- Department of Rehabilitation Medicine, The Third Affiliated Hospital, Sun Yat-sen University, Guangzhou, China
Background: Exosomes, especially stem cell-derived exosomes, have been widely studied in pre-clinical research of ischemic stroke. However, their pooled effects remain inconclusive.
Methods: Relevant literature concerning the effects of exosomes on neurological performance in a rodent model of ischemic stroke was identified via searching electronic databases, including PubMed, Embase, and Web of Science. The primary outcomes included neurological function scores (NFS) and infarct volume (IV), and the secondary outcomes were several pro-inflammatory factors and terminal deoxynucleotidyl transferase deoxyuridine triphosphate nick end labeling-positive cells. Subgroup analyses regarding several factors potentially influencing the effects of exosomes on NFS and IV were also conducted.
Results: We identified 21 experiments from 18 studies in the meta-analysis. Pooled analyses showed the positive and significant effects of exosomes on NFS (standardized mean difference −2.79; 95% confidence interval −3.81 to −1.76) and IV (standardized mean difference −3.16; 95% confidence interval −4.18 to −2.15). Our data revealed that the effects of exosomes on neurological outcomes in rodent stroke models might be related to routes of administration and exosomes sources. In addition, there was significant attenuation in pro-inflammatory factors, including interleukin-6, tumor necrosis factor-α and interleukin-1β, and terminal deoxynucleotidyl transferase deoxyuridine triphosphate nick end labeling-positive cells when undergoing exosomes treatment.
Conclusion: Cell-derived exosomes treatment demonstrated statistically significant improvements in structural and neurological function recovery in animal models of ischemic stroke. Our results also provide relatively robust evidence supporting cell-derived exosomes as a promising therapy to promote neurological recovery in stroke individuals.
Introduction
Ischemic stroke continues to be a leading cause of adult death and permanent disability throughout the world (Chen L. et al., 2016). In most instances, ischemic stroke is caused by blood-vessel occlusion, most commonly due to an embolus or local thrombosis (Dirnagl et al., 1999). Given the limitation of available therapeutic options, patients still retain long-term dysfunction after stroke, although they have received recombinant tissue plasminogen activator or endovascular intervention (Schwamm et al., 2013; Nogueira et al., 2018).
To replace the loss of the functional neurons, stem cell transplantation represents a promising treatment option (Boltze et al., 2014). Several studies have identified that transplantation of mesenchymal stem cells (MSCs) not only facilitated functional recovery in rodents after stroke but also improved neurological outcomes for post-stroke survivors (Liu et al., 2011; Levy et al., 2019). However, only a small percentage of MSCs engrafted into the ischemic hemisphere exerted neuroprotective effects (Acosta et al., 2015; Gervois et al., 2016). In addition, cell-based therapy was found with several potential risks and limitations, such as tumor formation, occlusion in the microvasculature, and weak capacity to cross the blood–brain barrier (Lukomska et al., 2019).
Emerging studies demonstrated that exosomes released from MSCs are the major biological principle underlying the therapeutic benefits of MSC transplantation (Xin et al., 2013b; Doeppner et al., 2015). Exosomes are nanosized vesicles with a size of 40 to 150 nm and secreted by different cell types. They play a pivotal role in mediating intercellular communication by delivering a variety of functional biomolecules, including messenger RNAs, microRNAs (miRNAs), long non-coding RNA, proteins, and lipids, to recipient cells (Raposo and Stoorvogel, 2013; Tkach and Théry, 2016). Until now, exosomal miRNAs have been investigated more fully than other cargo of exosomes in the light of their functional significance. Indeed, increasing data showed that exosomal miRNAs improved neurological function and promoted neurovascular remodeling in the ischemic brain (Xin et al., 2013b, 2017a). Furthermore, nanometer-sized exosomes are admitted to cross the blood–brain barrier and prevent tumor formation. Therefore, cell-derived exosomes are expected to become an efficient and safe treatment for ischemic stroke.
A considerable number of animal studies have been performed to explore the efficacy of exosomes on an ischemic stroke model, but they have various cell origins, administration routes, injection doses, and timepoints of intervention and evaluation (Song et al., 2019; Zhao et al., 2020). However, there are a few clinical trials concerning cell-derived exosomes in the treatment of ischemic stroke, and most of them are in the initial recruitment stage of the trials. To characterize and quantify the pre-clinical evidence and provide a vital theoretical basis for clinical application, we therefore aimed to conduct this meta-analysis of animal studies.
Materials and Methods
The protocol was established according to the Preferred Reporting Items for Systematic Review and Meta-Analysis guidelines (Moher et al., 2009).
Search Strategy
We conducted a literature search in the electronic database, including PubMed, Embase, and Web of Science. The search strategy was as follows: (“exosomes” or “exosome” or “exosomal” or “secretome”) and (“brain ischemia” or “cerebral ischemia” or “ischemia stroke” or “brain infarct” or “cerebral infarct” or “middle cerebral artery occlusion” or “MCAO”). All of the search strategies were performed from the initiation to June 2020, and the literature was published in English. The references of selected articles were further searched by hand to obtain additional citations.
Study Selection
Two authors (MH and ZH) independently abstracted all data from any eligible publication, according to a standard protocol. Discrepancies were resolved by discussion with the third reviewer (HZ). The inclusion criteria for this research were (1) population-rodent models with ischemic stroke (transient or permanent); (2) intervention unmodified cell-derived exosomes; (3) comparison-vehicle, phosphate-buffered saline, or positive control drug or no treatment; and (4) outcome measure—the primary outcomes were neurological function score (NFS) and infarct volume (IV). The secondary outcomes were the percentage of apoptotic cells, levels of interleukin (IL)-1β, IL-6, and tumor necrosis factor (TNF)-α. The exclusion criteria were (1) ischemic stroke was not conducted on rodent models; (2) artificially modified cell-derived exosomes; (3) repeated data or insufficient information; (4) the intervention was a combination of exosomes and another drug; and (5) clinical articles, case reports, commentary, reviews, conference abstracts, correspondence, expert opinion, and in vitro studies.
Data Extraction
The following data extraction from the included studies was conducted independently by two reviewers (MH and ZH). The following details were collected: (1) name of the first author and the publication year; (2) country or region; (3) animal sex and species; (4) number of animals in the study, control group, and exosomes treatment group; (5) comorbid status of animals; (6) method of an ischemic stroke model; (7) occlusion time of animal with ischemic stroke; (8) treatment time; (9) origins of exosome; (10) administration route of exosome; (11) administration dose of exosome; (12) measurement time; and (13) outcome measures. For included studies without available numerical values in the text, we contacted with authors twice by email and performed the WebPlotDigitizer software (https://automeris.io/WebPlotDigitizer/) to extract data from the figures or graphs (Burda et al., 2017).
Quality Assessment
The methodological quality of each eligible studies was assessed independently by two investigators (MH and ZH) via using the 10-item Collaborative Approach to Meta-Analysis and Review of Animal Data from Experimental Studies quality checklist and detailed items as follows: A, publication in a peer-reviewed journal; B, statement of temperature control; C, randomization to treatment or control group; D, blinding of the ischemic model establishment; E, blinding of outcome assessment; F, anesthetic without obvious intrinsic vascular protection activity; G, an appropriate animal model such as advanced age, diabetic, or hypertensive; H, estimation of the sample size; I, compliance with animal welfare regulations; and J, declaration of potential conflicts of interest (Zhao et al., 2020).
Effect Sizes Estimation
The effect size was calculated using standardized mean difference (SMD) with 95% confidence intervals (95% CIs) for continuous outcomes because a single outcome measure was assessed and reported across trials using different measurement tools. For quantitative synthesis, the pooled effect estimation was calculated by comparing the change from baseline with the endpoint of the study between the intervention group and the control group.
The primary outcome was served to assess functional and structural recovery, which included NFSs and IV. If more than one measure for NFS were used in an individual trial, a modified neurological severity score was considered as a priority outcome measure because it is more appropriate to reflect neurological impairment; otherwise, neurological severity score and various behavioral tests (adhesive removal test, foot-fault test). We choose the 2,3,5-triphenyl tetrazolium chloride method as IV assessment after considering MRI and cresyl violet staining. Several pro-inflammatory factors, such as IL-6, TNF-α, and IL-1β, and the percentage of terminal deoxynucleotidyl transferase deoxyuridine triphosphate nick end labeling-positive cells, were detected as the secondary outcomes. Besides, several subgroup analyses were conducted to investigate whether study characteristics (administration routes, type of ischemia, exosomes sources, intervention time, and different species) had the effects on exosomes in improving neurological performance via using random-effects models. The authors were contacted twice by email for original data if the published study data were insufficient for data analyses.
Statistical Analysis
All statistical analyses were performed by Review Manager 5.3 (The Cochrane Collaboration, Oxford, UK) and Stata version 14.0 (Stata Corp, College Station, TX, USA). We used the SMD with the 95% CI to record the continuous outcomes. Statistical heterogeneity was analyzed by using the I-square test. A random-effects model test was performed when heterogeneity was significant (I2 > 50%). Subgroup and sensitivity were conducted to investigate the origin of heterogeneity. A value of p < 0.05 was considered statistically significant.
Results
Search Results and Study Characteristics
The process of study selection is outlined in Figure 1. A total of 18 studies with 21 comparisons satisfied the inclusion criteria (Xin et al., 2013a, 2017a,b; Chen K. H. et al., 2016; Jiang et al., 2018; Deng et al., 2019; Li et al., 2019; Nalamolu et al., 2019a,b; Pei et al., 2019; Song et al., 2019; Sun et al., 2019; Venkat et al., 2019; Zheng et al., 2019; Li G. et al., 2020; Ling et al., 2020; Safakheil and Safakheil, 2020; Zhao et al., 2020). The characteristics of the included studies are presented in Table 1. Moreover, the Preferred Reporting Items for Systematic Review and Meta-Analysis flow diagram was showed in Supplementary Table 1. All these studies were published between 2013 and 2020, and 367 animals were included in this meta-analysis. Among the included studies, six of them used bone marrow mesenchymal stromal cells—exosome (Xin et al., 2013a, 2017a,b; Deng et al., 2019; Safakheil and Safakheil, 2020; Zhao et al., 2020), one adopted adipose-derived mesenchymal stem cells—exosome (Chen K. H. et al., 2016), one applied human umbilical cord mesenchymal stem cells—exosome (Li G. et al., 2020), one used human umbilical cord blood mesenchymal stromal cells—exosome (Nalamolu et al., 2019b), one used adipose-derived stem cells—exosome(Jiang et al., 2018), one used neural stem cells—exosome (Sun et al., 2019), one used human urine-derived stem cells—exosome (Ling et al., 2020), one used mouse brain endothelial cells—exosome (Venkat et al., 2019), one used macrophages—exosome (Zheng et al., 2019), one used microglia—exosome, and one used astrocytes—exosome (Pei et al., 2019; Song et al., 2019), and the two remaining used another origin of exosomes (Li et al., 2019; Nalamolu et al., 2019a).
The ischemic stroke model was established with a unifilar nylon suture (Xin et al., 2013a, 2017a,b; Chen K. H. et al., 2016; Jiang et al., 2018; Deng et al., 2019; Nalamolu et al., 2019a,b; Pei et al., 2019; Song et al., 2019; Sun et al., 2019; Zheng et al., 2019; Li G. et al., 2020; Ling et al., 2020; Safakheil and Safakheil, 2020; Zhao et al., 2020), bipolar electrocoagulation forceps (Li et al., 2019), or thrombus (Venkat et al., 2019). A total of 13 studies used the transient ischemic stroke model (Xin et al., 2013a, 2017a,b; Chen K. H. et al., 2016; Deng et al., 2019; Nalamolu et al., 2019a,b; Song et al., 2019; Sun et al., 2019; Zheng et al., 2019; Ling et al., 2020; Safakheil and Safakheil, 2020; Zhao et al., 2020), and four studies used the permanent model (Jiang et al., 2018; Li et al., 2019; Pei et al., 2019; Venkat et al., 2019). Most studies chose to perform exosomes injection immediately after the injury model was established (Jiang et al., 2018; Li et al., 2019; Nalamolu et al., 2019a,b; Song et al., 2019; Zheng et al., 2019; Li G. et al., 2020), and several studies reported the same dose of exosomes (100 μg) injected intravenously (Xin et al., 2013a, 2017a,b; Chen K. H. et al., 2016; Safakheil and Safakheil, 2020). A variety of miRNA types have been reported in exosomes, such as miR-17-92 (Xin et al., 2017a), miR-126 (Venkat et al., 2019), miR-124 (Song et al., 2019), miR-26a (Ling et al., 2020), miR-26b-5p (Li G. et al., 2020), miR-30d-5p (Jiang et al., 2018), and miR-138-5p (Deng et al., 2019).
Quality Assessment
The median quality score across the 18 studies was 5.5, and the range was from 4 to 7. The most widely met criteria included peer-reviewed publications (100% of studies), appropriate animal model (100% of studies), statement of compliance with animal welfare regulations (100% of studies), and statement of potential conflict of interests (100% of studies). Moreover, 72.22% of the included studies were randomly divided into the treatment and control groups, whereas the randomization was not mentioned in the remaining studies (27.78%). However, the calculation of sample size, the blinded induction of stroke model, and the blinded assessment of the experimental outcome had not been reported in all included studies. Further details of the study quality score are presented in Table 2.
Primary Outcomes
Neurological Function Scores
Fourteen studies with 17 comparisons reported the NFSs. The pooled analysis showed that exosomes can significantly improve the neurological function compared with the control (SMD −2.79; 95% CI −3.81 to −1.76; p < 0.001; I2 = 91.3%; Figure 2A). The subgroup analysis showed that all cell-derived exosomes are effective in improving neurological function (Figure 2B). Moreover, the subgroup analysis showed that all administrational routes help improve the neurological function, except for internal jugular vein administration (Figure 2C). In addition, the subgroup analysis presented that immediate or delayed treatment with cell-derived exosomes could improve the neural recovery after transient or permanent stroke (Supplementary Figures 1A,B; Supplementary Table 2). The sensitivity analysis showed that none of the single studies significantly influenced the result (Supplementary Figure 1C).
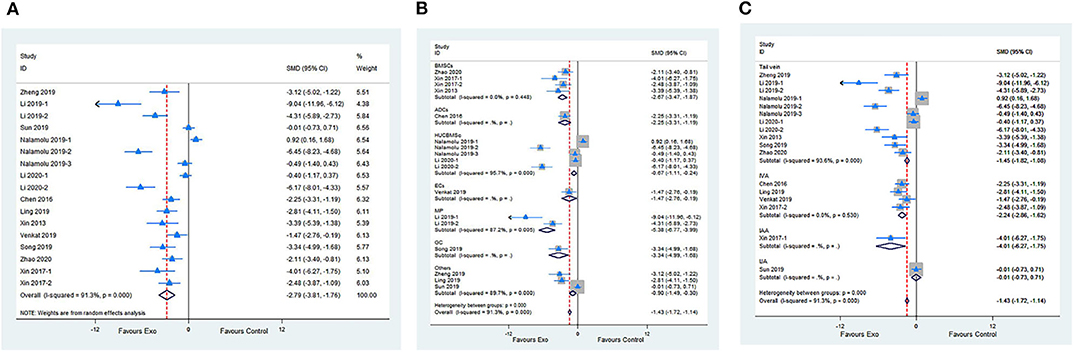
Figure 2. (A) Forest plot shows the efficacy of exosomes in improving the neurological function in the ischemic stroke model. (B) Forest plot shows the efficacy of exosomes derived from various cells in improving the neurological function in the ischemic stroke model. (C) Forest plot shows the efficacy of exosomes via different administrational routes in improving the neurological function in the ischemic stroke model.
Infarct Volume
Meta-analysis of 15 studies with 18 comparisons showed significant effects of exosomes for reducing IV compared with control groups (SMD −3.16; 95% CI −4.18 to −2.15; p < 0.001; I2 = 90.8%; Figure 3A). The subgroup analysis presented that all cell-derived exosomes benefit from reducing IV except for mouse brain endothelial cells (Figure 3B). Moreover, the subgroup analysis showed that all administrational routes effectively reduce IV (Figure 3C). In addition, the subgroup analysis indicated that immediate or delayed treatment with cell-derived exosomes reduced IV after transient or permanent stroke (Supplementary Figures 2A,B; Supplementary Table 2). None of the single studies significantly influenced the result via sensitivity analysis (Supplementary Figure 2C).
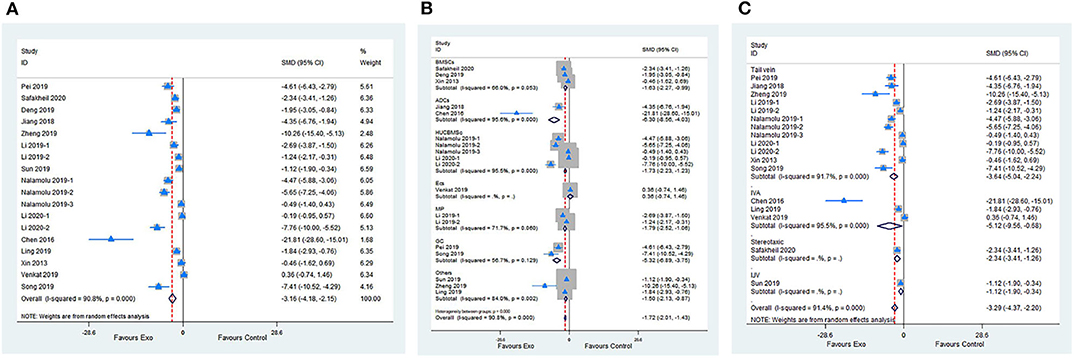
Figure 3. (A) Forest plot shows the efficacy of exosomes in reducing infarct volume in the ischemic stroke model. (B) Forest plot shows the efficacy of exosomes derived from various cells in reducing infarct volume in the ischemic stroke model. (C) Forest plot shows the efficacy of exosomes via different administrational routes in reducing infarct volume in the ischemic stroke model.
Secondary Outcomes
Five studies evaluated the anti-inflammatory effect of exosomes by measuring IL-6 levels compared with the control. Meta-analysis presented a significant reduction in the exosome group (SMD −2.9; 95% CI −4.58 to −1.22; p < 0.001; I2 = 85.8%; Figure 4A). A meta-analysis of seven studies with eight comparisons indicated that the level of TNF-α was significantly decreased in the exosome group compared with the control group (SMD −3.10; 95% CI −3.94 to −2.26; p < 0.05; I2 = 52.9%; Figure 4B). Moreover, four studies with five comparisons assessed the IL-1β level. Meta-analysis showed a significant decrease in the exosome treatment group (SMD −2.29; 95% CI −3.97 to −0.61; p < 0.001; I2 = 85.1%; Figure 4C). A meta-analysis of four studies showed a significant reduction of terminal deoxynucleotidyl transferase deoxyuridine triphosphate nick end labeling-positive cell percentage between the exosome group and the control group (SMD −3.20; 95% CI −4.29 to −2.1; I2 = 47.8%; p = 0.125; Figure 4D; Supplementary Table 2).
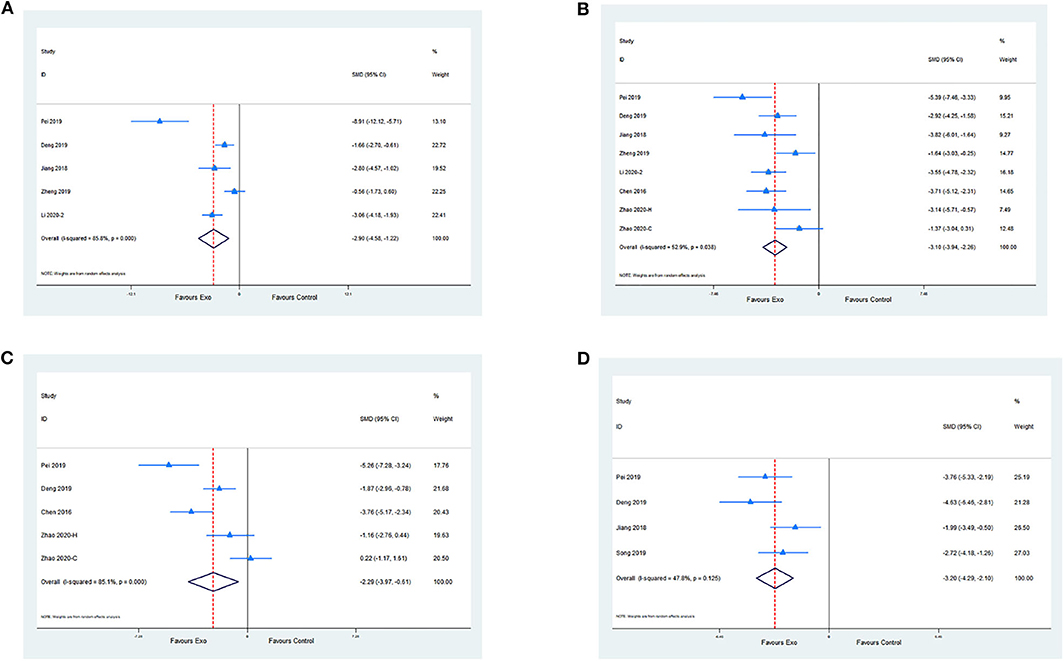
Figure 4. (A) Pooled estimate of exosomes on anti-inflammation, according to IL-6. (B) Pooled estimate of exosomes on anti-inflammation, according to TNF-α. (C) Pooled estimate of exosomes on anti-inflammation according to IL-1β. (D) Pooled estimate of exosomes on anti-apoptosis according to terminal deoxynucleotidyl transferase deoxyuridine triphosphate nick end labeling-positive cells.
Publication Bias
The funnel plot was conducted to examine publication bias for the primary outcomes. Potential publication bias of primary outcomes was tested using Egger's test (p < 0.0001; Figures 5A,B). The publication bias was more likely to generate from different types of exosome applications or inconsistent measurements. Hence, we performed several subgroup analyses to figure out the potential sources of publication bias, which are described above in primary outcomes.
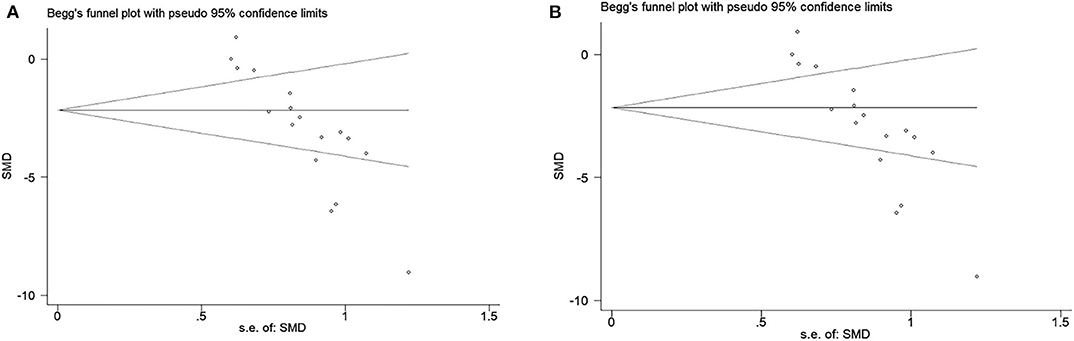
Figure 5. (A) Beggs funnel plot with neural function score. (B) Beggs funnel plot with infarct volume.
Discussion
To the best of our knowledge, this is the first meta-analysis to systematically describe ischemic stroke with unmodified cell-derived exosomes treatment. A meta-analysis of 18 studies with 21 comparisons presented a comprehensive summary of the pooled effect of cell-derived exosomes on rodents with ischemic stroke. The available evidence from our included studies indicated that stem cell-derived exosomes substantially improved neurological function and reduced IV in a rodent with cerebral ischemia. Moreover, pooled analyses demonstrated that exosome therapy not only ameliorates the inflammatory response but also reduces cell apoptosis after ischemic stroke. Therefore, our meta-analysis provided crucial clues for human clinical trials on exosomes and ischemic stroke.
A previous meta-analysis evaluated the efficacy of extracellular vesicles therapy for stroke (Thomas et al., 2020). Both modified and unmodified cell-derived extracellular vesicles were included to explore their benefits in ischemic and hemorrhagic stroke in this publication, whereas it was tough to identify the real therapeutic effects induced by extracellular vesicles or signaling molecule added artificially. In contrast, we specifically investigated the effects of unmodified cell-derived exosomes on an ischemic stroke to reduce the sources of heterogeneity and improve the overall quality of the included studies. Moreover, our meta-analysis further evaluated the effect of exosomes on pro-inflammatory cytokines and cell apoptosis, providing useful information for further laboratory studies and clinical trials.
In the current study, the subgroup analysis showed that all administrational routes helped improve the neurological function, except for internal jugular vein administration. The result that internal jugular vein administration is not effective might be relevant to the short interval between exosome intervention (2 h) and functional assessment (24 h) and/or the relative insensitivity of a four-point test using neurologic deficit score. Besides, the organ sequestration of circulating exosomes injected intravenously or inadequate dosing may be contributed to this negative result (Sun et al., 2019). In this meta-analysis, 12 included studies applied to the rats, whereas nine studies used mice. The subgroup analysis showed that either mice or rats have significant and positive effects on IVs and neurological scores (Supplementary Figure 3), but the effects of exosomes on IV between-group difference did reach statistical significance (QB = 7.38, P = 0.007), which demonstrated in terms of reducing IVs, a larger treatment effect of exosomes on rats than mice.
Remarkably, all studies were performed on male rodents. Indeed, hormone and sex hormone-independent mechanisms played a central role in regulating sex differences in stroke (Wilson, 2013). Immune response, a part of sex hormone-independent mechanisms, is a vital factor in determining the difference for males and females to respond to experimental stroke (Dotson and Offner, 2017). A study reported by Dotson et al. revealed that splenectomy before middle cerebral artery occlusion significantly decreased the peripheral macrophages/monocytes and activated T cells in male C57BL/6J mice but not female, which resulted in attenuation of neurological impairment in male mice but not female (Dotson et al., 2015).
Exosomes, endosome-derived nanosized membrane vesicles, are released by most cell types. Moreover, exosomes are vital carriers for regulating angiogenesis, neurogenesis, inflammation, and cell apoptosis (Deng et al., 2019; Tian et al., 2019; Ling et al., 2020; Zhao et al., 2020). Emerging studies demonstrated that stem cell transplantation improved neurological recovery after ischemic stroke, and a previous meta-analysis showed that stem cell therapy was safe and effective in animals and humans with cerebral ischemia (Xin et al., 2013a). More importantly, recent studies reported no significant difference in efficacy between stem cell treatment and stem cell-derived exosomes in a rodent model with ischemic stroke. Stem cell-derived exosomes take part in cell-to-cell communication and are considered as the paracrine effectors of cell-based therapy by transferring a great deal of signaling factors including proteins, lipids, mRNA, and non-coding RNAs (such as miRNAs) (Liang et al., 2014; Xin et al., 2014). Compared with cell-based treatment, cell-derived exosomes have lower immunogenicity. In addition, exosome administration could reduce or avoid some of the risks related to cell translation, such as tumorigenesis and cytokine release syndrome (Phinney and Pittenger, 2017). Thus, exosomes seem to be a promising therapy for the recovery of ischemic stroke.
Increasing studies have demonstrated that RNAs derived from exosomes are the critical component for their therapeutic effect. As the content of exosomes, miRNAs are ~22 nucleotides in length and function as non-coding RNAs regulating the level of protein expression by modulating mRNA translation (Ha and Kim, 2014). The miRNAs participate in post-transcriptional regulation of gene expressions typically by binding to the 3′-untranslated region of mRNA sequences (Rupaimoole and Slack, 2017). Given the dramatic effects of miRNA, exosomal miRNAs have gained more attention than proteins and lipids. More and more studies demonstrated that the exosomal miRNAs were involved in the processes of neurological recovery and neural remodeling after stroke (Xin et al., 2017a; Zhang et al., 2019).
In our included studies, there are numerous miRNAs correlated with the neuroprotective effects of exosomes, such as miR-17-92 (Xin et al., 2017a), miR-126 (Venkat et al., 2019), miR-124 (Song et al., 2019), miR-26a (Ling et al., 2020), miR-26b-5p (Li G. et al., 2020), miR-30d-5p (Jiang et al., 2018), and miR-138-5p (Deng et al., 2019). For example, miR-17-92 contained in exosomes enhanced neural plasticity and improved neurological function after stroke, possibly via downregulating PTEN expression and subsequent activating its downstream proteins, the PI3K/Akt/mTOR/GSK-3β signaling pathway (Xin et al., 2017a). Song et al. (2019) demonstrated that M2 microglia-derived exosomes alleviated ischemia-reperfusion injury and protected neuronal survival, and the underlying mechanism might be relevant to exosomal miR-124 and its downstream target ubiquitin-specific protease 14. Moreover, a study reported by Venkat et al. (2019) demonstrated that endothelial cells-Exo promoted capillary tube formation and improved axonal remodeling. However, the downregulation of miR-126 in endothelial cells-Exo reduced Exo-afforded effects of capillary tube formation and axonal regeneration. Thus, it means that exosomal miRNAs play a key role in the neuroprotective function and neural recovery after stroke.
Inflammation is widely involved in the pathogenesis of numerous cerebrovascular disorders, such as ischemic stroke and intracerebral hemorrhage (Kleinig and Vink, 2009), leading to secondary injury to the brain. Increasing evidence demonstrated that microglia-modulated inflammation actively played a part in cerebral infarction (Cai et al., 2017). When ischemic stroke occurred, microglia were polarized toward the M1 phenotype, which produces multiple pro-inflammatory cytokines, including IL-6, TNF-α, and IL-1β, thus aggravating neurofunctional impairment (Tobin et al., 2014). In contrast, the microglia M2 phenotype promotes the secretion of anti-inflammatory cytokines, such as IL-4 and IL-10, thus alleviating neuroinflammation and improving neuronal survival (Schmieder et al., 2012). Li et al. reported that the pro-inflammatory cytokines TNF-α, IL-1β, and IL-6 in the brain tissue were significantly elevated in the rats with ischemic stroke (Li S. et al., 2020).
In this study, six included studies demonstrated that exosome treatment significantly decreased the production of pro-inflammatory factors in brain tissue after cerebral ischemia (Chen K. H. et al., 2016; Deng et al., 2019; Pei et al., 2019; Zheng et al., 2019; Li G. et al., 2020; Zhao et al., 2020). On the other hand, Zhao et al. found that exosome treatment promoted the secretion of anti-inflammatory cytokines (TGF-β and IL-10) in the ischemic hemisphere and repressed microglia M1 polarization to inhibit microglial inflammation via regulating the CysLT 2R-ERK1/2 pathway (Zhao et al., 2020). Moreover, a study reported by Jiang et al. demonstrated that exosomes secreted from adipose-derived stem cells upregulated the expression of anti-inflammatory cytokines (IL-4 and IL-10) and enhanced M2 microglia/macrophage polarization (Jiang et al., 2018). Therefore, exosomes exert a potential neuroprotective effect partially through attenuating brain inflammation.
The ischemic core and penumbra are two separate vital areas of the brain during ischemic stroke. The ischemic core experiences an extreme and rapid decrease of blood flow with irreversible cell death a few minutes after cerebral ischemia. However, apoptosis within the penumbra may appear after several hours or days with reversible cell death (Bandera et al., 2006). Thus, apoptosis is another essential part of the pathogenesis of ischemic stroke, and inhibition of apoptosis could alleviate cerebral injury in stroke models (Uzdensky, 2019). Our meta-analysis demonstrated that cell-derived exosomes contributed to the reduction of cell apoptosis. Song et al. indicated that treatment with M2 microglia-derived exosomes greatly attenuated apoptotic neurons and protected from ischemia-reperfusion injury after stroke compared with phosphate-buffered saline treatment (Song et al., 2019). Moreover, Pei et al. (2019) demonstrated that astrocyte-derived exosomes significantly decreased middle cerebral artery occlusion that induced neuron apoptosis via inhibiting autophagy in experimental ischemic stroke. Additional research reported by Xin et al. (2017a) demonstrated that exosomes not only promoted neurogenesis and oligodendrogenesis but also improved neuronal dendrite plasticity in the ischemic boundary zone, which is possibly related to the PI3K/Akt/mTOR/GSK-3β signaling pathway. Besides, endothelial cell-derived exosomes significantly increased artery diameter and vessel density in the ischemic boundary zone compared with stroke mice without any treatment (Venkat et al., 2019).
Exosomes are emerging as essential intercellular carriers in exerting neurorestorative effects after stroke (Tian et al., 2018; Song et al., 2019). The present meta-analysis result showed that both immediate and delayed treatments with cell-derived exosomes provide therapeutic benefits. Moreover, almost all cell-derived exosomes are effective in improving neurological recovery. However, there are multiple translational challenges needed to be solved before the clinical application of cell-derived exosomes. Firstly, the approach of isolation and storage may have an impact on the characteristics and quality of exosomes (Maroto et al., 2017). Appropriate methods of isolation and storage need to be standardized. Secondly, it is vital to develop the optimal methods to produce a sufficient quantity of exosomes to meet the clinical requirement. Thirdly, in most studies, the follow-up time was <1 month. Thus, the long-term effects of exosomes are a pivotal challenge that needs to be further explored. Fourthly, dose-response and therapeutic window researches are required before the clinical application.
Our study has several potential limitations. First, the earliest start time of intervention available in large human clinical trials was a median of 45 min after ischemic stroke onset (Saver et al., 2015). However, there were ten animal experiments adopting exosome therapy immediately after brain ischemia, which may lead to the overestimation of exosome intervention effects achievable. Second, the ischemic stroke model was performed on young, healthy male rodents in most of the included studies. Nevertheless, ischemic stroke usually occurs in elderly male or female individuals with several risk factors of cerebrovascular diseases (such as hypertension, hyperlipemia, and diabetes), indicating that the heterogenicity of the post-stroke patient requires more complicated interventions. Third, it is important to realize that scaling the production of exosomes for human clinical trials would be required. The general consensus on exosome isolation, purification, and normalization procedures would overcome the crucial bottlenecks in translational studies. Finally, several limitations for this meta-analysis itself should be considered. On the one hand, the heterogeneity between the included studies cannot be evidently decreased, even if we performed subgroup and sensitivity analyses. On the other hand, we performed the data extraction from graphics using WebPlotDigitizer software, which may alter the original data and affect the results.
Conclusion
Pooled data from the present meta-analysis demonstrated that cell-derived exosomes improve neurological function and reduce IV in rodent models of ischemic stroke. Furthermore, we found that cell-derived exosomes have potential neuroprotective effects, which were mainly mediated through anti-inflammation and anti-apoptosis. Despite the fact that some factors, such as publication bias and study quality, may affect the validity of positive results, this meta-analysis provides important clues to translate new therapeutic options for cerebral ischemia.
Data Availability Statement
The original contributions generated for the study are included in the article/Supplementary Materials, further inquiries can be directed to the Corresponding author.
Author Contributions
MH and ZH carried out the design, collected the data, performed the statistical analyses, and drafted the manuscript. CX and LL assisted with statistical analyses and drafted the manuscript. LC, SC, and TL participated in the collection of data. HZ conceived the design of the study and contributed to draft the manuscript. All authors contributed to the article and approved the submitted version.
Funding
This work was supported by grants from the National Natural Science Foundation of China (81972151 and 81572228) and the Guangdong Basic and Applied Basic Research Foundation (2019A1515011106).
Conflict of Interest
The authors declare that the research was conducted in the absence of any commercial or financial relationships that could be construed as a potential conflict of interest.
Supplementary Material
The Supplementary Material for this article can be found online at: https://www.frontiersin.org/articles/10.3389/fncel.2020.593130/full#supplementary-material
Supplementary Figure 1. (A) The forest plot shows the efficacy of exosomes via different administrational time in improving the neurological function in the ischemic stroke model. (B) The forest plot shows the efficacy of exosomes to different types of stroke in improving the neurological function in the ischemic stroke model. (C) The sensitivity analysis of included studies in neurological function scores.
Supplementary Figure 2. (A) The forest plot shows the efficacy of exosomes via different administrational time in reducing infarct volume in the ischemic stroke model. (B) The forest plot shows the efficacy of exosomes to different types of stroke in reducing infarct volume in the ischemic stroke model. (C) The sensitivity analysis of included studies in infarct volume.
Supplementary Figure 3. (A) The forest plot shows the efficacy of exosomes in improving the neurological function in rats and mice with ischemic stroke. (B) The forest plot shows the efficacy of exosomes in reducing infarct volume in rats and mice with ischemic stroke.
Supplementary Table 1. PRISMA 2009 checklist.
Supplementary Table 2. Meta-analytic results from included studies.
References
Acosta, S. A., Tajiri, N., Hoover, J., Kaneko, Y., and Borlongan, C. V. (2015). Intravenous bone marrow stem cell grafts preferentially migrate to spleen and abrogate chronic inflammation in stroke. Stroke 46, 2616–2627. doi: 10.1161/STROKEAHA.115.009854
Bandera, E., Botteri, M., Minelli, C., Sutton, A., Abrams, K. R., and Latronico, N. (2006). Cerebral blood flow threshold of ischemic penumbra and infarct core in acute ischemic stroke: a systematic review. Stroke 37, 1334–1339. doi: 10.1161/01.STR.0000217418.29609.22
Boltze, J., Lukomska, B., and Jolkkonen, J. (2014). Mesenchymal stromal cells in stroke: improvement of motor recovery or functional compensation? J. Cereb. Blood Flow Metab. 34, 1420–1421. doi: 10.1038/jcbfm.2014.94
Burda, B. U., O'Connor, E. A., Webber, E. M., Redmond, N., and Perdue, L. A. (2017). Estimating data from figures with a web-based program: considerations for a systematic review. Res. Synth. Methods 8, 258–262. doi: 10.1002/jrsm.1232
Cai, J., Xu, D., Bai, X., Pan, R., Wang, B., Sun, S., et al. (2017). Curcumin mitigates cerebral vasospasm and early brain injury following subarachnoid hemorrhage via inhibiting cerebral inflammation. Brain Behav. 7:e00790. doi: 10.1002/brb3.790
Chen, K. H., Chen, C. H., Wallace, C. G., Yuen, C. M., Kao, G. S., Chen, Y. L., et al. (2016). Intravenous administration of xenogenic adipose-derived mesenchymal stem cells (ADMSC) and ADMSC-derived exosomes markedly reduced brain infarct volume and preserved neurological function in rat after acute ischemic stroke. Oncotarget 7, 74537–74556. doi: 10.18632/oncotarget.12902
Chen, L., Zhang, G., Khan, A. A., Guo, X., and Gu, Y. (2016). Clinical efficacy and meta-analysis of stem cell therapies for patients with brain ischemia. Stem Cells Int. 2016:6129579. doi: 10.1155/2016/6129579
Deng, Y., Chen, D., Gao, F., Lv, H., Zhang, G., Sun, X., et al. (2019). Exosomes derived from microRNA-138-5p-overexpressing bone marrow-derived mesenchymal stem cells confer neuroprotection to astrocytes following ischemic stroke via inhibition of LCN2. J. Biol. Eng. 13:71. doi: 10.1186/s13036-019-0193-0
Dirnagl, U., Iadecola, C., and Moskowitz, M. A. (1999). Pathobiology of ischaemic stroke: an integrated view. Trends Neurosci. 22, 391–397. doi: 10.1016/S0166-2236(99)01401-0
Doeppner, T. R., Herz, J., Görgens, A., Schlechter, J., Ludwig, A. K., Radtke, S., et al. (2015). Extracellular vesicles improve post-stroke neuroregeneration and prevent postischemic immunosuppression. Stem Cells Transl. Med. 4, 1131–1143. doi: 10.5966/sctm.2015-0078
Dotson, A. L., and Offner, H. (2017). Sex differences in the immune response to experimental stroke: implications for translational research. J. Neurosci. Res. 95, 437–446. doi: 10.1002/jnr.23784
Dotson, A. L., Wang, J., Saugstad, J., Murphy, S. J., and Offner, H. (2015). Splenectomy reduces infarct volume and neuroinflammation in male but not female mice in experimental stroke. J. Neuroimmunol. 278, 289–298. doi: 10.1016/j.jneuroim.2014.11.020
Gervois, P., Wolfs, E., Ratajczak, J., Dillen, Y., Vangansewinkel, T., Hilkens, P., et al. (2016). Stem Cell-Based Therapies for ischemic stroke: preclinical results and the potential of imaging-assisted evaluation of donor cell fate and mechanisms of brain regeneration. Med. Res. Rev 36, 1080–1126. doi: 10.1002/med.21400
Ha, M., and Kim, V. N. (2014). Regulation of microRNA biogenesis. Nat. Rev. Mol. Cell Biol. 15, 509–524. doi: 10.1038/nrm3838
Jiang, M., Wang, H., Jin, M., Yang, X., Ji, H., Jiang, Y., et al. (2018). Exosomes from MiR-30d-5p-ADSCs reverse acute ischemic stroke-induced, autophagy-mediated brain injury by promoting m2 microglial/macrophage polarization. Cell. Physiol. Biochem. 47, 864–878. doi: 10.1159/000490078
Kleinig, T. J., and Vink, R. (2009). Suppression of inflammation in ischemic and hemorrhagic stroke: therapeutic options. Curr. Opin. Neurol. 22, 294–301. doi: 10.1097/WCO.0b013e32832b4db3
Levy, M. L., Crawford, J. R., Dib, N., Verkh, L., Tankovich, N., and Cramer, S. C. (2019). Phase I/II study of safety and preliminary efficacy of intravenous allogeneic mesenchymal stem cells in chronic stroke. Stroke 50, 2835–2841. doi: 10.1161/STROKEAHA.119.026318
Li, G., Xiao, L., Qin, H., Zhuang, Q., Zhang, W., Liu, L., et al. (2020). Exosomes-carried microRNA-26b-5p regulates microglia M1 polarization after cerebral ischemia/reperfusion. Cell Cycle 19, 1022–1035. doi: 10.1080/15384101.2020.1743912
Li, S., Lu, Y., Ding, D., Ma, Z., Xing, X., Hua, X., et al. (2020). Fibroblast growth factor 2 contributes to the effect of salidroside on dendritic and synaptic plasticity after cerebral ischemia/reperfusion injury. Aging 12, 10951–10968. doi: 10.18632/aging.103308
Li, Y., Ren, C., Li, H., Jiang, F., Wang, L., Xia, C., et al. (2019). Role of exosomes induced by remote ischemic preconditioning in neuroprotection against cerebral ischemia. Neuroreport 30, 834–841. doi: 10.1097/WNR.0000000000001280
Liang, X., Ding, Y., Zhang, Y., Tse, H. F., and Lian, Q. (2014). Paracrine mechanisms of mesenchymal stem cell-based therapy: current status and perspectives. Cell Transplant 23, 1045–1059. doi: 10.3727/096368913X667709
Ling, X., Zhang, G., Xia, Y., Zhu, Q., Zhang, J., Li, Q., et al. (2020). Exosomes from human urine-derived stem cells enhanced neurogenesis via miR-26a/HDAC6 axis after ischaemic stroke. J. Cell. Mol. Med. 24, 640–654. doi: 10.1111/jcmm.14774
Liu, Z., Li, Y., Zhang, R. L., Cui, Y., and Chopp, M. (2011). Bone marrow stromal cells promote skilled motor recovery and enhance contralesional axonal connections after ischemic stroke in adult mice. Stroke 42, 740–744. doi: 10.1161/STROKEAHA.110.607226
Lukomska, B., Stanaszek, L., Zuba-Surma, E., Legosz, P., Sarzynska, S., and Drela, K. (2019). Challenges and controversies in human mesenchymal stem cell therapy. Stem Cells Int. 2019:9628536. doi: 10.1155/2019/9628536
Maroto, R., Zhao, Y., Jamaluddin, M., Popov, V. L., Wang, H., Kalubowilage, M., et al. (2017). Effects of storage temperature on airway exosome integrity for diagnostic and functional analyses. J. Extracell. Vesicles 6:1359478. doi: 10.1080/20013078.2017.1359478
Moher, D., Liberati, A., Tetzlaff, J., and Altman, D. G. (2009). Preferred reporting items for systematic reviews and meta-analyses: the PRISMA statement. PLoS Med. 6:e1000097. doi: 10.1371/journal.pmed.1000097
Nalamolu, K. R., Venkatesh, I., Mohandass, A., Klopfenstein, J. D., Pinson, D. M., Wang, D. Z., et al. (2019a). Exosomes secreted by the cocultures of normal and oxygen-glucose-deprived stem cells improve post-stroke outcome. Neuromolecular Med. 21, 529–539. doi: 10.1007/s12017-019-08540-y
Nalamolu, K. R., Venkatesh, I., Mohandass, A., Klopfenstein, J. D., Pinson, D. M., Wang, D. Z., et al. (2019b). Exosomes treatment mitigates ischemic brain damage but does not improve post-stroke neurological outcome. Cell. Physiol. Biochem. 52, 1280–1291. doi: 10.33594/000000090
Nogueira, R. G., Jadhav, A. P., Haussen, D. C., Bonafe, A., Budzik, R. F., Bhuva, P., et al. (2018). Thrombectomy 6 to 24 hours after stroke with a mismatch between deficit and infarct. N. Engl. J. Med. 378, 11–21. doi: 10.1056/NEJMoa1706442
Pei, X., Li, Y., Zhu, L., and Zhou, Z. (2019). Astrocyte-derived exosomes suppress autophagy and ameliorate neuronal damage in experimental ischemic stroke. Exp. Cell Res. 382:111474. doi: 10.1016/j.yexcr.2019.06.019
Phinney, D. G., and Pittenger, M. F. (2017). Concise review: MSC-derived exosomes for cell-free therapy. Stem Cells 35, 851–858. doi: 10.1002/stem.2575
Raposo, G., and Stoorvogel, W. (2013). Extracellular vesicles: exosomes, microvesicles, and friends. J. Cell Biol 200, 373–383. doi: 10.1083/jcb.201211138
Rupaimoole, R., and Slack, F. J. (2017). MicroRNA therapeutics: towards a new era for the management of cancer and other diseases. Nat. Rev. Drug Discov. 16, 203–222. doi: 10.1038/nrd.2016.246
Safakheil, M., and Safakheil, H. (2020). The effect of exosomes derived from bone marrow stem cells in combination with rosuvastatin on functional recovery and neuroprotection in rats after ischemic stroke. J. Mol. Neurosci. 70, 724–737. doi: 10.1007/s12031-020-01483-1
Saver, J. L., Starkman, S., Eckstein, M., Stratton, S. J., Pratt, F. D., Hamilton, S., et al. (2015). Prehospital use of magnesium sulfate as neuroprotection in acute stroke. N. Engl. J. Med. 372, 528–536. doi: 10.1056/NEJMoa1408827
Schmieder, A., Michel, J., Schönhaar, K., Goerdt, S., and Schledzewski, K. (2012). Differentiation and gene expression profile of tumor-associated macrophages. Semin. Cancer Biol. 22, 289–297. doi: 10.1016/j.semcancer.2012.02.002
Schwamm, L. H., Ali, S. F., Reeves, M. J., Smith, E. E., Saver, J. L., Messe, S., et al. (2013). Temporal trends in patient characteristics and treatment with intravenous thrombolysis among acute ischemic stroke patients at Get With The Guidelines-Stroke hospitals. Circ. Cardiovasc. Qual. Outcomes 6, 543–549. doi: 10.1161/CIRCOUTCOMES.111.000095
Song, Y., Li, Z., He, T., Qu, M., Jiang, L., Li, W., et al. (2019). M2 microglia-derived exosomes protect the mouse brain from ischemia-reperfusion injury via exosomal miR-124. Theranostics 9, 2910–2923. doi: 10.7150/thno.30879
Sun, X., Jung, J. H., Arvola, O., Santoso, M. R., Giffard, R. G., Yang, P. C., et al. (2019). Stem cell-derived exosomes protect astrocyte cultures from in vitro ischemia and decrease injury as post-stroke intravenous therapy. Front. Cell. Neurosci. 13:394. doi: 10.3389/fncel.2019.00394
Thomas, J. M., Cunningham, C. J., Lawrence, C. B., Pinteaux, E., and Allan, S. M. (2020). Therapeutic potential of extracellular vesicles in preclinical stroke models: a systematic review and meta-analysis. BMJ Open Sci. 4:e100047. doi: 10.1136/bmjos-2019-100047
Tian, T., Zhang, H. X., He, C. P., Fan, S., Zhu, Y. L., Qi, C., et al. (2018). Surface functionalized exosomes as targeted drug delivery vehicles for cerebral ischemia therapy. Biomaterials 150, 137–149. doi: 10.1016/j.biomaterials.2017.10.012
Tian, Y., Zhu, P., Liu, S., Jin, Z., Li, D., Zhao, H., et al. (2019). IL-4-polarized BV2 microglia cells promote angiogenesis by secreting exosomes. Adv. Clin. Exp. Med. 28, 421–430. doi: 10.17219/acem/91826
Tkach, M., and Théry, C. (2016). Communication by extracellular vesicles: where we are and where we need to go. Cell 164, 1226–1232. doi: 10.1016/j.cell.2016.01.043
Tobin, M. K., Bonds, J. A., Minshall, R. D., Pelligrino, D. A., Testai, F. D., and Lazarov, O. (2014). Neurogenesis and inflammation after ischemic stroke: what is known and where we go from here. J. Cereb. Blood Flow Metab. 34, 1573–1584. doi: 10.1038/jcbfm.2014.130
Uzdensky, A. B. (2019). Apoptosis regulation in the penumbra after ischemic stroke: expression of pro- and antiapoptotic proteins. Apoptosis 24, 687–702. doi: 10.1007/s10495-019-01556-6
Venkat, P., Cui, C., Chopp, M., Zacharek, A., Wang, F., Landschoot-Ward, J., et al. (2019). MiR-126 mediates brain endothelial cell exosome treatment-induced neurorestorative effects after stroke in type 2 diabetes mellitus mice. Stroke 50, 2865–2874. doi: 10.1161/STROKEAHA.119.025371
Wilson, M. E. (2013). Stroke: understanding the differences between males and females. Pflugers Arch. 465, 595–600. doi: 10.1007/s00424-013-1260-x
Xin, H., Katakowski, M., Wang, F., Qian, J. Y., Liu, X. S., Ali, M. M., et al. (2017a). MicroRNA cluster miR-17-92 cluster in exosomes enhance neuroplasticity and functional recovery after stroke in rats. Stroke 48, 747–753. doi: 10.1161/STROKEAHA.116.015204
Xin, H., Li, Y., and Chopp, M. (2014). Exosomes/miRNAs as mediating cell-based therapy of stroke. Front. Cell. Neurosci. 8:377. doi: 10.3389/fncel.2014.00377
Xin, H., Li, Y., Cui, Y., Yang, J. J., Zhang, Z. G., and Chopp, M. (2013a). Systemic administration of exosomes released from mesenchymal stromal cells promote functional recovery and neurovascular plasticity after stroke in rats. J. Cereb. Blood Flow Metab. 33, 1711–1715. doi: 10.1038/jcbfm.2013.152
Xin, H., Li, Y., Liu, Z., Wang, X., Shang, X., Cui, Y., et al. (2013b). MiR-133b promotes neural plasticity and functional recovery after treatment of stroke with multipotent mesenchymal stromal cells in rats via transfer of exosome-enriched extracellular particles. Stem Cells 31, 2737–2746. doi: 10.1002/stem.1409
Xin, H., Wang, F., Li, Y., Lu, Q. E., Cheung, W. L., Zhang, Y., et al. (2017b). Secondary release of exosomes from astrocytes contributes to the increase in neural plasticity and improvement of functional recovery after stroke in rats treated with exosomes harvested from microRNA 133b-overexpressing multipotent mesenchymal stromal cells. Cell Transplant. 26, 243–257. doi: 10.3727/096368916X693031
Zhang, H., Wu, J., Wu, J., Fan, Q., Zhou, J., Wu, J., et al. (2019). Exosome-mediated targeted delivery of miR-210 for angiogenic therapy after cerebral ischemia in mice. J. Nanobiotechnology 17:29. doi: 10.1186/s12951-019-0461-7
Zhao, Y., Gan, Y., Xu, G., Yin, G., and Liu, D. (2020). MSCs-derived exosomes attenuate acute brain injury and inhibit microglial inflammation by reversing cysLT2R-ERK1/2 mediated microglia M1 polarization. Neurochem. Res. 45, 1180–1190. doi: 10.1007/s11064-020-02998-0
Keywords: exosomes, animal models, ischemic stroke, cell-derived exosomes, meta-analysis
Citation: Huang M, Hong Z, Xiao C, Li L, Chen L, Cheng S, Lei T and Zheng H (2020) Effects of Exosomes on Neurological Function Recovery for Ischemic Stroke in Pre-clinical Studies: A Meta-analysis. Front. Cell. Neurosci. 14:593130. doi: 10.3389/fncel.2020.593130
Received: 09 August 2020; Accepted: 23 October 2020;
Published: 26 November 2020.
Edited by:
Raymond Ching-Bong Wong, Centre for Eye Research Australia, AustraliaReviewed by:
Burak Yulug, Alanya Alaaddin Keykubat University, TurkeyMuge Yemisci, Hacettepe University, Turkey
Copyright © 2020 Huang, Hong, Xiao, Li, Chen, Cheng, Lei and Zheng. This is an open-access article distributed under the terms of the Creative Commons Attribution License (CC BY). The use, distribution or reproduction in other forums is permitted, provided the original author(s) and the copyright owner(s) are credited and that the original publication in this journal is cited, in accordance with accepted academic practice. No use, distribution or reproduction is permitted which does not comply with these terms.
*Correspondence: Haiqing Zheng, emhlbmdoYWlxaW5nMDkwOSYjeDAwMDQwO2FsaXl1bi5jb20=
†These authors have contributed equally to this work