- 1Key Laboratory of Medical Electrophysiology of Ministry of Education and Medical Electrophysiological Key Laboratory of Sichuan Province, Institute of Cardiovascular Research, Southwest Medical University, Luzhou, China
- 2Department of Histology and Embryology, School of Basic Medical Sciences, Southwest Medical University, Luzhou, China
- 3Department of Neurosurgery, Affiliated Hospital of Southwest Medical University, Luzhou, China
- 4Neurosurgical Clinical Research Center of Sichuan Province, Luzhou, China
A presynaptic protein closely related to Parkinson's disease (PD), α-synuclein (α-Syn), has been studied extensively regarding its pathogenic mechanisms. As a physiological protein in presynapses, however, α-Syn's physiological function remains unclear. Its location in nerve terminals and effects on membrane fusion also imply its functional role in synaptic transmission, including its possible interaction with high-curvature membranes via its N-terminus and amorphous C-terminus. PD-related mutants that disrupt the membrane interaction (e.g., A30P and G51D) additionally suggest a relationship between α-Syn's pathogenic mechanisms and physiological roles through the membrane binding. Here, we summarize recent research on how α-Syn and its variants interact with membranes and influence synaptic transmission. We list several membrane-related connections between the protein's physiological function and the pathological mechanisms that stand to expand current understandings of α-Syn.
Introduction
The protein α-Synuclein is highly soluble and plays a central role in the pathogenesis in Parkinson's disease and other synucleinopathies. With only 140 amino acids, α-Synuclein (α-Syn) is a small peripheral membrane protein that localizes specifically to the axon terminal in neurons (Maroteaux et al., 1988; George et al., 1995; Iwai et al., 1995; Bendor et al., 2013). In α-Syn's unusual, unique structure (Figure 1), its highly conserved N-terminus contains seven 11-mer repeats (residues 1–95) with the KTKEGV consensus sequence, similar to apolipoprotein, that forms three turns of an amphipathic α-helix and mediates α-protrusion associated with membranes of synuclein and lipids (Davidson et al., 1998; Eliezer et al., 2001; Bussell and Eliezer, 2003; Chandra et al., 2003; Bussell et al., 2005). Strangely, all identified mutations related to synucleinopathies (e.g., A30P, E46K, H50Q, G51D, A53E, and A53T) are located in the N-terminal domain (Polymeropoulos et al., 1997; Krüger et al., 1998; Zarranz et al., 2004; Appel-Cresswell et al., 2013; Lesage et al., 2013; Proukakis et al., 2013; Pasanen et al., 2014). Beyond that, five of them are clustered in eight residues, which suggests the potential for a pathology of lipid binding or even a lack of lipid binding to α-Syn. Aside from that domain, the NAC domain (i.e., residues 60–95) is responsible for α-Syn's aggregation (Uéda et al., 1993) and aids the detection of lipid properties (Fusco et al., 2014). Meanwhile, the C-terminus (i.e., residues 96–140), a highly acidic and largely unstructured domain (Davidson et al., 1998; Bussell and Eliezer, 2003; Ulmer et al., 2005), is the target of various post-translational modifications (Oueslati et al., 2010). It is also considered to bind to proteins, ions, polycations, and polyamines (Paik et al., 1999; Nielsen et al., 2001; Fernández et al., 2004; Brown, 2007), as well as to modulate α-Syn's binding to membranes (Sevcsik et al., 2011) and protect it from aggregation (Crowther et al., 1998; Park et al., 2002; Hoyer et al., 2004).
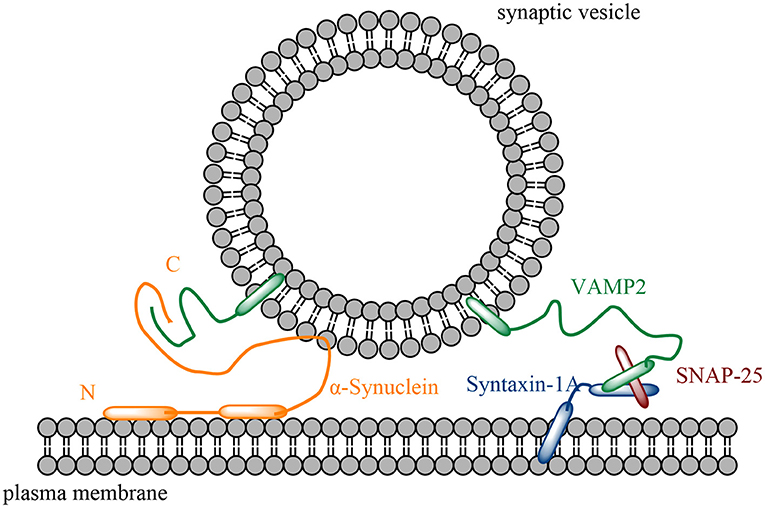
Figure 1. Schematic diagram of α-Syn in SNARE (a complex composed of syntaxin-1A, VAMP2 and SNAP-25)-mediated membrane interaction. α-Syn's N-terminus forms two helices to interact with the plasma membrane, while α-Syn interacts with VAMP2 at the C-terminus.
Located in presynapses, α-Syn could interact with synaptic vesicles and act as a molecular chaperone of soluble N-ethylmaleimide-sensitive factor attachment protein receptor (SNARE) complexes (George et al., 1995). Although such evidence indicates α-Syn's role in neurotransmitter release and synaptic plasticity, its precise function remains unclear. α-Syn is also absent in worms, fruit flies, and yeasts, which suggests that it is not generally required for synaptic transmission or membrane trafficking (Abeliovich et al., 2000; Chandra et al., 2004; Burre et al., 2010; Jensen et al., 2011). Therefore, given existing research, we have summarized the current knowledge about the interaction of α-Syn with membranes, how variants of α-Syn affect the interaction with membranes, and how α-Syn affects synaptic transmission. We also discuss the possible mechanism of the transition between physiological function and pathological mechanism.
Physiological Mechanism of Membrane Fusion
As a basic life process, membrane fusion involves subcellular compartmentation, cell growth, hormone secretion, and neurotransmission (Wickner and Schekman, 2008). Membrane fusion begins with the gradual fusion of the outer and inner leaflets between two membranes, which causes lipids and other contents (e.g., proteins, glycoprotein, and glycolipid) to mix. From there, it consists of multiple distinct stages, including tethering, docking and priming, hemifusion, and full fusion (Tian et al., 2019).
Critical evidence concerning proteins active in membrane fusion has accumulated in the literature to date. Notably, SNAREs are considered to represent core fusion elements that assembled in a four-helix bundle structure, releasing energy such that the membranes enter into close proximity and eventually undergo membrane fusion (Sutton et al., 1998). Proteins such as α-Syn and cysteine string protein α (CSPα) affect SNARE complexes, while the interaction between lipid molecules and proteins plays a key role in regulating membrane fusion (Brunger et al., 2015; Wang et al., 2016b; Bao et al., 2018; Das et al., 2020).
α-Syn's Interaction With Membranes
In view of its enriched expression in neuronal cells, many endogenous roles for α-Syn have been proposed, including ones that involve controlling synaptic vesicle release (Snead and Eliezer, 2014), modulating secretory pathways (Wang and Hay, 2015), and even regulating vesicle transport (Jensen et al., 1998). Another well-described biochemical property of α-Syn is membrane binding associated with structural switching.
α-Syn's Function in Synaptic Transmission
In vitro research has shown that α-Syn inhibits membrane fusion by stabilizing the lipid packing of stressed bilayers independently of other protein factors that may be involved in the fusion machinery of membranes (Kamp et al., 2010). At the same time, α-Syn was found to significantly promote the clustering of protein-reconstituted liposomes that mimic synaptic vesicles, albeit with little effect on Ca2+-triggered fusion in a single vesicle-vesicle system with reconstituted neuronal SNAREs, synaptotagmin-1, and complexin-1 (Diao et al., 2013). It was also found to participate in vesicle aggregation, initiation, fusion (i.e., assembly of SNARE complex), and recycling (i.e., disassembly of SNARE complex), all of which relate to membrane fusion (Lashuel et al., 2013).
Studies have shown that α-Syn functions within various aspects of synaptic transmission. For one, it promotes the assembly of SNARE complexes by directly interacting with N-terminus of VAMP2 through the highly flexible, negatively charged C-terminal region (Burre et al., 2010). For another, via its N-terminal region, α-Syn's membrane anchoring is also essential to the process (Wang et al., 2016a). α-Syn may also cooperate with CSPα to maintain SNARE proteins assembly and neurotransmission (Hou et al., 2017). Additional evidence suggests that native α-Syn does not compromise the efficiency of synaptic vesicle exocytosis but does help to increase the availability of synthetic vesicles at the synapse (Diao et al., 2013). Recently, studies show both VAMP2 and synapsin cooperate to promote clustering of SVs and regulating SV recycling with different mechanisms (Atias et al., 2019; Sun et al., 2019). However, other research has indicated that α-Syn knockout exerts little effect on synaptic transmission (Nemani et al., 2010) and that α-Syn's overexpression reduces the release of neurotransmitters by disrupting vesicle docking in exocytosis (Larsen et al., 2006). Further still, because αβγ-synuclein triple-knockout mice lacking synucleins developed age-dependent neurological impairments, exhibited decreased SNARE-complex assembly, and died prematurely, synucleins may also sustain normal SNARE complex assembly in presynaptic terminals during aging (Burre et al., 2010).
Other research has revealed that the promotive effect of α-Syn on SNARE-dependent bilayer merging mainly occurs via the enhancement of vesicle docking (Hawk et al., 2019). Recent work also revealed no significant changes observed in merging efficiency, the ratio of instant-to-delayed merger events, or the kinetics of bilayer merging other than the frequency of vesicle docking, especially since α-Syn stimulates vesicle docking without altering the dynamics of bilayer mergers in lipid mixing (Hawk et al., 2019). To that, researchers have contributed the hypothesis that α-Syn binds to vesicle-associated membrane protein 2 (VAMP2) by using its unstructured C-terminus, while simultaneously interacting with the target plasma membrane via its amphipathic N-terminal region, thereby aiding the recruitment of synaptic vesicles to the plasma membrane. The α-Syn's C-terminus is critical to promoting vesicle docking, because after truncating the C-terminus, the docking process of vesicles was inhibited, and overexpression had destroyed the accumulation of vesicles within the synapse (Lou et al., 2017). On the contrary, it had been found that the inhibition of docking by α-Syn is coupled with the α-Syn's membrane binding but not with the interaction with VAMP2, although the fusion inhibition by α-Syn oligomers at much lower concentrations requires α-Syn's interaction with VAMP2 (Lai et al., 2014). Once aggregated, the multivalent oligomeric species containing multiple binding sites can bind to VAMP2 on the vesicle, which renders VAMP2 unable to interact with t-SNARE (i.e., syntaxin-1A and SNAP-25) on the plasma membrane (Choi et al., 2013) and thus severely reduces the possibility of bilayer merging. In addition, some convincing evidence suggests that α-Syn promotes the filling of vesicles by directly interacting with and modulating vesicular monoamine transporter 2 and the reuptake of dopamine via the dopamine transporter (Lee et al., 2001; Wersinger and Sidhu, 2003; Guo et al., 2008; Swant et al., 2011; Butler et al., 2015). Latest studies also suggest that α-Syn can promote endocytosis by increasing phosphatidylinositol 4,5-bisphosphate level (Schechter et al., 2020). Those findings may stimulate new ideas for further examination of the process of synaptic transmission.
Fusion Pore Regulation
In general, fusion begins with the formation of a narrow pore called a fusion pore that allows water, solute, and membrane to move between compartments (Brose et al., 2019). A fusion pore may grow, contract, or close, all under the influence of both mechanical forces and biological cues (Brose et al., 2019). In that context, α-Syn's aggregation has been shown to cause the formation of oligomeric intermediates that interact with membranes to form fusion pores, while α-Syn itself seems to partly accelerate the opening of the pores (Logan et al., 2017). During exocytosis, synaptic vesicles also form fusion pores that dilate before fully collapsing into the plasma membrane. However, because fusion pores can also reclose during kiss-and-run events in which vesicles are immediately regenerated (Alabi and Tsien, 2013), the regulation of the membrane curvature may affect the behavior of fusion pores. If so, then because α-Syn can bind to anionic membranes with high curvatures (Davidson et al., 1998; Jensen et al., 2011; Pranke et al., 2011), overexpression of α-Syn has been shown to influence the behavior of exocytotic fusion pores. The dilation of fusion pores would thus be expected to limit the release of neuromodulators that dissociate slowly from luminal matrices (e.g., monoamines and peptides), but without affecting classical transmitters such as glutamate that can rapidly escape, even through small pores. However, α-Syn has increased the number of SNARE complexes (Burre et al., 2010), which may also heighten the force that drives the dilation of fusion pores and thus promotes cargo release (Shi et al., 2012). In view of those dynamics, it is difficult to reconcile the observed inhibition of cargo release and the notion that α-Syn chaperones SNARE complexes. Altogether, α-Syn appears to play a dual role. On the one hand, it inhibits membrane fusion in vivo and in vitro by directly acting on lipid bilayers. On the other hand, it may promote the accumulation of SNARE complexes by inhibiting exocytosis, thereby preventing the disassembly of complexes present on vesicles primed for fusion (Kamp et al., 2010, Nuscher et al., 2004; Braun and Sachs, 2015).
Modulation With Ca2+
The primary location of α-Syn is at the presynaptic terminal, where calcium fluctuations can occur in concentrations in the hundreds of μM. It is well established that calcium ions play an important role in triggering synaptic transmission, and studies have shown that calcium binds to α-Syn at its C-terminus, where Ca2+ can regulate the role of α-Syn and the plasma membrane. In vitro experiments have confirmed that different concentrations of calcium ions exert different effects on proteins in plasma membranes (e.g., presynaptic membrane), and it is well known that cytosolic Ca2+ regulates vesicle docking, priming, fusion, and the expansion of fusion pores (Man et al., 2015). Still other work has shown that α-Syn interacts with plasma membranes in a specific structure and affects calcium signal transduction, while β-sheet-rich-poly α-Syn can cause Ca2+ deregulation and Ca2+-dependent cell death (Angelova et al., 2016). Added to that, a recent study has demonstrated α-Syn's novel effects on mobilizing the release of Ca2+ from thapsigargin-sensitive Ca2+ pools to enhance the ATP-induced increase of Ca2+ concentration, which enhances vesicle fusion. At the same time, soluble α-Syn elevates the same release of Ca2+ from thapsigargin-sensitive Ca2+ pools to enhance ATP-induced fusion, which reveals α-Syn's novel role in coupling vesicles to specific Ca2+ microdomains. By contrast, aggregated α-Syn, in a Ca2+-independent pathway, inhibits vesicle priming but does not affect the dilation of fusion pores (Huang et al., 2018).
Influence of α-Syn's Different Truncated Ends on Membrane Interaction
α-Syn has an N-terminal membrane-binding region that binds to phospholipid bilayers and a C-terminal region that interacts with VAMP2 (Lou et al., 2017). Via its N-terminus and amorphous C-terminus, α-Syn can also interact with high-curvature membranes. At both ends, however, truncation affects α-Syn's interaction with membranes to varying degrees, hence the interest among researchers in analyzing how α-Syn's different truncated ends affect membrane interaction.
Several studies have furnished support for an emerging view that α-Syn's N-terminal region plays an anchoring role in membrane interaction, namely by modulating α-Syn's physiological as well as pathological role (Diao et al., 2013; Fusco et al., 2016; O'Leary and Lee, 2019). Since then, additional research has indicated that approximately 14 N-terminal residues enter anionic membranes at a skewed angle of insertion, which relates to the helical region's folding onto the membrane's surface, thereby synergistically establishing the joint α-Syn–lipid structures (Cholak et al., 2020). Meanwhile, the deletion of residues 2–14 reduces α-Syn's membrane localization in mammalian cells, which indicates that the N-terminal anchor exerts an impact in vivo. All of that evidence shows that avidity within the N-terminal anchor couples N-terminal insertion and helical surface binding, both of which are crucial for α-Syn's interaction with membranes and cellular localization and may even affect membrane fusion (Cholak et al., 2020). Fusion has also proven to fail with a truncated α-Syn due to its lacking the charged C-terminal domain (Kamp et al., 2010). Last, genomic editing to disrupt α-Syn's N-terminal domain, which is important for membrane association, induced mitochondrial elongation without changes in fusion-fission protein levels, thereby suggesting that αSyn plays a direct physiological role in maintaining the size of mitochondria (Pozo Devoto et al., 2017).
Influence of Mutants on Membrane Interaction
Studies have additionally revealed that α-Syn factors into neurodegenerative disorders (Logan et al., 2017) and that various secondary structures of α-Syn are involved in physiological and pathological processes (El-Agnaf et al., 1998; Conway et al., 2000; Lashuel et al., 2002; Uversky, 2007; Burré et al., 2014, 2015; Wang et al., 2014). For those reasons, knowing the effect of Parkinson's disease-related mutants that disrupt membrane interaction is important to understanding the possible relationship between pathogenic mechanisms and physiological roles at play in membrane binding. Research has shown that the A53T mutation had the highest membrane affinity with wild-type α-Syn, even compared to A30P (Perlmutter et al., 2009). Meanwhile, the increased affinity of the E46K mutant for vesicles containing negatively charged lipids has also been observed to induce an additional hydrogen bond between the protein and either the detergent or the lipid. Even so, the literature on those topics remains slim, and other possible mechanisms of the effects of PD-related mutants indeed warrant further investigation.
Discussion
As a type of membrane-based interaction, membrane fusion is vital to the release of neurotransmitters and plays a chief role in transmitter mechanisms. As a protein that can regulate membrane fusion, α-Syn is essential in the normal function of synapses, especially in synaptic transmission. Since changes in α-Syn can affect its function of regulating membrane fusion and thus affect synaptic transmission—and its changes may also contribute to some neurodegenerative diseases—a more profound understanding of α-Syn is critically needed.
Physiologically, as a chaperone, α-Syn participates in the assembly of SNARE complexes and may perform other functions as well. Pathologically, by contrast, α-Syn misfolds into neurotoxic aggregates that mediate neurodegeneration and propagate between neurons (Burré et al., 2014). Synuclein also forms a perplexing web of interactions with lipids, trafficking machinery, and other regulatory factors (Wang and Hay, 2015). Moreover, the protein's effects on endocytosis, exocytosis, and vesicle circulation are closely related to synaptic transmission. In the central nervous system, the release of calcium-dependent neurotransmitters regulates the roles of α-Syn and membrane, which affect not only the interaction between α-Syn and synaptic vesicles, but also neurotransmission, a qualitative release process. Therefore, the aggregation of α-Syn may relate to α-Syn's toxic effect, which stands to inspire new ideas for drug targets able to prevent that effect. On top of that, other experiments could study the effect of PD-related mutants on fusion to provide insights into the treatment of neurodegenerative diseases more generally.
Author Contributions
WD, CL, and YZ conceived the perspective of the work. CL and YZ drafted the manuscript. CL designed the figure. All authors revised and approved the final version of the manuscript.
Funding
This work was supported by National Natural Science Foundation of China (31871031), Ministry of Science and Technology of the People's Republic of China (2019YFE0120600), Department of Science and Technology of Sichuan Province (2019YJ0481).
Conflict of Interest
The authors declare that the research was conducted in the absence of any commercial or financial relationships that could be construed as a potential conflict of interest.
References
Abeliovich, A., Schmitz, Y., Fariñas, I., Choi-Lundberg, D., Ho, W. H., Castillo, P. E., et al. (2000). Mice lacking alpha-synuclein display functional deficits in the nigrostriatal dopamine system. Neuron 25, 239–252. doi: 10.1016/S0896-6273(00)80886-7
Alabi, A. A., and Tsien, R. W. (2013). Perspectives on kiss-and-run: role in exocytosis, endocytosis, and neurotransmission. Annu. Rev. Physiol. 75, 393–422. doi: 10.1146/annurev-physiol-020911-153305
Angelova, P. R., Ludtmann, M. H., Horrocks, M. H., Negoda, A., Cremades, N., Klenerman, D., et al. (2016). Ca2+ is a key factor in α-synuclein-induced neurotoxicity. J. Cell Sci. 129, 1792–1801. doi: 10.1242/jcs.180737
Appel-Cresswell, S., Vilarino-Guell, C., Encarnacion, M., Sherman, H., Yu, I., Shah, B., et al. (2013). Alpha-synuclein p.H50Q, a novel pathogenic mutation for Parkinson's disease. Mov. Disord. 28, 811–813. doi: 10.1002/mds.25421
Atias, M., Tevet, Y., Sun, J., Stavsky, A., Tal, S., Kahn, J., et al. (2019). Synapsins regulate alpha-synuclein functions. Proc. Natl. Acad. Sci. U.S.A. 116, 11116–11118. doi: 10.1073/pnas.1903054116
Bao, H., Das, D., Courtney, N. A., Jiang, Y., Briguglio, J. S., Lou, X., et al. (2018). Dynamics and number of trans-SNARE complexes determine nascent fusion pore properties. Nature 554, 260–263. doi: 10.1038/nature25481
Bendor, J. T., Logan, T. P., and Edwards, R. H. (2013). The function of alpha-synuclein. Neuron 79, 1044–1066. doi: 10.1016/j.neuron.2013.09.004
Braun, A. R., and Sachs, J. N. (2015). α-Synuclein reduces tension and increases undulations in simulations of small unilamellar vesicles. Biophys. J. 108, 1848–1851. doi: 10.1016/j.bpj.2015.03.029
Brose, N., Brunger, A., Cafiso, D., Chapman, E. R., Diao, J., Hughson, F. M., et al. (2019). Synaptic vesicle fusion: today and beyond. Nat. Struct. Mol. Biol. 26, 663–668. doi: 10.1038/s41594-019-0277-z
Brown, D. R. (2007). Interactions between metals and alpha-synuclein—function or artefact? FEBS J. 274, 3766–3774. doi: 10.1111/j.1742-4658.2007.05917.x
Brunger, A. T., Cipriano, D. J., and Diao, J. (2015). Towards reconstitution of membrane fusion mediated by SNAREs and other synaptic proteins. Crit. Rev. Biochem. Mol. Biol. 50, 231–241. doi: 10.3109/10409238.2015.1023252
Burré, J., Sharma, M., and Südhof, T. C. (2014). α-Synuclein assembles into higher-order multimers upon membrane binding to promote SNARE complex formation. Proc. Natl. Acad. Sci. U.S.A. 111, E4274–4283. doi: 10.1073/pnas.1416598111
Burré, J., Sharma, M., and Südhof, T. C. (2015). Definition of a molecular pathway mediating α-synuclein neurotoxicity. J. Neurosci. 35, 5221–5232. doi: 10.1523/JNEUROSCI.4650-14.2015
Burre, J., Sharma, M., Tsetsenis, T., Buchman, V., Etherton, M. R., and Sudhof, T. C. (2010). Alpha-synuclein promotes SNARE-complex assembly in vivo and in vitro. Science 329, 1663–1667. doi: 10.1126/science.1195227
Bussell, R. Jr., and Eliezer, D. (2003). A structural and functional role for 11-mer repeats in alpha-synuclein and other exchangeable lipid binding proteins. J. Mol. Biol. 329, 763–778. doi: 10.1016/S0022-2836(03)00520-5
Bussell, R. Jr., Ramlall, T. F., and Eliezer, D. (2005). Helix periodicity, topology, and dynamics of membrane-associated alpha-synuclein. Protein Sci. 14, 862–872. doi: 10.1110/ps.041255905
Butler, B., Goodwin, S., Saha, K., Becker, J., Sambo, D., Davari, P., et al. (2015). Dopamine transporter activity is modulated by α-synuclein. J. Biol. Chem. 290:19009. doi: 10.1074/jbc.A115.639880
Chandra, S., Chen, X., Rizo, J., Jahn, R., and Südhof, T. C. (2003). A broken alpha -helix in folded alpha -Synuclein. J. Biol. Chem. 278, 15313–15318. doi: 10.1074/jbc.M213128200
Chandra, S., Fornai, F., Kwon, H. B., Yazdani, U., Atasoy, D., Liu, X., et al. (2004). Double-knockout mice for alpha- and beta-synucleins: effect on synaptic functions. Proc. Natl. Acad. Sci U.S.A. 101, 14966–14971. doi: 10.1073/pnas.0406283101
Choi, B. K., Choi, M. G., Kim, J. Y., Yang, Y., Lai, Y., Kweon, D. H., et al. (2013). Large α-synuclein oligomers inhibit neuronal SNARE-mediated vesicle docking. Proc. Natl. Acad. Sci. U.S.A. 110, 4087–4092. doi: 10.1073/pnas.1218424110
Cholak, E., Bugge, K., Khondker, A., Gauger, K., Pedraz-Cuesta, E., Pedersen, M. E., et al. (2020). Avidity within the N-terminal anchor drives α-synuclein membrane interaction and insertion. FASEB J. 7462–7482. doi: 10.1096/fj.202000107R
Conway, K. A., Harper, J. D., and Lansbury, P. T. Jr. (2000). Fibrils formed in vitro from alpha-synuclein and two mutant forms linked to Parkinson's disease are typical amyloid. Biochemistry 39, 2552–2563. doi: 10.1021/bi991447r
Crowther, R. A., Jakes, R., Spillantini, M. G., and Goedert, M. (1998). Synthetic filaments assembled from C-terminally truncated alpha-synuclein. FEBS Lett. 436, 309–312. doi: 10.1016/S0014-5793(98)01146-6
Das, D., Bao, H., Courtney, K. C., Wu, L., and Chapman, E. R. (2020). Resolving kinetic intermediates during the regulated assembly and disassembly of fusion pores. Nat. Commun.11:231. doi: 10.1038/s41467-019-14072-7
Davidson, W. S., Jonas, A., Clayton, D. F., and George, J. M. (1998). Stabilization of alpha-synuclein secondary structure upon binding to synthetic membranes. J. Biol. Chem. 273, 9443–9449. doi: 10.1074/jbc.273.16.9443
Diao, J., Burre, J., Vivona, S., Cipriano, D. J., Sharma, M., Kyoung, M., et al. (2013). Native alpha-synuclein induces clustering of synaptic-vesicle mimics via binding to phospholipids and synaptobrevin-2/VAMP2. Elife 2:e00592. doi: 10.7554/eLife.00592
El-Agnaf, O. M., Jakes, R., Curran, M. D., Middleton, D., Ingenito, R., Bianchi, E., et al. (1998). Aggregates from mutant and wild-type alpha-synuclein proteins and NAC peptide induce apoptotic cell death in human neuroblastoma cells by formation of beta-sheet and amyloid-like filaments. FEBS Lett. 440, 71–75. doi: 10.1016/S0014-5793(98)01418-5
Eliezer, D., Kutluay, E., Bussell, R. Jr., and Browne, G. (2001). Conformational properties of alpha-synuclein in its free and lipid-associated states. J. Mol. Biol. 307, 1061–1073. doi: 10.1006/jmbi.2001.4538
Fernández, C. O., Hoyer, W., Zweckstetter, M., Jares-Erijman, E. A., Subramaniam, V., Griesinger, C., et al. (2004). NMR of alpha-synuclein-polyamine complexes elucidates the mechanism and kinetics of induced aggregation. EMBO J. 23, 2039–2046. doi: 10.1038/sj.emboj.7600211
Fusco, G., De Simone, A., Arosio, P., Vendruscolo, M., Veglia, G., and Dobson, C. M. (2016). Structural ensembles of membrane-bound α-synuclein reveal the molecular determinants of synaptic vesicle affinity. Sci. Rep. 6:27125. doi: 10.1038/srep27125
Fusco, G., De Simone, A., Gopinath, T., Vostrikov, V., Vendruscolo, M., Dobson, C. M., et al. (2014). Direct observation of the three regions in α-synuclein that determine its membrane-bound behaviour. Nat. Commun. 5:3827. doi: 10.1038/ncomms4827
George, J. M., Jin, H., Woods, W. S., and Clayton, D. F. (1995). Characterization of a novel protein regulated during the critical period for song learning in the zebra finch. Neuron 15, 361–372. doi: 10.1016/0896-6273(95)90040-3
Guo, J. T., Chen, A. Q., Kong, Q., Zhu, H., Ma, C. M., and Qin, C. (2008). Inhibition of vesicular monoamine transporter-2 activity in alpha-synuclein stably transfected SH-SY5Y cells. Cell Mol. Neurobiol. 28, 35–47. doi: 10.1007/s10571-007-9227-0
Hawk, B. J. D., Khounlo, R., and Shin, Y. K. (2019). Alpha-synuclein continues to enhance SNARE-dependent vesicle docking at exorbitant concentrations. Front. Neurosci. 13:216. doi: 10.3389/fnins.2019.00216
Hou, C., Wang, Y., Liu, J., Wang, C., and Long, J. (2017). Neurodegenerative disease related proteins have negative effects on SNARE-mediated membrane fusion in pathological confirmation. Front. Mol. Neurosci.10:66. doi: 10.3389/fnmol.2017.00066
Hoyer, W., Cherny, D., Subramaniam, V., and Jovin, T. M. (2004). Impact of the acidic C-terminal region comprising amino acids 109-140 on alpha-synuclein aggregation in vitro. Biochemistry 43, 16233–16242. doi: 10.1021/bi048453u
Huang, C. C., Chiu, T. Y., Lee, T. Y., Hsieh, H. J., Lin, C. C., and Kao, L. S. (2018). Soluble α-synuclein facilitates priming and fusion by releasing Ca(2+) from the thapsigargin-sensitive Ca(2+) pool in PC12 cells. J. Cell. Sci. 131:213017. doi: 10.1242/jcs.213017
Iwai, A., Masliah, E., Yoshimoto, M., Ge, N., Flanagan, L., de Silva, H. A., et al. (1995). The precursor protein of non-A beta component of Alzheimer's disease amyloid is a presynaptic protein of the central nervous system. Neuron 14, 467–475. doi: 10.1016/0896-6273(95)90302-X
Jensen, M. B., Bhatia, V. K., Jao, C. C., Rasmussen, J. E., Pedersen, S. L., Jensen, K. J., et al. (2011). Membrane curvature sensing by amphipathic helices: a single liposome study using α-synuclein and annexin B12. J. Biol. Chem. 286, 42603–42614. doi: 10.1074/jbc.M111.271130
Jensen, P. H., Nielsen, M. S., Jakes, R., Dotti, C. G., and Goedert, M. (1998). Binding of alpha-synuclein to brain vesicles is abolished by familial Parkinson's disease mutation. J. Biol. Chem. 273, 26292–26294. doi: 10.1074/jbc.273.41.26292
Kamp, F., Exner, N., Lutz, A. K., Wender, N., Hegermann, J., Brunner, B., et al. (2010). Inhibition of mitochondrial fusion by α-synuclein is rescued by PINK1, Parkin and DJ-1. EMBO J. 29, 3571–3589. doi: 10.1038/emboj.2010.223
Krüger, R., Kuhn, W., Müller, T., Woitalla, D., Graeber, M., Kösel, S., et al. (1998). Ala30Pro mutation in the gene encoding alpha-synuclein in Parkinson's disease. Nat. Genet. 18, 106–108. doi: 10.1038/ng0298-106
Lai, Y., Kim, S., Varkey, J., Lou, X., Song, J. K., Diao, J., et al. (2014). Nonaggregated alpha-synuclein influences SNARE-dependent vesicle docking via membrane binding. Biochemistry 53, 3889–3896. doi: 10.1021/bi5002536
Larsen, K. E., Schmitz, Y., Troyer, M. D., Mosharov, E., Dietrich, P., Quazi, A. Z., et al. (2006). Alpha-synuclein overexpression in PC12 and chromaffin cells impairs catecholamine release by interfering with a late step in exocytosis. J. Neurosci. 26, 11915–11922. doi: 10.1523/JNEUROSCI.3821-06.2006
Lashuel, H. A., Overk, C. R., Oueslati, A., and Masliah, E. (2013). The many faces of alpha-synuclein: from structure and toxicity to therapeutic target. Nat. Rev. Neurosci. 14, 38–48. doi: 10.1038/nrn3406
Lashuel, H. A., Petre, B. M., Wall, J., Simon, M., Nowak, R. J., Walz, T., et al. (2002). Alpha-synuclein, especially the Parkinson's disease-associated mutants, forms pore-like annular and tubular protofibrils. J. Mol. Biol. 322, 1089–1102. doi: 10.1016/S0022-2836(02)00735-0
Lee, F. J., Liu, F., Pristupa, Z. B., and Niznik, H. B. (2001). Direct binding and functional coupling of alpha-synuclein to the dopamine transporters accelerate dopamine-induced apoptosis. FASEB J. 15, 916–926. doi: 10.1096/fsb2fj000334com
Lesage, S., Anheim, M., Letournel, F., Bousset, L., Honoré, A, Rozas, N., et al. (2013). G51D α-synuclein mutation causes a novel parkinsonian-pyramidal syndrome. Ann. Neurol. 73, 459–471. doi: 10.1002/ana.23894
Logan, T., Bendor, J., Toupin, C., Thorn, K., and Edwards, R. H. (2017). α-Synuclein promotes dilation of the exocytotic fusion pore. Nat. Neurosci. 20, 681–689. doi: 10.1038/nn.4529
Lou, X., Kim, J., Hawk, B. J., and Shin, Y. K. (2017). α-Synuclein may cross-bridge v-SNARE and acidic phospholipids to facilitate SNARE-dependent vesicle docking. Biochem. J. 474, 2039–2049. doi: 10.1042/BCJ20170200
Man, K. N., Imig, C., Walter, A. M., Pinheiro, P. S., Stevens, D. R., Rettig, J., et al. (2015). Identification of a Munc13-sensitive step in chromaffin cell large dense-core vesicle exocytosis. Elife 4:19. doi: 10.7554/eLife.10635.019
Maroteaux, L., Campanelli, J. T., and Scheller, R. H. (1988). Synuclein: a neuron-specific protein localized to the nucleus and presynaptic nerve terminal. J. Neurosci. 8, 2804–2815. doi: 10.1523/JNEUROSCI.08-08-02804.1988
Nemani, V. M., Lu, W., Berge, V., Nakamura, K., Onoa, B., Lee, M. K., et al. (2010). Increased expression of alpha-synuclein reduces neurotransmitter release by inhibiting synaptic vesicle reclustering after endocytosis. Neuron 65, 66–79. doi: 10.1016/j.neuron.2009.12.023
Nielsen, M. S., Vorum, H., Lindersson, E., and Jensen, P. H. (2001). Ca2+ binding to alpha-synuclein regulates ligand binding and oligomerization. J. Biol. Chem. 276, 22680–22684. doi: 10.1074/jbc.M101181200
Nuscher, B., Kamp, F., Mehnert, T., Odoy, S., Haass, C., Kahle, P. J., et al. (2004). Alpha-synuclein has a high affinity for packing defects in a bilayer membrane: a thermodynamics study. J. Biol. Chem. 279, 21966–21975. doi: 10.1074/jbc.M401076200
O'Leary, E. I., and Lee, J. C. (2019). Interplay between α-synuclein amyloid formation and membrane structure. Biochim. Biophys. Acta Proteins Proteom. 1867, 483–491. doi: 10.1016/j.bbapap.2018.09.012
Oueslati, A., Fournier, M., and Lashuel, H. A. (2010). Role of post-translational modifications in modulating the structure, function and toxicity of alpha-synuclein: implications for Parkinson's disease pathogenesis and therapies. Prog. Brain Res. 183, 115–145. doi: 10.1016/S0079-6123(10)83007-9
Paik, S. R., Shin, H. J., Lee, J. H., Chang, C. S., and Kim, J. (1999). Copper(II)-induced self-oligomerization of alpha-synuclein. Biochem. J. 340, 821–828. doi: 10.1042/bj3400821
Park, S. M., Jung, H. Y., Kim, T. D., Park, J. H., Yang, C. H., and Kim, J. (2002). Distinct roles of the N-terminal-binding domain and the C-terminal-solubilizing domain of alpha-synuclein, a molecular chaperone. J. Biol. Chem. 277, 28512–28520. doi: 10.1074/jbc.M111971200
Pasanen, P., Myllykangas, L., Siitonen, M., Raunio, A., Kaakkola, S., Lyytinen, J., et al. (2014). Novel α-synuclein mutation A53E associated with atypical multiple system atrophy and Parkinson's disease-type pathology. Neurobiol. Aging 35, 2180.e2181–2185. doi: 10.1016/j.neurobiolaging.2014.03.024
Perlmutter, J. D., Braun, A. R., and Sachs, J. N. (2009). Curvature dynamics of alpha-synuclein familial Parkinson disease mutants: molecular simulations of the micelle- and bilayer-bound forms. J. Biol. Chem. 284, 7177–7189. doi: 10.1074/jbc.M808895200
Polymeropoulos, M. H., Lavedan, C., Leroy, E., Ide, S. E., Dehejia, A., Dutra, A., et al. (1997). Mutation in the alpha-synuclein gene identified in families with Parkinson's disease. Science 276, 2045–2047. doi: 10.1126/science.276.5321.2045
Pozo Devoto, V. M., Dimopoulos, N., Alloatti, M., Pardi, M. B., Saez, T. M., Otero, M. G., et al. (2017). αSynuclein control of mitochondrial homeostasis in human-derived neurons is disrupted by mutations associated with Parkinson's disease. Sci. Rep. 7:5042. doi: 10.1038/s41598-017-05334-9
Pranke, I. M., Morello, V., Bigay, J., Gibson, K., Verbavatz, J. M., Antonny, B., et al. (2011). α-Synuclein and ALPS motifs are membrane curvature sensors whose contrasting chemistry mediates selective vesicle binding. J. Cell. Biol. 194, 89–103. doi: 10.1083/jcb.201011118
Proukakis, C., Dudzik, C. G., Brier, T., MacKay, D. S., Cooper, J. M., Millhauser, G. L., et al. (2013). A novel α-synuclein missense mutation in Parkinson disease. Neurology 80, 1062–1064. doi: 10.1212/WNL.0b013e31828727ba
Schechter, M., Atias, M., Abd Elhadi, S., Davidi, D., Gitler, D., and Sharon, R. (2020). α-Synuclein facilitates endocytosis by elevating the steady-state levels of phosphatidylinositol 4,5-bisphosphate. J. Biol. Chem. 295, 18076–18090. doi: 10.1074/jbc.RA120.015319
Sevcsik, E., Trexler, A. J., Dunn, J. M., and Rhoades, E. (2011). Allostery in a disordered protein: oxidative modifications to α-synuclein act distally to regulate membrane binding. J. Am. Chem. Soc. 133, 7152–7158. doi: 10.1021/ja2009554
Shi, L., Shen, Q. T., Kiel, A., Wang, J., Wang, H. W., Melia, T. J., et al. (2012). SNARE proteins: one to fuse and three to keep the nascent fusion pore open. Science 335, 1355–1359. doi: 10.1126/science.1214984
Snead, D., and Eliezer, D. (2014). Alpha-synuclein function and dysfunction on cellular membranes. Exp. Neurobiol. 23, 292–313. doi: 10.5607/en.2014.23.4.292
Sun, J., Wang, L., Bao, H., Premi, S., Das, U., Chapman, E. R., et al. (2019). Functional cooperation of α-synuclein and VAMP2 in synaptic vesicle recycling. Proc. Natl. Acad. Sci. U.S.A. 116, 11113–11115. doi: 10.1073/pnas.1903049116
Sutton, R. B., Fasshauer, D., Jahn, R., and Brunger, A. T. (1998). Crystal structure of a SNARE complex involved in synaptic exocytosis at 2.4 A resolution. Nature 395, 347–353. doi: 10.1038/26412
Swant, J., Goodwin, J. S., North, A., Ali, A. A., Gamble-George, J., Chirwa, S., et al. (2011). α-Synuclein stimulates a dopamine transporter-dependent chloride current and modulates the activity of the transporter. J. Biol. Chem. 86, 43933–43943. doi: 10.1074/jbc.M111.241232
Tian, Z., Gong, J., Crowe, M., Lei, M., Li, D., Ji, B., et al. (2019). Biochemical studies of membrane fusion at the single-particle level. Prog. Lipid Res. 73, 92–100. doi: 10.1016/j.plipres.2019.01.001
Uéda, K., Fukushima, H., Masliah, E., Xia, Y., Iwai, A., Yoshimoto, M., et al. (1993). Molecular cloning of cDNA encoding an unrecognized component of amyloid in Alzheimer disease. Proc. Natl. Acad. Sci. U.S.A. 90, 11282–11286. doi: 10.1073/pnas.90.23.11282
Ulmer, T. S., Bax, A., Cole, N. B., and Nussbaum, R. L. (2005). Structure and dynamics of micelle-bound human alpha-synuclein. J. Biol. Chem. 280, 9595–9603. doi: 10.1074/jbc.M411805200
Uversky, V. N. (2007). Neuropathology, biochemistry, and biophysics of alpha-synuclein aggregation. J. Neurochem. 103, 17–37. doi: 10.1111/j.1471-4159.2007.04764.x
Wang, C., Zhao, C., Li, D., Tian, Z., Lai, Y., Diao, J., et al. (2016a). Versatile structures of α-synuclein. Front. Mol. Neurosci. 9:48. doi: 10.3389/fnmol.2016.00048
Wang, L., Das, U., Scott, D. A., Tang, Y., McLean, P. J., and Roy, S. (2014). α-synuclein multimers cluster synaptic vesicles and attenuate recycling. Curr. Biol. 24,:2319–2326. doi: 10.1016/j.cub.2014.08.027
Wang, T., and Hay, J. C. (2015). Alpha-synuclein toxicity in the early secretory pathway: how it drives neurodegeneration in parkinsons disease. Front. Neurosci. 9:433. doi: 10.3389/fnins.2015.00433
Wang, Y., Li, L., Hou, C., Lai, Y., Long, J., Liu, J., et al. (2016b). SNARE-mediated membrane fusion in autophagy. Semin. Cell Dev. Biol. 60, 97–104. doi: 10.1016/j.semcdb.2016.07.009
Wersinger, C., and Sidhu, A. (2003). Attenuation of dopamine transporter activity by alpha-synuclein. Neurosci. Lett. 340, 189–192. doi: 10.1016/S0304-3940(03)00097-1
Wickner, W., and Schekman, R. (2008). Membrane fusion. Nat. Struct. Mol. Biol. 15, 658–664. doi: 10.1038/nsmb.1451
Keywords: Parkinson's disease, alpha-synuclein, membrane, SNAREs, synaptic transmission
Citation: Liu C, Zhao Y, Xi H, Jiang J, Yu Y and Dong W (2021) The Membrane Interaction of Alpha-Synuclein. Front. Cell. Neurosci. 15:633727. doi: 10.3389/fncel.2021.633727
Received: 26 November 2020; Accepted: 28 January 2021;
Published: 04 March 2021.
Edited by:
Jiajie Diao, University of Cincinnati, United StatesReviewed by:
Huan Bao, The Scripps Research Institute, United StatesShijun Weng, Fudan University, China
Fushun Wang, Nanjing University of Chinese Medicine, China
Copyright © 2021 Liu, Zhao, Xi, Jiang, Yu and Dong. This is an open-access article distributed under the terms of the Creative Commons Attribution License (CC BY). The use, distribution or reproduction in other forums is permitted, provided the original author(s) and the copyright owner(s) are credited and that the original publication in this journal is cited, in accordance with accepted academic practice. No use, distribution or reproduction is permitted which does not comply with these terms.
*Correspondence: Wei Dong, ZG9uZ3dlaUBzd211LmVkdS5jbg==
†These authors have contributed equally to this work