- 1Department of Anesthesiology, Guanghua School of Stomatology, Hospital of Stomatology, Sun Yat-sen University, Guangzhou, China
- 2Guangdong Provincial Key Laboratory of Stomatology, Institute of Stomatological Research, Sun Yat-sen University, Guangzhou, China
Mast cells (MCs) are immune cells and are widely distributed throughout the body. MCs are not only classically viewed as effector cells of some allergic diseases but also participate in host defense, innate and acquired immunity, homeostatic responses, and immunoregulation. Mounting evidence indicates that activation of MCs releasing numerous vasoactive and inflammatory mediators has effects on the nervous system and has been involved in different pain conditions. Here, we review the latest advances made about the implication of MCs in pain. Possible cellular and molecular mechanisms regarding the crosstalk between MC and the nervous system in the initiation and maintenance of pain are also discussed.
Introduction
Pain is a hallmark of inflammation that can be either protective or detrimental during acute or chronic stages. The development and maintenance of chronic pain are involved in neuronal sensitization (Ji et al., 2016). It has long been postulated that interactions between the nervous system and immune system contribute to the pathophysiology of pain. Following intense noxious stimulation, neuropeptides and neurotransmitters released by nociceptors result in neurogenic inflammation and the recruitment of immune cells, whereas infiltrated immune cells release mediators to enhanced responsiveness of sensory neurons. Such positive feedback loops may underlie pain induction (Liu et al., 2021).
Considerable evidence suggests that mast cells (MCs), effectors of innate immunity and local inflammation, regulate pain signaling, for example, by secreting mediators that activate nearby nerves based on their histological proximity (Chompunud Na Ayudhya et al., 2020; Aguilera-Lizarraga et al., 2021). Here, we discuss the role of MCs in pain initiation and maintenance via MC-neuron crosstalk. Possible molecular mechanisms and resolution of pain associated with MC are demonstrated. Importantly, the identification of the pathological role of MCs in neuroimmune interactions will provide us novel strategies operative in pain.
Mast Cell Basics
MCs derive from CD34/CD117-expressing multipotent hematopoietic precursor cells in the bone marrow, which circulate in the bloodstream and are transited out of the circulation to the peripheral tissues where they attain their maturity (Metcalfe et al., 1997; Figure 1). Mature MCs can exert instant effects on vascular function (Albert-Bayo et al., 2019) and sensory neurons as they are in close proximity to vasculature and nerve fibers innervating derma (Morellini et al., 2018), visceral organs (Barbara et al., 2004), meninges (Levy et al., 2007; Hassler et al., 2019), brain parenchyma (Ocak et al., 2019), and hypothalamus (Edvinsson et al., 1977).
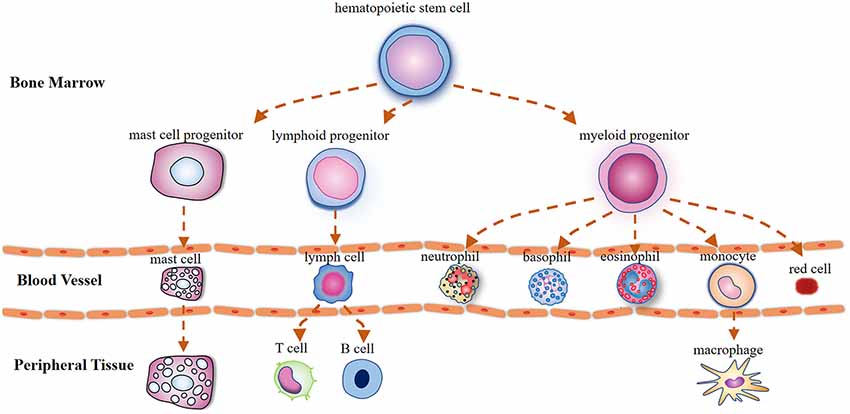
Figure 1. Illustration outlining the mast cells (MCs) differentiation trajectories. Mast cells, lymphocytes, and myeloid cells are derived from pluripotent hematopoietic progenitors in the bone marrow. Unlike basophils that attain their maturity in the circulation, mast cell precursors circulate in the bloodstream as immature cells and are transited out to the peripheral tissues where they mature under the influence of growth factors.
MCs can be activated through a variety of mechanisms. Of these, allergens and pathogens acting on their respective receptors expressed on MCs, such as the high-affinity immunoglobulin E receptor and toll-like receptor, represents the classical model of MC activation (González-de-Olano and Álvarez-Twose, 2018). Notably, MCs can be activated by membrane receptors that can not only detect thermal and physical stimuli [e.g., the transient receptor potential vanilloid (TRPV) family] (Zhang D. et al., 2012; Solís-López et al., 2017), but also detect a variety of endogenous mediators, including neuropeptides and neurotransmitters released by nociceptive neurons [e.g., Mrgprb2/X2, a G protein-coupled receptor responsive to substance P (SP)] (Green et al., 2019).
Following activation, MCs release their granule-stored mediators and then secret re-synthesized granules as a late response, called “de novo synthesis” (Vukman et al., 2017). The former process is termed “degranulation”, in which MCs release pre-formed granules within minutes. These mediators include biogenic amines (e.g., histamine and serotonin), proteases (e.g., tryptase and chymase), proteoglycans (e.g., heparin) tumor necrosis factor alpha (TNFα), leukotrienes, cytokines, and chemokines that facilitate the migration of other immune cells (González-de-Olano and Álvarez-Twose, 2018). They can be recognized in tissues with toluidine blue staining due to the large cytoplasmic granules (mainly heparin) in cells (Eady, 1976).
Mast Cell Involved in Painful Conditions
MCs are located in the vicinity of nociceptive C-fibers and may interact with nerve endings through the “synapse like” connection (Suzuki et al., 2004). Increased MCs were observed in patients with headaches (Friesen et al., 2018), non-cardiac chest pain (Lee et al., 2014), and self-injurious behavior-associated pain (Symons et al., 2009). Pain-like behaviors have been found to be MC-associated, including models of post-fracture nociception (Li et al., 2012), cancer pain (Lam and Schmidt, 2010; Yu et al., 2019), postoperative pain (Oliveira et al., 2013), fibromyalgia (muscle pain; Theoharides et al., 2019), sickle cell anemia-associated pain (Vang et al., 2015) and visceral hypersensitivity, as is indicated in irritable bowel syndromes (Di Nardo et al., 2014), chronic pelvic pain (Done et al., 2012), interstitial cystitis (IC; Wang et al., 2016; Martin Jensen et al., 2018), and neonatal maternal separation (Chen et al., 2021). Mastocytosis, characterized by constitutive hyperactivity of MC, is often accompanied by pain syndromes (Giannetti and Filice, 2021). Additionally, MC stabilizers significantly attenuate hyperalgesia in inflammatory pain models induced by formalin (Nakajima et al., 2009), potamotrygon venom (Kimura et al., 2018), nerve growth factor (NGF), and dynorphin (Kissel et al., 2017).
Taken as a whole, the results indicate that MCs play an important role in different painful conditions, although some studies showed that depletion or stabilization of MC did not display pain-relieving effect in models induced by complete Freund’s adjuvant, carrageenan, formalin, NGF, or nociceptin/orphanin (McDougall and Larson, 2006; Xanthos et al., 2011; Lopes et al., 2017; Magnusdottir et al., 2018). Whether MC plays a critical role in nociceptive processing remains to be elucidated.
Mechanistic Insights into The Dialog Between Neuron and Mast Cell
MCs are well recognized for their sufficient role in inflammation but much less is known about their contributions to pain pathways. MC may increase the excitability of nociceptors by releasing pro-nociceptive molecules, whose receptors are expressed on sensory neurons (Loewendorf et al., 2016). Mediators released by nociceptive sensory neurons, in turn, regulate the maturation, recruitment, and degranulation of MCs through the activation of their respective membrane receptors on MCs (Serhan and Basso, 2019; Koyuncu Irmak et al., 2019; Figure 2).
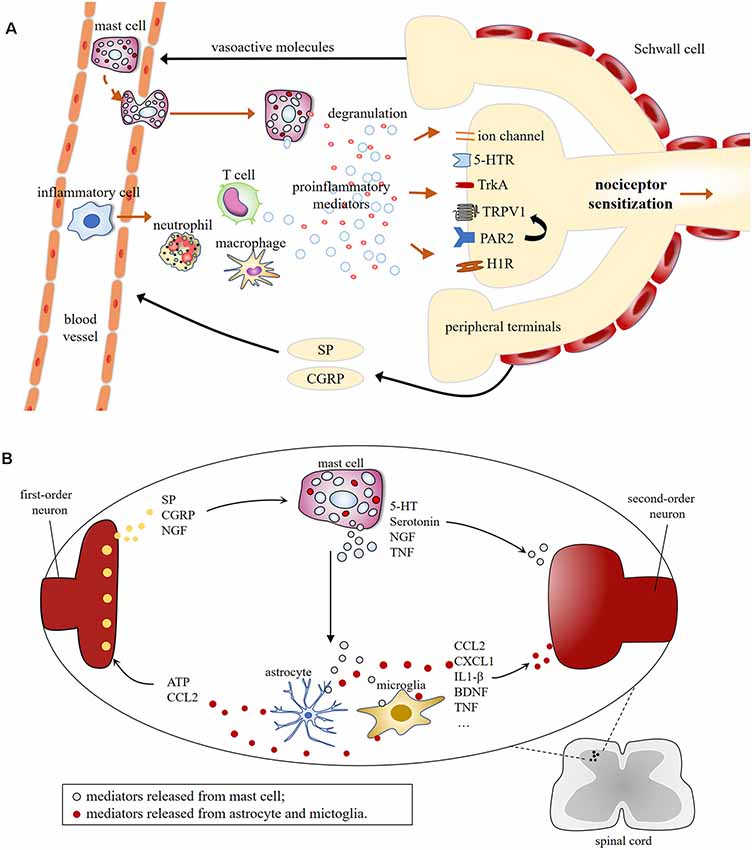
Figure 2. (A) Schematic illustration of mast cell involvement in peripheral sensitization in the terminals of nociceptive primary afferents. Mast cell degranulation induces the production of proinflammatory mediators [e.g., 5-HT, TNF, nerve growth factor (NGF), histamine, tryptase], resulting in nociceptive neurons release vasoactive neuropeptides, which in turn, leads to the recruitment of immune cells, including mast cells, macrophage, neutrophil, T cell, etc. This leads to the possibility of positive feedback loop, which could lead to chronic pain. (B) Molecular mechanisms of central sensitization induced by mast cells degranulation in first-order excitatory synapses, where communications between neuronal and non-neuronal cells occur. Central terminals of nociceptors release inflammatory factors that activate the second-order neurons and non-neuronal cells including mast cells, which induces neuronal activation via producing proinflammatory cytokines and chemokines [e.g., TNF, interleukins (IL)-1β, CCL2, CXCL1], and granular components, such as 5-HT and serotonin.
Neuropeptides
Neuropeptides, critical inducers of neurogenic inflammation, are primarily released from nociceptors following intense noxious stimulation and/or activation of different molecular sensors, such as TRP channels (e.g., TRPV1; Sousa-Valente and Brain, 2018; Yakubova and Davidyuk, 2021), G protein-coupled receptors [proteinase-activated receptor 2 (PAR2) and Mrgprb2] (Wei et al., 2016), sodium channels (Nav1.9; Bonnet et al., 2019), and mechanosensitive Piezo receptors (Mikhailov et al., 2019).
SP and calcitonin gene-related peptide (CGRP) are two of the pivotal neuropeptides implicated in neurogenic inflammation and pain. Recent evidence suggests that SP promotes the recruitment of innate immune cells and the release of pro-inflammatory mediators via activation of the Mrgprb2 receptor expressed by MCs (Green et al., 2019). A recent report reveals a regulatory effect of CGRP in MCs using RNA-sequencing, in which differentially expressed genes are enriched in biological processes associated with transcription, MC activation, and proliferation after CGRP treatment (Sun et al., 2020). Although MCs abundantly express receptors for neuropeptides (Le et al., 2016), however, many neuropeptides have less well-defined roles in MC-mediated pain.
In turn, MCs may exacerbate inflammation and pain signals via modulating SP production. MCs reside in the microenvironment where SP- immunoreactive nerve fibers are located and modify the degradation of SP by releasing tryptase and chymase (Caughey et al., 1988). Pharmacological inhibition on MCs significantly reduces the release of SP and ameliorates hyperalgesia in sickle mice (Vincent et al., 2013). Identification of the modulatory effects of MCs on SP and CGRP may provide insights into the neuro-immune interaction, but not exclusively, pain hypersensitivity.
Serotonin
Serotonin, or 5-hydroxytryptamine (5-HT), is a neurotransmitter that distributes mainly in the central nervous system and it is involved in the regulation of numerous behavioral and physiological processes, such as perception, memory, and mood (Bamalan and Al Khalili, 2020). Recent studies suggest that serotonin can be released from peripheral MC and promote pain during tissue injury (Sommer, 2004).
The expression level of 5-HT was upregulated in pain models induced by acute inflammation (Nakajima et al., 2009), surgery (Oliveira et al., 2011), and migraine (Koroleva et al., 2019), which can be significantly attenuated by MC stabilizer or MC deficiency. Patients with abdominal pain showed a significantly increased release of 5-HT, which has a significant correlation with MCs counts and the severity of pain (Taylor et al., 2010; Cremon et al., 2011).
5-HT is also a powerful neuromodulator with a receptor-dependent effect. Several subtypes of serotonin receptors, such as 5-HT(1)A (Coelho et al., 1998), 5-HT(3) (Yan et al., 2014), and 5-HT(2A) receptors (Oliveira et al., 2011), have been found associated with nociceptive responses mediated by MC. Selected tricyclic antidepressants, capable of inhibiting 5-HT secretion from MCs, are well introduced in chronic pain treatment, which expand our understanding of mechanisms underlying the pathophysiology of pain (Ferjan and Lipnik-Stangelj, 2013).
Histamine
Histamine is present within all bodily tissues, stored in secretory vesicles that are released by MCs and basophils. Histamine regulates various physiological and pathological processes, such as autoimmune conditions, vasodilation, hematopoiesis, and neurotransmission (Obara et al., 2020), which are facilitated by binding to histamine H 1, H2, H3, and H4 receptors that differ in their tissue expression patterns and functions (Obara et al., 2020; Patel and Mohiuddin, 2020).
Accumulating evidence indicates that MC-derived histamine serves as mediator to pain. Treatment with MC stabilizers and/or histamine antagonists significantly ameliorates vincristine/paclitaxel-induced hyperalgesia (Gao et al., 2016; Schneider, 2017). Blockade of H1 receptor in pain models with increased MCs infiltration inhibits or reduces prostatitis-associated pelvic pain (Done et al., 2012), visceral hypersensitivity (Barbara et al., 2007), venom-induced mechanical allodynia (Lauria et al., 2018), and post-operative nociception (Oliveira et al., 2011). H2 receptors also indicated in hyperalgesia and allodynia mediated by MC histamine in inflammatory pain (Massaad et al., 2004), vincristine-induced neuropathic pain (Jaggi et al., 2017), and IC pain (Rudick et al., 2008). Given the efficacy of histamine antagonists in treating hyperalgesia, inhibition on MC degranulation may provide a promising target in pain control (Obara et al., 2020).
Tryptase
Tryptase is a trypsin-like serine protease produced by MCs. It serves as a marker of MC activation. The release of tryptase has been proven to be attributed to activation of Kit receptor in MCs (Grimbaldeston et al., 2005; Ammendola et al., 2013; Chen et al., 2021).
MC tryptases are essential for inflammation and nociceptive responses (Hoffmeister et al., 2011; Borbély et al., 2016). Clinically, there was a significant correlation between the intensity of pain and tryptase levels in patients who are with the complex regional pain syndrome (Huygen et al., 2004). Increased level of tryptase in the incised tissue was detected in most patients who were undergoing moderate-to-severe pain for up to 1 month (Pepper et al., 2013). Tryptase may be involved in pain through cleaving and activating its receptor PAR2 expressed on sensory neurons (Anaf et al., 2006; Bunnett, 2006). As pretreatment with PAR2 antagonist was capable of attenuating chronic visceral hyperalgesia (Roman et al., 2014), preventing postoperative nociception (Oliveira et al., 2013), and abolishing cancer-dependent allodynia (Lam and Schmidt, 2010).
Some studies revealed that tryptase-PAR2 may affect neurogenic inflammation and pain transmission via regulating the activity of TRP ankyrin 1 and TRPV1, TRPV4 channels of sensory neurons (Dai et al., 2004, 2007; Zhao et al., 2014), by phospholipase C, protein kinase A, and protein kinase C-dependent mechanisms (Chen et al., 2011). Moreover, MC tryptase activates neutrophil (de Almeida et al., 2020) and microglia (Zhang S. et al., 2012), which are important culprits for inflammation and exerts an active role in pain (Tsuda, 2018). MC tryptase has been implicated in peripheral and central sensitization, albeit there remain large gaps in our knowledge about the tryptase-mediated mechanism of nociception.
Cytokines
Cytokines are synthesized mainly by the immune and nervous system and are responsible for the regulation of differentiation, inflammation, immune responses, cell apoptosis, and necrosis via transmitting signals between cells (Totsch and Sorge, 2017; Zahari et al., 2017). Additionally, cytokines contribute significantly to pain arising from nociceptor activation. A range of cytokines, including TNFα, interleukins (IL)-1beta, IL-6, IL-17, granulocyte macrophage colony-stimulating factor (GM-CSF), have been shown to play prominent roles in sensitizing neuronal cells via their specific receptors (Cook et al., 2018).
Non-neuronal cells, such as MCs, monocytes, lymphocytes, are producers of TNF (Grivennikov et al., 2005). A previous finding has identified MCs as an important source of both preformed and immunologically inducible TNF implicated in different biological responses (Gordon and Galli, 1990). After being activated, MCs rapidly secret granule-stored TNF through degranulation and then release the de novo synthesized TNF 24 h later (Zhang B. et al., 2012). TNFα, as a neuro-sensitizing molecule, causes neurogenic inflammation and a lowering of the threshold to stimulation (Wheeler et al., 2014), which may be attributed to activation of cyclooxygenase and the p38 MAP kinase (Zhang et al., 2011). TNFα binds to its receptors and initiates the generation and release of inflammatory mediators produced by immune cells, including MCs (Yang et al., 2018). However, a study of IC pain models that displays an increased number of MCs fails to suggest a role for TNFα in initiating nociception (Rudick et al., 2008).
IL-33 (Martin Jensen et al., 2018) and IL-1beta that secreted from MCs in response to inflammatory molecules, such as lipopolysaccharide and SP, may involve in the processing of local inflammation and hypersensitivity (Coelho et al., 2000; Ebenezer et al., 2018; Taracanova et al., 2018). The neuro-sensitizing effects of some inflammatory cytokines generated and secreted from MCs, such as IL-2, IL-5, IL-6, IL-9, IL-10, IL-11, IL-16, IL-37 and platelet-derived growth factor (Mukai et al., 2018; Conti et al., 2019), need to be validated.
NGF
NGF is believed to be an important mediator in peripheral hyperalgesia (Pezet and McMahon, 2006). NGF is stored and released by a range of cell types, such as MCs, macrophages, and the sensory and sympathetic neurons (Bandtlow et al., 1987; Liu et al., 2021).
A vitro study reveals that MCs can synthesize, store, and release NGF in response to antigen/IgE stimulation (Leon et al., 1994), while NGF induces human MCs differentiation, maturation, and degranulation (Skaper, 2017). On the one hand, NGF released from MC have profound implications in pain-associated pathobiology, such as osteoarthritis pain (Sousa-Valente et al., 2018) and visceral hypersensitivity (Li et al., 2019). MC-derived NGF may participate in long–lasting peripheral sensitization by governing the enteric synaptic plasticity (Zhang et al., 2018). On the other hand, as MCs express receptors for NGF (Tam et al., 1997), endogenous NGF can elicit the degranulation of MCs, which may be relevant to the early stages of peripheral sensitization and inflammation (Marshall et al., 1990; Groneberg et al., 2005; Sousa-Valente et al., 2018) as well as central sensitization (Kissel et al., 2017).
From the foregoing, it can be concluded that the crosstalk between NGF and MCs may contribute to tissue inflammation and hyperalgesia via amplifying each other’s effects. However, the detailed mechanisms of their interaction warrant further research.
Conclusion
The recent flood of evidence demonstrates the involvement of MCs in painful conditions and suggests a possible mechanism of MCs to pain pathobiology. Noxious stimuli can rapidly activate resident MCs at the injured site, where they release neuro-sensitizing molecules that induce peripheral sensitization, local inflammation, and the recruitment of other immune cells. Meanwhile, MCs interact with mediators that are critical for the maintenance of pain. MCs also modulate nociception centrally via enhancing neuronal sensitivity and altering the permeability of the blood-brain barrier (Esposito et al., 2001), allowing the infiltration of additional cells (Figure 2).
The involvement of the immune system in pain appears to be more common than once thought, as common analgesics are often not sufficient to control pain associated with MC activation (Butterfield, 2009; Aich et al., 2015). Systemic MC activation disease (MCAD) is characterized by the accumulation of genetically altered dysfunctional MCs with the abnormal release of these cells’ mediators. Although therapeutic alternatives in MCAD patients with pain are drugs that profoundly stabilize MCs, it remains a challenge considering its adverse effects on human beings (Wirz and Molderings, 2017). Based on the demonstrated efficacy in pain, analgesics that can significantly mitigate MC degranulation, such as morphine (Vincent et al., 2016), Palmitoylethanolamide (D’Amico and Impellizzeri, 2020), and ketotifen (Klooker et al., 2010), are promising for treating all those painful conditions in which MC activation is the main cause. Pharmacological targeting of MC proliferation, specific surface antigens, and downstream signaling pathways, in addition to stabilizing MCs, may improve analgesics therapy (Molderings et al., 2016).
Given that MC serves as important source of proinflammatory mediators in sustained nociceptive sensitization, new strategies to manipulate crosstalk between neurons and MC hold considerable promise. However, mechanisms of pain are still emerging, and the molecular mechanisms of MC-mediated pain are worth exploring.
Author Contributions
WF and LM designed and drafted the manuscript and figures. QL analyzed the data. QL, FH, and HH revised the manuscript. All authors contributed to the article and approved the submitted version.
Funding
This work was supported partly by the National Natural Science Foundation of China-Guangdong Joint Fund (No. 81771098 and 81541153).
Conflict of Interest
The authors declare that the research was conducted in the absence of any commercial or financial relationships that could be construed as a potential conflict of interest.
References
Aguilera-Lizarraga, J., Florens, M. V., and Viola, M. F. (2021). Local immune response to food antigens drives meal-induced abdominal pain. Nature 590, 151–156. doi: 10.1038/s41586-020-03118-2
Aich, A., Afrin, L. B., and Gupta, K. (2015). Mast Cell-Mediated Mechanisms of Nociception. Int. J. Mol. Sci. 16, 29069–29092. doi: 10.3390/ijms161226151
Albert-Bayo, M., Paracuellos, I., González-Castro, A. M., Rodríguez-Urrutia, A., Rodríguez-Lagunas, M. J., Alonso-Cotoner, C., et al. (2019). Intestinal mucosal mast cells: key modulators of barrier function and homeostasis. Cells 8:135. doi: 10.3390/cells8020135
Ammendola, M., Sacco, R., Sammarco, G., Donato, G., Zuccalà, V., Romano, R., et al. (2013). Mast cells positive to tryptase and c-kit receptor expressing cells correlates with angiogenesis in gastric cancer patients surgically treated. Gastroenterol. Res. Pract. 2013:703163. doi: 10.1155/2013/703163
Anaf, V., Chapron, C., El Nakadi, I., De Moor, V., Simonart, T., Noël, J. C., et al. (2006). Pain, mast cells and nerves in peritoneal, ovarian and deep infiltrating endometriosis. Fertil. Steril. 86, 1336–1343. doi: 10.1016/j.fertnstert.2006.03.057
Bamalan, O. A., and Al Khalili, Y. (2020). “Physiology serotonin,” in StatPearls [Internet], (Treasure Island, FL: StatPearls Publishing). Available online at: https://www.ncbi.nlm.nih.gov/books/NBK545168/.
Bandtlow, C. E., Heumann, R., Schwab, M. E., and Thoenen, H. (1987). Cellular localization of nerve growth factor synthesis by in situ hybridization. Embo J. 6, 891–899.
Barbara, G., Stanghellini, V., De Giorgio, R., Cremon, C., Cottrell, G. S., Santini, D., et al. (2004). Activated mast cells in proximity to colonic nerves correlate with abdominal pain in irritable bowel syndrome. Gastroenterology 126, 693–702. doi: 10.1053/j.gastro.2003.11.055
Barbara, G., Wang, B., Stanghellini, V., de Giorgio, R., Cremon, C., Di Nardo, G., et al. (2007). Mast cell-dependent excitation of visceral-nociceptive sensory neurons in irritable bowel syndrome. Gastroenterology 132, 26–37. doi: 10.1053/j.gastro.2006.11.039
Bonnet, C., Hao, J., Osorio, N., Donnet, A., Penalba, V., Ruel, J., et al. (2019). Maladaptive activation of Nav1.9 channels by nitric oxide causes triptan-induced medication overuse headache. Nat. Commun. 10:4253. doi: 10.1038/s41467-019-12197-3
Borbély, É, Sándor, K., Markovics, A., Kemény, Á, Pintér, E., Szolcsányi, J., et al. (2016). Role of capsaicin-sensitive nerves and tachykinins in mast cell tryptase-induced inflammation of murine knees. Inflamm. Res. 65, 725–736. doi: 10.1007/s00011-016-0954-x
Bunnett, N. W. (2006). Protease-activated receptors: how proteases signal to cells to cause inflammation and pain. Semin. Thromb. Hemost. 32, 39–48. doi: 10.1055/s-2006-939553
Butterfield, J. H. (2009). Survey of aspirin administration in systemic mastocytosis. Prostaglandins Other Lipid Mediat. 88, 122–124. doi: 10.1016/j.prostaglandins.2009.01.001
Caughey, G. H., Leidig, F., Viro, N. F., and Nadel, J. A. (1988). Substance P and vasoactive intestinal peptide degradation by mast cell tryptase and chymase. J. Pharmacol. Exp. Ther. 244, 133–137.
Chen, Y., Yang, C., and Wang, Z. J. (2011). Proteinase-activated receptor 2 sensitizes transient receptor potential vanilloid 1, transient receptor potential vanilloid 4 and transient receptor potential ankyrin 1 in paclitaxel-induced neuropathic pain. Neuroscience 193, 440–451. doi: 10.1016/j.neuroscience.2011.06.085
Chen, Z., Zhou, T., Zhang, Y., Dong, H., and Jin, W. (2021). Mast cells in the paraventricular nucleus participate in visceral hypersensitivity induced by neonatal maternal separation. Behav. Brain Res. 402:113113. doi: 10.1016/j.bbr.2020.113113
Chompunud Na Ayudhya, C., Roy, S., Thapaliya, M., and Ali, H. (2020). Roles of a mast cell-specific receptor MRGPRX2 in host defense and inflammation. J. Dent. Res. 99, 882–890. doi: 10.1177/0022034520919107
Coelho, A. M., Fioramonti, J., and Buéno, L. (2000). Systemic lipopolysaccharide influences rectal sensitivity in rats: role of mast cells, cytokines and vagus nerve. Am. J. Physiol. Gastrointest. Liver Physiol. 279, G781–G790. doi: 10.1152/ajpgi.2000.279.4.G781
Coelho, A. M., Fioramonti, J., and Bueno, L. (1998). Mast cell degranulation induces delayed rectal allodynia in rats: role of histamine and 5-HT. Dig. Dis. Sci. 43, 727–737. doi: 10.1023/a:1018853728251
Conti, P., D’Ovidio, C., Conti, C., Gallenga, C. E., Lauritano, D., Caraffa, A., et al. (2019). Progression in migraine: role of mast cells and pro-inflammatory and anti-inflammatory cytokines. Eur. J. Pharmacol. 844, 87–94. doi: 10.1016/j.ejphar.2018.12.004
Cook, A. D., Christensen, A. D., Tewari, D., McMahon, S. B., and Hamilton, J. A. (2018). Immune cytokines and their receptors in inflammatory pain. Trends Immunol. 39, 240–255. doi: 10.1016/j.it.2017.12.003
Cremon, C., Carini, G., Wang, B., Vasina, V., Cogliandro, R. F., De Giorgio, R., et al. (2011). Intestinal serotonin release, sensory neuron activation and abdominal pain in irritable bowel syndrome. Am. J. Gastroenterol. 106, 1290–1298. doi: 10.1038/ajg.2011.86
D’Amico, R., and Impellizzeri, D. (2020). ALIAmides update: palmitoylethanolamide and its formulations on management of peripheral neuropathic pain. Int. J. Mol. Sci. 21:5330. doi: 10.3390/ijms21155330
Dai, Y., Moriyama, T., Higashi, T., Togashi, K., Kobayashi, K., Yamanaka, H., et al. (2004). Proteinase-activated receptor 2-mediated potentiation of transient receptor potential vanilloid subfamily 1 activity reveals a mechanism for proteinase-induced inflammatory pain. J. Neurosci. 24, 4293–4299. doi: 10.1523/JNEUROSCI.0454-04.2004
Dai, Y., Wang, S., Tominaga, M., Yamamoto, S., Fukuoka, T., Higashi, T., et al. (2007). Sensitization of TRPA1 by PAR2 contributes to the sensation of inflammatory pain. J. Clin. Invest. 117, 1979–1987. doi: 10.1172/JCI30951
de Almeida, A. D., Silva, I. S., Fernandes-Braga, W., LimaFilho, A. C. M., Florentino, R. O. M., Barra, A., et al. (2020). A role for mast cells and mast cell tryptase in driving neutrophil recruitment in LPS-induced lung inflammation via protease-activated receptor 2 in mice. Inflamm. Res. 69, 1059–1070. doi: 10.1007/s00011-020-01376-4
Di Nardo, G., Barbara, G., Cucchiara, S., Cremon, C., Shulman, R. J., Isoldi, S., et al. (2014). Neuroimmune interactions at different intestinal sites are related to abdominal pain symptoms in children with IBS. Neurogastroenterol. Motil. 26, 196–204. doi: 10.1111/nmo.12250
Done, J. D., Rudick, C. N., Quick, M. L., Schaeffer, A. J., and Thumbikat, P. (2012). Role of mast cells in male chronic pelvic pain. J. Urol. 187, 1473–1482. doi: 10.1016/j.juro.2011.11.116
Eady, R. A. (1976). The mast cells: distribution and morphology. Clin. Exp. Dermatol. 1, 313–321. doi: 10.1111/j.1365-2230.1976.tb01436.x
Ebenezer, A. J., Prasad, K., Rajan, S., and Thangam, E. B. (2018). Silencing of H4R inhibits the production of IL-1β through SAPK/JNK signaling in human mast cells. J. Recept. Signal. Transduct. Res. 38, 204–212. doi: 10.1080/10799893.2018.1468783
Edvinsson, L., Cervós-Navarro, J., Larsson, L. I., Owman, C., and Rönnberg, A. L. (1977). Regional distribution of mast cells containing histamine, dopamine, or 5-hydroxytryptamine in the mammalian brain. Neurology 27, 878–883. doi: 10.1212/wnl.27.9.878
Esposito, P., Gheorghe, D., Kandere, K., Pang, X., Connolly, R., Jacobson, S., et al. (2001). Acute stress increases permeability of the blood-brain-barrier through activation of brain mast cells. Brain Res. 888, 117–127. doi: 10.1016/s0006-8993(00)03026-2
Ferjan, I., and Lipnik-Stangelj, M. (2013). Chronic pain treatment: the influence of tricyclic antidepressants on serotonin release and uptake in mast cells. Mediators Inflamm. 2013:340473. doi: 10.1155/2013/340473
Friesen, C., Singh, M., Singh, V., and Schurman, J. V. (2018). An observational study of headaches in children and adolescents with functional abdominal pain: relationship to mucosal inflammation and gastrointestinal and somatic symptoms. Medicine (Baltimore) 97:e11395. doi: 10.1097/MD.0000000000011395
Gao, W., Zan, Y., Wang, Z. J., Hu, X. Y., and Huang, F. (2016). Quercetin ameliorates paclitaxel-induced neuropathic pain by stabilizing mast cells and subsequently blocking PKCepsilon-dependent activation of TRPV1. Acta Pharmacol. Sin. 37, 1166–1177. doi: 10.1038/aps.2016.58
Giannetti, A., and Filice, E. (2021). Mast cell activation disorders. Medicina 57:124. doi: 10.3390/medicina57020124
González-de-Olano, D., and Álvarez-Twose, I. (2018). Mast cells as key players in allergy and inflammation. J. Investig. Allergol. Clin. Immunol. 28, 365–378. doi: 10.18176/jiaci.0327
Gordon, J. R., and Galli, S. J. (1990). Mast cells as a source of both preformed and immunologically inducible TNF-alpha/cachectin. Nature 346, 274–276. doi: 10.1038/346274a0
Green, D. P., Limjunyawong, N., Gour, N., Pundir, P., and Dong, X. (2019). A mast-cell-specific receptor mediates neurogenic inflammation and pain. Neuron 101, 412–420.e3. doi: 10.1016/j.neuron.2019.01.012
Grimbaldeston, M. A., Chen, C. C., Piliponsky, A. M., Tsai, M., Tam, S. Y., and Galli, S. J. (2005). Mast cell-deficient W-sash c-kit mutant Kit W-sh/W-sh mice as a model for investigating mast cell biology in vivo. Am. J. Pathol. 167, 835–848. doi: 10.1016/S0002-9440(10)62055-X
Grivennikov, S. I., Tumanov, A. V., Liepinsh, D. J., Kruglov, A. A., Marakusha, B. I., Shakhov, A. N., et al. (2005). Distinct and nonredundant in vivo functions of TNF produced by t cells and macrophages/neutrophils: protective and deleterious effects. Immunity 22, 93–104. doi: 10.1016/j.immuni.2004.11.016
Groneberg, D. A., Serowka, F., Peckenschneider, N., Artuc, M., Grützkau, A., Fischer, A., et al. (2005). Gene expression and regulation of nerve growth factor in atopic dermatitis mast cells and the human mast cell line-1. J. Neuroimmunol. 161, 87–92. doi: 10.1016/j.jneuroim.2004.12.019
Hassler, S. N., Ahmad, F. B., Burgos-Vega, C. C., Boitano, S., Vagner, J., Price, T. J., et al. (2019). Protease activated receptor 2 (PAR2) activation causes migraine-like pain behaviors in mice. Cephalalgia 39, 111–122. doi: 10.1177/0333102418779548
Hoffmeister, C., Trevisan, G., Rossato, M. F., de Oliveira, S. M., Gomez, M. V., and Ferreira, J. (2011). Role of TRPV1 in nociception and edema induced by monosodium urate crystals in rats. Pain 152, 1777–1788. doi: 10.1016/j.pain.2011.03.025
Huygen, F. J., Ramdhani, N., van Toorenenbergen, A., Klein, J., and Zijlstra, F. J. (2004). Mast cells are involved in inflammatory reactions during Complex Regional Pain Syndrome type 1. Immunol. Lett. 91, 147–154. doi: 10.1016/j.imlet.2003.11.013
Jaggi, A. S., Kaur, G., Bali, A., and Singh, N. (2017). Pharmacological investigations on mast cell stabilizer and histamine receptor antagonists in vincristine-induced neuropathic pain. Naunyn Schmiedebergs Arch. Pharmacol. 390, 1087–1096. doi: 10.1007/s00210-017-1426-8
Ji, R. R., Chamessian, A., and Zhang, Y. Q. (2016). Pain regulation by non-neuronal cells and inflammation. Science 354, 572–577. doi: 10.1126/science.aaf8924
Kimura, L. F., Santos-Neto, M., Barbaro, K. C., and Picolo, G. (2018). Potamotrygon motoro stingray venom induces both neurogenic and inflammatory pain behavior in rodents. Toxicon 150, 168–174. doi: 10.1016/j.toxicon.2018.05.018
Kissel, C. L., Kovács, K. J., and Larson, A. A. (2017). Evidence for the modulation of nociception in mice by central mast cells. Eur. J. Pain 21, 1743–1755. doi: 10.1002/ejp.1086
Klooker, T. K., Braak, B., Koopman, K. E., Welting, O., Wouters, M. M., van der Heide, S., et al. (2010). The mast cell stabiliser ketotifen decreases visceral hypersensitivity and improves intestinal symptoms in patients with irritable bowel syndrome. Gut 59, 1213–1221. doi: 10.1136/gut.2010.213108
Koroleva, K., Gafurov, O., Guselnikova, V., Nurkhametova, D., Giniatullina, R., Sitdikova, G., et al. (2019). Meningeal mast cells contribute to ATP-induced nociceptive firing in trigeminal nerve terminals: direct and indirect purinergic mechanisms triggering migraine pain. Front. Cell. Neurosci. 13:195. doi: 10.3389/fncel.2019.00195
Koyuncu Irmak, D., Kilinc, E., and Tore, F. (2019). Shared fate of meningeal mast cells and sensory neurons in migraine. Exp. Dermatol. 13:136. doi: 10.3389/fncel.2019.00136
Lam, D. K., and Schmidt, B. L. (2010). Serine proteases and protease-activated receptor 2-dependent allodynia: a novel cancer pain pathway. Pain 149, 263–272. doi: 10.1016/j.pain.2010.02.010
Lauria, P. S. S., Silva, E., Casais, L. L., do Espirito-Santo, R. F., de Souza, C. M. V., Zingali, R. B., et al. (2018). Pain-like behaviors and local mechanisms involved in the nociception experimentally induced by Latrodectus curacaviensis spider venom. Toxicol. Lett. 299, 67–75. doi: 10.1016/j.toxlet.2018.09.008
Le, D. D., Schmit, D., Heck, S., Omlor, A. J., Sester, M., Herr, C., et al. (2016). Increase of mast cell-nerve association and neuropeptide receptor expression on mast cells in perennial allergic rhinitis. Neuroimmunomodulation 23, 261–270. doi: 10.1159/000453068
Lee, H., Chung, H., Park, J. C., Shin, S. K., Lee, S. K., Lee, Y. C., et al. (2014). Heterogeneity of mucosal mast cell infiltration in subgroups of patients with esophageal chest pain. Neurogastroenterol. Motil. 26, 786–793. doi: 10.1111/nmo.12325
Leon, A., Buriani, A., Dal Toso, R., Fabris, M., Romanello, S., Aloe, L., et al. (1994). Mast cells synthesize, store and release nerve growth factor. Proc. Natl. Acad. Sci. U S A 91, 3739–3743. doi: 10.1073/pnas.91.9.3739
Levy, D., Burstein, R., Kainz, V., Jakubowski, M., and Strassman, A. M. (2007). Mast cell degranulation activates a pain pathway underlying migraine headache. Pain 130, 166–176. doi: 10.1016/j.pain.2007.03.012
Li, W. T., Luo, Q. Q., Wang, B., Chen, X., Yan, X. J., Qiu, H. Y., et al. (2019). Bile acids induce visceral hypersensitivity via mucosal mast cell-to-nociceptor signaling that involves the farnesoid X receptor/nerve growth factor/transient receptor potential vanilloid 1 axis. FASEB J. 33, 2435–2450. doi: 10.1096/fj.201800935RR
Li, W. W., Guo, T. Z., Liang, D. Y., Sun, Y., Kingery, W. S., Clark, J. D., et al. (2012). Substance P signaling controls mast cell activation, degranulation and nociceptive sensitization in a rat fracture model of complex regional pain syndrome. Anesthesiology 116, 882–895. doi: 10.1097/ALN.0b013e31824bb303
Liu, J. A., Yu, J., and Cheung, C. W. (2021). Immune actions on the peripheral nervous system in pain. Int. J. Mol. Sci. 22:1448. doi: 10.3390/ijms22031448
Loewendorf, A. I., Matynia, A., Saribekyan, H., Gross, N., Csete, M., Harrington, M., et al. (2016). Roads less traveled: sexual dimorphism and mast cell contributions to migraine pathology. Front. Immunol. 7:140. doi: 10.3389/fimmu.2016.00140
Lopes, D. M., Denk, F., Chisholm, K. I., Suddason, T., Durrieux, C., Thakur, M., et al. (2017). Peripheral inflammatory pain sensitisation is independent of mast cell activation in male mice. Pain 158, 1314–1322. doi: 10.1097/j.pain.0000000000000917
Magnusdottir, E. I., Grujic, M., Roers, A., Hartmann, K., Pejler, G., Lagerstrom, M. C., et al. (2018). Mouse mast cells and mast cell proteases do not play a significant role in acute tissue injury pain induced by formalin. Mol. Pain 14:1744806918808161. doi: 10.1177/1744806918808161
Marshall, J. S., Stead, R. H., McSharry, C., Nielsen, L., and Bienenstock, J. (1990). The role of mast cell degranulation products in mast cell hyperplasia. I. Mechanism of action of nerve growth factor. J. Immunol. 144, 1886–1892.
Martin Jensen, M., Jia, W., Schults, A. J., Ye, X., Prestwich, G. D., Oottamasathien, S., et al. (2018). IL-33 mast cell axis is central in LL-37 induced bladder inflammation and pain in a murine interstitial cystitis model. Cytokine 110, 420–427. doi: 10.1016/j.cyto.2018.05.012
Massaad, C. A., Safieh-Garabedian, B., Poole, S., Atweh, S. F., Jabbur, S. J., Saadé, N. E., et al. (2004). Involvement of substance P, CGRP and histamine in the hyperalgesia and cytokine upregulation induced by intraplantar injection of capsaicin in rats. J. Neuroimmunol. 153, 171–182. doi: 10.1016/j.jneuroim.2004.05.007
McDougall, J. J., and Larson, S. E. (2006). Nociceptin/orphanin FQ evokes knee joint pain in rats via a mast cell independent mechanism. Neurosci. Lett. 398, 135–138. doi: 10.1016/j.neulet.2005.12.066
Metcalfe, D. D., Baram, D., and Mekori, Y. A. (1997). Mast cells. Physiol. Rev. 77, 1033–1079. doi: 10.1152/physrev.1997.77.4.1033
Mikhailov, N., Leskinen, J., Fagerlund, I., Poguzhelskaya, E., Giniatullina, R., Gafurov, O., et al. (2019). Mechanosensitive meningeal nociception via Piezo channels: implications for pulsatile pain in migraine. Neuropharmacology 149, 113–123. doi: 10.1016/j.neuropharm.2019.02.015
Molderings, G. J., Haenisch, B., Brettner, S., Homann, J., Menzen, M., Dumoulin, F. L., et al. (2016). Pharmacological treatment options for mast cell activation disease. Naunyn Schmiedebergs Arch. Pharmacol. 389, 671–694. doi: 10.1007/s00210-016-1247-1
Morellini, N., Finch, P. M., Goebel, A., and Drummond, P. D. (2018). Dermal nerve fibre and mast cell density and proximity of mast cells to nerve fibres in the skin of patients with complex regional pain syndrome. Pain 159, 2021–2029. doi: 10.1097/j.pain.0000000000001304
Mukai, K., Tsai, M., and Saito, H. (2018). Mast cells as sources of cytokines, chemokines and growth factors. Immunol. Rev. 282, 121–150. doi: 10.1111/imr.12634
Nakajima, K., Obata, H., Ito, N., Goto, F., and Saito, S. (2009). The nociceptive mechanism of 5-hydroxytryptamine released into the peripheral tissue in acute inflammatory pain in rats. Eur. J. Pain 13, 441–447. doi: 10.1016/j.ejpain.2008.06.007
Obara, I., Telezhkin, V., Alrashdi, I., and Chazot, P. L. (2020). Histamine, histamine receptors and neuropathic pain relief. Br. J. Pharmacol. 177, 580–599. doi: 10.1111/bph.14696
Ocak, U., Ocak, P. E., Wang, A., Zhang, J. H., Boling, W., Wu, P., et al. (2019). Targeting mast cell as a neuroprotective strategy. Brain Inj. 33, 723–733. doi: 10.1080/02699052.2018.1556807
Oliveira, S. M., Drewes, C. C., Silva, C. R., Trevisan, G., Boschen, S. L., Moreira, C. G., et al. (2011). Involvement of mast cells in a mouse model of postoperative pain. Eur. J. Pharmacol. 672, 88–95. doi: 10.1016/j.ejphar.2011.10.001
Oliveira, S. M., Silva, C. R., and Ferreira, J. (2013). Critical role of protease-activated receptor 2 activation by mast cell tryptase in the development of postoperative pain. Anesthesiology 118, 679–690. doi: 10.1097/ALN.0b013e31827d415f
Patel, R. H., and Mohiuddin, S. S. (2020). “Biochemistry histamine,” in StatPearls [Internet], (Treasure Island, FL: StatPearls Publishing). Available online at: https://www.ncbi.nlm.nih.gov/books/NBK557790/.
Pepper, A., Li, W., Kingery, W. S., Angst, M. S., Curtin, C. M., and Clark, J. D. (2013). Changes resembling complex regional pain syndrome following surgery and immobilization. J. Pain 14, 516–524. doi: 10.1016/j.jpain.2013.01.004
Pezet, S., and McMahon, S. B. (2006). Neurotrophins: mediators and modulators of pain. Annu. Rev. Neurosci. 29, 507–538. doi: 10.1146/annurev.neuro.29.051605.112929
Roman, K., Done, J. D., Schaeffer, A. J., Murphy, S. F., and Thumbikat, P. (2014). Tryptase-PAR2 axis in experimental autoimmune prostatitis, a model for chronic pelvic pain syndrome. Pain 155, 1328–1338. doi: 10.1016/j.pain.2014.04.009
Rudick, C. N., Bryce, P. J., Guichelaar, L. A., Berry, R. E., and Klumpp, D. J. (2008). Mast cell-derived histamine mediates cystitis pain. PLoS One 3:e2096. doi: 10.1371/journal.pone.0002096
Schneider, E. H. (2017). New perspectives for a well-known biogenic amine: mast cell-derived histamine as pathophysiological agent in vincristine-induced neuropathic pain. Naunyn Schmiedebergs Arch. Pharmacol. 390, 1085–1086. doi: 10.1007/s00210-017-1427-7
Serhan, N., and Basso, L. (2019). House dust mites activate nociceptor-mast cell clusters to drive type 2 skin inflammation. Nat. Immunol. 20, 1435–1443. doi: 10.1038/s41590-019-0493-z
Skaper, S. D. (2017). Nerve growth factor: a neuroimmune crosstalk mediator for all seasons. Immunology 151, 1–15. doi: 10.1111/imm.12717
Solís-López, A., Kriebs, U., Marx, A., Mannebach, S., Liedtke, W. B., Caterina, M. J., et al. (2017). Analysis of TRPV channel activation by stimulation of FCεRI and MRGPR receptors in mouse peritoneal mast cells. PLoS One 12:e0171366. doi: 10.1371/journal.pone.0171366
Sommer, C. (2004). Serotonin in pain and analgesia: actions in the periphery. Mol. Neurobiol. 30, 117–125. doi: 10.1385/MN:30:2:117
Sousa-Valente, J., and Brain, S. D. (2018). A historical perspective on the role of sensory nerves in neurogenic inflammation. Semin. Immunopathol. 40, 229–236. doi: 10.1007/s00281-018-0673-1
Sousa-Valente, J., Calvo, L., Vacca, V., Simeoli, R., Arévalo, J. C., Malcangio, M., et al. (2018). Role of TrkA signalling and mast cells in the initiation of osteoarthritis pain in the monoiodoacetate model. Osteoarthritis Cartilage 26, 84–94. doi: 10.1016/j.joca.2017.08.006
Sun, H., Ma, Y., An, S., and Wang, Z. (2020). Altered gene expression signatures by calcitonin gene-related peptide promoted mast cell activity in the colon of stress-induced visceral hyperalgesia mice. Neurogastroenterol. Motil. 31:e14073. doi: 10.1111/nmo.14073
Suzuki, A., Suzuki, R., Furuno, T., Teshima, R., and Nakanishi, M. (2004). N-cadherin plays a role in the synapse-like structures between mast cells and neurites. Biol. Pharm. Bull. 27, 1891–1894. doi: 10.1248/bpb.27.1891
Symons, F. J., Wendelschafer-Crabb, G., Kennedy, W., Heeth, W., and Bodfish, J. W. (2009). Degranulated mast cells in the skin of adults with self-injurious behavior and neurodevelopmental disorders. Brain Behav. Immun. 23, 365–370. doi: 10.1016/j.bbi.2008.11.003
Tam, S. Y., Tsai, M., Yamaguchi, M., Yano, K., Butterfield, J. H., Galli, S. J., et al. (1997). Expression of functional TrkA receptor tyrosine kinase in the HMC-1 human mast cell line and in human mast cells. Blood 90, 1807–1820. doi: 10.1182/blood.v90.5.1807
Taracanova, A., Tsilioni, I., Conti, P., Norwitz, E. R., and Leeman, S. E. (2018). Substance P and IL-33 administered together stimulate a marked secretion of IL-1β from human mast cells, inhibited by methoxyluteolin. Proc. Natl. Acad. Sci. U S A 115, E9381–e9390. doi: 10.1073/pnas.1810133115
Taylor, T. J., Youssef, N. N., Shankar, R., Kleiner, D. E., and Henderson, W. A. (2010). The association of mast cells and serotonin in children with chronic abdominal pain of unknown etiology. BMC Res. Notes 3:265. doi: 10.1186/1756-0500-3-265
Theoharides, T. C., Tsilioni, I., and Bawazeer, M. (2019). Mast Cells, Neuroinflammation and Pain in Fibromyalgia Syndrome. Front. Cell. Neurosci. 13:353. doi: 10.3389/fncel.2019.00353
Totsch, S. K., and Sorge, R. E. (2017). Immune system involvement in specific pain conditions. Mol. Pain 13:1744806917724559. doi: 10.1177/1744806917724559
Tsuda, M. (2018). Microglia in the CNS and Neuropathic Pain. Adv. Exp. Med. Biol. 1099, 77–91. doi: 10.1007/978-981-13-1756-9_7
Vang, D., Paul, J. A., Nguyen, J., Tran, H., Vincent, L., Yasuda, D., et al. (2015). Small-molecule nociceptin receptor agonist ameliorates mast cell activation and pain in sickle mice. Haematologica 100, 1517–1525. doi: 10.3324/haematol.2015.128736
Vincent, L., Vang, D., Nguyen, J., Benson, B., Lei, J., and Gupta, K. (2016). Cannabinoid receptor-specific mechanisms to alleviate pain in sickle cell anemia via inhibition of mast cell activation and neurogenic inflammation. Haematologica 101, 566–577. doi: 10.3324/haematol.2015.136523
Vincent, L., Vang, D., Nguyen, J., Gupta, M., Luk, K., Ericson, M. E., et al. (2013). Mast cell activation contributes to sickle cell pathobiology and pain in mice. Blood 122, 1853–1862. doi: 10.1182/blood-2013-04-498105
Vukman, K. V., Försönits, A., Oszvald, Á., Tóth, E. Á., and Buzás, E. I. (2017). Mast cell secretome: soluble and vesicular components. Semin. Cell. Dev. Biol. 67, 65–73. doi: 10.1016/j.semcdb.2017.02.002
Wang, X., Liu, W., O’Donnell, M., Lutgendorf, S., Bradley, C., Schrepf, A., et al. (2016). Evidence for the role of mast cells in cystitis-associated lower urinary tract dysfunction: a multidisciplinary approach to the study of chronic pelvic pain research network animal model study. PLoS One 11:e0168772. doi: 10.1371/journal.pone.0168772
Wei, H., Wei, Y., Tian, F., Niu, T., and Yi, G. (2016). Blocking proteinase-activated receptor 2 alleviated neuropathic pain evoked by spinal cord injury. Physiol. Res. 65, 145–153. doi: 10.33549/physiolres.933104
Wheeler, M. A., Heffner, D.L., Kim, S., Espy, S. M., Spano, A. J., Cleland, C. L., et al. (2014). TNF-α/TNFR1 signaling is required for the development and function of primary nociceptors. Neuron 82, 587–602. doi: 10.1016/j.neuron.2014.04.009
Wirz, S., and Molderings, G. J. (2017). A practical guide for treatment of pain in patients with systemic mast cell activation disease. Pain Physician 20, E849–E861. doi: 10.36076/ppj.20.5.e849
Xanthos, D. N., Gaderer, S., Drdla, R., Nuro, E., Abramova, A., Ellmeier, W., et al. (2011). Central nervous system mast cells in peripheral inflammatory nociception. Mol. Pain 7:42. doi: 10.1186/1744-8069-7-42
Yakubova, A., and Davidyuk, Y. (2021). Searching for predictors of migraine chronification: a pilot study of 1911A>G polymorphism of TRPV1 gene in episodic versus chronic migraine. J. Mol. Neurosci. 71, 618–624. doi: 10.1007/s12031-020-01683-9
Yan, X. J., Feng, C. C., Liu, Q., Zhang, L. Y., Dong, X., Liu, Z. L., et al. (2014). Vagal afferents mediate antinociception of estrogen in a rat model of visceral pain: the involvement of intestinal mucosal mast cells and 5-hydroxytryptamine 3 signaling. J. Pain 15, 204–217. doi: 10.1016/j.jpain.2013.10.012
Yang, S., Wang, J., Brand, D. D., and Zheng, S. G. (2018). Role of TNF-TNF receptor 2 signal in regulatory T cells and its therapeutic implications. Front. Immunol. 9:784. doi: 10.3389/fimmu.2018.00784
Yu, D., Zhu, J., Zhu, M., Wei, K., Chen, Q., Wu, X., et al. (2019). Inhibition of mast cell degranulation relieves visceral hypersensitivity induced by pancreatic carcinoma in mice. J. Mol. Neurosci. 69, 235–245. doi: 10.1007/s12031-019-01352-6
Zahari, W., Hashim, S. N., Yusof, M. F., Osman, Z. F., Kannan, T. P., Mokhtar, K. I., et al. (2017). Immunomodulatory effect of cytokines in the differentiation of mesenchymal stem cells: a review. Curr. Stem Cell. Res. Ther. 12, 197–206. doi: 10.2174/1574888X11666160614103404
Zhang, B., Weng, Z., Sismanopoulos, N., Asadi, S., Therianou, A., Alysandratos, K. D., et al. (2012). Mitochondria distinguish granule-stored from de novo synthesized tumor necrosis factor secretion in human mast cells. Int. Arch. Allergy Immunol. 159, 23–32. doi: 10.1159/000335178
Zhang, D., Spielmann, A., Wang, L., Ding, G., Huang, F., Gu, Q., et al. (2012). Mast-cell degranulation induced by physical stimuli involves the activation of transient-receptor-potential channel TRPV2. Physiol. Res. 61, 113–124. doi: 10.33549/physiolres.932053
Zhang, L., Song, J., Bai, T., Wang, R., and Hou, X. (2018). Sustained pain hypersensitivity in the stressed colon: Role of mast cell-derived nerve growth factor-mediated enteric synaptic plasticity. Neurogastroenterol. Motil. 30:e13430. doi: 10.1111/nmo.13430
Zhang, S., Zeng, X., Yang, H., Hu, G., and He, S. (2012). Mast cell tryptase induces microglia activation via protease-activated receptor 2 signaling. Cell. Physiol. Biochem. 29, 931–940. doi: 10.1159/000171029
Zhang, X. C., Kainz, V., Burstein, R., and Levy, D. (2011). Tumor necrosis factor-α induces sensitization of meningeal nociceptors mediated via local COX and p38 MAP kinase actions. Pain 152, 140–149. doi: 10.1016/j.pain.2010.10.002
Keywords: mast cells, pain, hyperalgesia, inflammation, immunoregulation
Citation: Mai L, Liu Q, Huang F, He H and Fan W (2021) Involvement of Mast Cells in the Pathophysiology of Pain. Front. Cell. Neurosci. 15:665066. doi: 10.3389/fncel.2021.665066
Received: 07 February 2021; Accepted: 07 May 2021;
Published: 10 June 2021.
Edited by:
Giuseppina D’Alessandro, Sapienza University of Rome, ItalyReviewed by:
Albert Rizvanov, Kazan Federal University, RussiaRossella Paolini, Sapienza University of Rome, Italy
Copyright © 2021 Mai, Liu, Huang, He and Fan. This is an open-access article distributed under the terms of the Creative Commons Attribution License (CC BY). The use, distribution or reproduction in other forums is permitted, provided the original author(s) and the copyright owner(s) are credited and that the original publication in this journal is cited, in accordance with accepted academic practice. No use, distribution or reproduction is permitted which does not comply with these terms.
*Correspondence: Wenguo Fan, fanweng@mail.sysu.edu.cn