- 1Behavioral and Systems Neuroscience Program, Department of Psychology, Rutgers University, New Brunswick, NJ, USA
- 2Joint Graduate Program in Toxicology, Rutgers University, New Brunswick, NJ, USA
The neuropeptide, orphanin FQ/nociceptin (OFQ/N or simply, nociceptin), is expressed in both neuronal and non-neuronal tissue, including the immune system. In the brain, OFQ/N has been investigated in relation to stress, anxiety, learning and memory, and addiction. More recently, it has also been found that OFQ/N influences glial cell functions, including oligodendrocytes, astrocytes, and microglial cells. However, this latter research is relatively small, but potentially important, when observations regarding the relationship of OFQ/N to stress and emotional functions is taken into consideration and integrated with the growing evidence for its involvement in cells that mediate inflammatory events. This review will first provide an overview and understanding of how OFQ/N has been implicated in the HPA axis response to stress, followed by an understanding of its influence on natural and learned anxiety-like behavior. What emerges from an examination of the literature is a neuropeptide that appears to counteract anxiogenic influences, but paradoxically, without attenuating HPA axis responses generated in response to stress. Studies utilized both central administration of OFQ/N, which was shown to activate the HPA axis, as well as antagonism of NOP-R, the OFQ/N receptor. In contrast, antagonist or transgenic OFQ/N or NOP-R knockout studies, showed augmentation of HPA axis responses to stress, suggesting that OFQ/N may be needed to control the magnitude of the HPA axis response to stress. Investigations of behavior in standard exploratory tests of anxiogenic behavior (eg., elevated plus maze) or learned fear responses have suggested that OFQ/N is needed to attenuate fear or anxiety-like behavior. However, some discrepant observations, in particular, those that involve appetitive behaviors, suggest a failure of NOP-R deletion to increase anxiety. However, it is also suggested that OFQ/N may operate in an anxiolytic manner when initial anxiogenic triggers (eg., the neuropeptide CRH) are initiated. Finally, the regulatory functions of OFQ/N in relation to emotion-related behaviors may serve to counteract potential neuroinflammatory events in the brain. This appears to be evident within the glial cell environment of the brain, since OFQ/N has been shown to reduce the production of proinflammatory cellular and cytokine events. Given that both OFQ/N and glial cells are activated in response to stress, it is possible that there is a possible convergence of these two systems that has important repercussions for behavior and neuroplasticity.
Introduction
Orphanin FQ/nociceptin (OFQ/N) is a heptadecapeptide most closely related to the endogenous kappa opioid peptide (KOP) dynorphin A (Meunier et al., 1995; Reinscheid et al., 1995). The peptide is referred interchangeably to either OFQ/N or nociceptin, due to its known hyperalgesic effects (Reinscheid et al., 1995). Early studies surrounding the isolation of this opioid-like neuropeptide revealed that OFQ/N lacks affinity for traditional opioid receptors (Bunzow et al., 1994; Mollereau et al., 1994; Reinscheid et al., 1996). Eventually it was shown that OFQ/N binds selectively to a G protein coupled receptor, the nociceptin opioid-like peptide (NOP) receptor (also known as ORL-1 or opioid-like orphan receptor 1), which resembles the classical opioid receptors in structure but does not bind opioid ligands (Bunzow et al., 1994; Fukuda et al., 1994; Mollereau et al., 1994; Wick et al., 1994). At the cellular level, activation of the NOP receptor (NOP-R) exerts inhibitory effects as it is negatively coupled to adenylyl cyclase (Mollereau et al., 1994; Wang et al., 1994), stimulates K+ conductance (Hawes et al., 2000) and inhibits high-voltage activated (HVA) N-type Ca2+ channels (Altier and Zamponi, 2008). As a result, OFQ/N binding to NOP-R reduces neuronal excitability and inhibits neurotransmitter release (Schlicker and Morari, 2000).
The OFQ/N peptide is derived through enzymatic cleavage from a larger precursor peptide, that also liberates other biologically active peptides (see Figure 1; Meunier et al., 1995; Okuda-Ashitaka et al., 1998; Reinscheid et al., 2000; Mogil and Pasternak, 2001). In this way, the nociceptin precursor, preproOFQ/N (ppOFQ/N), is similar to proopiomelanocortin (POMC), the precursor to several unrelated bioactive peptides (Meunier et al., 1995). More specifically, ppOFQ/N codes for two additional neuropeptides, nocistatin and NocII/OFQII, that lie immediately upstream and downstream, respectively, of OFQ/N (Meunier et al., 1995; Okuda-Ashitaka et al., 1998; Reinscheid et al., 2000). Less is known regarding the functional role of these neuropeptides, although there is evidence to suggest that nocistatin exerts biological effects opposite to those of OFQ/N (Okuda-Ashitaka et al., 1998; Gavioli et al., 2002).

FIGURE 1. Amino acid sequences for mouse (187 aa) and rat (181 aa) nociceptin precursors (ppOFQ/N). The signal peptide is labeled and shown in italics. Proteolytic cleavage sites are shown in red, bold font. Enzymatic cleavage may occur at multiple sites along the ppOFQ/N peptide generating three biologically active neuropeptides; OrphaninFQ/Nociceptin (blue), Nocistatin (orange) and NocII/OFQII (red; Mollereau et al., 1996).
High sequence homology between OFQ/N, NOP-R and components of the opioid superfamily prompted initial investigations into the role of OFQ/N in nociception (Meunier et al., 1995; Reinscheid et al., 1995; Rossi et al., 1998). Early findings revealed the relationship between nociceptin signaling and pain transmission to be complex as OFQ/N produced hyperalgesia when administered supraspinally (Mollereau et al., 1994; Reinscheid et al., 1995) and analgesia when administered at spinal sites (Rossi et al., 1998). Further investigation of the OFQ/N system revealed that both OFQ/N and its receptor are prominently expressed in stress-sensitive limbic and limbic-associated brain structures involved in processing emotionally relevant stimuli (Bunzow et al., 1994; Nothacker et al., 1996; Neal et al., 1999a, b; Pampusch et al., 2000). Since this suggested a potential role for OFQ/N in behaviors other than nociception, a good deal of effort has been directed to determining the role of OFQ/N in stress-related endocrine functions and behavior. Moreover, several reviews have highlighted the importance of OFQ/N in the modulation of reward, addiction and learning (Noda et al., 2000; Koob, 2008; Kuzmin et al., 2009; Zaveri, 2011).
In the current review, focus will be placed on the ability of OFQ/N to modulate anxiety-related behaviors and stress-induced neuroendocrine responses. In addition, the emerging role of OFQ/N in immune and/or inflammatory processes will be considered. There is considerable evidence that OFQ/N and NOP-R are expressed in endocrine (i.e., adrenal gland), and lymphoid organs and cells (e.g., blood leukocytes), including those considered to constitute “immune-like” functions in the CNS (Wang et al., 1994; Halford et al., 1995; Lachowicz et al., 1995; Nothacker et al., 1996; Peluso et al., 1998; Pampusch et al., 2000; Finley et al., 2008). Importantly, broad yet restricted expression of OFQ/N and NOP-R throughout the CNS and periphery suggests a modulatory role for the nociceptin system in regulation of emotional states, cognitive processes, and neuroendocrine and immune functions (Halford et al., 1995; Mamiya et al., 1998; Nabeshima et al., 1999; Devine et al., 2001; Leggett et al., 2009a). Accordingly, this paper will address the large but controversial literature concerning the role of OFQ/N in modulating physiological and behavioral responses to stress. In addition, the putative role of the nociceptin system in regulating neuroinflammatory processes will be discussed. This research is potentially important, given the relationship of OFQ/N to stress and emotional functions, and the increasing relevance of this to inflammatory events.
Orphanin FQ/Nociceptin and Responses to Psychogenic Stress
Stress as a physiological state originates from both systemic and psychogenic origins impacting nervous, endocrine and immune system functions. Of particular interest is the hypothalamic-pituitary-adrenal (HPA) axis, a major branch of the neuroendocrine system and the principle endocrine component of the stress response. Exposure to stress, both physical and psychological, activates the HPA axis, triggering a neuroendocrine cascade that culminates in the release of glucocorticoids [cortisol in humans and corticosterone (CORT) in rodents]. Briefly, stressor exposure activates neurosecretory cells of the paraventricular nucleus (PVN) of the hypothalamus, which secrete corticotropin-releasing hormone (CRH; also known as CRF) into the hypophyseal portal circulation. Once in the anterior pituitary, CRH stimulates CRH receptor I (CRH-RI) and induces the release of adrenocorticotropic hormone (ACTH), which is released into the systemic circulation, and subsequently stimulates the secretion of glucocorticoids from the adrenal cortex. A variety of stimuli can serve as stressors to the activation of the HPA axis, including psychogenic, physical and immunologic stressors. In most cases, such stressors engage higher level neural processes mediated by limbic forebrain structures, such as the amygdala, bed nucleus of the stria terminalis (BNST) and medial prefrontal cortex (mPFC), all of which either directly or indirectly form connections that ultimately impact the PVN (Ulrich-Lai and Herman, 2009).
The stress-induced elevation of CORT serves to mobilize energy stores and increase arousal. In this way, glucocorticoids are beneficial for short-term survival as they prepare animals to cope with real or perceived threats to homeostasis. However, prolonged exposure to glucocorticoids can, among other things, suppress normal immune function and impair cognition. For this reason, glucocorticoid release following stressor exposure is tightly regulated and involves integration of complex feed-forward and feedback signals at the level of the brain. Notably, circulating glucocorticoids provide feedback inhibition that is mediated, in part, by limbic-associated structures (Jankord and Herman, 2008; Ulrich-Lai and Herman, 2009). Specifically, while there are multiple levels of glucocorticoid feedback, binding of CORT to glucocorticoid receptors (GR) in the hippocampus and mPFC contributes to the inhibitory regulation of HPA axis activation (Herman et al., 2012).
Accumulating evidence strongly implicates the nociceptin system in regulation of the stress response in general, and emerging data suggest that OFQ/N may contribute to feed-forward regulation of the HPA axis specifically. Therefore, evidence is reviewed regarding the involvement of endogenous OFQ/N in the regulation of the HPA axis response to acute psychogenic stress. A summary of these findings is provided in Tables 1 and 2, as well as Figure 2.
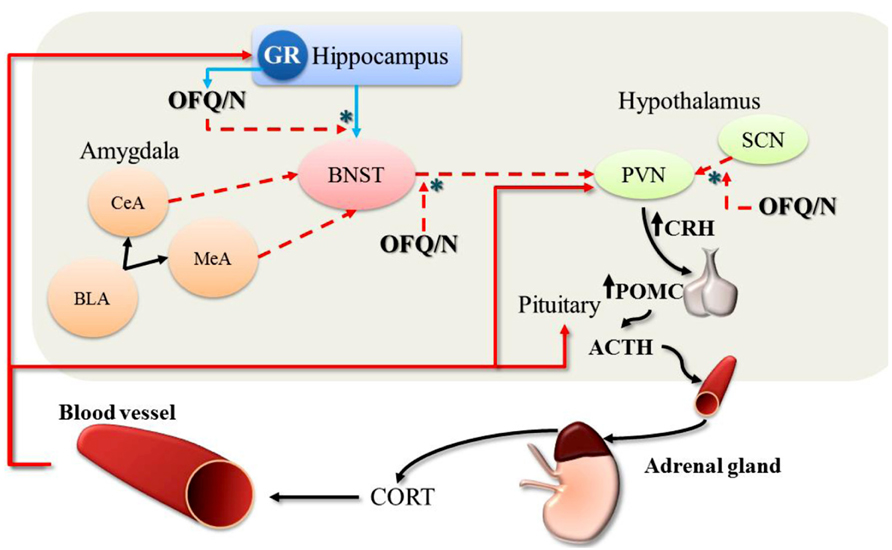
FIGURE 2. Feed-forward effects of OFQ/N on the hypothalamic-pituitary-adrenal (HPA) axis response to stress. The stimulatory effects of OFQ/N on the HPA axis are mediated through disinhibitory signaling (*) in multiple brain regions. Following activation of the HPA axis, CORT is released into circulation. At the level of the hippocampus, CORT binds to and stimulates GR which provides negative feedback control over HPA axis activity (red solid arrows). Binding of CORT to GR also induces the expression of OFQ/N, which binds to NOPr in the hippocampus and inhibits glutamatergic transmission (blue solid arrows) thereby removing trans-synaptic inhibition of the PVN. Nociceptin signaling also inhibits GABAergic outputs (red dashed arrows) from the BNST to the PVN, which attenuates the restraining influence of the BNST on PVN activity. Finally, nociceptin may also exert feed-forward effects on the HPA axis by suppressing inhibitory output from the SCN to the PVN. In this way, OFQ/N stimulates PVN neurons indirectly to produce CRH, which induces the expression of POMC and stimulates the release of ACTH. Collectively, these changes prolong or enhance the HPA axis response to stress. [Note: although depicted in this schematic for completeness, OFQ/N does not modulate amygdalar-outputs (CeA, central amygdala; MeA, medial amygdala; BLA, basolateral amygdala) to the PVN].
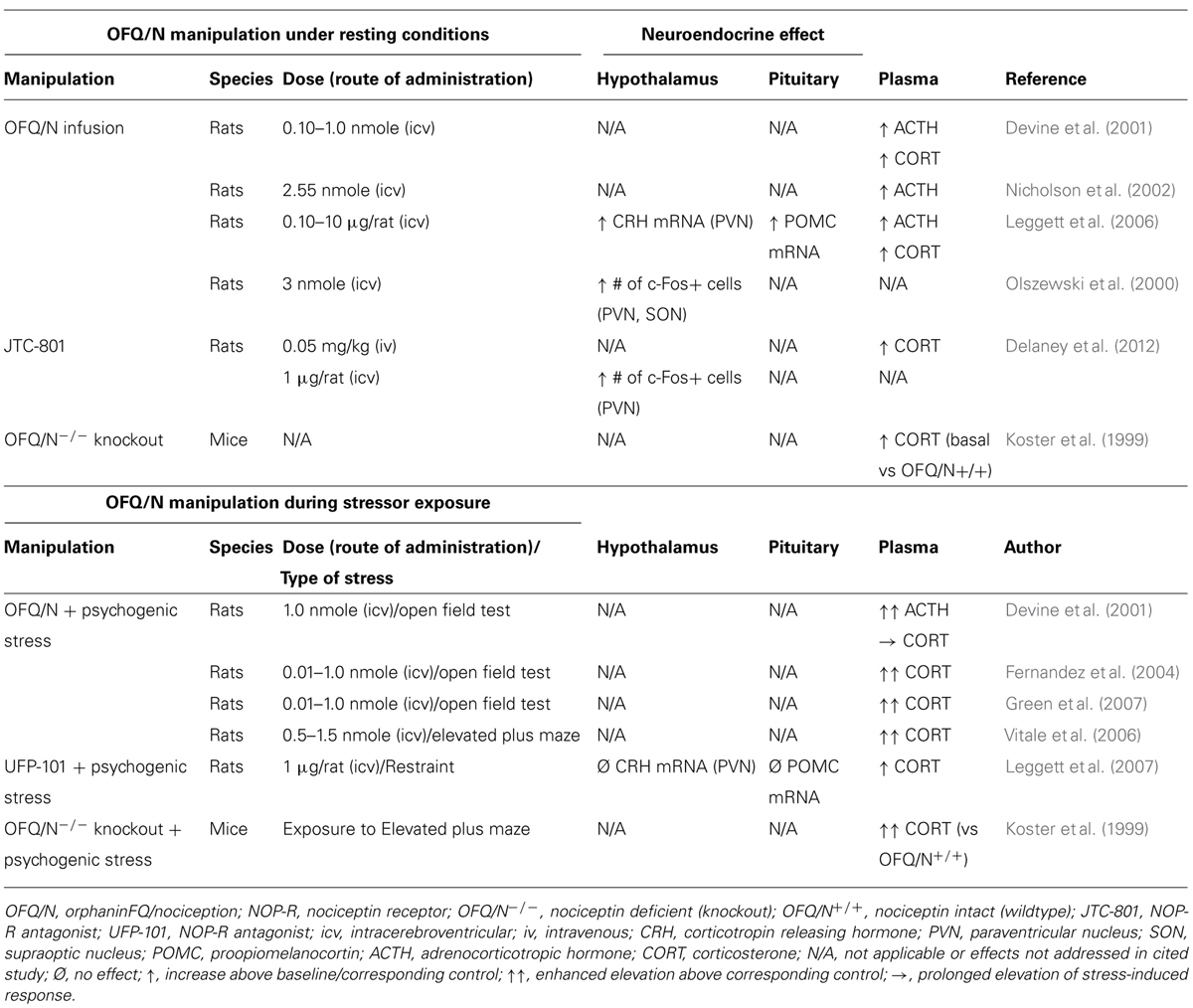
TABLE 1. Effects of manipulating the nociceptin system on the hypothalamic-pituitary-adrenal (HPA) axis response in stressed and non-stressed animals.
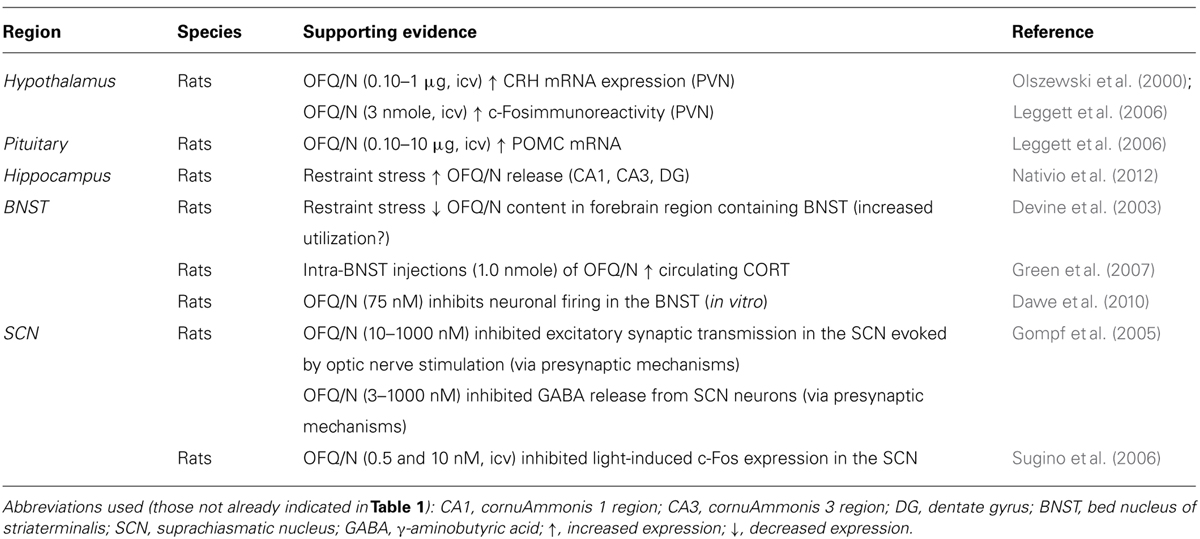
TABLE 2. Putative neural substrates mediating the stimulatory effects of OFQ/N on the hypothalamic-pituitary-adrenal (HPA) axis.
OFQ/N and the Hypothalamic-Pituitary-Adrenal Axis
Studies concerning the role of the nociceptin system in regulation of the HPA axis consistently demonstrate that central administration of OFQ/N elevates circulating ACTH and plasma CORT in unstressed rats (Devine et al., 2001; Nicholson et al., 2002; Leggett et al., 2006). Moreover, OFQ/N enhances endocrine responses during neophobic tests of anxiety such as exposure to the open field (OF; Devine et al., 2001; Fernandez et al., 2004; Green et al., 2007) and elevated plus maze (EPM; Vitale et al., 2006). Such findings implicate OFQ/N in feed-forward regulation of the HPA axis, and further suggest that nociceptin signaling may mediate or contribute to the HPA axis response to psychogenic stress.
In apparent contrast to these findings, mice lacking the nociceptin gene (OFQ/N-/-) exhibit elevated basal and post-stress levels of plasma CORT relative to wildtype mice, suggesting that OFQ/N serves to dampen HPA axis output (Koster et al., 1999). While seemingly at odds with one another, the discrepant findings of Koster et al. (1999) and others (Devine et al., 2001; Nicholson et al., 2002; Fernandez et al., 2004; Leggett et al., 2006; Vitale et al., 2006; Green et al., 2007) could be ascribed to several factors. First, enhanced HPA axis activity in OFQ/N-/- mice may be due to deficient OFQ/N signaling during key developmental processes rather than due to a lack of OFQ/N per se. In fact, ppOFQ/N and NOP-R mRNA transcripts are highly expressed during early, embryonic development, raising the possibility that OFQ/N signaling contributes to normal neuronal development (Ikeda et al., 1998). Of additional concern is that the ppOFQ/N gene, that was disrupted by Koster et al. (1999), encodes additional peptides that exert biological effects opposite to those of OFQ/N (Okuda-Ashitaka et al., 1998). Accordingly, the findings of Koster et al. (1999) might not be directly attributable to a loss of OFQ/N-NOP-R signaling.
These concerns notwithstanding, pharmacological blockade of NOP-R was shown to enhance stress-induced activation of the HPA axis (Leggett et al., 2007). Specifically, intracerebroventricular (icv) administration of the peptide NOP-R antagonist, UFP-101, enhanced and prolonged, respectively, ACTH and CORT responses to acute restraint (Leggett et al., 2007). This supports the notion that nociceptin signaling exerts a restraining effect on the HPA response to stressors (Koster et al., 1999), although under certain conditions UFP-101 acts as a partial agonist at the NOP-R receptor, potentially mimicking the effects of OFQ/N (Mahmoud et al., 2010). For example, under conditions in which NOP-R was highly expressed and constitutively active, UFP-101 inhibited Ca2+ currents in cultured, stellate ganglion neurons in a manner similar to that of OFQ/N (Mahmoud et al., 2010). On the other hand, UFP-101 blocked OFQ/N-mediated Ca2+ current inhibition in control cells expressing NOP-R at physiological levels (Mahmoud et al., 2010). Therefore, given its mixed pharmacological profile, it is uncertain whether in Leggett et al.’s (2007) study, UFP-101 enhanced restraint-stress induced activation of the HPA axis via partial agonist effects at NOP-R.
In a more recent study, the HPA modulatory effects of a different non-peptide NOP-R antagonist, JTC-801, were examined during exposure to an acute restraint stressor (Delaney et al., 2012). Although it did not further increase stress-induced levels of CORT, intravenous (iv) JTC-801 elevated plasma CORT in unstressed rats. This suggested that nociceptin tonically regulates, in an inhibitory fashion, basal activity of the HPA axis (Delaney et al., 2012), which contrasted with other evidence that UFP-101 did not alter basal HPA axis activity (Leggett et al., 2007). Once again, however, and like other NOP-R antagonists, JTC-801 exhibits a complex pharmacological profile including antagonist and inverse agonist actions (Mahmoud et al., 2010), and allosteric regulation (Sestili et al., 2004; Del Giudice et al., 2011). Therefore, other experimental factors could have influenced the effect of JTC-801 on the HPA axis including route of drug administration, dose-response characteristics, and the selectivity profile of JTC-801 at NOP-R (Delaney et al., 2012).
It seems, therefore, that pharmacological attempts to determine the role of OFQ/N in the HPA axis response to stress have been hampered by poorly selective NOP-R antagonists. Moreover, the lack of correspondence in methodology renders the results of these studies difficult to reconcile. Although this has created some uncertainty regarding whether OFQ/N is necessary for activation or inhibition of the HPA axis, it has not deterred efforts to identify the neural substrate(s) and signaling mechanism(s) that might underlie OFQ/N involvement in HPA axis regulation. One possibility is that nociceptin contributes to the synthesis and release of other major hormones or peptides that exert excitatory effects on the HPA axis. In fact, OFQ/N-induced activation of the HPA axis is paralleled by up-regulation of CRH mRNA in the PVN of the hypothalamus and increased expression of POMC mRNA in the pituitary (Leggett et al., 2006). Moreover, icv administration of OFQ/N reportedly increased Fos protein expression in the PVN (Olszewski et al., 2000). However, given that OFQ/N binding to NOP-R reduces neuronal excitability, inhibiting both glutamate and GABA release (Meis and Pape, 2001; Bianchi et al., 2004; Roberto and Siggins, 2006; Cruz et al., 2012), it is likely that activation of PVN neurons in response to OFQ/N occurs through indirect mechanisms.
It has been suggested that OFQ/N may drive HPA axis output by disrupting limbic feedback to the hypothalamus (Devine et al., 2001; Leggett et al., 2006; see Figure 2). It is recognized that the hippocampus expresses high levels of GR, and this serves as a major source of glucocorticoid-mediated feedback control over HPA axis output (Sapolsky et al., 1983). The hippocampal GR are bound by circulating CORT, one effect of which is enhancement of glutamatergic transmission (Jankord and Herman, 2008; Ulrich-Lai and Herman, 2009). This can result in trans-synaptic excitation of an inhibitory relay in the BNST that maintains and/or augments inhibition of the PVN (Jankord and Herman, 2008). This normal state of affairs, however, might be disrupted by OFQ/N (see Figure 2). Exposure of rats to acute restraint increases OFQ/N release in the hippocampus in an adrenal-dependent manner, and mimicked by CORT injection, suggesting that glucocorticoids are necessary for stress-induced hippocampal OFQ/N release (Nativio et al., 2012). However, it is not known whether this glucocorticoid-dependent hippocampal OFQ/N release subsequent to stressor exposure prolongs or enhances HPA axis activity.
Alternatively, there is evidence that OFQ/N release in the BNST may play a role in modulating the HPA axis. As mentioned, the neural circuitry for regulation of the HPA axis includes the BNST, which contains GABAergic cells that send afferents to the PVN and inhibit HPA responses to stress (Ulrich-Lai and Herman, 2009). Acute restraint stress was reported to reduce OFQ/N content in gross dissections of the basal forebrain region containing the BNST, and this was argued to reflect increased release and utilization of OFQ/N (Devine et al., 2003). Importantly, OFQ/N neurotransmission and stimulation of NOP-R in the BNST is thought to reduce GABAergic input to the PVN, which ultimately would prolong activation of the HPA axis and stress-induced elevations of CORT. Consistent with this hypothesis, OFQ/N elicits strong inhibition of BNST neuronal firing in vitro (Dawe et al., 2010). Moreover, unilateral, intra-BNST infusion of OFQ/N in rats dose-dependently enhanced the CORT response to mild psychogenic stress (exposure to an OF; Green et al., 2007). At the very least, therefore, it could be argued that OFQ/N-mediated signaling in the BNST has significant consequences on HPA axis functions precipitated by stressors; whether this also involves hippocampal recruitment as suggested above remains to be determined.
The amygdala has also received attention as a potential mediator of OFQ/N effects on the HPA axis (Green et al., 2007). A heterogeneous structure comprised of many nuclei, the amygdala is differentially recruited by systemic and psychogenic stressors, which affects the PVN indirectly through synaptic inputs in the BNST (Ulrich-Lai and Herman, 2009). The two major output nuclei of the amygdala (central and medial amygdala; CeA and MeA) contain inhibitory projection neurons that synapse onto GABAergic cell groups in the BNST (Ulrich-Lai and Herman, 2009). Therefore, by means of a disinhibitory process, activation of the CeA and/or MeA results in excitation rather than inhibition of the PVN. Interestingly, injections of OFQ/N into the amygdala did not alter the CORT response to a mild, psychogenic stressor (Green et al., 2007), in spite of other evidence that (1) social defeat stress induced NOP-R mRNA expression in the CeA and basomedial (BMA) nuclei of the amygdala (Green and Devine, 2009), (2) acute restraint stress reduced ppOFQ/N mRNA expression in the CeA (Delaney et al., 2012), and (3) icv administration of OFQ/N induced Fos protein expression in the CeA (Olszewski et al., 2000). Based on such evidence, it is not unlikely that OFQ/N signaling occurs in the amygdala, and can increase anxiety-like behavior (Green et al., 2007). However, such intra-amygdalar behavioral effects are likely to be dissociated from the neuroendocrine effects of OFQ/N, which most likely engage alternative pathways.
Finally, it has been suggested that the stimulatory effects of OFQ/N on glucocorticoid release arise from modulation of circadian inputs to the HPA axis (Devine et al., 2001; Leggett et al., 2007). In rodents, blood CORT concentrations are lowest during the light or diurnal phase of the circadian cycle, and peak in the nocturnal phase, when rodents display high levels of activity, including increased food and water intake. Similarly, CORT-mediated negative feedback regulation of ACTH varies across the circadian light/dark cycle. For example, exposure to stress during the nadir or low phase of the CORT circadian rhythm induces an ACTH response of greater magnitude than during the peak phase. A critical structure in the regulation of circadian rhythms is the suprachiasmatic nucleus (SCN) of the hypothalamus, which relays photic information from the retina to the PVN. Interestingly, NOP-R is prominently expressed in the SCN, and OFQ/N has been shown to alter the activity of SCN neurons both in vitro (Gompf et al., 2005) and in vivo (Sugino et al., 2006). For example, icv administration of OFQ/N suppressed light-induced c-Fos expression in the SCN of rats (Sugino et al., 2006), while OFQ/N inhibited both excitatory and inhibitory neurotransmission in SCN neurons (Gompf et al., 2005). In view of these findings, it has been hypothesized that OFQ/N might stimulate the HPA axis and enhance endocrine responses to acute stress by altering circadian input to the PVN across the light/dark cycle (Devine et al., 2001; Leggett et al., 2007). Although it is also likely that OFQ/N-dependent modulation of the HPA axis response to acute psychogenic stress occurs through additive actions in multiple limbic and extra-limbic sites (Devine et al., 2001; Green et al., 2007).
In summary, there is consistent evidence that OFQ/N is involved in the activation of the HPA axis. What is not clear at present is the precise mechanism by which this occurs. One factor that has not been fully investigated with regard to the HPA axis is the role of neurotransmitter systems regulated by OFQ/N. For example, there is evidence to suggest that OFQ/N exerts an inhibitory effect on the release of acetylcholine (ACh) in cortical, hippocampal and striatal brain regions (Itoh et al., 1999; Cavallini et al., 2003; Uezu et al., 2005). Cholinergic stimulatory effects on the HPA axis have long been recognized (Matta et al., 1998; Rhodes et al., 2001), and it might be expected that if OFQ/N exerts an inhibitory effect on ACh, this could be one mechanism by which an inhibition of the HPA axis is maintained. Indeed, the elevated and/or prolonged HPA axis responses observed in the absence of OFQ/N or loss of NOP-R signaling (Koster et al., 1999; Mallimo et al., 2010), fit this particular explanatory scheme. However, whether modulation of the cholinergic system is involved, remains to be determined.
OFQ/N and Regulation of Anxiety States
Anxiety as a mental state is characterized by heightened arousal, hypervigilance and the expression of defensive behaviors (e.g., escape or avoidance). Anxiety-responses are evoked in anticipation of threat and are accompanied by autonomic and endocrine activation. Collectively, these physiological and behavioral reactions are thought to be adaptive and prepare an individual to cope with unexpected or unavoidable situations. Although there is no shortage of peptides implicated in anxiety-like behavior, converging lines of evidence from rodent studies strongly implicate the nociceptin system in regulation of anxiety states. Notably, OFQ/N and NOP-R agonists have been shown to attenuate the expression of anxiety-like behaviors and in a manner comparable to that of conventional anxiolytics including diazepam (Jenck et al., 1997, 2000) and alaprozam (Jenck et al., 2000).
The attenuation of anxiety-like behaviors by OFQ/N stands in contrast to other evidence already discussed that nociceptin promotes feed-forward regulation of the HPA axis, which is often associated with anxiety states. Moreover, stimulation of the nociceptin system has also been demonstrated to enhance – rather than attenuate – the expression of anxiety-like behavior under specific conditions (Fernandez et al., 2004; Green et al., 2007). Therefore, it would appear that the relationship between the emotional and endocrine effects of nociceptin is not straightforward, nor is it clear whether the OFQ/N system is anxiolytic or anxiogenic. In the remainder of this section, these studies will be reviewed more closely, and are summarized in Tables 3 and 4.
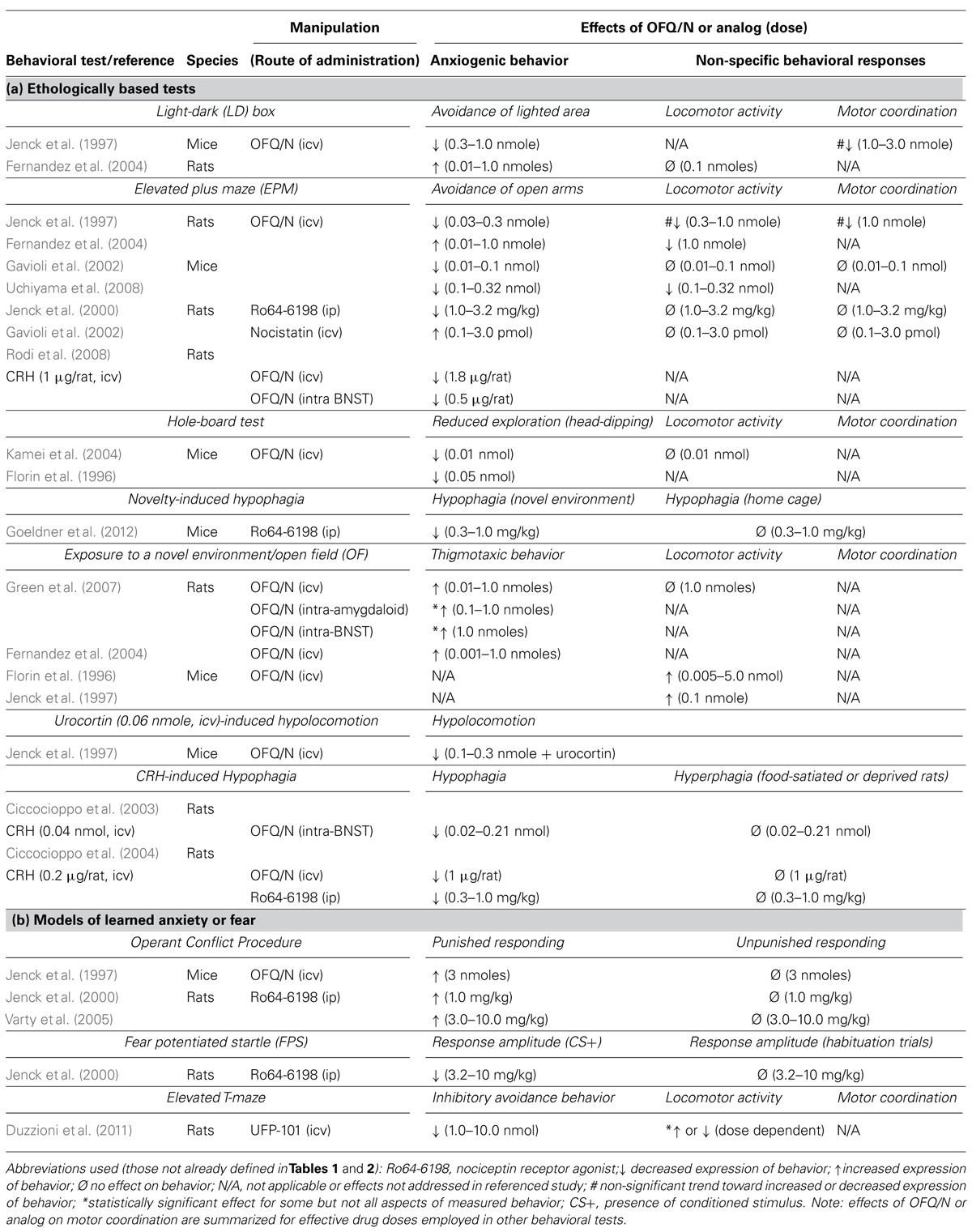
TABLE 3. Effects of manipulation of the nociceptin system on the expression of innate (a) and learned (b) anxiety-like behavior in rodents.
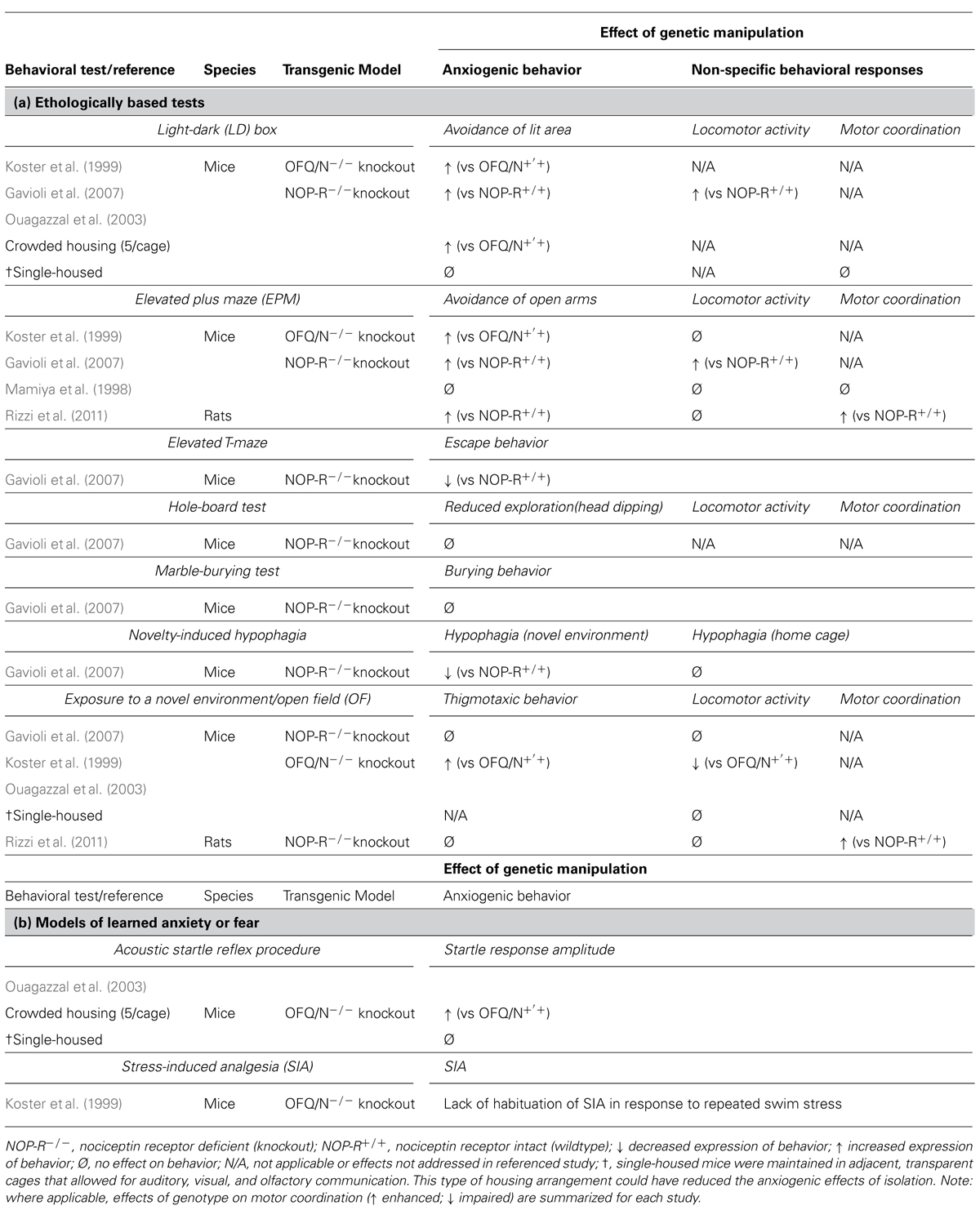
TABLE 4. Effects of targeted deletion of endogenous OFQ/N and NOP-R signaling on the expression of (a) innate and (b) learned anxiety-like or fear-like behavior in rodents.
The modulatory effects of OFQ/N have been extensively studied in a variety of tests that model different aspects of anxiety in rodents. For instance, in mice and rats central administration of OFQ/N increased the amount of time spent in the lighted compartment of a light-dark (LD) box and enhanced open arm exploration in the EPM test (Jenck et al., 1997; Gavioli et al., 2002; Uchiyama et al., 2008). Likewise, systemic administration to rats of Ro64-6198, a nonpeptide NOP-R agonist, that easily penetrates the blood-brain barrier, attenuated open-arm avoidance in the EPM (Jenck et al., 2000) and, in mice, increased the consumption of a familiar and highly palatable, liquid diet in a novel environment (Goeldner et al., 2012). These studies used ethologically based tests, which involved measures of unconditioned responses (e.g., approach-avoidance behavior) and are based on a rodent’s inherent fear of unprotected, brightly lit and unfamiliar spaces. Consequently, it is possible that OFQ/N may be effective in reducing anxiety-like behavior under conditions in which an anxiogenic response is unlearned or hard-wired.
One caveat, however, is that unconditional responding is susceptible to drug effects on general activity levels (Rodgers, 1997). Notably, the aforementioned studies used effective dose ranges at which OFQ/N has been reported to produce either hyper- or hypolocomotion in mice and rats (Reinscheid et al., 1995; Devine et al., 1996; Florin et al., 1996; Rizzi et al., 2001). Moreover, at higher doses, OFQ/N can even impair motor coordination and balance (Jenck et al., 1997, 2000). Indeed, dose-dependent effects of OFQ/N on locomotor activity are most apparent in the EPM. For instance, in mice,low doses of OFQ/N (0.01 nmol, icv) attenuated open arm avoidance without affecting other measures of locomotor activity (Gavioli et al., 2002), while higher doses of OFQ/N (0.1–0.3 nmol, icv) decreased open arm avoidance and also increased the total number of transitions between open and closed arms (Uchiyama et al., 2008). At high doses, therefore, the behavioral effects of OFQ/N may not be specific to the anxiolytic properties of the neuropeptide.
Similar concerns for the behavioral effects of OFQ/N were observed in the hole board and LD emergence tests (Florin et al., 1996; Jenck et al., 1997; Kamei et al., 2004). In the hole board test, OFQ/N was thought to attenuate anxiety by virtue of increasing head-dipping behavior (into the holes of the apparatus), and this occurred without changing general activity level (Kamei et al., 2004). Moreover, this occurred at the lowest dose tested (0.01 nmol, icv; Kamei et al., 2004). However, in other studies the increased exploration (i.e., head dipping) in the hole board test, as well as emergence into light in the LD box test, could not be differentiated from the locomotor-stimulating properties of the OFQ/N dose range tested (0.05–1.0 nmol, icv; Florin et al., 1996).
One further consideration is that the specific parameters of locomotion may inform interpretation of the locomotor effects of a given treatment. For instance, although OFQ/N (0.05–0.5 nmol, icv) produced a general hyperlocomotion in mice subjected to an unfamiliar or novel environment (Florin et al., 1996; Jenck et al., 1997), this was parsed into increased horizontal movement and increased vertical movement (e.g., rearings). Such a combination of movements might be considered an index of increased exploration and possibly reduced anxiety in animals. Indeed, when hypolocomotion was induced by the CRH receptor II agonist urocortin (a purported anxiogenic effect), OFQ/N (0.1–0.3 nmol, icv) reversed this effect, and in a manner comparable to that of diazepam (Jenck et al., 1997). This may support the concept introduced above with regard to ethological tests: that OFQ/N is anxiolytic during states of heighted anxiety, serving to interfere with other neurochemical systems that initially trigger the anxiety-like behavior.
The anxiolytic properties of OFQ/N signaling have also been demonstrated in models of learned anxiety and/or fear (Jenck et al., 1997, 2000; Varty et al., 2005). In one study, OFQ/N attenuated response inhibition in a modified Geller-Seifter conflict test (Jenck et al., 1997). In this test, food-deprived mice are first trained to lever-press for food reinforcement. After training, mice are subjected to alternating non-conflict and conflict sessions, in which bar presses are either reinforced with food (non-conflict) or result in food delivery and simultaneous foot-shock (conflict). In vehicle-treated mice, foot-shock resulted in a selective inhibition of lever-press behavior. Conversely, OFQ/N increased operant responding during conflict sessions (Jenck et al., 1997), as did Ro64-6198 in rats (Jenck et al., 2000; Varty et al., 2005). This suggests that OFQ/N may reduce the degree of task-specific anxiety associated with punished responding.
It should be noted that OFQ/N is a potent orexigenic agent (Pomonis et al., 1996; Stratford et al., 1997; Polidori et al., 2000). In fact, administration of OFQ/N into the lateral or third ventricles stimulates feeding in non-food deprived rats (Pomonis et al., 1996; Polidori et al., 2000). Moreover, site-specific microinjection of OFQ/N into brain regions implicated in the regulation of food intake (Stratford et al., 1997; Polidori et al., 2000) induces hyperphagia in free-feeding rats. Therefore, in tasks that involve appetitive behavior, it is possible that the hyperphagic effects of nociceptin contribute to changes in behavioral responses observed in food-deprived animals, such as those trained in the Geller-Seifter conflict test. However, since neither OFQ/N nor Ro64-6198 affected lever press behavior during non-conflict sessions (i.e., unpunished responding), it is likely that the OFQ/N-induced increase in punished responding is specific to the anxiolytic properties of OFQ/N (Jenck et al., 1997, 2000; Varty et al., 2005).
Finally, OFQ/N signaling attenuates anxiogenic behavior in other models of conditioned or learned emotional responses, such as the fear-potentiated startle procedure (Jenck et al., 2000). In this paradigm a previously neutral, conditional stimulus (CS; e.g., a light) is explicitly paired with the presentation of an aversive, unconditional stimulus (US; e.g., foot-shock). Following repeated CS-US presentations, an association between the two stimuli is formed such that the light comes to predict the occurrence of foot-shock. After conditioning, the startle response to an acoustic stimulus (e.g., a short burst of white noise) is measured in the absence (CS-) and presence (CS+) of the CS on alternating trials. The magnitude of startle potentiation, or increase in response amplitude on trials in which the CS is present (CS+ trials), relative to trials in which the CS is absent (CS-), is then taken as an index of learned fear. Thus, animals should exhibit a greater startle response on trials in which the CS is presented in conjunction with the acoustic stimulus. Consistent with the anxiolytic-like effects of OFQ/N and Ro64-6198 in the Geller-Seifter conflict test (Jenck et al., 1997, 2000; Varty et al., 2005), systemic administration of Ro64-6198 attenuated the magnitude of startle potentiation in rats (Jenck et al., 2000). This reinforced the notion that fear and/or anxiety can be reduced after stimulation of the OFQ/N receptor NOP.
The use of transgenic mice deficient in various components of the OFQ/N system have also suggested that OFQ/N is involved in emotion regulation. For example, mutant mice lacking the OFQ/N gene (OFQ/N-/-) exhibited increased avoidance behavior in the EPM (Koster et al., 1999). More specifically, OFQ/N-/- mice made fewer entries into and spent less time in the open arms of the EPM. Similarly, in the LD emergence test, OFQ/N-/- mice, when compared to wildtype mice, spent less time exploring, and showed a higher latency to first enter, the lighted compartment. Mutant mice also showed a reduction in free exploration in the OF test, as evidenced by increased thigmotaxic behavior and hypolocomotion (Koster et al., 1999). Collectively, these findings provided converging evidence that endogenous OFQ/N signaling mediates behavioral responding in models of innate anxiety.
Interestingly, there is evidence that the behavioral phenotype of OFQ/N-/- mice is influenced by social housing conditions (Koster et al., 1999; Ouagazzal et al., 2003). When maintained under crowded housing conditions (5 mice/cage), OFQ/N mutant mice displayed greater home-cage aggression and heightened emotional reactivity in unconditioned (LD box) and conditioned (acoustic startle) response tests. Interestingly, when mice were housed individually no behavioral differences were observed between the genotypes (Ouagazzal et al., 2003). Although isolation is also considered to be stressful the authors noted that single-housed mice were maintained in adjacent, transparent cages that allowed for auditory, visual, and olfactory communication. By providing some degree of interaction this type of housing arrangement could have reduced the anxiogenic effects of isolation. Nonetheless, these findings suggest that deficient OFQ/N signaling may confer a vulnerability to stress, and in this case, to the chronic stress produced by crowded housing conditions (Ouagazzal et al., 2003). More broadly, these data implicate endogenous OFQ/N in stressor-induced behavioral adaptation (Ouagazzal et al., 2003).
In good agreement with this idea, Koster et al. (1999) disrupted the ppOFQ/N gene, and reported that group-housed OFQ/N-/- mice failed to adapt to repeated stressor exposure. Specifically, mice were subjected to a forced swim stressor, and adaptation to repeated swim was assessed by habituation of stress-induced analgesia (SIA). In contrast to wildtype mice, OFQ/N-/- mice failed to adapt to repeated forced swim exposure and continued to exhibit SIA after each trial (Koster et al., 1999). These findings suggest that OFQ/N may be needed to promote behavioral resilience and maintenance of normal physiological functions during periods of repeated or chronic stress.
Though evidence from knockout studies corroborates the idea that OFQ/N likely serves to dampen the expression of anxiogenic-like behavior, it should be noted that the aforementioned use of OFQ/N-/- mice involved a disruption in the ppOFQ/N gene. As noted earlier, this precursor peptide is cleaved to form other biologically active peptides (Meunier et al., 1995; Okuda-Ashitaka et al., 1998; Reinscheid et al., 2000; Mogil and Pasternak, 2001), such as nocistatin, which can have opposite biological effects to that of OFQ/N (Okuda-Ashitaka et al., 1998; Gavioli et al., 2002). For example, central administration of nocistatin alone increased anxiogenic behavior in rats tested in the EPM, and furthermore, abrogated the anxiolytic-like effects of OFQ/N in this same test (Gavioli et al., 2002). Therefore, behavioral data from mice with disruptions to ppOFQ/N must be carefully interpreted.
Nonetheless, it is encouraging that targeted deletions of NOP-R can produce a similar anxiogenic profile in both mice (Gavioli et al., 2007) and rats (Rizzi et al., 2011). For instance, NOP-R knockout animals (NOP-R-/-) spend less time in the ostensibly anxiety-provoking open areas of the EPM (Gavioli et al., 2007; Rizzi et al., 2011) and the illuminated region of the LD box (Gavioli et al., 2007). However, this not a consistent observation (Mamiya et al., 1998; Gavioli et al., 2007). Whereas Gavioli et al. (2007) describe an anxiogenic-like profile for NOP-R-/- mice in the EPM, Mamiya et al. (1998) observed no behavioral differences between the wildtype and mutant genotypes also tested in the EPM. While not easily explained, it should be noted, that the genetic background on which the NOP-R mutation was generated varied between the two studies. As this is a critical factor in behavioral phenotypes of genetically modified mice, particularly with respect to anxiogenic behavior (Anisman et al., 2008), differences in background strain could have accounted for the disparate findings. For example, attending only to the data from wildtype mice, baseline levels of anxiety differed significantly between the studies (Mamiya et al., 1998; Gavioli et al., 2007), attesting to the importance of background genetics modifying the expression of gene deletions (Anisman et al., 2008).
Leaving aside the issue of background genetics in the interpretation of specific gene deletions, it should be emphasized that variations in the type of behavioral test can also reveal different results. For instance, Gavioli et al. (2007) report no behavioral differences between NOP-R+/+ and NOP-R-/- mice in the OF, hole board and marble burying tests. These findings stand in contrast to other evidence that pharmacological stimulation of NOP-R attenuates anxiogenic-related behaviors in the OF and hole board tests (Florin et al., 1996; Jenck et al., 1997, 2000; Kamei et al., 2004). However, a variety of factors could have accounted for the discrepant findings. As noted by Gavioli et al. (2007), differences in the experimental conditions between their study and others (Florin et al., 1996; Jenck et al., 1997, 2000; Kamei et al., 2004) could have limited their ability to detect differences in anxiogenic-like behavior between the genotypes. It is also possible that other biological mechanisms compensated for the loss of NOP-R signaling throughout development and that these compensatory systems were sufficient to support normal, behavioral responding under certain experimental conditions.
Adding to the confusion emerging from transgenic mouse studies, NOP-R-/- mice exhibited an anxiolytic-like profile when tested in the novelty-induced suppressed feeding behavior test (Gavioli et al., 2007). Briefly, in this test food-deprived mice are placed into the corner of an OF that, prior to testing, was baited with food pellets located in the centermost region of the OF. During testing, the latency to venture out and eat, and the amount consumed (over a 5 min period), was then scored for each animal. Immediately thereafter, mice were returned to their home cages where they were scored using the same behavioral parameters. Anxiety generated by the novel and ostensibly aversive environment (the OF) suppressed the motivation to seek and consume food, resulting in an increased latency to first eat, as well as a reduced food intake. However, upon returning to their home cages, animals quickly approached and consumed a greater amount of food than in the novel environment. Unexpectedly, NOP-R-/- mice exhibited a reduced latency to eat and consumed a greater amount of food than wildtype controls in the OF (Gavioli et al., 2007). This apparent anxiolytic phenotype of NOP-R-/- mice could not be accounted for by differences in appetite, as the body mass lost during deprivation was equivalent between the genotypes. Moreover, NOP-R-/- and NOP-R+/+ mice consumed the same amount of food when returned to their home cages (Gavioli et al., 2007).
A different study by Mallimo et al. (2010) used ORL-1-/- mice (i.e., NOP-R deficient) that received saline and immunologic treatment, and were tested within hours of treatment or several days later. Testing of mice included gustatory neophobia, OF behavior and exposure to a large and unfamiliar, novel object (to test for object neophobia). Of relevance to the current discussion is the behavior of the saline injected (ip) mice. In these mice, it was observed that relative to saline-injected ORL-1+/+ mice, gustatory neophobia was attenuated in ORL-1-/- mice, which is in keeping with the observations of Gavioli et al. (2007) discussed above. Furthermore, and again similar to Gavioli et al. (2007), OF behavior tested several days later was not different between the ORL-1-/- and ORL-1+/+ mice. However, in ORL-1-/- mice, approach and contact with a novel object placed into the OF, was significantly reduced, and time spent away from the object was significantly increased (Mallimo et al., 2010).
These observations suggest that the impact of OFQ/N receptor deletion produces a complicated phenotype that appears to depend on the type of testing conducted on the animals. In particular, the use of an appetitive paradigm, such as those described above, does not unmask an anxiety-like pattern of behavior in mice rendered NOP-R deficient. Furthermore, there are alternative interpretations of the data that could be posited. For instance, a shorter latency to enter the centermost region of an OF to consume food, may reflect impulsive behavior. In line with this idea, UFP-101, a NOP-R antagonist, reduced the latency to first exit the closed arm of an elevated T-maze (ETM) and enter into an open, unprotected arm (Duzzioni et al., 2011). Although a reduced latency could be considered an index of a diminished anxiety-like behavior, it could also be argued that rats with shorter exit latencies are more impulsive than animals demonstrating an appropriate, inhibitory avoidance response. Although speculative, this hypothesis could account for some of the inconsistencies observed in the behavioral phenotype of NOP-R-/- mice. At the very least, it is apparent that endogenous NOP-R differentially modulates anxiogenic-like behavior across different tests of anxiety, even when the behavioral methods employed intend to model the same aspects of anxiety (e.g., innate anxiety).
Concluding Points
In general, most of the behavioral evidence suggests that under conditions of stress, exposure to novel contexts, and learned anxiety and/or fear responses, OFQ/N is recruited to modulate the intensity or severity of behavioral change. Conceptually, it is tempting to think that OFQ/N serves as an attenuating influence on anxiety, although additional research is required to confirm this hypothesis, since not all of the data gathered to date fit neatly into this interpretation (Fernandez et al., 2004; Green et al., 2007). Still, it should be noted that the anxiogenic and anorectic effects of CRH are inhibited by OFQ/N (Ciccocioppo et al., 2003, 2004; Rodi et al., 2008), which reinforces the notion that OFQ/N functions as an opposing influence on neurochemical systems activated by stress. However, the precise effects of such opposition needs to be clarified. Interestingly, a recent study found that individuals who suffer from PTSD, and carry a single-nucleotide polymorphism for the human opioid receptor-like 1 gene (Oprl1), showed greater functional connectivity between the amygdala and the insula during exposure to fearful faces (Andero et al., 2013). Although not a therapeutic study, this study does provide the opportunity to determine whether PTSD symptoms might be better managed by administering OFQ/N receptor agonists. However, further work is going to be needed, since in the same report, ORL-1 receptor stimulation in mice prevented the consolidation of fear memory after an aversive event (ibid). How to implement treatments like this therapeutically without affecting other memory processes necessary for optimal cognitive functioning will require further analysis.
OFQ/N and Inflammation
It is well established that immune factors – primarily cytokines – can impact neurobiological functions under normal and clinical conditions (Dantzer et al., 2008). Cytokines are immune-derived intercellular messengers that are involved in systemic inflammation, and their identification in damaged brain tissue has focused attention on neuroinflammatory mechanisms of brain dysfunction, as well as their possible contributions to brain plasticity and repair. Brain cytokines originate from glial cells, such as astrocytes, oligodendrocytes, and in particular, microglia (Ransohoff and Perry, 2009; Graeber et al., 2011). The regulation of cytokine synthesis and release is carefully orchestrated, since changes in the magnitude and duration of cytokine levels may modify a range of cognitive and emotional functions, including those aligned with classical indices of depressive symptomatology. Indeed, studies of immune challenge (eg., using endotoxin or lipopolysaccharide, LPS), have shown a tripartite of proinflammatory cytokines - interleukin-1β (IL-1β), IL-6 and tumor necrosis factor-alpha (TNFα) – to be the most significant and potent influences on a variety of neural, endocrine and behavioral measures (Dantzer et al., 2008). However, it is now evident that these and other cytokines are part of the brain’s neurochemical milieu operating independently of immune challenge, and which can be elicited by neurotransmitter and neuropeptide systems activated by psychogenic stressors (Albrecht et al., 2010; Spulber and Schultzberg, 2010; Perez Nievas et al., 2011; Stipursky et al., 2011; Wager-Smith and Markou, 2011; Barnum et al., 2012; Santello et al., 2012).
An exciting development over the past decade is that OFQ/N is involved in regulation of neuroinflammatory and immunological processes. Orphanin FQ/nociceptin and its receptor are expressed in various peripheral tissues (Lachowicz et al., 1995; Mollereau et al., 1996) including immune cells and lymphoid organs (Wang et al., 1994), and similar to endogenous opioids, the OFQ/N system plays a role in immunomodulation (Halford et al., 1995; Kawashima et al., 2002; Kato et al., 2005; Rossi-George et al., 2005; Goldfarb et al., 2006; Miller and Fulford, 2007; Finley et al., 2008; Leggett et al., 2009b). Stimulation of NOP-R enhances or suppresses expression of proinflammatory cytokines (Buzas et al., 2002; Kawashima et al., 2002; Fu et al., 2006) or chemokines (Finley et al., 2008), stimulates chemotaxis (Serhan et al., 2001; Trombella et al., 2005), and modulates activation of the HPA axis in response to inflammatory stimuli (Leggett et al., 2009b; Mallimo et al., 2010). In general, the hypothesized role of the nociceptin system is believed to be anti-inflammatory, a function that may be exerted through regulation of cytokine expression. Figure 3 provides a schematic illustration of the major findings discussed below, regarding the immunomodulatory role of OFQ/N in the periphery and CNS.
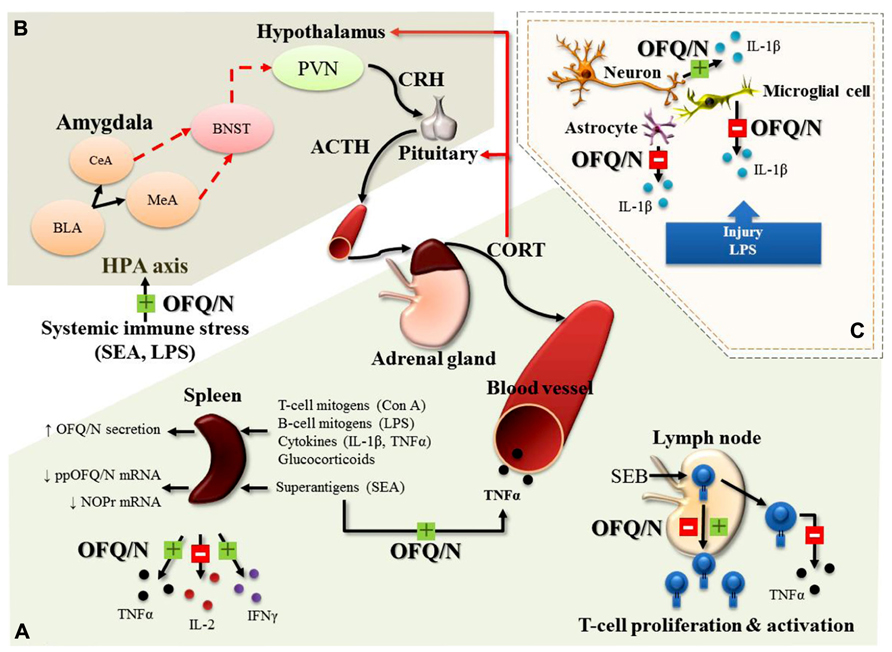
FIGURE 3. Major, immunomodulatory effects of OFQ/N in the periphery (A), feed-forward effects of OFQ/N on the hypothalamic-pituitary-adrenal (HPA) axis response to systemic immune stress (B) and immunomodulatory effects of OFQ/N in the central nervous system (CNS; C). In general, the hypothesized role of the nociceptin system is believed to be anti-inflammatory, a function that may be exerted through regulation of cytokine expression. In response to a wide variety of immunologic stimuli (Con A, concanavalin A; LPS, lipopolysaccharide; IL-1β, Interleukin-1 beta; TNFα, tumor necrosis factor alpha; SEA, staphylococcal enterotoxin A) OFQ/N inhibits (red box with minus) or enhances (green box with plus) the release of inflammatory cytokines (TNFα, interleukin-2; IL-2 and interferon gamma; IFNγ) from splenocytes and lymphocytes and enhances SEA-induced elevations in circulating TNFα. Nociceptin also modulates T-lymphocyte proliferation and activation following superantigenic challenge with staphylococcal enterotoxin B (SEB; A). In line with its feed-forward effects on the HPA axis response to psychogenic stress, OFQ/N exerts stimulatory effects on the HPA axis response to systemic immune stress. Specifically, nociceptin signaling prolongs elevations in circulating CORT induced by systemic administration of SEA or LPS (B). In the central nervous system (CNS), OFQ/N modulates the release inflammatory cytokines. Notably, nociceptin signaling appears to regulate, in a cell-specific manner, the expression of IL-1β. In response to traumatic injury or LPS, OFQ/N attenuates the release of IL-1β from microglial cells and astrocytes. Conversely, nociceptin signaling enhances the release of IL-1β from neurons (C). Abbreviations used: corticotropin releasing hormone; CRH, adrenocorticotropin hormone; ACTH, paraventricular nucleus; PVN, bed nucleus of stria terminalis; BNST, central amygdala; CeA, medial amygdala; MeA, basolateral amygdala; BLA, basolateral amygdala.
In the immune system, Miller and Fulford (2007) determined that OFQ/N secretion from lymphocytes and splenocytes (in vitro) is increased following exposure to various inflammatory mediators such as T (Concanavalin A; Con A) and B cell (LPS) mitogens, the proinflammatory cytokines IL-1β and TNFα, and synthetic glucocorticoids. Moreover, OFQ/N suppressed production of IL-2 from cultured splenocytes and significantly attenuated splenocyte proliferation in response to Con A stimulation, an effect that was inhibited by ORL-1 receptor antagonists (Miller and Fulford, 2007). There is also evidence to suggest that OFQ/N may have immunomodulatory properties in vivo. For instance, ORL-1-/-receptor deficient mice (ORL-1-/-) exhibited an exaggerated splenic IL-2 response 4 h after systemic challenge with the bacterial T lymphocyte “superantigen,” staphylococcal enterotoxin A (SEA; Mallimo et al., 2010). This finding is in good agreement with that of Miller and Fulford (2007) and the idea that signaling through the OFQ/N receptor limits the production of IL-2 following T cell activation.
On the other hand, intraperitoneal injection (ip) of OFQ/N to C57BL/6 mice, prior to challenge with SEA, produced an enhancement of splenic TNFα and IFNγ mRNA expression and increased plasma levels of TNFα (Goldfarb et al., 2006). Conversely, in the same study, ppOFQ/N-/- mice challenged with SEA showed diminished mRNA for TNFα and IFNγ (ibid). The stimulatory effect of OFQ/N on TNFα and IFNγ may be necessary to ensure optimal levels of cytokine production at later time points following T cell stimulation. In line with this idea, ORL-1-/- mice exhibited an attenuated splenic, TNFα response 4 h after systemic challenge with SEA (Mallimo et al., 2010). Collectively, these findings suggest that activation of the nociceptin system may play an important role in the balance of pro- and anti-inflammatory cytokine production. These effects of SEA may involve interactions at the level of T lymphocytes. That is, SEA is selective for T cells (Goldfarb et al., 2006), and T cells are known to express NOP-R, the receptor for OFQ/N (Peluso et al., 1998). Indeed, addition of OFQ/N to human T cell cultures stimulated with another superantigen, staphylococcal enterotoxin B (SEB), result in enhanced proliferation, but reduced TNFα production (Waits et al., 2004). Interestingly, restimulation of the same T cells resulted in OFQ/N causing a reduction in proliferative responding (ibid). A later study showed that cultured purified CD4+ T cells from human peripheral blood had attenuated proliferative responding to primary SEB exposure when OFQ/N was introduced into cell culture (Fiset et al., 2003; Easten et al., 2009). From these studies it is evident that OFQ/N has immunomodulatory properties that can target T cell function, although the direction of its effects on cytokine production and T cell proliferation require further characterization.
In keeping with in vivo studies that provided good evidence for OFQ/N-dependent immunomodulation (Goldfarb et al., 2006), additional studies are available to show the clinical applicability of these effects. In a rat study of experimental colitis, it was demonstrated that pathology and inflammatory cytokine production (eg., IL-1β) were diminished after two daily injections of low dose (0.02 and 0.2 nmol/Kg) OFQ/N, but were exacerbated when the dose was substantially increased (20 nmol/Kg; Petrella et al., 2013). The implications of this finding are consistent with an earlier observation that OFQ/N elevations may negatively impact survival during infection. That is, subsequent to the induction of sepsis by cecal ligation and puncture, OFQ/N administration to rats increased mortality, while NOP-R antagonist treatment attenuated various cellular and cytokine parameters of inflammation, and reduced mortality (Carvalho et al., 2008). Corresponding data in human clinical situations has been noted, with higher correlations of plasma OFQ/N in non-surviving septic patients suggesting that antagonism of OFQ/N may be a potential immunotherapeutic manipulation that may aid recovery (Serrano-Gomez et al., 2011). Overall, while little is known about the precise interactions between OFQ/N and the immune system, there is compelling evidence to explore this relationship further and elucidate the relevance of this to immunopathological and inflammatory disorders.
It is well established that systemic immune responses can produce changes in various neurobiological outcomes. In keeping with various CNS changes in neuropeptides and monamine neurotransmitters after immune challenge (Anisman et al., 2008), mice challenged with the bacterial T cell superantigen, SEA, showed increased ppOFQ/N mRNA in the hypothalamus and amygdala (Kawashima et al., 2002). In addition, SEA challenge activates the HPA axis and neuronal induction of the immediate early gene c-fos in various limbic brain regions, including the amygdala and hypothalamus (Rossi-George et al., 2005). Since these effects are known to depend on TNF (Rossi-George et al., 2005), it is possible that SEA-induced ppOFQ/N mRNA changes in the brain, are similarly dependent on the presence of TNF. Moreover, in the absence of ORL-1 signaling, the duration of the plasma CORT response to SEA (Mallimo et al., 2010) and to LPS (Leggett et al., 2009a) is reduced, suggesting that mobilization of central OFQ/N may promote a prolongation of the HPA axis response to systemic immune stress.
In addition to T cell antigens, strong proinflammatory inducers, such as LPS, which targets monocytes and macrophages, have been shown to induce OFQ/N production by cultured sensory neurons obtained from the dorsal root ganglion, an effect that appears to be dependent on the Toll-like receptor 4 (TLR4; Acosta and Davies, 2008). This may be relevant to nociception, as inflammation-related pain may be due to regulation of the hypoalgesic effects of OFQ/N, suggesting that NOP-R and OFQ/N are recruited by inflammatory stimuli to coordinate and control levels of pain perception (Depner et al., 2003; Chen and Sommer, 2007; Fu et al., 2007a). For instance, formalin and zymosan induce inflammation and induction of pain, although lower thresholds of pain sensitivity were evident in mice that lacked either ppOFQ/N and/or NOP-R (Depner et al., 2003). Furthermore, areas of inflammation in peripheral, as well as central, nervous tissue most likely attract infiltration of leukocytes (Prendergast and Anderton, 2009). Such cellular infiltrates may further contribute to the concentration of local neuron-derived OFQ/N, since neutrophils are known to produce OFQ/N (Fiset et al., 2003). Finally, as detailed below, astrocytes express NOP-R and cytokine production by astrocytes can be inhibited by OFQ/N, an effect which represents one mechanism by which OFQ/N may reduce pain sensitivity (Fu et al., 2007b).
In recent years there has been a resurgence of interest in glial cell function in the CNS, and in particular, how this contributes to and/or regulates neuroinflammatory processes in neurodegenerative conditions. The influence of OFQ/N on glial function has been suggested through a variety of different in vitro and in vivo studies. For example, NOP-R engagement may influence oligodendrocyte development, since the modulation of myelin basic protein concentrations subsequent to prenatal treatment with the opioid agonist, buprenorphine, were shown to be dependent on NOP-R expression (Eschenroeder et al., 2012). Similarly, with regard to astrocyte function, NOP-R knockout mice treated with methamphetamine have augmented GFAP expression in the striatum (Sakoori and Murphy, 2010), which is in keeping with other evidence that spinal cord astrocyte activation and in vitro cytokine production by astrocytes is attenuated by OFQ/N via ORL-1, which is expressed on astrocytes (Fu et al., 2007b). Interestingly, ppOFQ/N mRNA is increased in astrocytes treated with morphine (Takayama and Ueda, 2005), while LPS, and various proinflammatory cytokines (eg., tumor necrosis factor and interleukin-1) can increase astrocyte concentrations of immunoreactive OFQ/N and ppOFQ/N mRNA (Buzas et al., 2002). Finally, following neural trauma in rats, LPS treatment increased OFQ/N co-localized with brain astrocytes (Zhao et al., 2002). This observation was made in the context of a corresponding investigation of microglial cells, and is perhaps the only major instance where OFQ/N was linked to this intensively studied category of glial cells.
Microglia essentially are the tissue macrophages of the brain and spinal cord, and as such, likely exert an “immune-like” influence through phagocytosis and production of proinflammatory cytokines like TNFα and IL-1β. In the study by Zhao et al. (2002), rats given icv infusion of OFQ/N showed a significantly reduced number of activated microglia following neural trauma. In addition, LPS induced upregulation of IL-1β mRNA in cultured microglial cells, and this was inhibited by application of OFQ/N (Fu et al., 2006). This effect was blocked by co-administration of an ORL-1 receptor antagonist, confirming that downregulation of Il-1β mRNA transcripts in microglia of the CNS is ORL-1 dependent. However, when applied to cultured neurons, OFQ/N significantly increased LPS-induced upregulation of IL-1β mRNA (Fu et al., 2006). The differential effect of OFQ/N on IL-1β gene expression on microglial and neuronal preparations was suggested to reflect a disturbance in the endogenous synaptic structural arrangement between these cell types. Thus, microglia in vivo may exert a direct inhibitory action on neurons and when this connection is disturbed, neurons may upregulate IL-1β mRNA instead (Fu et al., 2006). This is a compelling hypothesis, that is hampered by a relative paucity of additional studies to corroborate and expand on the effects of OFQ/N on microglial cells.
In conclusion, although relatively small, there is a potentially significant literature relating OFQ/N to glial cell function. A compelling view that might be elaborated and pursued is that of OFQ/N mediated reduction of proinflammatory cytokine production during various conditions of trauma, degeneration and systemic inflammation due to infection and other immunologically relevant conditions (eg., autoimmune disease). It seems plausible, for example, that OFQ/N may also serve to modify the various sickness behaviors – cognitive, motoric and emotional – that arise from exposure to immunological stimuli. This would fit the overall – but not overwhelming – view that OFQ/N serves to attenuate stress-related behavioral reactions, as reviewed earlier. Extending this idea further, it has been demonstrated that stress can activate microglial cells in the brain (Sugama et al., 2007, 2009; Hinwood et al., 2012), and furthermore, stress can also increase the number of astrocytes that express IL-1β (Sugama et al., 2011). Given that psychogenic and immunologic stressors can increase OFQ/N expression in the brain (Kawashima et al., 2002; Nativio et al., 2012), it could be hypothesized that an increase in OFQ/N may serve to regulate glial cell activation and the production of cytokines, like IL-1β (Zhao et al., 2002). Whether such interactions exist between central OFQ/N changes and neuroinflammatory events remains to be determined.
Summary and Future Directions
The current review has focused on bringing together three elements of OFQ/N biology that are potentially linked, and may hold promise for future studies on brain health and neuroplasticity. Given that many of the studies addressing the role of OFQ/N on the HPA axis and anxiety-related behavior have focused on acute stressor exposure, greater attention is needed to address the relationship of OFQ/N to neuroplasticity during conditions of chronic stress. Chronic stress has long been associated as a contributor and/or trigger for anxiety and mood disorders(Charney, 2003; Feder et al., 2009), and the pursuit of the molecular and cellular targets of stress-activated neurotransmitter systems (eg., the monoamines) has shifted to the importance of neuroinflammatory cytokines and glial cells, which express receptors for multiple neurotransmitters (Kettenmann et al., 2011). Indeed, as stated above, microglial cells, are activated under a range of conditions, including strokes, physical trauma, demyelination, and neurodegeneration or neuronal cell death (Ransohoff and Perry, 2009). Of particular interest is that the activated phenotype of a microglial cell can be produced by in vitro exposure to neurotransmitters (eg., norepinephrine; Heneka et al., 2010), as well as psychogenic stressors, which increase neurotransmitter synthesis and release(Anisman et al., 2005). Indeed, psychogenic stressors alone, in the absence of immune activation in the periphery, can activate brain microglial cells and modify cytokine levels in the brain (Frank et al., 2007; Wohleb et al., 2011; Hinwood et al., 2012; Gibb et al., 2008, 2011). What is relevant and novel to this scenario is the knowledge that OFQ/N plays an immunomodulatory role that can modify a number of proinflammatory cytokines, including IL-1β, TNF-α and IL-2. This serves to move the relationship of OFQ/N to stress and behavior, into a scheme that also incorporates the contribution of neuroinflammatory functions that might be regulated or modified by OFQ/N. This new direction may yield clues for the treatment of neuropsychiatric clinical conditions, in which neuroinflammation is suspected of contributing to depression, cognitive decline and neurodegeneration.
Conflict of Interest Statement
The authors declare that the research was conducted in the absence of any commercial or financial relationships that could be construed as a potential conflict of interest.
References
Acosta, C., and Davies, A. (2008). Bacterial lipopolysaccharide regulates nociceptin expression in sensory neurons. J. Neurosci. Res. 86, 1077–1086. doi: 10.1002/jnr.21565
Albrecht, J., Sidoryk-Wegrzynowicz, M., Zielinska, M., and Aschner, M. (2010). Roles of glutamine in neurotransmission. Neuron Glia Biol. 6, 263–276. doi: 10.1017/S1740925X11000093
Altier, C., and Zamponi, G. W. (2008). Signaling complexes of voltage-gated calcium channels and G protein-coupled receptors. J. Recept. Signal Transduct. Res. 28, 71–81. doi: 10.1080/10799890801941947
Andero, R., Brothers, S. P., Jovanovic, T., Chen, Y. T., Salah-Uddin, H., Cameron, M., et al. (2013). Amygdala-dependent fear is regulated by Oprl1 in mice and humans with PTSD. Sci. Transl. Med. 5, 188ra173.
Anisman, H., Merali, Z., and Hayley, S. (2008). Neurotransmitter, peptide and cytokine processes in relation to depressive disorder: comorbidity between depression and neurodegenerative disorders. Prog. Neurobiol. 85, 1–74. doi: 10.1016/j.pneurobio.2008.01.004
Anisman, H., Merali, Z., Poulter, M. O., and Hayley, S. (2005). Cytokines as a precipitant of depressive illness: animal and human studies. Curr. Pharm. Des. 11, 963–972. doi: 10.2174/1381612053381701
Barnum, C. J., Pace, T. W., Hu, F., Neigh, G. N., and Tansey, M. G. (2012). Psychological stress in adolescent and adult mice increases neuroinflammation and attenuates the response to LPS challenge. J. Neuroinflammation 9, 9. doi: 10.1186/1742-2094-9-9
Bianchi, C., Marani, L., Barbieri, M., Marino, S., Beani, L., and Siniscalchi, A. (2004). Effects of nociceptin/orphanin FQ and endomorphin-1 on glutamate and GABA release, intracellular [Ca2+] and cell excitability in primary cultures of rat cortical neurons. Neuropharmacology 47, 873–883. doi: 10.1016/j.neuropharm.2004.06.017
Bunzow, J. R., Saez, C., Mortrud, M., Bouvier, C., Williams, J. T., Low, M., et al. (1994). Molecular cloning and tissue distribution of a putative member of the rat opioid receptor gene family that is not a mu, delta or kappa opioid receptor type. FEBS Lett. 347, 284–288. doi: 10.1016/0014-5793(94)00561-3
Buzas, B., Rosenberger, J., Kim, K. W., and Cox, B. M. (2002). Inflammatory mediators increase the expression of nociceptin/orphanin FQ in rat astrocytes in culture. Glia 39, 237–246. doi: 10.1002/glia.10106
Carvalho, D., Petronilho, F., Vuolo, F., Machado, R. A., Constantino, L., Guerrini, R., et al. (2008). The nociceptin/orphanin FQ-NOP receptor antagonist effects on an animal model of sepsis. Intensive Care Med. 34, 2284–2290. doi: 10.1007/s00134-008-1313-3
Cavallini, S., Marino, S., Beani, L., Bianchi, C., and Siniscalchi, A. (2003). Nociceptin inhibition of acetylcholine efflux from different brain areas. Neuroreport 14, 2167–2170. doi: 10.1097/00001756-200312020-00007
Charney, D. S. (2003). Neuroanatomical circuits modulating fear and anxiety behaviors. Acta Psychiatr. Scand. Suppl. 38–50. doi: 10.1034/j.1600-0447.108.s417.3.x
Chen, Y., and Sommer, C. (2007). Activation of the nociceptin opioid system in rat sensory neurons produces antinociceptive effects in inflammatory pain: involvement of inflammatory mediators. J. Neurosci. Res. 85, 1478–1488. doi: 10.1002/jnr.21272
Ciccocioppo, R., Cippitelli, A., Economidou, D., Fedeli, A., and Massi, M. (2004). Nociceptin/orphanin FQ acts as a functional antagonist of corticotropin-releasing factor to inhibit its anorectic effect. Physiol. Behav. 82, 63–68. doi: 10.1016/j.physbeh.2004.04.035
Ciccocioppo, R., Fedeli, A., Economidou, D., Policani, F., Weiss, F., and Massi, M. (2003). The bed nucleus is a neuroanatomical substrate for the anorectic effect of corticotropin-releasing factor and for its reversal by nociceptin/orphanin FQ. J. Neurosci. 23, 9445–9451.
Cruz, M. T., Herman, M. A., Kallupi, M., and Roberto, M. (2012). Nociceptin/orphanin FQ blockade of corticotropin-releasing factor-induced gamma-aminobutyric acid release in central amygdala is enhanced after chronic ethanol exposure. Biol. Psychiatry 71, 666–676. doi: 10.1016/j.biopsych.2011.10.032
Dantzer, R., O’Connor, J. C., Freund, G. G., Johnson, R. W., and Kelley, K. W. (2008). From inflammation to sickness and depression: when the immune system subjugates the brain. Nat. Rev. Neurosci. 9, 46–56. doi: 10.1038/nrn2297
Dawe, K. L., Wakerley, J. B., and Fulford, A. J. (2010). Nociceptin/orphanin FQ and the regulation of neuronal excitability in the rat bed nucleus of the stria terminalis: interaction with glucocorticoids. Stress 13, 516–527.
Del Giudice, M. R., Borioni, A., Bastanzio, G., Sbraccia, M., Mustazza, C., and Sestili, I. (2011). Synthesis and pharmacological evaluation of bivalent antagonists of the nociceptin opioid receptor. Eur. J. Med. Chem. 46, 1207–1221. doi: 10.1016/j.ejmech.2011.01.040
Delaney, G., Dawe, K. L., Hogan, R., Hunjan, T., Roper, J., Hazell, G., et al. (2012). Role of nociceptin/orphanin FQ and NOP receptors in the response to acute and repeated restraint stress in rats. J. Neuroendocrinol. 24, 1527–1541. doi: 10.1111/j.1365-2826.2012.02361.x
Depner, U. B., Reinscheid, R. K., Takeshima, H., Brune, K., and Zeilhofer, H. U. (2003). Normal sensitivity to acute pain, but increased inflammatory hyperalgesia in mice lacking the nociceptin precursor polypeptide or the nociceptin receptor. Eur. J. Neurosci. 17, 2381–2387. doi: 10.1046/j.1460-9568.2003.02676.x
Devine, D. P., Hoversten, M. T., Ueda, Y., and Akil, H. (2003). Nociceptin/orphanin FQ content is decreased in forebrain neurones during acute stress. J. Neuroendocrinol. 15, 69–74. doi: 10.1046/j.1365-2826.2003.00868.x
Devine, D. P., Taylor, L., Reinscheid, R. K., Monsma, F. J. Jr., Civelli, O., and Akil, H. (1996). Rats rapidly develop tolerance to the locomotor-inhibiting effects of the novel neuropeptide orphanin FQ. Neurochem. Res. 21, 1387–1396. doi: 10.1007/BF02532380
Devine, D. P., Watson, S. J., and Akil, H. (2001). Nociceptin/orphanin FQ regulates neuroendocrine function of the limbic-hypothalamic-pituitary-adrenal axis. Neuroscience 102, 541–553. doi: 10.1016/S0306-4522(00)00517-0
Duzzioni, M., Duarte, F. S., Leme, L. R., Gavioli, E. C. and De Lima, T. C. (2011) Anxiolytic-like effect of central administration of NOP receptor antagonist UFP-101 in rats submitted to the elevated T-maze. Behav. Brain Res. 222, 206–211. doi: 10.1016/j.bbr.2011.03.056
Easten, K. H., Harry, R. A., Purcell, W. M., and McLeod, J. D. (2009). Nociceptin-induced modulation of human T cell function. Peptides 30, 926–934. doi: 10.1016/j.peptides.2009.01.021
Eschenroeder, A. C., Vestal-Laborde, A. A., Sanchez, E. S., Robinson, S. E., and Sato-Bigbee, C. (2012). Oligodendrocyte responses to buprenorphine uncover novel and opposing roles of mu-opioid- and nociceptin/orphanin FQ receptors in cell development: implications for drug addiction treatment during pregnancy. Glia 60, 125–136. doi: 10.1002/glia.21253
Feder, A., Nestler, E. J., and Charney, D. S. (2009). Psychobiology and molecular genetics of resilience. Nat. Rev. Neurosci. 10, 446–457. doi: 10.1038/nrn2649
Fernandez, F., Misilmeri, M. A., Felger, J. C., and Devine, D. P. (2004). Nociceptin/orphanin FQ increases anxiety-related behavior and circulating levels of corticosterone during neophobic tests of anxiety. Neuropsychopharmacology 29, 59–71. doi: 10.1038/sj.npp.1300308
Finley, M. J., Happel, C. M., Kaminsky, D. E., and Rogers, T. J. (2008). Opioid and nociceptin receptors regulate cytokine and cytokine receptor expression. Cell. Immunol. 252, 146–154. doi: 10.1016/j.cellimm.2007.09.008
Fiset, M. E., Gilbert, C., Poubelle, P. E., and Pouliot, M. (2003). Human neutrophils as a source of nociceptin: a novel link between pain and inflammation. Biochemistry 42, 10498–10505. doi: 10.1021/bi0300635
Florin, S., Suaudeau, C., Meunier, J. C., and Costentin, J. (1996). Nociceptin stimulates locomotion and exploratory behaviour in mice. Eur. J. Pharmacol. 317, 9–13. doi: 10.1016/S0014-2999(96)00707-8
Frank, M. G., Baratta, M. V., Sprunger, D. B., Watkins, L. R., and Maier, S. F. (2007). Microglia serve as a neuroimmune substrate for stress-induced potentiation of CNS pro-inflammatory cytokine responses. Brain Behav. Immun. 21, 47–59. doi: 10.1016/j.bbi.2006.03.005
Fu, X., Wang, Y. Q., Wang, J., Yu, J., and Wu, G. C. (2007a). Changes in expression of nociceptin/orphanin FQ and its receptor in spinal dorsal horn during electroacupuncture treatment for peripheral inflammatory pain in rats. Peptides 28, 1220–1228. doi: 10.1016/j.peptides.2007.03.022
Fu, X., Zhu, Z. H., Wang, Y. Q., and Wu, G. C. (2007b). Regulation of proinflammatory cytokines gene expression by nociceptin/orphanin FQ in the spinal cord and the cultured astrocytes. Neuroscience 144, 275–285. doi: 10.1016/j.neuroscience.2006.09.016
Fu, X., Wang, Y. Q., and Wu, G. C. (2006). Involvement of nociceptin/orphanin FQ and its receptor in electroacupuncture-produced anti-hyperalgesia in rats with peripheral inflammation. Brain Res. 1078, 212–218. doi: 10.1016/j.brainres.2006.01.026
Fukuda, K., Kato, S., Mori, K., Nishi, M., Takeshima, H., Iwabe, N., et al. (1994). cDNA cloning and regional distribution of a novel member of the opioid receptor family. FEBS Lett. 343, 42–46. doi: 10.1016/0014-5793(94)80603-9
Gavioli, E. C., Rae, G. A., Calo, G., Guerrini, R., and De Lima, T. C. (2002). Central injections of nocistatin or its C-terminal hexapeptide exert anxiogenic-like effect on behaviour of mice in the plus-maze test. Br. J. Pharmacol. 136, 764–772. doi: 10.1038/sj.bjp.0704739
Gavioli, E. C., Rizzi, A., Marzola, G., Zucchini, S., Regoli, D., and Calo, G. (2007). Altered anxiety-related behavior in nociceptin/orphanin FQ receptor gene knockout mice. Peptides 28, 1229–1239. doi: 10.1016/j.peptides.2007.04.012
Gibb, J., Hayley, S., Gandhi, R., Poulter, M. O., and Anisman, H. (2008). Synergistic and additive actions of a psychosocial stressor and endotoxin challenge: circulating and brain cytokines, plasma corticosterone and behavioral changes in mice. Brain Behav. Immun. 22, 573–589. doi: 10.1016/j.bbi.2007.12.001
Gibb, J., Hayley, S., Poulter, M. O., and Anisman, H. (2011). Effects of stressors and immune activating agents on peripheral and central cytokines in mouse strains that differ in stressor responsivity. Brain Behav. Immun. 25, 468–482. doi: 10.1016/j.bbi.2010.11.008
Goeldner, C., Spooren, W., Wichmann, J., and Prinssen, E. P. (2012). Further characterization of the prototypical nociceptin/orphanin FQ peptide receptor agonist Ro 64-6198 in rodent models of conflict anxiety and despair. Psychopharmacology (Berl.) 222, 203–214. doi: 10.1007/s00213-012-2636-x
Goldfarb, Y., Reinscheid, R. K., and Kusnecov, A. W. (2006). Orphanin FQ/nociceptin interactions with the immune system in vivo: gene expression changes in lymphoid organs and regulation of the cytokine response to staphylococcal enterotoxin A. J. Neuroimmunol. 176, 76–85. doi: 10.1016/j.jneuroim.2006.04.008
Gompf, H. S., Moldavan, M. G., Irwin, R. P., and Allen, C. N. (2005). Nociceptin/orphanin FQ (N/OFQ) inhibits excitatory and inhibitory synaptic signaling in the suprachiasmatic nucleus (SCN). Neuroscience 132, 955–965. doi: 10.1016/j.neuroscience.2004.11.057
Graeber, M. B., Li, W., and Rodriguez, M. L. (2011). Role of microglia in CNS inflammation. FEBS Lett. 585, 3798–3805. doi: 10.1016/j.febslet.2011.08.033
Green, M. K., Barbieri, E. V., Brown, B. D., Chen, K. W., and Devine, D. P. (2007). Roles of the bed nucleus of stria terminalis and of the amygdala in N/OFQ-mediated anxiety and HPA axis activation. Neuropeptides 41, 399–410. doi: 10.1016/j.npep.2007.09.002
Green, M. K., and Devine, D. P. (2009). Nociceptin/orphanin FQ and NOP receptor gene regulation after acute or repeated social defeat stress. Neuropeptides 43, 507–514. doi: 10.1016/j.npep.2009.08.003
Halford, W. P., Gebhardt, B. M., and Carr, D. J. (1995). Functional role and sequence analysis of a lymphocyte orphan opioid receptor. J. Neuroimmunol. 59, 91–101. doi: 10.1016/0165-5728(95)00030-6
Hawes, B. E., Graziano, M. P., and Lambert, D. G. (2000). Cellular actions of nociceptin: transduction mechanisms. Peptides 21, 961–967. doi: 10.1016/S0196-9781(00)00232-1
Heneka, M. T., Nadrigny, F., Regen, T., Martinez-Hernandez, A., Dumitrescu-Ozimek, L., Terwel, D., et al. (2010). Locus ceruleus controls Alzheimer’s disease pathology by modulating microglial functions through norepinephrine. Proc. Natl. Acad. Sci. U.S.A. 107, 6058–6063. doi: 10.1073/pnas.0909586107
Herman, J. P., McKlveen, J. M., Solomon, M. B., Carvalho-Netto, E., and Myers, B. (2012). Neural regulation of the stress response: glucocorticoid feedback mechanisms. Braz. J. Med. Biol. Res. 45, 292–298. doi: 10.1590/S0100-879X2012007500041
Hinwood, M., Morandini, J., Day, T. A., and Walker, F. R. (2012). Evidence that microglia mediate the neurobiological effects of chronic psychological stress on the medial prefrontal cortex. Cereb. Cortex 22, 1442–1454. doi: 10.1093/cercor/bhr229
Ikeda, K., Watanabe, M., Ichikawa, T., Kobayashi, T., Yano, R., and Kumanishi, T. (1998). Distribution of prepro-nociceptin/orphanin FQ mRNA and its receptor mRNA in developing and adult mouse central nervous systems. J. Comp. Neurol. 399, 139–151. doi: 10.1002/(SICI)1096-9861(19980914)399:1<139::AID-CNE11>3.0.CO;2-C
Itoh, K., Konya, H., Takai, E., Masuda, H., and Nagai, K. (1999). Modification of acetylcholine release by nociceptin in conscious rat striatum. Brain Res. 845, 242–245. doi: 10.1016/S0006-8993(99)01954-X
Jankord, R., and Herman, J. P. (2008). Limbic regulation of hypothalamo-pituitary-adrenocortical function during acute and chronic stress. Ann. N. Y. Acad. Sci. 1148, 64–73. doi: 10.1196/annals.1410.012
Jenck, F., Moreau, J. L., Martin, J. R., Kilpatrick, G. J., Reinscheid, R. K., Monsma, F. J. Jr., et al. (1997). Orphanin FQ acts as an anxiolytic to attenuate behavioral responses to stress. Proc. Natl. Acad. Sci. U.S.A. 94, 14854–14858. doi: 10.1073/pnas.94.26.14854
Jenck, F., Wichmann, J., Dautzenberg, F. M., Moreau, J. L., Ouagazzal, A. M., Martin, J. R., et al. (2000). A synthetic agonist at the orphanin FQ/nociceptin receptor ORL1: anxiolytic profile in the rat. Proc. Natl. Acad. Sci. U.S.A. 97, 4938–4943. doi: 10.1073/pnas.090514397
Kamei, J., Matsunawa, Y., Miyata, S., Tanaka, S., and Saitoh, A. (2004). Effects of nociceptin on the exploratory behavior of mice in the hole-board test. Eur. J. Pharmacol. 489, 77–87. doi: 10.1016/j.ejphar.2003.12.020
Kato, S., Tsuzuki, Y., Hokari, R., Okada, Y., Miyazaki, J., Matsuzaki, K., et al. (2005). Role of nociceptin/orphanin FQ (Noc/oFQ) in murine experimental colitis. J. Neuroimmunol. 161, 21–28. doi: 10.1016/j.jneuroim.2004.12.006
Kawashima, N., Fugate, J., and Kusnecov, A. W. (2002). Immunological challenge modulates brain orphanin FQ/nociceptin and nociceptive behavior. Brain Res. 949, 71–78. doi: 10.1016/S0006-8993(02)02966-9
Kettenmann, H., Hanisch, U. K., Noda, M., and Verkhratsky, A. (2011). Physiology of microglia. Physiol. Rev. 91, 461–553. doi: 10.1152/physrev.00011.2010
Koob, G. F. (2008). A role for brain stress systems in addiction. Neuron 59, 11–34. doi: 10.1016/j.neuron.2008.06.012
Koster, A., Montkowski, A., Schulz, S., Stube, E. M., Knaudt, K., Jenck, F., et al. (1999). Targeted disruption of the orphanin FQ/nociceptin gene increases stress susceptibility and impairs stress adaptation in mice. Proc. Natl. Acad. Sci. U.S.A. 96, 10444–10449. doi: 10.1073/pnas.96.18.10444
Kuzmin, A., Madjid, N., Johansson, B., Terenius, L., and Ogren, S. O. (2009). The nociceptin system and hippocampal cognition in mice: a pharmacological and genetic analysis. Brain Res. 1305(Suppl.), S7–S19. doi: 10.1016/j.brainres.2009.09.075
Lachowicz, J. E., Shen, Y., Monsma, F. J. Jr., and Sibley, D. R. (1995). Molecular cloning of a novel G protein-coupled receptor related to the opiate receptor family. J. Neurochem. 64, 34–40. doi: 10.1046/j.1471-4159.1995.64010034.x
Leggett, J. D., Dawe, K. L., Jessop, D. S., and Fulford, A. J. (2009a). Endogenous nociceptin/orphanin FQ system involvement in hypothalamic-pituitary-adrenal axis responses: relevance to models of inflammation. J. Neuroendocrinol. 21, 888–897. doi: 10.1111/j.1365-2826.2009.01912.x
Leggett, J. D., Dawe, K. L., Jessop, D. S., and Fulford, A. J. (2009b). Endogenous nociceptin/orphanin FQ system involvement in HPA axis responses: relevance to models of inflammation. J. Neuroendocrinol. 21, 888–897. doi: 10.1111/j.1365-2826.2009.01912.x
Leggett, J. D., Harbuz, M. S., Jessop, D. S., and Fulford, A. J. (2006). The nociceptin receptor antagonist [Nphe1,Arg14,Lys15]nociceptin/ orphanin FQ-NH2 blocks the stimulatory effects of nociceptin/orphanin FQ on the HPA axis in rats. Neuroscience 141, 2051–2057. doi: 10.1016/j.neuroscience.2006.05.036
Leggett, J. D., Jessop, D. S., and Fulford, A. J. (2007). The nociceptin/orphanin FQ antagonist UFP-101 differentially modulates the glucocorticoid response to restraint stress in rats during the peak and nadir phases of the hypothalamo-pituitary-adrenal axis circadian rhythm. Neuroscience 147, 757–764. doi: 10.1016/j.neuroscience.2007.04.010
Mahmoud, S., Margas, W., Trapella, C., Calo, G., and Ruiz-Velasco, V. (2010). Modulation of silent and constitutively active nociceptin/orphanin FQ receptors by potent receptor antagonists and Na+ ions in rat sympathetic neurons. Mol. Pharmacol. 77, 804–817. doi: 10.1124/mol.109.062208
Mallimo, E. M., Ansonoff, M. A., Pintar, J. E., and Kusnecov, A. W. (2010). Role of opioid receptor like-1 receptor in modulation of endocrine, immunological, and behavioral responses to the T-cell superantigen staphylococcal enterotoxin A. J. Neuroimmunol. 218, 48–56. doi: 10.1016/j.jneuroim.2009.10.014
Mamiya, T., Noda, Y., Nishi, M., Takeshima, H., and Nabeshima, T. (1998). Enhancement of spatial attention in nociceptin/orphanin FQ receptor-knockout mice. Brain Res. 783, 236–240. doi: 10.1016/S0006-8993(97)01406-6
Matta, S. G., Fu, Y., Valentine, J. D., and Sharp, B. M. (1998). Response of the hypothalamo-pituitary-adrenal axis to nicotine. Psychoneuroendocrinology 23, 103–113. doi: 10.1016/S0306-4530(97)00079-6
Meis, S., and Pape, H. C. (2001). Control of glutamate and GABA release by nociceptin/orphanin FQ in the rat lateral amygdala. J. Physiol. 532, 701–712. doi: 10.1111/j.1469-7793.2001.0701e.x
Meunier, J. C., Mollereau, C., Toll, L., Suaudeau, C., Moisand, C., Alvinerie, P., et al. (1995). Isolation and structure of the endogenous agonist of opioid receptor-like ORL1 receptor. Nature 377, 532–535. doi: 10.1038/377532a0
Miller, T. R., and Fulford, A. J. (2007). Regulation of nociceptin/orphaninFQ secretion by immune cells and functional modulation of interleukin-2. Peptides 28, 2243–2252. doi: 10.1016/j.peptides.2007.09.004
Mogil, J. S., and Pasternak, G. W. (2001). The molecular and behavioral pharmacology of the orphaninFQ/nociceptin peptide and receptor family. Pharmacol. Rev. 53, 381–415.
Mollereau, C., Parmentier, M., Mailleux, P., Butour, J. L., Moisand, C., Chalon, P., et al. (1994). ORL1, a novel member of the opioid receptor family. Cloning, functional expression and localization. FEBS Lett. 341, 33–38. doi: 10.1016/0014-5793(94)80235-1
Mollereau, C., Simons, M. J., Soularue, P., Liners, F., Vassart, G., Meunier, J. C., et al. (1996). Structure, tissue distribution, and chromosomal localization of the prepronociceptin gene. Proc. Natl. Acad. Sci. U.S.A. 93, 8666–8670. doi: 10.1073/pnas.93.16.8666
Nabeshima, T., Noda, Y., and Mamiya, T. (1999). The role of nociceptin in cognition. Brain Res. 848, 167–173. doi: 10.1016/S0006-8993(99)01906-X
Nativio, P., Pascale, E., Maffei, A., Scaccianoce, S., and Passarelli, F. (2012). Effect of stress on hippocampal nociceptin expression in the rat. Stress 15, 378–384. doi: 10.3109/10253890.2011.627071
Neal, C. R. Jr., Mansour, A., Reinscheid, R., Nothacker, H. P., Civelli, O., Akil, H., et al. (1999a). Opioid receptor-like (ORL1) receptor distribution in the rat central nervous system: comparison of ORL1 receptor mRNA expression with (125)I-[(14)Tyr]-orphanin FQ binding. J. Comp. Neurol. 412, 563–605. doi: 10.1002/(SICI)1096-9861(19991004)412:4<563::AID-CNE2>3.0.CO;2-Z
Neal, C. R. Jr., Mansour, A., Reinscheid, R., Nothacker, H. P., Civelli, O., and Watson, S. J. Jr. (1999b). Localization of orphanin FQ (nociceptin) peptide and messenger RNA in the central nervous system of the rat. J. Comp. Neurol. 406, 503–547. doi: 10.1002/(SICI)1096-9861(19990419)406:4<503::AID-CNE7>3.0.CO;2-P
Nicholson, J. R., Akil, H., and Watson, S. J. (2002). Orphanin FQ-induced hyperphagia is mediated by corticosterone and central glucocorticoid receptors. Neuroscience 115, 637–643. doi: 10.1016/S0306-4522(02)00290-7
Noda, Y., Mamiya, T., Manabe, T., Nishi, M., Takeshima, H., and Nabeshima, T. (2000). Role of nociceptin systems in learning and memory. Peptides 21, 1063–1069. doi: 10.1016/S0196-9781(00)00244-8
Nothacker, H. P., Reinscheid, R. K., Mansour, A., Henningsen, R. A., Ardati, A., Monsma, F. J. Jr., et al. (1996). Primary structure and tissue distribution of the orphanin FQ precursor. Proc. Natl. Acad. Sci. U.S.A. 93, 8677–8682. doi: 10.1073/pnas.93.16.8677
Okuda-Ashitaka, E., Minami, T., Tachibana, S., Yoshihara, Y., Nishiuchi, Y., Kimura, T., et al. (1998). Nocistatin, a peptide that blocks nociceptin action in pain transmission. Nature 392, 286–289. doi: 10.1038/32660
Olszewski, P. K., Billington, C. J., and Levine, A. S. (2000). Fos expression in feeding-related brain areas following intracerebroventricular administration of orphanin FQ in rats. Brain Res. 855, 171–175. doi: 10.1016/S0006-8993(99)02239-8
Ouagazzal, A. M., Moreau, J. L., Pauly-Evers, M., and Jenck, F. (2003). Impact of environmental housing conditions on the emotional responses of mice deficient for nociceptin/orphanin FQ peptide precursor gene. Behav. Brain Res. 144, 111–117. doi: 10.1016/S0166-4328(03)00066-4
Pampusch, M. S., Serie, J. R., Osinski, M. A., Seybold, V. S., Murtaugh, M. P., and Brown, D. R. (2000). Expression of nociceptin/OFQ receptor and prepro-nociceptin/OFQ in lymphoid tissues. Peptides 21, 1865–1870. doi: 10.1016/S0196-9781(00)00332-6
Peluso, J., LaForge, K. S., Matthes, H. W., Kreek, M. J., Kieffer, B. L., and Gaveriaux-Ruff, C. (1998). Distribution of nociceptin/orphanin FQ receptor transcript in human central nervous system and immune cells. J. Neuroimmunol. 81, 184–192. doi: 10.1016/S0165-5728(97)00178-1
Perez Nievas, B. G., Hammerschmidt, T., Kummer, M. P., Terwel, D., Leza, J. C., and Heneka, M. T. (2011). Restraint stress increases neuroinflammation independently of amyloid beta levels in amyloid precursor protein/PS1 transgenic mice. J. Neurochem. 116, 43–52. doi: 10.1111/j.1471-4159.2010.07083.x
Petrella, C., Giuli, C., Broccardo, M., Eutamene, H., Cartier, C., Leveque, M., et al. (2013). Protective and worsening peripheral nociceptin/orphanin FQ receptor-mediated effect in a rat model of experimental colitis. Pharmacol. Res. 70, 72–79. doi: 10.1016/j.phrs.2013.01.004
Polidori, C., de Caro, G., and Massi, M. (2000). The hyperphagic effect of nociceptin/orphanin FQ in rats. Peptides 21, 1051–1062. doi: 10.1016/S0196-9781(00)00243-6
Pomonis, J. D., Billington, C. J., and Levine, A. S. (1996). Orphanin FQ, agonist of orphan opioid receptor ORL1, stimulates feeding in rats. Neuroreport 8, 369–371. doi: 10.1097/00001756-199612200-00072
Prendergast, C. T., and Anderton, S. M. (2009). Immune cell entry to central nervous system – current understanding and prospective therapeutic targets. Endocr. Metab. Immune Disord. Drug Targets 9, 315–327. doi: 10.2174/187153009789839219
Ransohoff, R. M., and Perry, V. H. (2009). Microglial physiology: unique stimuli, specialized responses. Annu. Rev. Immunol. 27, 119–145. doi: 10.1146/annurev.immunol.021908.132528
Reinscheid, R. K., Ardati, A., Monsma, F. J. Jr., and Civelli, O. (1996). Structure-activity relationship studies on the novel neuropeptide orphanin FQ. J. Biol. Chem. 271, 14163–14168. doi: 10.1074/jbc.271.24.14163
Reinscheid, R. K., Nothacker, H., and Civelli, O. (2000). The orphanin FQ/nociceptin gene: structure, tissue distribution of expression and functional implications obtained from knockout mice. Peptides 21, 901–906. doi: 10.1016/S0196-9781(00)00226-6
Reinscheid, R. K., Nothacker, H. P., Bourson, A., Ardati, A., Henningsen, R. A., Bunzow, J. R., et al. (1995). Orphanin FQ: a neuropeptide that activates an opioidlike G protein-coupled receptor. Science 270, 792–794. doi: 10.1126/science.270.5237.792
Rhodes, M. E., O’Toole, S. M., Czambel, R. K., and Rubin, R. T. (2001). Male-female differences in rat hypothalamic-pituitary-adrenal axis responses to nicotine stimulation. Brain Res. Bull. 54, 681–688. doi: 10.1016/S0361-9230(01)00488-9
Rizzi, A., Bigoni, R., Marzola, G., Guerrini, R., Salvadori, S., Regoli, D., et al. (2001). Characterization of the locomotor activity-inhibiting effect of nociceptin/orphanin FQ in mice. Naunyn Schmiedebergs Arch. Pharmacol. 363, 161–165. doi: 10.1007/s002100000358
Rizzi, A., Molinari, S., Marti, M., Marzola, G., and Calo, G. (2011). Nociceptin/orphanin FQ receptor knockout rats: in vitro and in vivo studies. Neuropharmacology 60, 572–579. doi: 10.1016/j.neuropharm.2010.12.010
Roberto, M., and Siggins, G. R. (2006). Nociceptin/orphanin FQ presynaptically decreases GABAergic transmission and blocks the ethanol-induced increase of GABA release in central amygdala. Proc. Natl. Acad. Sci. U.S.A. 103, 9715–9720. doi: 10.1073/pnas.0601899103
Rodgers, R. J. (1997). Animal models of ‘anxiety’: where next? Behav. Pharmacol. 8, 477–496; discussion 497–504. doi: 10.1097/00008877-199711000-00003
Rodi, D., Zucchini, S., Simonato, M., Cifani, C., Massi, M., and Polidori, C. (2008). Functional antagonism between nociceptin/orphanin FQ (N/OFQ) and corticotropin-releasing factor (CRF) in the rat brain: evidence for involvement of the bed nucleus of the stria terminalis. Psychopharmacology (Berl.) 196, 523–531. doi: 10.1007/s00213-007-0985-7
Rossi, G. C., Perlmutter, M., Leventhal, L., Talatti, A., and Pasternak, G. W. (1998). Orphanin FQ/nociceptin analgesia in the rat. Brain Res. 792, 327–330. doi: 10.1016/S0006-8993(97)01490-X
Rossi-George, A., Urbach, D., Colas, D., Goldfarb, Y., and Kusnecov, A. W. (2005). Neuronal, endocrine, and anorexic responses to the T-cell superantigen staphylococcal enterotoxin A: dependence on tumor necrosis factor-alpha. J. Neurosci. 25, 5314–5322. doi: 10.1523/JNEUROSCI.0687-05.2005
Sakoori, K., and Murphy, N. P. (2010). Reduced degeneration of dopaminergic terminals and accentuated astrocyte activation by high dose methamphetamine administration in nociceptin receptor knock out mice. Neurosci. Lett. 469, 309–313. doi: 10.1016/j.neulet.2009.12.014
Santello, M., Cali, C., and Bezzi, P. (2012). Gliotransmission and the tripartite synapse. Adv. Exp. Med. Biol. 970, 307–331. doi: 10.1007/978-3-7091-0932-8_14
Sapolsky, R. M., McEwen, B. S., and Rainbow, T. C. (1983). Quantitative autoradiography of [3H]corticosterone receptors in rat brain. Brain Res. 271, 331–334. doi: 10.1016/0006-8993(83)90295-0
Schlicker, E., and Morari, M. (2000). Nociceptin/orphanin FQ and neurotransmitter release in the central nervous system. Peptides 21, 1023–1029. doi: 10.1016/S0196-9781(00)00233-3
Serhan, C. N., Fierro, I. M., Chiang, N., and Pouliot, M. (2001). Cutting edge: nociceptin stimulates neutrophil chemotaxis and recruitment: inhibition by aspirin-triggered-15-epi-lipoxin A4. J. Immunol. 166, 3650–3654.
Serrano-Gomez, A., Thompson, J. P., and Lambert, D. G. (2011). Nociceptin/orphanin FQ in inflammation and sepsis. Br. J. Anaesth. 106, 6–12. doi: 10.1093/bja/aeq337
Sestili, I., Borioni, A., Mustazza, C., Rodomonte, A., Turchetto, L., Sbraccia, M., et al. (2004). A new synthetic approach of N-(4-amino-2-methylquinolin-6-yl)-2-(4-ethylphenoxymethyl)benzamide (JTC-801) and its analogues and their pharmacological evaluation as nociceptin receptor (NOP) antagonists. Eur. J. Med. Chem. 39, 1047–1057. doi: 10.1016/j.ejmech.2004.09.009
Spulber, S., and Schultzberg, M. (2010). Connection between inflammatory processes and transmittor function-modulatory effects of interleukin-1. Prog. Neurobiol. 90, 256–262. doi: 10.1016/j.pneurobio.2009.10.015
Stipursky, J., Romao, L., Tortelli, V., Neto, V. M., and Gomes, F. C. (2011). Neuron-glia signaling: Implications for astrocyte differentiation and synapse formation. Life Sci. 89, 524–531. doi: 10.1016/j.lfs.2011.04.005
Stratford, T. R., Holahan, M. R., and Kelley, A. E. (1997). Injections of nociceptin into nucleus accumbens shell or ventromedial hypothalamic nucleus increase food intake. Neuroreport 8, 423–426. doi: 10.1097/00001756-199701200-00009
Sugama, S., Fujita, M., Hashimoto, M., and Conti, B. (2007). Stress induced morphological microglial activation in the rodent brain: involvement of interleukin-18. Neuroscience 146, 1388–1399. doi: 10.1016/j.neuroscience.2007.02.043
Sugama, S., Takenouchi, T., Fujita, M., Conti, B., and Hashimoto, M. (2009). Differential microglial activation between acute stress and lipopolysaccharide treatment. J. Neuroimmunol. 207, 24–31. doi: 10.1016/j.jneuroim.2008.11.007
Sugama, S., Takenouchi, T., Sekiyama, K., Kitani, H., and Hashimoto, M. (2011). Immunological responses of astroglia in the rat brain under acute stress: interleukin 1 beta co-localized in astroglia. Neuroscience 192, 429–437. doi: 10.1016/j.neuroscience.2011.06.051
Sugino, T., Shimazoe, T., Ikeda, M., and Watanabe, S. (2006). Role of nociceptin and opioid receptor like 1 on entrainment function in the rat suprachiasmatic nucleus. Neuroscience 137, 537–544. doi: 10.1016/j.neuroscience.2005.08.085
Takayama, N., and Ueda, H. (2005). Morphine-induced overexpression of prepro-nociceptin/orphanin FQ in cultured astrocytes. Peptides 26, 2513–2517. doi: 10.1016/j.peptides.2005.05.005
Trombella, S., Vergura, R., Falzarano, S., Guerrini, R., Calo, G., and Spisani, S. (2005). Nociceptin/orphanin FQ stimulates human monocyte chemotaxis via NOP receptor activation. Peptides 26, 1497–1502. doi: 10.1016/j.peptides.2005.03.015
Uchiyama, H., Yamaguchi, T., Toda, A., Hiranita, T., Watanabe, S., and Eyanagi, R. (2008). Involvement of the GABA/benzodiazepine receptor in the axiolytic-like effect of nociceptin/orphanin FQ. Eur. J. Pharmacol. 590, 185–189. doi: 10.1016/j.ejphar.2008.05.031
Uezu, K., Sano, A., Sei, H., Toida, K., Houtani, T., Sugimoto, T., et al. (2005). Enhanced hippocampal acetylcholine release in nociceptin-receptor knockout mice. Brain Res. 1050, 118–123. doi: 10.1016/j.brainres.2005.05.044
Ulrich-Lai, Y. M., and Herman, J. P. (2009). Neural regulation of endocrine and autonomic stress responses. Nat. Rev. Neurosci. 10, 397–409. doi: 10.1038/nrn2647
Varty, G. B., Hyde, L. A., Hodgson, R. A., Lu, S. X., McCool, M. F., Kazdoba, T. M., et al. (2005). Characterization of the nociceptin receptor (ORL-1) agonist, Ro64-6198, in tests of anxiety across multiple species. Psychopharmacology (Berl.) 182, 132–143. doi: 10.1007/s00213-005-0041-4
Vitale, G., Arletti, R., Ruggieri, V., Cifani, C., and Massi, M. (2006). Anxiolytic-like effects of nociceptin/orphanin FQ in the elevated plus maze and in the conditioned defensive burying test in rats. Peptides 27, 2193–2200. doi: 10.1016/j.peptides.2006.04.003
Wager-Smith, K., and Markou, A. (2011). Depression: a repair response to stress-induced neuronal microdamage that can grade into a chronic neuroinflammatory condition? Neurosci. Biobehav. Rev. 35, 742–764. doi: 10.1016/j.neubiorev.2010.09.010
Waits, P. S., Purcell, W. M., Fulford, A. J., and McLeod, J. D. (2004). Nociceptin/orphanin FQ modulates human T cell function in vitro. J. Neuroimmunol. 149, 110–120. doi: 10.1016/j.jneuroim.2003.12.018
Wang, J. B., Johnson, P. S., Imai, Y., Persico, A. M., Ozenberger, B. A., Eppler, C. M., et al. (1994). cDNA cloning of an orphan opiate receptor gene family member and its splice variant. FEBS Lett. 348, 75–79. doi: 10.1016/0014-5793(94)00557-5
Wick, M. J., Minnerath, S. R., Lin, X., Elde, R., Law, P. Y., and Loh, H. H. (1994). Isolation of a novel cDNA encoding a putative membrane receptor with high homology to the cloned mu, delta, and kappa opioid receptors. Brain Res. Mol. Brain Res. 27, 37–44. doi: 10.1016/0169-328X(94)90181-3
Wohleb, E. S., Hanke, M. L., Corona, A. W., Powell, N. D., Stiner, L. M., Bailey, M. T., et al. (2011). beta-Adrenergic receptor antagonism prevents anxiety-like behavior and microglial reactivity induced by repeated social defeat. J. Neurosci. 31, 6277–6288. doi: 10.1523/JNEUROSCI.0450-11.2011
Zaveri, N. T. (2011). The nociceptin/orphanin FQ receptor (NOP) as a target for drug abuse medications. Curr. Top. Med. Chem 11, 1151–1156. doi: 10.2174/156802611795371341
Keywords: orphanin FQ, nociceptin, HPA axis, fear, astrocytes, microglia, cytokines, immune system
Citation: Mallimo EM and Kusnecov AW (2013) The role of orphanin FQ/nociceptin in neuroplasticity: relationship to stress, anxiety and neuroinflammation. Front. Cell. Neurosci. 7:173. doi: 10.3389/fncel.2013.00173
Received: 18 May 2013; Accepted: 14 September 2013;
Published online: 08 October 2013.
Edited by:
Shawn Hayley, Carleton University, CanadaCopyright © 2013 Mallimo and Kusnecov. This is an open-access article distributed under the terms of the Creative Commons Attribution License (CC BY). The use, distribution or reproduction in other forums is permitted, provided the original author(s) or licensor are credited and that the original publication in this journal is cited, in accordance with accepted academic practice. No use, distribution or reproduction is permitted which does not comply with these terms.
*Correspondence: Alexander W. Kusnecov, Behavioral and Systems Neuroscience Program, Department of Psychology, Rutgers University, 152 Frelinghuysen Road, New Brunswick, NJ 08855, USA e-mail:a3VzbmVjb3ZAcmNpLnJ1dGdlcnMuZWR1