar Bergmann glia (Nimmerjahn et al., 2009). Three distinct types of Ca2+ signals were defined in this seminal study. First, ‘bursts’ are spontaneous radially spreading Ca2+ elevations that depend on purinergic signaling and can also be detected in anesthetized rats and mice (Hoogland et al., 2009). Since they are not at all, or extremely rarely detected in awake mice through chronic cranial windows after sufficient tissue recovery from surgery (Paukert et al., 2014), they may be triggered by trauma-associated ATP release. Second, ‘sparkles’ are spontaneous, low-frequency Ca2+ elevations that occur asynchronously in individual Bergmann glia process domains and persist at lower frequency under isoflurane anesthesia. These events may correspond to microdomain Ca2+ activity in protoplasmic astrocytes which will be discussed below. Finally, ‘flares’ are a robust, whole-cell Ca2+ transient encompassing Bergmann glia in the entire field of view, are detectable only in awake mice, and relate to the onset of motor activity.
The underlying mechanism of this motor activity-associated astroglia Ca2+ elevation remained elusive in these initial studies. It is now known that locomotion is associated with sufficient arousal to trigger the release of norepinephrine and acetylcholine. In mouse primary visual cortex for example, noradrenergic signaling is responsible for tonic depolarization of the membrane potential of layer 2/3 and 4 excitatory neurons as well as interneurons during locomotion (Polack et al., 2013). Locomotion also evokes a Ca2+ rise in vasoactive intestinal peptide (VIP)-positive neurons, which depends on activation of nicotinic acetylcholine receptors (Fu et al., 2014). Finally, direct observation of terminal Ca2+ dynamics was accomplished by expression of the genetically-encoded Ca2+ indicator (GECI) GCaMP6s in mouse noradrenergic and cholinergic neurons, respectively. Locomotion is accompanied by Ca2+ elevations in both types of terminals in primary visual and auditory cortices (Reimer et al., 2016).
Electrical stimulation of brainstem nuclei in anesthetized mice revealed the powerful potential of neuromodulators for coordinated Ca2+ activation of astroglia. Stimulation of locus coeruleus triggers norepinephrine release and leads to subsequent Ca2+ activation of somatosensory cortex astrocytes (Bekar et al., 2008). Stimulation of nucleus basalis of Meynert induces acetylcholine release and causes a Ca2+ activation of astrocytes in somatosensory cortex (Takata et al., 2011), primary visual cortex (Chen et al., 2012), and hippocampus (Navarrete et al., 2012). Consistent with the idea that vigilance-dependent astroglia Ca2+ activation may be mediated by norepinephrine, it was found that locomotion as well as isometric muscle contractions, such as during a freezing response, induce widespread Ca2+ elevations in mouse cerebellar Bergmann glia, an occurrence previously described as ‘flares.’ Vigilance-dependent Bergmann glia responses are highly correlated with astrocyte Ca2+ elevations in primary visual cortex (Paukert et al., 2014). In the same study, pharmacological experiments revealed the importance of α1-adrenergic receptors for Bergmann glia Ca2+ activation. α1-adrenergic receptors had been independently found to be required for whisker stimulation- and air puff-induced Ca2+ activation of mouse somatosensory cortex astrocytes (Ding et al., 2013). Notably, locomotion-induced widespread Bergmann glia Ca2+ elevations have been studied in the molecular layer of cerebellar cortex where cross-sections of Bergmann glia processes are captured (Nimmerjahn et al., 2009; Paukert et al., 2014). Consistent with this finding, locomotion/startle-induced Ca2+ elevations in cortical astrocytes encompass processes in addition to somata (Paukert et al., 2014; Srinivasan et al., 2015; Ye et al., 2017; King et al., 2020).
Recently, use of global as well as cell type-specific gene deletion revealed that α1A-adrenergic receptors on Bergmann glia are required for vigilance-dependent Ca2+ activation (Ye et al., 2020). The generated conditional knockout mouse line in combination with the inducible Aldh1l1−CreERT2 mouse line (Srinivasan et al., 2016) led to a loss of >94% of Adra1a mRNA in the cerebellum, indicating that within the cerebellum, α1A-adrenergic receptors are potentially exclusively expressed in astroglia. Despite almost complete loss of Bergmann glia Ca2+ elevations in the global Adra1a knockout mouse, the loss of responsiveness in the conditional mouse more than 1 month following Cre recombination was approximately 40%, suggesting an extended stability of some receptors and highlights an obstacle for the interpretation of negative behavioral data. An independent study found that knock-out of α1A-adrenergic receptors in mouse Hes5-positive astrocytes of superficial laminae of the spinal dorsal horn strongly reduced Ca2+ elevations in those cells in response to intraplantar injection of capsaicin (Kohro et al., 2020).
Noradrenergic signaling to astroglia is evolutionarily highly conserved. Indeed, the first experimental evidence for direct signaling of neuromodulators through astroglia was obtained from studying Drosophila astrocytes (Ma et al., 2016). Olfactory-driven larval chemotaxis as well as touch-induced startle responses induce somatic Ca2+ elevations in astrocytes that are mediated by the release of the Drosophila norepinephrine analogs tyramine and octopamine from Tdc2-positive neurons through binding to their receptor on astrocytes. In zebrafish, radial astrocytes accumulate information about futile motor activity through α1B-adrenergic receptors (Mu et al., 2019). Astroglia Ca2+ elevations encompassing whole cells can also occur spontaneously. In the developing mouse cochlea, clusters of supporting cells undergo Ca2+ elevations that depend on the activation of P2Y1 receptors (Tritsch et al., 2007; Babola et al., 2020). Astroglia express many more G protein-coupled receptors (Kofuji and Araque, 2020). Most if not all of them contribute to the diverse pool of astroglia microdomain Ca2+ dynamics that will be discussed in the next section. It is currently unclear and awaits further investigation whether they also modulate norepinephrine-mediated, vigilance-dependent global astroglia Ca2+ elevations, independently cause global astroglia Ca2+ elevations in yet to be defined behavioral context, or exclusively contribute to microdomain activity.
The astroglia network is anatomically complex and dynamic. A single astroglia contacts multiple neuronal cell bodies, hundreds of processes, and tens of thousands of synapses (Ventura and Harris, 1999). In addition, a considerable body of experimental findings suggests functional interactions between astrocytes and neurons at the synaptic level, termed gliotransmission, most of which have been determined to depend on astrocyte Ca2+ elevations (Araque et al., 2014). This feature has inspired investigations in subcellular, localized Ca2+ dynamics restricted to small portions of astroglia processes. First observed in acute cerebellar slices, ‘microdomain’ Ca2+ transients in Bergmann glia processes occur spontaneously or in response to electrical stimulation of parallel fibers, which are glutamatergic axons of granule cells passing by Purkinje cell dendrites, molecular layer interneurons and Bergmann glia processes (Grosche et al., 1999). Bergmann glia express GluA2 lacking, Ca2+-permeable α-amino-3-hydroxy-5-methyl-4-isoxazolepropionic acid (AMPA) type glutamate receptors (Burnashev et al., 1992; Müller et al., 1992) and the Gq protein-coupled purinergic receptor P2Y1 (Rudolph et al., 2016). The fast component of Ca2+ elevation following parallel fiber stimulation has been ascribed to AMPA receptors (Piet and Jahr, 2007) and a second slower component of Ca2+ elevation depends on mGlu1-dependent release of ATP from molecular layer interneurons (Beierlein and Regehr, 2006; Piet and Jahr, 2007).
In awake mice, the Bergmann glia Ca2+ signal equivalent of microdomain events was previously termed ‘sparkles’ (Nimmerjahn et al., 2009). They are spontaneous Ca2+ elevations spatially restricted to individual main process branches that anatomically appear as palisades. Sparkles persist at a lower frequency during inhibition of action potential firing with tetrodotoxin (TTX), during inhibition of glutamatergic receptors, and during isoflurane general anesthesia. These features suggest that a considerable portion of sparkles resemble intrinsically-generated Ca2+ elevations that have been previously described in cortical astrocytes of anesthetized mice (Takata and Hirase, 2008).
Two major experimental obstacles have limited the appreciation of microdomain Ca2+ dynamics. The paucity in cytosolic volume of the finest astroglia processes limits the availability of synthetic Ca2+ indicators as well as cytosolic GECIs in those compartments and reduces the signal-to-noise level. The other difficulty is in distinguishing between a microdomain Ca2+ event and global Ca2+ signaling events spreading into the microdomain’s spatial territory. For instance, locomotion-associated ‘flares’ prevent determining if ‘sparkles’ are enhanced during locomotion (Nimmerjahn et al., 2009). A significant advancement came with the introduction of membrane-tethered GECIs (Shigetomi et al., 2010a,b), which detect miniature Ca2+ fluctuations in astroglia localized near the plasma membrane and within the finest processes with only minimum volume of cytoplasm. These ‘spotty’ Ca2+ transients have more variable kinetics including faster responses than global vigilance-dependent responses described above, and occur asynchronously (Srinivasan et al., 2015; Agarwal et al., 2017). Some asynchrony of microdomain Ca2+ events may be explained by the biochemical compartmentation of nodal structural elements often localized in proximity to dendritic spines, a discovery recently made with super-resolution microscopy within the meshwork of astrocyte fine processes (Arizono et al., 2020).
By expressing the membrane-anchored GECI in acute hippocampal slices and organotypic culture, respectively, it was deduced that TRPA1 is required for astrocyte microdomain responses and for setting the basal Ca2+ level (Shigetomi et al., 2011, 2013; Jackson and Robinson, 2015). Using transgenic mice expressing a membrane-anchored GECI in astrocytes confirmed that TRPA1 contributes to the basal hippocampal astrocyte Ca2+ level, but transient microdomain Ca2+ events are not sensitive to pharmacological inhibition of TRPA1, Ca2+ release-activated channels (CRACs), ryanodine receptors, voltage-dependent Ca2+ channels or Na+-Ca2+ exchangers (Agarwal et al., 2017). Instead, consistent with the considerable subcellular co-localization of microdomain Ca2+ events and mitochondria (Jackson and Robinson, 2015; Agarwal et al., 2017), inhibition of the mitochondrial permeability transition pore (mPTP) dramatically reduces the frequency of microdomain Ca2+ events (Agarwal et al., 2017). Stochastic opening of the mPTP causes brief bursts of reactive oxygen species (ROS) production in mitochondria (Wang et al., 2008). In turn, excessive ROS production can open mPTP (Vercesi et al., 1997; Duchen, 2000). Remarkably, microdomain Ca2+ events, like global, vigilance-dependent Ca2+ responses, are also evolutionarily conserved. In Drosophila astrocytes, ROS-regulated TrpML is responsible for transient microdomain Ca2+ elevations (Ma and Freeman, 2020). ROS facilitate IP3R-mediated Ca2+ release from stores (Bánsághi et al., 2014), and further promote mPTP opening (Agarwal et al., 2017).
IP3R2 plays an important role for microdomain Ca2+ events because the frequency of those events is strongly reduced in ITPR2–/– mice (Srinivasan et al., 2015; Agarwal et al., 2017). In the absence of any circuit stimulation, IP3R-dependent facilitation of microdomain Ca2+ events appears to be not dependent on vesicular neurotransmitter release since incubation with veratridine and bafilomycin A1, for depleting vesicle content, does not affect the frequency of those events (Agarwal et al., 2017). On the other hand, it has been demonstrated that electrical stimulation of individual hippocampal axons in acute slices can evoke microdomain Ca2+ elevations (Bindocci et al., 2017) and that whisker stimulation, air puff stimulation, and locomotion can facilitate microdomain Ca2+ events (Srinivasan et al., 2015; Agarwal et al., 2017; Stobart et al., 2018). Certainly, it needs to be noted that, as mentioned above, it may be difficult to distinguish between highly coordinated microdomain Ca2+ activity and predominantly IP3R2-dependent global whole cell Ca2+ activity. Intriguingly, sensory stimulation and arousal can enhance microdomain activity even in ITPR2–/– mice, and norepinephrine can still enhance microdomain event frequency in the absence of IP3R2 (Agarwal et al., 2017) but the α1-adrenergic receptor antagonist prazosin does not inhibit this enhancement (Srinivasan et al., 2015). Thus, if this is a direct effect of norepinephrine on astrocytes through non-type 2 IP3 receptors (Sherwood et al., 2017), it is possible that α2A-adrenergic receptors, whose mRNA is expressed in astrocytes (Zhang et al., 2014), could contribute to facilitation of microdomain Ca2+ events.
The most convincing and complete evidence if and how astroglia Ca2+ dynamics influence circuit activity and behavior stems from experimental approaches that allow a combination of the following three components: (1) visualization of astroglia Ca2+ dynamics in awake organisms during behavior, (2) reduction or abolishment of the behavior-dependence of Ca2+ dynamics through astroglia-specific genetic deletion of a signaling pathway component, and (3) the detection of consequences for circuit activity and/or behavior.
Employing such a three-component approach has revealed the crucial role of noradrenergic signaling in the context of widespread whole-astrocyte Ca2+ elevations. Its importance is further demonstrated by its evolutionary conservation. Sensory stimulation (olfaction) or startling touch of Drosophila larvae triggers release of the Drosophila adrenergic transmitter analogs octopamine and tyramine from Tdc2-positive neurons (Ma et al., 2016). Octopamine/tyramine (Oct/Tyr) bind directly to astrocyte Oct/TyrR causing extracellular Ca2+ influx through the transient receptor potential (TRP) channel Water witch (Wtrw). Somatic astrocyte Ca2+ elevation, through an unknown mechanism, potentially causes release of ATP, which following breakdown to adenosine, activates the inhibitory AdoR on dopaminergic neurons, relieving chemotaxis and startle-induced reversal behaviors from dopaminergic inhibition (Ma et al., 2016). Notably, this study provided the first experimental evidence that neuromodulators can signal directly through astroglia. In addition to Drosophila, futile motor activity in zebrafish causes incremental Ca2+ elevations in radial astrocytes ultimately leading to a ‘giving up’ change in behavioral state (Mu et al., 2019). Mismatch between swimming as motor action and visual virtual feedback, a paradigm previously employed in studies of mouse primary visual cortex (Keller et al., 2012), activates noradrenergic neurons and leads to a delayed global Ca2+ elevation in radial astrocytes. This astrocyte Ca2+ activation is dependent on α1B-adrenergic receptors and through the combination of opto-/chemogenetics and laser cell ablation it has been found that astrocyte Ca2+ activation, through a yet unknown mechanism, activates inhibitory neurons that trigger a cessation of swimming attempts (Mu et al., 2019).
In mouse cerebellar Bergmann glia, it is α1A-adrenergic receptors that are responsible for vigilance-dependent Ca2+ activation (Ye et al., 2020). Remarkably, despite almost complete loss of locomotion-induced Bergmann glia Ca2+ elevations in global Adra1a knockout mice, the mice do not display any motor coordination deficit. Consistent with this finding, acute exposure to ethanol (2 g/kg i.p.), which suppresses locomotion-induced norepinephrine release, results in considerable loss of Bergmann glia Ca2+ responsiveness that lasts for more than 45 min whereas ataxic motor coordination, present 15 min after onset of exposure to ethanol, is completely recovered 30 min later (Ye et al., 2020). These findings suggest that vigilance-dependent Bergmann glia global Ca2+ elevations may play a role in cognitive function of the cerebellum rather than motor coordination; however, it needs to be noted that direct evidence for this is still missing.
An independently developed Adra1a conditional knockout mouse line was used to investigate the role of α1A-adrenergic receptors in Hes5-positive astrocytes in superficial laminae of the spinal dorsal horn (supSDH) (Kohro et al., 2020). Although the completeness of reduction of ipsilateral, intraplantar capsaicin-induced Hes5-positive supSDH astrocyte Ca2+ elevation in Adra1a conditional knockout mice was not tested, strikingly, it was found that mechanosensory hypersensitivity was abolished. The precise mechanism how α1A-adrenergic receptor activation in Hes5-positive supSDH astrocytes triggers mechanosensory hypersensitivity remains elusive. One possible mechanism is based on the concept of gliotransmission where D-serine, besides glutamate and ATP, is the most frequently proposed signaling molecule (Yang et al., 2003; Panatier et al., 2006; Henneberger et al., 2010), but see Wolosker et al. (2016), Mothet et al. (2019). D-serine may function as a diffusible messenger following exogenous activation of P2Y1 receptors on lamina I SDH astrocytes to spread gliogenic long-term potentiation (LTP) beyond individual synapses (Kronschläger et al., 2016). It is conceivable that α1A-adrenergic receptors in Hes5-positive supSDH astrocytes could substitute the experimental role of P2Y1 receptors and control D-serine signaling. Indeed, Kohro et al. (2020) found that exogenously administered D-serine is sufficient to cause mechanosensory hypersensitivity. However, they did not detect extracellular elevations of D-serine in Hes5-positive supSDH astrocytes even following the drastic activation of a Designer Receptors Exclusively Activated by Designer Drugs (DREADD), specifically the humanized Gq protein-coupled muscarinic receptor type 3-based DREADD (hM3Dq). This finding could either mean that even excessive Ca2+ elevation in Hes5-positive supSDH astrocytes does not modulate extracellular D-serine signaling, or it could suggest that the detection method was not sensitive enough to take advantage of the Adra1a conditional knockout mouse line to imperatively link capsaicin-induced, noradrenergic Ca2+ activation of Hes5-positive supSDH astrocytes to D-serine signaling.
Robust noradrenergic signaling to astroglia in awake mice establishes a translational potential. Monai et al. (2016) found that a 10 min episode of transcranial direct current stimulation (tDCS), which is employed to ameliorate symptoms in patients of major depression among other neuropsychiatric and neurological conditions, when applied to mouse visual cortex, induces an α1-adrenergic receptor-dependent widespread, global Ca2+ elevation in astrocytes but not in neurons (Monai et al., 2016). Despite the absence of tDCS-induced neuronal Ca2+ elevation, a single 10 min tDCS application is sufficient for IP3R2-dependent induction of an hours-lasting potentiation of visually evoked potentials.
For many astroglia receptors it remains to be defined in which awake behavioral context they are activated. This requires investigation of whether they lead to global astroglia Ca2+ activation like noradrenergic receptors, whether they potentiate noradrenergic responses, or whether they exclusively contribute to microdomain Ca2+ events. For example, there is strong evidence that electrical stimulation of cholinergic nuclei and fibers in anesthetized mice leads to muscarinic receptor-dependent global Ca2+ elevation in somatosensory cortex, primary visual cortex and hippocampal astrocytes affecting local circuit plasticity (Takata et al., 2011; Chen et al., 2012; Navarrete et al., 2012). Given that cholinergic signaling is engaged during states of heightened vigilance (Polack et al., 2013; Fu et al., 2014; Reimer et al., 2016), it is somewhat surprising that no widespread, heightened vigilance-dependent global cortical astrocyte Ca2+ elevations persist following pharmacological inhibition of α1-adrenergic receptors (Ding et al., 2013; Srinivasan et al., 2015). Also, indirect evidence from a detailed study of sleep states suggests that acetylcholine may not directly lead to a Ca2+ rise in cortical astrocytes (Bojarskaite et al., 2020) though a fraction of somatosensory cortex astrocyte microdomain Ca2+ events in awake mice is sensitive to the muscarinic receptor antagonist atropine (Stobart et al., 2018). This highlights that caution is warranted when electrical stimulation of fiber tracts is employed. For instance, with cerebellar granule cell-Purkinje cell glutamatergic synapses, whether one stimulates bundles of neighboring axons in the molecular layer or whether one excites dispersed axons by stimulating in the granule cell layer determines if glutamate spillover leads to endocannabinoid-dependent short-term plasticity (Marcaggi and Attwell, 2005). For this reason, in Tables 1, 2 we emphasize the difference between behavior-induced (sensory stimulation or behavioral state) versus electrical stimulation- or excitatory opto- or chemogenetic stimulation-induced astroglia Ca2+ dynamics.
A very elegant study revealed that wakefulness/vigilance-dependent release of acetylcholine leads to circadian oscillations in hippocampal extracellular D-serine levels (Papouin et al., 2017). D-serine circadian oscillations were abolished when the gene for α7 nicotinic acetylcholine receptors (nAChRs) was specifically deleted from hippocampal CA1 astrocytes. Extracellular L-serine does not follow circadian oscillations, indicating that circadian D-serine fluctuations originate from astrocytes. Remarkably, α7nAChR-dependent D-serine release causes the glycine-binding site of N-methyl D-aspartate receptors (NMDARs) to reach saturation during the active/wakeful phase of the mouse. This finding raises the possibility that transient vigilance-independent D-serine release may be less impactful during the active phase, when NMDAR glycine-binding sites appear to be saturated, than during sleep, and may offer an explanation why several phenotypes arising from astroglia-selective reductions in Ca2+ dynamics have been found linked to the regulation of sleep (Halassa et al., 2009; Foley et al., 2017; Bojarskaite et al., 2020; Ingiosi et al., 2020).
Glutamatergic signaling to astrocytes has been intensively studied in juvenile rodent hippocampus initially with a significant emphasis on metabotropic glutamate receptor mGlu5 signaling (Rose et al., 2018). In vivo topical pharmacology experiments have suggested that mGlu5 activation on somatosensory cortex astrocytes contributes to contralateral forepaw stimulation- or local electrical stimulation-induced arteriole dilation leading to functional hyperemia (Zonta et al., 2003; Takano et al., 2006). However, while this does not exclude mGlu5 signaling in astrocytic endfeet where microdomain Ca2+ events are spared, it has been demonstrated that in ITPR2–/– mice where the majority of Ca2+ elevations in soma and main processes as well as a considerable portion of fine process microdomain events are abolished, functional hyperemia is not impaired (Nizar et al., 2013; Bonder and McCarthy, 2014). More recent work has even revealed the reverse flow of information: cortical astrocyte endfeet in contact with arterioles sense functional hyperemia-associated vasodilation and endothelial nitric oxide production and respond with enhanced Ca2+ elevations (Tran et al., 2018).
Developmental expression analysis of rodent mGlu5 in mouse cortical astrocytes revealed steep downregulation during the third and fourth postnatal weeks leaving the Gi/o protein-coupled receptor mGlu2/3 as the predominant astrocyte metabotropic glutamate receptor (Sun et al., 2013). The developmental downregulation of cortical astrocyte mGlu5 expression is also subject to pathological dysregulation. In a mouse model of Fragile X syndrome, microRNA miR-128-3p is expressed at an elevated level in astroglia and causes accelerated loss of mGlu5 function (Men et al., 2020). Similar to cortical astrocyte downregulation of mGlu5 expression, adult mouse hippocampal mossy fiber stimulation-evoked astrocyte Ca2+ elevations as well as the frequency of spontaneous microdomain Ca2+ events are not inhibited by the antagonist for mGlu5 but are sensitive to antagonists for mGlu2/3 and for the metabotropic γ-amino butyric acid (GABA) receptor GABABR (Haustein et al., 2014). In contrast to their action in neurons, Gi/o protein-coupled receptors in astroglia often enhance Ca2+ elevations, most likely through direct initiation and/or facilitation of IP3R opening by the βγ subunit (Zeng et al., 2003; Durkee et al., 2019; Nagai et al., 2019), but see (Kol et al., 2020).
Regarding in vivo consequences of glutamatergic signaling to astroglia, AMPA receptor function in cerebellar Bergmann glia is among the better understood examples. Two distinct strategies have been pursued to reduce or eliminate AMPA receptor-mediated Ca2+ influx. As demonstrated in one study, viral overexpression of the GluA2 subunit in Bergmann glia renders AMPA receptors Ca2+ impermeable, impairs the close ensheathment of glutamatergic synapses onto Purkinje cells by Bergmann glia processes and consequently, slows glutamate clearance (Iino et al., 2001). Developmental pruning of the climbing fiber, one of the two major excitatory inputs to the cerebellar cortex, is also impaired. The second strategy was use of conditional knockout of the two predominant AMPA receptor subunits expressed in Bergmann glia, GluA1 and GluA4. Twelve weeks after inducing gene deletion, Bergmann glia processes were morphologically altered, synaptic glutamate clearance impaired, and miniature excitatory postsynaptic currents in Purkinje cells were less frequent (Saab et al., 2012). Moreover, the mice exhibited a higher propensity toward missteps on the Erasmus Ladder, which is a fine motor coordination task, and impaired eyeblink conditioned responses. Together, these findings indicate that Ca2+ influx through AMPA receptors is an important signal for establishment and maintenance of proper synaptic function in the cerebellar cortex.
ATP is among the most reliable agonists to trigger global Ca2+ elevations as well as enhance microdomain Ca2+ events in astroglia in vitro. In vivo, some of the best understood mechanisms of purinergic signaling through astroglia are during development, pathological reactivity and functional hyperemia. Before hearing onset, astroglia-like inner supporting cells (ISCs) spontaneously release ATP which activates P2Y1 autoreceptors and together with intense gap junction coupling among ISCs, intracellular Ca2+ rises in clusters of neighboring cells (Babola et al., 2020). The Ca2+ elevation leads to opening of Ca2+-activated chloride channel TMEM16A which extrudes chloride, drives potassium efflux, and causes transient depolarization of neighboring inner hair cells (Wang et al., 2015). Bursts of action potentials in spiral ganglion neuron (SGN) afferents that innervate neighboring inner hair cells propagate throughout the pre-hearing auditory pathway preserving later tonotopic representation (Babola et al., 2018). ISC-initiated transient potassium elevations can even excite neighboring SGN afferents directly when inner hair cell-SGN afferent synaptic transmission is impaired (Babola et al., 2018). It is thought that this astroglia-mediated spontaneous and transient activation of the auditory pathway allows for circuit maturation so that sensory processing may begin when the ear canal opens.
In adult rodents, P2Y1 receptors participate in astrocyte-to-neuron signaling in brainstem-mediated baroreception and response. It has been found that experimentally increasing intracranial pressure leads to increased venous pressure, reduced difference in brain arterio-venous pressure, and decreases the brain blood flow, which ultimately causes a Ca2+ elevation in brainstem astrocytes that are in proximity to central sympathetic autonomous nervous system centers (Marina et al., 2020). In response, the heart rate and the mean arterial pressure increase for homeostatic regulation of brain blood flow. This astrocytic regulatory response was impaired when vesicular release was hampered by overexpressing dominant-negative soluble N-ethyl-maleimide-sensitive factor attachment protein receptor (dn-SNARE). The Gourine laboratory studied the pressure sensing mechanism in more detail in an in vitro preparation and proposed a model for the initial pressure-dependent ATP release mechanism based on an interaction between TRPV4 ion channels and connexin 43. After finding that P2Y1 autoreceptors appear to serve as signal amplifiers, they deduced that vesicular release of ATP from brainstem astrocytes is the signal that excites central sympathetic centers (Turovsky et al., 2020). Astrocyte P2Y1 receptors have also been found to account for the enhanced microdomain Ca2+ event frequency in cortical astrocytes of anesthetized mouse models of Alzheimer’s disease (Kuchibhotla et al., 2009; Delekate et al., 2014).
Ionotropic receptors are likewise utilized by astrocytes to receive purinergic signals. Functional hyperemia occurs differently at the capillary level, which is controlled by astroglia Ca2+ dynamics, and the arteriole level, which occurs through interneuron nitric oxide release (Mishra et al., 2016). Astroglia-mediated, neuronally evoked dilation of capillaries occurs when Ca2+ influx through P2X1 receptors activates phospholipase D2 and diacylglycerol lipase to generate arachidonic acid. The regulation of functional hyperemia in the rat retina (Biesecker et al., 2016) is consistent with the concept of astroglia mediation of functional hyperemia at the level of capillaries (Mishra et al., 2016) but not arterioles (Nizar et al., 2013; Bonder and McCarthy, 2014). Light flickering induces Ca2+ elevations in Müller cell fine processes in contact with capillaries and in those in contact with arterioles. In ITPR2–/– mice with impaired light flickering-induced Ca2+ elevations in both types of fine processes, vasodilation is impaired only with capillaries (Biesecker et al., 2016).
Circuit and behavior analysis following astroglia-specific gene deletion of the cannabinoid receptor type 1 (CB1R) settled an earlier controversy about the expression of this receptor in astrocytes (Stella, 2004; Han et al., 2012). In addition to mGlu2/3 receptors and GABAB receptors, Gi/o protein-coupled CB1R activation also leads to astrocyte Ca2+ elevations (Navarrete and Araque, 2008, 2010; Robin et al., 2018). It still remains to be determined whether in awake behaving mice CB1R activation leads to global, whole-cell Ca2+ elevation as seen in slice experiments following neuronal depolarization or CB1R agonist application, or to increased frequency of microdomain Ca2+ events.
Depending on intensity and duration of hippocampal astrocyte CB1R activation, different effects on local circuit activity and behavior have been observed. Han et al. (2012) tested the hypothesis that astrocyte CB1Rs could account for the known detrimental effect of acute cannabinoid exposure for spatial working memory (Lichtman and Martin, 1996). They found that acute cannabinoid exposure is required during induction, but not for expression of in vivo hippocampal CA3-CA1 long-term depression (LTD) (Han et al., 2012). Cannabinoids cause LTD through astrocyte CB1R but not through glutamatergic or GABAergic neuron CB1R. LTD occurs through internalization of postsynaptic AMPA receptors that is independent of mGlu1 activation but dependent on NMDA receptor activation. The impairment in spatial working memory follows the same pharmacological profile as the impairment of astrocyte CB1R-mediated LTD (Han et al., 2012). A recent study on NMDA receptor-dependent, postsynaptic expression of CA3-CA1 hippocampal LTD further demonstrated that this circuit phenomenon depends on the astrocytic activation of the p38α variant of mitogen-activated protein kinase (MAPK) (Navarrete et al., 2019).
In contrast, endogenous activation of astrocyte CB1Rs leads to synaptic potentiation. The retrograde diffusion of endogenous cannabinoids following postsynaptic depolarization-induced production causes inhibition of subsequent neurotransmitter release from presynaptic terminals that express CB1Rs, a phenomenon known as depolarization-induced suppression of inhibition (DSI) or excitation (DSE) (Maejima et al., 2001; Wilson and Nicoll, 2001; Alger, 2002; Kreitzer et al., 2002; Ohno-Shosaku et al., 2002). An investigation for a role of hippocampal astrocytes in endocannabinoid-mediated short-term plasticity found that while a 5 s depolarization of CA1 pyramidal neurons to 0 V induces homoneuronal DSE of Schaffer collateral input, neighboring pyramidal neurons experience heteroneuronal synaptic potentiation (Navarrete and Araque, 2010). Several lines of evidence pointed at astrocyte CB1Rs being responsible for the tens of seconds lasting synaptic potentiation. This was abolished following depletion of intracellular Ca2+ stores with thapsigargin, while DSE was not. Also, it was inhibited by loading astrocytes with the high-affinity Ca2+ chelator BAPTA or the G-protein inhibitor GDPβS, a procedure leading toward enhanced DSE. While pyramidal neuron depolarization-induced astrocyte Ca2+ elevations were insensitive to inhibition of mGlu1, synaptic potentiation was inhibited, suggesting presynaptic mGlu1 activation downstream of astrocyte Ca2+ elevation is responsible for synaptic potentiation (Navarrete and Araque, 2010).
Recently, it has been demonstrated that novel object recognition is strongly impaired in astroglia-specific CB1R knockout mice (Robin et al., 2018). Hippocampal NMDA receptor function was found to be required for novel object recognition, and both NMDA receptors as well as astrocyte CB1Rs were required for in vivo high-frequency stimulation-induced long-term potentiation (LTP). Consistent with the dependence on NMDA receptors, high-frequency stimulation-induced LTP as well as novel object recognition could be rescued in astroglia-specific CB1R knockout mice by substituting with saturating concentration of D-serine (Robin et al., 2018). This finding suggests that astrocyte CB1R signaling is important for hippocampal LTP and novel object recognition via controlling the availability of extracellular D-serine. This conclusion, however, may have some interesting implications. As discussed earlier, it has recently been found that circadian/wakefulness-dependent cholinergic signaling through hippocampal astrocytes controls mean extracellular availability of D-serine (Papouin et al., 2017). Six hours into the active phase in environmental enrichment housing, extracellular D-serine levels saturate the glycine binding site of NMDA receptors, whereas the occupancy of this site reaches a minimum toward the end of the sleep phase (Papouin et al., 2017). The novel object recognition study here was conducted 7–10 h into the sleep phase, and indeed NMDA receptors were not saturated with endogenous D-serine (Robin et al., 2018). Therefore, it will also be important to explore the impact of hippocampal astrocyte CB1R signaling on novel object recognition during the active phase when animals are more likely to encounter object recognition challenges.
In the hippocampal CA3-CA1 microcircuit, astrocyte GABAB receptor signaling plays a role in counterbalancing GABAergic interneuron-mediated inhibition of Shaffer collateral glutamate release (Perea et al., 2016). At states of low interneuron excitation (individual action potential firing) Shaffer collateral glutamate release is inhibited in a GABAA receptor-dependent manner. In contrast, when interneurons fire bursts of action potentials, GABAB receptors on astrocytes are recruited, as astrocyte-specific knockout experiments confirmed, leading to enhanced Ca2+ signaling and group 1 mGlu receptor-dependent enhancement of Shaffer collateral glutamate release. Astrocyte-specific GABAB receptor knockout was sufficient to reduce the power of hippocampal theta and low gamma oscillations (30–60 Hz) in anesthetized mice (Perea et al., 2016). The contribution of astrocyte-dependent Ca2+ signaling to low gamma oscillations is consistent with findings from transgenic mice with astrocyte-selective overexpression of tetanus toxin (Lee et al., 2014). Here the reduction of low gamma oscillations was, however, only significant in awake mice, not during sleep.
A recent study explored the circuit and behavioral consequences of astrocyte-specific knockout of GABAB receptors in prefrontal cortex (Mederos et al., 2021). They also found a reduction of low gamma oscillations in active awake mice, but not in resting mice. Low gamma oscillations may reflect synchronization of the neuronal population and has been associated with working memory processing in prefrontal cortex (Buzśaki and Wang, 2012). Indeed, mice lacking GABAB receptors in prefrontal cortex astrocytes have difficulty making correct decisions during the T-maze alternating behavioral task, a paradigm testing working memory performance (Mederos et al., 2021). A more detailed cellular analysis of the circuit function revealed that astrocyte GABAB receptors, IP3R2-dependently, potentiate parvalbumin-positive interneuron-driven inhibitory postsynaptic currents in local neurons. Using astrocyte-specific overexpression of melanopsin1 for optical control of Gq protein-coupled receptor activation exclusively in astrocytes (Mederos et al., 2019), they not only rescued goal-directed behavior in astrocyte-GABAB receptor knockout mice but further enhanced goal-directed behavior in wildtype mice (Mederos et al., 2021). This observation is in contrast with disruptive consequences of astrocyte-specific activation of exogenous Gi protein-coupled receptors in striatal astrocytes, which also enhances Ca2+ dynamics but leads to hyperactivity of the mice (Nagai et al., 2019). It indicates that brain region, subcellular location of receptors within astroglia, and duration of activation likely all have inherent importance.
The mitochondrial PTP appears to be a major determinant of spontaneous, intrinsically regulated astroglia microdomain Ca2+ fluctuations (Agarwal et al., 2017). The intrinsic nature of these signaling events likely stems from metabolic control (Jackson and Robinson, 2015; Agarwal et al., 2017; Oheim et al., 2018). In addition, the promiscuous regulation of mPTP activity by ROS, Ca2+, and IP3R among others suggests that stochastic activation of the many G protein-coupled astrocyte receptors in the presence of ambient receptor ligands at low basal concentrations or in their absence may account for the considerable bafilomycin A1-insensitive, IP3R2-dependent portion of microdomain Ca2+ events (Agarwal et al., 2017). To further this complexity, vigilance/arousal-dependent, predominantly noradrenergic signaling, which is critical for whole-cell, widespread Ca2+ elevations (Ding et al., 2013; Paukert et al., 2014; Ye et al., 2020), also contributes to microdomain events (Agarwal et al., 2017), as does local neuronal activity through activation of various receptors or ion channels (Agarwal et al., 2017; Bindocci et al., 2017; Stobart et al., 2018). The complexity of astroglia microdomain Ca2+ dynamics so far has made it impossible to genetically manipulate selectively microdomain activity while leaving basal Ca2+ as well as whole-cell Ca2+ elevations intact. More refined mechanistic insight into what happens in astroglia mitochondria during distinct behavioral and environmental states will be required to design gentle but highly specific genetic manipulations.
In the midst of this challenge, the Drosophila model system offers an opportunity to selectively target astrocyte microdomain Ca2+ events (Ma and Freeman, 2020). In contrast to mammalian microdomain events, apart from the ineffective neurotransmitters including glutamate, acetylcholine, GABA and octopamine, only tyramine, which corresponds to mammalian dopamine, enhances the frequency of Drosophila TrpML-mediated Ca2+ transients by approximately 40%. Around half of the microdomain events are associated with the tracheal system, which in Drosophila, consists of a network that terminates in filopodia throughout the nervous system and facilitates oxygen delivery. Retractions of tracheal filopodia are often preceded by astrocyte microdomain events. Inhibiting or genetically deleting TrpML in astrocytes eliminates microdomain Ca2+ events and increases ROS concentration and results in growth of the tracheal system, suggestive of reduced filopodia retractions (Ma and Freeman, 2020). Together, these findings suggest that astroglia microdomain Ca2+ dynamics are not only an evolutionary conserved phenomenon, but may serve a conserved functional role as homeostatic element within the metabolic state of the microenvironment. As we will discuss below, a way of studying preferentially microdomain Ca2+ events in mammalian astroglia could be represented by sleep studies, where vigilance/arousal-dependent whole cell Ca2+ events, like under general anesthesia, are strongly reduced, if not absent (Bojarskaite et al., 2020; Ingiosi et al., 2020).
Drosophila astroglia have granted insight into diverse mechanisms of how basal Ca2+ controls local circuit excitability and behavior. In cortex glia, which are located in brain areas densely populated with cell bodies but no synapses, the genetic deletion of the Na+/Ca2+, K+ exchanger zydeco leads to elevation of intracellular Ca2+ accompanied by a proneness to seizure activity (Melom and Littleton, 2013). The constant elevation of Ca2+ causes the intrinsic microdomain Ca2+ activity to disappear, possibly through masking it. Intracellular Ca2+ elevation in cortex glia is mechanistically linked to seizure activity by activation of calcineurin, subsequent endocytosis of two-pore K+ channel sandman, and impaired extracellular buffering of K+ (Weiss et al., 2019). The elevated extracellular K+ will depolarize surrounding excitable cells and bring their membrane potential closer to the threshold of excitation.
In Drosophila astrocytes, which are located in areas containing synapses, intracellular Ca2+ elevation triggers a different signaling pathway resulting in paralysis (Zhang et al., 2017). It was found that Ca2+ elevation increases the expression of Rab11, a GABA plasma membrane transporter (GAT) regulator, facilitating endocytosis of GAT, impairing synaptic GABA clearance, and leading to paralysis (Zhang et al., 2017). An analogous mechanism was uncovered in mouse striatal astrocytes where experimental reduction of intracellular Ca2+ leads to enhanced GABA clearance and reduced tonic inhibition of the surrounding microcircuitry, ultimately causing excessive self-grooming (Yu et al., 2018). Mouse astroglia can also control tonic inhibition through GABA release via Ca2+-activated anion channel bestrophin-1. This mechanism has been proposed for cerebellar Bergmann glia controlling excitability of granule cells (Lee et al., 2010) and for astrocytes in the striatum controlling excitability of medium spiny neurons (Yoon et al., 2014). Astroglia-mediated tonic inhibition has been associated with limited performance on the rotarod paradigm (Woo et al., 2018). Also, bestrophin-1 has been implicated in vesicle-independent astrocytic release of glutamate and D-serine (Oh and Lee, 2017).
IP3R2 is an astroglia Ca2+ signaling junction point. It is by far the most abundant IP3R subtype in astroglia (Sharp et al., 1999; Holtzclaw et al., 2002; Petravicz et al., 2008). Upon gene deletion, whole-cell Ca2+ elevations are almost completely abolished and also the frequency of microdomain events is considerably reduced (Fiacco et al., 2007; Srinivasan et al., 2015; Agarwal et al., 2017), suggesting that through its interaction with mPTP, it is a major pathway how G protein-coupled receptors facilitate microdomain events. The importance of IP3R2 for astroglia Ca2+ dynamics is further emphasized by the finding that it serves not only Gq protein-coupled receptors but also Gi/o protein-coupled receptors for intracellular Ca2+ release (Durkee et al., 2019). Given the apparent importance of IP3R2 for astroglia Ca2+ dynamics, it came as a surprise that a series of acute hippocampal slice studies of baseline and stimulated synaptic function and synaptic plasticity found no consequences of IP3R2 gene deletion (Fiacco et al., 2007; Petravicz et al., 2008; Agulhon et al., 2010). These findings triggered more refined slice physiology studies that applied astrocyte-specific knockout of endogenous receptors and the dissection of concomitant excitatory as well as inhibitory or potentiating as well as depressing circuit mechanisms (e.g., Perea et al., 2016). In addition, the study of spontaneous as well as agonist-induced hippocampal astrocyte Ca2+ dynamics from acute slices of mice carrying a combined gene deletion of IP3R2 and IP3R3 suggests that non-type 2 IP3Rs may contribute to astroglia Ca2+ elevations (Sherwood et al., 2017). Not only at the level of slice physiology, also at the behavioral level the consequences of IP3R2 gene deletion have been less impressive than one might have expected. A battery of behavioral tests with astrocyte-specific IP3R2 gene deletion mice revealed no significant difference to wildtype littermates when testing anxiety, depressive behavior, motor coordination, exploratory behavior, sensory motor gating with the acoustic startle response and prepulse inhibition, and with learning and memory using the Morris water maze (Petravicz et al., 2014).
On the other hand, recent years have also seen a considerable number of findings demonstrating how loss of IP3R2 function does affect developmental synaptic pruning, neurovascular coupling, cortical plasticity and memory, and the regulation of sleep. Similar to the climbing fiber synapse in the cerebellum mentioned earlier (Iino et al., 2001), the principal trigeminal nucleus-ventral posteromedial thalamic nucleus synapse serves as a model of developmental synaptic pruning since between postnatal day 7 (P7) and P16, 7–8 inputs are refined into a single mature input (Yang et al., 2016). Using a combination of acute slice electrophysiology and immunocytochemistry it has been found that this synapse elimination is incomplete in ITPR2–/– mice, leaving on average more than two functional inputs at P16. Local administration of ATP or the P2Y1 receptor agonist MRS-2365 rescued synapse elimination, but global P2Y1 receptor gene deletion made all rescue attempts fail (Yang et al., 2016). This suggests a model where IP3R2-dependent astrocytic ATP release acts through P2Y1 receptors to facilitate synapse elimination.
In awake behaving mice, learning and memory can be controlled by astroglia Ca2+ dynamics at different time scales. Padmashri et al. (2015) used an inducible astroglia-specific IP3R2 gene deletion approach to test whether motor skill learning is affected. Even though the conditional knockout approach reduced astrocyte Ca2+ responses to the application of ATP only by approximately 50%, knockout mice reached an earlier ceiling of the success rate in a forelimb reaching-task after 3 of 5 days. They also found a linear relationship between residual Ca2+ responsiveness and preservation in motor skill learning (Padmashri et al., 2015). Pinto-Duarte et al. (2019) did not find an effect of global IP3R2 gene deletion on novel object recognition, fear memory, or spatial memory within 2–5 days. But in all three tests, they found a considerable impairment 2–4 weeks later. Since such a remote memory deficit might represent impaired memory consolidation, they investigated hippocampal Schaffer collateral-to-CA1 field potential LTD in acute slices. They found impaired LTD in ITPR2–/– mice, which could be rescued with exogenous D-serine, but not when preincubated with the degrading enzyme D-amino acid oxidase (DAAO) or with NMDA receptor inhibition (Pinto-Duarte et al., 2019). Astroglia Ca2+ dynamics can also acutely shape cortical neuronal population activity in response to sensory stimulation (Lines et al., 2020). Monitoring somatosensory cortex gamma activity revealed that sensory stimulation induces a transient increase in power that attenuates to a steady-state level for the duration of the stimulation. In ITPR2–/– mice, the attenuation is diminished. This is unlikely due to developmental changes, since acute elevation of astroglia Ca2+ via astroglia-specific activation of hM3Dq reduces the gamma activity (Lines et al., 2020).
Several studies have focused on the role of astroglia Ca2+ signaling during sleep. A very elegant recent study combined monitoring of somatosensory cortex astrocyte Ca2+ dynamics, locomotion activity of head-fixed mice, whisking activity, as well as cortical electrical activity and muscle activity in ITPR2–/– mice and wildtype littermates (Bojarskaite et al., 2020). In the analysis of sleep states, they distinguished between slow wave sleep with a further distinction between non-rapid eye movement (NREM) sleep and intermediate state (IS) sleep, and rapid eye movement (REM) sleep. As expected for a behavioral state lacking significant arousal, astrocyte Ca2+ dynamics during sleep are dominated by microdomain events. Preceding the state transition from either of the two slow wave sleep states (NREM or IS) to wakefulness, there is an enhanced IP3R2-dependent Ca2+ elevation. This finding is consistent with an independent recent astroglia Ca2+ imaging study where intracellular Ca2+ release was attenuated by astroglia-specific gene deletion of stromal interaction molecule 1 (STIM1) (Ingiosi et al., 2020). The transition from slow wave sleep to wakefulness is when enhanced norepinephrine release is expected (Takahashi et al., 2010). Surprisingly, neither study found a Ca2+ elevation during REM sleep (Bojarskaite et al., 2020; Ingiosi et al., 2020) when elevated levels of extracellular acetylcholine are expected (Jasper and Tessier, 1971). This finding, together with the inhibition of global widespread cortical astroglia Ca2+ elevations during awake states of heightened vigilance by α1-adrenergic receptor antagonists (Ding et al., 2013; Srinivasan et al., 2015) raise the possibility that acetylcholine cannot directly cause significant cortical astroglia Ca2+ elevations. Another finding in ITPR2–/– mice was increased frequency of sleep spindles during IS sleep, which may cause impaired memory consolidation (Bojarskaite et al., 2020). This finding may provide a mechanistic explanation for impaired remote memory in ITPR2–/– mice discussed above (Pinto-Duarte et al., 2019) or for impaired remote memory in consequence of manipulation of astroglia Ca2+ dynamics using the humanized Gi protein-coupled muscarinic receptor type 4 DREADD (hM4Di) discussed below (Kol et al., 2020). Bojarskaite et al. (2020) also found considerably reduced delta electrocorticogram power during NREM sleep in ITPR2–/– mice, which indicates reduced sleep pressure, which is consistent with the first study that reported astrocyte control of sleep pressure and the impact of sleep deprivation (Halassa et al., 2009). This study by Halassa et al. (2009) used astrocyte-specific overexpression of dn-SNARE to impair the proposed vesicular release of ATP and to reduce occupancy of adenosine A1 receptors, which are important for mediating sleep pressure. Consistent findings on sleep pressure and sleep deprivation were obtained by Ingiosi et al. (2020). Finally, Bojarskaite et al. (2020) found that loss of IP3R2 function reduces the dwell time but increases the frequency of both slow wave sleep states, leaving REM sleep unaltered. This finding is almost opposite to the outcome of a study where IP3 signaling was directly attenuated by astroglia-specific overexpression of the venus-tagged IP3 5’phosphatase (VIPP) (Foley et al., 2017). There they found an increased number of REM sleep bouts with no difference in dwell time and no change in NREM sleep. The discrepancy in results may be explained by the fact that IP3R2 gene deletion will considerably attenuate intracellular Ca2+ release mediated by possibly all astrocyte G protein-coupled receptors. In contrast, hydrolysis of IP3 is not expected to interfere with Gi/o βγ subunit binding to IP3R2. Therefore, it is tempting to speculate that activation of α2-adrenergic receptors, or of any other astrocyte Gi/o protein-coupled receptor in the study by Foley et al. (2017) could account for the difference in sleep regulation among these two studies.
In recent years, astroglia-specific overexpression of exogenous actuators for manipulation and interrogation of astroglia physiology has become very popular (Table 2). The advantages of such approaches are immediately clear since almost all receptors on astroglia are also present on neurons and other neural cells. Limitations of the utility of exogenous actuators need to be considered in light of the downstream signaling events that follow the astroglia-specific initiation. Actuators which remove an endogenous signal may arguably be regarded as less invasive. One example is the CalEx constitutively active plasmalemmal Ca2+ pump and will be discussed below. For actuators that represent the overexpression of an exogenous G protein-coupled receptor that elevates Ca2+, one needs to consider the physiological dynamic range of the expected signal regarding expression level, its subcellular location, and duration/pattern of ligand application. Predictions of the consequences of overexpression of ion channels, some with ion selectivity for which no physiological counterpart is known in astroglia, a cell type with ion buffering function, are most complex. Nevertheless, these are very powerful tools that may inform follow-up studies focused on the physiological role of specific endogenous astroglia signaling pathways.
To inhibit microdomain events despite their mechanistic diversity, Yu et al. (2018) modified human plasma membrane Ca2+ ATPase pump (hPMCA) isoform 2, w/b splice variant with excised cytosolic interaction domains (hPMCA2w/b), resulting in a modified pump that constitutively extrudes cytosolic Ca2+. The model was coined “CalEx” due to its function in Ca2+ extrusion2 (Yu et al., 2018). Behavioral assays of mice expressing CalEx in striatal astrocytes revealed that reducing whole-cell and microdomain Ca2+ dynamics results in excessive self-grooming behavior indicative of an obsessive-compulsive disorder phenotype. The underlying circuit mechanism was identified as low astrocyte Ca2+ leading to reduced expression of Rab11a, which leads to less internalization of the GABA transporter GAT-3, more GABA uptake, lower ambient GABA concentration and less tonic inhibition (Yu et al., 2018). This mechanism is conserved in Drosophila astrocytes (Zhang et al., 2017), but the relationship between basal mouse astrocyte Ca2+ set by TRPA1 and surface expression of GAT-3 in co-culture with neurons is reversed (Shigetomi et al., 2011). CalEx overexpressed in mouse prefrontal cortex astrocytes was used in comparison with hM3Dq3, the Gq protein-coupled DREADD, to study the effects of manipulating astrocyte Ca2+ on ethanol’s motivational, stimulatory and sedating impact (Erickson et al., 2020). It was found that while boosting astrocyte Ca2+ dynamics enhances all negative effects such as ethanol consumption, excessive locomotor stimulation in response to low dose ethanol and prolonged loss of righting reflex (LORR) due to high dose ethanol sedation, reducing astrocyte Ca2+ dynamics with CalEx has protective, opposite effects. The aggravated sedating effect of enhanced astrocyte Ca2+ could be reversed by inhibiting adenosine A1 receptors (Erickson et al., 2020), suggesting that hM3Dq activation in prefrontal cortex astrocytes may trigger ATP release. In the light of these findings it is interesting to note that the suppression of vigilance-dependent astroglia Ca2+ activation by ethanol inhibition of norepinephrine release (Ye et al., 2020) may play a homeostatic protective role during acute ethanol intoxication.
The Goshen group recently expressed hM4Di4 in hippocampal CA1 astrocytes to inhibit their Ca2+ elevations (Kol et al., 2020). This is remarkable, because Gi/o protein-coupled receptors usually enhance Ca2+ dynamics in astroglia. Others indeed found that hM4Di when expressed in hippocampal CA1 astrocytes enhances Ca2+ signals (Durkee et al., 2019), and this is consistent with striatal astrocytes (Nagai et al., 2019). One could imagine two possible explanations for this discrepancy. It is possible that the expression level, which could then also affect the subcellular location, was different. Or, since they used the mode in order to present the quantification of Ca2+ events, which represents the global maximum in the amplitude frequency distribution but completely ignores local maxima, it may be interesting to see the full distribution in response sizes. Nevertheless, in this elegant study they found that the CA1-to-anterior cingulate cortex (ACC) projection is critical for remote memory but not for recent memory. Inhibiting CA1 astrocyte Ca2+ dynamics then disrupts remote memory (Kol et al., 2020). These results are consistent with the recent finding that IP3R2 gene deletion impairs remote but not recent memory (Pinto-Duarte et al., 2019).
The mas-related genes Gq protein-coupled orphan receptor MrgA1, which is naturally only expressed in some nociceptive neurons (Han et al., 2002), was the first xenoreceptor used for astroglia-specific Ca2+ activation (Fiacco et al., 2007). When expressed in cerebellar Bergmann glia of anesthetized mice, it was found that application of its peptide ligand, the Phe-Met-Arg-Phe (FMRF)amide, a whole-cell Ca2+ elevation is accompanied by extracellular reduction in potassium concentration leading to a temporary hyperexcitability of Purkinje cells, the cerebellar principal neurons (Wang et al., 2012); however, see Smith et al. (2018). A different study used the hyperpolarizing optogenetic actuator Archaerhodopsin (Arch)5 in cortical astrocytes of awake mice and found that Arch light-activation causes Ca2+ elevations that, in terms of their spatial restriction, resemble microdomain events, but last tens of seconds (Poskanzer and Yuste, 2016). The mechanism of how Arch leads to Ca2+ elevation remains elusive. As a consequence of astrocyte Arch activation, extracellular glutamate spikes can be detected accompanying slow oscillatory and more synchronized neuronal activity (Poskanzer and Yuste, 2016).
Activation of hM3Dq and the α1-adrenergic receptor-based opsin Opto-Gq (Airan et al., 2009) in hippocampal CA1 astrocytes to elevate Ca2+ has been demonstrated to be sufficient to induce NMDA receptor-dependent LTP and contextual memory (Adamsky et al., 2018). In contrast, hM3Dq activation in neurons has a detrimental effect on memory performance (Adamsky et al., 2018). Activation of hM4Di in striatal astrocytes leads to enhanced Ca2+ elevations and hyperactivity (Nagai et al., 2019). Transcriptomic analysis of activated astrocytes revealed the reactivation of thrombospondin 1, which is an important factor during developmental synaptogenesis (Christopherson et al., 2005). Treatment with gabapentin, antagonist of the thrombospondin 1 receptor, rescues all morphological as well as all functional changes (Nagai et al., 2019). They further demonstrated that hM4Di activation in astrocytes can be used to compensate phenotypically for mouse disease models which display reduced astrocytic Ca2+ activity, such as the Huntington’s disease mouse model (Yu et al., 2020). Durkee et al. (2019) conducted a systematic comparison of the effects of hM3Dq and hM4Di when expressed in neurons or astrocytes. They found that hM4Di, like GABABR activation, elevates Ca2+ levels in astrocytes in an IP3R2-dependent manner. Yet, the phospholipase C inhibitor U73122 failed to inhibit hM4Di as well as GABABR responses in astrocytes. This suggests the possibility that in general, Gi/o protein-coupled receptors in astroglia couple via the βγ subunit to the IP3R2 (Zeng et al., 2003; Durkee et al., 2019).
Considerable progress has been made in our understanding of the role of astroglia Ca2+ dynamics for the processing of information in the brain. Behavioral state transitions occur constantly, ranging from different sleep states to various levels of vigilance during wakefulness and are disrupted in psychiatric disease. Emerging experimental findings, which are schematized in Figure 1, emphasize the importance of the particular behavioral state that determines which molecular mechanisms predominate the respective astroglia Ca2+ signal, what spatial and temporal pattern it assumes, and how it feeds back to behavior. While the precise astroglia Ca2+ signals in unanesthetized animals have not yet been defined in vivo for many of the discussed receptors, it appears that a significant portion of astroglia Ca2+ dynamics are representations of neuromodulator signaling and are primed for a role in informing neural populations about the behavioral and environmental context. Since dependence of astroglia Ca2+ activation on behavioral state relies on variable release of neuromodulator and not on variable intrinsic responsiveness of astroglia, it is possible that exogenous astroglia-specific actuators have led to more robust phenotypes because the limited spatial and temporal precision of this approach allows the recruitment of astroglia Ca2+ activation out of behavioral or environmental context. On the other hand, it is encouraging that several similarities in phenotypes have been independently defined. For example, the importance of astroglia Ca2+ dynamics in memory consolidation allowing remote memory formation has been discovered using the IP3R2 gene deletion model as well as independently using inhibitory DREADD overexpression in hippocampal astrocytes. Similarly, a role for astroglia Ca2+ dynamics in developmental synapse elimination was discovered using the same IP3R2 gene deletion model, and independently it was found that developmental synaptogenic cues can be reactivated by exogenous enhancement of astroglia Ca2+ dynamics in adult mice. It will be important to combine insight from exogenous activation studies with our increasing understanding of the behavioral and environmental context when endogenous astroglia signaling pathways are naturally engaged to revise and customize conventional behavioral paradigms for testing the physiological impact of astroglia Ca2+ dynamics. This will be a prerequisite for recognizing alterations in astroglia Ca2+ dynamics and for understanding how they can contribute to nervous system disease.
Figure 1. Diagram of behavioral state-dependent activation of noradrenergic and cholinergic systems and their modulation of astroglia Ca2+ dynamics during sleep, rest, and vigilance. Astrocytes in primary visual cortex and somatosensory cortex, Hes5-positive astrocytes in spinal dorsal horn, and cerebellar Bergmann glia exhibit norepinephrine-dependent global Ca2+ rises during states of heightened vigilance. In Bergmann glia and Hes5-positive astrocytes of spinal dorsal horn, this arousal-associated Ca2+ signal is mediated by α1A-adrenergic receptors. Cholinergic activation of hippocampal astrocytes through α7 nicotinic acetylcholine receptors has been found to modulate D-serine concentration depending on the sleep-wake state. LC, locus coeruleus; LDT, laterodorsal tegmental nucleus; PPN, pedunculopontine nucleus; MS, medial septal nucleus; DB, diagonal band of Broca; NBM, nucleus basalis of Meynert; SI, substantia innominata.
All authors listed have made a substantial, direct and intellectual contribution to the work, and approved it for publication.
EL was supported by a T32NS082145 fellowship. This work was supported by R01AA025128, R01MH113780, and The Robert J. Kleberg, Jr. and Helen C. Kleberg Foundation to MP.
The authors declare that the research was conducted in the absence of any commercial or financial relationships that could be construed as a potential conflict of interest.
Astroglia Ca2+ dynamics and their consequences for neural circuit function and behavior has become a rapidly proliferating research area. Many important contributions could not be covered in this review. However, we hope that the organization of this review will help to place other studies in context.
Adamsky, A., Kol, A., Kreisel, T., Doron, A., Ozeri-Engelhard, N., Melcer, T., et al. (2018). Astrocytic activation generates De Novo neuronal potentiation and memory enhancement. Cell 174, 59.e14–71.e14. doi: 10.1016/j.cell.2018.05.002
Agarwal, A., Wu, P.-H., Hughes, E. G., Fukaya, M., Tischfield, M. A., Langseth, A. J., et al. (2017). Transient opening of the mitochondrial permeability transition pore induces microdomain calcium transients in astrocyte processes. Neuron 93, 587.e7–605.e7. doi: 10.1016/j.neuron.2016.12.034
Agulhon, C., Fiacco, T. A., and McCarthy, K. D. (2010). Hippocampal short- and long-term plasticity are not modulated by astrocyte Ca2+ signaling. Science 327, 1250–1254. doi: 10.1126/science.1184821
Airan, R. D., Thompson, K. R., Fenno, L. E., Bernstein, H., and Deisseroth, K. (2009). Temporally precise in vivo control of intracellular signalling. Nature 458, 1025–1029. doi: 10.1038/nature07926
Alger, B. E. (2002). Retrograde signaling in the regulation of synaptic transmission: focus on endocannabinoids. Prog. Neurobiol. 68, 247–286. doi: 10.1016/S0301-0082(02)00080-1
Araque, A., Carmignoto, G., Haydon, P. G., Oliet, S. H. R., Robitaille, R., and Volterra, A. (2014). Gliotransmitters travel in time and space. Neuron 81, 728–739. doi: 10.1016/j.neuron.2014.02.007
Araque, A., Parpura, V., Sanzgiri, R. P., and Haydon, P. G. (1999). Tripartite synapses: glia, the unacknowledged partner. Trends Neurosci. 22, 208–215. doi: 10.1016/S0166-2236(98)01349-6
Arizono, M., Inavalli, V. V. G. K., Panatier, A., Pfeiffer, T., Angibaud, J., Levet, F., et al. (2020). Structural basis of astrocytic Ca2+ signals at tripartite synapses. Nat. Commun. 11:1906. doi: 10.1038/s41467-020-15648-4
Babola, T. A., Kersbergen, C. J., Wang, H. C., and Bergles, D. E. (2020). Purinergic signaling in cochlear supporting cells reduces hair cell excitability by increasing the extracellular space. eLife 9:e52160. doi: 10.7554/eLife.52160
Babola, T. A., Li, S., Gribizis, A., Lee, B. J., Issa, J. B., Wang, H. C., et al. (2018). Homeostatic control of spontaneous activity in the developing auditory system. Neuron 99, 511.e5–524.e5. doi: 10.1016/j.neuron.2018.07.004
Bánsághi, S., Golenár, T., Madesh, M., Csordás, G., RamachandraRao, S., Sharma, K., et al. (2014). Isoform- and species-specific control of inositol 1,4,5-trisphosphate (IP3) receptors by reactive oxygen species. J. Biol. Chem. 289, 8170–8181. doi: 10.1074/jbc.M113.504159
Beierlein, M., and Regehr, W. G. (2006). Brief bursts of parallel fiber activity trigger calcium signals in bergmann glia. J. Neurosci. 26, 6958–6967.
Bekar, L. K., He, W., and Nedergaard, M. (2008). Locus coeruleus alpha-adrenergic-mediated activation of cortical astrocytes in vivo. Cereb. Cortex 18, 2789–2795. doi: 10.1093/cercor/bhn040
Biesecker, K. R., Srienc, A. I., Shimoda, A. M., Agarwal, A., Bergles, D. E., Kofuji, P., et al. (2016). Glial cell calcium signaling mediates capillary regulation of blood flow in the retina. J. Neurosci. 36, 9435–9445. doi: 10.1523/JNEUROSCI.1782-16.2016
Bindocci, E., Savtchouk, I., Liaudet, N., Becker, D., Carriero, G., and Volterra, A. (2017). Three-dimensional Ca 2+ imaging advances understanding of astrocyte biology. Science 356:eaai8185. doi: 10.1126/science.aai8185
Bojarskaite, L., Bjørnstad, D. M., Pettersen, K. H., Cunen, C., Hermansen, G. H., Åbjørsbråten, K. S., et al. (2020). Astrocytic Ca2+ signaling is reduced during sleep and is involved in the regulation of slow wave sleep. Nat. Commun. 11:3240. doi: 10.1038/s41467-020-17062-2
Bonder, D. E., and McCarthy, K. D. (2014). Astrocytic Gq-GPCR-linked IP3R-dependent Ca2+ signaling does not mediate neurovascular coupling in mouse visual cortex in vivo. J. Neurosci. 34, 13139–13150. doi: 10.1523/JNEUROSCI.2591-14.2014
Burnashev, N., Khodorova, A., Jonas, P., Helm, P. J., Wisden, W., Monyer, H., et al. (1992). Calcium-permeable AMPA-kainate receptors in fusiform cerebellar glial cells. Science 256, 1566–1570. doi: 10.1126/science.1317970
Buzśaki, G., and Wang, X. J. (2012). Mechanisms of gamma oscillations. Annu. Rev. Neurosci. 35, 203–225. doi: 10.1146/annurev-neuro-062111-150444
Chen, N., Sugihara, H., Sharma, J., Perea, G., Petravicz, J., Le, C., et al. (2012). Nucleus basalis-enabled stimulus-specific plasticity in the visual cortex is mediated by astrocytes. Proc. Natl. Acad. Sci. U.S.A. 109, E2832–E2841. doi: 10.1073/pnas.1206557109
Christopherson, K. S., Ullian, E. M., Stokes, C. C. A., Mullowney, C. E., Hell, J. W., Agah, A., et al. (2005). Thrombospondins are astrocyte-secreted proteins that promote CNS synaptogenesis. Cell 120, 421–433. doi: 10.1016/j.cell.2004.12.020
Delekate, A., Füchtemeier, M., Schumacher, T., Ulbrich, C., Foddis, M., and Petzold, G. C. (2014). Metabotropic P2Y1 receptor signalling mediates astrocytic hyperactivity in vivo in an Alzheimer’s disease mouse model. Nat. Commun. 5:5422. doi: 10.1038/ncomms6422
Ding, F., O’Donnell, J., Thrane, A. S., Zeppenfeld, D., Kang, H., Xie, L., et al. (2013). α1-Adrenergic receptors mediate coordinated Ca2+ signaling of cortical astrocytes in awake, behaving mice. Cell Calcium 54, 387–394. doi: 10.1016/j.ceca.2013.09.001
Dombeck, D. A., Khabbaz, A. N., Collman, F., Adelman, T. L., and Tank, D. W. (2007). Imaging large-scale neural activity with cellular resolution in awake, mobile mice. Neuron 56, 43–57. doi: 10.1016/j.neuron.2007.08.003
Duchen, M. R. (2000). Mitochondria and Ca2+ in cell physiology and pathophysiology. Cell Calcium 28, 339–348. doi: 10.1054/ceca.2000.0170
Durkee, C. A., Covelo, A., Lines, J., Kofuji, P., Aguilar, J., and Araque, A. (2019). Gi/o protein-coupled receptors inhibit neurons but activate astrocytes and stimulate gliotransmission. Glia 67, 1076–1093. doi: 10.1002/glia.23589
Erickson, E. K., DaCosta, A. J., Mason, S. C., Blednov, Y. A., Mayfield, R. D., and Harris, R. A. (2020). Cortical astrocytes regulate ethanol consumption and intoxication in mice. Neuropsychopharmacology 46, 500–508. doi: 10.1038/s41386-020-0721-0
Fiacco, T. A., Agulhon, C., Taves, S. R., Petravicz, J., Casper, K. B., Dong, X., et al. (2007). Selective stimulation of astrocyte calcium in situ does not affect neuronal excitatory synaptic activity. Neuron 54, 611–626. doi: 10.1016/j.neuron.2007.04.032
Fiacco, T. A., and McCarthy, K. D. (2018). Multiple lines of evidence indicate that gliotransmission does not occur under physiological conditions. J. Neurosci. 38, 3–13. doi: 10.1523/JNEUROSCI.0016-17.2017
Foley, J., Blutstein, T., Lee, S., Erneux, C., Halassa, M. M., and Haydon, P. (2017). Astrocytic IP3/Ca2+ signaling modulates theta rhythm and REM Sleep. Front. Neural Circuits 11:3. doi: 10.3389/fncir.2017.00003
Franze, K., Grosche, J., Skatchkov, S. N., Schinkinger, S., Foja, C., Schild, D., et al. (2007). Müller cells are living optical fibers in the vertebrate retina. Proc. Natl. Acad. Sci. U.S.A. 104, 8287–8292. doi: 10.1073/pnas.0611180104
Fu, Y., Tucciarone, J. M., Espinosa, J. S., Sheng, N., Darcy, D. P., Nicoll, R. A., et al. (2014). A cortical circuit for gain control by behavioral state. Cell 156, 1139–1152. doi: 10.1016/j.cell.2014.01.050
Giaume, C., and McCarthy, K. D. (1996). Control of gap-junctional communication in astrocytic networks. Trends Neurosci. 19, 319–325. doi: 10.1016/0166-2236(96)10046-1
Grosche, J., Matyash, V., Möller, T., Verkhratsky, A., Reichenbach, A., and Kettenmann, H. (1999). Microdomains for neuron-glia interaction: parallel fiber signaling to Bergmann glial cells. Nat. Neurosci. 2, 139–143.
Halassa, M. M., Florian, C., Fellin, T., Munoz, J. R., Lee, S.-Y., Abel, T., et al. (2009). Astrocytic modulation of sleep homeostasis and cognitive consequences of sleep loss. Neuron 61, 213–219. doi: 10.1016/j.neuron.2008.11.024
Han, J., Kesner, P., Metna-Laurent, M., Duan, T., Xu, L., Georges, F., et al. (2012). Acute cannabinoids impair working memory through astroglial CB1 receptor modulation of hippocampal LTD. Cell 148, 1039–1050. doi: 10.1016/j.cell.2012.01.037
Han, S. K., Dong, X., Hwang, J. I., Zylka, M. J., Anderson, D. J., and Simon, M. I. (2002). Orphan G protein-coupled receptors Mrga1 and Mrgc11 are distinctively activated by RF-amide-related peptides through the Gαq/11 pathway. Proc. Natl. Acad. Sci. U.S.A. 99, 14740–14745. doi: 10.1073/pnas.192565799
Haustein, M. D., Kracun, S., Lu, X.-H., Shih, T., Jackson-Weaver, O., Tong, X., et al. (2014). Conditions and constraints for astrocyte calcium signaling in the hippocampal mossy fiber pathway. Neuron 82, 413–429. doi: 10.1016/j.neuron.2014.02.041
Henneberger, C., Papouin, T., Oliet, S. H. R., and Rusakov, D. A. (2010). Long-term potentiation depends on release of d-serine from astrocytes. Nature 463, 232–236. doi: 10.1038/nature08673
Holtzclaw, L. A., Pandhit, S., Bare, D. J., Mignery, G. A., and Russell, J. T. (2002). Astrocytes in adult rat brain express type 2 inositol 1,4,5-trisphosphate receptors. Glia 39, 69–84. doi: 10.1002/glia.10085
Hoogland, T. M., Kuhn, B., Göbel, W., Huang, W., Nakai, J., Helmchen, F., et al. (2009). Radially expanding transglial calcium waves in the intact cerebellum. Proc. Natl. Acad. Sci. U.S.A. 106, 3496–3501.
Iino, M., Goto, K., Kakegawa, W., Okado, H., Sudo, M., Ishiuchi, S., et al. (2001). Glia-synapse interaction through Ca2+-permeable AMPA receptors in Bergmann glia. Science 292, 926–929.
Ingiosi, A. M., Hayworth, C. R., Harvey, D. O., Singletary, K. G., Rempe, M. J., Wisor, J. P., et al. (2020). A role for astroglial calcium in mammalian sleep and sleep regulation. Curr. Biol. 30, 1–11. doi: 10.1016/j.cub.2020.08.052
Jackson, J. G., and Robinson, M. B. (2015). Reciprocal regulation of mitochondrial dynamics and calcium signaling in astrocyte processes. J. Neurosci. 35, 15199–15213. doi: 10.1523/JNEUROSCI.2049-15.2015
Jasper, H. H., and Tessier, J. (1971). Acetylcholine liberation from cerebral cortex during paradoxical (REM) sleep. Science 172, 601–602. doi: 10.1126/science.172.3983.601
Keller, G. B., Bonhoeffer, T., and Hübener, M. (2012). Sensorimotor mismatch signals in primary visual cortex of the behaving mouse. Neuron 74, 809–815. doi: 10.1016/j.neuron.2012.03.040
King, C. M., Bohmbach, K., Minge, D., Delekate, A., Zheng, K., Reynolds, J., et al. (2020). Local Resting Ca2+ controls the scale of astroglial Ca2+ Signals. Cell Rep. 30, 3466.e5–3477.e5. doi: 10.1016/j.celrep.2020.02.043
Kofuji, P., and Araque, A. (2020). G-Protein-Coupled receptors in astrocyte–neuron communication. Neuroscience 456, 71–84. doi: 10.1016/j.neuroscience.2020.03.025
Köhler, S., Winkler, U., and Hirrlinger, J. (2019). Heterogeneity of astrocytes in grey and white matter. Neurochem. Res. 46, 3–14. doi: 10.1007/s11064-019-02926-x
Kohro, Y., Matsuda, T., Yoshihara, K., Kohno, K., Koga, K., Katsuragi, R., et al. (2020). Spinal astrocytes in superficial laminae gate brainstem descending control of mechanosensory hypersensitivity. Nat. Neurosci. 23, 1376–1387. doi: 10.1038/s41593-020-00713-4
Kol, A., Adamsky, A., Groysman, M., Kreisel, T., London, M., and Goshen, I. (2020). Astrocytes contribute to remote memory formation by modulating hippocampal–cortical communication during learning. Nat. Neurosci. 23, 1229–1239. doi: 10.1038/s41593-020-0679-6
Kreitzer, A. C., Carter, A. G., and Regehr, W. G. (2002). Inhibition of interneuron firing extends the spread of endocannabinoid signaling in the cerebellum. Neuron 34, 787–796. doi: 10.1016/S0896-6273(02)00695-5
Kronschläger, M. T., Drdla-Schutting, R., Gassner, M., Honsek, S. D., Teuchmann, H. L., and Sandkühler, J. (2016). Gliogenic LTP spreads widely in nociceptive pathways. Science 354, 1144–1148. doi: 10.1126/science.aah5715
Kuchibhotla, K. V., Lattarulo, C. R., Hyman, B. T., and Bacskai, B. J. (2009). Synchronous hyperactivity and intercellular calcium waves in astrocytes in Alzheimer mice. Science 323, 1211–1215. doi: 10.1126/science.1169096
Kuffler, S. W. (1967). Neuroglial cells: physiological properties and a potassium mediated effect of neuronal activity on the glial membrane potential. Proc. R. Soc. Lond. Ser. B Biol. Sci. 168, 1–21. doi: 10.1098/rspb.1967.0047
Lee, H. S., Ghetti, A., Pinto-Duarte, A., Wang, X., Dziewczapolski, G., Galimi, F., et al. (2014). Astrocytes contribute to gamma oscillations and recognition memory. Proc. Natl. Acad. Sci. U.S.A. 111, E3343–E3352. doi: 10.1073/pnas.1410893111
Lee, S., Yoon, B.-E., Berglund, K., Oh, S.-J., Park, H., Shin, H.-S., et al. (2010). Channel-mediated tonic GABA release from glia. Science 330, 790–796. doi: 10.1126/science.1184334
Lichtman, A. H., and Martin, B. R. (1996). Δ9-Tetrahydrocannabinol impairs spatial memory through a cannabinoid receptor mechanism. Psychopharmacology 126, 125–131. doi: 10.1007/BF02246347
Lines, J., Martin, E. D., Kofuji, P., Aguilar, J., and Araque, A. (2020). Astrocytes modulate sensory-evoked neuronal network activity. Nat. Commun. 11:3689. doi: 10.1038/s41467-020-17536-3
Ma, Z., and Freeman, M. R. (2020). TrpML-mediated astrocyte microdomain Ca2+ transients regulate astrocyte-tracheal interactions. eLife 9:e58952. doi: 10.7554/eLife.58952
Ma, Z., Stork, T., Bergles, D. E., and Freeman, M. R. (2016). Neuromodulators signal through astrocytes to alter neural circuit activity and behaviour. Nature 539, 428–432. doi: 10.1038/nature20145
Maejima, T., Hashimoto, K., Yoshida, T., Aiba, A., and Kano, M. (2001). Presynaptic inhibition caused by retrograde signal from metabotropic glutamate to cannabinoid receptors. Neuron 31, 463–475. doi: 10.1016/S0896-6273(01)00375-0
Marcaggi, P., and Attwell, D. (2005). Endocannabinoid signaling depends on the spatial pattern of synapse activation. Nat. Neurosci. 8, 776–781. doi: 10.1038/nn1458
Marina, N., Christie, I. N., Korsak, A., Doronin, M., Brazhe, A., Hosford, P. S., et al. (2020). Astrocytes monitor cerebral perfusion and control systemic circulation to maintain brain blood flow. Nat. Commun. 11:131. doi: 10.1038/s41467-019-13956-y
McCaslin, A. F. H., Chen, B. R., Radosevich, A. J., Cauli, B., and Hillman, E. M. C. (2011). In vivo 3D morphology of astrocyte-vasculature interactions in the somatosensory cortex: Implications for neurovascular coupling. J. Cereb. Blood Flow Metab. 31, 795–806. doi: 10.1038/jcbfm.2010.204
Mederos, S., Hernández-Vivanco, A., Ramírez-Franco, J., Martín-Fernández, M., Navarrete, M., Yang, A., et al. (2019). Melanopsin for precise optogenetic activation of astrocyte-neuron networks. Glia 67, 915–934. doi: 10.1002/glia.23580
Mederos, S., Sánchez-Puelles, C., Esparza, J., Valero, M., Ponomarenko, A., and Perea, G. (2021). GABAergic signaling to astrocytes in the prefrontal cortex sustains goal-directed behaviors. Nat. Neurosci. 24, 82–92. doi: 10.1038/s41593-020-00752-x
Melom, J. E., and Littleton, J. T. (2013). Mutation of a NCKX eliminates glial microdomain calcium oscillations and enhances seizure susceptibility. J. Neurosci. 33, 1169–1178. doi: 10.1523/JNEUROSCI.3920-12.2013
Men, Y., Ye, L., Risgaard, R. D., Promes, V., Zhao, X., Paukert, M., et al. (2020). Astroglial FMRP deficiency cell-autonomously up-regulates miR-128 and disrupts developmental astroglial mGluR5 signaling. Proc. Natl. Acad. Sci. U.S.A. 117, 25092–25103. doi: 10.1073/pnas.2014080117
Mishra, A., Reynolds, J. P., Chen, Y., Gourine, A. V., Rusakov, D. A., and Attwell, D. (2016). Astrocytes mediate neurovascular signaling to capillary pericytes but not to arterioles. Nat. Neurosci. 19, 1619–1627. doi: 10.1038/nn.4428
Monai, H., Ohkura, M., Tanaka, M., Oe, Y., Konno, A., Hirai, H., et al. (2016). Calcium imaging reveals glial involvement in transcranial direct current stimulation-induced plasticity in mouse brain. Nat. Commun. 7:11100. doi: 10.1038/ncomms11100
Mothet, J. P., Billard, J. M., Pollegioni, L., Coyle, J. T., and Sweedler, J. V. (2019). Investigating brain d-serine: advocacy for good practices. Acta Physiol. 226:e13257. doi: 10.1111/apha.13257
Mu, Y., Bennett, D. V., Rubinov, M., Narayan, S., Yang, C.-T., Tanimoto, M., et al. (2019). Glia accumulate evidence that actions are futile and suppress unsuccessful behavior. Cell 178, 27.e19–43.e19. doi: 10.1016/j.cell.2019.05.050
Müller, T., Möller, T., Berger, T., Schnitzer, J., and Kettenmann, H. (1992). Calcium entry through kainate receptors and resulting potassium-channel blockade in Bergmann glial cells. Science 256, 1563–1566.
Nagai, J., Rajbhandari, A. K., Gangwani, M. R., Hachisuka, A., Coppola, G., Masmanidis, S. C., et al. (2019). Hyperactivity with disrupted attention by activation of an astrocyte synaptogenic cue. Cell 177, 1280.e20–1292.e20. doi: 10.1016/j.cell.2019.03.019
Navarrete, M., and Araque, A. (2008). Endocannabinoids mediate neuron-astrocyte communication. Neuron 57, 883–893. doi: 10.1016/j.neuron.2008.01.029
Navarrete, M., and Araque, A. (2010). Endocannabinoids potentiate synaptic transmission through stimulation of astrocytes. Neuron 68, 113–126. doi: 10.1016/j.neuron.2010.08.043
Navarrete, M., Cuartero, M. I., Palenzuela, R., Draffin, J. E., Konomi, A., Serra, I., et al. (2019). Astrocytic p38α MAPK drives NMDA receptor-dependent long-term depression and modulates long-term memory. Nat. Commun. 10:2968. doi: 10.1038/s41467-019-10830-9
Navarrete, M., Perea, G., de Sevilla, D. F., Gómez-Gonzalo, M., Núñez, A., Martín, E. D., et al. (2012). Astrocytes mediate in vivo cholinergic-induced synaptic plasticity. PLoS Biol. 10:e1001259. doi: 10.1371/journal.pbio.1001259
Nimmerjahn, A., Mukamel, E. A., and Schnitzer, M. J. (2009). Motor behavior activates Bergmann glial networks. Neuron 62, 400–412.
Nizar, K., Uhlirova, H., Tian, P., Saisan, P. A., Cheng, Q., Reznichenko, L., et al. (2013). In vivo stimulus-induced vasodilation occurs without IP3 receptor activation and may precede astrocytic calcium increase. J. Neurosci. 33, 8411–8422. doi: 10.1523/JNEUROSCI.3285-12.2013
Oh, S. J., and Lee, C. J. (2017). Distribution and function of the Bestrophin-1 (Best1) channel in the brain. Exp. Neurobiol. 26, 113–121. doi: 10.5607/en.2017.26.3.113
Oheim, M., Schmidt, E., and Hirrlinger, J. (2018). Local energy on demand: are ‘spontaneous’ astrocytic Ca2+-microdomains the regulatory unit for astrocyte-neuron metabolic cooperation? Brain Res. Bull. 136, 54–64. doi: 10.1016/j.brainresbull.2017.04.011
Ohno-Shosaku, T., Tsubokawa, H., Mizushima, I., Yoneda, N., Zimmer, A., and Kano, M. (2002). Presynaptic cannabinoid sensitivity is a major determinant of depolarization-induced retrograde suppression at hippocampal synapses. J. Neurosci. 22, 3864–3872. doi: 10.1523/jneurosci.22-10-03864.2002
Padmashri, R., Suresh, A., Boska, M. D., and Dunaevsky, A. (2015). Motor-skill learning is dependent on astrocytic activity. Neural Plast 2015:938023. doi: 10.1155/2015/938023
Panatier, A., Theodosis, D. T., Mothet, J. P., Touquet, B., Pollegioni, L., Poulain, D. A., et al. (2006). Glia-Derived d-Serine controls NMDA receptor activity and synaptic memory. Cell 125, 775–784. doi: 10.1016/j.cell.2006.02.051
Papouin, T., Dunphy, J. M., Tolman, M., Dineley, K. T., and Haydon, P. G. (2017). Septal cholinergic neuromodulation tunes the astrocyte-dependent gating of hippocampal NMDA Receptors to wakefulness. Neuron 94, 840.e7–854.e7. doi: 10.1016/j.neuron.2017.04.021
Paukert, M., Agarwal, A., Cha, J., Doze, V. A., Kang, J. U., and Bergles, D. E. (2014). Norepinephrine controls astroglial responsiveness to local circuit activity. Neuron 82, 1263–1270. doi: 10.1016/j.neuron.2014.04.038
Perea, G., Gómez, R., Mederos, S., Covelo, A., Ballesteros, J. J., Schlosser, L., et al. (2016). Activity-dependent switch of GABAergic inhibition into glutamatergic excitation in astrocyte-neuron networks. eLife 5:e20362. doi: 10.7554/eLife.20362
Petravicz, J., Boyt, K. M., and McCarthy, K. D. (2014). Astrocyte IP3R2-dependent Ca2+ signaling is not a major modulator of neuronal pathways governing behavior. Front. Behav. Neurosci. 8:384. doi: 10.3389/fnbeh.2014.00384
Petravicz, J., Fiacco, T. A., and McCarthy, K. D. (2008). Loss of IP3 receptor-dependent Ca2+ increases in hippocampal astrocytes does not affect baseline CA1 pyramidal neuron synaptic activity. J. Neurosci. 28, 4967–4973. doi: 10.1523/JNEUROSCI.5572-07.2008
Piet, R., and Jahr, C. E. (2007). Glutamatergic and purinergic receptor-mediated calcium transients in Bergmann glial cells. J. Neurosci. 27, 4027–4035.
Pinto-Duarte, A., Roberts, A. J., Ouyang, K., and Sejnowski, T. J. (2019). Impairments in remote memory caused by the lack of Type 2 IP3 receptors. Glia 67, 1976–1989. doi: 10.1002/glia.23679
Polack, P.-O., Friedman, J., and Golshani, P. (2013). Cellular mechanisms of brain state-dependent gain modulation in visual cortex. Nat. Neurosci. 16, 1331–1339. doi: 10.1038/nn.3464
Poskanzer, K. E., and Yuste, R. (2016). Astrocytes regulate cortical state switching in vivo. Proc. Natl. Acad. Sci. U.S.A. 113, E2675–E2684. doi: 10.1073/pnas.1520759113
Ransom, B. R., and Goldring, S. (1973). Ionic determinants of membrane potential of cells presumed to be glia in cerebral cortex of cat. J. Neurophysiol. 36, 855–868. doi: 10.1152/jn.1973.36.5.855
Reimer, J., McGinley, M. J., Liu, Y., Rodenkirch, C., Wang, Q., McCormick, D. A., et al. (2016). Pupil fluctuations track rapid changes in adrenergic and cholinergic activity in cortex. Nat. Commun. 7:13289. doi: 10.1038/ncomms13289
Robin, L. M., Oliveira da Cruz, J. F., Langlais, V. C., Martin-Fernandez, M., Metna-Laurent, M., Busquets-Garcia, A., et al. (2018). Astroglial CB1 receptors determine synaptic d-serine availability to enable recognition memory. Neuron 98, 935.e5–944.e5. doi: 10.1016/j.neuron.2018.04.034
Rose, C. R., Felix, L., Zeug, A., Dietrich, D., Reiner, A., and Henneberger, C. (2018). Astroglial glutamate signaling and uptake in the hippocampus. Front. Mol. Neurosci. 10:451. doi: 10.3389/fnmol.2017.00451
Rudolph, R., Jahn, H. M., Courjaret, R., Messemer, N., Kirchhoff, F., and Deitmer, J. W. (2016). The inhibitory input to mouse cerebellar Purkinje cells is reciprocally modulated by Bergmann glial P2Y1 and AMPA receptor signaling. Glia 64, 1265–1280. doi: 10.1002/glia.22999
Saab, A. S., Neumeyer, A., Jahn, H. M., Cupido, A., Šimek, A. A. M., Boele, H.-J., et al. (2012). Bergmann glial AMPA receptors are required for fine motor coordination. Science 337, 749–753. doi: 10.1126/science.1221140
Savtchouk, I., and Volterra, A. (2018). Gliotransmission: beyond black-and-white. J. Neurosci. 38, 14–25. doi: 10.1523/JNEUROSCI.0017-17.2017
Sharp, A. H., Nucifora, F. C., Blondel, O., Sheppard, C. A., Zhang, C., Snyder, S. H., et al. (1999). Differential cellular expression of isoforms of inositol 1,4,5- triphosphate receptors in neurons and glia in brain. J. Comp. Neurol. 406, 207–220. doi: 10.1002/(SICI)1096-9861(19990405)406:2<207:AID-CNE6<3.0.CO;2-7
Sherwood, M. W., Arizono, M., Hisatsune, C., Bannai, H., Ebisui, E., Sherwood, J. L., et al. (2017). Astrocytic IP3Rs: contribution to Ca2+ signalling and hippocampal LTP. Glia 65, 502–513. doi: 10.1002/glia.23107
Shigetomi, E., Jackson-Weaver, O., Huckstepp, R. T., O’Dell, T. J., and Khakh, B. S. (2013). TRPA1 channels are regulators of astrocyte basal calcium levels and long-term potentiation via constitutive d-serine release. J. Neurosci. 33, 10143–10153. doi: 10.1523/JNEUROSCI.5779-12.2013
Shigetomi, E., Kracun, S., and Khakh, B. S. (2010a). Monitoring astrocyte calcium microdomains with improved membrane targeted GCaMP reporters. Neuron Glia Biol. 6, 183–191. doi: 10.1017/S1740925X10000219
Shigetomi, E., Kracun, S., Sofroniew, M. V., and Khakh, B. S. (2010b). A genetically targeted optical sensor to monitor calcium signals in astrocyte processes. Nat. Neurosci. 13, 759–766. doi: 10.1038/nn.2557
Shigetomi, E., Tong, X., Kwan, K. Y., Corey, D. P., and Khakh, B. S. (2011). TRPA1 channels regulate astrocyte resting calcium and inhibitory synapse efficacy through GAT-3. Nat. Neurosci. 15, 70–80. doi: 10.1038/nn.3000
Siegel, A., Reichenbach, A., Hanke, S., Senitz, D., Brauer, K., and Smith, T. G. (1991). Comparative morphometry of Bergmann glial (Golgi epithelial) cells - A Golgi study. Anat. Embryol. 183, 605–612. doi: 10.1007/BF00187909
Smith, N. A., Kress, B. T., Lu, Y., Chandler-Militello, D., Benraiss, A., and Nedergaard, M. (2018). Fluorescent Ca2+ indicators directly inhibit the Na, K-ATPase and disrupt cellular functions. Sci. Signal. 11:eaal2039. doi: 10.1126/scisignal.aal2039
Srinivasan, R., Huang, B. S., Venugopal, S., Johnston, A. D., Chai, H., Zeng, H., et al. (2015). Ca2+ signaling in astrocytes from Ip3r2−/− mice in brain slices and during startle responses in vivo. Nat. Neurosci. 18, 708–717. doi: 10.1038/nn.4001
Srinivasan, R., Lu, T.-Y., Chai, H., Xu, J., Huang, B. S., Golshani, P., et al. (2016). New transgenic mouse lines for selectively targeting astrocytes and studying calcium signals in astrocyte processes In Situ and In Vivo. Neuron 92, 1181–1195. doi: 10.1016/j.neuron.2016.11.030
Stobart, J. L., Ferrari, K. D., Barrett, M. J. P., Glück, C., Stobart, M. J., Zuend, M., et al. (2018). Cortical circuit activity evokes rapid astrocyte calcium signals on a similar timescale to neurons. Neuron 98, 726.e4–735.e4. doi: 10.1016/j.neuron.2018.03.050
Sun, W., McConnell, E., Pare, J.-F., Xu, Q., Chen, M., Peng, W., et al. (2013). Glutamate-dependent neuroglial calcium signaling differs between young and adult brain. Science 339, 197–200. doi: 10.1126/science.1226740
Takahashi, K., Kayama, Y., Lin, J. S., and Sakai, K. (2010). Locus coeruleus neuronal activity during the sleep-waking cycle in mice. Neuroscience 169, 1115–1126. doi: 10.1016/j.neuroscience.2010.06.009
Takano, T., He, W., Han, X., Wang, F., Xu, Q., Wang, X., et al. (2014). Rapid manifestation of reactive astrogliosis in acute hippocampal brain slices. Glia 62, 78–95. doi: 10.1002/glia.22588
Takano, T., Tian, G. F., Peng, W., Lou, N., Libionka, W., Han, X., et al. (2006). Astrocyte-mediated control of cerebral blood flow. Nat. Neurosci. 9, 260–267. doi: 10.1038/nn1623
Takata, N., and Hirase, H. (2008). Cortical layer 1 and layer 2/3 astrocytes exhibit distinct calcium dynamics in vivo. PLoS One 3:e0002525. doi: 10.1371/journal.pone.0002525
Takata, N., Mishima, T., Hisatsune, C., Nagai, T., Ebisui, E., Mikoshiba, K., et al. (2011). Astrocyte calcium signaling transforms cholinergic modulation to cortical plasticity in vivo. J. Neurosci. 31, 18155–18165. doi: 10.1523/JNEUROSCI.5289-11.2011
Theis, M., and Giaume, C. (2012). Connexin-based intercellular communication and astrocyte heterogeneity. Brain Res. 1487, 88–98. doi: 10.1016/j.brainres.2012.06.045
Thrane, A. S., Rangroo Thrane, V., Zeppenfeld, D., Lou, N., Xu, Q., Nagelhus, E. A., et al. (2012). General anesthesia selectively disrupts astrocyte calcium signaling in the awake mouse cortex. Proc. Natl. Acad. Sci. U.S.A. 109, 18974–18979. doi: 10.1073/pnas.1209448109
Tran, C. H. T., Peringod, G., and Gordon, G. R. (2018). astrocytes integrate behavioral state and vascular signals during functional hyperemia. Neuron 100, 1133.e3–1148.e3. doi: 10.1016/j.neuron.2018.09.045
Tritsch, N. X., Yi, E., Gale, J. E., Glowatzki, E., and Bergles, D. E. (2007). The origin of spontaneous activity in the developing auditory system. Nature 450, 50–55. doi: 10.1038/nature06233
Turovsky, E. A., Braga, A., Yu, Y., Esteras, N., Korsak, A., Theparambil, S. M., et al. (2020). Mechanosensory signaling in astrocytes. J. Neurosci. 40, 9364–9371. doi: 10.1523/JNEUROSCI.1249-20.2020
Ventura, R., and Harris, K. M. (1999). Three-dimensional relationships between hippocampal synapses and astrocytes. J. Neurosci. 19, 6897–6906. doi: 10.1523/jneurosci.19-16-06897.1999
Vercesi, A. E., Kowaltowski, A. J., Grijalba, M. T., Meinicke, A. R., and Castilho, R. F. (1997). The role of reactive oxygen species in mitochondrial permeability transition. Biosci. Rep. 17, 43–52. doi: 10.1023/A:1027335217774
Verkhratsky, A., and Nedergaard, M. (2018). Physiology of astroglia. Physiol. Rev. 98, 239–389. doi: 10.1152/physrev.00042.2016
Wang, F., Xu, Q., Wang, W., Takano, T., and Nedergaard, M. (2012). Bergmann glia modulate cerebellar Purkinje cell bistability via Ca2+-dependent K+ uptake. Proc. Natl. Acad. Sci. U.S.A. 109, 7911–7916. doi: 10.1073/pnas.1120380109
Wang, H. C., Lin, C. C., Cheung, R., Zhang-Hooks, Y., Agarwal, A., Ellis-Davies, G., et al. (2015). Spontaneous activity of cochlear hair cells triggered by fluid secretion mechanism in adjacent support cells. Cell 163, 1348–1359. doi: 10.1016/j.cell.2015.10.070
Wang, W., Fang, H., Groom, L., Cheng, A., Zhang, W., Liu, J., et al. (2008). Superoxide flashes in single mitochondria. Cell 134, 279–290. doi: 10.1016/j.cell.2008.06.017
Weiss, S., Melom, J. E., Ormerod, K. G., Zhang, Y. V., and Littleton, J. T. (2019). Glial Ca2+signaling links endocytosis to K+buffering around neuronal somas to regulate excitability. eLife 8:e44186. doi: 10.7554/eLife.44186
Wilson, R. I., and Nicoll, R. A. (2001). Endogenous cannabinoids mediate retrograde signalling at hippocampal synapses. Nature 410, 588–592. doi: 10.1038/35069076
Wolosker, H., Balu, D. T., and Coyle, J. T. (2016). The rise and fall of the D-Serine-mediated gliotransmission hypothesis. Trends Neurosci. 39, 712–721. doi: 10.1016/j.tins.2016.09.007
Woo, J., Min, J. O., Kang, D. S., Kim, Y. S., Jung, G. H., Park, H. J., et al. (2018). Control of motor coordination by astrocytic tonic GABA release through modulation of excitation/inhibition balance in cerebellum. Proc. Natl. Acad. Sci. U.S.A. 115, 5004–5009. doi: 10.1073/pnas.1721187115
Yang, J., Yang, H., Liu, Y., Li, X., Qin, L., Lou, H., et al. (2016). Astrocytes contribute to synapse elimination via type 2 inositol 1,4,5-trisphosphate receptor-dependent release of ATP. eLife 5:e15043. doi: 10.7554/eLife.15043
Yang, Y., Ge, W., Chen, Y., Zhang, Z., Shen, W., Wu, C., et al. (2003). Contribution of astrocytes to hippocampal long-term potentiation through release of D-serine. Proc. Natl. Acad. Sci. U.S.A. 100, 15194–15199. doi: 10.1073/pnas.2431073100
Ye, L., Haroon, M. A., Salinas, A., and Paukert, M. (2017). Comparison of GCaMP3 and GCaMP6f for studying astrocyte Ca2+ dynamics in the awake mouse brain. PLoS One 12:e0181113. doi: 10.1371/journal.pone.0181113
Ye, L., Orynbayev, M., Zhu, X., Lim, E. Y., Dereddi, R. R., Agarwal, A., et al. (2020). Ethanol abolishes vigilance-dependent astroglia network activation in mice by inhibiting norepinephrine release. Nat. Commun. 11:6157. doi: 10.1038/s41467-020-19475-5
Yoon, B. E., Woo, J., Chun, Y. E., Chun, H., Jo, S., Bae, J. Y., et al. (2014). Glial GABA, synthesized by monoamine oxidase B, mediates tonic inhibition. J. Physiol. 592, 4951–4968. doi: 10.1113/jphysiol.2014.278754
Yu, X., Nagai, J., Marti-Solano, M., Soto, J. S., Coppola, G., Babu, M. M., et al. (2020). Context-specific striatal astrocyte molecular responses are phenotypically exploitable. Neuron 108, 1146.e10–1162.e10. doi: 10.1016/j.neuron.2020.09.021
Yu, X., Taylor, A. M. W., Nagai, J., Golshani, P., Evans, C. J., Coppola, G., et al. (2018). Reducing astrocyte calcium signaling in vivo alters striatal microcircuits and causes repetitive behavior. Neuron 99, 1170.e9–1187.e9. doi: 10.1016/j.neuron.2018.08.015
Zeng, W., Mak, D. O. D., Li, Q., Shin, D. M., Foskett, J. K., and Muallem, S. (2003). A new mode of Ca2+ signaling by G protein-coupled receptors: gating of IP3 receptor Ca2+ release channels by Gβγ. Curr. Biol. 13, 872–876. doi: 10.1016/S0960-9822(03)00330-0
Zhang, Y., Chen, K., Sloan, S. A., Bennett, M. L., Scholze, A. R., O’Keeffe, S., et al. (2014). An RNA-Sequencing transcriptome and splicing database of glia, neurons, and vascular cells of the cerebral cortex. J. Neurosci. 34, 11929–11947. doi: 10.1523/JNEUROSCI.1860-14.2014
Zhang, Y. V., Ormerod, K. G., and Littleton, J. T. (2017). Astrocyte Ca2+ influx negatively regulates neuronal activity. eNeuro 4:ENEURO.0340-16.2017. doi: 10.1523/ENEURO.0340-16.2017
Keywords: astroglia, behavioral state, calcium, astrocyte, neuromodulation, norepinephrine, Bergmann glia, vigilance
Citation: Lim EY, Ye L and Paukert M (2021) Potential and Realized Impact of Astroglia Ca2 + Dynamics on Circuit Function and Behavior. Front. Cell. Neurosci. 15:682888. doi: 10.3389/fncel.2021.682888
Received: 19 March 2021; Accepted: 03 May 2021;
Published: 07 June 2021.
Edited by:
Rolf Sprengel, Max Planck Institute for Medical Research (MPIMF), GermanyReviewed by:
Fritjof Helmchen, University of Zurich, SwitzerlandCopyright © 2021 Lim, Ye and Paukert. This is an open-access article distributed under the terms of the Creative Commons Attribution License (CC BY). The use, distribution or reproduction in other forums is permitted, provided the original author(s) and the copyright owner(s) are credited and that the original publication in this journal is cited, in accordance with accepted academic practice. No use, distribution or reproduction is permitted which does not comply with these terms.
*Correspondence: Martin Paukert, cGF1a2VydG1AdXRoc2NzYS5lZHU=
Disclaimer: All claims expressed in this article are solely those of the authors and do not necessarily represent those of their affiliated organizations, or those of the publisher, the editors and the reviewers. Any product that may be evaluated in this article or claim that may be made by its manufacturer is not guaranteed or endorsed by the publisher.
Research integrity at Frontiers
Learn more about the work of our research integrity team to safeguard the quality of each article we publish.