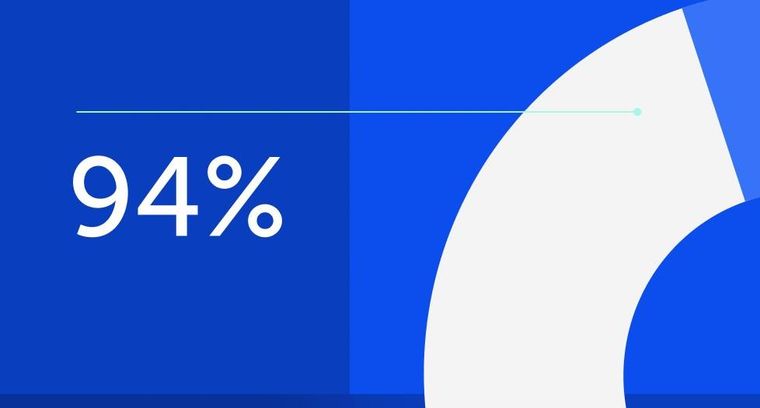
94% of researchers rate our articles as excellent or good
Learn more about the work of our research integrity team to safeguard the quality of each article we publish.
Find out more
ORIGINAL RESEARCH article
Front. Cell. Neurosci., 09 April 2019
Sec. Non-Neuronal Cells
Volume 13 - 2019 | https://doi.org/10.3389/fncel.2019.00109
This article is part of the Research TopicDual role of Microglia in Health and Disease: Pushing the Balance Towards RepairView all 13 articles
Accumulation of mutated superoxide dismutase 1 (mSOD1) in amyotrophic lateral sclerosis (ALS) involves injury to motor neurons (MNs), activation of glial cells and immune unbalance. However, neuroinflammation, besides its detrimental effects, also plays beneficial roles in ALS pathophysiology. Therefore, the targeting of microglia to modulate the release of inflammatory neurotoxic mediators and their exosomal dissemination, while strengthening cell neuroprotective properties, has gained growing interest. We used the N9 microglia cell line to identify phenotype diversity upon the overexpression of wild-type (WT; hSOD1WT) and mutated G93A (hSOD1G93A) protein. To investigate how each transduced cell respond to an inflammatory stimulus, N9 microglia were treated with lipopolysaccharide (LPS). Glycoursodeoxycholic acid (GUDCA) and dipeptidyl vinyl sulfone (VS), known to exert neuroprotective properties, were tested for their immunoregulatory properties. Reduced Fizz1, IL-10 and TLR4 mRNAs were observed in both transduced cells. However, in contrast with hSOD1WT-induced decreased of inflammatory markers, microglia transduced with hSOD1G93A showed upregulation of pro-inflammatory (TNF-α/IL-1β/HMGB1/S100B/iNOS) and membrane receptors (MFG-E8/RAGE). Importantly, their derived exosomes were enriched in HMGB1 and SOD1. When inflammatory-associated miRNAs were evaluated, increased miR-146a in cells with overexpressed hSOD1WT was not recapitulated in their exosomes, whereas hSOD1G93A triggered elevated exosomal miR-155/miR-146a, but no changes in cells. LPS stimulus increased M1/M2 associated markers in the naïve microglia, including MFG-E8, miR-155 and miR-146a, whose expression was decreased in both hSOD1WT and hSOD1G93A cells treated with LPS. Treatment with GUDCA or VS led to a decrease of TNF-α, IL-1β, HMGB1, S100B and miR-155 in hSOD1G93A microglia. Only GUDCA was able to increase cellular IL-10, RAGE and TLR4, together with miR-21, while decreased exosomal miR-155 cargo. Conversely, VS reduced MMP-2/MMP-9 activation, as well as upregulated MFG-E8 and miR-146a, while producing miR-21 shuttling into exosomes. The current study supports the powerful role of overexpressed hSOD1WT in attenuating M1/M2 activation, and that of hSOD1G93A in switching microglia from the steady state into a reactive phenotype with low responsiveness to stimuli. This work further reveals GUDCA and VS as promising modulators of microglia immune response by eliciting common and compound-specific molecular mechanisms that may promote neuroregeneration.
Amyotrophic lateral sclerosis (ALS) is a fatal motor neuron (MN) disease that affects both upper MNs, in the motor cortex, and lower MNs, in the brainstem and spinal cord. The major hallmark of this disease is the accumulation of intracellular protein inclusions in MNs, thought to be caused by mutations, protein damage such as oxidation, or protein seeding (Chiò et al., 2013; Robberecht and Philips, 2013). Among the several genes linked to ALS, there is evidence supporting a pathogenic role for Cu/Zn superoxide dismutase 1 (SOD1) in 20% of familial ALS cases and 3% of sporadic cases (Krüger et al., 2016). In fact, mutations in SOD1 are associated with a toxic gain of function that leads to protein misfolding and aggregation of the protein intracellularly (Rotunno and Bosco, 2013). More importantly, the uptake of misfolded and aggregated mutant SOD1 by other cells induces aggregation of endogenous mutant SOD1 and wild-type (WT) SOD1 protein (Münch et al., 2011; Sundaramoorthy et al., 2013). Small extracellular vesicles, here also designated as exosomes, have particular relevance as part of the cell-to-cell signaling mechanisms. Actually, once released, these vesicles can be taken up by nearby cells or travel long distances, affecting cellular function in either physiological or pathological ways (Sarko and McKinney, 2017). Misfolded and mutant SOD1 can be released via exocytosis, upon apoptosis or, as recently reported, inside exosomes (Gomes et al., 2007; Basso et al., 2013; Silverman et al., 2016). Once released into the extracellular space, mutant SOD1 activates microglia (Zhao et al., 2010) and we recently showed that the engulfment of exosomes released from mutant SOD1 MNs by microglia leads to the activation of inflammatory signaling pathways and loss of their phagocytic ability (Pinto et al., 2017). Despite decades of research and several studies pointing to SOD1 toxic function as the main player in ALS pathogenesis, the exact role of SOD1WT and the impact of the mutated form in microglia function remains unclear. The relevance of microglia in the onset and progression of ALS is increasingly recognized and different polarized activated phenotypes were found in several models of ALS. In the majority of the studies using mutated SOD1 models, microglia overactivation was shown to contribute for ALS progression (Beers et al., 2006; Boillée et al., 2006). Two types of microglial activation have been considered, the classical M1 phenotype associated with the release of pro-inflammatory molecules and activation of receptors, and the M2 phenotype related with the secretion of anti-inflammatory mediators and growth factors, contributing to the repair and neuroprotection (Brites and Fernandes, 2015; Komine and Yamanaka, 2015). However, the latest knowledge points to the coexistence of different heterogeneous states and mixed phenotypes (Tang and Le, 2016; Pinto et al., 2017), and anti-inflammatory strategies have been replaced by the concept of active immunomodulation (Pena-Altamira et al., 2016). Actually, microglia activation was described as having both beneficial and injurious effects in ALS, depending on the relative prevalence of harmful and protective genes, on the ALS disease model and on the state of disease progression (Liao et al., 2012; Brites and Vaz, 2014; Gravel et al., 2016). In this sense, while the reduction of microgliosis was shown to slow ALS progression in the mutated SOD1 mice (Martínez-Muriana et al., 2016), reactive microglia was protective to MN degeneration in a mouse model of TDP-43 proteinopathy (Spiller et al., 2018), reinforcing the relevance of microglia reactivity and function in the ALS context.
Inflammatory-associated microRNAs (inflamma-miRNAs) are without doubt a new paradigm for understanding immunoregulation and inflammation. They showed to be important mediators of macrophages/microglia polarization and were found as part of microglia exosomal cargo, thus being able to modulate other cells (Alexander et al., 2015; Cardoso et al., 2016; Cunha et al., 2016; Fernandes et al., 2018). One of the miRNAs that gained particular attention in ALS is miRNA(miR)-155, already described in fALS and sALS patients (Koval et al., 2013), and also in the pre-symptomatic mutated superoxide dismutase 1 (mSOD1) mice, even before MN loss (Cunha et al., 2018), pointing this miRNA as a promising biomarker in ALS. Interestingly, targeting of miR-155 restored microglial proper functions in mSOD1 mice and prolonged mice survival (Butovsky et al., 2015), suggesting the benefits of molecules targeting miR-155 levels to be used as therapeutic strategies.
Currently, there are still no specific targets and effective therapies for ALS, due to the involvement of several multifactorial pathophysiological mechanisms. The only available treatments licensed by the Food and Drug Administration (FDA) are riluzole, since 1996, and the new compound, Edaravone (Abe et al., 2017; Cruz, 2018). Our previous studies have demonstrated that glycoursodeoxycholic acid (GUDCA), a conjugated bile acid, has antioxidant, anti-inflammatory and neuroprotective effects in a cellular model of mutant SOD1 neurodegeneration (Vaz et al., 2015). Lately, we also showed that dipeptidyl vinyl sulfone (VS), a small molecule with inhibitory cysteine protease activity, was able to prevent amyloid-β(Aβ)-induced microglia-inflammatory signaling pathways in the N9 microglia cell line, by inhibiting high mobility group box protein 1 (HMGB1) and interleukin(IL)-1β production (Falcão et al., 2017). Surprisingly, VS was also able to rescue miR-155 overexpression, thus indicating its potential in miR-155 immunoregulation.
Mice overexpressing human SOD1WT have been used as controls, but some studies revealed the existence of abnormalities in the cerebellum (Afshar et al., 2017) and others that hastened the disease in G85R transgenic mice (Wang et al., 2009), highlighting that exaggerated expression of SOD1 is clearly not physiological. In fact, overexpression of both SOD1 and SOD1G93A genes account for an elevation of SOD1 protein levels and changes in iron metabolism genes expression (Gajowiak et al., 2015). However, in the N9 murine microglia, SOD1 overexpression decreased the release of tumor necrosis factor α (TNF-α) and IL-6 upon stimulation with lipopolysaccharide (LPS; Dimayuga et al., 2007), and that of mutant SOD1 in BV-2 microglial cell lines increased TNF-α secretion and their neurotoxic potential (Liu et al., 2009). It is thus essential to significantly expand our knowledge on the molecular mechanisms involved in ALS microglia dysfunctional properties to target key steps with novel drugs. In the present study, we assessed the inflammatory phenotype diversity of N9 murine microglia upon transduction to promote the overexpression of human SOD1, WT (hSOD1WT) or mutated G93A (hSOD1G93A) protein, relatively to naïve cells. To have a better insight on how such transduction may differently compromise the microglia response to an inflammatory stimulus, we treated these cells with LPS and evaluated a set of inflammatory and anti-inflammatory mediators, as well as inflamma-miRNAs. Finally, we assessed the potential immunoregulatory properties of GUDCA and VS.
Data revealed that both hSOD1WT and hSOD1G93A overexpression in N9-microglia induced morphological shape alterations and reduction of M2 markers, compatible with an activated cell. Major inflammatory genes were only increased in the hSOD1G93A cells, which released exosomes enriched in miR-155 and miR-146a, as well as in HMGB1 and SOD1 mRNAs. Interestingly, transduced cells showed a defective stimulus-response to LPS, in contrast with the naïve cells that presented exacerbated inflammatory markers. We also provide evidence that GUDCA and VS exert immunomodulatory properties over the hSOD1G93A microglia, but that their benefits are mediated by molecular pathways that are specific of each compound. Thus, we may conclude that depending on the key molecular cascades to be modulated towards neuroprotection, GUDCA and VS can be effective therapeutic strategies to restore microglial function in ALS.
We used the N9 microglial cell line (Cunha et al., 2016; Pinto et al., 2017). These cells are reported to present features similar to microglia in primary cultures, such as phagocytosis and inflammation-related features (Righi et al., 1989; Bruce-Keller et al., 2000; Fleisher-Berkovich et al., 2010). Lentiviral particles were produced by co-transfections of HEK293T cells with the packaging plasmids pGal-pol and pRev, the envelope plasmid pVSV-G and the lentiviral expression vectors plvAcGFP-hSOD1wt/plvAcGFP-hSOD1G93A (plasmids #27138 and #27142, respectively, Addgene, Cambridge, USA; Stevens et al., 2010), at a ratio of 3:2:1:4, using X-tremeGene HP, according to the manufacturer’s instructions (Roche, Mannhein, Germany; Simões et al., 2015; Pereira et al., 2016). Twenty-four hours after transfection, cell media was changed. Supernatants containing lentiviral particles were collected after 48 and 72 h, filtered using a 0.22 μm sterile filter and stored at −80°C. To stably overexpress hSOD1WT and hSOD1G93A in N9 microglia cell line, cells were seeded in 6-well plates at a density of 3 × 105 cells/well and, 24 h after plating, cells were transduced by adding the supernatants containing lentiviral particles. Media was changed 4–5 times to eliminate all the lentiviral particles (Simões et al., 2015; Pereira et al., 2016). Stable cell lines were purified by cell sorting of green fluorescent protein (GFP) expression (BDFACSAria, BD Biosciences) and the percentage of GFP-positive cells was monitored in the GUAVA flow cytometer. The percentage of GFP-expressing cells was >80% in all experiments (Figure 1). Cells were maintained in Roswell Park Memorial Institute (RPMI) medium supplemented with 10% fetal bovine serum (FBS), 1% L-glutamine and 1% Penicillin/Streptomycin. For each experiment, cells were plated at a concentration of 1 × 105 cells/ml (Cunha et al., 2016). RPMI was purchased from Sigma-Aldrich (St. Louis, MO, USA). FBS, L-glutamine and Penicillin/Streptomycin were purchased from Biochrom AG (Berlin, Germany).
Figure 1. Schematic representation of cell transfection for lentiviral production and further generation of N9 cells expressing hSOD1WT-green fluorescent protein (GFP) and hSOD1G93A-GFP protein. HEK293T cells were incubated with the packaging plasmids pGal-pol and pRev, the envelop plasmid pVSV-G and the lentiviral expression vectors plvAcGFP-hSOD1WT and plvAcGFP-hSOD1G93A, in order to produce lentivirus carrying single-strand RNAs (ssRNA) containing the sequence for human superoxide dismutase 1 wild-type (WT; hSOD1WT), and carrying the G93A mutation (hSOD1G93A). Cell supernatants were collected and incubated with N9 naïve cells, then reverse transcriptase converted the ssRNA into double-strand RNA (dsRNA) that entered the cell nucleus and stably integrated the genome.
After 24 h of plating, cellular media was changed and either hSOD1WT and hSOD1G93A cells were maintained for 48 h, treated or not with LPS (300 ng/ml). Non-transduced N9 cells (naïve) were used as controls, and also treated or not with LPS. In another set of experiments, hSOD1G93A cells were incubated with GUDCA (50 μM) or with VS (10 μM), also during 48 h.
Exosomes were obtained from the extracellular media of N9 naïve, hSOD1WT and N9 hSOD1G93A cells, according to our previous publication (Pinto et al., 2017). Briefly, after the time of incubation, extracellular media was centrifuged at 1,000 g for 10 min, to remove cell debris. Then, the supernatant was transferred to a different tube and centrifuged at 16,000 g for 1 h to pellet microvesicles. The recovered supernatant was filtered in a 0.22 μm pore filter, transferred to an ultracentrifuge tube and centrifuged at 100,000 g for 2 h, to pellet exosomes. Afterward, the pellet of exosomes was resuspended in phosphate-buffered saline (PBS) and centrifuged one last time at 100,000 g for 2 h, in order to wash the pellet. All the procedure was performed at 4°C. Exosome pellet was resuspended in lysis buffer for further RNA isolation.
Exosomes were visualized by transmission electron microscopy (TEM); for negative staining TEM, 10 μL of samples were mounted on Formvar/carbon film-coated mesh nickel grids (Electron Microscopy Sciences, Hatfield, PA, USA) and left standing for 2 min. The liquid in excess was removed with filter paper, and 10 μL of 1% uranyl acetate was added on to the grids and left standing for 10 s, after which, the liquid in excess was removed with filter paper. Visualization was carried out on a JEOL JEM 1400 TEM at 120 kV (Tokyo, Japan). Images were digitally recorded using a CCD digital camera Orious 1100W Tokyo, Japan at the HEMS/i3S of the University of Porto.
Cells were collected in Cell Lysis Buffer and protein concentration was determined using a protein assay kit (Bio-Rad, Hercules, CA, USA) according to manufacturer’s specifications. Then, 50 μg of protein were separated in a 12% polyacrylamide electrophoresis gel (SDS-PAGE) and transferred to a nitrocellulose membrane. After blocking with 5% (w/v) nonfat milk solution, membranes were incubated with the following primary antibodies: rabbit anti-SOD1 (1:500), mouse anti-GFP (1:1,000 both from Santa Cruz Biotechnology®) or anti-β-actin (1:5,000), from Sigma, all diluted in 5% (w/v) BSA overnight at 4°C, followed by the secondary antibodies goat anti-rabbit or goat anti-mouse, respectively, both HRP-linked (1:5,000 Santa Cruz Biotechnology®) diluted in blocking solution. Chemiluminescence detection was performed by using Western BrightTM Sirius (K- 12043-D10, Advansta, Menlo Park, CA, USA) and bands were visualized in the ChemiDocTM XRS System (Bio-Rad; Vaz et al., 2015). In parallel, we performed western blot analysis in isolated exosomes to evaluate the expression of Alix, Flotillin-1 and CD63 by using the same procedure, 20 μg of total protein and specific antibodies (mouse anti-Alix, Cell Signaling, mouse anti-flotillin-1, BD Biosciences, goat anti-CD63, Santa Cruz Biotechnology). In this case, we used Amido Black staining as the loading control.
Total RNA was extracted from N9 microglia using TRIzol® (LifeTechnologies, Carlsbad, CA, USA), according to manufacturer’s instructions. RNA inside exosomes was extracted using miRCURY Isolation Kit—Cell (#300110, Exiqon, Vedbaek, Denmark). Total RNA was quantified using Nanodrop ND-100 Spectrophotometer (NanoDrop Technologies, Wilmington, DE, USA) and conversion to cDNA was performed with GRS cDNA Synthesis Master Mix (GRiSP, Porto, Portugal). Quantitative Real Time-PCR (qRT-PCR) was performed on a QuantStudio 7 Flex Real-Time PCR System (Applied Biosystems) using an Xpert Fast Sybr Blue (GRiSP). qRT-PCR was accomplished under optimized conditions: 50°C for 2 min followed by 95°C for 2 min and finally 50 cycles at 95°C for 5 s and 62°C for 30 s. In order to verify the specificity of the amplification, a melt-curve analysis was performed, immediately after the amplification protocol. Non-specific products of PCR were not found in any case. Results were normalized to β-actin and expressed as fold change. The sequences used for primers are represented in Supplementary Table S1. For miRNA analysis, conversion of cDNA was achieved with the universal cDNA Synthesis Kit (#203301, Exiqon; Cunha et al., 2018). The Power SYBR® Green PCR Master Mix (Applied Biosystems) was used in combination with predesigned primers (Exiqon), represented in Supplementary Table S1, using SNORD110, U6 and RNU1A1 as reference genes. The reaction conditions consisted of polymerase activation/denaturation and well-factor determination at 95°C for 10 min, followed by 50 amplification cycles at 95°C for 10 s and 60°C for 1 min (ramp-rate 1.6°/s). Relative mRNA and miRNA concentrations were calculated using the ΔΔCT equation and quantification of target miRNAs was made in comparison to the geometric average of the three reference genes. In addition, we used the synthetic RNA template spike-in (UniSp6) as a positive control to ensure the quality of the reaction and subsequent evaluations. All samples were measured in duplicate.
Activities of MMP-2 and MMP-9 were determined in the N9 extracellular media, either alone or after incubation with LPS, GUDCA or VS, by performing a SDS-PAGE zymography in 0.1% gelatin-10% acrylamide gels, under non-reducing conditions (Silva et al., 2010). Briefly, after electrophoresis, the gels were washed for 1 h in a solution containing 2.5% Triton-X-100 to remove SDS and to renature the MMP species in the gel and then incubated at 37°C to induce gelatin lysis (buffer: 50 mM Tris pH 7.4, 5 mM CaCl2, 1 μM ZnCl2) overnight. Gels were then stained with 0.5% Coomassie Brilliant Blue R-250 (Sigma-Aldrich) and destained in 30% ethanol/10% acetic acid/H2O (v/v). Gelatinase activity, detected as a white band on a blue background, was measured using the Image LabTM analysis software (Bio-Rad).
Levels of nitric oxide (NO) were estimated by measuring the concentration of nitrites (), a product of NO metabolism, in the extracellular media of N9 cells. Extracellular medium, free from cellular debris, was mixed with Griess reagent [1% (w/v) sulfanilamide in 5% H3PO4 and 0.1% (w/v) N-1 naphtylethylenediamine, all from Sigma-Aldrich, in a proportion of 1:1 (v/v)] in 96-well tissue culture plates for 10 min in the dark, at room temperature (Vaz et al., 2015). The absorbance at 540 nm was determined using a microplate reader (Bio-Rad Laboratories; Hercules, CA, USA). A calibration curve was used for each assay. All samples were measured in duplicate.
Results of at least three independent experiments were expressed as mean ± SEM. Comparisons between N9 naïve and N9 naïve incubated with LPS were made using unpaired two-tailed Student’s t-test. Comparisons between the different groups were made by one-way ANOVA followed by multiple comparisons Bonferroni post hoc correction using GraphPad Prism 7 (GraphPad Software, San Diego, CA, USA). P-values of 0.05 were considered statistically significant.
To achieve SOD1 overexpression in microglia, N9 naïve cells were transduced with hSOD1WT and hSOD1G93A coupled with a GFP tail, as detailed in the methods section, which allowed us to monitor protein expression over time. As previously observed in our group, Western blot analysis showed the presence of mouse SOD1 protein expression at 16 kDa in all samples (Vaz et al., 2015). In N9 hSOD1WT and hSOD1G93A microglia, hSOD1 expression was verified at ~48 kDa, consistent with the weight of hSOD1 coupled with the GFP tail (Figure 2A). In addition, the 27 kDa band corresponding to GFP alone (indicated in additional supporting information) was only detected in cells transduced with GFP alone upon incubation with anti-GFP antibody. To note that, although appearing slightly higher in N9 hSOD1WT microglia, we did not find significant differences between hSOD1WT and hSOD1G93A expression, also confirmed by the percentage of GFP-positive cells checked routinely in the GUAVA flow cytometer, as well as by immunocytochemistry (Figure 2B). We additionally evaluated cellular morphology, phagocytic ability and cell viability to assess the impact of hSOD1 transduction in these cells. However, no differences were found between hSOD1WT and hSOD1G93A cells (Supplementary Figure S1).
Figure 2. Transduced N9 microglia efficiently express the human SOD1 protein. The presence of hSOD1 WT and carrying the G93A mutation (hSOD1WT and hSOD1G93A, respectively), coupled with the green fluorescent protein tail (hSOD1-GFP), as well as the expression of GFP was confirmed by Western blot and immunocytochemistry. (A) Western blot analysis indicates the presence of mouse SOD1, as well as hSOD1-GFP at a higher weight, while GFP expression in only observed for cells transduced with GFP not fused with hSOD1. Representative results from one blot are shown and β-actin was used as a loading control. Samples ran in the same gel at the same conditions. (B) Expression of hSOD1-GFP was confirmed by immunocytochemistry (green fluorescence). Nuclear staining was achieved with Hoechst dye (in blue). Representative results of one experiment are shown. Scale bar represents 20 μm.
In our previous work, we demonstrated that neuronal exosomes from hSOD1G93A cells carry a content of inflamma-miRNAs similar to the cells of origin, facilitating the shuttle of miR-124 from neuron to microglia, while induce phenotypic alterations and cellular reactivity in recipient microglia cells (Pinto et al., 2017). Therefore, in the current study, we isolated exosomes from N9, either naïve or overexpressing hSOD1WT or hSOD1G93A. We have confirmed the presence of Alix, Flotillin-1 and the tetraspanin CD63 in exosomal lysates (Figure 3A). Diameter exosome size was ~100 nm and cup-shape morphology was obtained by TEM (Figure 3B).
Figure 3. Exosomes released by N9 cells, either naïve or expressing hSOD1WT or hSOD1G93A show similar markers, size and shape. Exosomes were isolated from the extracellular media of N9 cells, either naïve or overexpressing human hSOD1WT and hSOD1G93A, as described in methods. (A) Western blot analysis indicates the presence of common exosome markers Alix, Flotillin-1, and CD63. (B) Representative images obtained by negative-staining transmission electron microscopy (TEM) of exosomes are depicted evidencing cup shape morphology and that the majority of vesicles have a diameter of close to 100 nm. Scale bar represents 100 nm.
Although SOD1 mutations are reported in fALS cases (Barber and Shaw, 2010), the effect of mutant SOD1G93A specifically in microglia is not completely clarified. Recently, reactive microglia evidenced to exert neuroprotective functions in a mouse model of TDP-43 proteinopathy (Spiller et al., 2018), highlighting the beneficial role that microglia activation may also have in neurodegenerative diseases. To explore the reactive signature of WT and mutant SOD1 in N9 microglia, we evaluated the gene expression of specific inflammatory markers in naïve and in transduced cells overexpressing hSOD1. When compared to naïve cells, N9 hSOD1WT microglia showed decreased levels of pro-inflammatory markers (usually associated to the M1 phenotype, TNF-α/IL-1β/S100B/iNOS), as well as anti-inflammatory markers (related to the M2 phenotype, Arg1/SOCS1/Fizz1/IL-10; Figures 4A,B). Although some anti-inflammatory markers were similar to naïve cells (Arg1 and SOCS1) or reduced in a similar way to those observed in N9 hSOD1WT microglia (Fizz1 and IL-10), N9 hSOD1G93A cells showed an increase in the pro-inflammatory markers that were studied. More importantly, some of these markers (TNF-α, IL-1β and HMGB1) were not only above the levels obtained in N9 hSOD1WT cells, but also above those of naïve cells, which indicate a stressed microglia that may contribute to either a depressed or exacerbated response, depending on the inflammatory stimulus (Figures 4A,B). Since we did not find significant differences between the levels of such markers in N9 transduced with GFP alone (Supplementary Figure S2), we may assume that the changes observed are due to the presence of hSOD1WT or hSOD1G93A and not because of the lentiviral infection. Moreover, increased expression of membrane surface receptors, like MFG-E8 and RAGE, was only evident in N9 hSOD1G93A microglia, when compared to N9 hSOD1WT microglia, while TLR-4 expression was reduced in both cells (Figure 4C). Interestingly, we observed that exosomes may also be involved in the propagation of the alarmin HMGB1 and the SOD1 protein, whose gene levels were found elevated in N9 hSOD1G93A microglia, thus contrasting with N9 hSOD1WT cells (Figure 4D). This result is consistent with previous findings where SOD1 was described to be transported into the extracellular media in exosomes and further collected by other cells (Silverman et al., 2016). This finding suggests that mutated microglia also contribute to chronic inflammatory status through the transport of HMGB1 in their released exosomes, never described to be carried by microglial-derived exosomes.
Figure 4. N9 hSOD1G93A microglia have a pro-inflammatory phenotype with an exacerbated MFG-E8 and RAGE gene expression, and originate exosomes enriched in HMGB1 and SOD1. Cellular mRNA expression of pro-inflammatory markers (A), anti-inflammatory markers (B) and membrane surface receptors (C) was assessed by quantitative Real-Time PCR (qRT-PCR). The dashed line represents the average value (N9 naïve cells). (D) mRNA expression was also evaluated in exosomes by qRT-PCR and N9 hSOD1WT cells were used as a control. Results are mean (± SEM) from at least three independent experiments. *p < 0.05 and **p < 0.01 vs. N9 naïve microglia; $p < 0.05 and $$p < 0.01 vs. N9 hSOD1WT microglia; one-way ANOVA (Bonferroni post hoc correction). ##p < 0.01 vs. N9 hSOD1WT cells; unpaired Student’s t-test (two-tailed).
Inflamma-miRNAs are reported to have a modulatory role in microglia activation (Cardoso et al., 2012; Saba et al., 2012) and to circulate as part of exosomal cargo (Alexander et al., 2015; Fernandes et al., 2018). In this study, we were mainly interested in assessing for the first time the differential expression of miR-155, miR-146a, miR-125b and miR-21 in N9 cells transduced with WT and mutant SOD1 relatively to the naïve ones, which are known to mediate macrophage polarization (Essandoh et al., 2016), CNS inflammation (Su et al., 2016) and to be expressed in the spinal cord of SOD1G93A mice at the symptomatic stage (Cunha et al., 2018). Decreased levels of miR-125b and miR-21, when compared with the naïve cells (as well as cells transduced with GFP, Supplementary Figure S2), were found in WT and mutated microglia (Figure 5A), and may account to a compromised response to an inflammatory stimulus (Tili et al., 2007; Parisi et al., 2016), thus turning microglia more susceptible to activation (Barnett et al., 2016; Cardoso et al., 2016). Regarding the pro-inflammatory miR-155 and its negative regulator miR-146a, only the last one was increased in N9 hSOD1WT microglia. While upregulated levels of miR-146a in hSOD1WT cells may act as a negative regulator of miR-155 increase, thus accounting for their normal content in exosomes, selective release of miR-155 and miR-146a from hSOD1G93A N9 cells into exosomes (Figure 5B) may drive disease progression in the mSOD1 ALS model. MiRNAs that are overrepresented in exosomes in comparison to levels in the cell indicate a selective exported mechanism (Bell and Taylor, 2017).
Figure 5. N9 hSOD1WT microglia highly express miR-146a, while N9 hSOD1G93A cells actively package miR-155 and miR-146a into their exosomes. Inflammatory microRNAs (miRNA, miR) expression in both cells (A) and exosomes (B) were analyzed by qRT-PCR. N9 naïve cells were used as a control and the dashed line represents the average value. Results are mean (± SEM) from at least three independent experiments. *p < 0.05 and **p < 0.01 vs. N9 naïve microglia; $p < 0.05 and $$p < 0.01 vs. N9 hSOD1WT microglia; one-way ANOVA (Bonferroni post hoc correction).
It was demonstrated that SOD1WT overexpression decreases ROS production and reduces neurotoxic inflammatory markers, even in the presence of LPS (Dimayuga et al., 2007). Therefore, we thought that would be interesting to further explore differences in hSOD1 microglia reactivity towards LPS treatment relatively to naïve cells, to better understand microglial behavior when facing an inflammatory environment in the context of ALS. As indicated in Table 1, incubation of naïve cells with LPS induced an activated phenotype with increased TNF-α, HMGB1 and S100B, but not of IL-1β or iNOS gene expression. Upregulation of Fizz1 and MFG-E8 was notorious, together with increased extracellular MMP-9 activation and NO production. Curiously, we found a decrease in the gene expression of S100B and RAGE. Stimulation with LPS also upregulated miR-155, miR-146a and miR-21, and downregulated miR-125b in cells. Interestingly, they were all found reduced in respective cell-derived exosomes, with the exception of miR-146a. However, either hSOD1WT or hSOD1G93A cells revealed lower expression of pro- and anti-inflammatory genes upon LPS challenge, than naïve N9 microglia.
Table 1. Differences in gene and miRNA expression, as well as in soluble factors, after exposure of naïve and transduced N9 microglia to lipopolysaccharide (LPS).
It is important to note that GFP-transduced cells were able to respond to LPS in a similar manner to naïve N9 in terms of pro- or anti-inflammatory markers we used, as well as in miRNAs expression (Supplementary Figure S2), indicating that the differences in transduced cells do not derive from lentiviral transduction or GFP expression. In a similar way, MFG-E8 and RAGE mRNA levels were found downregulated in both transduced cells, in comparison with the LPS-treated naïve ones, while no significant differences were obtained for extracellular MMP-9, MMP-2 and NO levels. The same defective pattern was observed for inflamma-miRNAs, though an increase of miR-21 was observed in LPS-treated hSOD1WT cells and of miR-125b in hSOD1G93A transduced microglia. To highlight, however, that the selective exported mechanism of miR-155 into exosomes was notorious in the hSOD1WT cells, contrasting with its low expression in mSOD1 cells and their exosomes after stimulation with LPS, further attesting their increased inability to develop an inflammatory response against damage.
The alterations observed in the expression of pro- and anti-inflammatory-associated genes and miRNAs, as well as the inability to mount an inflammatory response when facing an inflammatory stimulus in the microglia transduced with hSOD1G93A, led us to test two different compounds described as immunomodulators in glial cells (Fernandes et al., 2007; Falcão et al., 2017). Neither of them caused identifiable changes in cell viability (Supplementary Figure S3) and both were similarly efficient in decreasing the expression of genes associated to a pro-inflammatory response, such as TNF-α, IL-1β, HMGB1 and S100B (Figure 6A). Interestingly, we found that MMP-2 and MMP-9 activation was merely downregulated by VS in hSOD1G93A cells (Figure 6B), supporting its specificity over that of GUDCA to inhibit these pro-inflammatory mediators. This may have therapeutic relevance since the elevation of MMP-9/-2 expression was observed in the spinal cord of SOD1G93A mice (Fang et al., 2010), and that of MMP-9 in NSC-34 MNs overexpressing hSOD1G93A (Vaz et al., 2015). Though that both VS and GUDCA showed to reduce the expression of the anti-inflammatory associated genes Arg1 and SOCS1, only GUDCA significantly enhanced the expression of IL-10 mRNA (Figure 6C), revealing its potential to counteract inflammation whenever it is exacerbated. Unique potential therapeutic benefits of VS were also found for its ability in increasing the gene expression of MFG-E8 (Figure 6D), a protein that has been described as promoting microglia phagocytosis (Lei et al., 2013). Further studies should elucidate whether activation of RAGE and TLR-4 by GUDCA, as we here observed, will have benefits in restoring the immune response of hSOD1G93A cells. Additionally, both VS and GUDCA showed to be equally efficient in decreasing intracellular miR-155, while miR-21 was selectively enhanced by GUDCA and miR-146a by VS (Figure 7A), again reinforcing their action through distinct pathways. Sorting into exosomes was also different considering that GUDCA was again effective in sustaining dissemination of miR-155 and inflammation by exosomes released from hSOD1G93A cells. In addition, exosome-shuttling of miR-21, indicated to have protective effects against oxidative stress (Shi et al., 2018), merely occurred by VS treatment (Figure 7B).
Figure 6. Pro-inflammatory markers in N9 hSOD1G93A microglia are downregulated by glycoursodeoxycholic acid (GUDCA) and dipeptidyl vinyl sulfone (VS), with GUDCA specifically upregulating IL-10, RAGE and TLR-4 genes, and VS promoting the increase of MFG-E8 and the decrease of MMP-2 and MMP-9 activation. N9 hSOD1G93A microglia were incubated with 50 μM of GUDCA or 10 μM of VS for 48 h. Cellular mRNA expression of pro-inflammatory markers (A), anti-inflammatory markers (C) and membrane surface receptors (D) was evaluated by qRT-PCR. (B) Activation of MMP-2 and MMP-9 was assessed by gelatin zymography assay. The intensity of the bands was quantified using computerized image analysis (Image LabTM software). Non-treated mutant cells were used as control and the dashed line represents average values. Results are mean (± SEM) from at least three independent experiments. *p < 0.05 and **p < 0.01 vs. N9 hSOD1G93A microglia; $p < 0.05 and $$p < 0.01 vs. N9 hSOD1G93A + GUDCA; one-way ANOVA (Bonferroni post hoc correction).
Figure 7. Treatment of N9 hSOD1G93A microglia with glycoursodeoxycholic acid (GUDCA) and dipeptidil vinyl sulfone (VS) decreases miR-155, with GUDCA specifically upregulating miR-21 in cells, and VS promoting the increase of miR-146a in cells and of miR-21 in exosomes. After 48 h incubation of N9 hSOD1G93A microglia with 50 μM of GUDCA or 10 μM of VS, inflammatory microRNAs (miRNA, miR) in both cells (A) and exosomes (B) were analyzed by qRT-PCR. Non-treated mutant cells were used as control and the dashed line represents the average values. Results are mean (± SEM) from at least three independent experiments. *p < 0.05 and **p < 0.01 vs. N9 hSOD1G93A microglia; $p < 0.05 and $$p < 0.01 vs. N9 hSOD1G93A + GUDCA; one-way ANOVA (Bonferroni post hoc correction).
These novel data provide clear evidence of the beneficial contribution of VS and GUDCA as immunoregulatory compounds in the hSOD1G93A N9 microglia, by sharing similar modulatory properties, but overall by additionally counteracting distinct inflammatory signaling pathways.
The role of glial cells in neurodegenerative diseases, particularly in ALS, has been thoroughly investigated and discussed, with evidences that these cells are key contributors to the neuroinflammation processes and disease progression (Lasiene and Yamanaka, 2011). In this study, we focused on microglia since they have a deregulated inflammatory profile when expressing mutant SOD1 (Beers et al., 2006; Boillée et al., 2006). To create an ALS cellular model based in SOD1 mutations, we transduced the N9 microglia cell line in order to overexpress the hSOD1WT or hSOD1G93A gene. Although some studies have pointed that elevated and not physiological levels of SOD1WT may cause the neurodegeneration and disease onset in the SOD1 ALS mouse model (Wang et al., 2009; Afshar et al., 2017), the transduction process of WT and mutant hSOD1 in our cellular model did not cause any loss of cell viability. Furthermore, N9 hSOD1WT cells presented a higher viability when compared to N9 naïve cells, which may derive from the antioxidant properties of SOD1 protein, thus conferring increased resistance to cell death.
A study using the N9 microglia cell line demonstrated that SOD1 overexpression was able to decrease superoxide and NO production, as well as to attenuate the ability of activated microglia to induce toxicity in cultured neurons (Dimayuga et al., 2007). Here, we observed a decrease in the selected pro- and anti-inflammatory genes evaluated upon hSOD1WT expression, reflecting a deactivation process of the N9 microglia. The same was not observed in the case of hSOD1G93A overexpression, which led to heterogeneous activation and polarization of the cells. In fact, microglia isolated from the spinal cord of the mSOD1 model is characterized by an M1/M2 dichotomy (Chiu et al., 2013), but no clear information was provided regarding the cortical brain microglia (Righi et al., 1989), as the N9 model used in our study. In addition, a recent study showed that microglia activation leads to increased expression of pro-inflammatory genes that is accompanied by the expression of some anti-inflammatory genes, as well (Lively and Schlichter, 2018). Interestingly, when looking at the miRNA profile of transduced cells, miR-146a was only found increased in N9 hSOD1WT cells, which may determine the inhibition of the inflammatory response by targeting the NF-κB pathway with consequent downregulation of the alarmin S100B and the pro-inflammatory cytokines. Since NF-κB activation is necessary for the induction of several miRNAs, such as miR-125b and miR-21, this can explain the reduced levels of these miRNAs in N9 hSOD1WT cells. On the other hand, their downregulation in hSOD1G93A N9 microglia may reveal that cells became reactive and lose their ability to counteract an additional stress stimulus, considering the anti-inflammatory role of these miRNAs (Tili et al., 2007; Ma et al., 2011; Cardoso et al., 2016). In accordance with these findings, N9 hSOD1G93A cells revealed an overexpression of the RAGE receptor. Such result may derive from the activated inflammatory cascade subsequent to the binding of HMGB1 to RAGE, based on the elevated gene expression of such alarmin in these cells, with consequent increased expression of TNF-α, and IL-1β, as here observed by us. Indeed, increased expression of pro-inflammatory mediators in SOD1G93A mice has already been described (Hensley et al., 2006; Jeyachandran et al., 2015) and mutant SOD1G93A microglia was reported to be more neurotoxic than WT microglia, also due to the increased production of ROS and TNF-α pro-inflammatory cytokine (Liu et al., 2009). Additionally, WT and mutated SOD1 overexpression cause a decrease in Fizz1 and IL-10 expression genes, suggesting a delayed response of the transduced microglia toward repair mechanisms during neuroinflammation.
Furthermore, in the mutant SOD1 mouse model, as well as in fALS and sALS patients, miR-155 was found to be elevated and its targeting revealed to restore the dysfunctional microglia and to attenuate disease progression in the ALS mouse model (Koval et al., 2013; Butovsky et al., 2015; Cunha et al., 2018). In N9 hSOD1G93A microglia, despite the similar endogenous levels of miR-155 and miR-146a relatively to naïve cells, these miRNAs were found to be shuttled into exosomes, similarly to our previous observations on N9 microglia polarization by LPS (Cunha et al., 2016). Our findings in hSOD1G93A overexpressing cells highlight the exacerbated expression of inflammatory genes indicating that cells carrying such mutation are more reactive than the N9 hSOD1WT microglia, probably influencing the surrounding cells and contributing to an overall inflammatory environment. In accordance, gene expression analysis in exosomes from mutated cells also revealed that HMGB1 and SOD1 are transported as part of their cargo, together with miR-155 and miR-146a, contributing to the dissemination of inflammatory mediators and aggravating the neuroinflammation status. These results are supported by previous studies showing that mutant/misfolded SOD1 is released into exosomes and is propagated to different cells, while also inducing exosome formation and release from cells (Basso et al., 2013; Grad et al., 2014; Silverman et al., 2016). HMGB1 is described as being secreted either in apoptotic bodies (Bell et al., 2006; Buzas et al., 2014), or in cell-derived exosomes (Sheller-Miller et al., 2017), but never mentioned to be carried in microglia-derived exosomes, as we now report. Although MFG-E8 is not a typical pro-inflammatory mediator, activated microglia upregulate MFG-E8, which has been related with the phagocytosis of viable neurons (Fricker et al., 2012) and with the production of pro-inflammatory cytokines (Liu et al., 2013). However, MFG-E8 role appears to be dose-dependent, since it is also proposed that a high concentration of MFG-E8 blocks the binding between apoptotic cells and microglia/macrophages, thus leading to the inhibition of phagocytosis (Yamaguchi et al., 2008). This may indicate that, in an inflammatory state, in which these cells appear to be, N9 hSOD1G93A microglia can either cause neuronal death or be unable to restore the homeostatic balance, contributing to ALS pathogenesis.
Overexpression of hSOD1WT and hSOD1G93A, although useful to understand ALS underlying mechanisms, are not physiological models. In this sense, only the transduced cells with GFP stimulated with LPS reproduced the M1 polarization found in the naïve cells after 24 h treatment (Cunha et al., 2016), while a defective stimulation and depressed resolving capacity after LPS pro-inflammatory activation was found in the transduced N9 hSOD1WT and hSOD1G93A microglia. We may then hypothesize that these microglia may become inefficient protectors of the brain against endogenous damage and pathogens. Actually, when compared with the response of the naïve cells towards LPS, hSOD1WT and hSOD1G93A N9 microglia clearly revealed a decrease in the majority of pro- and anti-inflammatory mediators. Moreover, intracellular inflammatory-associated miRNAs were downregulated, including miR-155 and miR-146a, which are both involved in the NF-κB pathway, by being upregulated upon inflammatory stimulus, and by acting as a negative feedback regulator, respectively (Mann et al., 2017). Though SOD1 overexpression was indicated to reduce neurotoxic inflammatory signaling in microglia (Dimayuga et al., 2007), we could still observe an increased exosomal content in miR-155 meaning that immunomodulatory effects were conserved, a finding not observed in exosomes from the mutated N9 microglia. Instead, their enrichment in miR-125b was shown to account for decreased MN survival in ALS, once miR-125b was found to be neurotoxic and to be upregulated in the lumbar spinal cord of SOD1G93A mice (Parisi et al., 2016).
After concluding that hSOD1G93A N9 microglia show upregulated pro-inflammatory markers, downregulated resolving genes, and exosomal enriched cargo in HMGB1 and SOD1, we tested the immunoregulatory properties of GUDCA and VS in rescuing the dysfunctional phenotype of the mutated microglia towards a more neuroprotective one, based on previous results (Vaz et al., 2015; Falcão et al., 2017). Our data demonstrate that both GUDCA and VS promote inflammatory resolution leading to a reduction of TNF-α, IL-1β, HMGB1, S100B and miR-155 expression, corroborating their anti-inflammatory properties (Fernandes et al., 2007; Vaz et al., 2015; Ko et al., 2017) in other cells and disease models. Interestingly, we observed a significant increase of the anti-inflammatory cytokine IL-10, together with miR-21 and membrane receptors RAGE and TLR-4 only in GUDCA-treated cells. This is not without precedent, since GUDCA was demonstrated to inhibit the production of TNF-α and IL-1β in astroglial cells by preventing the maturation of these cytokines and their consequent release in an experimental model of jaundice, thus preventing astroglial reactivity (Fernandes et al., 2007). Also, miR-21 was reported to promote an anti-inflammatory response by increasing IL-10 production (Sheedy et al., 2010). In this way, GUDCA may be acting through the activation of the receptor TLR-4, leading to the expression of this miRNA that, consequently, promotes an anti-inflammatory response. The decrease of miR-155 encapsulation in exosomes by GUDCA may further concur to attenuate the stimulation produced by the hSOD1G93A overexpression in N9 cells. Regarding VS, similarly to what we observed in N9-microglia exposed to Aβ peptide (Falcão et al., 2017), this compound showed its anti-inflammatory properties by reducing miR-155, HMGB1 and IL-1β, as well as MMP-2 and MMP-9 activation. Additionally, VS increased the cellular expression of miR-146a and decreased that of miR-155, probably as a consequence of NF-κB pathway inhibition and its immunoregulatory properties (Su et al., 2016). Furthermore, VS significantly promoted the expression of MFG-E8 that drives microglia phagocytosis (Liu et al., 2013) and the shuttle of miR-21 into exosomes in hSOD1G93A N9 microglia, which was reported to negatively regulate the secondary inflammatory response of microglia (Wang et al., 2018) by acting in recipient cells. The inhibition of the pro-inflammatory response by miR-146a upregulation may also favor the specific downregulation of MMP-2 and MMP-9 (Könnecke and Bechmann, 2013), potentially resulting in the restoration of immune homeostasis and highlighting VS as a microglia-targeted candidate in ALS. We may then conclude that, although through different mechanisms of action, both GUDCA and VS show beneficial therapeutic properties for immunomodulation towards neuroprotection in ALS. Importantly, because each of the compounds showed specificity to unique microglia targets, that may open new possibilities to modulate precise microglia subtypes.
Overall, and as schematically represented in Figure 8, our findings clarify the importance of hSOD1WT in the host cell, by having calming and antioxidant effects. Besides inducing the expression of pro-inflammatory genes, hSOD1G93A protein decreased the expression of anti-inflammatory genes, thus increasing the reactivity of the cell. In addition, by decreasing microglia repairing ability in damaging conditions, the expression of the mutated protein may further contribute to compromised homeostatic balance and failure in neuroregenerative processes. Finally, we propose that GUDCA and VS are potential pharmacological approaches in single or combined therapeutic strategies for ALS, due to their multi-target effects and active microglia immunomodulation.
Figure 8. Schematic representation of the dysregulated inflammatory-associated mediators and immunomodulation benefits by GUDCA or VS in N9 cells transduced with hSOD1WT and hSOD1G93A protein. Overexpression of hSOD1WT causes a downregulation of pro- and anti-inflammatory genes in N9 cells and upregulation of miR-146a. In contrast, hSOD1G93A transduction causes increased gene expression of pro-inflammatory mediators, thus leading to an activated microglia population. Both transduced cells reveal a decrease of miR-125b and miR-21. To note that the release of exosomes containing miR-155 and miR-146a, as well as HMGB1 and SOD1 by N9 hSOD1G93A cells may determine the propagation of inflammation. Treatment with GUDCA or VS effectively modulates the gene expression of pro-inflammatory molecules, together with the increase of anti-inflammatory miRNAs. GUDCA increases IL-10 and cellular miR-21, with a substantial reduction of exosomal miR-155, while VS decreases MMP-2 and MMP-9 activation and determines the shuttle of miR-21 into exosomes. In sum, GUDCA and VS are relevant immunoregulators of the dysfunctional microglia in mutated superoxide dismutase 1 (mSOD1) ALS, and their differential target specificity may be envisaged as very promising to recover specific disease-related subtypes. GUDCA, glycoursodeoxycholic acid; HMGB1, high mobility group box protein 1; miR, microRNA; MMP, matrix metalloproteinase; SOD1, superoxide dismutase 1; VS, dipeptidyl vinyl sulfone.
DB and AV conceived and designed the experiments. AV and CC implemented and optimized the transduction procedures. RM supervised the chemical synthesis of dipeptidyl vinyl sulfone. AV, SP, CE and LC carried out the experiments. SP, AV and DB analyzed the data and wrote the manuscript. DB critically reviewed the content and approved the final version for publication.
This work was funded by Research Grant of the Santa Casa Scientific Research Program on ALS (SCML-Project ELA-2015-002 to DB), by Fundação para a Ciência e a Tecnologia (FCT), through project PTDC/MED-NEU/31395/2017 (to DB) and grant UID/DTP/04138/2013 (to iMed.ULisboa), as well as by the Programa Operacional Regional de Lisboa and the Programa Operacional Competitividade e Internacionalização (LISBOA-01-0145-FEDER-031395 to DB), and individual fellowship (SFRH/BPD/76590/2011 to AV).
The authors declare that the research was conducted in the absence of any commercial or financial relationships that could be construed as a potential conflict of interest.
We thank Diane Pereira and Inês Moranguinho from iMed.ULisboa, for providing plasmids that allowed us to produce the lentiviral particles; Dr. Elsa Rodrigues, iMed.ULisboa, for the anti-GFP antibody and for helping in the interpretation of the data obtained with such antibody. We also thank Dr. Rui Fernandes and A.R. Malheiro, i3S—Instituto de Investigação e Inovação em Saúde and HEMS/IBMC—Histology and Electron Microscopy Service, Universidade do Porto for acquisition and analysis of TEM images.
The Supplementary Material for this article can be found online at: https://www.frontiersin.org/articles/10.3389/fncel.2019.00109/full#supplementary-material
Abe, K., Aoki, M., Tsuji, S., Itoyama, Y., Sobue, G., Togo, M., et al. (2017). Safety and efficacy of edaravone in well defined patients with amyotrophic lateral sclerosis: a randomised, double-blind, placebo-controlled trial. Lancet Neurol. 16, 505–512. doi: 10.1016/S1474-4422(17)30115-1
Afshar, P., Ashtari, N., Jiao, X., Rahimi-Balaei, M., Zhang, X., Yaganeh, B., et al. (2017). Overexpression of human SOD1 leads to discrete defects in the cerebellar architecture in the mouse. Front. Neuroanat. 11:22. doi: 10.3389/fnana.2017.00022
Alexander, M., Hu, R., Runtsch, M. C., Kagele, D. A., Mosbruger, T. L., Tolmachova, T., et al. (2015). Exosome-delivered microRNAs modulate the inflammatory response to endotoxin. Nat. Commun. 6, 7321–7321. doi: 10.1038/ncomms8321
Barber, S. C., and Shaw, P. J. (2010). Oxidative stress in ALS: key role in motor neuron injury and therapeutic target. Free Radic. Biol. Med. 48, 629–641. doi: 10.1016/j.freeradbiomed.2009.11.018
Barnett, R. E., Conklin, D. J., Ryan, L., Keskey, R. C., Ramjee, V., Sepulveda, E. A., et al. (2016). Anti-inflammatory effects of miR-21 in the macrophage response to peritonitis. J. Leukoc. Biol. 99, 361–371. doi: 10.1189/jlb.4A1014-489R
Basso, M., Pozzi, S., Tortarolo, M., Fiordaliso, F., Bisighini, C., Pasetto, L., et al. (2013). Mutant copper-zinc superoxide dismutase (SOD1) induces protein secretion pathway alterations and exosome release in astrocytes: implications for disease spreading and motor neuron pathology in Amyotrophic Lateral Sclerosis. J. Biol. Chem. 288, 15699–15711. doi: 10.1074/jbc.m112.425066
Beers, D. R., Henkel, J. S., Xiao, Q., Zhao, W., Wang, J., Yen, A. A., et al. (2006). Wild-type microglia extend survival in PU.1 knockout mice with familial amyotrophic lateral sclerosis. Proc. Natl. Acad. Sci. U S A 103, 16021–16026. doi: 10.1073/pnas.0607423103
Bell, C. W., Jiang, W., Reich, C. F. III., and Pisetsky, D. S. (2006). The extracellular release of HMGB1 during apoptotic cell death. Am. J. Physiol. Cell Physiol. 291, C1318–C1325. doi: 10.1152/ajpcell.00616.2005
Bell, E., and Taylor, M. A. (2017). Functional roles for exosomal MicroRNAs in the tumour microenvironment. Comput. Struct. Biotechnol. J. 15, 8–13. doi: 10.1016/j.csbj.2016.10.005
Boillée, S., Yamanaka, K., Lobsiger, C. S., Copeland, N. G., Jenkins, N. A., Kassiotis, G., et al. (2006). Onset and progression in inherited ALS determined by motor neurons and microglia. Science 312, 1389–1392. doi: 10.1126/science.1123511
Brites, D., and Fernandes, A. (2015). Neuroinflammation and depression: microglia activation, extracellular microvesicles and microRNA dysregulation. Front. Cell. Neurosci. 9:476. doi: 10.3389/fncel.2015.00476
Brites, D., and Vaz, A. R. (2014). Microglia centered pathogenesis in ALS: insights in cell interconnectivity. Front. Cell. Neurosci. 8, 117–117. doi: 10.3389/fncel.2014.00117
Bruce-Keller, A. J., Keeling, J. L., Keller, J. N., Huang, F. F., Camondola, S., and Mattson, M. P. (2000). Antiinflammatory effects of estrogen on microglial activation. Endocrinology 141, 3646–3656. doi: 10.1210/en.141.10.3646
Butovsky, O., Jedrychowski, M. P., Cialic, R., Krasemann, S., Murugaiyan, G., Fanek, Z., et al. (2015). Targeting miR-155 restores abnormal microglia and attenuates disease in SOD1 mice. Ann. Neurol. 77, 75–99. doi: 10.1002/ana.24304
Buzas, E. I., György, B., Nagy, G., Falus, A., and Gay, S. (2014). Emerging role of extracellular vesicles in inflammatory diseases. Nat. Rev. Rheumatol. 10, 356–364. doi: 10.1038/nrrheum.2014.19
Cardoso, A. L., Guedes, J. R., and de Lima, M. C. P. (2016). Role of microRNAs in the regulation of innate immune cells under neuroinflammatory conditions. Curr. Opin. Pharmacol. 26, 1–9. doi: 10.1016/j.coph.2015.09.001
Cardoso, A. L., Guedes, J. R., Pereira de Almeida, L., and Pedroso de Lima, M. C. (2012). miR-155 modulates microglia-mediated immune response by down-regulating SOCS-1 and promoting cytokine and nitric oxide production. Immunology 135, 73–88. doi: 10.1111/j.1365-2567.2011.03514.x
Chiò, A., Traynor, B. J., Collins, J., Simeone, J. C., Goldstein, L. A., and White, L. A. (2013). Global epidemiology of amyotrophic lateral sclerosis: a systematic review of the published literature. Neuroepidemiology 41, 118–130. doi: 10.1159/000351153
Chiu, I. M., Morimoto, E. T., Goodarzi, H., Liao, J. T., O’Keeffe, S., Phatnani, H. P., et al. (2013). A neurodegeneration-specific gene-expression signature of acutely isolated microglia from an amyotrophic lateral sclerosis mouse model. Cell Rep. 4, 385–401. doi: 10.1016/j.celrep.2013.06.018
Cruz, M. P. (2018). Edaravone (Radicava): a novel neuroprotective agent for the treatment of amyotrophic lateral sclerosis. PT 43, 25–28.
Cunha, C., Gomes, C., Vaz, A. R., and Brites, D. (2016). Exploring new inflammatory biomarkers and pathways during LPS-induced M1 polarization. Mediators Inflamm. 2016:6986175. doi: 10.1155/2016/6986175
Cunha, C., Santos, C., Gomes, C., Fernandes, A., Correia, A. M., Sebastiao, A. M., et al. (2018). Downregulated glia interplay and increased miRNA-155 as promising markers to track ALS at an early stage. Mol. Neurobiol. 55, 4207–4224. doi: 10.1007/s12035-017-0631-2
Dimayuga, F. O., Wang, C., Clark, J. M., Dimayuga, E. R., Dimayuga, V. M., and Bruce-Keller, A. J. (2007). SOD1 overexpression alters ROS production and reduces neurotoxic inflammatory signaling in microglial cells. J. Neuroimmunol. 182, 89–99. doi: 10.1016/j.jneuroim.2006.10.003
Essandoh, K., Li, Y., Huo, J., and Fan, G. C. (2016). MiRNA-mediated macrophage polarization and its potential role in the regulation of inflammatory response. Shock 46, 122–131. doi: 10.1097/SHK.0000000000000604
Falcão, A. S., Carvalho, L. A. R., Lidónio, G., Vaz, A. R., Lucas, S. D., Moreira, R., et al. (2017). Dipeptidyl vinyl sulfone as a novel chemical tool to inhibit HMGB1/NLRP3-inflammasome and inflamma-miRs in Aβ-mediated microglial inflammation. ACS Chem. Neurosci. 8, 89–99. doi: 10.1021/acschemneuro.6b00250
Fang, L., Teuchert, M., Huber-Abel, F., Schattauer, D., Hendrich, C., Dorst, J., et al. (2010). MMP-2 and MMP-9 are elevated in spinal cord and skin in a mouse model of ALS. J. Neurol. Sci. 294, 51–56. doi: 10.1016/j.jns.2010.04.005
Fernandes, A., Ribeiro, A. R., Monteiro, M., Garcia, G., Vaz, A. R., and Brites, D. (2018). Secretome from SH-SY5Y APPSwecells trigger time-dependent CHME3 microglia activation phenotypes, ultimately leading to miR-21 exosome shuttling. Biochimie 155, 67–82. doi: 10.1016/j.biochi.2018.05.015
Fernandes, A., Vaz, A. R., Falcão, A. S., Silva, R. F. M., Brito, M. A., and Brites, D. (2007). Glycoursodeoxycholic acid and interleukin-10 modulate the reactivity of rat cortical astrocytes to unconjugated bilirubin. J. Neuropathol. Exp. Neurol. 66, 789–798. doi: 10.1097/nen.0b013e3181461c74
Fleisher-Berkovich, S., Filipovich-Rimon, T., Ben-Shmuel, S., Hülsmann, C., Kummer, M. P., and Heneka, M. T. (2010). Distinct modulation of microglial amyloid β phagocytosis and migration by neuropeptides. J. Neuroinflammation 7:61. doi: 10.1186/1742-2094-7-61
Fricker, M., Neher, J. J., Zhao, J. W., Théry, C., Tolkovsky, A. M., and Brown, G. C. (2012). MFG-E8 mediates primary phagocytosis of viable neurons during neuroinflammation. J. Neurosci. 32, 2657–2666. doi: 10.1523/JNEUROSCI.4837-11.2012
Gajowiak, A., Styś, A., Starzyński, R. R., Bednarz, A., Lenartowicz, M., Staroń, R., et al. (2015). Mice overexpressing both non-mutated human SOD1 and mutated SOD1(G93A) genes: a competent experimental model for studying iron metabolism in amyotrophic lateral sclerosis. Front. Mol. Neurosci. 8:82. doi: 10.3389/fnmol.2015.00082
Gomes, C., Keller, S., Altevogt, P., and Costa, J. (2007). Evidence for secretion of Cu,Zn superoxide dismutase via exosomes from a cell model of amyotrophic lateral sclerosis. Neurosci. Lett. 428, 43–46. doi: 10.1016/j.neulet.2007.09.024
Grad, L. I., Yerbury, J. J., Turner, B. J., Guest, W. C., Pokrishevsky, E., O’Neill, M. A., et al. (2014). Intercellular propagated misfolding of wild-type Cu/Zn superoxide dismutase occurs via exosome-dependent and -independent mechanisms. Proc. Natl. Acad. Sci. U S A 111, 3620–3625. doi: 10.1073/pnas.1312245111
Gravel, M., Béland, L. C., Soucy, G., Abdelhamid, E., Rahimian, R., Gravel, C., et al. (2016). IL-10 controls early microglial phenotypes and disease onset in ALS caused by misfolded superoxide dismutase 1. J. Neurosci. 36, 1031–1048. doi: 10.1523/JNEUROSCI.0854-15.2016
Hensley, K., Abdel-Moaty, H., Hunter, J., Mhatre, M., Mou, S., Nguyen, K., et al. (2006). Primary glia expressing the G93A-SOD1 mutation present a neuroinflammatory phenotype and provide a cellular system for studies of glial inflammation. J. Neuroinflammation 3:2. doi: 10.1186/1742-2094-3-2
Jeyachandran, A., Mertens, B., McKissick, E. A., and Mitchell, C. S. (2015). Type I Vs. type II cytokine levels as a function of sod1 g93a mouse amyotrophic lateral sclerosis disease progression. Front. Cell. Neurosci. 9:462. doi: 10.3389/fncel.2015.00462
Ko, W. K., Lee, S. H., Kim, S. J., Jo, M. J., Kumar, H., Han, I. B., et al. (2017). Anti-inflammatory effects of ursodeoxycholic acid by lipopolysaccharide-stimulated inflammatory responses in RAW 264.7 macrophages. PLoS One 12:e0180673. doi: 10.1371/journal.pone.0180673
Komine, O., and Yamanaka, K. (2015). Neuroinflammation in motor neuron disease. Nagoya J. Med. Sci. 77, 537–549.
Könnecke, H., and Bechmann, I. (2013). The role of microglia and matrix metalloproteinases involvement in neuroinflammation and gliomas. Clin. Dev. Immunol. 2013:914104. doi: 10.1155/2013/914104
Koval, E. D., Shaner, C., Zhang, P., du Maine, X., Fischer, K., Tay, J., et al. (2013). Method for widespread microRNA-155 inhibition prolongs survival in ALS-model mice. Hum. Mol. Genet. 22, 4127–4135. doi: 10.1093/hmg/ddt261
Krüger, S., Battke, F., Sprecher, A., Munz, M., Synofzik, M., Schöls, L., et al. (2016). Rare variants in neurodegeneration associated genes revealed by targeted panel sequencing in a german ALS cohort. Front. Mol. Neurosci. 9:92. doi: 10.3389/fnmol.2016.00092
Lasiene, J., and Yamanaka, K. (2011). Glial cells in amyotrophic lateral sclerosis. Neurol. Res. Int. 2011, 718987–718987. doi: 10.1155/2011/718987
Lei, C., Lin, S., Zhang, C., Tao, W., Dong, W., Hao, Z., et al. (2013). High-mobility group box1 protein promotes neuroinflammation after intracerebral hemorrhage in rats. Neuroscience 228, 190–199. doi: 10.1016/j.neuroscience.2012.10.023
Liao, B., Zhao, W., Beers, D. R., Henkel, J. S., and Appel, S. H. (2012). Transformation from a neuroprotective to a neurotoxic microglial phenotype in a mouse model of ALS. Exp. Neurol. 237, 147–152. doi: 10.1016/j.expneurol.2012.06.011
Liu, Y., Hao, W., Dawson, A., Liu, S., and Fassbender, K. (2009). Expression of amyotrophic lateral sclerosis-linked SOD1 mutant increases the neurotoxic potential of microglia via TLR2. J. Biol. Chem. 284, 3691–3699. doi: 10.1074/jbc.M804446200
Liu, Y., Yang, X., Guo, C., Nie, P., Liu, Y., and Ma, J. (2013). Essential role of MFG-E8 for phagocytic properties of microglial cells. PLoS One 8:e55754. doi: 10.1371/journal.pone.0055754
Lively, S., and Schlichter, L. C. (2018). Microglia responses to pro-inflammatory stimuli (LPS, IFNγ+TNFα) and reprogramming by resolving cytokines (IL-4, IL-10). Front. Cell. Neurosci. 12:215. doi: 10.3389/fncel.2018.00215
Ma, X., Becker Buscaglia, L. E., Barker, J. R., and Li, Y. (2011). MicroRNAs in NF-kappaB signaling. J. Mol. Cell Biol. 3, 159–166. doi: 10.1093/jmcb/mjr007
Mann, M., Mehta, A., Zhao, J. L., Lee, K., Marinov, G. K., Garcia-Flores, Y., et al. (2017). An NF-κB-microRNA regulatory network tunes macrophage inflammatory responses. Nat. Commun. 8:851. doi: 10.1038/s41467-017-00972-z
Martínez-Muriana, A., Mancuso, R., Francos-Quijorna, I., Olmos-Alonso, A., Osta, R., Perry, V. H., et al. (2016). CSF1R blockade slows the progression of amyotrophic lateral sclerosis by reducing microgliosis and invasion of macrophages into peripheral nerves. Sci. Rep. 6:25663. doi: 10.1038/srep25663
Münch, C., O’Brien, J., and Bertolotti, A. (2011). Prion-like propagation of mutant superoxide dismutase-1 misfolding in neuronal cells. Proc. Natl. Acad. Sci. U S A 108, 3548–3553. doi: 10.1073/pnas.1017275108
Parisi, C., Napoli, G., Amadio, S., Spalloni, A., Apolloni, S., Longone, P., et al. (2016). MicroRNA-125b regulates microglia activation and motor neuron death in ALS. Cell Death Differ. 23, 531–541. doi: 10.1038/cdd.2015.153
Pena-Altamira, E., Prati, F., Massenzio, F., Virgili, M., Contestabile, A., Bolognesi, M. L., et al. (2016). Changing paradigm to target microglia in neurodegenerative diseases: from anti-inflammatory strategy to active immunomodulation. Expert Opin. Ther. Targets 20, 627–640. doi: 10.1517/14728222.2016.1121237
Pereira, D. M., Simões, A. E. S., Gomes, S. E., Castro, R. E., Carvalho, T., Rodrigues, C. M. P., et al. (2016). MEK5/ERK5 signaling inhibition increases colon cancer cell sensitivity to 5-fluorouracil through a p53-dependent mechanism. Oncotarget 7, 34322–34340. doi: 10.18632/oncotarget.9107
Pinto, S., Cunha, C., Barbosa, M., Vaz, A. R., and Brites, D. (2017). Exosomes from NSC-34 cells transfected with hSOD1–G93A are enriched in mir-124 and drive alterations in microglia phenotype. Front. Neurosci. 11:273. doi: 10.3389/fnins.2017.00273
Righi, M., Mori, L., De Libero, G., Sironi, M., Biondi, A., Mantovani, A., et al. (1989). Monokine production by microglial cell clones. Eur. J. Immunol. 19, 1443–1448. doi: 10.1002/eji.1830190815
Robberecht, W., and Philips, T. (2013). The changing scene of amyotrophic lateral sclerosis. Nat. Rev. Neurosci. 14, 248–264. doi: 10.1038/nrn3430
Rotunno, M. S., and Bosco, D. A. (2013). An emerging role for misfolded wild-type SOD1 in sporadic ALS pathogenesis. Front. Cell. Neurosci. 7:253. doi: 10.3389/fncel.2013.00253
Saba, R., Gushue, S., Huzarewich, R. L. C. H., Manguiat, K., Medina, S., Robertson, C., et al. (2012). MicroRNA 146a (miR-146a) is over-expressed during prion disease and modulates the innate immune response and the microglial activation state. PLoS One 7:e30832. doi: 10.1371/journal.pone.0030832
Sarko, D. K., and McKinney, C. E. (2017). Exosomes: origins and therapeutic potential for neurodegenerative disease. Front. Neurosci. 11:82. doi: 10.3389/fnins.2017.00082
Sheedy, F. J., Palsson-McDermott, E., Hennessy, E. J., Martin, C., O’Leary, J. J., Ruan, Q., et al. (2010). Negative regulation of TLR4 via targeting of the proinflammatory tumor suppressor PDCD4 by the microRNA miR-21. Nat. Immunol. 11, 141–147. doi: 10.1038/ni.1828
Sheller-Miller, S., Urrabaz-Garza, R., Saade, G., and Menon, R. (2017). Damage-associated molecular pattern markers HMGB1 and cell-free fetal telomere fragments in oxidative-stressed amnion epithelial cell-derived exosomes. J. Reprod. Immunol. 123, 3–11. doi: 10.1016/j.jri.2017.08.003
Shi, B., Wang, Y., Zhao, R., Long, X., Deng, W., and Wang, Z. (2018). Bone marrow mesenchymal stem cell-derived exosomal miR-21 protects C-kit+ cardiac stem cells from oxidative injury through the PTEN/PI3K/Akt axis. PLoS One 13:e0191616. doi: 10.1371/journal.pone.0191616
Silva, S. L., Vaz, A. R., Barateiro, A., Falcão, A. S., Fernandes, A., Brito, M. A., et al. (2010). Features of bilirubin-induced reactive microglia: from phagocytosis to inflammation. Neurobiol. Dis. 40, 663–675. doi: 10.1016/j.nbd.2010.08.010
Silverman, J. M., Fernando, S. M., Grad, L. I., Hill, A. F., Turner, B. J., Yerbury, J. J., et al. (2016). Disease mechanisms in ALS: misfolded sod1 transferred through exosome-dependent and exosome-independent pathways. Cell. Mol. Neurobiol. 36, 377–381. doi: 10.1007/s10571-015-0294-3
Simões, A. E. S., Pereira, D. M., Gomes, S. E., Brito, H., Carvalho, T., French, A., et al. (2015). Aberrant MEK5/ERK5 signalling contributes to human colon cancer progression via NF-κB activation. Cell Death Dis. 6:e1718. doi: 10.1038/cddis.2015.83
Spiller, K. J., Restrepo, C. R., Khan, T., Dominique, M. A., Fang, T. C., Canter, R. G., et al. (2018). Microglia-mediated recovery from ALS-relevant motor neuron degeneration in a mouse model of TDP-43 proteinopathy. Nat. Neurosci. 21, 329–340. doi: 10.1038/s41593-018-0083-7
Stevens, J. C., Chia, R., Hendriks, W. T., Bros-Facer, V., van Minnen, J., Martin, J. E., et al. (2010). Modification of superoxide dismutase 1 (SOD1) properties by a GFP tag—implications for research into amyotrophic lateral sclerosis (ALS). PLoS One 5:e9541. doi: 10.1371/journal.pone.0009541
Su, W., Aloi, M. S., and Garden, G. A. (2016). MicroRNAs mediating CNS inflammation: small regulators with powerful potential. Brain Behav. Immun. 52, 1–8. doi: 10.1016/j.bbi.2015.07.003
Sundaramoorthy, V., Walker, A. K., Yerbury, J., Soo, K. Y., Farg, M. A., Hoang, V., et al. (2013). Extracellular wildtype and mutant SOD1 induces ER-Golgi pathology characteristic of amyotrophic lateral sclerosis in neuronal cells. Cell. Mol. Life Sci. 70, 4181–4195. doi: 10.1007/s00018-013-1385-2
Tang, Y., and Le, W. (2016). Differential roles of M1 and M2 microglia in neurodegenerative diseases. Mol. Neurobiol. 53, 1181–1194. doi: 10.1007/s12035-014-9070-5
Tili, E., Michaille, J.-J., Cimino, A., Costinean, S., Dumitru, C. D., Adair, B., et al. (2007). Modulation of miR-155 and miR-125b levels following lipopolysaccharide/TNF-α stimulation and their possible roles in regulating the response to endotoxin shock. J. Immunol. 179, 5082–5089. doi: 10.4049/jimmunol.179.8.5082
Vaz, A. R., Cunha, C., Gomes, C., Schmucki, N., Barbosa, M., and Brites, D. (2015). Glycoursodeoxycholic acid reduces matrix metalloproteinase-9 and caspase-9 activation in a cellular model of superoxide dismutase-1 neurodegeneration. Mol. Neurobiol. 51, 864–877. doi: 10.1007/s12035-014-8731-8
Wang, L., Deng, H. X., Grisotti, G., Zhai, H., Siddique, T., and Roos, R. P. (2009). Wild-type SOD1 overexpression accelerates disease onset of a G85R SOD1 mouse. Hum. Mol. Genet. 18, 1642–1651. doi: 10.1093/hmg/ddp085
Wang, M., Mungur, R., Lan, P., Wang, P., and Wan, S. (2018). MicroRNA-21 and microRNA-146a negatively regulate the secondary inflammatory response of microglia after intracerebral hemorrhage. Int. J. Clin. Exp. Pathol. 11, 3348–3356.
Yamaguchi, H., Takagi, J., Miyamae, T., Yokota, S., Fujimoto, T., Nakamura, S., et al. (2008). Milk fat globule EGF factor 8 in the serum of human patients of systemic lupus erythematosus. J. Leukoc. Biol. 83, 1300–1307. doi: 10.1189/jlb.1107730
Keywords: amyotrophic lateral sclerosis, mutant SOD1G93A, microglia reactivity, inflammatory-associated microRNAs, glycoursodeoxycholic acid, vinyl sulfone
Citation: Vaz AR, Pinto S, Ezequiel C, Cunha C, Carvalho LA, Moreira R and Brites D (2019) Phenotypic Effects of Wild-Type and Mutant SOD1 Expression in N9 Murine Microglia at Steady State, Inflammatory and Immunomodulatory Conditions. Front. Cell. Neurosci. 13:109. doi: 10.3389/fncel.2019.00109
Received: 31 October 2018; Accepted: 05 March 2019;
Published: 09 April 2019.
Edited by:
Raquel Ferreira, Universidade da Beira Interior, PortugalReviewed by:
Adrian Israelson, Ben-Gurion University of the Negev, IsraelCopyright © 2019 Vaz, Pinto, Ezequiel, Cunha, Carvalho, Moreira and Brites. This is an open-access article distributed under the terms of the Creative Commons Attribution License (CC BY). The use, distribution or reproduction in other forums is permitted, provided the original author(s) and the copyright owner(s) are credited and that the original publication in this journal is cited, in accordance with accepted academic practice. No use, distribution or reproduction is permitted which does not comply with these terms.
*Correspondence: Dora Brites, ZGJyaXRlc0BmZi51bGlzYm9hLnB0
Disclaimer: All claims expressed in this article are solely those of the authors and do not necessarily represent those of their affiliated organizations, or those of the publisher, the editors and the reviewers. Any product that may be evaluated in this article or claim that may be made by its manufacturer is not guaranteed or endorsed by the publisher.
Research integrity at Frontiers
Learn more about the work of our research integrity team to safeguard the quality of each article we publish.