- 1Department of Cell Biology and Neuroscience, Istituto Superiore di Sanità, Rome, Italy
- 2Department of Life Sciences, University of Modena and Reggio Emilia, Modena, Italy
- 3Department of Physiology and Pharmacology, Sapienza University, Rome, Italy
A commentary on
Dynamic microglial alterations underlie stress-induced depressive-like behavior and suppressed neurogenesis
by Kreisel, T., Frank, M. G., Licht, T., Reshef, R., Ben-Menachem-Zidon, O., Baratta, M. V., et al. (2014). Mol. Psychiatry 19, 699–709.
Major Depression (MD) affects 10–15% of the population worldwide and constitutes an enormous medical, societal, and economic burden. One of the prominent causes of such burden is the very limited understanding of the mechanisms underlying the psychopathology (Belmaker and Agam, 2008). Among the most relevant factors determining the onset of MD is the quality of the living environment, such as exposure to stressful life events (Cohen et al., 2007; Davidson and McEwen, 2012). In addition, increased inflammation, associated to conditions such as infection and immunotherapy, is reported to increase the likelihood to show depressive symptomatology (Dantzer et al., 2008; Han and Yu, 2014).
The nervous and the immune systems are engaged in an intense bidirectional interplay, along the ongoing changes in the living environment (Yirmiya and Goshen, 2011; McCusker and Kelley, 2013). In this perspective, microglia, the resident immune cells of the brain, have recently attracted a lot of interest in the biological psychiatry field (Muller, 2014). These cells can modify their features and function according to the inputs from the environment. Indeed, equipped with receptors for a plethora of molecules microglia cells can sense environmental changes over a time scale of minutes and respond performing diverse functions which, accordingly to the context, might result as either beneficial or harmful (Kierdorf and Prinz, 2013; Siskova and Tremblay, 2013), though factors regulating microglial transition across different functional states are not well-defined. For instance, these cells respond to sensory and behavioral experience (e.g., deprivation of visual stimuli, environmental enrichment) by modulating their interactions with neuronal circuits, notably regulating processes such as adult hippocampal neurogenesis (Ekdahl, 2012; Reshef et al., 2014) and elimination and formation of synapses (Paolicelli et al., 2011; Tremblay et al., 2011; Parkhurst et al., 2013; Sierra et al., 2014).
The role of microglia in interfacing environmental stimuli and changes in brain function has suggested that these cells may underlay the interplay between environmental stimuli and vulnerability to MD. In this context, the preclinical study performed by the group headed by Raz Yirmiya provides an interesting demonstration of the role played by the microglial cells in mediating the effects of stress on depression. Authors showed that stress exposure produced dynamic bi-directional alterations in microglia status that, in turn, are causally involved in stress-induced depressive-like behavior in rodents. They explored the effects of stress in three brain regions: the hippocampal dentate gyrus, the medial prefrontal cortex and the somatosensory cortex. They found that mice and rats exposed to unpredictable stress (US) show microglia modifications, mainly in the dentate gyrus of the hippocampal system, which are dependent on the duration of the US. Indeed, they demonstrated that a short-term exposure (1–2 days) to US results in a transient induction of microglia proliferation and activation (i.e., microglial cells assume an activated morphology and increased expression of activation markers) via IL-1\ IL-1R mediated signaling. However, following 3–4 days of US, a microglia decline was observed in stressed animals compared to controls as indicated by high levels of caspase-3 (a protease that mediates the execution-phase of apoptosis) and DNA fragmentation (a key feature of apoptosis). Moreover, they found that chronic (5 weeks) US exposure induced depressive-like behavior (namely, decreased sucrose preference and social exploration) associated with a lowered number of microglia cells, reduction in length of microglial processes and soma area, and decreased expression of microglial markers (i.e., Iba-1 and CD11b). In addition, Yirmiya and collaborators showed, through pharmacological manipulations, that counteracting the bi-directional changes in microglial activity induced by stress can prevent or reverse the depressive-like phenotype. In particular, the block of microglial activation during the early phase of stress exposure through administration of minocycline (a commonly used drug to inhibit microglial activation) or imipramine (an antidepressant with anti-inflammatory properties; Alboni et al., 2013) prevents, at long-term, specific stress-induced effects on microglia and behavior. Whereas, during the late phase of stress exposure, when microglial decline occurs, the stimulation of microglia through administration of lipopolysaccharide, macrophage colony-stimulating factor or granulocytes-macrophage colony-stimulating factor reverses the stress-induced depressive-like behaviors. These observations support a causal role for the dynamic microglial changes in triggering and maintaining depressive-like symptoms under stress conditions and suggest an inverted U-shaped relationship between time-dependent microglial status and behavioral outcome (Goshen et al., 2007; Kreisel et al., 2014).
Overall, the results of this paper open a new perspective about the role of microglia in the interplay between the quality of the environment and vulnerability to MD. We strengthen this finding, stressing the idea that microglia plays a key role in such interplay not only actively participating but being integral part of brain plasticity (Figure 1). Therefore, along with neuronal plasticity, microglial plasticity should be considered as a key component of the brain plasticity processes. In this perspective, neuronal plasticity, and brain plasticity are not synonyms, but the latter emerges from the plastic capabilities of both neurons and microglia, and glia in general, and from their interaction. Accordingly, it is warranted to explore brain plasticity in a comprehensive fashion, assessing neuronal plasticity (e.g., synaptic strength, spine, and dendritic modifications) in parallel with the dynamic changes in microglial status (e.g., morphological changes, phagocytic activity). This approach may lead to unravel novel molecular and cellular mechanisms underlying the onset and progression of psychopathologies to be exploited as targets for innovative therapeutic strategies.
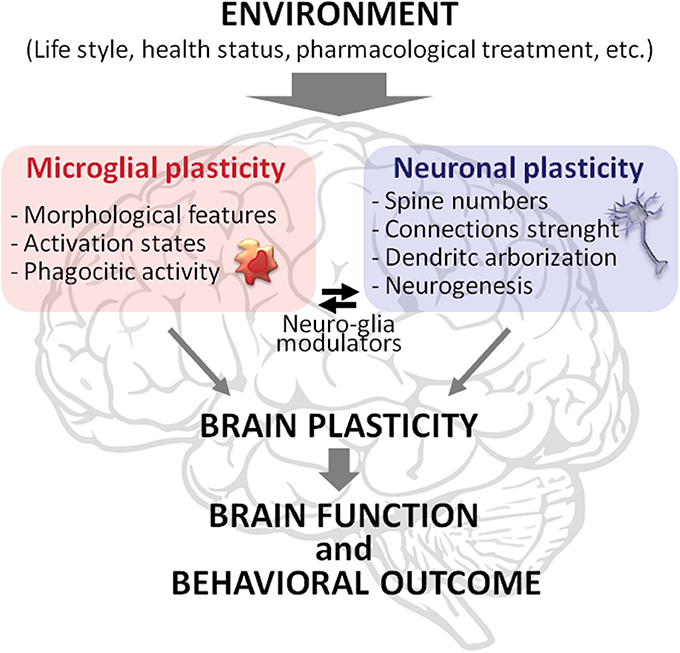
Figure 1. Schematic representation of brain plasticity as a complex phenomenon emerging from the plastic capabilities of microglia and neurons and their interaction. Microglia and neurons modify their morphology and functionality in response to environmental stimuli, such as exercise, diet, social interactions, health conditions, and pharmacological treatments. The modifications in these two cell types are intertwined. For instance, microglial phagocytic activity strongly affects neuronal spine remodeling while compounds released by neurons influence microglia status. These processes allow brain function and behavioral outcome to be tuned to the features of the environment.
Conflict of Interest Statement
The authors declare that the research was conducted in the absence of any commercial or financial relationships that could be construed as a potential conflict of interest.
References
Alboni, S., Benatti, C., Montanari, C., Tascedda, F., and Brunello, N. (2013). Chronic antidepressant treatments resulted in altered expression of genes involved in inflammation in the rat hypothalamus. Eur. J. Pharmacol. 721, 158–167. doi: 10.1016/j.ejphar.2013.08.046
Pubmed Abstract | Pubmed Full Text | CrossRef Full Text | Google Scholar
Belmaker, R. H., and Agam, G. (2008). Major depressive disorder. N. Engl. J. Med. 358, 55–68. doi: 10.1056/NEJMra073096
Pubmed Abstract | Pubmed Full Text | CrossRef Full Text | Google Scholar
Cohen, S., Janicki-Deverts, D., and Miller, G. E. (2007). Psychological stress and disease. JAMA 298, 1685–1687. doi: 10.1001/jama.298.14.1685
Pubmed Abstract | Pubmed Full Text | CrossRef Full Text | Google Scholar
Dantzer, R., O'connor, J. C., Freund, G. G., Johnson, R. W., and Kelley, K. W. (2008). From inflammation to sickness and depression: when the immune system subjugates the brain. Nat. Rev. Neurosci. 9, 46–56. doi: 10.1038/nrn2297
Pubmed Abstract | Pubmed Full Text | CrossRef Full Text | Google Scholar
Davidson, R. J., and McEwen, B. S. (2012). Social influences on neuroplasticity: stress and interventions to promote well-being. Nat. Neurosci. 15, 689–695. doi: 10.1038/nn.3093
Pubmed Abstract | Pubmed Full Text | CrossRef Full Text | Google Scholar
Ekdahl, C. T. (2012). Microglial activation - tuning and pruning adult neurogenesis. Front. Pharmacol. 3:41. doi: 10.3389/fphar.2012.00041
Pubmed Abstract | Pubmed Full Text | CrossRef Full Text | Google Scholar
Goshen, I., Kreisel, T., Ounallah-Saad, H., Renbaum, P., Zalzstein, Y., Ben-Hur, T., et al. (2007). A dual role for interleukin-1 in hippocampal-dependent memory processes. Psychoneuroendocrinology 32, 1106–1115. doi: 10.1016/j.psyneuen.2007.09.004
Pubmed Abstract | Pubmed Full Text | CrossRef Full Text | Google Scholar
Han, Q. Q., and Yu, J. (2014). Inflammation: a mechanism of depression? Neurosci. Bull. 30, 515–523. doi: 10.1007/s12264-013-1439-3
Pubmed Abstract | Pubmed Full Text | CrossRef Full Text | Google Scholar
Kierdorf, K., and Prinz, M. (2013). Factors regulating microglia activation. Front. Cell Neurosci. 7:44. doi: 10.3389/fncel.2013.00044
Pubmed Abstract | Pubmed Full Text | CrossRef Full Text | Google Scholar
Kreisel, T., Frank, M. G., Licht, T., Reshef, R., Ben-Menachem-Zidon, O., Baratta, M. V., et al. (2014). Dynamic microglial alterations underlie stress-induced depressive-like behavior and suppressed neurogenesis. Mol. Psychiatry 19, 699–709. doi: 10.1038/mp.2013.155
Pubmed Abstract | Pubmed Full Text | CrossRef Full Text | Google Scholar
McCusker, R. H., and Kelley, K. W. (2013). Immune-neural connections: how the immune system's response to infectious agents influences behavior. J. Exp. Biol. 216, 84–98. doi: 10.1242/jeb.073411
Pubmed Abstract | Pubmed Full Text | CrossRef Full Text | Google Scholar
Muller, N. (2014). Immunology of major depression. Neuroimmunomodulation 21, 123–130. doi: 10.1159/000356540
Paolicelli, R. C., Bolasco, G., Pagani, F., Maggi, L., Scianni, M., Panzanelli, P., et al. (2011). Synaptic pruning by microglia is necessary for normal brain development. Science 333, 1456–1458. doi: 10.1126/science.1202529
Pubmed Abstract | Pubmed Full Text | CrossRef Full Text | Google Scholar
Parkhurst, C. N., Yang, G., Ninan, I., Savas, J. N., Yates, J. R. 3rd. Lafaille, J. J., et al. (2013). Microglia promote learning-dependent synapse formation through brain-derived neurotrophic factor. Cell 155, 1596–1609. doi: 10.1016/j.cell.2013.11.030
Pubmed Abstract | Pubmed Full Text | CrossRef Full Text | Google Scholar
Reshef, R., Kreisel, T., Beroukhim Kay, D., and Yirmiya, R. (2014). Microglia and their CX3CR1 signaling are involved in hippocampal- but not olfactory bulb-related memory and neurogenesis. Brain Behav. Immun. 41, 239–250. doi: 10.1016/j.bbi.2014.04.009
Pubmed Abstract | Pubmed Full Text | CrossRef Full Text | Google Scholar
Sierra, A., Tremblay, M. E., and Wake, H. (2014). Never-resting microglia: physiological roles in the healthy brain and pathological implications. Front. Cell. Neurosci. 8:240. doi: 10.3389/fncel.2014.00240
Pubmed Abstract | Pubmed Full Text | CrossRef Full Text | Google Scholar
Siskova, Z., and Tremblay, M. E. (2013). Microglia and synapse: interactions in health and neurodegeneration. Neural Plast. 2013:425845. doi: 10.1155/2013/425845
Pubmed Abstract | Pubmed Full Text | CrossRef Full Text | Google Scholar
Tremblay, M. E., Stevens, B., Sierra, A., Wake, H., Bessis, A., and Nimmerjahn, A. (2011). The role of microglia in the healthy brain. J. Neurosci. 31, 16064–16069. doi: 10.1523/JNEUROSCI.4158-11.2011
Pubmed Abstract | Pubmed Full Text | CrossRef Full Text | Google Scholar
Yirmiya, R., and Goshen, I. (2011). Immune modulation of learning, memory, neural plasticity and neurogenesis. Brain Behav. Immun. 25, 181–213. doi: 10.1016/j.bbi.2010.10.015
Pubmed Abstract | Pubmed Full Text | CrossRef Full Text | Google Scholar
Keywords: microglia, plasticity, environment, depression, behavior, stress
Citation: Branchi I, Alboni S and Maggi L (2014) The role of microglia in mediating the effect of the environment in brain plasticity and behavior. Front. Cell. Neurosci. 8:390. doi: 10.3389/fncel.2014.00390
Received: 06 October 2014; Accepted: 31 October 2014;
Published online: 18 November 2014.
Edited by:
Luisa Pinto, University of Minho, PortugalReviewed by:
Hiroaki Wake, National Institute for Basic Biology, JapanCatarina A. Gomes, University of Coimbra, Portugal
Copyright © 2014 Branchi, Alboni and Maggi. This is an open-access article distributed under the terms of the Creative Commons Attribution License (CC BY). The use, distribution or reproduction in other forums is permitted, provided the original author(s) or licensor are credited and that the original publication in this journal is cited, in accordance with accepted academic practice. No use, distribution or reproduction is permitted which does not comply with these terms.
*Correspondence: laura.maggi@uniroma1.it