- 1Department of Life Science, University of Modena and Reggio Emilia, Modena, Italy
- 2Department of Pharmacological and Biomolecular Sciences, University of Milan, Milan, Italy
Changes in GABAergic inhibition occur during physiological processes, during response to drugs and in various pathologies. These changes can be achieved through direct allosteric modifications at the γ-amino butyric acid (GABA) type A (GABAA) receptor protein level, or by altering the synthesis, trafficking and stability of the receptor. Neurosteroids (NSs) and protein kinase C (PKC) are potent modulators of GABAA receptors and their effects are presumably intermingled, even though evidence for this hypothesis is only partially explored. However, several PKC isoforms are able to phosphorylate the GABAA receptor, producing different functional effects. We focused on the ε isoform, that has been correlated to the sensitivity of the GABAA receptor to allosteric modulators and whose expression may be regulated in peripheral sensory neurons by NSs. The cross-talk between PKC-ε and NSs, leading to changes in GABAA receptor functionality, is considered and discussed in this perspective.
Introduction
GABAA receptors are fundamental for fast synaptic inhibition in the brain. Given the role of these receptors in synaptic transmission, all the mechanisms that regulate their activity are of primary importance. One that is particularly significant is receptor phosphorylation. Kinases represent a superfamily of isoenzymes that, through protein phosphorylation, regulate several cellular processes, including proliferation, differentiation, tumorigenesis, cytoskeletal remodeling, receptor function and synaptic transmission modulation (Battaini, 2001). This superfamily comprises the protein kinase (PK) A, G and C, which are serine/threonine phosphotransferases.
Protein kinase C (PKC) is one of the most significant kinases for GABAA receptor modulation. Phosphorylation of the receptor can produce different effects, ranging from enhancement to inhibition of protein function, depending on the subtype of subunit targeted and on the location of the sites being phosphorylated (Moss and Smart, 1996).
In particular, among PKC enzymes there are conventional (α, βI, βII and Υ) and new (δ, ε, η and θ) isoforms (Nishizuka, 1995), that are differently expressed in the nervous system. The differences among PKC isoforms contribute to the large range of functions they may perform.
Some PKCs directly bind to the intracellular domain of specific GABAA receptor subunits, providing in this way a rapid regulation of the receptor activity by all the intracellular pathways that activate this kinase (Brandon et al., 1999).
PKC-Epsilon (PKC-ε)
PKC-ε is classified as a novel isoform present in the brain, found mainly in the cerebral cortex, cerebellum, hippocampus and, in small amount, in non-nervous peripheral tissues (Saito et al., 1993; Chen et al., 2000).
The characterization of PKC-ε null mice has provided insights into the role of PKC-ε in the central nervous system (CNS; Hodge et al., 1999). Animals lacking PKC-ε show hypersensitivity to the behavioral effects of allosteric modulators of GABAA receptors, such as ethanol and neurosteroids (NSs; Hodge et al., 2002). Among NSs, the progesterone metabolite Allopregnanolone (Allo) is one of the most important endogenous steroid in the CNS (Baulieu and Robel, 1990), as changes in its concentration correlate with physiological and pathological conditions(Maguire and Mody, 2007; Luchetti et al., 2011).
In vitro studies on cortical synaptosomes demonstrate that a peptide able to inhibit PKC-ε translocation (Khasar et al., 1999) produces an increase in Allo sensitivity (Hodge et al., 1999). Similarly, in primary cultures of cortical neurons Allo is more effective in potentiating GABA-evoked current when the cells are intracellularly perfused with this same inhibitory peptide (Figure 1A). From analysis of the dose-response curves of this Allo effect, it is evident that the potency of NSs remains unchanged after blocking PKC-ε translocation, whereas the efficacy is increased (Figure 1B). We cannot determine whether the receptors mediating the increased response to Allo were at synaptic or extrasynaptic sites. However, it is possible that a selective phosphorylation by PKC-ε occurs, in this case the kinase activity determines selective changes only in synaptic or in tonic currents. The use of a heterologous system, expressing different GABAA receptor subunits, or specific agonists for “tonic receptors” could provide the answer to this issue.
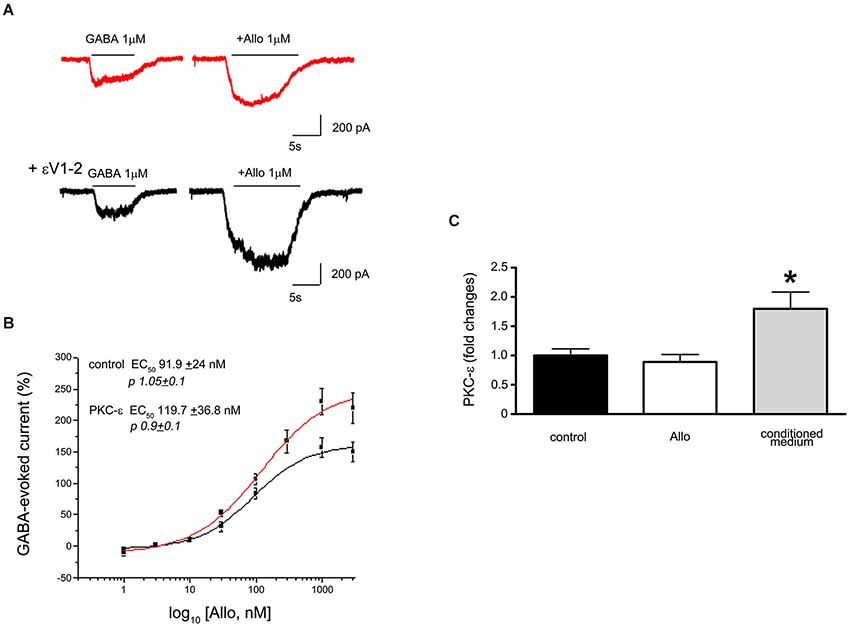
Figure 1. (A) Electrophysiological traces showing the effect of Allo on GABA-evoked currents recorded from cortical neurons in culture (7DIV). The upper trace shows a recording from a control cell (red) and the lower trace is from a cell intracellularly perfused with the peptide εV1–2, a PKC-ε inhibitor. (B) Dose response curves of Allo effect in control and in the presence of the peptide eV1–2. Each point is the mean+/−SE of 8–15 cells. PKC-ε blockade did not change the potency of Allo but affected its efficacy. (C) Assessment of PKC-ε gene expression changes (as fold changes) in primary culture of dorsal root ganglia (DRG) neuron after 24 h exposure to 10−6 M Allo (white column), or to the culture conditioned medium obtained from Schwann cell cultures treated with 10−6 M Allo (gray column). The relative quantification of mRNA was obtained by quantitative real time PCR. Data were normalized to the housekeeping genes α-tubulin and β2-microglobulin and expressed as difference (ΔΔCt) vs. controls, then averaged for each experimental group. The black column represents the controls (DRG neurons treated with vehicle, ethanol). Experiments were repeated at least three times (*p < 0.05 Anova Test).
Recent studies showed that PKC-ε action depends on the phosphorylation of the GABAA receptor at the level of Ser327 of the γ2 subunit (Qi et al., 2007), in turn regulating the response of the receptors to allosteric modulators. Furthermore, PKC-ε kinase controls GABAA receptor trafficking through the N-Ethylmaleimide-Sensitive Factor (NESF)-signaling pathway. Indeed, as suggested by the changes in Allo efficacy from in vitro studies (Figure 1B), the activation of PKC-ε is able to decrease cell surface expression of these receptors (Chou et al., 2010).
NSs and Phosphorylation
Allo, and other NSs that are positive modulators of GABAA receptors, potentiate GABA-evoked chloride current through an increase in the channel opening probability (Puia et al., 1990; Twyman and Macdonald, 1992; Zhu and Vicini, 1997). This results in a prolongation in the decay time of inhibitory post-synaptic currents (IPSCs; Harrison et al., 1987; Fáncsik et al., 2000). However, NSs also act on extrasynaptic GABAA receptors, causing large effects on δ-containing receptors that mediate the tonic current in certain brain regions (Belelli et al., 2002; Stell et al., 2003).
Interestingly, the desensitization of GABAA receptors plays an important role in NSs’ modulation (Zhu and Vicini, 1997), suggesting that the receptor needs to be in a “specific” state to be responsive to these endogenous NSs.
Furthermore, NSs produce long-lasting changes in the efficacy of GABAergic neurotransmission by modulating the phosphorylation of synaptic and extrasynaptic receptors. Indeed, in a recent paper, Abramian et al. showed a new molecular mechanism by which NSs change the efficacy of GABAergic inhibition by increasing surface expression of specific GABAA receptors (mainly containing α4 subunits), responsible for the tonic current in hippocampus (Abramian et al., 2014). They suggested that this component of GABAergic neurotransmission may be a key regulator of excitability. In this way the phosphorylation process may alter the function and/or trafficking of GABAA receptors, thus changing the efficacy of GABAA-mediated inhibition.
Interestingly other allosteric modulators of GABAA receptors, i.e., benzodiazepine, may also change GABA signaling, influencing the diffusion and clustering of receptors at synapses (Lévi et al., 2015). Conversely, it was shown that the potentiating effect of NSs can be decreased after stimulation of the PKC signaling pathway, either in physiological (Brussaard et al., 2000; Brussaard and Koksma, 2003; Maguire et al., 2005; Oberlander et al., 2012) or pathological conditions (Mtchedlishvili et al., 2001; Kia et al., 2011). For example, the phosphorylation state of the GABAA receptor changes during pregnancy or over the estrous cycle, a phenomenon that can compromise GABAA modulation by NS action.
The modulatory efficacy of NSs is decreased in dentate granule cells from epileptic rats (Mtchedlishvili et al., 2001). This agrees with the observation that brain and cell specific changes in GABAA receptors may occur in several epileptic models (Schwarzer et al., 1997; Fritschy et al., 1999; Peng et al., 2004) and in the temporal lobe of human epileptic subjects (Loup et al., 2000; Ferando and Mody, 2012). Recent studies show changes in the phosphorylation of GABAA receptors after kindling; these modifications together with changes in GABAA subunit composition could account for a decreased responsiveness to NSs (Kia et al., 2011; Carver et al., 2014). The diminished sensitivity to endogenous positive allosteric modulators, such as Allo, increases susceptibility to seizures, similarly to what happens in women with catamenial epilepsy, where seizures occur more frequently before the onset of menses and NS levels fall due to progesterone crash (Reddy, 2009).
Also important is the cross-talk among PKC-ε, GABAA receptors and NSs in pain perception. NS production is stimulated as a result of inflammatory pain (Poisbeau et al., 2005), and accordingly changes in PKC-ε expression were observed during pathological pain (Parada et al., 2003). Vergnano et al. proposed that the 3α-5α-NSs (such as Allo) could be part of an endogenous compensatory mechanism, in response to sustained activation of the spinal nociceptive system that occurs during pathological conditions (Vergnano et al., 2007).
The persistence of incoming pain messages, and the associated increases in intracellular Ca2+ concentration, could induce a strong stimulation of Ca2+-dependent PKC. This leads to a functional block of GABAA receptors in their current state. Therefore, PKC prevents further 3α-5α-NS dependent potentiation, without decreasing the basal modulatory effect of 3α-5α-NSs (Vergnano et al., 2007).
PKC-ε exerts a modulatory action on the pain pathways acting on CNS neurons, but also at the peripheral nervous system (PNS) level, for instance on dorsal root ganglia (DRG) neurons. Indeed, PKC-ε may alter the permeability of Na-type Ca2+ channels in DRG, and it has been shown to enhance nociception (Van Kolen et al., 2008). The capability of endogenous mediators to regulate PKC-ε gene expression has already been demonstrated for several molecules, including the neuropeptide ghrelin, thyroid hormones, the apolipoprotein E3 and some miRNAs (Rybin and Steinberg, 1996; Alipour et al., 2011; Sen et al., 2012). However, no data reported on the possible modulation exerted by NSs on PKC-ε gene expression. Recent studies by qRT-PCR analysis evaluated the possible modulation of PKC-ε expression in DRG neuronal cultures following 24-h treatment with Allo (10−6 M). Allo did not change PKC-ε expression under basal conditions, but was significantly upregulated in DRG neurons exposed to the culture medium from Allo-treated Schwann cells (Figure 1C). These findings suggest that Allo-treated Schwann cells can release one or more factors able to modulate PKC-ε expression in DRG neurons. However, Schwann cells also express basal levels of PKC-ε (Borghini et al., 1994). Overall, we speculate that these mechanisms identify novel putative circuits involved in the regulation of pain processes at PNS and spinal cord levels.
Given that Allo is considered one key factor in the modulation of peripheral pain pathways, its capability to regulate PKC-ε is promising and opens new perspectives for the identification of the basic mechanisms regulating chronic pain onset.
Conclusions
The activity of GABAA receptors must be finely regulated in the CNS. For this reason when NSs increase GABAA receptor activity, the “system” tries to re-equilibrate by activating different PKs. The increase in neuronal activity that occurs by activating L-type Ca2+ channels leads to a Ca2+/calmodulin-dependent PK type II phosphorylation of the GABAA receptor β3 subunit. This in turn produces a rapid insertion of receptors in the membrane, with a consequent increase in tonic current (Saliba et al., 2012). Similarly, NSs promote GABAA receptor phosphorylation, leading to an increase in extrasynaptic receptor expression (Abramian et al., 2014).
These findings shed light on a new type of modulatory activity played by NSs. Indeed, NSs not only allosterically modulate GABAA receptor function and synthesis, but can also regulate membrane trafficking of the receptor protein, which is particularly important in determining synapse efficacy. Another NS, pregnenolone sulphate, uses the same “strategy” to modulate N-Methyl-D-aspartate (NMDA) receptor-mediated neurotransmission. Indeed, its effects are determined by direct modulation of the NMDA receptor, but also by increasing receptor expression on the cell surface (Kostakis et al., 2013).
Interestingly, in physio-pathological situations, or after pharmacological treatments, NSs (endogenous and exogenous) and PKC activities may vary a lot. The precise role of functional cross-talk between these “modulators” (Adams et al., 2015) and how these interactions can affect GABAA receptor function are still a matter of investigation.
In Figure 2 we summarize some of the interrelationships among these three players. We believe that it is important to keep in mind these pathways. Eventually, shedding light on other presently unknown cross-talks will help to better understand the mechanisms underlying some neuropathologies, as well as unraveling the mechanisms of action of novel GABAA modulating drugs. However, a complex picture emerges from the recent findings. The pharmacological response to endogenous molecules or to exogenous drugs results from the dynamic interrelationship between modulators and receptor proteins. This cross-talk can produce different responses, depending on factors such as cellular distribution or subtype and phosphorylation state of the receptor involved.
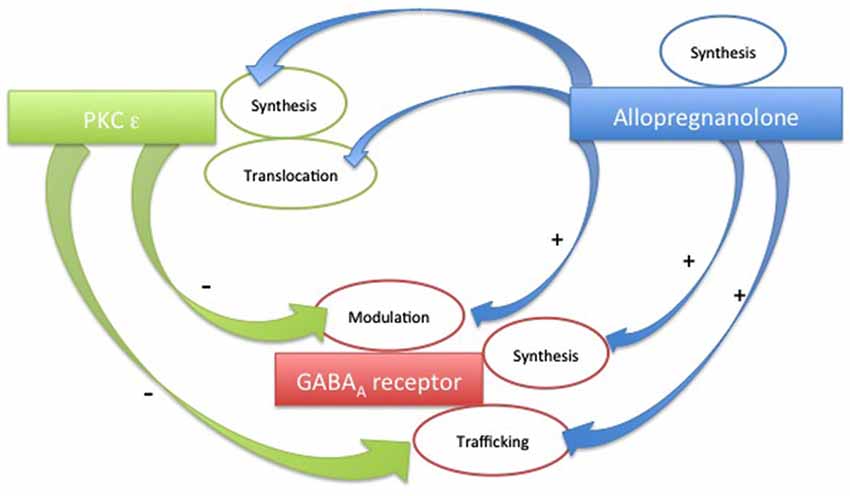
Figure 2. Schematic plot of the possible cross-talks among PKC-ε, Allopregnanolone and GABAA receptors.
As a general perspective, the importance of GABAA receptor phosphorylation is particularly relevant when a pharmacological treatment involving allosteric GABAA receptor modulators, such as NSs, is started. However, it should be emphasized that GABAA receptor rearrangement at the synapse level, and/or changes in receptor subunit composition, can lead to different pharmacological effects. Altogether, these hypotheses should be taken into account to better understand the complex behavior of NSs at the level of neuronal circuitries (Puia et al., 2012) and in in vivo studies.
Conflict of Interest Statement
The authors declare that the research was conducted in the absence of any commercial or financial relationships that could be construed as a potential conflict of interest.
Acknowledgments
This work has been supported by Association Francaise Contre les Myopathies (AFM Grant n° 16342/2012) to V.M. We thank Prof. Giulia Curia and M.Sc. Richard JP Smith for helpful revision of the manuscript.
References
Abramian, A. M., Comenencia-Ortiz, E., Modgil, A., Vien, T. N., Nakamura, Y., Moore, Y. E., et al. (2014). Neurosteroids promote phosphorylation and membrane insertion of extrasynaptic GABAA receptors. Proc. Natl. Acad. Sci. U S A 111, 7132–7137. doi: 10.1073/pnas.1403285111
PubMed Abstract | Full Text | CrossRef Full Text | Google Scholar
Adams, J. M., Thomas, P., and Smart, T. G. (2015). Modulation of neurosteroid potentiation by protein kinases at synaptic- and extrasynaptic-type GABAA receptors. Neuropharmacology 88, 63–73. doi: 10.1016/j.neuropharm.2014.09.021
PubMed Abstract | Full Text | CrossRef Full Text | Google Scholar
Alipour, M. R., Aliparasti, M. R., Keyhanmanesh, R., Almasi, S., Halimi, M., Ansarin, K., et al. (2011). Effect of ghrelin on protein kinase C-ε and protein kinase C-δ gene expression in the pulmonary arterial smooth muscles of chronic hypoxic rats. J. Endocrinol. Invest. 34, e369–e373. doi: 10.3275/8056
PubMed Abstract | Full Text | CrossRef Full Text | Google Scholar
Battaini, F. (2001). Protein kinase C isoforms as therapeutic targets in nervous system disease states. Pharmacol. Res. 44, 353–361. doi: 10.1006/phrs.2001.0893
PubMed Abstract | Full Text | CrossRef Full Text | Google Scholar
Baulieu, E. E., and Robel, P. (1990). Neurosteroids: a new brain function? J. Steroid Biochem. Mol. Biol. 37, 395–403. doi: 10.1016/0960-0760(90)90490-C
PubMed Abstract | Full Text | CrossRef Full Text | Google Scholar
Belelli, D., Casula, A., Ling, A., and Lambert, J. J. (2002). The influence of subunit composition on the interaction of neurosteroids and GABAA receptors. Neuropharmacology 43, 651–661. doi: 10.1016/s0028-3908(02)00172-7
PubMed Abstract | Full Text | CrossRef Full Text | Google Scholar
Borghini, I., Ania-Lahuerta, A., Regazzi, R., Ferrari, G., Gjinovci, A., Wollheim, C. B., et al. (1994). α, βI, βII, δ and ε protein kinase C isoforms and compound activity in the sciatic nerve of normal and diabetic rats. J. Neurochem. 62, 686–696. doi: 10.1046/j.1471-4159.1994.62020686.x
PubMed Abstract | Full Text | CrossRef Full Text | Google Scholar
Brandon, N. J., Uren, J. M., Kittler, J. T., Wang, H., Olsen, R., Parker, P. J., et al. (1999). Subunit-specific association of protein kinase C and the receptor for activated C kinase with GABAA receptors. J. Neurosci. 19, 9228–9234.
Brussaard, A. B., and Koksma, J. J. (2003). Conditional regulation of neurosteroid sensitivity of GABAA receptors. Ann. N Y Acad. Sci. 1007, 29–36. doi: 10.1196/annals.1286.003
PubMed Abstract | Full Text | CrossRef Full Text | Google Scholar
Brussaard, A. B., Wossink, J., Lodder, J. C., and Kits, K. S. (2000). Progesterone-metabolite prevents protein kinase C-dependent modulation of gamma-aminobutyric acid type A receptors in oxytocin neurons. Proc. Natl. Acad. Sci. U S A 97, 3625–3630. doi: 10.1073/pnas.050424697
PubMed Abstract | Full Text | CrossRef Full Text | Google Scholar
Carver, C. M., Wu, X., Gangisetty, O., and Reddy, D. S. (2014). Perimenstrual-like hormonal regulation of extrasynaptic δ-containing GABAA receptors mediating tonic inhibition and neurosteroid sensitivity. J. Neurosci. 34, 14181–14197. doi: 10.1523/JNEUROSCI.0596-14.2014
PubMed Abstract | Full Text | CrossRef Full Text | Google Scholar
Chen, G., Masana, M. I., and Manji, H. K. (2000). Lithium regulates PKC-mediated intracellular cross-talk and gene expression in the CNS in vivo. Bipolar. Disord. 2, 217–236. doi: 10.1034/j.1399-5618.2000.20303.x
PubMed Abstract | Full Text | CrossRef Full Text | Google Scholar
Chou, W. H., Wang, D., McMahon, T., Qi, Z. H., Song, M., Zhang, C., et al. (2010). GABAA receptor trafficking is regulated by protein kinase C(epsilon) and the N- ethylmaleimide-sensitive factor. J. Neurosci. 30, 13955–13965. doi: 10.1523/JNEUROSCI.0270-10.2010
PubMed Abstract | Full Text | CrossRef Full Text | Google Scholar
Fáncsik, A., Linn, D. M., and Tasker, J. G. (2000). Neurosteroid modulation of GABA IPSCs is phosphorylation dependent. J. Neurosci. 20, 3067–3075.
Ferando, I., and Mody, I. (2012). GABAA receptor modulation by neurosteroids in models of temporal lobe epilepsies. Epilepsia 53(Suppl. 9), 89–101. doi: 10.1111/epi.12038
PubMed Abstract | Full Text | CrossRef Full Text | Google Scholar
Fritschy, J. M., Kiener, T., Bouilleret, V., and Loup, F. (1999). GABAergic neurons and GABAA receptors in temporal lobe epilepsy. Neurochem. Int. 34, 435–445. doi: 10.1016/s0197-0186(99)00040-6
PubMed Abstract | Full Text | CrossRef Full Text | Google Scholar
Harrison, N. L., Vicini, S., and Barker, J. L. (1987). A steroid anesthetic prolongs inhibitory postsynaptic currents in cultured rat hippocampal neurons. J. Neurosci. 7, 604–609.
Hodge, C. W., Mehmert, K. K., Kelley, S. P., McMahon, T., Haywood, A., Olive, M. F., et al. (1999). Supersensitivity to allosteric GABA(A) receptor modulators and alcohol in mice lacking PKC epsilon. Nat. Neurosci. 2, 997–1002. doi: 10.1038/14795
PubMed Abstract | Full Text | CrossRef Full Text | Google Scholar
Hodge, C. W., Raber, J., McMahon, T., Walter, H., Sanchez-Perez, A. M., Olive, M. F., et al. (2002). Decreased anxiety-like behavior, reduced stress hormones and neurosteroid supersensitivity in mice lacking PKC epsilon. J. Clin. Invest. 110, 1003–1010. doi: 10.1172/jci15903
PubMed Abstract | Full Text | CrossRef Full Text | Google Scholar
Khasar, S. G., Lin, Y. H., Martin, A., Dadgar, J., McMahon, T., Wang, D., et al. (1999). A novel nociceptor signaling pathway revealed in protein kinase C epsilon mutant mice. Neuron 24, 253–260. doi: 10.1016/s0896-6273(00)80837-5
PubMed Abstract | Full Text | CrossRef Full Text | Google Scholar
Kia, A., Ribeiro, F., Nelson, R., Gavrilovici, C., Ferguson, S. S., and Poulter, M. O. (2011). Kindling alters neurosteroid-induced modulation of phasic and tonic GABAA receptor-mediated currents: role of phosphorylation. J. Neurochem. 116, 1043–1056. doi: 10.1111/j.1471-4159.2010.07156.x
PubMed Abstract | Full Text | CrossRef Full Text | Google Scholar
Kostakis, E., Smith, C., Jang, M. K., Martin, S. C., Richards, K. G., Russek, S. J., et al. (2013). The neuroactive steroid pregnenolone sulfate stimulates trafficking of functional N-methyl D-aspartate receptors to the cell surface via a noncanonical, G protein and Ca2+-dependent mechanism. Mol. Pharmacol. 84, 261–274. doi: 10.1124/mol.113.085696
PubMed Abstract | Full Text | CrossRef Full Text | Google Scholar
Lévi, S., Le Roux, N., Eugène, E., and Poncer, J. C. (2015). Benzodiazepine ligands rapidly influence GABAA receptor diffusion and clustering at hippocampal inhibitory synapses. Neuropharmacology 88, 199–208. doi: 10.1016/j.neuropharm.2014.06.002
PubMed Abstract | Full Text | CrossRef Full Text | Google Scholar
Loup, F., Wieser, H. G., Yonekawa, Y., Aguzzi, A., and Fritschy, J. M. (2000). Selective alterations in GABAA receptor subtypes in human temporal lobe epilepsy. J. Neurosci. 20, 5401–5419.
Luchetti, S., Huitinga, I., and Swaab, D. F. (2011). Neurosteroid and GABA-A receptor alterations in Alzheimer’s disease, Parkinson’s disease and multiple sclerosis. Neuroscience 191, 6–21. doi: 10.1016/j.neuroscience.2011.04.010
PubMed Abstract | Full Text | CrossRef Full Text | Google Scholar
Maguire, J., and Mody, I. (2007). Neurosteroid synthesis-mediated regulation of GABAA receptors: relevance to the ovarian cycle and stress. J. Neurosci. 27, 2155–2162. doi: 10.1523/jneurosci.4945-06.2007
PubMed Abstract | Full Text | CrossRef Full Text | Google Scholar
Maguire, J. L., Stell, B. M., Rafizadeh, M., and Mody, I. (2005). Ovarian cycle-linked changes in GABA(A) receptors mediating tonic inhibition alter seizure susceptibility and anxiety. Nat. Neurosci. 8, 797–804. doi: 10.1038/nn1469
PubMed Abstract | Full Text | CrossRef Full Text | Google Scholar
Moss, S. J., and Smart, T. J. (1996). Modulation of amino acid-gated ion channels by protein phosphorylation. Int. Rev. Neurobiol. 39, 1–52. doi: 10.1016/S0074-7742(08)60662-5
PubMed Abstract | Full Text | CrossRef Full Text | Google Scholar
Mtchedlishvili, Z., Bertram, E. H., and Kapur, J. (2001). Diminished allopregnanolone enhancement of GABA(A) receptor currents in a rat model of chronic temporal lobe epilepsy. J. Physiol. 537, 453–465. doi: 10.1111/j.1469-7793.2001.00453.x
PubMed Abstract | Full Text | CrossRef Full Text | Google Scholar
Nishizuka, Y. (1995). Protein kinase C and lipid signaling for sustained cellular responses. FASEB J. 9, 484–496.
Oberlander, J. G., Porter, D. M., Onakomaiya, M. M., Penatti, C. A., Vithlani, M., Moss, S. J., et al. (2012). Estrous cycle variations in GABA(A) receptor phosphorylation enable rapid modulation by anabolic androgenic steroids in the medial preoptic area. Neuroscience 226, 397–410. doi: 10.1016/j.neuroscience.2012.09.014
PubMed Abstract | Full Text | CrossRef Full Text | Google Scholar
Parada, C. A., Yeh, J. J., Reichling, D. B., and Levine, J. D. (2003). Transient attenuation of protein kinase C epsilon can terminate a chronic hyperalgesic state in the rat. Neuroscience 120, 219–226. doi: 10.1016/s0306-4522(03)00267-7
PubMed Abstract | Full Text | CrossRef Full Text | Google Scholar
Peng, Z., Huang, C. S., Stell, B. M., Mody, I., and Houser, C. R. (2004). The altered expression of the delta subunit of the GABAA receptorin a mouse model of temporal lobe epilepsy. J. Neurosci. 24, 8629–8639. doi: 10.1523/jneurosci.2877-04.2004
PubMed Abstract | Full Text | CrossRef Full Text | Google Scholar
Poisbeau, P., Patte-Mensah, C., Keller, A. F., Barrot, M., Breton, J. D., Luis-Delgado, O. E., et al. (2005). Inflammatory pain upregulates spinal inhibition via endogenous neurosteroid production. J. Neurosci. 25, 11768–11776. doi: 10.1523/jneurosci.3841-05.2005
PubMed Abstract | Full Text | CrossRef Full Text | Google Scholar
Puia, G., Gullo, F., Dossi, E., Lecchi, M., and Wanke, E. (2012). Novel modulatory effects of neurosteroids and benzodiazepines on excitatory and inhibitory neurons excitability: a multi-electrode array recording study. Front. Neural Circuits 6:94. doi: 10.3389/fncir.2012.00094
PubMed Abstract | Full Text | CrossRef Full Text | Google Scholar
Puia, G., Santi, M. R., Vicini, S., Pritchet, D. B., Purdy, R. H., Paul, S. M., et al. (1990). Neurosteroids act on recombinant human GABAA receptors. Neuron 4, 759–765. doi: 10.1016/0896-6273(90)90202-q
PubMed Abstract | Full Text | CrossRef Full Text | Google Scholar
Qi, Z. H., Song, M., Wallace, M. J., Wang, D., Newton, P. M., McMahon, T., et al. (2007). Protein kinase C epsilon regulates gamma-aminobutyrate type A receptor sensitivity to ethanol and benzodiazepines through phosphorylation of gamma2 subunits. J. Biol. Chem. 282, 33052–33063. doi: 10.1074/jbc.m707233200
PubMed Abstract | Full Text | CrossRef Full Text | Google Scholar
Reddy, D. S. (2009). The role of neurosteroids in the pathophysiology and treatment of catamenial epilepsy. Epilepsy Res. 85, 1–30. doi: 10.1016/j.eplepsyres.2009.02.017
PubMed Abstract | Full Text | CrossRef Full Text | Google Scholar
Rybin, V., and Steinberg, S. F. (1996). Thyroid hormone represses protein kinase C isoform expression and activity in rat cardiac myocytes. Circ. Res. 79, 388–398. doi: 10.1161/01.res.79.3.388
PubMed Abstract | Full Text | CrossRef Full Text | Google Scholar
Saito, N., Itouji, A., Totani, Y., Osawa, I., Koide, H., Fujisawa, N., et al. (1993). Cellular and intracellular localization of epsilon-subspecies of protein kinase C in the rat brain; presynaptic localization of the ε-subspecies. Brain Res. 607, 241–248. doi: 10.1016/0006-8993(93)91512-q
PubMed Abstract | Full Text | CrossRef Full Text | Google Scholar
Saliba, R. S., Kretschmannova, K., and Moss, S. J. (2012). Activity-dependent phosphorylation of GABAA receptors regulates receptor insertion and tonic current. EMBO J. 31, 2937–2951. doi: 10.1038/emboj.2012.109
PubMed Abstract | Full Text | CrossRef Full Text | Google Scholar
Schwarzer, C., Tsunashima, K., Wanzenböck, C., Fuchs, K., Sieghart, W., and Sperk, G. (1997). GABAA receptor subunits in the rat hippocampus. II. Altered distribution in kainic acid-induced temporal lobe epilepsy. Neuroscience 80, 1001–1017. doi: 10.1016/s0306-4522(97)00145-0
PubMed Abstract | Full Text | CrossRef Full Text | Google Scholar
Sen, A., Alkon, D. L., and Nelson, T. J. (2012). Apolipoprotein E3 (ApoE3) but not ApoE4 protects against synaptic loss through increased expression of protein kinase C epsilon. J. Biol. Chem. 287, 15947–15958. doi: 10.1074/jbc.M111.312710
PubMed Abstract | Full Text | CrossRef Full Text | Google Scholar
Stell, B. M., Brickley, S. G., Tang, C. Y., Farrant, M., and Mody, I. (2003). Neuroactive steroids reduce neuronal excitability by selectively enhancing tonic inhibition mediated by delta subunit-containing GABAA receptors. Proc. Natl. Acad. Sci. U S A 100, 14439–14444. doi: 10.1073/pnas.2435457100
PubMed Abstract | Full Text | CrossRef Full Text | Google Scholar
Twyman, R. E., and Macdonald, R. L. (1992). Neurosteroid regulation of GABAA receptor single-channel kinetic properties of mouse spinal cord neurons in culture. J. Physiol. 456, 215–245. doi: 10.1113/jphysiol.1992.sp019334
PubMed Abstract | Full Text | CrossRef Full Text | Google Scholar
Van Kolen, K., Pullan, S., Neefs, J. M., and Dautzenberg, F. M. (2008). Nociceptive and behavioural sensitisation by protein kinase C epsilon signalling in the CNS. J. Neurochem. 104, 1–13.
Vergnano, A. M., Schlichter, R., and Poisbeau, P. (2007). PKC activation sets an upper limit to the functional plasticity of GABAergic transmission induced by endogenous neurosteroids. Eur. J. Neurosci. 26, 1173–1182. doi: 10.1111/j.1460-9568.2007.05746.x
PubMed Abstract | Full Text | CrossRef Full Text | Google Scholar
Keywords: GABAA receptor, phosphorylation site, neurosteroids, receptor traffiking, PKCepsilon
Citation: Puia G, Ravazzini F, Castelnovo LF and Magnaghi V (2015) PKCε and allopregnanolone: functional cross-talk at the GABAA receptor level. Front. Cell. Neurosci. 9:83. doi: 10.3389/fncel.2015.00083
Received: 22 December 2014; Accepted: 23 February 2015;
Published online: 19 March 2015.
Edited by:
Jonathan Mapelli, University of Modena and Reggio Emilia, ItalyReviewed by:
Stefano Vicini, Georgetown University School of Medicine, USAPaul Andrew Davies, Tufts University, USA
Copyright © 2015 Puia, Ravazzini, Castelnovo and Magnaghi. This is an open-access article distributed under the terms of the Creative Commons Attribution License (CC BY). The use, distribution and reproduction in other forums is permitted, provided the original author(s) or licensor are credited and that the original publication in this journal is cited, in accordance with accepted academic practice. No use, distribution or reproduction is permitted which does not comply with these terms.
*Correspondence: Giulia Puia, Department of Life Science, University of Modena and Reggio Emilia, Via Campi 287, Modena, 41100, Italy Tel: +39-0592055135Z2l1bGlhLnB1amFAdW5pbW9yZS5pdA==