- 1Center for Mobility and Rehabilitation Engineering Research, Kessler Foundation, West Orange, NJ, United States
- 2Department of Physical Medicine and Rehabilitation, Rutgers—New Jersey Medical School, Newark, NJ, United States
- 3Research Staff Children's Specialized Hospital New Brunswick, New Brunswick, NJ, United States
- 4Department of Psychology, Montclair State University, Montclair, NJ, United States
Acquired brain injury (ABI) is a leading cause of ambulation deficits in the United States every year. ABI (stroke, traumatic brain injury and cerebral palsy) results in ambulation deficits with residual gait and balance deviations persisting even after 1 year. Current research is focused on evaluating the effect of robotic exoskeleton devices (RD) for overground gait and balance training. In order to understand the device effectiveness on neuroplasticity, it is important to understand RD effectiveness in the context of both downstream (functional, biomechanical and physiological) and upstream (cortical) metrics. The review identifies gaps in research areas and suggests recommendations for future research. We carefully delineate between the preliminary studies and randomized clinical trials in the interpretation of existing evidence. We present a comprehensive review of the clinical and pre-clinical research that evaluated therapeutic effects of RDs using various domains, diagnosis and stage of recovery.
1. Introduction
Acquired brain injury (ABI) is a leading cause of ambulation deficits affecting people in the United States every year (Centers for Disease Control and Prevention, 2016; Menon and Bryant, 2019; CDC Stroke Statistics, 2020; Alliance BI, 2023). An ABI is an injury to the brain that is not hereditary, or degenerative (Menon and Bryant, 2019; BIA, 2021). The injury results in a change to the brain's neuronal activity, which may affect the physical integrity, metabolic activity, or functional ability of nerve cells in the brain, and in turn affects function (BIA, 2021). For the purpose of this review ABI refers to a diagnosis of stroke, traumatic brain injury, and cerebral palsy.
Sixty-five percent of individuals diagnosed with ABI (stroke, traumatic brain injury, and cerebral palsy) have mobility deficits; despite rehabilitation, over half of them present with functional ambulation deficits even after 1 year, limiting their community ambulation, independence, and activities of daily living (ADL) (Wade and Hewer, 1987; Friedman, 1990). Regaining ambulation is a priority in adults and children with ABI to improve their participation and quality of life (QOL) (Rudberg et al., 2021).
Post-ABI gait and balance rehabilitation is based on the theory that consistent, repeated task-specific practice will lead to recovery of function (Partridge et al., 2000; Cooke et al., 2010). Some of the critical parameters for improving mobility post-ABI are task-specific, repetitive practices that are progressively more challenging (Langhorne et al., 2009). Wearable robotic devices for over-ground walking offer an alternative modality for rehabilitation, because they can facilitate task-specific, repetitive practice that is progressively more challenging for individuals with acute and chronic ABI.
The past decade has witnessed a dramatic growth in the study and application of wearable robotic devices (RDs) for over-ground gait training in individuals with ABI (Canela et al., 2013; Murray et al., 2015; Federici et al., 2016; Louie and Eng, 2016; Kozlowski et al., 2017; Lefeber et al., 2017; Lerner et al., 2017a; Patané et al., 2017; Molteni et al., 2018; Androwis et al., 2019; Karunakaran et al., 2019; Moucheboeuf et al., 2020). RDs can provide trajectory guidance and assistance at various joints individually (hip, knee, ankle) or in combination (multi-joint), to assist, resist, or augment muscle torque (Dollar and Herr, 2008; Yan et al., 2015; Esquenazi et al., 2017; Iandolo et al., 2019). Some RDs also provide rigid support for stability and static balance (Ekso, ReWalk, HAL, etc.) to keep the users in an upright posture (Dollar and Herr, 2008; Yan et al., 2015; Esquenazi et al., 2017; Iandolo et al., 2019). These exoskeletons have a rigid structure at the joints and/or links. They may provide hip or/and back support to keep the users in the upright position. Though these robots do not provide dynamic balance control. This upright posture is very important during gait training to provide quality repetitions especially in people who require maximum assistance from therapists. Therapy requirements differ based on time since injury and deficits (Kwakkel et al., 2004; Neural Plasticity After Acquired Brain Injury, 2022). It is well established that recovery plateaus with time, which is why repetitive practice should start as early as possible to change the trajectory of recovery (Kwakkel et al., 2004; Neural Plasticity After Acquired Brain Injury, 2022). RDs are capable of early mobilization, providing consistent repetitive physical therapy by assisting users with severe gait and balance deficits early after ABI. With time (i.e., in chronic stages of ABI), many patients develop compensatory mechanisms such as circumduction, steppage gait, hip hiking, toe walking, to successfully ambulate (Kerrigan et al., 2000; Williams et al., 2009; Winter, 2009; Kemu, 2010; Perry and Burnfield, 2010; Dubin, 2014; Sheffler and Chae, 2015). These pathological deviations from healthy walking result in slower walking speed, shorter step length, decreased symmetry, reduced gait and balance adaptability, and increased risk of falls (Kerrigan et al., 2000; Williams et al., 2009; Winter, 2009; Kemu, 2010; Perry and Burnfield, 2010; Dubin, 2014; Sheffler and Chae, 2015). One of the goals of therapy is to reduce these compensatory mechanisms and train individuals to perform healthy and efficient overground ambulation. RDs are functionally capable of providing this training and can be useful in both the acute and chronic stages of recovery (Calabrò et al., 2018; Molteni et al., 2018, 2021; Nolan et al., 2020; Rojek et al., 2020).
Despite rapid progress in robotic exoskeleton design and technology, limited data is available on the evaluation of RD efficacy with regard to children and adults diagnosed with ABI. In order to fully understand the effects of RDs, it is imperative to answer questions related to their utilization, such as: (a) How does early RD therapy change the recovery curve?; (b) Who should use multi-joint RD vs. single joint RD?; (c) Does a person with low deficits benefit from multi-joint or single joint RD therapy during the acute stages of recovery?; (d) Would it be beneficial to use a single joint robot over multi-joint robot to target a deficit or reduce a compensatory mechanism?; and (e) How does providing assistance/resistance change the way we learn? In order to answer these questions, we need to understand: (1) the effect of RDs on functional recovery, as well as biomechanical, physiological, and cortical mechanisms, and (2) the effect of mechanical and software (control) characteristics of RDs on time since injury, as well as on the various deficits. To date, most published studies have analyzed functional recovery, but there is limited research on the effect of RD over-ground gait training on biomechanical, physiological, and cortical mechanisms in children and adults with ABI. Biomechanical and physiological outcomes quantitatively reflect the underlying impairment in joint mechanisms, inter-limb coordination or balance mechanisms, and their recovery. Understanding the changes in gait and balance mechanisms will help us understand the reasons for the observed functional changes and will help us to better understand recovery. Research on structural and functional changes in the cortical and subcortical levels will help us understand the underlying mechanisms of neuroplasticity. Therefore, comprehensive efficacy studies across parameters will help us understand the effects of RDs and how to improve rehabilitation strategies. Most of the available literature reviews on exoskeleton research have focused on design and development activities in terms of electromechanical design or software controllers to provide optimal and efficient device (Dollar and Herr, 2008; Viteckova et al., 2013; Shi et al., 2019; Lee et al., 2020). Other reviews were on gait trainers/non-overground robotic devices that are very different from overground robotic exoskeletons (Moucheboeuf et al., 2020). Several reviews had a narrower focus; such as reviews on only randomized clinical trials or reviews of safety, ease of use, or feasibility of use in clinical environments (Mehrholz and Pohl, 2012; Poli et al., 2013; Federici et al., 2015, 2016; Schwartz and Meiner, 2015; Wall et al., 2015; Louie and Eng, 2016; Alias et al., 2017; Hill et al., 2017; Jayaraman et al., 2017; Lefeber et al., 2017; Bruni et al., 2018; Mehrholz et al., 2018; Molteni et al., 2018; Weber and Stein, 2018; Postol et al., 2019; Moucheboeuf et al., 2020; Pinto-Fernandez et al., 2020; Swank et al., 2020a; Dijkers et al., 2021; Sale et al., 2021). Though they provide a great insight into the usage of the device, they do not help us to understand the relationship between training, neuroplasticity, and functional recovery.
This review is targeted at researchers and developers in the field of robotic neurorehabilitation. The goal is to provide a comprehensive review of state of the science, i.e., the clinical and pre-clinical research on the therapeutic effects of various over-ground gait training RDs, and to identify gaps in research areas in order to identify directions for further investigation. We present and discuss existing assessments in terms of functional, clinical, biomechanical, physiological, and cortical mechanisms. We also provide guidelines and recommendations for clinical and pre-clinical research, taking into account the clinical needs of the patient population.
2. Methodology
This review was conducted in accordance with the framework proposed by Moher et al. (2009). PubMed, and Scopus databases were accessed and searched from inception to July 31, 2021. We combined the search terms (lower extremity exoskeletons OR lower limb exoskeleton OR gait exoskeleton OR exoskeleton ambulation OR exoskeleton walking), with humans and English language as limits. All duplicates between the search criteria were removed.
Inclusion criteria were full-text, peer-reviewed articles that used a powered robotic exoskeleton device (RD) with adults and children post acquired brain injury as an intervention for overground gait rehabilitation. Articles were included if they reported functional outcomes (e.g., speed, distance, independence, etc.), clinical outcomes [e.g., Functional Independence Measure (FIM), Gross Motor Function Classification (GMFC), etc.], biomechanical outcomes (e.g., kinematic, kinetic, temporal-spatial, etc.), physiological [e.g., Electromyography (EMG), etc.] and neurological [e.g., Magnetic Resonance Imaging (MRI), functional Magnetic Resonance Imaging (fMRI), functional Near Infrared Spectroscopy (fNIRS), etc.].
Lower extremity RDs are herein operationally defined as a wearable robotic device that actuates at least one of the three lower extremity joints (hip, knee, and ankle) during overground gait either unilaterally or bilaterally in one or more movement planes'. Articles were excluded if they were on neurological conditions other than ABI; articles on industrial and military applications; reported only technology development; reported only orthotic effect of RD; reported only feasibility of usage; included only healthy participants; utilized a treadmill-based device; or if only an abstract was available. Titles and abstracts were screened for relevance by two authors according to the inclusion and exclusion criteria above. In the event of conflict, a third author was consulted for resolution. Full-texts were then screened, and reference lists of all selected articles were searched for additional studies. Included articles were then examined to extract data regarding study design, RD, participant characteristics, intervention, training period, outcome measures, adverse effects, and results. We examined the changes in functional, clinical, biomechanical, physiological, and neurological outcomes published in the qualifying literature.
A total of 6,908 articles were retrieved using the search criteria. After removing the articles based on the inclusion and exclusion criteria (Figure 1), 57 articles remained and were included for this review.
3. Results
Figure 2 shows all the studies included in this review to give an overview of the state of the science in the field of therapeutic exoskeletons. RD Research is sparce in TBI and CP diagnosis, with no randomized clinical trials (RCT's) or intervention studies with a control group currently available in these two populations. In stroke, about half the studies have a control group (i.e., randomized control trial, randomized cross over, intervention study with control and retrospective study). Figure 3 shows the average age by population. Studies on CP (6 studies < 18 years; 2 studies < 31 years) and TBI (ages >13 and < 30 years) have predominantly been on pediatric and young adults. On the other hand, all stroke studies have been on middle age to older adults (ages >35 and < 80 years). Studies on CP had participants with quadriplegia (1 study), and diplegia (7 studies), while all participants in TBI and stroke had one sided weakness (hemiplegia). Age, affected side, sex, and diagnoses are detailed in Table 1.
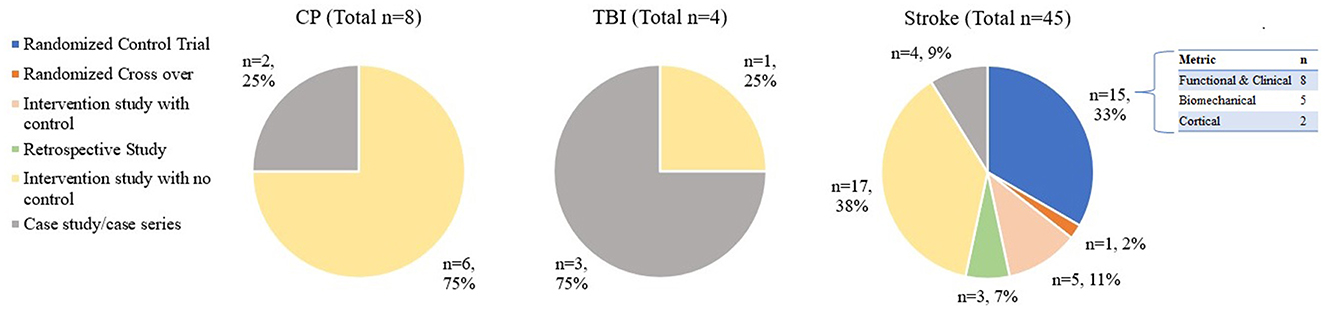
Figure 2. Studies divided based on population and further divided based on type of study. Some of the studies with Biomechanical and cortical outcomes also presented functional and studies with functional outcome also presented biomechanical metrics as secondary outcomes. Please refer to Table 1 for all the outcomes measures.
The review results have been divided based on the diagnosis [cerebral palsy (CP), traumatic brain injury (TBI), and stroke]. The review is further divided based on outcomes metrics (functional and clinical, physiological and biomechanical, and neurological). The soft RD's were reviewed separately from rigid exoskeletons. Table 2 describes the known technical characteristics of all the exoskeletons reviewed in this article. Table 1 describes the studies reviewed in this article. Abbreviations are listed in Table 3.
3.1. Cerebral palsy
RD sessions across studies were quantified for the cerebral palsy (CP) population to demonstrate the dosing effect, and is shown in Figure 4A. There is variability across these limited studies in terms of dosing (number of sessions); 62% of the studies were between 5 and 10 sessions. The distribution of number of participants across all studies in CP was quantified in order to understand the generalizability and impact of RD evidence (Figure 4B).
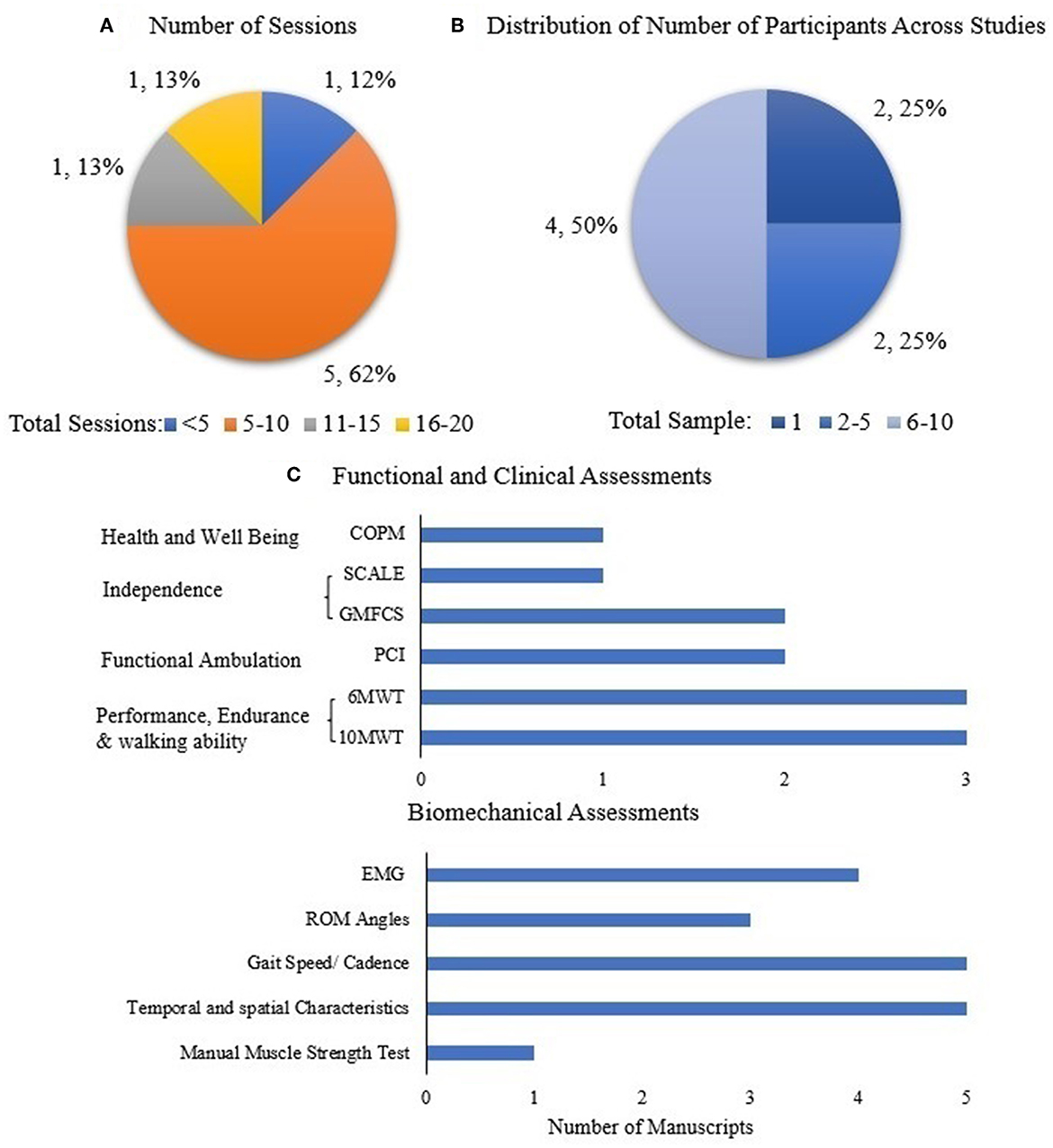
Figure 4. (A) Number of sessions across all studies and (B) distribution of number of participants across all studies in CP population. Data is represented as the number of studies and as percentage with respect to the total number of studies. (C) Assessments used across all studies in CP population.
Figure 4C shows the distribution of functional and clinical, and biomechanical assessments across all studies (case study, intervention) in the CP Population. In the limited number of studies in CP population, the most widely used outcomes are performance, endurance and walking ability (10MWT, 6MWT, TUG), as well as spatial-temporal characteristics and cadence.
3.1.1. Rigid exoskeletons
3.1.1.1. Biomechanical and physiological outcomes
Utilization of RD (HAL) gait training in children and adults with CP was evaluated. The preliminary analysis demonstrated improvements in functional and biomechanical outcomes such as gait speed, step length, and cadence in children and adults with no change in GMFM (Ueno et al., 2019). A case study on clinical and physiological metrics also showed that 6 minute walk test (6MWT), gross motor functional measure (GMFM), and Canadian Occupational Performance Measure (COPM) increased, while Physiological Cost Index (PCI) declined after the RD (HAL) intervention (Kuroda et al., 2020).
Similar results were seen while using RD (CPWalker) to restore ambulation. An improvement in biomechanical metrics including step length (Bayón et al., 2016), spatial-temporal parameters (Bayón et al., 2018), and cadence (Bayón et al., 2016), as well as functional and clinical outcomes, such as speed (Bayón et al., 2016, 2018), D and E dimensions (assessed together) of the GMFM-88 scale (Bayón et al., 2018), endurance (6MWT) (Bayón et al., 2018), and strength at the hip and knee (Bayón et al., 2018).
Lerner et al. (2017a) designed a novel RD (ultraflex system) that provides on-demand assistance for knee extension while preserving (or enhancing) muscle activity of the user in CP patients to improve crouch gait. The results from an initial evaluation showed an increase in peak knee extension (Lerner et al., 2017b). The powered exoskeleton significantly altered lower extremity kinematics and reduced the amount of crouch compared to the baseline (BL) condition, resulting in a gait trajectory similar to normal walking (Lerner et al., 2017b). Lerner et al. showed that the knee extension RD for crouch gait increased vastus lateralus (VL) and semitendinosus (SEMI-T) activity during swing and stance respectively on both the affected and unaffected limb (Lerner et al., 2017b). The variability in VL and SEMI-T were low after continued use of this RD (Bulea et al., 2018).
Research on bilateral ankle (Adaptive Ankle) RD showed that participants improved their walking speed and stride length with a corresponding increase in soleus (SO) and VL muscle activity, where SO activity was 39% similar to unimpaired individuals after RD training (Fang et al., 2020).
3.2. Traumatic brain injury
RD sessions across studies were quantified for the TBI population to demonstrate the dosing effect, and were found to be 12 sessions across the limited number of pre-clinical studies. The distribution of number of participants across all studies in TBI was quantified in order to understand the generalizability and impact of RD evidence (Figure 5A).
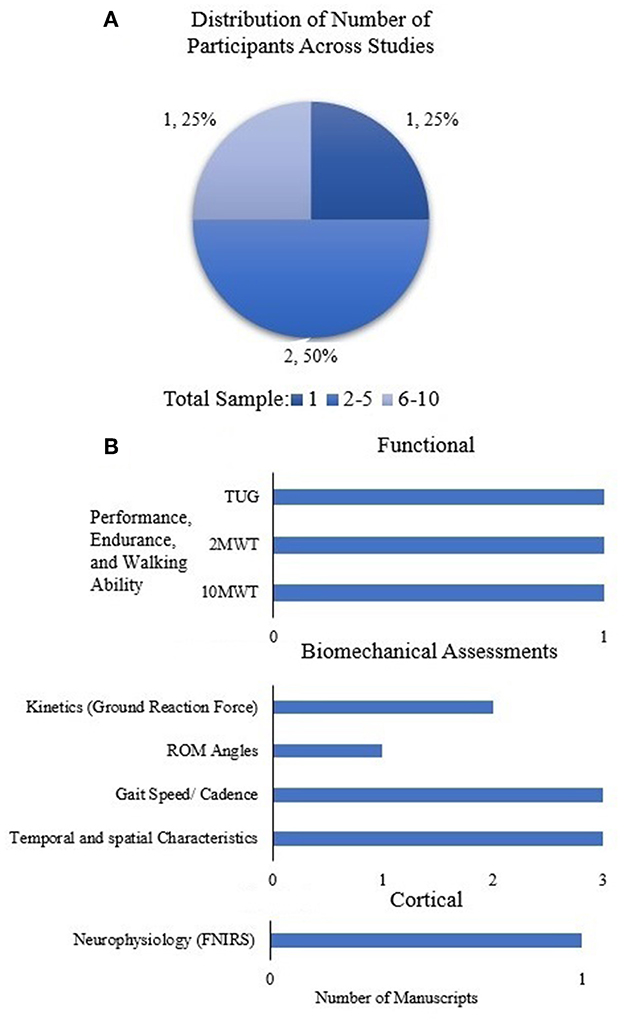
Figure 5. (A) Number of participants across all studies in TBI. Data is represented as the number of studies and as percentage with respect to the total number of studies. (B) Assessments used across all studies in TBI population.
Figure 5B shows the distribution of functional and clinical, biomechanical, and cortical assessments across all studies (case study, intervention) in the TBI population.
3.2.1. Rigid exoskeletons
3.2.1.1. Biomechanical outcomes
3.2.1.1.1. Effects of RD in individuals with acute TBI
A case study by Nolan et al. (2018) showed that 4 weeks of RD (Ekso) training in a single young adult with acute TBI had a therapeutic effect after utilizing RD. The participant had a consistent prolonged stance phase bilaterally and performed a more symmetrical gait cycle. RD training resulted in reduced joint angle variability, increased plantar flexion and dorsiflexion, and increased bilateral symmetry, but with decreased walking speed, step length, and swing time (Nolan et al., 2018). There was also an increased compensatory mechanism of hip circumduction.
3.2.1.1.2. Effects of RD in individuals with chronic TBI
TBI research by Karunakaran et al. (2019, 2020a) evaluated the effect of 4 weeks of RD training on gait mechanisms in adolescents and adults with chronic ABI. The results showed that there could be potential long-term effects of improved linearity of loading during initial double support, healthy bilateral loading characteristics, improvement in spatial symmetry, swing time, stance time, and step length with an associated increase in speed, due to RD gait training (Karunakaran et al., 2019, 2020a).
3.2.1.2. Neurological outcomes
3.2.1.2.1. Effects of RD in individuals with chronic TBI
The same group also evaluated the neurophysiological response to RD training in a case study with a participant diagnosed with TBI (Karunakaran et al., 2020b). The results showed that at follow-up there was decreased activity in motor cortex, pre-motor cortex, and supplementary motor area (SMA) with corresponding improvement in gait and balance [improved gait speed and timed up and go (TUG)], suggesting that the participant required less attentional resources to perform the walking task (Karunakaran et al., 2020b).
3.3. Stroke
RD sessions across studies were quantified for the stroke population to demonstrate the dosing effect, and are shown in Figure 6. There is variability across studies in terms of dosing (number of sessions); 80% of the studies were between 5 and 20 sessions. The distribution of the number of participants across all studies in stroke was quantified in order to understand the generalizability and impact of RD evidence. Eighty five percent of the studies had a sample of < 50 participants. This includes intervention and control groups (Figure 6).
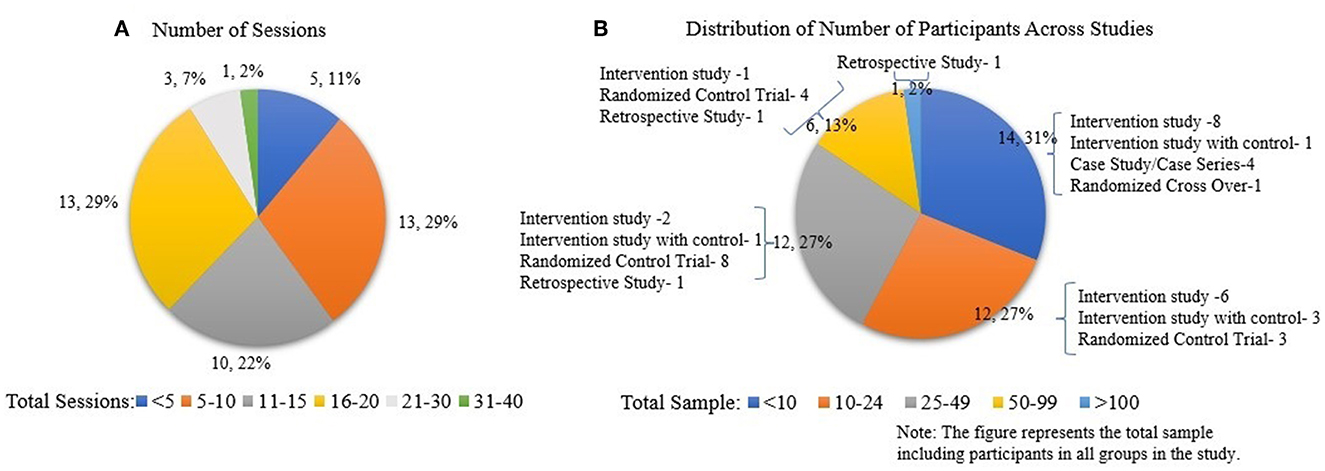
Figure 6. (A) Number of sessions across all studies and (B) distribution of number of participants across all studies in stroke population. Data is represented as the number of studies and as percentage with respect to the total number of studies.
Figure 7 shows the organization of stroke research in this paper.
3.3.1. Rigid exoskeletons
Figure 8 shows the different outcomes used in the RCTs and the randomized crossover trial for rigid exoskeletons. Most of the RCTs in stroke focus on understanding the effects of RDs, using mostly functional and clinical measures, with very few in other domains. Table 4 shows the distribution of functional and clinical assessments across all studies (RCT, case study, intervention, retrospective, and cross over). The most widely used outcome measures across all studies were performance, endurance and walking ability measures (10MWT, 6MWT, TUG), followed by balance (BBS) and functional ambulation (FAC). Compared to functional outcomes, only a limited number of studies evaluated biomechanical and cortical outcomes. The most widely used biomechanical outcomes are spatial-temporal characteristics and cadence.
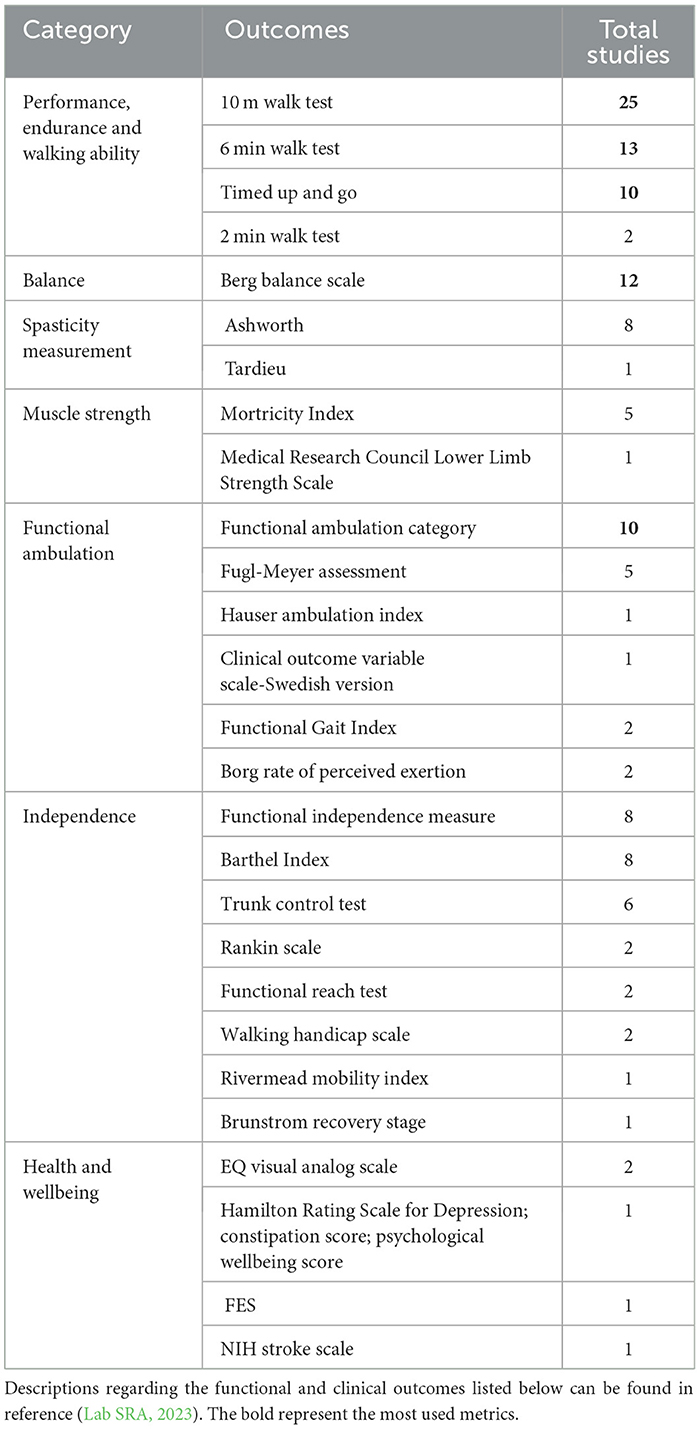
Table 4. Distribution of studies based on functional and clinical assessment categories across all studies for Rigid RD.
3.3.1.1. Functional and clinical outcomes
3.3.1.1.1. Effects of RD in individuals with acute stroke
A retrospective study showed that individuals with acute stroke who received RD (Ekso) training walked twice the distance compared to the standard of care/conventional gait training (SOC/CGT) group during their inpatient physical rehabilitation, though both groups received the same duration of training (time spent in inpatient rehabilitation training session) and similar dosing (Nolan et al., 2020). The RD group also increased their motor FIM score (change from admission to discharge) and motor FIM efficiency compared to the SOC group, though both groups were matched for admission motor FIM scores (Nolan et al., 2020). Similar study evaluating the therapeutic effect on functional ambulation in adults with acute stroke after RD (Ekso) gait training (Karunakaran et al., 2021). The results showed that RD provided high dose training and that there were significant improvements in 10 meter walk test (10MWT), 6MWT, and TUG at follow-up compared to baseline (Karunakaran et al., 2021). Utilization of RD (Ekso) in 96 individuals with acute stroke showed that participants increased their “walk” time and number of steps from session 1 to 5, followed by a plateau from session 5 onwards (with most sessions lasting about 20 mins). Significant differences were observed in Stroke Rehabilitation Assessment of Movement, but weren't observed in FIM in this study (Swank et al., 2020b). In another set of studies, the HAL RD system provided intensive, repetitive gait training in hemiparetic patients in people with acute stroke. All patients improved their walking ability during the training period, as reflected by the 10MWT (from 111.5 to 40 s in median) and the Functional Amblation Categories (FAC) (from 0 to 1.5 score in median) (Nilsson et al., 2014). Similarly, there were significant differences in the Brunstrom recovery stage, FIM total, and FIM motor subscore in the RD (HAL) group compared to SOC at discharge, though there were no significant differences on rehabilitation admission between the groups (Taki et al., 2020). There were also no significant differences in the global disability and score change, defined by modified Rankin Scale, between the groups (Taki et al., 2020). Research on the effects of 4 weeks of BEAR-H1 RD training with conventional training in acute and subacute stroke (Li et al., 2021) showed that there were significant improvements in 6MWT, Fugl-Meyer Assessment-Lower extremity (FMA-LE), gait speed, cadence, step length, and cycle duration at follow-up compared to baseline as well as in the RD group compared to conventional training group with no change in FAC and Modified Ashworth Scale (MAS) between groups (Li et al., 2021).
3.3.1.1.2. Effects of RD in individuals with subacute stroke
Research using RD (EKSO) has shown significant improvements in functional outcomes (6MWT (Molteni et al., 2017; Goffredo et al., 2019), and 10MWT (Molteni et al., 2017; Goffredo et al., 2019), as well as in clinical outcomes [Barthel Index (BI) (Goffredo et al., 2019), Motricity Index (MI) (Molteni et al., 2017; Goffredo et al., 2019), FAC (Molteni et al., 2017; Goffredo et al., 2019), and Walking Handicap Scale (WHS) (Goffredo et al., 2019)], but no change was observed in the MAS (Goffredo et al., 2019) and the Trunk control test (TCT) (Molteni et al., 2017; Goffredo et al., 2019) in individuals with subacute stroke. Also, clinical trials in individuals with sub-acute stroke showed that 6MWT, MI-Affected Limb, 10MWT, mBI, MAS-Affected Limb, FAC, and WHS showed similar results in both conventional training and training with RD (Ekso) (Molteni et al., 2021).
Research using RD (HAL) gait training increased the walking speed and the distance walked during 2-minute walk test (2MWT), cadence, and stride length in subacute stroke (Mizukami et al., 2016). The Berg Balance Scale (BBS), FMA, FAC, and PCI also improved, but not significantly (Mizukami et al., 2016). A randomized clinical trial showed significant improvement in FAC and that the improvement was retained 2 months post-intervention, while maximum walking speed, stride, cadence, 6MWT, TUG, and FMA of the lower extremity did not show an effect (Watanabe et al., 2017). Research using an RD (wearable ankle) showed FAC and walking speed improved after using the RD compared to the control group (Yeung et al., 2021).
3.3.1.1.3. Effects of RD in individuals with chronic stroke
In individuals with chronic stroke, MI, FAC, 10MWT and 6MWT showed improvements, while the Ashworth scale and WHS did not show any improvements after RD (Ekso) training (Molteni et al., 2017). A similar study comparing RD (Ekso) training to the control group also showed minimal clinically important difference (MCID) in 10MWT and 6MWT (Schröder et al., 2019). A randomized study in individuals with chronic stroke showed that the RD (Ekso) group improved in all Coping Orientation to Problems Experienced outcomes, FIM, 10MWT, TUG, and River Mobility Index (RMI), as well as achieved a greater improvement in constipation and QoL than SOC (De Luca et al., 2020). A pilot study compared the wearable RD (Ekso) with both end effector training and SOC. The results showed no significant difference between the three groups (Pournajaf et al., 2019). Although statistical significance was not obtained, pre-post differences in the RD and end effector groups in TUG, 10MWT, and 6MWT were higher than the MCID values reported in the literature. These differences were not found in the SOC group (Pournajaf et al., 2019).
All patients who received HAL training showed significant improvements in 10MWT, cadence, number of steps, TUG, functional reach (FRT), and BBS compared to SOC in chronic stroke patients (Yoshimoto et al., 2015). Similarly, a longitudinal study by Tanaka et al. showed that gait speed, stride length, cadence, and 2MWT were significantly increased after the RD (HAL) gait training, and the effects were retained after 3 months in chronic stroke patients (Tanaka et al., 2019). Similarly, a case study on a participant with chronic stroke showed improvement in 10MWT, TUG, FRT, two-step test, and BBS after RD (HAL) training, and the improvements were retained at 2 month follow-up (Yoshimoto et al., 2016). RD (HAL) intervention significantly improved gait speed, cadence, BBS, and the number of steps assessed by the 10MWT in individuals with chronic stroke. The TUG also improved though was not statistically significant (Kawamoto et al., 2013). The individuals were further divided into independent ambulatory and dependent ambulatory subgroups. Both groups showed significant change in BBS while only the dependent ambulatory subgroup showed statistically significant differences in walking speed, cadence, and number of steps (Kawamoto et al., 2013). Preliminary study on RD (Indego) with participants with chronic TBI and stroke showed improvements in speed and endurance in 10MWT and 6MWT respectively. Some of the participants also improved their FAC level (Jyräkoski et al., 2021).
Preliminary investigation of the RD (H2) gait training in the three chronic stroke patients showed that the participants performed a more symmetric gait in RD training and there were slight improvements in 6MWT, TUG, and FMA-LE in all the participants after training (Bortole et al., 2015). Researchers investigated the effectiveness of the developed RD (Robot-assisted ankle-foot-orthosis) on chronic stroke patients (Yeung et al., 2018). The results showed improvement in FAC and 10MWT while wearing RD while FMA also improved from pre to post-training. There were no significant differences found in MAS, BBS, and 6MWT (Yeung et al., 2018). Walking with passive RD (Exoband) showed that walking distance and gait speed increased in people with stroke after training compared to baseline (Panizzolo et al., 2021). Rehabilitation using ExoAtlet RD in chronic stroke showed that the speed, BBS, and TS showed improvement after RD training (Kovalenko et al., 2021).
3.3.1.2. Biomechanical and physiological outcomes
Research has found a median perceived exertion on the Borg Scale after RD training (Ekso), with a statistically significant change in walking time, standing time, and number of steps with the progression of gait training and MAS in sub-acute stroke patients (Høyer et al., 2020). This study shows somewhat above fairly light exertion training with RD. The study also showed that participants walked greater distances and achieved more steps throughout the training sessions, but with lessened cardiorespiratory strain during RD training. The authors suggest that RD-assisted gait training is less energy consuming and less cardiorespiratory stressful than walking without RD-assistance, encouraging more repetitions in participants. This, in turn, could strengthen motor learning and control (Høyer et al., 2020).
Analysis of the load distribution on feet with open and closed eyes in both groups [RD (Ekso GT) and SOC] showed a small and non-significant tendency to reduce the amount of uninvolved limb loading after therapy, which may indicate gradual improvement in limb loading symmetry (Rojek et al., 2020). Results also indicate that after training with the RD, load distribution between the limbs was better than in SOC (Rojek et al., 2020), though both groups improved their loading characteristics after training. In addition, observed changes in BI and RMI also showed a significant improvement in the RD group compared to the control group (Rojek et al., 2020).
A preliminary study showed that one session of RD (Vanderbilt) training in individuals with stroke resulted in improvements in gait speed, spatial symmetry, and step length (Murray et al., 2014, 2015). The effect of the RD (SMAS) on the biomechanical metrics were evaluated (Buesing et al., 2015). The results showed a significantly large increase in step length and spatial symmetry in the SMAS group than the control group. There was an increase in velocity, stride length and step length on both impaired and non-impaired sides with an associated decrease in swing time on the affected side and double support time for both groups (Buesing et al., 2015).
Researchers quantified improvement of lateral symmetry after using single-leg RD (HAL) using EMG in acute stroke patients (Tan et al., 2018). The results showed a significant increase in similarity between lateral synergies of patients with a corresponding increase in gait measures like walking speed, step length, step cadence, stance duration, and percentage of gait cycle (Tan et al., 2018). In addition, improvements in FIM-locomotion, FIM-motor, and FMA scores were also observed (Tan et al., 2018). Researchers utilized muscle synergy analysis to show gait symmetry in subacute stroke patients that underwent RD (HAL) gait training (Tan et al., 2020). The results showed no significant differences in muscle synergy symmetry between RD and SOC groups though the timing of muscle synergies was symmetrical in the HAL group but not in the control group. Intergroup comparisons of symmetry in muscle synergies and their timings were not significantly different. This could be due to large variability in recovery in the control group. Finally, stance time ratio was not observed to improve in both groups after their respective therapies (Tan et al., 2020). There was a significant increase in FIM-locomotion, FIM-motor, and FMA scores in both the HAL group and control group (Tan et al., 2020).
Muscle strength changes after use of RD (UG0210) using the manual muscle strength test of the tibialis anterior muscle in acute and subacute stroke patients were evaluated. No significant difference was observed between control and RD groups but both groups improved after training (Zhang et al., 2020).
The preliminary study evaluated the effect of RD (Ekso) training on EMG and functional gait in individuals with subacute stroke (Infarinato et al., 2021). MI-affected limb, FAC showed significant improvement, while MAS-Affected Limb, TCT, and 10MWT did not show a change after training (Infarinato et al., 2021). Bilateral Symmetry and mean root mean square improved in TA and co-contraction decreased in proximal muscles after RD training (Infarinato et al., 2021).
Increases in strength in the paretic muscles were noted, along with increases in stability, functional level, and walking speed in the group with RD (ExoAtlet) compared to without RD (Kotov et al., 2021). Comparison of stabilometric also showed improved outcomes in RD compared to without RD (Kotov et al., 2021). An increase in stride length and decrease in gait speed was observed after RD training in acute stroke (Kotov et al., 2021). The effect of RD (Ekso) on neuromuscular coordination was evaluated in individuals with chronic stroke and compared to healthy controls (Zhu et al., 2021). Spatial-temporal parameters, kinematics, and muscle synergy pattern were analyzed. The results showed the motor modules for steadfast walking were described by four distinct motor modules described in heathy controls and three modules in the paretic leg of the stroke patients. Muscle coordination complexity, module composition, and activation timing were preserved after the training. In contrast, walking with the RD altered the stroke subjects' synergy pattern, especially on the paretic side. The changes were dominated by the activation profile modulation toward the normal pattern observed in the healthy controls (Zhu et al., 2021).
Researchers determined whether the newly developed hip-assist robot (GEMS) was effective in improving biomechanical and physiological outcomes in stroke patients (Lee et al., 2019). Gait speed, cadence, stride length, gait symmetry, sEMG of bilateral RF, BF, TA, and MG, cardiopulmonary metabolic energy efficiency, FMA, fall efficacy scale, and BBS were measured. The results showed an improvement in all biomechanical outcomes, except gait symmetry in the RD group. There was an improvement in all muscle activity in RD and only in RF for CGT. There was a decrease in metabolic energy, FMA, and Fall Efficacy Scale for RD (Lee et al., 2019).
Robotic leg orthosis (RLO) is an actuated RD that provided assistance for extension and flexion. Preliminary data showed improved clinical (BBS and LE-FMA) outcomes (Li et al., 2015). The participants also improved their cadence, step length, and walking speed. The EMG results showed there was an increase in the normalized root mean square values of the MG and BF on the affected side. Additionally, EMG activities of the agonist and antagonist pair (i.e., RF and BF) appeared to return to similar levels after training. The peak moment of hip flexor, knee extensor, and plantar flexor, which all contributed to push-off power, were found to have increased after 3 weeks of training (Li et al., 2015).
3.3.1.3. Neurological outcomes
RD (Ekso) training, in hemiplegic chronic stroke patients, resulted in greater improvement with medium to large effect size in 10MW, RMI, TUG, CSE and sensory-motor integration (SMI) using Transcranial Magnetic Stimulation (TMS), frontoparietal effective connectivity (FPEC) using EEG, than SOC (Calabrò et al., 2018). These changes were accompanied by improved stance/swing ratio, gait cycle duration, reduced limb asymmetries, and hip and knee muscle activation. The strengthening of FPEC, the increase of SMI in the affected side, and the decrease of SMI in the unaffected side were the most important factors correlated with the clinical improvement. The RD induced a reshape of CSE of both hemispheres, whereas SOC change mainly pertained to the affected CSE. This research concluded that RD induced a more evident remodulation of SMI between the hemispheres as compared to SOC (Calabrò et al., 2018).
EEG was used to understand short-term changes due to RD (Ekso) training in people with chronic stroke. Study findings showed a strong relation between lesion lateralization, dominance and connectivity modulations (Molteni et al., 2020). Right-hemisphere (non-dominant) stroke participants showed an increase of connectivity Node strength (NS) over the contralesional motor cortex and ipsilesional prefrontal cortex after exoskeleton training. They displayed a modification of connectivity after RD-assisted gait toward a pattern similar to one described during ankle dorsiflexion in able-bodied persons suggesting short term neuroplasticity. In left-hemisphere stroke participants (dominant hemisphere lesion), the connectivity pattern is different. Even after RD-assisted gait, both connectivity betweenness centrality (BC) and NS point to a preeminent role of the vertex node, that is, the scalp field projection of the neurons discharging in the lower limb area. In these persons, a foot task does not activate a complex network, but only a focal activity over the corresponding motor cortex, thereby suggesting a lower degree of local efficiency and reorganization (Molteni et al., 2020).
Research by Jayaraman et al. showed that significant functional improvement in walking speed, berg balance scale, and endurance with an associated change in corticomotor excitability (CME) using TMS after intervention with the RD compared to the control group (Jayaraman et al., 2019). This suggests that RD may promote greater walking speed, endurance, balance, and CME than control.
3.3.2. Soft exoskeletons
Figure 9 shows the distribution of functional and clinical and cortical assessments across all studies in the stroke population. In the limited number of studies utilizing soft RD in the stroke population, the most widely used outcomes are performance, endurance and walking ability measures (10MWT, 2MWT).
3.3.2.1. Functional and clinical outcomes
The feasibility study on RD (ReWalk Restore) showed that individuals with stroke increased their walking speed with and without RD after 5 days of RD training (Awad et al., 2020). It is noteworthy that 36% of study participants achieved a large meaningful increase (i.e., ≥ 0.10 m/s) in their unassisted maximum walking speed after only 5 days of training (Awad et al., 2020). Preliminary feasibility study on RD (Myosuit) showed improvements in walking speed while the distance in 2MWT reduced after four sessions of RD training (Haufe et al., 2020).
Randomized clinical trial in people with subacute stroke showed the RD (Regent) significantly improved gait speed and BBS. In addition, improvements were also observed in FIM and BI (Monticone et al., 2013).
3.3.2.2. Neurological outcomes
Cortical reorganization in 14 patients with chronic stroke after the use of Regent RD was analyzed using fMRI using a passive sensorimotor paradigm imitating the support loading during slow walking (Saenko et al., 2016). The results showed that the temporal characteristics of walking improved, which was accompanied by a decrease in the activation zones of the inferior parietal lobules, especially in the healthy hemisphere, and a significant increase in the activation zones of the primary sensorimotor and supplementary motor areas (Saenko et al., 2016). Functional connectivity analysis showed significant changes in intrahemispheric and interhemispheric interactions (Saenko et al., 2016).
Navigated TMS (nTMS) was used to assess the changes in gait cortical control after using RD (Regent) for 10 sessions in individuals with chronic stroke (Poydasheva et al., 2016). Results showed increased speed with no change in FMA. During nTMS, a reduction was recorded in the average latency of evoked motor response from the affected hemisphere, as well as various patterns of changes in the size and localization of cortical representations of the leg muscles (Poydasheva et al., 2016).
4. Discussion
Current research is focused on improving gait and balance mechanisms using RDs for rehabilitation (Federici et al., 2016; Louie and Eng, 2016; Lefeber et al., 2017; Molteni et al., 2018; Moucheboeuf et al., 2020). These robotic devices may provide support for gait and/or balance, assist with joint movements, or reduce the use of compensatory mechanisms during walking. Most of the reviews on RD research have focused on design and development activities in terms of electromechanical design or software controllers with a view to provide optimal control (Dollar and Herr, 2008; Viteckova et al., 2013; Shi et al., 2019; Lee et al., 2020). Many reviews were on gait trainers/non-overground robotic devices, which are very different from overground robotic RDs (Moucheboeuf et al., 2020). Other reviews had a narrower focus; such as reviews on only randomized clinical trials or reviews of safety, ease of use, or feasibility of use in clinical environments (Mehrholz and Pohl, 2012; Poli et al., 2013; Federici et al., 2015, 2016; Schwartz and Meiner, 2015; Wall et al., 2015; Louie and Eng, 2016; Alias et al., 2017; Hill et al., 2017; Jayaraman et al., 2017; Lefeber et al., 2017; Bruni et al., 2018; Mehrholz et al., 2018; Molteni et al., 2018; Weber and Stein, 2018; Postol et al., 2019; Moucheboeuf et al., 2020; Pinto-Fernandez et al., 2020; Swank et al., 2020a; Dijkers et al., 2021; Sale et al., 2021). Though they provide a great insight into the usage of the device, they do not help us to understand the relationship between training, neuroplasticity, and functional recovery. In this paper, we have reviewed various preliminary and pre-clinical, as well as clinical, research that evaluated RDs using functional, clinical, biomechanical, physiological, and cortical outcomes. We carefully delineate between the preliminary studies and RCTs in interpretation of existing evidence. The goal was to provide a review of the state of the science and to provide directions for further investigation. Another feature of this review is that for the first time the downstream (functional, biomechanical and physiological) and upstream (cortical) metrics used to evaluate various RDs in ABI are presented. In order to understand both device effectiveness and neuroplasticity, it is important to understand RD effectiveness in the context of all these metrics. This paper provides a comprehensive review of the clinical and pre-clinical research on the therapeutic (pre-post) effects of various over-ground gait training RDs. This review lays the foundation to understand the knowledge gaps that currently exists in RD rehabilitation research. Though there is initial preliminary evidence on efficacy of RD, a comprehensive randomized controlled clinical trial is required to fully understand the therapeutic effects of RD in ABI.
Our review shows that RDs could be used for gait therapy across age groups, based on the ability to fit into exoskeletons. It has also shown that RDs could be used across deficit characteristics (i.e., diplegia, hemiplegia, and quadriplegia). The selection of an RD would be dependent on the amount of support and control the user requires from the robot. A non-ambulatory participant with ABI would require maximal assistance with rigid supports to walk and balance. On the other hand, an ambulatory participant may require assistance to perform accurate symmetrical gait with limited supports (soft RD), allowing for multidimensional walking and balance. Further research is required to precisely determine the optimal use of rigid and soft RDs at different stages of recovery to allow for treatment progression.
A healthy walking control mechanism involves supporting the body weight and providing stability in the forward and lateral directions during forward progression (Winter, 2009; Perry and Burnfield, 2010). In each step, stability and balance are associated with progression (Winter, 2009; Perry and Burnfield, 2010). The control mechanism needs to be able to scan the environment for obstacles and adjust the gait pattern and balance in order to adapt to the environment. Individuals with ABI often present with loss of voluntary movement, loss of movement co-ordination, muscle weakness, etc., resulting in gait and balance control mechanism deficits (Kerrigan et al., 2000; Williams et al., 2009; Winter, 2009; Kemu, 2010; Perry and Burnfield, 2010; Dubin, 2014; Sheffler and Chae, 2015). Compensatory mechanisms like steppage gait, hip hiking, toe walking, crouch gait, etc. are used to ambulate successfully (Kerrigan et al., 2000; Williams et al., 2009; Winter, 2009; Kemu, 2010; Perry and Burnfield, 2010; Dubin, 2014; Sheffler and Chae, 2015). These pathological deviations from healthy walking result in slower walking speed, shorter step length, reduced gait and balance adaptability, and increased risk of falls (Kerrigan et al., 2000; Williams et al., 2009; Winter, 2009; Kemu, 2010; Perry and Burnfield, 2010; Dubin, 2014; Sheffler and Chae, 2015).
The last decade has seen an enormous growth in the development of RDs for neuro-rehabilitation (Dollar and Herr, 2008; Yan et al., 2015; Esquenazi et al., 2017; Iandolo et al., 2019). Various software (variable assistance, resistance) and mechanical (soft and rigid structures with different actuator mechanisms) designs for RDs have enabled the provision of individual assistance, from supporting a particular segment or joint (multiple degrees of freedom) to complete control and stability to perform gait and balance (Dollar and Herr, 2008; Yan et al., 2015; Esquenazi et al., 2017; Iandolo et al., 2019). There is increasing evidence showing the feasibility and safety of various RDs in ABI (Dollar and Herr, 2008; Yan et al., 2015; Esquenazi et al., 2017; Iandolo et al., 2019). Therapies with RDs are combining intensive therapy with quality gait and balance training (Federici et al., 2016; Louie and Eng, 2016; Lefeber et al., 2017; Molteni et al., 2018; Moucheboeuf et al., 2020).
Research has shown preliminary evidence for the efficacy of using RDs for CP rehabilitation (Bayón et al., 2016, 2018; Lerner et al., 2017a,b; Bulea et al., 2018; Ueno et al., 2019; Fang et al., 2020; Kuroda et al., 2020). RDs in CP have been designed to target either deficit or function. Both kinds of RDs have shown moderate improvement in functional outcomes (such as speed and endurance, Figure 4) and biomechanical outcomes (such as spatial-temporal characteristics, joint angles, and EMG activation, Figure 4) in preliminary studies. Though this is promising, rigorous randomized controlled trials with large sample size on the use of RDs for CP rehabilitation are largely missing to date.
Research on the usage of RDs in TBI (Figure 5) has been scarce. Research is mostly on providing initial evidence on biomechanical characteristics with associated cortical changes after use of RDs in TBI for restoration of gait and balance (Nolan et al., 2018; Karunakaran et al., 2019, 2020a,b). Pre-clinical and randomized clinical trials are lacking for RD use in individuals with TBI, making it hard to assess the efficacy of RDs in TBI patients.
Recently, there has been an increase in pre-clinical and clinical research to understand the functional and clinical changes after RD use in both acute and chronic stages of stroke. The most commonly studied exoskeletons were the Ekso and the HAL (Nilsson et al., 2014; Mizukami et al., 2016; Molteni et al., 2017, 2021; Watanabe et al., 2017; Goffredo et al., 2019; Nolan et al., 2020; Swank et al., 2020b; Taki et al., 2020; Karunakaran et al., 2021; Yeung et al., 2021). Multiple studies have shown that RD increased the walking distance and walking speed during acute and sub-acute stroke rehabilitation (Molteni et al., 2017, 2021; Watanabe et al., 2017; Goffredo et al., 2019; Karunakaran et al., 2021). The results from studies using Ekso RD have shown improvements in Stroke Rehabilitation Assessment of Movement (Swank et al., 2020b), with one of the studies showing significant improvement in FIM (Nolan et al., 2020), while the other did not (Swank et al., 2020b). Results from HAL RD in acute stroke rehabilitation have shown improvements in speed FAC, FIM, and BRs (Nilsson et al., 2014; Taki et al., 2020). A study using BEAR-H1 RD also showed improvement in functional metrics such as speed, endurance and FMA-LA (Li et al., 2021). Multiple studies have shown that Ekso and HAL improved speed, endurance, balance, and functional ambulatory metrics, but not in spasticity index, though not all studies showed significant differences between the control group and the RD gait training group (Nilsson et al., 2014; Mizukami et al., 2016; Molteni et al., 2017, 2021; Watanabe et al., 2017; Goffredo et al., 2019; Nolan et al., 2020; Swank et al., 2020b; Taki et al., 2020; Karunakaran et al., 2021; Yeung et al., 2021). These mixed results in the metric could be due to various characteristics such as dosing (number of steps) differences, the number of RD sessions (Figure 6A), low sample size (Figure 6B) or the differences in therapy focus (deficit focused, intensity focused or joint focused, etc.) gait training (Partridge et al., 2000; Peurala et al., 2009; Hornby et al., 2016). In order to understand the differences in results, randomized control trials with large sample sizes with these characteristics as covariates needs to be conducted. Current studies suggest that utilization of these exoskeletons may increase functional outcomes during the acute and sub-acute stages of recovery when used over multiple sessions. Though Ekso and HAL have very different control mechanisms, both exoskeletons provide bilateral support and assistance to perform gait in the sagittal plane. Further research is required to understand the effect of different control mechanisms on recovery in these individual devices, as well as between these devices. The effect of “number of steps” and “number of sessions” required to induce significant change also needs to be studied in order to better understand the effects and efficacy of each RD.
Similar results have also been observed in chronic stroke. Various RDs such as Ekso, HAL, Ankle RD's, ExoAtlet, ReWalk Restore, Myosuit, and H2 have shown improvement in speed, endurance, and balance; though not all metrics were significant across all RDs (Kawamoto et al., 2013; Bortole et al., 2015; Yoshimoto et al., 2015, 2016; Molteni et al., 2017; Yeung et al., 2018; Pournajaf et al., 2019; Schröder et al., 2019; Tanaka et al., 2019; Awad et al., 2020; De Luca et al., 2020; Haufe et al., 2020; Jyräkoski et al., 2021; Kovalenko et al., 2021; Panizzolo et al., 2021). The results do suggest that repeated consistent practice provided by RDs has the potential to induce recovery even during the chronic phase of stroke, where spontaneous plasticity reduces and recovery trajectory is slow. Even though the assistance provided to the joints, control mechanism, as well as support provided by the RDs were different, each of the RDs showed changes in recovery of function. Stroke can present with deficits across multiple joints. Each of the RDs may target different deficits. It would be beneficial to understand how the different control mechanisms compare at targeting particular deficits to induce recovery and also to understand if it is beneficial to target single or multi-joints based on the deficits.
Physiological outcomes using RD have been measured through self-report exertion (Borg), heart rate, and measures of oxygen consumption. In people with sub-acute stroke, the perceived exertion was above fairly light exertion based on self-reported measures (Høyer et al., 2020). One of the studies reported less energy expenditure with RD (Ekso) training (Mehrholz et al., 2018). The intensity may be varied by varying the RD control settings, resulting in increased user effort. Future studies need to evaluate the effect of RD control settings on user effort and exertion. During the sub-acute stages of recovery, where the user might need maximum assistance, RDs might need to provide more assistance across the joints to facilitate the user to perform higher quality or correct gait. This in turn might reduce the perceived exertion, while allowing the user to perform more consistent and accurate repetitive steps. In addition, exertion, and cardiorespiratory load depend on various other factors such as level of assistance, various robotic control mechanisms, and type of robot. Current research is sparse on analyzing the exertion and cardio-respiratory strain due to RD training in both acute and chronic stroke. Future research is needed to address this shortcoming. Analyzing the cardio-respiratory strain at various assistance levels across different RDs is necessary to truly understand the effect of RD assistance on intensity (exertion and cardio-respiratory load).
Research on multiple RDs from initial studies suggests improved step length, gait symmetry, and gait kinematic after RD use (Monticone et al., 2013; Murray et al., 2014, 2015; Buesing et al., 2015). Similarly, loading characteristics and loading symmetry improved with RD training (Rojek et al., 2020); though it has also been observed that a single leg version of an RD resulted in improved step length with reduced symmetry. Though randomized controlled trials are needed to verify these results.
Preliminary research on EMG activity has shown improved muscle activity and muscle synergies with improved biomechanical and functional parameters in full-scale RDs; though significant differences were observed across studies (Li et al., 2015; Tan et al., 2018, 2020; Lee et al., 2019; Zhang et al., 2020; Infarinato et al., 2021; Kotov et al., 2021; Zhu et al., 2021). Though initial evidence shows improved biomechanical characteristics with RD use, there is still a need to understand RD impact on different biomechanical metrics, such as spatial-temporal characteristics, loading characteristics, EMG, and kinematics. The impact of the number of joint actuations, actuation assistance, as well as the difference between the actuation of both legs and single leg, needs to be studied in order to completely understand the effects of RDs on biomechanical characteristics. In addition, the effect of RDs on the different compensatory mechanisms needs to be studied. What is more, and warrants further study, is the fact that each person might have varying deficits at varying joints and may require customized therapy. This may require assistance or correction at various joints at varying levels. Hence, it is important to not only understand the effect of RDs on the population or on broad measures of ABI, but also their effects with regard to deficit levels. This will help us develop better rehabilitation RDs by customizing interventions.
Initial research on upstream mechanisms showed that CSE, CME, SMI, frontoparietal effective connectivity (FPEC) was better after RD training than conventional gait training in the preliminary analysis (Calabrò et al., 2018; Jayaraman et al., 2019). RD induced a more evident remodulation of SMI between the hemispheres as compared to conventional training. Node strength (NS) over the contralesional motor cortex and ipsilesional prefrontal cortex after exoskeleton training improved and displayed a modification of connectivity after RD assisted gait (Molteni et al., 2020). Preliminary analysis shows that RD is effective in inducing positive cortical re-organization and improved CSE (Poydasheva et al., 2016; Saenko et al., 2016; Calabrò et al., 2018; Jayaraman et al., 2019; Molteni et al., 2020). Understanding the relationship between lesion, and its effects on cortical activity changes as well as recovery due to RD and its effects on cortical activity changes and evaluating its relationship to biomechanical characteristics will help us optimize rehabilitation.
4.1. Future directions
The mechanical and software (control) capabilities of each RD are widespread and still evolving with the technology. RDs can be classified as deficit-targeted or function-targeted devices. Deficit-targeted devices focus on a single joint, while function-targeted devices target gait and balance mechanisms. Both kinds of RDs have shown improvement in ambulation, but the comparative effectiveness of such devices needs to be more comprehensively evaluated. It is unclear whether it is more beneficial to use single joint targeted devices or to target gait and balance function, both overall and/or under which circumstances. In addition, the control strategies vary between RDs. Thus, the training could be altered to provide varying levels of assistance (complete to no assistance) or resistance or trajectory guidance. The effect of these varying control strategies on the different stages of recovery also need to be analyzed. In addition, more comprehensive analysis of the length of training, the amount of training (number of sessions, number of steps), intensity, the effect of assistance, and how and when to reduce assistance and how this affects recovery is needed in order to guide the future trajectory of ABI rehabilitation. In order to answer such questions, comprehensive evaluations of various devices need to be compiled, and their effects on downstream and upstream metrics need to be evaluated.
Research has not addressed the effect of age, time since injury, or patient level of injury on recovery after using RDs. Since recovery plateaus during the chronic stages of ABI, it is important to understand the effects of time since injury. Neurological recovery might be influenced by age, especially with younger individuals having different sequelae influenced by their development; resulting in different patterns of recovery. The severity and location of injury (cortical or sub-cortical, localized vs. diffused, various ROIs) might influence the trajectory of recovery. Injury characteristics might influence the functional connectivity related to gait and balance mechanisms, resulting in varying biomechanical and physiological deficits and recovery. All these factors warrant research in their own right and are a necessary prerequisite for a more comprehensive understanding of post-ABI recovery.
RD research is still evolving. We have provided foundation of the preliminary evidence for over ground robotic devices in order to build more rigorously controlled research study as well as RCTs. The review also lays out the systematic framework to evaluate these devices based on diagnosis, domain (functional, biomechanical and neurological) and based on stage of recovery. We need to first evaluate the RDs for each pathology in each domain separately throughout the different stages of recovery. This will help us determine responders and non-responders in various diseases, domain, and recovery phases. This will help us narrow the devices for translation into clinical practice.
Author contributions
SP, CB, and EL assisted with sorting the manuscript based on the inclusion/exclusion criteria. KK validated the selected articles based on the inclusion/exclusion criteria and drafted the manuscript. KN and SS reviewed the manuscript. All authors contributed to the article and approved the submitted version.
Conflict of interest
The authors declare that the research was conducted in the absence of any commercial or financial relationships that could be construed as a potential conflict of interest.
Publisher's note
All claims expressed in this article are solely those of the authors and do not necessarily represent those of their affiliated organizations, or those of the publisher, the editors and the reviewers. Any product that may be evaluated in this article, or claim that may be made by its manufacturer, is not guaranteed or endorsed by the publisher.
References
Alias, N. A., Huq, M. S., Ibrahim, B. S. K. K., and Omar, R. (2017). The efficacy of state of the art overground gait rehabilitation robotics: a bird's eye view. Proc. Comput. Sci. 105, 365–370. doi: 10.1016/j.procs.2017.01.235
Alliance BI (2023). TBI Statistics. Available at: https://biact.org/understanding-brain-injury/facts-statistics/ (accessed April 5, 2023).
Androwis, G. J., Kwasnica, M. A., Niewrzol, P., Popok, P., Fakhoury, F. N., Sandroff, B. M., et al. (2019). “Mobility and cognitive improvements resulted from overground robotic exoskeleton gait-training in persons with MS,” in Proceedings of the Annual International Conference of the IEEE Engineering in Medicine and Biology Society, EMBS, 4454–4457. doi: 10.1109/EMBC.2019.8857029
Awad, L. N., Esquenazi, A., Francisco, G. E., Nolan, K. J., and Jayaraman, A. (2020). The ReWalk ReStoreTM soft robotic exosuit: a multi-site clinical trial of the safety, reliability, and feasibility of exosuit-augmented post-stroke gait rehabilitation. J. Neuroeng. Rehabil. 17, 1–11. doi: 10.1186/s12984-020-00702-5
Bayón, C., Lerma, S., Ramírez, O., Serrano, J. I., Castillo, M. D. D., Raya, R., et al. (2016). Locomotor training through a novel robotic platform for gait rehabilitation in pediatric population: short report. J. Neuroeng. Rehabil. 13, 1–6. doi: 10.1186/s12984-016-0206-x
Bayón, C., Martín-Lorenzo, T., Moral-Saiz, B., Ramírez, Ó., Pérez-Somarriba, Á., Lerma-Lara, S., et al. (2018). A robot-based gait training therapy for pediatric population with cerebral palsy: goal setting, proposal and preliminary clinical implementation. J. Neuroeng. Rehabil. 15, 69. doi: 10.1186/s12984-018-0412-9
BIA (2021). ABI vs. TBI: What is the Difference? Available online at: https://www.biausa.org/brain-injury/about-brain-injury/nbiic/what-is-the-difference-between-an-acquired-brain-injury-and-a-traumatic-brain-injury (accessed March 24, 2021).
Bortole, M., Venkatakrishnan, A., Zhu, F., Moreno, J. C., Francisco, G. E., Pons, J. L., et al. (2015). The H2 robotic exoskeleton for gait rehabilitation after stroke: early findings from a clinical study Wearable robotics in clinical testing. J. Neuroeng. Rehabil. 12, 1–14. doi: 10.1186/s12984-015-0048-y
Bruni, M. F., Melegari, C., De Cola, M. C., Bramanti, A., Bramanti, P., Calabrò, R. S., et al. (2018). What does best evidence tell us about robotic gait rehabilitation in stroke patients: a systematic review and meta-analysis. J. Clin. Neurosci. 48, 11–17. doi: 10.1016/j.jocn.2017.10.048
Buesing, C., Fisch, G., O'Donnell, M., Shahidi, I., Thomas, L., Mummidisetty, C. K., et al. (2015). Effects of a wearable exoskeleton stride management assist system (SMA®) on spatiotemporal gait characteristics in individuals after stroke: a randomized controlled trial. J. Neuroeng. Rehabil. 12, 69. doi: 10.1186/s12984-015-0062-0
Bulea, T. C., Lerner, Z. F., and Damiano, D. L. (2018). “Repeatability of EMG activity during exoskeleton assisted walking in children with cerebral palsy: implications for real time adaptable control,” in Proceedings of the Annual International Conference of the IEEE Engineering in Medicine and Biology Society, EMBS (Institute of Electrical and Electronics Engineers Inc.), 2801–2804. doi: 10.1109/EMBC.2018.8512799
Calabrò, R. S., Naro, A., Russo, M., Bramanti, P., Carioti, L., Balletta, T., et al. (2018). Shaping neuroplasticity by using powered exoskeletons in patients with stroke: a randomized clinical trial. J. Neuroeng. Rehabil. 15, 35. doi: 10.1186/s12984-018-0377-8
Canela, M., del Ama, A. J., and Pons, J. L. (2013). Design of a pediatric exoskeleton for the rehabilitation of the physical disabilities caused by cerebral palsy. Biosyst. Biorobot. 1, 255–258. doi: 10.1007/978-3-642-34546-3_40
CDC Stroke Statistics (2020). Available online at: https://www.cdc.gov/stroke/statistics_maps.htm (accessed March 7, 2020).
Centers for Disease Control Prevention (2016). Data and Statistics for Cerebral Palsy. 1–6. Available online at: https://www.cdc.gov/ncbddd/index.html (accessed August 6, 2022).
Cooke, E. V., Mares, K., Clark, A., Tallis, R. C., and Pomeroy, V. M. (2010). The effects of increased dose of exercise-based therapies to enhance motor recovery after stroke: a systematic review and meta-analysis. BMC Med. 8, 1–13. doi: 10.1186/1741-7015-8-60
De Luca, R., Maresca, G., Balletta, T., Cannavò, A., Leonardi, S., Latella, D., et al. (2020). Does overground robotic gait training improve non-motor outcomes in patients with chronic stroke? Findings from a pilot study. J. Clin. Neurosci. 81, 240–245. doi: 10.1016/j.jocn.2020.09.070
Dijkers, M. P., Akers, K. G., Dieffenbach, S., and Galen, S. S. (2021). Systematic reviews of clinical benefits of exoskeleton use for gait and mobility in neurologic disorders: a tertiary study. Arch. Phys. Med. Rehabil. 102, 300–313. doi: 10.1016/j.apmr.2019.01.025
Dollar, A. M., and Herr, H. (2008). Lower extremity exoskeletons and active orthoses: challenges and state-of-the-art. IEEE Trans. Robot. 24, 144–158. doi: 10.1109/TRO.2008.915453
Dubin, A. (2014). Gait. The role of the ankle and foot in walking. Med. Clin. North Am. 98, 205–211. doi: 10.1016/j.mcna.2013.10.002
Esquenazi, A., Talaty, M., and Jayaraman, A. (2017). Powered exoskeletons for walking assistance in persons with central nervous system injuries: a narrative review. PMR 9, 46–62. doi: 10.1016/j.pmrj.2016.07.534
Fang, Y., Orekhov, G., and Lerner, Z. F. (2020). Adaptive ankle exoskeleton gait training demonstrates acute neuromuscular and spatiotemporal benefits for individuals with cerebral palsy: a pilot study. Gait Posture. 95, 256–263. doi: 10.1016/j.gaitpost.2020.11.005
Federici, S., Meloni, F., and Bracalenti, M. (2016). Gait rehabilitation with exoskeletons. Handb. Hum. Motion 2016, 1–38. doi: 10.1007/978-3-319-30808-1_80-1
Federici, S., Meloni, F., Bracalenti, M., and Filippis, M. L. (2015). The effectiveness of powered, active lower limb exoskeletons in neurorehabilitation: a systematic review. NeuroRehabilitation 37, 321–340. doi: 10.3233/NRE-151265
Friedman, P. J. (1990). Gait recovery after hemiplegic stroke. Disabil. Rehabil. 12, 119–122. doi: 10.3109/03790799009166265
Goffredo, M., Guanziroli, E., Pournajaf, S., Gaffuri, M., Gasperini, G., Filoni, S., et al. (2019). Overground Wearable powered exoskeleton for gait training in subacute stroke subjects: clinical and gait assessments. Eur. J. Phys. Rehabil. Med. 55, 710–721. doi: 10.23736/S1973-9087.19.05574-6
Haufe, F. L., Schmidt, K., Duarte, J. E., Wolf, P., Riener, R., Xiloyannis, M., et al. (2020). Activity-based training with the Myosuit: a safety and feasibility study across diverse gait disorders. J. Neuroeng. Rehabil. 17, 1–11. doi: 10.1186/s12984-020-00765-4
Hill, D., Holloway, C. S., Ramirez, D. Z. M., Smitham, P., and Pappas, Y. (2017). What are user perspectives of exoskeleton technology? A literature review. Int. J. Technol. Assess. Health Care 33, 160–167. doi: 10.1017/S0266462317000460
Hornby, T. G., Moore, J. L., Lovell, L., and Roth, E. J. (2016). Influence of skill and exercise training parameters on locomotor recovery during stroke rehabilitation. Curr. Opin. Neurol. 29, 677–683. doi: 10.1097/WCO.0000000000000397
Høyer, E., Opheim, A., and Jørgensen, V. (2020). Implementing the exoskeleton Ekso GT TM for gait rehabilitation in a stroke unit—feasibility, functional benefits and patient experiences. Disabil. Rehabil. Assist. Technol. 17, 473–479. doi: 10.1080/17483107.2020.1800110
Iandolo, R., Marini, F., Semprini, M., Laffranchi, M., Mugnosso, M., Cherif, A., et al. (2019). Perspectives and challenges in robotic neurorehabilitation. Appl. Sci. 9, 3183. doi: 10.3390/app9153183
Infarinato, F., Romano, P., Goffredo, M., Ottaviani, M., Galafate, D., Gison, A., et al. (2021). Functional gait recovery after a combination of conventional therapy and overground robot-assisted gait training is not associated with significant changes in muscle activation pattern: an EMG preliminary study on subjects subacute post stroke. Brain Sci. 11, 448. doi: 10.3390/brainsci11040448
Jayaraman, A., Burt, S., and Rymer, W. Z. (2017). Use of lower-limb robotics to enhance practice and participation in individuals with neurological conditions. Pediatr. Phys. Therapy 29, S48–S56. doi: 10.1097/PEP.0000000000000379
Jayaraman, A., O'Brien, M. K., Madhavan, S., Mummidisetty, C. K., Roth, H. R., Hohl, K., et al. (2019). Stride management assist exoskeleton vs functional gait training in stroke: a randomized trial. Neurology 92, E263–E273. doi: 10.1212/WNL.0000000000006782
Jyräkoski, T., Merilampi, S., Puustinen, J., and Kärki, A. (2021). Over-ground robotic lower limb exoskeleton in neurological gait rehabilitation: user experiences and effects on walking ability. Technol. Disabil. 33, 53–63. doi: 10.3233/TAD-200284
Karunakaran, K. K., Ehrenberg, N., Cheng, J., Bentley, K., and Nolan, K. J. (2020a). Kinetic gait changes after robotic exoskeleton training in adolescents and young adults with acquired brain injury. Appl. Bionics Biomech. 2020. doi: 10.1155/2020/8845772
Karunakaran, K. K., Ehrenberg, N., Cheng, J., and Nolan, K. J. (2019). “Effects of robotic exoskeleton gait training on an adolescent with brain injury,” in Proceedings of the Annual International Conference of the IEEE Engineering in Medicine and Biology Society, EMBS 4445–4448. doi: 10.1109/EMBC.2019.8856787
Karunakaran, K. K., Gute, S., Ames, G. R., Chervin, K., Dandola, C. M., Nolan, K. J., et al. (2021). Effect of robotic exoskeleton gait training during acute stroke on functional ambulation. NeuroRehabilitation Preprint 2021, 1–11. doi: 10.3233/NRE-210010
Karunakaran, K. K., Nisenson, D. M., and Nolan, K. J. (2020b). “Alterations in cortical activity due to robotic gait training in traumatic brain injury,” in Proceedings of the Annual International Conference of the IEEE Engineering in Medicine and Biology Society, EMBS (Institute of Electrical and Electronics Engineers Inc.) 2020, 3224–3227. doi: 10.1109/EMBC44109.2020.9175764
Kawamoto, H., Kamibayashi, K., Nakata, Y., Yamawaki, K., Ariyasu, R., Sankai, Y., et al. (2013). Pilot study of locomotion improvement using hybrid assistive limb in chronic stroke patients. BMC Neurol. 13, 141. doi: 10.1186/1471-2377-13-141
Kemu, M. (2010). Kinetics and kinematics of loading response in stroke patients (a review article). Ann. King Edward Med. Univ. 14, 143. doi: 10.21649/akemu.v14i4.6
Kerrigan, D. C., Frates, E. P., Rogan, S., and Riley, P. O. (2000). Hip hiking and circumduction: quantitative definitions. Am. J. Phys. Med. Rehabil. 79, 247–252. doi: 10.1097/00002060-200005000-00006
Kotov, S. V., Isakova, E. V., Lijdvoy, V. Y., Petrushanskaya, K. A., Pismennaya, E. V., Romanova, M. V., et al. (2021). Robotic restoration of gait function in patients in the early recovery period of stroke. Neurosci. Behav. Physiol. 51, 583–589. doi: 10.1007/s11055-021-01109-y
Kovalenko, A. P., Rodionov, A. S., Kremlyov, D. I., Averkiev, D. V., Lobzin, V. Y., Guseva, A. V., et al. (2021). Gait rehabilitation in patients with spastic hemiparesis: new opportunities. Neurol. Neuropsychiatry, Psychosom. 13, 56–64. doi: 10.14412/2074-2711-2021-2-56-64
Kozlowski, A. J., Fabian, M., Lad, D., and Delgado, A. D. (2017). Feasibility and safety of a powered exoskeleton for assisted walking for persons with multiple sclerosis: a single-group preliminary study. Arch. Phys. Med. Rehabil. 98, 1300–1307. doi: 10.1016/j.apmr.2017.02.010
Kuroda, M., Nakagawa, S., Mutsuzaki, H., Mataki, Y., Yoshikawa, K., Takahashi, K., et al. (2020). Robot-assisted gait training using a very small-sized Hybrid Assistive Limb® for pediatric cerebral palsy: a case report. Brain Dev. 42, 468–472. doi: 10.1016/j.braindev.2019.12.009
Kwakkel, G., Kollen, B., and Lindeman, E. (2004). Understanding the pattern of functional recovery after stroke: facts and theories. Restor. Neurol. Neurosci. 22, 281–299.
Lab SRA (2023). Rehab Measures. Available online at: https://www.sralab.org/rehabilitation-measures (accessed August 6, 2022).
Langhorne, P., Coupar, F., and Pollock, A. (2009). Motor recovery after stroke: a systematic review. Lancet Neurol. 8, 741–754. doi: 10.1016/S1474-4422(09)70150-4
Lee, H., Ferguson, P. W., and Rosen, J. (2020). Lower limb exoskeleton systems-overview. Wearable Robot. Syst. Appl. 2020, 207–229. doi: 10.1016/B978-0-12-814659-0.00011-4
Lee, H.-J., Lee, S-H., Seo, K., Lee, M., Chang, W. H., Choi, B.-O., et al. (2019). Training for walking efficiency with a wearable hip-assist robot in patients with stroke a pilot randomized controlled trial. Stroke 50, 3545–3552. doi: 10.1161/STROKEAHA.119.025950
Lefeber, N., De Keersmaecker, E., Troch, M., Lafosse, C., de Geus, B., Kerckhofs, E., et al. (2020). Robot-assisted overground walking: physiological responses and perceived exertion in nonambulatory stroke survivors. IEEE Robot. Autom. Mag. 27, 22–31. doi: 10.1109/MRA.2019.2939212
Lefeber, N., Swinnen, E., and Kerckhofs, E. (2017). The immediate effects of robot-assistance on energy consumption and cardiorespiratory load during walking compared to walking without robot-assistance: a systematic review. Disabil. Rehabil. Assist. Technol. 12, 657–671. doi: 10.1080/17483107.2016.1235620
Lerner, Z. F., Damiano, D. L., and Bulea, T. C. (2017b). A lower-extremity exoskeleton improves knee extension in children with crouch gait from cerebral palsy. Sci. Transl. Med. 9, aam9145. doi: 10.1126/scitranslmed.aam9145
Lerner, Z. F., Damiano, D. L., Park, H. S., Gravunder, A. J., and Bulea, T. C. A. (2017a). Robotic exoskeleton for treatment of crouch gait in children with cerebral palsy: design and initial application. IEEE Trans. Neural Syst. Rehabil. Eng. 25, 650–659. doi: 10.1109/TNSRE.2016.2595501
Li, D.-X., Zha, F.-B., Long, J.-J., Liu, F., Cao, J., Wang, Y.-L., et al. (2021). Effect of robot assisted gait training on motor and walking function in patients with subacute stroke: a random controlled study. J. Stroke Cerebrovasc. Dis. 30, 105807. doi: 10.1016/j.jstrokecerebrovasdis.2021.105807
Li, L., Ding, L., Chen, N., Mao, Y., and Huang, D. (2015). Improved walking ability with wearable robot-assisted training in patients suffering chronic stroke. Biomed. Mater. Eng. 26, S329–S340. doi: 10.3233/BME-151320
Louie, D. R., and Eng, J. J. (2016). Powered robotic exoskeletons in post-stroke rehabilitation of gait: a scoping review. J. Neuroeng. Rehabil. 13, 1–10. doi: 10.1186/s12984-016-0162-5
Mehrholz, J., and Pohl, M. (2012). Electromechanical-assisted gait training after stroke: a systematic review comparing end-effector and exoskeleton devices. J. Rehabil. Med. 44, 193–199. doi: 10.2340/16501977-0943
Mehrholz, J., Pohl, M., Kugler, J., and Elsner, B. (2018). OriginalArbeit: Verbesserung der Gehfähigkeit nach Schlaganfall. Dtsch. Arztebl. Int. 115, 639–645. doi: 10.3238/arztebl.2018.0639
Menon, D. K., and Bryant, C. (2019). Time for change in acquired brain injury. Lancet Neurol. 18, 28. doi: 10.1016/S1474-4422(18)30463-0
Mizukami, M., Yoshikawa, K., Kawamoto, H., Sano, A., Koseki, K., Asakwa, Y., et al. (2016). Disability and rehabilitation: assistive technology gait training of subacute stroke patients using a hybrid assistive limb: a pilot study. Disabil. Rehabil. Assist. Technol. 12, 197–204. doi: 10.3109/17483107.2015.1129455
Moher, D., Liberati, A., Tetzlaff, J., Altman, D. G., and PRISMA Group (2009). Preferred reporting items for systematic reviews and meta-analyses: the PRISMA statement. PLoS Med. 6, 1–6. doi: 10.1371/journal.pmed.1000097
Molteni, F., Formaggio, E., Bosco, A., Guanziroli, E., Piccione, F., Masiero, S., et al. (2020). Brain connectivity modulation after exoskeleton-assisted gait in chronic hemiplegic stroke survivors: a pilot study. Am. J. Phys. Med. Rehabil. 99, 694–700. doi: 10.1097/PHM.0000000000001395
Molteni, F., Gasperini, G., Cannaviello, G., and Guanziroli, E. (2018). Exoskeleton and end-effector robots for upper and lower limbs rehabilitation: narrative review. PMR 10, S174–S188. doi: 10.1016/j.pmrj.2018.06.005
Molteni, F., Gasperini, G., Gaffuri, M., Colombo, M., Giovanzana, C., Lorenzon, C., et al. (2017). Wearable robotic exoskeleton for overground gait training in sub-acute and chronic hemiparetic stroke patients: preliminary results. Eur. J. Phys. Rehabil. Med. 53, 676–684. doi: 10.23736/S1973-9087.17.04591-9
Molteni, F., Guanziroli, E., Goffredo, M., Calabrò, R. S., Pournajaf, S., Gaffuri, M., et al. (2021). Gait recovery with an overground powered exoskeleton: a randomized controlled trial on subacute stroke subjects. Brain Sci. 11, 1–14. doi: 10.3390/brainsci11010104
Monticone, M., Ambrosini, E., Ferrante, S., and Colombo, R. (2013). 'Regent Suit' training improves recovery of motor and daily living activities in subjects with subacute stroke: a randomized controlled trial. Clin. Rehabil. 27, 792–802. doi: 10.1177/0269215513478228
Moucheboeuf, G., Griffier, R., Gasq, D., Glize, B., Bouyer, L., Dehail, P., et al. (2020). Effects of robotic gait training after stroke: a meta-analysis. Ann. Phys. Rehabil. Med. 63, 518–534. doi: 10.1016/j.rehab.2020.02.008
Murray, S. A., Ha, K. H., and Goldfarb, M. (2014). “An assistive controller for a lower-limb exoskeleton for rehabilitation after stroke, and preliminary assessment thereof,” in 2014 36th Annual International Conference of the IEEE Engineering in Medicine and Biology Society, EMBC 2014 (Institute of Electrical and Electronics Engineers Inc.), 4083–4086. doi: 10.1109/EMBC.2014.6944521
Murray, S. A., Ha, K. H., Hartigan, C., and Goldfarb, M. (2015). An assistive control approach for a lower-limb exoskeleton to facilitate recovery of walking following stroke. IEEE Trans. Neural Syst. Rehabil. Eng. 23, 441–449. doi: 10.1109/TNSRE.2014.2346193
Neural Plasticity After Acquired Brain Injury (2022). Evidence from Functional Neuroimaging—Rutgers University Libraries. Available online at: https://rutgers.primo.exlibrisgroup.com/discovery/fulldisplay?docid=cdi_proquest_miscellaneous_850563284andcontext=PCandvid=01RUT_INST:01RUTandlang=enandsearch_scope=MyInst_and_CI_2andadaptor=PrimoCentralandtab=Everything_except_researchandquery=any,contains,NeuralPlasticityAfterAcquiredBrainInjury:EvidencefromFunctionalNeuroimagingandmode=basic (accessed July 1, 2022).
Nilsson, A., Vreede, K. S., Häglund, V., Kawamoto, H., Sankai, Y., Borg, J., et al. (2014). Gait training early after stroke with a new exoskeleton-the hybrid assistive limb: a study of safety and feasibility. JNER J. NeuroEng. Rehabil. 11, 1–11. doi: 10.1186/1743-0003-11-92
Nolan, K. J., Karunakaran, K. K., Chervin, K., Monfett, M. R., Bapineedu, R. K., Jasey, N. N., et al. (2020). Robotic exoskeleton gait training during acute stroke inpatient rehabilitation. Front. Neurorobot. 14, 581815. doi: 10.3389/fnbot.2020.581815
Nolan, K. J., Karunakaran, K. K., Ehrenberg, N., and Kesten, A. G. (2018). Robotic exoskeleton gait training for inpatient rehabilitation in a young adult with traumatic brain injury. Proc. Annu. Int. Conf. IEEE Eng. Med. Biol. Soc. EMBS 2018, 2809–2812. doi: 10.1109/EMBC.2018.8512745
Panizzolo, F. A., Cimino, S., Pettenello, E., Belfiore, A., Petrone, N., Marcolin, G., et al. (2021). Effect of a passive hip exoskeleton on walking distance in neurological patients. Assist. Technol. 34, 527–532. doi: 10.1080/10400435.2021.1880494
Partridge, C., Mackenzie, M., Edwards, S., Reid, A., Jayawardena, S., Guck, N., et al. (2000). Is dosage of physiotherapy a critical factor in deciding patterns of recovery from stroke: a pragmatic randomized controlled trial. Physiother. Res. Int. 5, 230–240. doi: 10.1002/pri.203
Patané, F., Rossi, S., Del Sette, F., Taborri, J., and Cappa, P. (2017). WAKE-Up exoskeleton to assist children with cerebral palsy: design and preliminary evaluation in level walking. IEEE Trans. Neural Syst. Rehabil. Eng. 25, 906–916. doi: 10.1109/TNSRE.2017.2651404
Perry, J., and Burnfield, J. M. (2010). Gait Analysis: Normal and Pathological Function. California: Slack.
Peurala, S. H., Airaksinen, O., Huuskonen, P., Jäkälä, P., Juhakoski, M., Sandell, K., et al. (2009). Effects of intensive therapy using gait trainer or floor walking exercises early after stroke. J. Rehabil. Med. 41, 166–173. doi: 10.2340/16501977-0304
Pinto-Fernandez, D., Torricelli, D., Sanchez-Villamanan, M. D. C., Aller, F., Mombaur, K., Conti, R., et al. (2020). Performance evaluation of lower limb exoskeletons: a systematic review. IEEE Trans. Neural Syst. Rehabil. Eng. 28, 1573–1583. doi: 10.1109/TNSRE.2020.2989481
Poli, P., Morone, G., Rosati, G., and Masiero, S. (2013). Robotic technologies and rehabilitation: new tools for stroke patients' therapy. BioMed Res. Int. 2013. doi: 10.1155/2013/153872
Postol, N., Marquez, J., Spartalis, S., Bivard, A., and Spratt, N. J. (2019). Do powered over-ground lower limb robotic exoskeletons affect outcomes in the rehabilitation of people with acquired brain injury? Disabil. Rehabil. Assist. Technol. 14, 764–775. doi: 10.1080/17483107.2018.1499137
Pournajaf, S., Calabrò, R. S., Naro, A., Goffredo, M., Aprile, I., Tamburella, F., et al. (2019). Stroke gait rehabilitation: a comparison of end-effector, overground exoskeleton, and conventional gait training. Appl. Sci. 9, 2627. doi: 10.3390/app9132627
Poydasheva, A. G., Saenko, I. A., Chervyakov, A. V., Zmeykina, E. A., Lukmanov, R. H., Chernikova, L. A., et al. (2016). Evaluation of changes in the cortical gait control in post-stroke patients induced by the use of the “Regent” soft exoskeleton complex (SEC) by navigated transcranial magnetic stimulation. Hum. Physiol. 42, 252–257. doi: 10.1134/S0362119716030142
Rojek, A., Mika, A., Oleksy, Ł., Stolarczyk, A., and Kielnar, R. (2020). Effects of exoskeleton gait training on balance, load distribution, and functional status in stroke: a randomized controlled trial. Front. Neurol. 10, 1344. doi: 10.3389/fneur.2019.01344
Rudberg, A. S., Berge, E., Laska, A. C., Jutterström, S., Näsman, P., Sunnerhagen, K. S., et al. (2021). Stroke survivors' priorities for research related to life after stroke. Top. Stroke Rehabil. 28, 1–6. doi: 10.1080/10749357.2020.1789829
Saenko, I. V., Morozova, S. N., Zmeykina, E. A., Konovalov, R. N., Chervyakov, A. V., Poydasheva, A. G., et al. (2016). Changes in functional connectivity of motor zones in the course of treatment with a regent multimodal complex exoskeleton in neurorehabilitation of post-stroke patients. Hum. Physiol. 42, 54–60. doi: 10.1134/S036211971601014X
Sale, P., Franceschini, M., Waldner, A., and Hesse, S. (2021). Use of the Robot Assisted Gait Therapy in Rehabilitation of Patients With Stroke and Spinal Cord Injury. Available online at: https://www.researchgate.net/publication/224867329_Use_of_the_robot_assisted_gait_therapy_in_rehabilitation_of_patients_with_stroke_and_spinal_cord_injury (accessed December 21, 2021).
Schröder, J., Kenis, S., Goos, K., Truijen, S., and Saeys, W. (2019). The effects of exoskeleton-assisted overground gait training in chronic stroke—a pilot study. Biosyst. Biorobot. 21, 464–468. doi: 10.1007/978-3-030-01845-0_93
Schwartz, I., and Meiner, Z. (2015). Robotic-assisted gait training in neurological patients: who may benefit? Ann. Biomed. Eng. 43, 1260–1269. doi: 10.1007/s10439-015-1283-x
Sheffler, L. R., and Chae, J. (2015). Hemiparetic gait. Phys. Med. Rehabil. Clin. North Am. 26, 611–623. doi: 10.1016/j.pmr.2015.06.006
Shi, D., Zhang, W., Zhang, W., and Ding, X. A. (2019). Review on lower limb rehabilitation exoskeleton robots. Chinese J. Mech. Eng. 32, 1–11. doi: 10.1186/s10033-019-0389-8
Swank, C., Galvan, C., DiPasquale, J., Callender, L., Sikka, S., Driver, S., et al. (2020a). Lessons learned from robotic gait training during rehabilitation: Therapeutic and medical severity considerations over 3 years. Technol. Disabil. 32, 103–110. doi: 10.3233/TAD-190248
Swank, C., Trammell, M., Bennett, M., Ochoa, C., Callender, L., Sikka, S., et al. (2020b). The utilization of an overground robotic exoskeleton for gait training during inpatient rehabilitation—single-center retrospective findings. Int. J. Rehabil. Res. 43, 206–213. doi: 10.1097/MRR.0000000000000409
Taki, S., Imura, T., Iwamoto, Y., Imada, N., Tanaka, R., Araki, H., et al. (2020). Effects of exoskeletal lower limb robot training on the activities of daily living in stroke patients: retrospective pre-post comparison using propensity score matched analysis. J. Stroke Cerebrovasc. Dis. 29, 105176. doi: 10.1016/j.jstrokecerebrovasdis.2020.105176
Tan, C. K., Kadone, H., Watanabe, H., Marushima, A., Hada, Y., Yamazaki, M., et al. (2020). Differences in muscle synergy symmetry between subacute post-stroke patients with bioelectrically-controlled exoskeleton gait training and conventional gait training. Front. Bioeng. Biotechnol. 8, 770. doi: 10.3389/fbioe.2020.00770
Tan, C. K., Kadone, H., Watanabe, H., Marushima, A., Yamazaki, M., Sankai, Y., et al. (2018). Lateral symmetry of synergies in Lower Limb muscles of acute post-stroke patients after robotic intervention. Front. Neurosci. 12, 276. doi: 10.3389/fnins.2018.00276
Tanaka, H., Nankaku, M., Nishikawa, T., Yonezawa, H., Mori, H., Kikuchi, T., et al. (2019). A follow-up study of the effect of training using the hybrid assistive limb on gait ability in chronic stroke patients. Top. Stroke Rehabil. 26, 491–496. doi: 10.1080/10749357.2019.1640001
Ueno, T., Watanabe, H., Kawamoto, H., Shimizu, Y., Endo, A., Shimizu, T., et al. (2019). Feasibility and safety of Robot Suit HAL treatment for adolescents and adults with cerebral palsy. J. Clin. Neurosci. 68, 101–104. doi: 10.1016/j.jocn.2019.07.026
Viteckova, S., Kutilek, P., and Jirina, M. (2013). Wearable lower limb robotics: a review. Biocybern. Biomed. Eng. 33, 96–105. doi: 10.1016/j.bbe.2013.03.005
Wade, D. T., and Hewer, R. L. (1987). Functional abilities after stroke: measurement, natural history and prognosis. J. Neurol. Neurosurg. Psychiatry 50, 177–182. doi: 10.1136/jnnp.50.2.177
Wall, A., Borg, J., and Palmcrantz, S. (2015). Clinical application of the hybrid assistive limb (Hal) for gait training—a systematic review. Front. Syst. Neurosci. 9, 48. doi: 10.3389/fnsys.2015.00048
Watanabe, H., Goto, R., Tanaka, N., Matsumura, A., and Yanagi, H. (2017). Effects of gait training using the Hybrid Assistive Limb® in recovery-phase stroke patients: a 2-month follow-up, randomized, controlled study. NeuroRehabilitation 40, 363–367. doi: 10.3233/NRE-161424
Weber, L. M., and Stein, J. (2018). The use of robots in stroke rehabilitation: a narrative review. NeuroRehabilitation 43, 99–110. doi: 10.3233/NRE-172408
Williams, G., Morris, M. E., Schache, A., and McCrory, P. R. (2009). Incidence of gait abnormalities after traumatic brain injury. Arch. Phys. Med. Rehabil. 90, 587–593. doi: 10.1016/j.apmr.2008.10.013
Winter, D. A. (2009). Biomechanics and Motor Control of Human Movement: Fourth Edition. New York, NY: John Wiley and Sons. doi: 10.1002/9780470549148
Yan, T., Cempini, M., Oddo, C. M., and Vitiello, N. (2015). Review of assistive strategies in powered lower-limb orthoses and exoskeletons. Rob. Auton. Syst. 64, 120–136. doi: 10.1016/j.robot.2014.09.032
Yeung, L.-F., Lau, C. C. Y., Lai, C. W. K., Soo, Y. O. Y., Chan, M.-L., Tong, R. K. Y., et al. (2021). Effects of wearable ankle robotics for stair and over-ground training on sub-acute stroke: a randomized controlled trial. J. NeuroEngineering Rehabil. 18, 1–10. doi: 10.1186/s12984-021-00814-6
Yeung, L.-F., Ockenfeld, C., Pang, M.-K., Wai, H.-W., Soo, O.-Y., Li, S.-W., et al. (2018). Randomized controlled trial of robot-assisted gait training with dorsiflexion assistance on chronic stroke patients wearing ankle-foot-orthosis. J. Neuroeng. Rehabil. 15, 1–12. doi: 10.1186/s12984-018-0394-7
Yoshimoto, T., Shimizu, I., and Hiroi, Y. (2016). Sustained effects of once-a-week gait training with hybrid assistive limb for rehabilitation in chronic stroke: case study. J. Phys. Therapy Sci. 28, 2684. doi: 10.1589/jpts.28.2684
Yoshimoto, T., Shimizu, I., Hiroi, Y., Kawaki, M., Sato, D., Nagasawa, M., et al. (2015). Feasibility and efficacy of high-speed gait training with a voluntary driven exoskeleton robot for gait and balance dysfunction in patients with chronic stroke: nonrandomized pilot study with concurrent control. Int. J. Rehabil. Res. 38, 338–343. doi: 10.1097/MRR.0000000000000132
Zhang, J., Wang, T., Zhao, Q., and Liu, S. (2020). Impacts of a lower limb exoskeleton robot on the muscle strength of tibialis anterior muscle in stroke patients. E3S Web Conf. 185, 03036. doi: 10.1051/e3sconf/202018503036
Keywords: cerebral palsy, traumatic brain injury, robotic exoskeleton, gait, balance, rehabilitation, stroke
Citation: Karunakaran KK, Pamula SD, Bach CP, Legelen E, Saleh S and Nolan KJ (2023) Lower extremity robotic exoskeleton devices for overground ambulation recovery in acquired brain injury—A review. Front. Neurorobot. 17:1014616. doi: 10.3389/fnbot.2023.1014616
Received: 08 August 2022; Accepted: 27 March 2023;
Published: 25 May 2023.
Edited by:
Fabrizio Patane, University Niccolò Cusano, ItalyReviewed by:
Ljubica Konstantinovic, Faculty of Medicine, University of Belgrade, SerbiaIlaria Mileti, University Niccolò Cusano, Italy
Copyright © 2023 Karunakaran, Pamula, Bach, Legelen, Saleh and Nolan. This is an open-access article distributed under the terms of the Creative Commons Attribution License (CC BY). The use, distribution or reproduction in other forums is permitted, provided the original author(s) and the copyright owner(s) are credited and that the original publication in this journal is cited, in accordance with accepted academic practice. No use, distribution or reproduction is permitted which does not comply with these terms.
*Correspondence: Kiran K. Karunakaran, kkarunakaran@kesslerfoundation.org