- 1Faculty of Rehabilitation, School of Health Sciences, Fujita Health University, Toyoake, Aichi, Japan
- 2Department of Rehabilitation Medicine I, School of Medicine, Fujita Health University, Toyoake, Aichi, Japan
- 3Department of Rehabilitation, Fujita Health University Hospital, Toyoake, Aichi, Japan
Introduction: We aimed to explore the effect of gait training using Welwalk on gait patterns by comparing differences in gait patterns between robotic-assisted gait training using Welwalk and gait training using an orthosis in individuals with hemiparetic stroke.
Methods: This study included 23 individuals with hemiparetic stroke who underwent gait training with Welwalk combined with overground gait training using an orthosis. Three-dimensional motion analysis on a treadmill was performed under two conditions for each participant: during gait training with Welwalk and with the ankle-foot orthosis. The spatiotemporal parameters and gait patterns were compared between the two conditions.
Results: The affected step length was significantly longer, the step width was significantly wider, and the affected single support phase ratio was significantly higher in the Welwalk condition than in the orthosis condition. The index values of abnormal gait patterns were significantly lower while using Welwalk than in the orthosis condition. The following four indices were lower in the Welwalk condition: contralateral vaulting, insufficient knee flexion, excessive hip external rotation during the paretic swing phase, and paretic forefoot contact.
Discussion: Gait training using Welwalk increased the affected step length, step width, and single support phase while suppressing abnormal gait patterns as compared to gait training using the ankle-foot orthosis. This study suggests that gait training using Welwalk may promote a more efficient gait pattern reacquisition that suppresses abnormal gait patterns.
Trial registration: Prospectively registered in the Japan Registry of Clinical Trials (https://jrct.niph.go.jp; jRCTs042180152).
1. Introduction
Gait disorders after stroke are commonly faced by patients. Many people with severe motor paralysis after a stroke require assistance while walking (Wade et al., 1985). Although they achieve independent walking, the risk of falling remains owing to walking instability, and decreased walking speed limits activities of daily living (Perry et al., 1995; Blennerhassett et al., 2012). Dysfunction of the paralyzed leg (Dean and Kautz, 2015) and lack of balance function (Lorenze et al., 1958; Chen et al., 2005) have been reported as causes of gait instability in patients with post-stroke hemiparesis. Spatiotemporal parameters, such as decreased stride length and shortened single support phase ratio of the affected side during walking, are altered in individuals with hemiparetic stroke compared to those with a normal gait (Perry et al., 1995; Chen et al., 2005). Furthermore, individuals with hemiparetic stroke show compensatory movements due to decreased clearance caused by insufficient hip and knee flexion and ankle dorsiflexion (Lorenze et al., 1958; Woolley, 2001; Lamontagne et al., 2002; Chen et al., 2005). The abnormal gait pattern of individuals with hemiparetic stroke is not determined solely by the recovery of motor paralysis, but rather, it is strategically learned during the gait training process (Huitema et al., 2004). Therefore, the acquired gait pattern depends on the content of the walking training. The search for the optimal method of gait training is an important issue.
Several methods of gait training have been proposed for individuals with post-stroke hemiparesis, including high-speed waking training speed and partial body weight support treadmill training with functional electrical stimulation (Pohl et al., 2002: Wang et al., 2022). One of the standard approaches to gait training in individuals with hemiparetic stroke—lower-limb orthosis—is used to improve gait patterns in patients with hemiparesis after stroke at any stage of gait training (Veerbeek et al., 2014; Johnston et al., 2021). Furthermore, its efficacy has been verified (Woolley, 2001; Tyson et al., 2013). Gait training using an ankle-foot orthosis (AFO) in individuals with hemiparetic stroke increases the gait speed, stride length, and ratio of the affected single-leg support phase (Danielsson and Sunnerhagen, 2004; Thijssen et al., 2007; Nikamp et al., 2017). Furthermore, it increases clearance during the swing phase by maintaining the dorsiflexion angle of the ankle joint and improving the hip and knee angles during the stance phase (Tyson et al., 2013; Nikamp et al., 2017). Lately, robots have been used for gait training during rehabilitation. The efficacy of robot-assisted gait training (RAGT) has been reported (Mehrholz et al., 2020), which increases gait independence and gait speed, and changes the spatiotemporal parameters of gait (Hidler et al., 2009; Westlake and Patten, 2009). Individuals with hemiparesis who received conventional gait training combined with RAGT exhibited a less compensatory gait pattern at discharge compared to conventional gait training alone in a matched control (Katoh et al., 2020).
However, most of these studies compared different individuals. Thus, the changes in gait pattern observed in RAGT compared to using an orthosis simultaneously in the same individuals with post-stroke hemiparesis at the same time are unclear. Clarifying the differences in gait characteristics influenced by training conditions of the same individual is important to assess the mechanism of efficacy of RAGT.
This prospective study explained the differences in gait patterns between two conditions: robot-assisted gait training and gait training using an orthosis in individuals with post-stroke hemiparesis. We hypothesized that RAGT elicits a spatiotemporal parameter closer to normal and a gait pattern with suppressed compensatory movement than when using an orthosis, thanks to robotic assistance.
2. Materials and methods
2.1. Study design and setting
This prospective study was approved by the Fujita Health University Ethics Review Committee (CR22-003) and all participants provided written consent before the study. This study included 23 individuals with post-stroke hemiparesis admitted to Fujita Health University Hospital who met the criteria for the use of Welwalk (Supplementary Table 1) and underwent three-dimensional gait analyses on a treadmill under two conditions (with Welwalk and with an AFO) within 1 week. Specifically, from April 2018 to March 2021, 80 individuals met the criteria for the use of Welwalk; 23 of whom were able to complete a gait analysis for this study. This study compared the gait patterns of the participants under the two conditions cross-sectionally.
2.2. Participants
The participants were given a combination of gait training using a lower-limb orthosis and RAGT with Welwalk. The physician and therapist determined the start time of RAGT, starting as early as possible after the onset of the stroke. The frequency of the RAGT was 5–6 days a week for 40 min per day. The primary target of gait training using Welwalk was to improve gait independence. Therefore, based on the idea of assist-as-needed (Srivastava et al., 2015), during the training, the parameters of assistance function of Welwalk were adjusted to minimize therapist assistance, followed by minimizing robot assistance. For example, the knee extension assist level was adjusted according to each patient's risk of falling, increased if excessive knee flexion occurred frequently during the stance phase, and decreased if sufficient knee extension was consistently obtained. In addition to RAGT, the participants received rehabilitation, including physical therapy, occupational therapy, and speech-language therapy, if needed, 6–7 days a week. In total, including RAGT, the participants underwent rehabilitation for 3 h per day. Physical therapy interventions other than RAGT were not controlled, and the therapist was in charge of each participant performing walking and standing training using orthotics.
2.3. Robotic apparatus used
Welwalk (WW-2000, WW-1000, Toyota Motor Corporation, Japan) (Figure 1) was used for the RAGT (Hirano et al., 2017). Welwalk is a walking exercise assistance system in which a knee-ankle-foot orthosis-type robot is attached to a paralyzed leg and the patient walks on a treadmill using a safety suspension. An improvement in walking independence when using Welwalk for individuals with post-stroke hemiparesis has been reported (Tomida et al., 2019; Ii et al., 2020). The robot leg can assist in supporting knee extension with ten levels of assistance during the stance phase (knee extension assist). The maximum assistance level can hold the participant's knee in the extension position preventing knee bending during normal walking (assist level 10: the target torque is approximately 80 Nm). The minimum assistance level does not assist knee extension during the stance phase (assist level 1: the target torque is approximately 2 Nm). During the swing phase, the wire pulled the leg of the robot (swing assist). The traction force can be adjusted to six levels in the vertical direction and three levels in the anteroposterior direction. The left and right positions of the swing assist can be adjusted in four steps, allowing adjustment of adduction/abduction and internal/external rotation of the hip joint during the affected swing phase. The vertical assistance force is the same at level 2 as the weight of the robot's leg, and changes by 0.5 kg for every 1-level change. In addition, the robotic knee joint flexed and extended during the swing phase to easily swing the paralyzed leg. The flexion angle, time, and timing of the beginning of knee flexion were also adjusted. The ankle joint of the robotic leg can be set to a limited angle of plantar dorsiflexion like an orthosis. As a visual feedback item for participants, the front monitor can be switched between a mirror-like image, an image from the sagittal plane, and an image showing only the feet to improve gait posture and pattern. The treadmill could be adjusted from 0.2–3.0 km/h. During training, the therapist stood behind the participants and adjusted the settings according to their specific gait pattern (Hirano et al., 2017).
2.4. Gait analysis
The primary outcome was the change in spatio-temporal parameters during walking on the treadmill. The secondary outcomes were the index values of 12 abnormal gait patterns that typically appear in individuals with post-stroke hemiparesis (Table 1) (Itoh et al., 2012; Tanikawa et al., 2016, 2021; Hishikawa et al., 2018). Analyses were performed when participants were able to walk without the help of a therapist during the gait training period in both training conditions: with Welwalk and with an AFO. The order of gait analysis was not specific. To avoid the influence of fatigue, gait analyses were not conducted consecutively for both conditions. The types of orthoses used were determined by the physician and the therapist in charge.
A three-dimensional motion analysis system (Kinema Tracer, KISSEICOMTEC, Matsumoto, Japan) was used for the measurements. In the three-dimensional motion analysis, color markers were attached bilaterally to the shoulder (acromion), hip joint (at one-third of the distance from the great trochanter on a line connecting the anterior superior iliac spine and the great trochanter), knee joint (at the mid-point of the anteroposterior diameter of the lateral femoral epicondyle), ankle joint (at the lateral malleolus), and toes (at the heads of the fifth metatarsal) at 12 locations in the orthosis condition. In the Welwalk condition, two of the marker positions in the orthosis condition, the knee joint and ankle joint centers, were affixed to the robot leg. The distance to the actual knee joint and ankle joint position from the marker was measured and corrected to calculate and analyze the same joint positions as in the orthosis condition.
Each measurement was performed for 20 seconds at a sampling frequency of 60 Hz. In the orthosis condition, measurements were performed on the treadmill at a comfortable walking velocity for level walking or at 70% of this comfortable walking velocity if the patient had difficulty walking at that velocity. Measurements in the Welwalk condition were performed at a comfortable walking velocity on Welwalk and optimal assistance settings. In both conditions, all participants walked while viewing their walking posture on a monitor in front of them, and they used a handrail during the measurement. Additionally, the values of the robot's swing assist and knee extension assist were recorded in the Welwalk condition.
2.5. Statistical analysis
First, spatiotemporal parameters, including walking velocity, cadence, stride length, step length, and step width, were compared between the Welwalk and orthosis conditions. Second, the crude time and percentage of each phase of the gait cycle were compared between the two conditions. Each gait cycle was divided into four phases: affected initial double support, affected single support, unaffected initial double support, and affected swing. Finally, the 12 indices of abnormal gait patterns between the two conditions were compared.
Comparisons were performed using a paired t-test or Wilcoxon signed-rank test, according to the type of variable. Statistical analyses were performed using SPSS version 21 software (IBM Corp., Armonk, NY, USA). P-values < 0.05 were considered statistically significant.
3. Results
Participant demographic data are presented in Table 2. The median Stroke Impairment Assessment Set lower extremity motor function total score (hip-flexion test, knee-extension test, foot-pat test) as the degree of paralysis was 5 (4–6), indicating moderate motor paralysis (Chino et al., 1994). The robot assists in the Welwalk condition ranged from 1 to 10 (median, 6) for the swing assist and from 1 to 6 (median, 3) for the knee extension assist. In the orthosis condition, all participants used the Remodeled Adjustable Posterior Strut (RAPS)-AFO (Mizuno et al., 2005).
A comparison of the spatiotemporal parameters between the two conditions is presented in Table 3. Walking velocity and cadence showed no significant difference between the two conditions (P = 0.485 and P = 0.130, respectively). Stride length and affected step length were significantly longer in the Welwalk condition than in the orthosis condition (P < 0.00 and P = 0.001, respectively). The width of the steps was significantly wider in the Welwalk condition than in the orthosis condition (P < 0.001).
Comparisons of gait cycles are presented in Table 4. The percentage of affected single supports was significantly higher in the Welwalk condition than in the orthosis condition (P = 0.016). There were no significant differences in other parameters (Table 4).
Of the 12 index characteristics of hemiparetic stroke calculated from the three-dimensional motion analysis, the values of forefoot contact, contralateral vaulting, insufficient knee flexion during the swing phase, and excessive hip external rotation were lower in the Welwalk condition than in the orthosis condition (P < 0.001, P = 0.001, P < 0.001, and P = 0.011, respectively). There were no significant differences in the other eight indices between the two conditions (Figure 2).
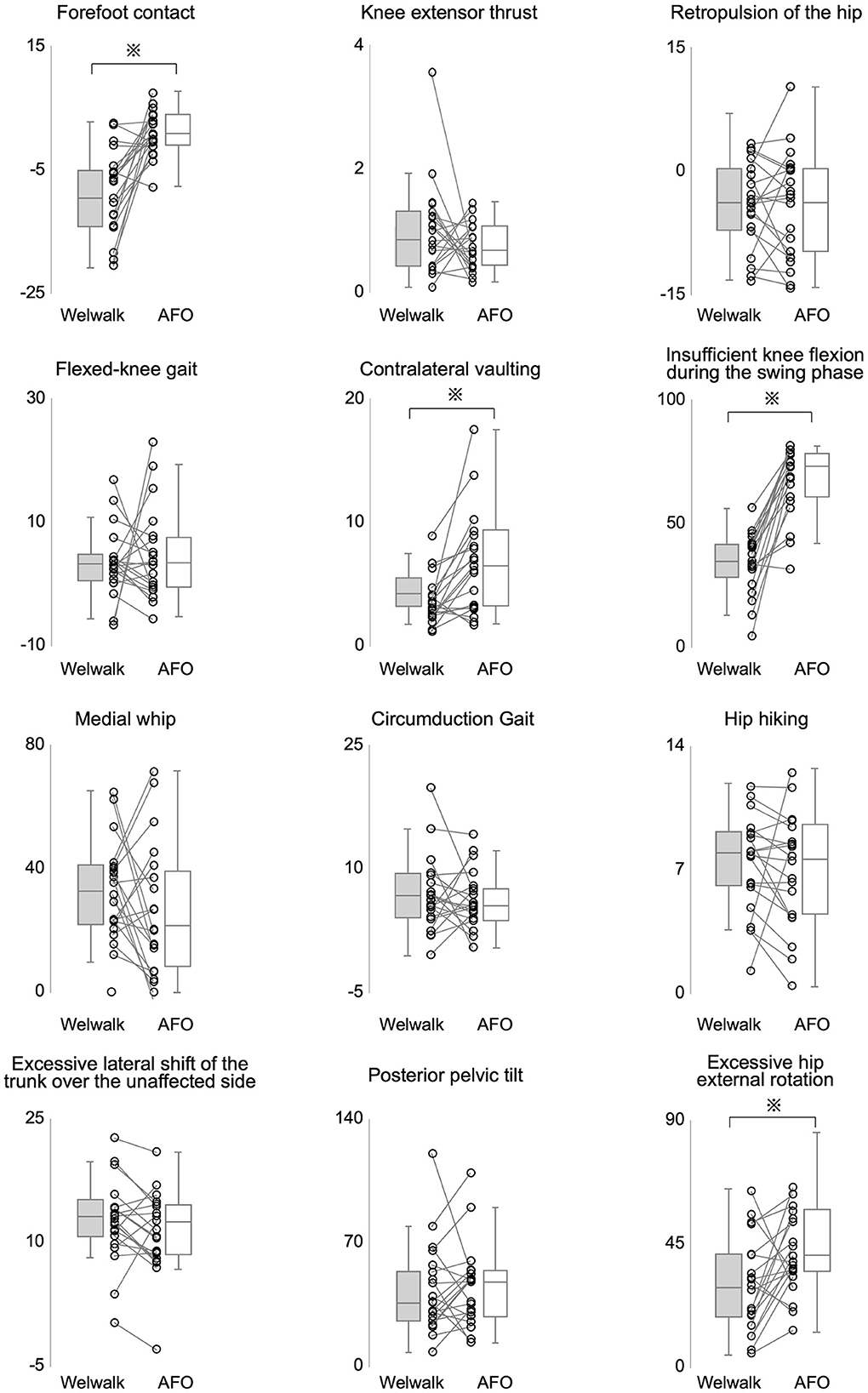
Figure 2. Comparison of the severities of abnormal gait patterns. A comparison of index values representing the severity of abnormal gait patterns between the Welwalk and the orthosis conditions is shown. The vertical axis represents the index values. All index values were compared using the Wilcoxon-signed rank test. *P-values < 0.05 were considered statistically significant.
4. Discussion
This study investigated the effects of Welwalk use on gait patterns in individuals with post-stroke hemiparesis by comparing the gait patterns between two conditions: robotic-assisted gait training using Welwalk and using an orthosis. The present results showed that gait training using Welwalk can increase the affected step length, step width, and single support phase time by suppressing abnormal gait patterns during gait compared to gait training using an orthosis.
Among the basic spatiotemporal parameters of walking, stride length and affected step length were significantly longer while using the Welwalk than the orthosis. The swing assist provided by Welwalk might have compensated for the dysfunction of the affected leg swing when the affected side had low swing ability due to paresis. The swing assist and knee flexion during swing were adjusted to ensure clearance and reduce the risk of falls; however, this might have also indirectly influenced stride extension.
Regarding step width, a previous study reported that the step width of Japanese healthy adults was 23.3 cm (Mukaino et al., 2016). The individuals with post-stroke hemiparesis who were able to walk independently displayed wider step widths compared to healthy individuals, reported to be 28.2 cm (Mukaino et al., 2016) and 29.8 cm (Abe et al., 2009). The participants in our study showed a narrower step width in both conditions (Welwalk and orthosis) than those previously reported in healthy individuals (Mukaino et al., 2016). This finding may be attributed to the different conditions between the present study and previous studies (Abe et al., 2009; Mukaino et al., 2016) regarding the time after stroke onset, walking speed, and use of a handrail. In a longitudinal study of gait parameters in hemiparetic stroke, no change in step width was observed after stroke onset (Chow and Stokic, 2021). It was also reported that changing walking speed did not change the step width. Therefore, we consider that the effect of handrail use contributed to this result (Kao et al., 2014). All participants in our study used a handrail, while participants in previous studies did not. Handrails have been reported to reduce step width by approximately 25% (IJmker et al., 2015). Welwalk showed a wider step width when compared to the orthosis. This difference is thought to be due to the adjustability of the step width with Welwalk. The adjustment functions of Welwalk enable the therapist to adjust the left-right position of the paralyzed side swing by changing the position of swing assist and to prompt the patient to position the paralyzed foot contact on the monitor for visual feedback (Hirano et al., 2017). Individuals with stroke are considered to widen their step width to increase walking stability (Hak et al., 2013), and training with a wider step width leads to increased walking stability (McAndrew Young and Dingwell, 2012). This may contribute to the faster independence of walking achieved in RAGT with Welwalk than in walking training with an orthosis (Ii et al., 2020).
Regarding the temporal parameters of the gait cycle, the percentage of affected single support phase was significantly larger in the Welwalk condition than in the orthosis condition. This suggests that the function of the knee extension assist of the Welwalk is useful for individuals with post-stroke hemiparesis when the use of an AFO is insufficient to provide stability in the stance phase. Individuals with post-stroke hemiparesis had been reported to tend to shorten the affected single support phase ratio due to decreased stance support and balance on the paralyzed support (Goldie et al., 2001). Furthermore, the single-support phase ratio of the affected side increased as gait training progressed and was associated with increased walking speed in individuals with post-stroke hemiparesis (De Quervain et al., 1996; Goldie et al., 2001). In RAGT with Welwalk, the participants could walk at a gait cycle similar to that of the later stage when training had progressed, and their gait ability had improved.
Regarding gait patterns, four indices (insufficient knee flexion, forefoot contact, excessive hip external rotation, and contralateral vaulting during the paretic swing phase) were significantly lower in the Welwalk condition than in the orthosis condition. Because this index value represents the degree of abnormal gait pattern, the lower the value, the closer it is to a normal gait pattern. Thus, the Welwalk condition elicits a gait pattern closer to normal, suggesting that the patient can walk with a suppressed abnormal gait pattern. Insufficient knee flexion during the swing phase is caused by decreased hip flexor output and spasticity of the rectus femoris muscle (Perry et al., 2010) and is considered a factor leading to decreased toe clearance (Gage et al., 1987). In the Welwalk condition, the direct knee joint flexion motion of the robot during the swing phase could maintain clearance and reduce abnormal gait patterns. Forefoot contact is caused by insufficient knee extension before initial contact, reduced ankle dorsiflexor output, and spasticity of the plantar flexor muscle (Perry et al., 2010). Therefore, it is believed that the lack of knee extension prior to initial contact was compensated for by knee extension in the terminal swing by Welwalk, leading to a reduction in forefoot contact. Excessive hip external rotation is determined by the direction of the foot during the swing leg (Tanikawa et al., 2016), and is thought to be caused by reduced hip flexor output (Lorenze et al., 1958). In the Welwalk condition, the swing assists and knee flexion exerted by the robotic assist allowed for an easier paretic leg swinging, which indirectly reduced external rotation of the hip during the swing phase. Contralateral vaulting during the paretic swing phase is a compensatory movement for decreased paretic toe clearance owing to the output of the affected hip flexor (Woolley, 2001; Cruz and Dhaher, 2009; Matsuda et al., 2017). In the Welwalk condition, this compensatory movement might have been indirectly decreased by the robot's swing assist and knee flexion movements to maintain toe clearance.
The present study showed that RAGT using Welwalk increased the step length of the affected side and step width, and affected the single support phase while suppressing abnormal gait patterns during gait compared to gait training using the AFO. A longer step length is associated with greater joint moments of hip extension than a narrow step length and greater output of the gluteus maximus, gluteus medius, and other muscles involved in stance stability (Lim et al., 2017). Increasing the affected single support phase may also enhance the function of the proximal lower extremity (including the muscle around the hip), which contributes to the support of the stance phase (Gottschalk et al., 1989). RAGT using Welwalk may be more effective than gait training using AFO because it promotes increased muscle output around the hip joint, as it increases stride length and single support phase time during walking. The gait pattern during the training process is considered to lead to definitively acquired gait pattern. Changes in gait pattern specific to patients with hemiparesis can lead to gait instability (Dean and Kautz, 2015). Repeated gait training with suppressing abnormal gait patterns specific to hemiparesis is meaningful because it may eventually lead to improved gait stability and energy efficiency (Platts et al., 2006; Polese et al., 2018). RAGT combined with conventional rehabilitation in individuals with hemiparesis has previously been shown to improve independence while walking (Mehrholz et al., 2020; Moucheboeuf et al., 2020). Our study suggests that gait training with Welwalk may lead to a more efficient gait pattern with suppression of abnormal patterns in addition to an increased proportion of individuals with post-stroke hemiparesis who achieve gait independence.
The limitations of this study were that it was conducted at a single institution and only cross-sectionally. The training doses in the orthosis and Welwalk conditions before the measurement were not investigated. The multiple mechanisms of robotic assistance are associated with changes in various gait parameters, but their correspondence remains unclear. Furthermore, a longitudinal study with dose monitoring of each condition will elucidate the relationship between the type of training and the definitively acquired gait pattern.
5. Conclusion
We investigated the effect of RAGT using Welwalk on gait patterns by comparing gait patterns using Welwalk and AFO in individuals with post-stroke hemiparesis who received training for the use of Welwalk and an orthosis device. Compared to gait training using the AFO, RAGT using Welwalk can increase step length, step width, and single support phase time in the affected side while suppressing abnormal gait patterns.
Data availability statement
The raw data supporting the conclusions of this article will be made available by the authors, without undue reservation.
Ethics statement
The studies involving human participants were reviewed and approved by Fujita Health University Ethics Review Committee. The patients/participants provided their written informed consent to participate in this study.
Author contributions
TI, SH, and YO designed this study, interpreted the data, and reviewed the manuscript. TI and DI collected and analyzed the data. TI and SH wrote the manuscript. All authors have read and approved the final manuscript.
Funding
This study was partially supported by the Toyota Motor Corporation through the loan of a Welwalk WW-2000 device and the Japan Society for the Promotion of Science (JSPS) KAKENHI, Grant No. 16K01476. The funder was not involved in the study design, data collection, analysis and interpretation, the writing of this article, or the decision to submit it for publication.
Conflict of interest
The authors declare that the research was conducted in the absence of any commercial or financial relationships that could be construed as a potential conflict of interest.
Publisher's note
All claims expressed in this article are solely those of the authors and do not necessarily represent those of their affiliated organizations, or those of the publisher, the editors and the reviewers. Any product that may be evaluated in this article, or claim that may be made by its manufacturer, is not guaranteed or endorsed by the publisher.
Supplementary material
The Supplementary Material for this article can be found online at: https://www.frontiersin.org/articles/10.3389/fnbot.2023.1151623/full#supplementary-material
Abbreviations
AFO, Ankle foot orthosis; RAGT, Robot-assisted gait training; FIM, Functional Independence Measure [Data Management Service of the Uniform Data System for Medical Rehabilitation and the Center for Functional Assessment Research: Guide for use of the uniform data set for medical rehabilitation (ver. 3.0), 1990]; SIAS, Stroke Impairment Assessment Set.
References
Abe, H., Michimata, A., Sugawara, K., Sugaya, N., and Izumi, S. (2009). Improving gait stability in stroke hemiplegic patients with a plastic ankle-foot orthosis. Tohoku. J. Exp. Med. 218, 193–199. doi: 10.1620/tjem.218.193
Blennerhassett, J. M., Dite, W., Ramage, E. R., and Richmond, M. E. (2012). Changes in balance and walking from stroke rehabilitation to the community: a follow-up observational study. Arch. Phys. Med. Rehabil. 93, 1782–1787. doi: 10.1016/j.apmr.2012.04.005
Chen, G., Patten, C., Kothari, D. H., and Zajac, F. E. (2005). Gait differences between individuals with post-stroke hemiparesis and non-disabled controls at matched speeds. Gait. Posture. 22, 51–56. doi: 10.1016/j.gaitpost.2004.06.009
Chino, N., Sonoda, S., Domen, K., Saitoh, E., and Kimura, A. (1994). Stroke Impairment Assessment Set (SIAS) A new evaluation instrument for stroke patients. J. Rehabil. Med. 31, 119–125. doi: 10.2490/jjrm1963.31.119
Chow, J. W., and Stokic, D. S. (2021). Longitudinal changes in temporospatial gait characteristics during the first year post-stroke. Brain. Sci. 11, 1648. doi: 10.3390/brainsci11121648
Cruz, T. H., and Dhaher, Y. Y. (2009). Impact of ankle-foot-orthosis on frontal plane behaviors post-stroke. Gait. Posture. 30, 312–316. doi: 10.1016/j.gaitpost.2009.05.018
Danielsson, A., and Sunnerhagen, K. S. (2004). Energy expenditure in stroke subjects walking with a carbon composite ankle foot orthosis. J. Rehabil. Med. 36, 165–168. doi: 10.1080/16501970410025126
De Quervain, I. A., Simon, S. R., Leurgans, S., Pease, W. S., and McAllister, D. (1996). Gait pattern in the early recovery period after stroke. J. Bone Joint Surg. Am. 78, 1506–1514. doi: 10.2106/00004623-199610000-00008
Dean, J. C., and Kautz, S. A. (2015). Foot placement control and gait instability among people with stroke. J. Rehabil. Res. Dev. 52, 577–590. doi: 10.1682/JRRD.2014.09.0207
Gage, J. R., Perry, J., Hicks, R. R., Koop, S., and Werntz, J. R. (1987). Rectus femoris transfer to improve knee function of children with cerebral palsy. Dev. Med. Child Neurol. 29, 159–166. doi: 10.1111/j.1469-8749.1987.tb02131.x
Goldie, P. A., Matyas, T. A., and Evans, O. M. (2001). Gait after stroke: initial deficit and changes in temporal patterns for each gait phase. Arch. Phys. Med. Rehabil. 82, 1057–1065. doi: 10.1053/apmr.2001.25085
Gottschalk, F., Kourosh, F., and Leveau, B. (1989). The functional anatomy of tensor fasciae latae and gluteus medius and minimus. J. Anat. 166: 179–189.
Hak, L., Houdijk, H., Werff, P., Prins, M., Mert, A., Beek, P. J., et al. (2013). Stepping strategies used by post-stroke individuals to maintain margins of stability during walking. Clin. Biomech. 28, 1041–1048. doi: 10.1016/j.clinbiomech.2013.10.010
Hidler, J., Nichols, D., Pelliccio, M., Brady, K., Campbell, D. D., Kahn, J. H., et al. (2009). Multicenter randomized clinical trial evaluating the effectiveness of the Lokomat in subacute stroke. Neurorehabil. Neural. Repair. 23, 5–13. doi: 10.1177/1545968308326632
Hirano, S., Saitoh, E., Tanabe, S., Tanikawa, H., Sasaki, S., Kato, D., et al. (2017). The features of Gait Exercise Assist Robot: Precise assist control and enriched feedback. NeuroRehabilitation. 41, 77–84. doi: 10.3233/NRE-171459
Hishikawa, N., Tanikawa, H., Ohtsuka, K., Mukaino, M., Inagaki, K., Matsuda, F., et al. (2018). Quantitative assessment of knee extensor thrust, flexed-knee gait, insufficient knee flexion during the swing phase, and medial whip in hemiplegia using three-dimensional treadmill gait analysis. Top. Stroke. Rehabil. 25, 548–553. doi: 10.1080/10749357.2018.1497272
Huitema, R. B., Hof, A. L., Mulder, T., Brouwer, W. H., Dekker, R., and Postema, K. (2004). Functional recovery of gait and joint kinematics after right hemispheric stroke. Arch. Phys. Med. Rehabil. 85, 1982–1988. doi: 10.1016/j.apmr.2004.04.036
Ii, T., Hirano, S., Tanabe, S., Saitoh, E., Yamada, J., Mukaino, M., et al. (2020). Robot-assisted gait training using welwalk in hemiparetic stroke patients: an effectiveness study with matched control. J. Stroke Cerebrovasc. Dis. 29, 105377. doi: 10.1016/j.jstrokecerebrovasdis.2020.105377
IJmker, T., Lamoth, C. J., Houdijk, H., Tolsma, M., van der Woude, L. H., Daffertshofer, A., et al. (2015). Effects of handrail hold and light touch on energetics, step parameters, and neuromuscular activity during walking after stroke. J. Neuroeng. Rehabil. 12, 70. doi: 10.1186/s12984-015-0051-3
Itoh, N., Kagaya, H., Saitoh, E., Ohtsuka, K., Yamada, J., Tanikawa, H., et al. (2012). Quantitative assessment of circumduction, hip hiking, and forefoot contact gait using Lissajous figures. Jpn. J. Nurs. Sci. 3, 78–84. doi: 10.11336/jjcrs.3.78
Johnston, T., Keller, S., Denzer-Weiler, C., and Brown, L. (2021). A clinical practice guideline for the use of ankle-foot orthoses and functional electrical stimulation post-stroke. J. Neural Phys. Ther. 45:112–196. doi: 10.1097/NPT.0000000000000347
Kao, P. C., Dingwell, J. B., Higginson, J. S., and Binder-Macleod, S. (2014). Dynamic instability during post-stroke hemiparetic walking. Gait. Posture. 40, 457–463. doi: 10.1016/j.gaitpost.2014.05.014
Katoh, D., Tanikawa, H., Hirano, S., Mukaino, M., Yamada, J., Sasaki, S., et al. (2020). The effect of using Gait Exercise Assist Robot (GEAR) on gait pattern in stroke patients: a cross-sectional pilot study. Top Stroke Rehabil. 27, 103–109. doi: 10.1080/10749357.2019.1660080
Lamontagne, A., Malouin, F., Richards, C. L., and Dumas, F. (2002). Mechanisms of disturbed motor control in ankle weakness during gait after stroke. Gait. Posture. 15, 244–255. doi: 10.1016/S0966-6362(01)00190-4
Lim, Y. P., Lin, Y. C., and Pandy, M. G. (2017). Effects of step length and step frequency on lower-limb muscle function in human Gait. J. Biomech. 57, 1–7. doi: 10.1016/j.jbiomech.2017.03.004
Lorenze, E. J., Derosa, A. J., and Keenan, E. L. (1958). Ambulation problems in hemiplegia. Arch. Phys. Med. Rehabil. 39, 366–370.
Matsuda, F., Mukaino, M., Ohtsuka, K., Tanikawa, H., Tsuchiyama, K., Teranishi, T., et al. (2017). Biomechanical factors behind toe clearance during the swing phase in hemiparetic patients. Top. Stroke. Rehabil. 24, 177–182. doi: 10.1080/10749357.2016.1234192
McAndrew Young, P. M., and Dingwell, J. B. (2012). Voluntary changes in step width and step length during human walking affect dynamic margins of stability. Gait. Posture. 36, 214–219. doi: 10.1016/j.gaitpost.2012.02.020
Mehrholz, J., Thomas, S., Kugler, J., Pohl, M., and Elsner, B. (2020). Electromechanical-assisted training for walking after stroke. Cochrane. Database. Syst. Rev. 10, CD006185. doi: 10.1002/14651858.CD006185.pub5
Mizuno, M., Saitoh, E., Iwata, E., Okada, M., Teranishi, T., Itoh, M., et al. (2005). The development of a new posterior strut AFO with an adjustable joint: its concept and a consideration of basic function. Bull. Jap. Soc. Prosthet. Orthot. 21, 225–233. doi: 10.11267/jspo1985.21.225
Moucheboeuf, G., Griffier, R., Gasq, D., Glize, B., Bouyer, L., Dehail, P., et al. (2020). Effects of robotic gait training after stroke: A meta-analysis. Ann. Phys. Rehabil. Med. 63, 518–534. doi: 10.1016/j.rehab.2020.02.008
Mukaino, M., Ohtsuka, K., Tsuchiyama, K., Matsuda, F., Inagaki, K., Yamada, J., et al. (2016). Feasibility of a simplified, clinically oriented, three-dimensional gait analysis system for the gait evaluation of stroke patients. Prog. Rehabil. Med. 1, 20160001. doi: 10.2490/prm.20160001
Nikamp, C. D. M., Hobbelink, M. S. H., van der Palen, J., Hermens, H. J., Rietman, J. S., and Buurke, J. H. (2017). A randomized controlled trial on providing ankle-foot orthoses in patients with (sub-)acute stroke: Short-term kinematic and spatiotemporal effects and effects of timing. Gait. Posture. 55, 15–22. doi: 10.1016/j.gaitpost.2017.03.028
Perry, J., Burnfield, J. M., Cabico, L. M., and Ebscohost. (2010). Gait Analysis: Normal and Pathological Function (Version Second edition). Thorofare, NJ: SLACK.
Perry, J., Garrett, M., Gronley, J. K., and Mulroy, S. J. (1995). Classification of walking handicap in the stroke population. Stroke. 26, 982–989. doi: 10.1161/01.STR.26.6.982
Platts, M. M., Rafferty, D., and Paul, L. (2006). Metabolic cost of overground gait in younger stroke patients and healthy controls. Med. Sci. Sports. Exerc. 38, 1041–1046. doi: 10.1249/01.mss.0000222829.34111.9c
Pohl, M., Mehrholz, J., Ritschel, C., and Ruckriem, S. (2002). Speed-dependent treadmill training in ambulatory hemiparetic stroke patients: a randomized controlled trial. Stroke. 33, 553–558. doi: 10.1161/hs0202.102365
Polese, J. C., Ada, L., and Teixeira-Salmela, L. F. (2018). Relationship between oxygen cost of walking and level of walking disability after stroke: an experimental study. Physiother. Res. Int. 23, 1688. doi: 10.1002/pri.1688
Srivastava, S., Kao, P. C., Kim, S. H., Stegall, P., Zanotto, D., Higginson, J. S., et al. (2015). Assist-as-needed robot-aided gait training improves walking function in individuals following stroke. IEEE. Trans. Neural. Syst. Rehabil. Eng. 23, 956–963. doi: 10.1109/TNSRE.2014.2360822
Tanikawa, H., Inagaki, K., Ohtsuka, k., Matsuda, F., Mukaino, M., Yamada, J., et al. (2021). Validity of quantitative assessment of posterior pelvic tilt and contralateral vaulting in hemiplegia using 3D treadmill gait analysis. Top. Stroke. Rehabil. 28, 96–103. doi: 10.1080/10749357.2020.1783919
Tanikawa, H., Ohtsuka, K., Mukaino, M., Inagaki, K., Matsuda, F., Teranishi, T., et al. (2016). Quantitative assessment of retropulsion of the hip, excessive hip external rotation, and excessive lateral shift of the trunk over the unaffected side in hemiplegia using three-dimensional treadmill gait analysis. Top. Stroke. Rehabil. 23, 311–317. doi: 10.1080/10749357.2016.1156361
Thijssen, D. H., Paulus, R., van Uden, C. J., Kooloos, J. G., and Hopman, M. T. (2007). Decreased energy cost and improved gait pattern using a new orthosis in persons with long-term stroke. Arch. Phys. Med. Rehabil. 88, 181–186. doi: 10.1016/j.apmr.2006.11.014
Tomida, K., Sonoda, S., Hirano, S., Suzuki, A., Tanino, G., Kawakami, K., et al. (2019). Randomized controlled trial of gait training using gait exercise assist robot (GEAR) in stroke patients with hemiplegia. J. Stroke. Cerebrovasc. Dis. 28, 2421–2428. doi: 10.1016/j.jstrokecerebrovasdis.2019.06.030
Tyson, S. F., Sadeghi-Demneh, E., and Nester, C. J. (2013). A systematic review and meta-analysis of the effect of an ankle-foot orthosis on gait biomechanics after stroke. Clin. Rehabil. 27, 879–891. doi: 10.1177/0269215513486497
Veerbeek, J. M., Wegen, E., Peppen, R., Wees, P., Hendriks, E., Rietberg, M., et al. (2014). What is the evidence for physical therapy poststroke? A systematic review and meta-analysis. PLoS ONE. 9, e87987. doi: 10.1371/journal.pone.0087987
Wade, D. T., Wood, V. A., and Hewer, R. L. (1985). Recovery after stroke—the first 3 months. J. Neurol. Neurosurg. Psychiatry. 48, 7–13. doi: 10.1136/jnnp.48.1.7
Wang, J., Zhao, L., Gao, Y., Liu, C., Dong, X., and He, X. (2022). The difference between the effectiveness of body-weight-supported treadmill training combined with functional electrical stimulation and sole body-weight-supported treadmill training for improving gait parameters in stroke patients: A systematic review and meta-analysis. Front. Neural. 9, 1003723. doi: 10.3389/fneur.2022.1003723
Westlake, K. P., and Patten, C. (2009). Pilot study of Lokomat versus manual-assisted treadmill training for locomotor recovery post-stroke. J. Neuroeng. Rehabil. 6, 18. doi: 10.1186/1743-0003-6-18
Keywords: gait training, hemiparesis, rehabilitation, RAGT, robot, stroke
Citation: Ii T, Hirano S, Imoto D and Otaka Y (2023) Effect of gait training using Welwalk on gait pattern in individuals with hemiparetic stroke: a cross-sectional study. Front. Neurorobot. 17:1151623. doi: 10.3389/fnbot.2023.1151623
Received: 26 January 2023; Accepted: 29 March 2023;
Published: 17 April 2023.
Edited by:
Xianta Jiang, Memorial University of Newfoundland, CanadaReviewed by:
Takahiro Kagawa, Aichi Institute of Technology, JapanJiang Li Zhao, Sun Yat-sen University, China
Copyright © 2023 Ii, Hirano, Imoto and Otaka. This is an open-access article distributed under the terms of the Creative Commons Attribution License (CC BY). The use, distribution or reproduction in other forums is permitted, provided the original author(s) and the copyright owner(s) are credited and that the original publication in this journal is cited, in accordance with accepted academic practice. No use, distribution or reproduction is permitted which does not comply with these terms.
*Correspondence: Satoshi Hirano, c3NoaXJhbm8mI3gwMDA0MDtmdWppdGEtaHUuYWMuanA=