- 1Department of Neuroscience, Achucarro Basque Center for Neuroscience, CIBERNED and University of Basque Country, Leioa, Spain
- 2Institute of Anatomy and Cell Biology, Università Cattolica del Sacro Cuore, Rome, Italy
Extracellular ATP, related nucleotides and adenosine are among the earliest signaling molecules, operating in virtually all tissues and cells. Through their specific receptors, namely purinergic P1 for nucleosides and P2 for nucleotides, they are involved in a wide array of physiological effects ranging from neurotransmission and muscle contraction to endocrine secretion, vasodilation, immune response, and fertility. The purinergic system also participates in the proliferation and differentiation of stem cells from different niches. In particular, both mesenchymal stem cells (MSCs) and neural stem cells are endowed with several purinergic receptors and ecto-nucleotide metabolizing enzymes, and release extracellular purines that mediate autocrine and paracrine growth/proliferation, pro- or anti-apoptotic processes, differentiation-promoting effects and immunomodulatory actions. Here, we discuss the often opposing roles played by ATP and adenosine in adult neurogenesis in both physiological and pathological conditions, as well as in adipogenic and osteogenic MSC differentiation. We also focus on how purinergic ligands produced and released by transplanted stem cells can be regarded as ideal candidates to mediate the crosstalk with resident stem cell niches, promoting cell growth and survival, regulating inflammation and, therefore, contributing to local tissue homeostasis and repair.
Purinergic Ligands are Ancient and Widespread Mediators of Cell-to-Cell Communication
It is now widely accepted that in adult organisms stem cells contribute to tissue homeostasis and repair through paracrine mechanisms, along with a mere integration into existing tissue architecture (Wang et al., 2014). Trophic factors combined with immunomodulatory molecules often represent the main mechanism responsible for the functional improvements exerted by transplanted stem cells (Uccelli et al., 2008; Leatherman, 2013). Released nucleotides and nucleosides behave as trophic, differentiating, and immunomodulatory molecules in many physiological and pathological events, through autocrine and paracrine mechanisms (Glaser et al., 2012). Phylogenetically, purinergic ligands are considered ancient molecules involved in cell-to-cell communication, and their receptors are expressed by almost every cell type, even in very primitive organisms such as prokaryotes, protozoa, and early plants (Burnstock and Verkhratsky, 2010). Purinergic receptors are also among the first neurotransmitter receptors to be expressed during very early stages of ontogenetic development (Burnstock and Ulrich, 2011). This conserved and widespread use of purinergic ligands for intercellular communication is possibly due to the fact that nucleotides (and ATP in particular) are fundamental constituents of cells, being the most widely used high energy carrier molecules, and because they are the building blocks of nucleic acids. Cells therefore usually contain millimolar concentrations of intracellular ATP that can be discharged into the extracellular space by vesicular exocytosis, concentrative, and equilibrative transporters, connexin/pannexin hemichannels and uncontrolled leakage from injured cells (Lohman et al., 2012).
Once released into the extracellular environment, purinergic ligands behave as signal mediators, activating different subtypes of purinergic receptors. There are four subtypes of adenosine P1 receptors (A1, A2A, A2B, and A3), seven subtypes of nucleotide P2X ligand-gated ion channel receptors (P2X1–7) and eight subtypes of nucleotide P2Y metabotropic receptors (P2Y1, P2Y2, P2Y4, P2Y6, P2Y11, P2Y12, P2Y13, and P2Y14). The P1 and P2Y subtypes are classical seven-transmembrane domain receptors, whose action is mediated through G-proteins and intracellular second messengers, including Ca2+, cAMP, and InsP3 (Burnstock, 2007).
The effects of ATP and adenosine are usually opposite and the resulting signal cascade activated by extracellular nucleotides and nucleosides in target cells is the combinatorial resultant of their extracellular metabolism, uptake and binding to specific receptors (Volonté and D’Ambrosi, 2009). Ecto-nucleotide metabolizing enzymes (in particular ecto-nucleoside triphosphate phosphohydrolases, and ecto-5′-nucleotidase) are powerful tools to control the effects mediated by extracellular purines, as they switch off the signal induced by ATP on P2 receptors, hydrolyzing it into adenosine, thereby activating P1 receptors.
Because of their widespread presence and the broad array of functions they can mediate, it is not surprising that purinergic receptors are involved in many aspects of stem cell physiology: mesenchymal stem cells (MSCs) and neuronal progenitor cells (NPCs) release and respond to purinergic ligands with altered proliferation, migration, differentiation and apoptosis, and by regulating immune responses associated with their mobilization (Burnstock and Ulrich, 2011). In this review we will analyze how purinergic signaling behaves as a common paracrine pathway that activates MSCs and neural stem cells (NSCs) in both physiological and pathological conditions.
Dual Role of the Purinergic System in NSCs in Physiological and Pathological Conditions
Extracellular Purines Modulate Adult Neurogenesis
Neural progenitor cells in adult brain express different purinergic receptors. Indeed, mRNAs for P2X4 and P2X7 subtypes, all P2Y receptors except P2Y4 and P2Y11, and all P1 receptors, but A3, have been found in subventricular zone (SVZ)-derived primary neurospheres (Stafford et al., 2007; Table 1). Moreover, neural progenitor cells of both SVZ and subgranular zone neurogenic niches highly express the nucleotide-metabolizing enzymes ectonucleoside triphosphate diphosphohydrolase (NTPDase) 2 and the tissue-non-specific alkaline phosphatase (TNAP; Langer et al., 2007). Extracellular nucleotides generated by these enzymes in the SVZ produce a rapid and transient increase in intracellular calcium mainly through the activation of the metabotropic P2Y1 receptor (Mishra et al., 2006). The role of P2Y1 in modulating neurogenesis changes depending on the physiological conditions and the concomitant presence of EGF and FGF. In fact, specific stimulation of this receptor in NPCs increases cell proliferation and migration (Grimm et al., 2010), but only when the growth factor concentration is low or absent (Mishra et al., 2006; Boccazzi et al., 2014; Table 1; Figure 1A). Conversely, when the growth factor concentration is higher, activation of P2Y1 has an antiproliferative effect (Stafford et al., 2007; Table 1). It was recently demonstrated that infusion of ATP in rat SVZ selectively increases the proliferation of type C cells but not of type B or A (Suyama et al., 2012). This effect is counteracted by the selective P2Y1 antagonist 20-deoxy-N6-methyladenosine-30,50-bisphosphate (MRS2179) suggesting a specific role of the P2Y1 receptor in modulating the activity of transit amplifying cells. In line with this, an additional indication of P2Y1 receptor functioning comes from evidence that ATP secreted by astrocytes, even at basal levels, promotes the proliferation of neural progenitor cells through activation of the P2Y1 subunit (Cao et al., 2013; Figure 1A).
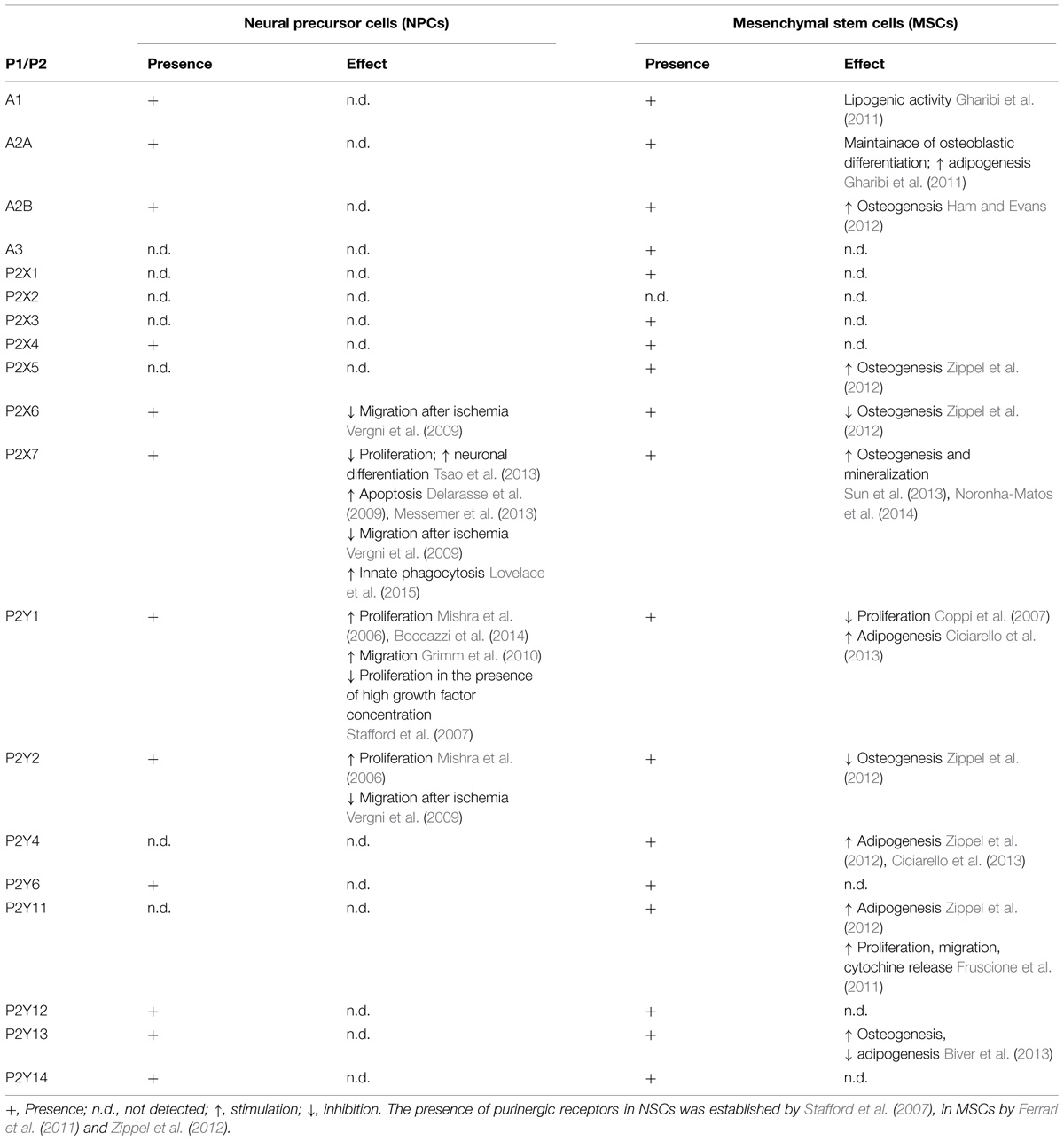
TABLE 1. Presence and function of purinergic P1 and P2 receptors in neural precursor cells and mesenchymal stem cells.
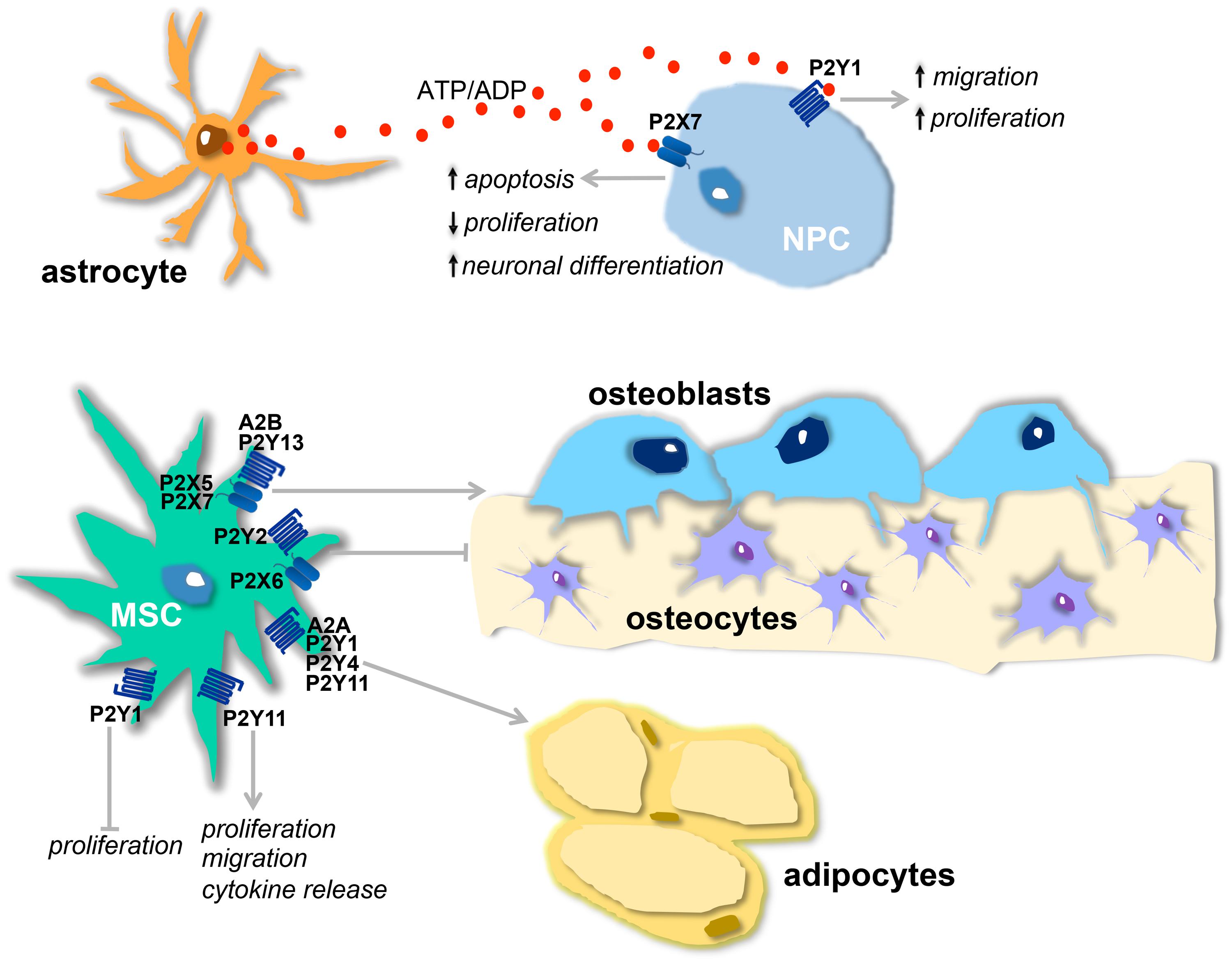
FIGURE 1. Physiological effects of purinergic receptors in neural and mesenchymal stem cells (MSCs). (A) Proposed model of purinergic receptor action on neurogenesis: ATP, released from astrocytes, and ADP, resulting from ATP hydrolysis, stimulate, respectively, P2X7 and P2Y1 receptors present on neural stem cells (NPCs). The activation of P2Y1 receptor leads to increased proliferation and migration and this effect is counterbalanced by P2X7 activation that decreases proliferation, induces neuronal differentiation, and apoptosis. (B) Osteogenic and adipogenic actions of purinergic receptors present on MSCs : A2B, P2Y13, P2X5, and P2X7 receptors stimulate osteogenesis, while P2X6 and P2Y2 are inhibitory. A2A, P2Y1, P2Y4 and P2Y11 receptors are adipogenic. P2Y11 receptor also induces migration, cytokine release, and proliferation. Proliferation is inhibited by P2Y1 receptor.
The effect of P2Y1 in stimulating the proliferation of progenitor cells and neurogenesis can be counterbalanced by activation of the P2X7 receptor (Figure 1A). This receptor subtype can regulate the homeostasis of the neurogenic niche, limiting excessive neuro- and glio-genesis by inhibiting proliferation and stimulating NPC differentiation (Tsao et al., 2013) and activating apoptotic mechanisms (Delarasse et al., 2009; Table 1). The P2X7 receptor expressed on neuroblasts can also contribute to the clearance of apoptotic cells by activating innate phagocytosis during early stages of neurogenesis (Lovelace et al., 2015; Table 1).
Extracellular Purines Affect NSC Response in Pathological Conditions
Massive release of extracellular ATP is one of the hallmarks of neurodegeneration. After a pathological event in the brain, such as ischemia or Parkinson’s disease all CNS cell types activate different purinergic receptors. P2X7, which is expressed mainly in microglia, astrocytes, and neurons, is the principal agent responsible for purinergic-induced excitotoxic cell death (Sperlagh et al., 2006). Activation of P2X7 in pathological conditions in neurons and astrocytes induces the formation of large pores which, together with pannexin channels, allow the passage of cations, the leakage of metabolites of up to 900 Da and further release of ATP. During an insult extracellular ATP can achieve millimolar concentrations in the extracellular space, determining sustained activation of purinergic receptors and an increase in intracellular calcium in target cells. The imbalance of calcium homeostasis in microglia results in the release of different interleukins, triggering a neuroinflammatory reaction (Sperlagh et al., 2006). However, the role of neuroinflammation in modulating neurogenesis during a pathological event is still debated. Inflammatory cytokines have both a positive and a negative effect on neurogenesis (Borsini et al., 2015) and the activation of purinergic receptors on microglia and astrocytes plays a relevant role in modulating their release. For example, microglial P2X7 activated by its specific agonists ATP and benzoyl-ATP during neuronal stress modulates the expression of NOD-like receptor (NLR) P3 inflammasome (Franceschini et al., 2015), sustaining the release of proinflammatory cytokines which, in turn, may contribute to the inhibition of progenitor cell activity. Conversely, the increase in P2X4 expression in astrocytes contributes to CNS remodeling after trauma and further increases synaptogenesis (Franke and Illes, 2006). Brain ischemia is also characterized by the release of inflammatory cytokines. After an ischemic insult the SVZ is able to release factors that can protect against cortical damage (Cavaliere et al., 2006) and the purinergic system can inhibit this function. Indeed, ATP released after brain insult overstimulates P2 receptors expressed in SVZ progenitor cells (mainly P2X6, P2X7, P2Y1, and P2Y2; Stafford et al., 2007; Vergni et al., 2009), inhibiting the migration of neuroblasts to the damaged cortex (Table 1). This process is further enhanced by a locally decreased production of the chemoattractant SDf-1alpha and may also be reversed by blocking the activation of microglia (Vergni et al., 2009). In this case, purines, together with other death signals released by damaged cells, counterbalance the response of progenitor cells recruited after damage (Messemer et al., 2013; Table 1).
The general assumption is that, during an insult, ATP can act as a detrimental pro-inflammatory signal, whereas adenosine, mainly through A1 and A3 receptors, usually has opposite properties (Fiebich et al., 2014). It is well known that ATP released after brain injury can be hydrolized by NTPDase2, which is highly expressed in the neural progenitor cell membrane (Gampe et al., 2015), and generate adenosine that, together with the adenosine released directly during brain damage, also has a modulatory effect on neurogenesis (Ulrich et al., 2012).
Finally, an important role in the modulation of NSC function following a stressful event is also exerted by orphan G protein-coupled receptors, which can be activated by extracellular nucleotides. This is the case of GPR17, a novel P2Y receptor specifically activated by both uracil nucleotides (UDP, UDP-glucose, and UDP-galactose) and cysteinyl-leukotrienes (cysLTs; Blasius et al., 1998; Ciana et al., 2006). GPR17 is also expressed in neural progenitor cells, mainly oligodendrocyte precursor cells, and acts as a regulatory factor in mediating oligodendrocyte response and neuronal death after brain ischemia (Lecca et al., 2008).
Purinergic Signaling in MSCs
Mesenchymal stem cells are self-renewing multipotent stem cells with the capacity to differentiate into chondrocytes, osteoblasts, or adipocytes. Numerous studies have shown that many molecules, inorganic compounds, and mechanical agents contribute to their commitment in the different lineages and it is now clear that there is an inverse relationship between their differentiation into osteoblatsts and into adipocytes. This balance is regulated by intersecting signaling pathways that converge on the regulation of two main transcription factors: peroxisome proliferator-activated receptor-γ (PPARγ) and Runt-related transcription factor 2 (Runx2), which are generally regarded as the master regulators of adipogenesis and osteogenesis, respectively (James, 2013).
Purinergic ligands have been widely described as early factors determining MSC fate (Glaser et al., 2012; Scarfi, 2014) but, while the role of the P1 receptors in MSC physiology is fairly clearly defined, the function of P2 receptors is more controversial, possibly because most of the 15 P2 receptor subtypes have been identified on MSCs (Zippel et al., 2012), it is often difficult to separate the effects of ATP from those of adenosine, and their function seems also to be influenced by the source of origin of the cells. To simplify, ATP can be considered both adipogenic and osteogenic, while its degradation product, adenosine, switches off adipogenic differentiation and has a prevalently osteogenic action (Gharibi et al., 2012; Ciciarello et al., 2013).
P1 Receptors on MSCs are Mostly Osteogenic and Immunomodulatory
Mesenchymal stem cells release adenosine and possess all P1 receptors (Evans et al., 2006), with A2B as the predominant subtype in undifferentiated cells and during osteoblastogenesis (Gharibi et al., 2011). Not only is adenosine released but most of it derives from the hydrolysis of ATP by ectonucleoside triphosphate diphosphohydrolase 1 (CD39) and ecto-5′-nucleotidase (CD73) activities that are abundantly present in the plasma membrane of MSCs (Sattler et al., 2011). Adenosine exerts an osteogenic action (Ham and Evans, 2012) mainly via the A2B receptor (Table 1; Figure 1B), its effects being canceled on pharmacological inhibition of this receptor subtype (He et al., 2013), and since overexpression of A2B receptors induces the synthesis of osteoblast-related genes (Runx2 and alkaline phosphatase; Gharibi et al., 2011). Consistently with these in vitro results, the knockout of CD73 in mice decreases osteoblast differentiation, resulting in osteopenia (Takedachi et al., 2012); A2B-deficient mice show impaired osteogenic differentiation, a mild osteopenic phenotype and impaired fracture physiology (Carroll et al., 2012); finally, loss of equilibrative nucleoside transporter 1 (ENT1) in mice, with consequent inhibition of adenosine reuptake, leads to ectopic calcification of spinal tissues (Warraich et al., 2013). Adenosine formation and activation of A2B receptors has also been strongly implicated in osteogenic differentiation induced by biomaterials containing calcium phosphate moieties (Shih et al., 2014). The A2A subunit has also been implicated in osteogenesis, being involved mainly in the maintenance of osteoblastic differentiation (Table 1) and this P1 subunit, together with the A1 receptor subtype, is also found upregulated during adipogenesis, influencing, respectively, differentiation (through upregulation of PPARγ; Figure 1) and lipogenic activity (Gharibi et al., 2011; Table 1).
The regenerative effects of MSCs largely depend on their capacity to regulate inflammation and tissue homeostasis via the secretion of an array of immunosuppressive factors, cytokines and growth and differentiation factors that may inhibit inflammatory responses and facilitate the proliferation and differentiation of progenitor cells in tissues in situ. P1 receptors are also involved in this aspect of MSC physiology following a pathological insult, being implicated in tissue repair and wound healing by stimulating local repair mechanisms and enhancing the accumulation of endothelial progenitor cells (Katebi et al., 2009). Released adenosine usually displays direct anti-inflammatory effects (Hasko and Pacher, 2008) blocking the proliferation of T-lymphocytes mainly through the A2A subtype, and the addition of A2A antagonists or CD39 inhibitors significantly counteracts this effect (Saldanha-Araujo et al., 2011; Sattler et al., 2011; Lee et al., 2014).
P2 Receptors have Pleiotropic Effects in MSCs
Human MSCs have been reported spontaneously to release ATP (Coppi et al., 2007) which, in a paracrine way, initiates and propagates intracellular Ca2+ waves, promoting the activation of transcription factors that are involved in cell differentiation (Kawano et al., 2006). ATP inhibits the proliferation of bone marrow (BM)-MSCs (Coppi et al., 2007) and stimulates their migration (Ferrari et al., 2011) and PPARγ levels through the activation of different P2X and P2Y receptor subunits (Omatsu-Kanbe et al., 2006; Zippel et al., 2012; Ciciarello et al., 2013; Table 1; Figure 1B). Together with this adipogenic role for extracellular nucleotides, it was recently demonstrated that P2 receptors are also involved in osteogenesis (Table 1) and up- or down-regulation of different P2 subtypes was observed in adipogenic and osteogenic differentiation of MSCs derived from adipose tissue and dental follicles (Zippel et al., 2012). In particular, P2Y13-deficient mice exhibit a decreased bone turnover associated with a reduction in the number of both osteoblasts and osteoclasts (Wang et al., 2014) and MSCs derived from these mice undergo a preferential adipogenic differentiation, showing that the P2Y13 receptor physiologically stimulates the differentiation of osteoblasts (Figure 1B) and inhibits that of adipocytes (Biver et al., 2013; Table 1). P2X7 receptor activation in BM-MSCs from post-menopausal women and following shockwave treatment also promotes osteogenic differentiation and mineralization (Sun et al., 2013; Noronha-Matos et al., 2014; Table 1; Figure 1B). Finally, it has been demonstrated that activation of P2Y11 receptor by NAD+ released from connexin hemichannels increases proliferation, migration, and cytokine release in BM-MSCs, sparing in this case osteogenic and adipogenic differentiation markers (Fruscione et al., 2011; Table 1; Figure 1B).
Purinergic Ligands may be Involved in the Crosstalk between NSCs and MSCs
In this review we have described how purinergic signaling is involved in the physiology of NSCs and MSCs, as both cell types produce and respond to nucleotides and nucleosides. Although purinergic receptors can mediate different effects in the two cell niches (Figure 1), in both cases purinergic signaling converges in the modulation of the immune response that is at the basis of stem cell recruitment, in particular after a stressful insult. The activation of P1 receptors is mainly immunosuppressive and trophic for stem cells, while the stimulation of P2 receptors is often proinflammatory and can enhance cell death pathways. Purinergic ligands produced and released by transplanted stem cells can behave as ideal candidates in promoting in situ cell growth and decreased apoptosis and in regulating inflammation. For example, although at present there is little evidence of transdifferentiation of MSCs into neurons, it is believed that the secretome of transplanted MSCs can empower surrounding cells to facilitate tissue repair also in CNS pathologies such as stroke, Parkinson’s disease, traumatic brain injury, and epilepsy (Kim et al., 2009; Joyce et al., 2010). With regard to epilepsy, a large body of literature demonstrates the supporting role of adenosine as an endogenous anticonvulsant agent involved in anti-epileptic and anti-apoptotic functions, also by promoting neurogenesis (Glaser et al., 2012; Boison, 2013). Although numerous adenosine agonists have been shown to be potent anticonvulsants in a wide array of animal models of epilepsy, they often produce serious systemic adverse events. An alternative strategy under investigation is to transplant MSCs engineered to release high amounts of adenosine in several models of epilepsy, in order to enhance the natural adenosinergic mechanism triggered by seizures. This approach is very attractive as it provides large amounts of adenosine in loco, limiting its action to the foci of seizure and it has indeed proved successful, as engineered MSCs produce a local boost of adenosine and trigger anti-epileptic and anti-apoptotic effects (Boison, 2009; Li et al., 2009; Huicong et al., 2013).
In an acute optic nerve injury model it was shown that MSCs exert neuroprotective and anti-inflammatory effects, also through the down-regulation of the P2X7 receptor in retinal ganglion cells (Chen et al., 2013). Conversely, it was recently shown that ATP released from light-depolarized astrocytes promotes the neuronal differentiation of MSCs through the activation of P2X receptors in vitro and in vivo (Tu et al., 2014). It is evident from these results that purinergic ligands activate shared pathways that can be involved in MSC and NSC crosstalk, thus allowing mesenchymal and neurogenic niches to become closer.
Conflict of Interest Statement
The authors declare that the research was conducted in the absence of any commercial or financial relationships that could be construed as a potential conflict of interest.
Acknowledgments
We are grateful to Professor Fabrizio Michetti for critical reading of the manuscript and to Margaret Starace for English editing. FC is supported by the Spanish Ministry of Economy (SAF2009-13463, SAF2013-45084-R), University of País Vasco (UPV/EHU), and CIBERNED. ND is funded by UCSC (linea D.1 2014 grant # 70201184).
References
Biver, G., Wang, N., Gartland, A., Orriss, I., Arnett, T. R., Boeynaems, J. M., et al. (2013). Role of the P2Y13 receptor in the differentiation of bone marrow stromal cells into osteoblasts and adipocytes. Stem Cells 31, 2747–2758. doi: 10.1002/stem.1411
Blasius, R., Weber, R. G., Lichter, P., and Ogilvie, A. (1998). A novel orphan G protein-coupled receptor primarily expressed in the brain is localized on human chromosomal band 2q21. J. Neurochem. 70, 1357–1365. doi: 10.1046/j.1471-4159.1998.70041357.x
Boccazzi, M., Rolando, C., Abbracchio, M. P., Buffo, A., and Ceruti, S. (2014). Purines regulate adult brain subventricular zone cell functions: contribution of reactive astrocytes. Glia 62, 428–439. doi: 10.1002/glia.22614
Boison, D. (2009). Engineered adenosine-releasing cells for epilepsy therapy: human mesenchymal stem cells and human embryonic stem cells. Neurotherapeutics 6, 278–283. doi: 10.1016/j.nurt.2008.12.001
Boison, D. (2013). Role of adenosine in status epilepticus: a potential new target? Epilepsia 54(Suppl. 6), 20–22. doi: 10.1111/epi.12268
Borsini, A., Zunszain, P. A., Thuret, S., and Pariante, C. M. (2015). The role of inflammatory cytokines as key modulators of neurogenesis. Trends Neurosci. 38, 145–157. doi: 10.1016/j.tins.2014.12.006
Burnstock, G. (2007). Purine and pyrimidine receptors. Cell. Mol. Life Sci. 64, 1471–1483. doi: 10.1007/s00018-007-6497-0
Burnstock, G., and Ulrich, H. (2011). Purinergic signaling in embryonic and stem cell development. Cell. Mol. Life Sci. 68, 1369–1394. doi: 10.1007/s00018-010-0614-1
Burnstock, G., and Verkhratsky, A. (2010). Long-term (trophic) purinergic signalling: purinoceptors control cell proliferation, differentiation and death. Cell Death Dis. 1:e9. doi: 10.1038/cddis.2009.11
Cao, X., Li, L. P., Qin, X. H., Li, S. J., Zhang, M., Wang, Q., et al. (2013). Astrocytic adenosine 5′-triphosphate release regulates the proliferation of neural stem cells in the adult hippocampus. Stem Cells 31, 1633–1643. doi: 10.1002/stem.1408
Carroll, S. H., Wigner, N. A., Kulkarni, N., Johnston-Cox, H., Gerstenfeld, L. C., and Ravid, K. (2012). A2B adenosine receptor promotes mesenchymal stem cell differentiation to osteoblasts and bone formation in vivo. J. Biol. Chem. 287, 15718–15727. doi: 10.1074/jbc.M112.344994
Cavaliere, F., Dinkel, K., and Reymann, K. (2006). The subventricular zone releases factors which can be protective in oxygen/glucose deprivation-induced cortical damage: an organotypic study. Exp. Neurol. 201, 66–74. doi: 10.1016/j.expneurol.2006.03.020
Chen, M., Xiang, Z., and Cai, J. (2013). The anti-apoptotic and neuro-protective effects of human umbilical cord blood mesenchymal stem cells (hUCB-MSCs) on acute optic nerve injury is transient. Brain Res. 1532, 63–75. doi: 10.1016/j.brainres.2013.07.037
Ciana, P., Fumagalli, M., Trincavelli, M. L., Verderio, C., Rosa, P., Lecca, D., et al. (2006). The orphan receptor GPR17 identified as a new dual uracil nucleotides/cysteinyl-leukotrienes receptor. EMBO J. 25, 4615–4627. doi: 10.1038/sj.emboj.7601341
Ciciarello, M., Zini, R., Rossi, L., Salvestrini, V., Ferrari, D., Manfredini, R., et al. (2013). Extracellular purines promote the differentiation of human bone marrow-derived mesenchymal stem cells to the osteogenic and adipogenic lineages. Stem Cells Dev. 22, 1097–1111. doi: 10.1089/scd.2012.0432
Coppi, E., Pugliese, A. M., Urbani, S., Melani, A., Cerbai, E., Mazzanti, B., et al. (2007). ATP modulates cell proliferation and elicits two different electrophysiological responses in human mesenchymal stem cells. Stem Cells 25, 1840–1849. doi: 10.1634/stemcells.2006-0669
Delarasse, C., Gonnord, P., Galante, M., Auger, R., Daniel, H., Motta, I., et al. (2009). Neural progenitor cell death is induced by extracellular ATP via ligation of P2X7 receptor. J. Neurochem. 109, 846–857. doi: 10.1111/j.1471-4159.2009.06008.x
Evans, B. A., Elford, C., Pexa, A., Francis, K., Hughes, A. C., Deussen, A., et al. (2006). Human osteoblast precursors produce extracellular adenosine, which modulates their secretion of IL-6 and osteoprotegerin. J. Bone Miner. Res. 21, 228–236. doi: 10.1359/JBMR.051021
Ferrari, D., Gulinelli, S., Salvestrini, V., Lucchetti, G., Zini, R., Manfredini, R., et al. (2011). Purinergic stimulation of human mesenchymal stem cells potentiates their chemotactic response to CXCL12 and increases the homing capacity and production of proinflammatory cytokines. Exp. Hematol. 39, 360–374, 374.e1-5. doi: 10.1016/j.exphem.2010.12.001
Fiebich, B. L., Akter, S., and Akundi, R. S. (2014). The two-hit hypothesis for neuroinflammation: role of exogenous ATP in modulating inflammation in the brain. Front. Cell. Neurosci 8:260. doi: 10.3389/fncel.2014.00260
Franceschini, A., Capece, M., Chiozzi, P., Falzoni, S., Sanz, J. M., Sarti, A. C., et al. (2015). The P2X7 receptor directly interacts with the NLRP3 inflammasome scaffold protein. FASEB J. doi: 10.1096/fj.14-268714 [Epub ahead of print].
Franke, H., and Illes, P. (2006). Involvement of P2 receptors in the growth and survival of neurons in the CNS. Pharmacol. Ther. 109, 297–324. doi: 10.1016/j.pharmthera.2005.06.002
Fruscione, F., Scarfi, S., Ferraris, C., Bruzzone, S., Benvenuto, F., Guida, L., et al. (2011). Regulation of human mesenchymal stem cell functions by an autocrine loop involving NAD+ release and P2Y11-mediated signaling. Stem Cells Dev. 20, 1183–1198. doi: 10.1089/scd.2010.0295
Gampe, K., Stefani, J., Hammer, K., Brendel, P., Potzsch, A., Enikolopov, G., et al. (2015). NTPDase2 and purinergic signaling control progenitor cell proliferation in neurogenic niches of the adult mouse brain. Stem Cells 33, 253–264. doi: 10.1002/stem.1846
Gharibi, B., Abraham, A. A., Ham, J., and Evans, B. A. (2011). Adenosine receptor subtype expression and activation influence the differentiation of mesenchymal stem cells to osteoblasts and adipocytes. J. Bone Miner. Res. 26, 2112–2124. doi: 10.1002/jbmr.424
Gharibi, B., Abraham, A. A., Ham, J., and Evans, B. A. (2012). Contrasting effects of A1 and A2b adenosine receptors on adipogenesis. Int J. Obes. (Lond.) 36, 397–406. doi: 10.1038/ijo.2011.129
Glaser, T., Cappellari, A. R., Pillat, M. M., Iser, I. C., Wink, M. R., Battastini, A. M., et al. (2012). Perspectives of purinergic signaling in stem cell differentiation and tissue regeneration. Purinergic Signal. 8, 523–537. doi: 10.1007/s11302-011-9282-3
Grimm, I., Ullsperger, S. N., and Zimmermann, H. (2010). Nucleotides and epidermal growth factor induce parallel cytoskeletal rearrangements and migration in cultured adult murine neural stem cells. Acta Physiol. (Oxf.) 199, 181–189. doi: 10.1111/j.1748-1716.2010.02092.x
Ham, J., and Evans, B. A. (2012). An emerging role for adenosine and its receptors in bone homeostasis. Front. Endocrinol. (Lausanne) 3:113. doi: 10.3389/fendo.2012.00113
Hasko, G., and Pacher, P. (2008). A2A receptors in inflammation and injury: lessons learned from transgenic animals. J. Leukoc. Biol. 83, 447–455. doi: 10.1189/jlb.0607359
He, W., Mazumder, A., Wilder, T., and Cronstein, B. N. (2013). Adenosine regulates bone metabolism via A1, A2A, and A2B receptors in bone marrow cells from normal humans and patients with multiple myeloma. FASEB J. 27, 3446–3454. doi: 10.1096/fj.13-231233
Huicong, K., Zheng, X., Furong, W., Zhouping, T., Feng, X., Qi, H., et al. (2013). The imbalanced expression of adenosine receptors in an epilepsy model corrected using targeted mesenchymal stem cell transplantation. Mol. Neurobiol. 48, 921–930. doi: 10.1007/s12035-013-8480-0
James, A. W. (2013). Review of signaling pathways governing MSC osteogenic and adipogenic differentiation. Scientifica (Cairo) 2013, 684736. doi: 10.1155/2013/684736
Joyce, N., Annett, G., Wirthlin, L., Olson, S., Bauer, G., and Nolta, J. A. (2010). Mesenchymal stem cells for the treatment of neurodegenerative disease. Regen. Med. 5, 933–946. doi: 10.2217/rme.10.72
Katebi, M., Soleimani, M., and Cronstein, B. N. (2009). Adenosine A2A receptors play an active role in mouse bone marrow-derived mesenchymal stem cell development. J. Leukoc. Biol. 85, 438–444. doi: 10.1189/jlb.0908520
Kawano, S., Otsu, K., Kuruma, A., Shoji, S., Yanagida, E., Muto, Y., et al. (2006). ATP autocrine/paracrine signaling induces calcium oscillations and NFAT activation in human mesenchymal stem cells. Cell Calcium 39, 313–324. doi: 10.1016/j.ceca.2005.11.008
Kim, Y. J., Park, H. J., Lee, G., Bang, O. Y., Ahn, Y. H., Joe, E., et al. (2009). Neuroprotective effects of human mesenchymal stem cells on dopaminergic neurons through anti-inflammatory action. Glia 57, 13–23. doi: 10.1002/glia.20731
Langer, D., Ikehara, Y., Takebayashi, H., Hawkes, R., and Zimmermann, H. (2007). The ectonucleotidases alkaline phosphatase and nucleoside triphosphate diphosphohydrolase 2 are associated with subsets of progenitor cell populations in the mouse embryonic, postnatal and adult neurogenic zones. Neuroscience 150, 863–879. doi: 10.1016/j.neuroscience.2007.07.064
Leatherman, J. (2013). Stem cells supporting other stem cells. Front. Genet. 4:257. doi: 10.3389/fgene.2013.00257
Lecca, D., Trincavelli, M. L., Gelosa, P., Sironi, L., Ciana, P., Fumagalli, M., et al. (2008). The recently identified P2Y-like receptor GPR17 is a sensor of brain damage and a new target for brain repair. PLoS ONE 3:e3579. doi: 10.1371/journal.pone.0003579
Lee, J. J., Jeong, H. J., Kim, M. K., Wee, W. R., Lee, W. W., Kim, S. U., et al. (2014). CD39-mediated effect of human bone marrow-derived mesenchymal stem cells on the human Th17 cell function. Purinergic Signal. 10, 357–365. doi: 10.1007/s11302-013-9385-0
Li, T., Ren, G., Kaplan, D. L., and Boison, D. (2009). Human mesenchymal stem cell grafts engineered to release adenosine reduce chronic seizures in a mouse model of CA3-selective epileptogenesis. Epilepsy Res. 84, 238–241. doi: 10.1016/j.eplepsyres.2009.01.002
Lohman, A. W., Billaud, M., and Isakson, B. E. (2012). Mechanisms of ATP release and signalling in the blood vessel wall. Cardiovasc. Res. 95, 269–280. doi: 10.1093/cvr/cvs187
Lovelace, M. D., Gu, B. J., Eamegdool, S. S., Weible, M. W. II, Wiley, J. S., Allen, D. G., et al. (2015). P2X7 receptors mediate innate phagocytosis by human neural precursor cells and neuroblasts. Stem Cells 33, 526–541. doi: 10.1002/stem.1864
Messemer, N., Kunert, C., Grohmann, M., Sobottka, H., Nieber, K., Zimmermann, H., et al. (2013). P2X7 receptors at adult neural progenitor cells of the mouse subventricular zone. Neuropharmacology 73, 122–137. doi: 10.1016/j.neuropharm.2013.05.017
Mishra, S. K., Braun, N., Shukla, V., Fullgrabe, M., Schomerus, C., Korf, H. W., et al. (2006). Extracellular nucleotide signaling in adult neural stem cells: synergism with growth factor-mediated cellular proliferation. Development 133, 675–684. doi: 10.1242/dev.02233
Noronha-Matos, J. B., Coimbra, J., Sa-E-Sousa, A., Rocha, R., Marinhas, J., Freitas, R., et al. (2014). P2X7-induced zeiosis promotes osteogenic differentiation and mineralization of postmenopausal bone marrow-derived mesenchymal stem cells. FASEB J. 28, 5208–5222. doi: 10.1096/fj.14-257923
Omatsu-Kanbe, M., Inoue, K., Fujii, Y., Yamamoto, T., Isono, T., Fujita, N., et al. (2006). Effect of ATP on preadipocyte migration and adipocyte differentiation by activating P2Y receptors in 3T3-L1 cells. Biochem. J. 393, 171–180. doi: 10.1042/BJ20051037
Saldanha-Araujo, F., Ferreira, F. I., Palma, P. V., Araujo, A. G., Queiroz, R. H., Covas, D. T., et al. (2011). Mesenchymal stromal cells up-regulate CD39 and increase adenosine production to suppress activated T-lymphocytes. Stem Cell Res. 7, 66–74. doi: 10.1016/j.scr.2011.04.001
Sattler, C., Steinsdoerfer, M., Offers, M., Fischer, E., Schierl, R., Heseler, K., et al. (2011). Inhibition of T-cell proliferation by murine multipotent mesenchymal stromal cells is mediated by CD39 expression and adenosine generation. Cell Transplant. 20, 1221–1230. doi: 10.3727/096368910X546553
Scarfi, S. (2014). Purinergic receptors and nucleotide processing ectoenzymes: their roles in regulating mesenchymal stem cell functions. World J. Stem Cells 6, 153–162. doi: 10.4252/wjsc.v6.i2.153
Shih, Y. R., Hwang, Y., Phadke, A., Kang, H., Hwang, N. S., Caro, E. J., et al. (2014). Calcium phosphate-bearing matrices induce osteogenic differentiation of stem cells through adenosine signaling. Proc. Natl. Acad. Sci. U.S.A. 111, 990–995. doi: 10.1073/pnas.1321717111
Sperlagh, B., Vizi, E. S., Wirkner, K., and Illes, P. (2006). P2X7 receptors in the nervous system. Prog. Neurobiol. 78, 327–346. doi: 10.1016/j.pneurobio.2006.03.007
Stafford, M. R., Bartlett, P. F., and Adams, D. J. (2007). Purinergic receptor activation inhibits mitogen-stimulated proliferation in primary neurospheres from the adult mouse subventricular zone. Mol. Cell. Neurosci. 35, 535–548. doi: 10.1016/j.mcn.2007.04.013
Sun, D., Junger, W. G., Yuan, C., Zhang, W., Bao, Y., Qin, D., et al. (2013). Shockwaves induce osteogenic differentiation of human mesenchymal stem cells through ATP release and activation of P2X7 receptors. Stem Cells 31, 1170–1180. doi: 10.1002/stem.1356
Suyama, S., Sunabori, T., Kanki, H., Sawamoto, K., Gachet, C., Koizumi, S., et al. (2012). Purinergic signaling promotes proliferation of adult mouse subventricular zone cells. J. Neurosci. 32, 9238–9247. doi: 10.1523/JNEUROSCI.4001-11.2012
Takedachi, M., Oohara, H., Smith, B. J., Iyama, M., Kobashi, M., Maeda, K., et al. (2012). CD73-generated adenosine promotes osteoblast differentiation. J. Cell. Physiol. 227, 2622–2631. doi: 10.1002/jcp.23001
Tsao, H. K., Chiu, P. H., and Sun, S. H. (2013). PKC-dependent ERK phosphorylation is essential for P2X7 receptor-mediated neuronal differentiation of neural progenitor cells. Cell Death Dis. 1, e751. doi: 10.1038/cddis.2013.274
Tu, J., Yang, F., Wan, J., Liu, Y., Zhang, I., Wu, B., et al. (2014). Light-controlled astrocytes promote human mesenchymal stem cells toward neuronal differentiation and improve the neurological deficit in stroke rats. Glia 62, 106–121. doi: 10.1002/glia.22590
Uccelli, A., Moretta, L., and Pistoia, V. (2008). Mesenchymal stem cells in health and disease. Nat. Rev. Immunol. 8, 726–736. doi: 10.1038/nri2395
Ulrich, H., Abbracchio, M. P., and Burnstock, G. (2012). Extrinsic purinergic regulation of neural stem/progenitor cells: implications for CNS development and repair. Stem Cell Rev. 8, 755–767. doi: 10.1007/s12015-012-9372-9
Vergni, D., Castiglione, F., Briani, M., Middei, S., Alberdi, E., Reymann, K. G., et al. (2009). A model of ischemia-induced neuroblast activation in the adult subventricular zone. PLoS ONE 4:e5278. doi: 10.1371/journal.pone.0005278
Volonté, C., and D’Ambrosi, N. (2009). Membrane compartments and purinergic signalling: the purinome, a complex interplay among ligands, degrading enzymes, receptors and transporters. FEBS J. 276, 318–329. doi: 10.1111/j.1742-4658.2008.06793.x
Wang, Y., Chen, X., Cao, W., and Shi, Y. (2014). Plasticity of mesenchymal stem cells in immunomodulation: pathological and therapeutic implications. Nat. Immunol. 15, 1009–1016. doi: 10.1038/ni.3002
Warraich, S., Bone, D. B., Quinonez, D., Ii, H., Choi, D. S., Holdsworth, D. W., et al. (2013). Loss of equilibrative nucleoside transporter 1 in mice leads to progressive ectopic mineralization of spinal tissues resembling diffuse idiopathic skeletal hyperostosis in humans. J. Bone Miner. Res. 28, 1135–1149. doi: 10.1002/jbmr.1826
Keywords: purinergic receptors, ATP, adenosine, mesenchymal stem cells, neural stem cells
Citation: Cavaliere F, Donno C and D’Ambrosi N (2015) Purinergic signaling: a common pathway for neural and mesenchymal stem cell maintenance and differentiation. Front. Cell. Neurosci. 9:211. doi: 10.3389/fncel.2015.00211
Received: 31 March 2015; Accepted: 16 May 2015;
Published online: 02 June 2015.
Edited by:
Gerald W. Zamponi, University of Calgary, CanadaReviewed by:
Robert Weissert, University of Regensburg, GermanyStephen Ferguson, Western University, Canada
Copyright © 2015 Cavaliere, Donno and D’Ambrosi. This is an open-access article distributed under the terms of the Creative Commons Attribution License (CC BY). The use, distribution or reproduction in other forums is permitted, provided the original author(s) or licensor are credited and that the original publication in this journal is cited, in accordance with accepted academic practice. No use, distribution or reproduction is permitted which does not comply with these terms.
*Correspondence: Nadia D’Ambrosi, Institute of Anatomy and Cell Biology, Università Cattolica del Sacro Cuore, Largo Francesco Vito, 1, 00168 Rome, Italy, nadia.dambrosi@rm.unicatt.it