- Department of Medical Pharmacology, Nagasaki University Graduate School of Biomedical Sciences, Nagasaki, Japan
Heteromers between mu opioid receptor (MOPr) and delta opioid receptor (DOPr) (i.e., MOPr-DOPr heteromer) have been found to be expressed in different brain regions, in the spinal cord, and in dorsal root ganglia. Recent studies on this heteromer reveal its important pathophysiological function in pain regulation including neuropathic pain; this suggests a role as a novel therapeutic target in chronic pain management. In addition, receptor transporter protein 4 (RTP4) has been shown to be involved in the intracellular maturation of the MOPr-DOPr heteromers. RTP4 appears to have unique distribution in vivo being highly expressed in sensory neurons and also macrophages; the latter are effector cells of the innate immune system that phagocytose foreign substances and secrete both pro-inflammatory and antimicrobial mediators; this suggests a possible contribution of RTP4 to neuronal immune-related pathological conditions such as neuropathic pain. Although RTP4 could be considered as an important therapeutic target in the management of pain via MOPr-DOPr heteromer, a few reports have supported this. This review will summarize the possible role or functions of the MOPr-DOPr heteromer and its regulatory molecule RTP4 in pain modulation at sensory neurons.
Introduction
In the nervous system including central and peripheral neurons, G-protein coupled receptors (GPCRs) play important roles in the signal transduction by neurotransmitters. For example, opioid receptors are widely distributed along the pain pathways and play a role in pain attenuation. Over the last decade studies have shown that heteromerization of GPCRs alters their pharmacological characteristics or their function (Gomes et al., 2016). That is, heteromers exhibit novel pharmacology, such as altered ligand-binding properties, G-protein coupling, and trafficking, that differs from that of homomers. Several groups including ours have focused on the physiological role of heteromerization between mu opioid receptors (MOPr) and delta opioid receptors (DOPr) and found that it may be involved in mechanisms modulating antinociceptive tolerance to morphine (He et al., 2011; Fujita et al., 2019). Thus early studies showed that DOPr antagonists decreased tolerance to the analgesic effects of morphine, a MOPr agonist (Abdelhamid and Takemori, 1991; Schiller, 2010), increased morphine-mediated antinociception (Gomes et al., 2004), and animals lacking DOPr or the gene-encoding preprotachykinin A do not exhibit morphine tolerance (Zhu et al., 1999; Guan et al., 2005). This was followed by studies by several groups that detected the presence of MOPr-DOPr heteromers and characterized their properties [reviewed in Rozenfeld and Devi (2011), Gomes et al. (2016), Ugur et al. (2018), Zhang et al. (2020)]. One of the studies showed that the MOPr-DOPr heteromer is constitutively associated with beta-arrestin, and treatment with DAMGO alone (a MOPr agonist) leads to sustained MAPK phosphorylation (characteristic of beta-arrestin-mediated phosphorylation), while a combination of DAMGO and TIPPψ (a DOPr antagonist) leads to a rapid and transient increase (characteristic of G protein-mediated signaling) as seen in cells expressing only MOPr (Rozenfeld and Devi, 2007). This signaling switch from G-protein (for MOPr) to beta-arrestin (for MOPr-DOPr heteromer) results in the cytoplasmic retention of MAPK and differential activation of downstream transcription factors (Rozenfeld and Devi, 2007). Interestingly, mice lacking beta-arrestin2 exhibit increased and prolonged morphine-induced antinociception (Bohn et al., 1999) and do not develop the antinociceptive tolerance to morphine (Bohn et al., 2000). The constitutive association of the MOPr-DOPr heteromer with beta-arrestin together with observations that chronic morphine exposure upregulates MOPr-DOPr heteromers in brain regions involved in pain signaling (e.g., rostroventral medulla and nucleus accumbens) (Gupta et al., 2010). Pierre et al. suggest the possibility that these heteromers play a pivotal role in the regulation of pain and antinociceptive tolerance by showing the co-localization of MOPr and DOPr after chronic morphine treatment (Pierre et al., 2019). Ligands targeting the MOPr-DOPr heteromer have been reported. These include bivalent ligand that contains MOPr agonist and DOPr antagonist pharmacophores separated by a spacer arm (MDAN series) and a small molecule ligand, CYM51010, that exhibit potent antinociception with reduced antinociceptive tolerance or dependence comparing to morphine (Daniels et al., 2005; Gomes et al., 2013; Fujita, 2020). Together these studies suggest that the MOPr-DOPr heteromer could be of therapeutic significance as a target for pain management.
Although not much is known about the mechanisms that regulate the biosynthesis and maturation of MOPr-DOPr heteromers, a receptor chaperone protein called receptor transporter protein 4 (RTP4), appears to play a key role in endogenous MOPr-DOPr heteromerization and in increasing MOPr-DOPr levels following long-term MOPr stimulation (Fujita et al., 2019). Furthermore, only 4 publications have reported the RTP4 interaction with GPCRs, among which 2 with MOPr-DOPr heteromer (Behrens et al., 2006; Decaillot et al., 2008; Mainland and Matsunami, 2012; Fujita et al., 2019). In these reports, as described above, the role of RTP4 as an endogenous chaperone molecule in regulation of MOPr-DOPr heteromers under physiological condition or under chronic morphine treatment has been clearly demonstrated (Decaillot et al., 2008; Fujita et al., 2019). Other 2 publications reported the role of RTP4 in regulation of taste receptors (Behrens et al., 2006; Mainland and Matsunami, 2012). They speculate that binding of RTP4 could expose an existing targeting motif within the receptor or mask an intracellular retention signal, while a detailed study on the mechanism by which the plasma membrane localization of taste receptors is regulated and on the exact roles of RTP4 will be necessary to understand how the function of receptors is achieved in vivo (Behrens et al., 2006). Furthermore, besides serving as a chaperone protein for the transport of GPCRs (Behrens et al., 2006; Decaillot et al., 2008; Gifford et al., 2008), RTP4 has been shown to have other physiological roles. That is, RTP4 seems to be an important marker in various cancers, is involved in hypertension and regulated by interferon as described and discussed by Fujita et al. (2019).
Interestingly, recent studies reveal that RTP4 expression is induced upon immune stimulation in vitro (Schoggins et al., 2011; Hoyo-Becerra et al., 2015; Nair et al., 2017; Dang et al., 2018); this suggests an effect on neuronal immune-related pathological states including neuropathic pain. In this review, we outline the possible role of the MOPr-DOPr heteromer and RTP4 in pain with an emphasis on neuropathic pain.
The Distribution of MOPr-DOPr Heteromer in the Central and Peripheral Neurons – The Possible Implications to the Inhibition of Pain Perception
By using a selective antibody targeting the MOPr-DOPr heteromer, Gupta et al. (2010) revealed its expression in several brain regions including cortex, hippocampus, hypothalamus, nucleus accumbens, prefrontal cortex, pons, striatum, and ventral tegmental area (Gupta et al., 2010). In addition, double-knockin animals expressing MOPr fused to the red fluorescent mcherry protein (MOPr-mcherry) and DOPr fused to the green fluorescent protein (DOPr-eGFP), Erbs et al. (2015) showed that both receptors are co-expressed in selective brain regions including the lateral hypothalamus and the rostroventral medulla, the main nucleus of the trapezoid body, the hippocampus, and the pons in agreement with the findings by Gupta et al. (2010). Moreover, studies with the double-knockin mice show that dependence to morphine leads to alterations in MOPr-DOPr heteromer expression in brain regions (Pierre et al., 2019). Interestingly, co-localization of MOPr-mcherry and DOPr-eGFP fluorescent signals were observed in GABAergic interneurons that control the firing rate of glutamatergic neurons; this suggests an involvement of MOPr-DOPr heteromers in the regulation of pain. This is supported by the co-expression of the two receptors in the high throughput nociceptive pathways that include the rostral ventral medulla, lateral parabrachial nucleus, spinal cord, and dorsal root ganglion (DRG) neurons, the pseudo-bipolar neurons, with a peripheral branch that innervates their target organ and a central branch that carries the somatosensory information to the spinal cord (Erbs et al., 2015). In situ hybridization, single-cell PCR, electrophysiology, and immunostaining studies also provide evidence for the coexistence of functional DOPr and MOPr in a subpopulation of small-diameter peptidergic DRG neurons (Wang et al., 2010; Guerrero-Alba et al., 2018). Finally, a pharmacologic study provided evidence that MOPr and DOPr colocalize on a functionally important population of TrkA positive peptidergic nociceptors (Joseph and Levine, 2010).
Recently studies have started to elucidate the therapeutic potential for MOPr-DOPr heteromers. A study by Fujita et al. (2014) suggested that MOPr-DOPr heteromer in enteric neurons as a therapeutic target for the treatment of diarrhea-predominant irritable bowel syndrome (Fujita et al., 2014). The study found that eluxadoline, an orally active mixed MOPr agonist DOPr antagonist, exhibits an anti-diarrheal effect, and in vitro studies revealed that the signaling profile of eluxadoline that can be partly blocked by MOPr-DOPr heteromer-selective antibodies. Moreover, the study reported that eluxadoline can block castor oil-induced diarrhea in wild type mice and this is attenuated in DOPr knockout (DOPr–/–) mice indicating the involvement of DOPr probably through MOPr-DOPr heteromerization in the in vivo effects of eluxadoline (Fujita et al., 2014). This suggests that the actions of eluxadoline could, at least in part, be due to targeting of MOPr-DOPr heteromers in the gut. Another study by Tiwari et al. (2020) suggested that MOPr-DOPr heteromer as a potential therapeutic target for the treatment of neuropathic pain. The authors observed significant upregulation of MOPr-DOPr heteromer expression in uninjured L4 DRG neurons after L5 spinal nerve ligation (SNL) in rats. Furthermore, they found that the MOPr-DOPr heteromer targeting ligand, CYM51010, significantly inhibited mechanical and heat hypersensitivity in this pain model (Tiwari et al., 2020). These results suggest that the spinal nerve injury increases MOPr-DOPr heteromer levels in uninjured DRG neurons, and that the heteromer may be a potential therapeutic target for relieving neuropathic pain in sensory neurons. However, further studies are needed to characterize in detail the contribution of MOPr-DOPr heteromer to the modulation of pain perception.
The Role of Receptor Transporter Protein 4 (RTP4) in the Regulation of MOPr-DOPr Heteromerization
The function and the maturation of GPCRs (and their heteromers) is known to be affected and regulated by receptor chaperone proteins (Ritter and Hall, 2009; Williams and Devi, 2010). Since the receptors must be properly folded after translation and, in most cases, transported to the plasma membrane to achieve functional activity, chaperones facilitating their transport significantly affect receptor function. Early studies with mammalian odorant receptors or taste receptors showed that receptor transporter protein family members (i.e., REEPs/RTPs) enhance receptor expression at the plasma membrane (Saito et al., 2004; Ritter and Hall, 2009). The analysis of RTP expression in mouse brain (Allen Brain Atlas)1 reveals that RTP4 is the most abundant subtype of the RTP family (RTP1-4) in the brain. RTP4 is known to promote cell-surface expression of a group of GPCRs involved in mediation of pain relief, bitter taste sense, or sense of smell (Saito et al., 2004; Behrens et al., 2006; Decaillot et al., 2008). RTP4 (249 amino acids) has a single transmembrane domain (228th to 248th amino acids) located near the C-terminal end. The N-terminal end is considered to be intracellular (1st to 227th amino acids) and the C-terminal end (249th amino acid) extracellular with no signal peptide (UniProt)2 (Figure 1A).
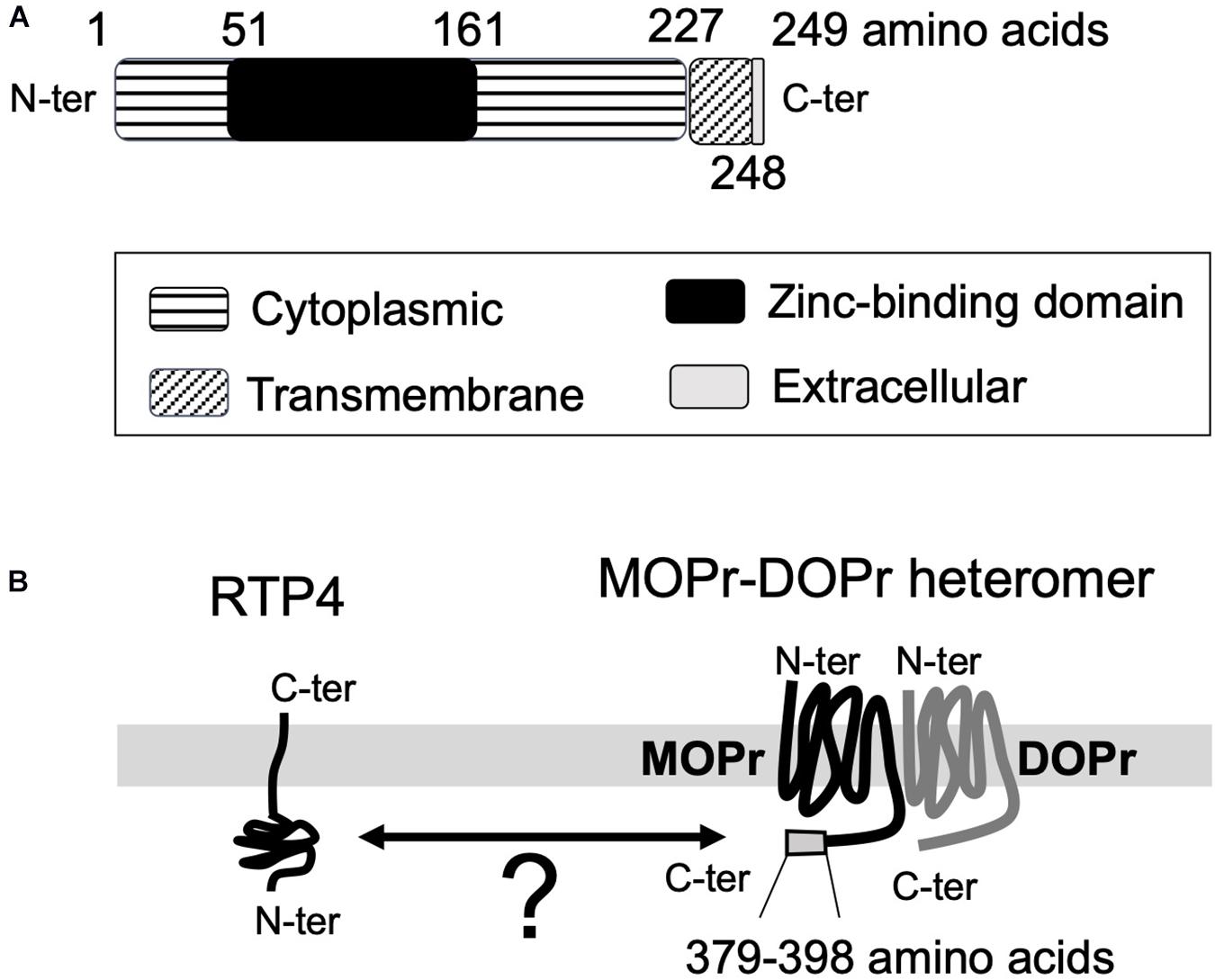
Figure 1. The topological information for RTP4 (A) and the schematic interaction between RTP4 and MOPr-DOPr heteromers (B). The data was obtained via UniProt data base (uniprot.org). RTP4 (249 amino acids) has a single predicted transmembrane domain (228th to 248th amino acids) located near the C-terminal end. It is considered that the N-terminal end is intracellular (1st to 227th amino acids) and the C-terminal end (249th amino acid) is extracellular, with no signal peptide (UniProt,https://www.uniprot.org/uniprot/Q9ER80) (A). Decaillot et al. (2008) have suggested that the interaction between RTP4 and MOPr-DOPr heteromer occurs in the cytoplasmic region since the region within the MOPr cytoplasmic C-terminal 29 amino acid residues (i.e., 370–398 aa) was necessary for interaction with RTP4 (B).
In the case of MOPr-DOPr heteromers, studies reported the requirement of RTP4 for efficient cell surface expression (Decaillot et al., 2008; Fujita et al., 2019) since in its absence a significant portion of the heteromer localized to the Golgi apparatus without being transported to the cell surface. On the other hand, the heterologous expression of RTP4 enhanced cell surface MOPr-DOPr heteromer localization (Decaillot et al., 2008); this was due to protection of the heteromer from ubiquitination and proteasomal degradation during folding and maturation. Although the details have not as yet been elucidated, the interaction between RTP4 and the MOPr-DOPr heteromer occurs via the cytoplasmic region of MOPr since cytoplasmic C-terminal 29 amino acid residues (i.e., 370–398 amino acids) of this receptor were necessary for interaction with RTP4 (Decaillot et al., 2008; Figure 1B). Interestingly, long-term MOPr stimulation by DAMGO enhanced the cell surface MOPr-DOPr heteromer expression; this was reversed by RTP4 siRNA (Fujita et al., 2019). More importantly, long-term treatment with DAMGO upregulates RTP4 levels. Upregulation in RTP4 levels and MOPr-DOPr heteromers is also seen in mice hypothalamus following chronic treatment with morphine that leads to antinociceptive tolerance (Fujita et al., 2019). While these studies show an important role for RTP4 in regulating cell surface expression of the MOPr-DOPr heteromer (Figure 2), it is still not clear if this chaperone remains associated with the heteromer at the plasma membrane and whether it modulates ligand binding to and signaling by the heteromer.
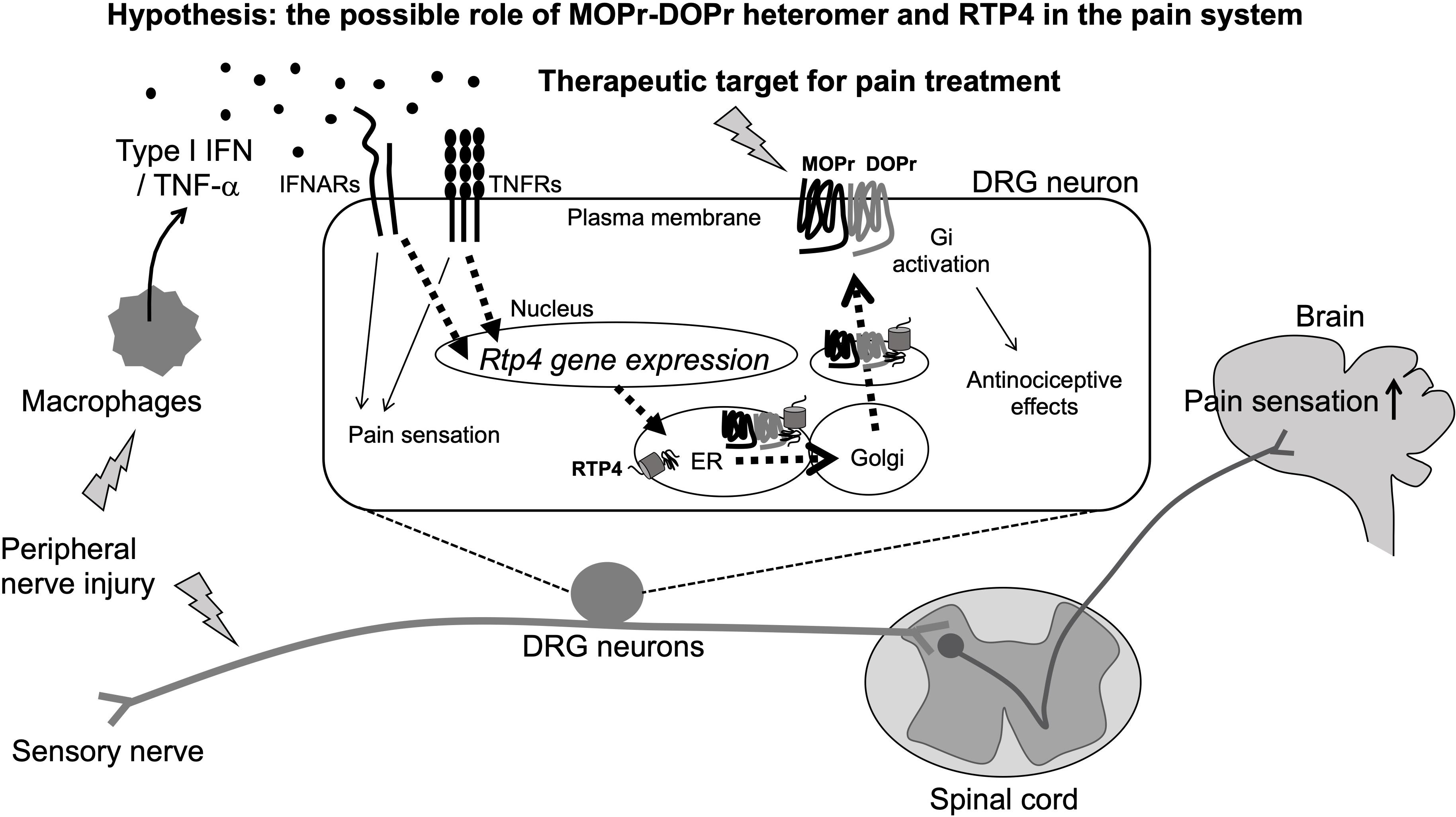
Figure 2. Schematic illustration of pain sensitization and the possible involvement of MOPr-DOPr heteromers and RTP4 in the regulation of pain sensation (Hypothesis). The peripheral nerve injury will stimulate peripheral immune response cells like macrophages that can produce type I IFN. RTP4 could be upregulated following the IFNARs/TNFRs activation in DRG neurons and thus induce the upregulation of MOPr-DOPr heteromer at the cell surface membrane (as a part of compensatory mechanisms occurring during neuropathic pain). Although the critical role for RTP4 and the MOPr-DOPr heteromer in pain sensitization at the spinal cord and the central nervous system is still not clear, the MOPr-DOPr heteromer and RTP4 could be a potent therapeutic target for pain treatment.
Possible Physiological Role of RTP4 in Pain Perception – The Involvement of Cytokine Mediated RTP4 Upregulation and MOPr-DOPr Heteromer Formation in Sensory Neurons
The database of the mouse brain3 provides important information about RTP4 gene expression in various types of neurons (including central and peripheral) and non-neuronal cells. Taking into account the excitatory neurons and inhibitory neurons in brain regions including cortex, hippocampus, midbrain, hindbrain, thalamus, hypothalamus, telencephalon, amygdala, pallidum, septal nucleus, and spinal cord, RTP4 is highly expressed in DRG neurons (both peptidergic and non-peptidergic), sympathetic (cholinergic and noradrenergic), enteric (nitrergic and cholinergic), and noradrenergic muscle neurons. Interestingly, a Gene Expression Omnibus (GEO)4 DataSets revealed an increase in RTP4 gene expression in L4 and L5 DRG neurons (both contralateral and ipsilateral) 28 days post-surgery in a rat model of L5 SNL neuropathic pain. This suggests a possible physiological role for RTP4 as a regulatory molecule for pain perception at sensory neurons, since other study also reported an increase of MOPr-DOPr heteromer formation at the cell surface of the DRG neurons in neuropathic pain animals (Tiwari et al., 2020; Figure 2). Thus, upregulation of RTP4 and MOPr-DOPr heteromer levels may be a part of compensatory mechanisms occurring during neuropathic pain. More experimental studies are needed to confirm this and well as the mechanisms involved in this process.
A possible mechanism for the upregulation of RTP4 in DRG neurons during neuropathic pain could involve “interferon (IFN).” This is because the RTP4 gene can be upregulated by type I IFN (IFN-α, IFN-β, IFN -ε, IFN-κ, IFN-ω, IFN-δ, IFN-ζ, and IFN-τ) leading to RTP4 being referred to as “IFN stimulated gene” (Schoggins et al., 2011; Hoyo-Becerra et al., 2015; Nair et al., 2017; Dang et al., 2018). Peripheral immune responding cell such as plasmacytoid dendritic cells or macrophages and the central glial cells like astrocytes or microglia have been identified as main producers of type I IFNs after viral infection, and the IFNs have been shown to affect neuronal survival, neurite outgrowth leading to modulation of the glutamatergic neurotransmission under some pathological conditions including multiple sclerosis and Alzheimer’s disease (reviewed in Blank and Prinz, 2017). Interestingly, type I IFN has been reported as one of the mediators of pain (Barragan-Iglesias et al., 2020; Figure 2). Type I IFN can activate their specific receptors (IFNRs, i.e., IFNAR1, IFNAR2) resulting in downstream activation of cellular signaling and a variety of physiological responses including pain regulation. Thus, Barragan-Iglesias et al. (2020) found that type I IFNs such as IFN-α and IFN-β will act directly on nociceptors in DRG neurons, where IFNARs are expressed, to activate JAK/STAT signaling pathways leading to increased phosphorylation of mitogen activated protein kinase and of eukaryotic initiation factor 4E to promote pain hypersensitivity. They hypothesized that this mechanism may be involved in the development of pain evoked by viral infections such as HIV or herpes virus. Also, TNF-α could be one of the additional regulatory molecules of RTP4 at the periphery since it has been reported to mediate the upregulation of RTP4 expression as a neuroinflammatory signals (Intlekofer et al., 2019). As it is well known, macrophages can release TNF-α (Roy et al., 1998; Eisenstein, 2019), thus it is speculated that several inflammatory cytokines including IFN and TNF-α will be released by peripheral immune responding cells and thus may lead to upregulation of the RTP4 in sensory neuronal cells. Since these cytokines positively regulate the development of neuropathic pain (Leung and Cahill, 2010), the upregulation of RTP4 and thus increase of MOPr-DOPr heteromer levels may be a part of compensatory mechanisms occurring during neuropathic pain as described above.
In the context of pain, the immune system and the activation of peripheral macrophages or of central microglia, of resident macrophage cells of the central nervous system, have been closely related to the development of neuropathic or inflammatory pain (Kiguchi et al., 2017; Xu et al., 2020). At the injured tissue, macrophage cells or microglia will be activated leading to induction of a variety of pro-inflammatory factors that directly or indirectly sensitize pain-processing neurons in the spinal dorsal horn, DRG, and in brain regions (e.g., cortex, thalamus, amygdala, and hypothalamus) in various models of neuropathic pain including nerve damage, diabetes, and chemotherapy models (Kiguchi et al., 2017; Xu et al., 2020). According to the database Bio GPS5, the gene expression of RTP4 is highly detected in macrophages compared to neurons indicating a possible physiological role of RTP4 in regulation of immune responses and the development of pain, although a direct role of RTP4 in the activation or accumulation of macrophages or microglia in tissues should be investigated.
Although RTP4 was initially characterized as a GPCR chaperone protein as described above (Saito et al., 2004; Behrens et al., 2006; Mainland and Matsunami, 2012), more recent publications clearly revealed its significant role in regulation of immune responses such as IFN-related antiviral immunity (Schoggins et al., 2011; Nair et al., 2017; Dang et al., 2018; Zarei Ghobadi et al., 2019; He et al., 2020). Thus, it is suggested that RTP4 could be induced by type I IFNs and work as a negative regulator of interferons pathways. Moreover, a recent paper demonstrated that RTP4 knockout (RTP4–/–) mice showed a higher production of type I IFN (He et al., 2020), suggesting an important role of RTP4 in positive regulation of the immune responses, which could be explored for disease treatment and management. Taken together, RTP4 is thought to be involved in either negative or positive regulation of IFN responses, and thus it is possible that RTP4 could be involved in the pain regulatory system.
Summary and Perspective
In this review, we summarized and hypothesized the important role of MOPr-DOPr heteromers in pain perception that is associated with neuropathic pain. Further, we suggest a possible role for RTP4, a chaperone protein of MOPr-DOPr heteromers, in pain. Although more studies are needed that show a direct contribution of MOPr-DOPr heteromers and RTP4 in neuropathic pain models, their important roles were suggested by several findings as follows.
1. MORr-DOPr heteromer and RTP4 appear to be found in DRG sensory neurons. 2. MOPr-DOPr heteromer is upregulated in the uninjured DRG by nerve injury. 3. RTP4 is a positive regulator of MOPr-DOPr heteromers in neuronal cells. 4. In DRG neurons, RTP4 can be upregulated by the nerve injury at the contralateral and the ipsilateral sites. 5. RTP4 has been shown to be upregulated by type I IFNs or TNF-α. 5. RTP4 is highly expressed in immune-related cells like macrophages that are known to produce cytokines including type I IFNs or TNF-α and to contribute to the development of neuropathic pain or pain sensation.
From these findings, we speculate that RTP4 may be a key molecule in the development of pain via modulating of MOPr-DOPr heteromer expression in neurons and thus this could be a potent therapeutic target for pain treatment. Although, the role of RTP4 in macrophages or microglia is not clear, it could contribute to the neuronal pain perception by activating the macrophages or microglia. Thus RTP4 and MOPr-DOPr heteromer are important novel therapeutic targets for the next generation pain therapeutics.
Author Contributions
The author confirms being the sole contributor of this work and has approved it for publication.
Funding
This work was supported by the Japan Society for the Promotion of Science KAKENHI (Grants 18K06894) and the Yamaguchi Memorial Foundation for the Promotion of Science on 2020.
Conflict of Interest
The author declares that the research was conducted in the absence of any commercial or financial relationships that could be construed as a potential conflict of interest.
Acknowledgments
The author is grateful to Dr. Ivone Gomes and Dr. Lakshmi A. Devi, Department of Pharmacology, Icahn School of Medicine at Mount Sinai, NYC, for careful review and editing of the manuscript. The author thanks Dr. Jun Aruga, Dr. Minoru Hatayama, and Dr. Hayato Matsunaga, Department of Medical Pharmacology, Nagasaki University Graduate School of Biomedical Sciences, Nagasaki, Japan, for reading and discussing on this manuscript.
Footnotes
- ^ https://mouse.brain-map.org/experiment/show/68444738
- ^ https://www.uniprot.org/uniprot/Q9ER80
- ^ http://mousebrain.org/genesearch.html
- ^ https://www.ncbi.nlm.nih.gov/geo/query/acc.cgi?acc=GSM63118
- ^ http://biogps.org/#goto=welcome
References
Abdelhamid, E. E., and Takemori, A. E. (1991). Characteristics of mu and delta opioid binding sites in striatal slices of morphine-tolerant and -dependent mice. Eur. J. Pharmacol 198, 157–163. doi: 10.1016/0014-2999(91)90615-w
Barragan-Iglesias, P., Franco-Enzastiga, U., Jeevakumar, V., Shiers, S., Wangzhou, A., Granados-Soto, V., et al. (2020). Type I interferons act directly on nociceptors to produce pain sensitization: implications for viral infection-induced pain. J. Neurosci. 40, 3517–3532. doi: 10.1523/jneurosci.3055-19.2020
Behrens, M., Bartelt, J., Reichling, C., Winnig, M., Kuhn, C., and Meyerhof, W. (2006). Members of RTP and REEP gene families influence functional bitter taste receptor expression. J. Biol. Chem. 281, 20650–20659. doi: 10.1074/jbc.m513637200
Blank, T., and Prinz, M. (2017). Type I interferon pathway in CNS homeostasis and neurological disorders. Glia 65, 1397–1406. doi: 10.1002/glia.23154
Bohn, L. M., Gainetdinov, R. R., Lin, F. T., Lefkowitz, R. J., and Caron, M. G. (2000). Mu-opioid receptor desensitization by beta-arrestin-2 determines morphine tolerance but not dependence. Nature 408, 720–723. doi: 10.1038/35047086
Bohn, L. M., Lefkowitz, R. J., Gainetdinov, R. R., Peppel, K., Caron, M. G., and Lin, F. T. (1999). Enhanced morphine analgesia in mice lacking beta-arrestin 2. Science 286, 2495–2498. doi: 10.1126/science.286.5449.2495
Dang, W., Xu, L., Yin, Y., Chen, S., Wang, W., Hakim, M. S., et al. (2018). IRF-1, RIG-I and MDA5 display potent antiviral activities against norovirus coordinately induced by different types of interferons. Antiviral Res. 155, 48–59. doi: 10.1016/j.antiviral.2018.05.004
Daniels, D. J., Lenard, N. R., Etienne, C. L., Law, P. Y., Roerig, S. C., and Portoghese, P. S. (2005). Opioid-induced tolerance and dependence in mice is modulated by the distance between pharmacophores in a bivalent ligand series. Proc. Natl. Acad. Sci. U.S.A. 102, 19208–19213. doi: 10.1073/pnas.0506627102
Decaillot, F. M., Rozenfeld, R., Gupta, A., and Devi, L. A. (2008). Cell surface targeting of mu-delta opioid receptor heterodimers by RTP4. Proc. Natl. Acad. Sci. U.S.A. 105, 16045–16050. doi: 10.1073/pnas.0804106105
Eisenstein, T. K. (2019). The role of opioid receptors in immune system function. Front. Immunol. 10:2904. doi: 10.3389/fimmu.2019.02904
Erbs, E., Faget, L., Scherrer, G., Matifas, A., Filliol, D., Vonesch, J. L., et al. (2015). A mu-delta opioid receptor brain atlas reveals neuronal co-occurrence in subcortical networks. Brain Struct. Funct. 220, 677–702. doi: 10.1007/s00429-014-0717-9
Fujita, W., Gomes, I., Dove, L. S., Prohaska, D., McIntyre, G., and Devi, L. A. (2014). Molecular characterization of eluxadoline as a potential ligand targeting mu-delta opioid receptor heteromers. Biochem. Pharmacol. 92, 448–456. doi: 10.1016/j.bcp.2014.09.015
Fujita, W., Yokote, M., Gomes, I., Gupta, A., Ueda, H., and Devi, L. A. (2019). Regulation of an opioid receptor chaperone protein. RTP4, by Morphine. Mol. Pharmacol. 95, 11–19. doi: 10.1124/mol.118.112987
Fujita, W. (2020). Aiming at ideal therapeutics- MOPr/DOPr or MOPr-DOPr heteromer-targeting ligand. Curr. Top. Med. Chem. 20. doi: 10.2174/1568026620666200423095231 [Epub ahead of print].
Gifford, C. A., Assiri, A. M., Satterfield, M. C., Spencer, T. E., and Ott, T. L. (2008). Receptor transporter protein 4 (RTP4) in endometrium, ovary, and peripheral blood leukocytes of pregnant and cyclic ewes. Biol. Reprod. 79, 518–524. doi: 10.1095/biolreprod.108.069468
Gomes, I., Ayoub, M. A., Fujita, W., Jaeger, W. C., Pfleger, K. D., and Devi, L. A. (2016). G protein-coupled receptor heteromers. Annu. Rev. Pharmacol. Toxicol. 56, 403–425.
Gomes, I., Fujita, W., Gupta, A., Saldanha, S. A., Negri, A., Pinello, C. E., et al. (2013). Identification of a mu-delta opioid receptor heteromer-biased agonist with antinociceptive activity. Proc. Natl. Acad. Sci. U.S.A. 110, 12072–12077. doi: 10.1073/pnas.1222044110
Gomes, I., Gupta, A., Filipovska, J., Szeto, H. H., Pintar, J. E., and Devi, L. A. (2004). A role for heterodimerization of mu and delta opiate receptors in enhancing morphine analgesia. Proc. Natl. Acad. Sci. U.S.A. 101, 5135–5139. doi: 10.1073/pnas.0307601101
Guan, J. S., Xu, Z. Z., Gao, H., He, S. Q., Ma, G. Q., Sun, T., et al. (2005). Interaction with vesicle luminal protachykinin regulates surface expression of delta-opioid receptors and opioid analgesia. Cell 122, 619–631. doi: 10.1016/j.cell.2005.06.010
Guerrero-Alba, R., Valdez-Morales, E. E., Jimenez-Vargas, N. N., Bron, R., Poole, D., Reed, D., et al. (2018). Co-expression of mu and delta opioid receptors by mouse colonic nociceptors. Br. J. Pharmacol. 175, 2622–2634. doi: 10.1111/bph.14222
Gupta, A., Mulder, J., Gomes, I., Rozenfeld, R., Bushlin, I., Ong, E., et al. (2010). Increased abundance of opioid receptor heteromers after chronic morphine administration. Sci. Signal. 3:ra54. doi: 10.1126/scisignal.2000807
He, S. Q., Zhang, Z. N., Guan, J. S., Liu, H. R., Zhao, B., Wang, H. B., et al. (2011). Facilitation of mu-opioid receptor activity by preventing delta-opioid receptor-mediated codegradation. Neuron 69, 120–131. doi: 10.1016/j.neuron.2010.12.001
He, X., Ashbrook, A. W., Du, Y., Wu, J., Hoffmann, H. H., Zhang, C., et al. (2020). RTP4 inhibits IFN-I response and enhances experimental cerebral malaria and neuropathology. Proc. Natl. Acad. Sci. U.S.A. 117, 19465–19474. doi: 10.1073/pnas.2006492117
Hoyo-Becerra, C., Liu, Z., Yao, J., Kaltwasser, B., Gerken, G., Hermann, D. M., et al. (2015). Rapid regulation of depression-associated genes in a new mouse model mimicking interferon-alpha-related depression in hepatitis C virus infection. Mol. Neurobiol. 52, 318–329. doi: 10.1007/s12035-014-8861-z
Intlekofer, K. A., Clements, K., Woods, H., Adams, H., Suvorov, A., and Petersen, S. L. (2019). Progesterone receptor membrane component 1 inhibits tumor necrosis factor alpha induction of gene expression in neural cells. PLoS One 14:e0215389. doi: 10.1371/journal.pone.0215389
Joseph, E. K., and Levine, J. D. (2010). Mu and delta opioid receptors on nociceptors attenuate mechanical hyperalgesia in rat. Neuroscience 171, 344–350. doi: 10.1016/j.neuroscience.2010.08.035
Kiguchi, N., Kobayashi, D., Saika, F., Matsuzaki, S., and Kishioka, S. (2017). Pharmacological regulation of neuropathic pain driven by inflammatory macrophages. Int. J. Mol. Sci. 18:2296. doi: 10.3390/ijms18112296
Leung, L., and Cahill, C. M. (2010). TNF-alpha and neuropathic pain–a review. J. Neuroinflammation 7:27. doi: 10.1186/1742-2094-7-27
Mainland, J., and Matsunami, H. (2012). RAMP like proteins : RTP and REEP family of proteins. Adv. Exp. Med. Biol. 744, 75–86. doi: 10.1007/978-1-4614-2364-5_7
Nair, S. R., Abraham, R., Sundaram, S., and Sreekumar, E. (2017). Interferon regulated gene (IRG) expression-signature in a mouse model of chikungunya virus neurovirulence. J. Neurovirol. 23, 886–902. doi: 10.1007/s13365-017-0583-3
Pierre, F., Ugur, M., Faivre, F., Doridot, S., Veinante, P., and Massotte, D. (2019). Morphine-dependent and abstinent mice are characterized by a broader distribution of the neurons co-expressing mu and delta opioid receptors. Neuropharmacology 152, 30–41. doi: 10.1016/j.neuropharm.2019.03.009
Ritter, S. L., and Hall, R. A. (2009). Fine-tuning of GPCR activity by receptor-interacting proteins. Nat. Rev. Mol. Cell Biol. 10, 819–830. doi: 10.1038/nrm2803
Roy, S., Barke, R. A., and Loh, H. H. (1998). MU-opioid receptor-knockout mice: role of mu-opioid receptor in morphine mediated immune functions. Brain Res. Mol. Brain Res. 61, 190–194. doi: 10.1016/s0169-328x(98)00212-5
Rozenfeld, R., and Devi, L. A. (2007). Receptor heterodimerization leads to a switch in signaling: beta-arrestin2-mediated ERK activation by mu-delta opioid receptor heterodimers. FASEB J. 21, 2455–2465. doi: 10.1096/fj.06-7793com
Rozenfeld, R., and Devi, L. A. (2011). Exploring a role for heteromerization in GPCR signalling specificity. Biochem. J. 433, 11–18. doi: 10.1042/bj20100458
Saito, H., Kubota, M., Roberts, R. W., Chi, Q., and Matsunami, H. (2004). RTP family members induce functional expression of mammalian odorant receptors. Cell 119, 679–691. doi: 10.1016/j.cell.2004.11.021
Schiller, P. W. (2010). Bi- or multifunctional opioid peptide drugs. Life Sci. 86, 598–603. doi: 10.1016/j.lfs.2009.02.025
Schoggins, J. W., Wilson, S. J., Panis, M., Murphy, M. Y., Jones, C. T., Bieniasz, P., et al. (2011). A diverse range of gene products are effectors of the type I interferon antiviral response. Nature 472, 481–485. doi: 10.1038/nature09907
Tiwari, V., He, S. Q., Huang, Q., Liang, L., Yang, F., Chen, Z., et al. (2020). Activation of micro-delta opioid receptor heteromers inhibits neuropathic pain behavior in rodents. Pain 161, 842–855. doi: 10.1097/j.pain.0000000000001768
Ugur, M., Derouiche, L., and Massotte, D. (2018). Heteromerization modulates mu opioid receptor functional properties in vivo. Front. Pharmacol. 9:1240. doi: 10.3389/fphar.2018.01240
Wang, H. B., Zhao, B., Zhong, Y. Q., Li, K. C., Li, Z. Y., Wang, Q., et al. (2010). Coexpression of delta- and mu-opioid receptors in nociceptive sensory neurons. Proc. Natl. Acad. Sci. U.S.A. 107, 13117–13122.
Williams, D., and Devi, L. A. (2010). Escorts take the lead molecular chaperones as therapeutic targets. Prog. Mol. Biol. Transl. Sci. 91, 121–149. doi: 10.1016/s1877-1173(10)91005-3
Xu, M., Bennett, D. L. H., Querol, L. A., Wu, L. J., Irani, S. R., Watson, J. C., et al. (2020). Pain and the immune system: emerging concepts of IgG-mediated autoimmune pain and immunotherapies. J. Neurol. Neurosurg. Psychiatry 91, 177–188. doi: 10.1136/jnnp-2018-318556
Zarei Ghobadi, M., Mozhgani, S. H., Farzanehpour, M., and Behzadian, F. (2019). Identifying novel biomarkers of the pediatric influenza infection by weighted co-expression network analysis. Virol. J. 16:124.
Zhang, L., Zhang, J. T., Hang, L., and Liu, T. (2020). Mu opioid receptor heterodimers emerge as novel therapeutic targets: recent progress and future perspective. Front. Pharmacol. 11:1078. doi: 10.3389/fphar.2020.01078
Keywords: opioid, RTP4, pain, DRG, heteromer
Citation: Fujita W (2020) The Possible Role of MOPr-DOPr Heteromers and Its Regulatory Protein RTP4 at Sensory Neurons in Relation to Pain Perception. Front. Cell. Neurosci. 14:609362. doi: 10.3389/fncel.2020.609362
Received: 23 September 2020; Accepted: 28 October 2020;
Published: 13 November 2020.
Edited by:
Takayoshi Masuoka, Kanazawa Medical University, JapanReviewed by:
Dominique Mssotte, Université de Strasbourg, FranceMichelino Puopolo, Stony Brook University, United States
Copyright © 2020 Fujita. This is an open-access article distributed under the terms of the Creative Commons Attribution License (CC BY). The use, distribution or reproduction in other forums is permitted, provided the original author(s) and the copyright owner(s) are credited and that the original publication in this journal is cited, in accordance with accepted academic practice. No use, distribution or reproduction is permitted which does not comply with these terms.
*Correspondence: Wakako Fujita, wakakofu@nagasaki-u.ac.jp