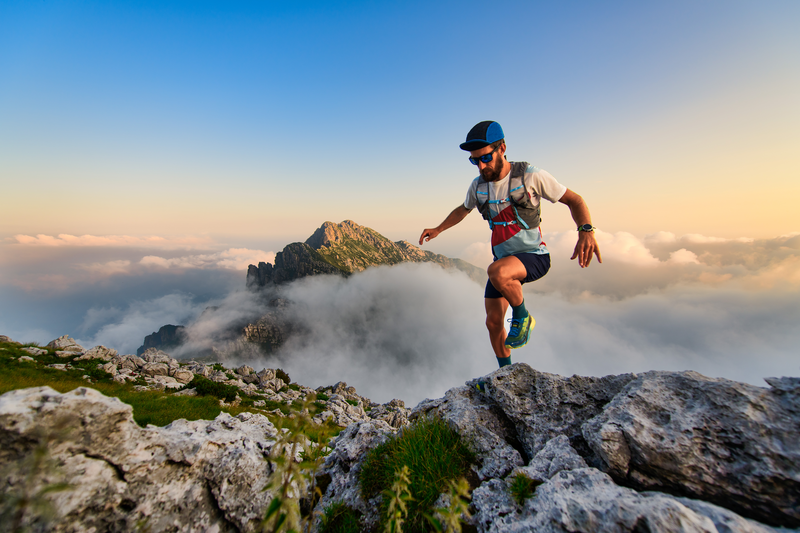
94% of researchers rate our articles as excellent or good
Learn more about the work of our research integrity team to safeguard the quality of each article we publish.
Find out more
ORIGINAL RESEARCH article
Front. Behav. Neurosci. , 04 December 2024
Sec. Learning and Memory
Volume 18 - 2024 | https://doi.org/10.3389/fnbeh.2024.1501377
Environmental Enrichment (EE) has received considerable attention for its potential to enhance cognitive and neurobiological outcomes in animal models. This bibliometric analysis offers a comprehensive evaluation of the EE research spanning from 1967 to 2024, utilizing data extracted from Scopus and analyzed through R and VOSviewer. The volume of publications, citation patterns, and collaborations were systematically reviewed, highlighting important contributions and emerging trends within the field of animal research. Core concepts of EE research are mapped, revealing key themes such as neuroplasticity, cognitive function, and behavioral outcomes. A significant increase in EE research is demonstrated, particularly after the year 2000, reflecting growing scientific and public interest in EE paradigms. This analysis provides insights into the global contributions and collaborative networks that have shaped EE studies over time. The role of EE in advancing the understanding of neurobiological, neurodevelopmental, and neurodegenerative processes is underscored. Influential contributors, leading countries, and high-impact journals in the field of EE are identified, offering a valuable resource for researchers seeking to understand or extend the current knowledge base. The strategic selection of keywords and rigorous data curation methods ensure that the findings accurately reflect the most impactful aspects of EE research in animals. This study serves as an essential reference for future explorations and applications of EE across disciplines. By providing a clear and structured overview of the field, this paper aims to serve as a foundation for ongoing and future research initiatives, encouraging more robust investigations and applications of EE to enhance cognitive and neurological health globally.
The Environmental Enrichment (EE) paradigm has been explored since the 1960s for its role in enhancing neural and cognitive functions in animal models (Vogel et al., 1967). Conventionally, EE includes objects that provide physical, cognitive, sensory, and social stimulation to improve overall mental health (Singhal et al., 2014; Baumans, 2005). EE has been extensively explored for its role in enhancing brain function. Studies have shown that EE increases the size and complexity of dendritic branches (Jung and Herms, 2014; Rojas et al., 2013; Beauquis et al., 2010; Leggio et al., 2005), promotes neurogenesis in the hippocampus (Beauquis et al., 2010; Fabel et al., 2009; Khalil, 2024; Rizzi et al., 2011), restores and upregulates synaptic plasticity (Aghighi Bidgoli et al., 2020; Stein et al., 2016; Kim et al., 2016), improves neurotransmitter function (Mora et al., 2007; Darna et al., 2015; Koh et al., 2007), and enhances expression of neurotrophic factors (Sun et al., 2010; Dandi et al., 2018; Birch et al., 2013). EE has also been shown to help in improving memory (Aghighi Bidgoli et al., 2020; Birch et al., 2013; Sahini et al., 2024), learning (Sahini et al., 2024; Bramati et al., 2023; Zentall, 2021), cognition (Zentall, 2021; Singhal et al., 2019a), and adaptability to stressful environments (Lehmann and Herkenham, 2011; Fan et al., 2021; Cabrera-Álvarez et al., 2024). However, much of the research on EE is somewhat fragmented with studies focusing on different aspects of EE using varied species of animals and methodologies over the years.
Bibliometric analysis is a systematic and quantitative approach to evaluate the current structure, development, and impact of research in specific fields (Ellegaard and Wallin, 2015; Donthu et al., 2021). It offers valuable insights into the trends and patterns of studies. By identifying key research themes, influential studies, and collaborative networks, this analysis helps to integrate diverse research patterns, guide future directions, and foster a more cohesive understanding of research impact and potential applications. To date, no bibliometric analysis has been conducted on EE research in animal models.
Hence, in this study, bibliometric techniques were utilized to analyze past and current research on EE, a growing area of study within neuroscience and related disciplines. By examining the volume of publications, citation patterns, and collaborations among authors and institutions, this analysis maps the scholarly network of EE research, identifies key contributors, and uncovers emerging trends in the field. It highlights the historical progression and scientific impact of EE, offering insights into its influence on fields such as neurodevelopmental disorders, mental health, and animal welfare. This broader perspective is essential for understanding how themes like neuroplasticity, behavior, and stress resilience have developed over time and for recognizing the interdisciplinary applications of EE beyond specific variables like brain function and behavior. By identifying knowledge gaps and underexplored areas, the analysis provides valuable insights to guide future research and advance the field in new directions, making it a crucial resource for researchers across disciplines.
To achieve this, Scopus was used as the database for article retrieval. The metadata of the collected articles were analyzed using R and VOSviewer software, both commonly used in bibliometric analyses (Derviş, 2019; McAllister et al., 2022). This helped us analyze keywords, authorship, co-authorship, co-citation, country distribution, and the impact of scientific journals. The analyses also allowed for the visualization of research networks and thematic clusters, providing valuable insights into the collaborative network and influential works that have shaped the research on EE over time.
Scopus, a scientific abstract and citation database of peer-reviewed literature from the academic publisher Elsevier, was utilized for searching and collecting articles related to EE (Burnham, 2006; Thelwall and Sud, 2022). A bibliometric analysis was then performed using the “Bibliometrix” package in R (version 4.4.1) and VOSviewer (version 1.6.20) to visualize the pooled metadata from the cataloged articles. EndNote (version 20) was used for referencing purposes. Table 1 shows the step-by-step process of data retrieval, handling, processing, analysis, and visualization involved in the bibliometric analysis.
Table 1. Steps involved in the bibliometric analysis process: data collection, curation, and visualization.
Scopus was selected as the primary database for data collection due to its comprehensive coverage of peer-reviewed, high-quality research publications and its strong reputation within the academic community (Burnham, 2006; Thelwall and Sud, 2022). Scopus provides robust indexing of a wide range of disciplines, including neuroscience, which is central to the topic of EE. It offers advanced citation tracking and a broad selection of journals, ensuring that the analysis captures key publications and the most influential studies in the field.
Google Scholar, while more comprehensive in terms of the range of documents indexed, does not offer the same level of quality control. Similarly, PubMed, while highly respected for biomedical research, is too specialized for the broader scope of EE. Additionally, Web of Science was excluded from the data collection sources due to its overlapping coverage with Scopus. By focusing on a single source, Scopus, efficiency in data handling was maintained while still capturing a comprehensive view of the relevant literature without the redundancy that might arise from using multiple similar databases.
The primary search terms used during the database queries were “environmental enrichment” and related terms, such as “enriched environment,” ensuring that the search remained centered around the core theme of the analysis, enhancing the accuracy of the retrieved dataset. This search yielded a total of 2,385 articles. The time range of the articles spanned from 1967 to August 2024. Unrelated subject areas such as Engineering, Social Sciences, Chemical Engineering, Earth and Planetary Sciences, Chemistry, Computer Science, Physics and Astronomy, Nursing, Materials Science, Energy, Arts and Humanities, Mathematics, Business Management and Accounting, Decision Sciences, and Dentistry were excluded from the Scopus search. The selection was limited to documents categorized as articles, reviews, and book chapters, and source types were restricted to journals, books, and book series. Additionally, only articles published in English were included.
Titles, and where necessary, abstracts, were reviewed to ascertain their direct relevance to the core themes of the bibliometric analysis. Articles that did not explicitly align with the EE theme were excluded. Specifically, articles were excluded if they did not explicitly focus on the core theme of EE, such as neuroplasticity, behavior, cognition, or other related biological and neurological outcomes. Studies that lacked direct application to the biological, cognitive, or behavioral aspects of EE were also excluded. For example, studies that mentioned “enrichment” in contexts unrelated to neuroscience or behavior (e.g., enrichment in environmental engineering) were excluded to maintain the biological focus of the analysis. Furthermore, studies addressing topics such as animal welfare or habitat design, without examining the biological or neurological effects of EE, were excluded, as they did not contribute to the core focus of the bibliometric analysis on the impact of EE on brain function and behavior. Some articles focusing on broader or less directly related topics, such as education or human animal social interactions, which fall under fields like psychology but may not explicitly deal with the core biological aspects of EE (e.g., neuroplasticity or brain function in animal models), were also excluded. Preliminary analysis using VOSviewer and R helped in the identification of additional keywords, which contributed to further refining the search and extraction of more relevant articles (refer to Appendix 1 for a comprehensive list of keywords). This rigorous selection process narrowed the dataset to 1,847 articles on Scopus, ensuring that retained studies were directly related to the analysis objectives. Appendix 2 shows the exported values from Scopus for each inclusion criteria. The metadata of these articles was then exported from Scopus as a CSV file for subsequent evaluation in R and VOSviewer.
The articles were included regardless of the availability of their full texts. This decision is aligned with the nature of bibliometric analysis, which primarily relies on metadata such as titles, abstracts, keywords, authors, and citation details rather than the full text content. Bibliometric tools like VOSviewer extract and analyze keyword frequencies, citation information, and authors details to uncover patterns and trends in the literature, making the full text of articles less crucial for this type of analysis. Furthermore, this inclusive approach helps mitigate potential bias that could arise from excluding significant studies due to access restrictions.
To streamline the dataset and reduce redundancy, a systematic approach was adopted using a thesaurus file to merge keywords representing the same concept for visualization purpose in VOSviewer. For instance, enriched environment, environmental enrichment, and environment enrichment were unified under “ee,” mice, mouse, rat, and rats were consolidated under the broader term “rodents,” behavior and behavior were considered together under the term “behavior,” and aging and aging were unified as “aging.” Similarly, anxiety and anxiety-like behavior were grouped under the term “anxiety.” This approach ensured comprehensive coverage of similar keywords written differently. Additionally, the variations in keyword forms, including singular and plural forms, as well as terms with or without apostrophes, were unified to avoid fragmentation. For instance, variations like Alzheimer Disease and Alzheimer's Disease were standardized to “Alzheimer's Disease,” and pig and pigs were merged into “pigs.”
Reducing the number of distinct nodes in the network helped minimizing visual clutter, making dominant research themes more discernible, as well as ensuring that fragmented studies were cohesively analyzed, providing a more accurate representation of the research activity in the field.
While conducting bibliometric analysis in VOSviewer, author keywords were chosen over index keywords to provide a more accurate reflection of the topics that researchers themselves consider central to their studies. Author keywords are directly provided by the original authors of the papers and are therefore more likely to represent the primary concepts and research areas the authors aimed to explore. This contrasts with index keywords, which may be assigned by databases based on broader classification systems and may not always reflect the specific focus of the research.
To ensure the robustness of the analysis, a minimum occurrence threshold of five was set for keywords to be included. This criterion was established to maintain a balance between dominant research topics while excluding terms that appear infrequently and may not be representative of trends in the field. Only keywords with a meaningful level of recurrence across multiple studies were considered, enhancing the reliability and interpretability of the co-occurrence network and thematic clustering generated by the software.
A minimum citation count of five was also established for authors and countries involved in the analysis. Setting a citation count threshold helped to filter out less influential works, focusing on those researchers and countries that have not only been prolific in terms of publication but have also made significant scholarly contributions in the field of EE. This helped us understand which researchers and regions are driving advancements and gaining recognition in this area of study.
Similarly, a minimum document threshold of five was established for authors, countries, and journals to be included in the co-authorship analysis. This threshold was implemented to identify and highlight the most active and collaborative authors, countries, and scientific journals in the field of EE. By doing so, the focus remained on contributors who not only have substantial involvement in EE research but also play pivotal roles in the dissemination and development of knowledge within the global scientific community through collaboration and publications in high-impact journals.
For the co-citation analysis, a minimum citation threshold of 200 was set for authors. Co-citation occurs when two or more authors are cited together in other works, indicating that their research is often referenced in conjunction with each other and may share thematic or methodological similarities. This analysis is instrumental in identifying influential authors whose work forms the basis for ongoing research within the field. The co-citation measure highlights the most impactful contributors whose research has been consistently acknowledged by peers. This complements the other bibliometric analyses, offering a well-rounded view of contemporary collaborations.
Finally, to maintain clarity and focus, the top 25 results in each category were selected for co-occurrence visualization. This methodological choice helped avoid visual clutter and ensures that the most significant contributors in the field are highlighted.
Link strength is a central metric in bibliometric and network analysis that quantifies the strength of the association between two elements in a co-occurrence network, such as authors, institutions, or keywords. It provides insight into how closely two elements are connected based on the frequency of their co-occurrence within the dataset. In this study, link strength was calculated to assess the relationships between various entities within EE research, allowing for the identification of influential collaborations, research themes, and trends.
Link strength is not limited to a simple binary relationship (i.e., whether or not two entities are connected) but it provides a continuous measure of the degree of association between entities. For example, a higher link strength between two authors suggests frequent collaboration, while a lower link strength suggests occasional collaboration. Similarly, when analyzing institutional linkages, a strong link indicates active collaboration between institutions on shared research projects, while weaker links reflect less frequent or occasional collaboration. In this study, by applying a threshold to the minimum number of occurrences required for inclusion in the network, only relevant and significant links were analyzed, ensuring that the most meaningful connections were highlighted. This filtering helped avoid the inclusion of less frequent or potentially less relevant associations.
To compute link strength, the software VOSviewer was used to generate co-occurrence networks which is a powerful tool for understanding the dynamics of a research field. In these networks, nodes represent entities (such as authors or institutions), and links between nodes represent the number of times those entities appear together within the same publication. The strength of the link is determined by the frequency of these co-occurrences: the more frequently two entities co-occur, the stronger the link between them. This is represented numerically by the link strength value. The link strength is represented visually as lines or edges connecting nodes, with the size of each node reflecting the frequency or magnitude of that entity's contribution to the dataset and thickness of each line corresponding to the magnitude of the link strength. Thicker lines indicate stronger relationships between entities, while thinner lines represent weaker or less frequent connections. Link strength, size of nodes and thickness of lines help in identifying clusters within the network, where groups of entities are more closely connected to each other than to the rest of the network. These clusters often represent specific research communities that are highly interconnected and are color coded in VOSviewer. For example, in collaboration analysis, different colors may represent various collaborative networks across geographic regions or research subfields. This clustering analysis allows for the identification of dominant research trends, leading contributors, and emergent fields of study. The size of a cluster indicates the significance of that group in the network, while the proximity of clusters to each other reveals the degree of interdisciplinary collaboration or overlap between research topics. A dense network, characterized by numerous and strong connections between nodes, suggests a highly collaborative field where entities frequently interact or co-publish. In contrast, a sparse network with fewer or weaker connections may indicate a more fragmented field, where research is conducted in isolated pockets with less interaction between entities. By quantifying and visualizing link strength and nodes, this study has provided a clear map of the collaborative landscape and intellectual structure within EE research.
Figure 1 depicts the annual scientific production of research in EE from 1967 through 2024. It shows a modest beginning with fewer than 10 publications annually until the late 1980s, followed by a gradual increase through the 1990s. A notable acceleration in publication volume began in the early 2000s, reflecting growing interest and recognition of EE significance in scientific research. This upward trend continued over the years, reaching a peak of 122 publications in 2022. The trend line from 2000 onwards suggests a period of particularly rapid growth, aligning with heightened scientific and public interest in the implications and applications of EE.
Figure 1. Annual scientific production on EE research. The graph displays the annual number of publications related to EE from 1967 to 2024.
The bar graph illustrates the distribution of corresponding authors focusing on EE research by country (Figure 2). The USA leads significantly with 403 corresponding authors, demonstrating a dominant role in EE research. Brazil follows with 143 authors, highlighting its substantial contribution to the field, particularly when compared to other nations. China, Spain, and the United Kingdom also show notable involvement with 99, 85, and 83 corresponding authors, respectively, underscoring their active research communities in this area.
Figure 2. Corresponding author count by country. The bar chart presents the number of corresponding authors contributing to EE research by country.
Canada, Australia, and Germany contribute with 78, 71, and 67 corresponding authors respectively, reflecting a strong engagement in EE studies. Italy and France, with 56 and 50 corresponding authors respectively, round out the list of the top 10 countries contributing to EE research. This geographic distribution indicates a broad international interest and varied contributions to the advancements in EE, reflecting its global relevance and interdisciplinary nature.
The bar chart illustrates the total number of citations received by all research articles on EE published by authors from various countries (Figure 3). The USA stands out prominently with a staggering 18,798 citations, illustrating its pivotal role and significant impact in EE research globally. Germany follows as a distant second with 4,359 citations, underscoring its substantial contributions to the field.
Figure 3. Total citations by country. This bar chart displays the total number of citations received by publications on EE research, categorized by country.
Canada and the United Kingdom are closely paired with 4,256 and 4,085 citations respectively, indicating their strong academic outputs and influential research in EE. Italy also shows a robust presence in the field with 3,696 citations, followed by Spain and France, which have accumulated 3,427 and 3,410 citations respectively.
Australia, Brazil, and the Netherlands round out the list of top 10 countries with 2,406, 2,146, and 1,736 citations respectively. These figures highlight the broad international interest and the recognition of work on EE originating from these regions by the global scientific community.
A total of 3,538 author keywords were identified, out of which 180 met the threshold criteria of a minimum occurrence of five in VOSviewer. Link strength in this analysis represents the frequency with which two keywords appear together across the dataset. For visualization purposes, the top 25 keywords were selected based on their occurrences and total link strength, indicating how strongly a keyword is associated with others in the network (Table 2).
The most central keyword, “ee,” appeared 1,050 times and had a total link strength of 1,107, suggesting that “ee” was not only frequently used but also had strong associations with other keywords. Other significant keywords include “behavior” with 164 occurrences and a link strength of 292, and “rodents” with 163 occurrences and a link strength of 272, reflecting the focus on behavioral studies and rodent models in EE research. Keywords such as “learning and memory” and “hippocampus” also featured prominently, with high occurrences and link strengths, underscoring the neurobiological dimensions explored in EE research. Higher link strength for these keywords signifies that they are frequently co-mentioned with other key terms, revealing how interconnected themes like memory and hippocampal function are within the broader scope of EE studies. The selective visualization helped highlight the most influential and recurrent themes within the extensive dataset, providing clear insights into the dominant research areas within the field of EE. By focusing on both occurrences and link strength, the analysis effectively illustrates not only which topics are most frequently studied but also how closely related they are to other research areas, revealing key thematic clusters.
As expected, the network visualization reveals the term “ee” as the central node. Surrounding this focal point, several key thematic clusters and terms have emerged, highlighting significant areas within the research landscape (Figure 4). In the figure, nodes represent individual keywords, and the size of each node corresponds to the frequency of occurrence of that keyword in the dataset. The lines or edges connecting the nodes represent the co-occurrence of keywords in the same publications. The thickness of these lines corresponds to the strength of association between the terms. Thicker lines indicate that the two keywords frequently appear together, while thinner lines suggest a weaker or less frequent association. The colors of the nodes and links (lines or edges) represent distinct clusters of related terms. The visual clusters formed by the interconnected nodes reveal the primary research domains and highlight the interdisciplinary nature of EE studies across neuroscience, behavior, and recovery from neurodegeneration or injury.
Figure 4. Keyword co-occurrence network in EE research. The network visualization shows the co-occurrence of keywords in EE research articles, with nodes representing individual keywords and edges representing co-occurrences between them. The size of each node corresponds to the frequency of the keyword's appearance, while the color represents different thematic clusters. The thickness of the edges represents the strength of co-occurrence of keywords, with thicker lines indicating a strong and more frequent association.
The cluster in green, containing keywords such as “hippocampus,” “synaptic plasticity,” “neuroplasticity,” “bdnf,” and “neurogenesis” are densely connected, emphasizing the significant focus on the neurobiological effects of EE. The strong links between these terms suggest a deep research interest in how EE influences brain structure and function, particularly in areas related to learning, memory, and cognitive resilience. The presence of keywords like “exercise,” “aging,” and “Alzheimer's disease” in this same cluster points to the diverse applications of EE across different life stages and conditions, including gerontology and neurodegenerative diseases.
Similarly, the red cluster captures keywords related to behavioral outcomes, including “behavior,” “anxiety,” “depression,” “stress,” and “aggression.” The connections among these terms illustrate the extensive study of role of EE in modulating behavior and emotional responses, often in the context of stress-related disorders and mental health. The thickness of the edges between these nodes further highlights the strong association and frequent co-mention of these terms in the EE literature.
The blue cluster reflects research focused on the role of EE in recovery from cognitive and physical impairments. Terms like “tbi” (traumatic brain injury), “Morris water maze,” and “functional recovery” are prominent, underscoring the application of EE in cognitive and functional recovery studies, particularly within rodent models.
Interestingly, the term “rodents” appears as a central node connected to both neurobiological and behavioral clusters, indicating the prevalent use of rodent models in EE research. Its connections to experimental outcomes such as the “Morris water maze” reinforce the central role of rodent-based studies in advancing our understanding of the effects of EE on cognition and behavior.
During our co-authorship analysis of EE research, a total of 7,339 authors were considered, but only 97 met the threshold of having authored a minimum of five co-authored publications on EE. Link strength in this analysis refers to the strength of the collaborative relationships between authors, measured by the number of publications they co-authored together. The analysis was then visualized focusing on the top 25 authors, chosen based on their number of documents, citations, and the strength of their collaborative links considered together (Table 3).
Table 3. Top 25 authors based on the number of documents, citations and total link strength from collaboration work.
Anthony E. Kline emerged as one of the most central figures with 31 documents, reflecting his extensive collaborative network, underscored by the highest total link strength of 65. A higher link strength demonstrates that Kline not only produces a large volume of work but also has deep, established collaborations, making him a pivotal figure in fostering collaborative research within the EE community. Lamberto Maffei also stood out with 15 documents and the highest citation count of 1,678 with co-authors, indicating both prolific output and substantial influence. Although his link strength is not as high as Kline's, his citation count reflects the significance of his research within the collaborative network. Notably, the network also includes other key contributors like Anthony J. Hannan, Marcello Solinas, Jeffery P. Cheng, and Corina O. Bondi, who have been instrumental in expanding the interdisciplinary reach of EE studies. The link strength among these authors signifies frequent and robust collaborations, showing how their joint efforts have driven the field forward. It showcases the deep interconnections between authors that foster scientific inquiry and highlights the importance of collaboration in advancing research within the EE community.
The VOSviewer visualization of author collaborations in EE research illustrates a vibrant network of scholars contributing to the field (Figure 5). The analysis identifies several key clusters of authors, each distinguished by different colors, representing collaborative groups that have significantly impacted EE studies. In this network, nodes represent individual authors, with the size of each node corresponding to the number of publications by that author. Edges or lines between the nodes represent co-authorships, indicating collaborations between authors. The thickness of the lines reflects the strength of the collaboration, where thicker lines represent frequent co-authorship or closer collaborative relationships.
Figure 5. Author collaboration network in EE research. The network visualization shows the collaboration patterns between authors in the field of EE research, with nodes representing individual authors and edges representing co-authorship links. The size of each node corresponds to the number of publications by that author, while the color represents different clusters of collaborative groups. The thickness of the edges represents the strength of collaborations, with thicker lines indicating more frequent co-authorships between authors.
The network is divided into several clusters, each represented by a different color, indicating groups of authors who frequently collaborate within a particular subfield of EE research. For example, red clusters signify a group of authors working on neuroplasticity and neurorehabilitation, including figures such as Anthony J. Hannan, Gerd Kempermann, and Lamberto Maffei. These authors often co-author papers related to neurogenesis, brain plasticity, and the role of EE in mental health, indicating a shared research focus. Green clusters represent another significant group, primarily focused on behavioral outcomes of EE, such as drug addiction and cognitive recovery. Marcello Solinas, Michael T. Bardo, and their collaborators are central figures in this group, as reflected by their relatively large nodes and thick connecting edges, indicating frequent and strong collaborations in this research area. Blue clusters focus on neuroprotection and the effects of EE on recovery from neurological disorders, with authors such as Anthony E. Kline and Francisco Mora playing pivotal roles. The proximity and thickness of the connecting lines between these authors reflect their interdisciplinary collaborations in fields like traumatic brain injury and neuroprotection, showcasing how different themes within EE research overlap and interact.
Anthony E. Kline has emerged as a pivotal figure, represented by the largest node, indicating his extensive collaborations and influence within the EE community. His work primarily connects various aspects of neurorehabilitation and neuroprotection, showing a strong interdisciplinary approach. Michael T. Bardo and Marcello Solinas represent major clusters focused on the behavioral sciences, exploring the implications of EE on drug addiction and cognitive functions. Lamberto Maffei and his collaborators have significantly contributed to understanding the neuroplasticity and sensory processing alterations induced by EE. Anthony J. Hannan has robust ties with his collaborators, particularly around genetic models and mental health outcomes, underscores the translational impact of EE research.
In the comprehensive bibliometric analysis on EE, a total of 129,389 author names appeared across the analyzed articles. With a stringent threshold set at a minimum of 200 co-citations (two publications are cited together by a third publication), 70 authors met this criterion. Link strength in this context represents the number of times two authors are co-cited together, indicating how frequently their works are referenced together in the same publication. The top 25 co-cited authors were selected for a more detailed visualization to highlight their contributions and the intricate network of intellectual collaboration within the field (Table 4).
Gerd Kempermann leads with 1,458 co-citations, underscoring his significant impact on studies related to neurogenesis and brain plasticity. His high link strength reflects the frequent co-citation of his work with other influential authors, signifying a central position in the intellectual network of EE research. Following closely is Fred H. Gage, with 1,393 co-citations, renowned for his work in similar domains. The strong link strength between Gage and other top authors indicates that his research is often referenced in conjunction with other prominent studies in the field, illustrating his intellectual influence. Anthony J. Hannan and Mark R. Rosenzweig, with 1,059 and 899 co-citations respectively, also emerge as key figures, particularly noted for their research on cognitive and neural plasticity influenced by environmental factors. The link strength of their co-citations highlights the close thematic relationship of their work with other major contributors in EE research, showing how their studies are frequently grouped with those of other leading figures when cited by the broader scientific community.
This analysis sheds light on the collaborative and intellectual relationships that shape the EE research field. The visualized network based on co-citation patterns reveals which authors works are most often studied together, providing insights into how foundational research clusters have formed and evolved over time.
For visualization purposes, the top 25 most co-cited authors were selected, representing the scholarly impact and network connections in EE research. In the co-citation network, nodes represent individual authors, and the size of each node reflects the number of co-citations associated with that author. Larger nodes, such as those representing Anthony E. Kline and Gerd Kempermann, indicate authors who have been frequently co-cited in the literature, marking them as central figures in EE research. Edges or lines between the nodes represent co-citations between authors, where thicker edges indicate more frequent co-citations, suggesting stronger conceptual or thematic links between the works of those authors. The visualization through VOSviewer revealed a dense network of co-citations among leading researchers, with several notable nodes indicating key figures (Figure 6).
Figure 6. Co-citation network of authors in EE research. The network visualization depicts co-citation relationships between authors frequently cited together in EE research. Nodes represent individual authors, with node size corresponding to the number of times an author has been co-cited, and edges indicating the frequency of co-citations between authors. The thickness of the edges represents the strength of co-citation ties, demonstrating intellectual connections and thematic overlap between authors.
Anthony E. Kline and Jeffrey P. Cheng, positioned on the extreme right of visualization, formed a prominent blue cluster, indicating their pivotal roles and frequent co-citations in the literature on neural rehabilitation and neuroplastic effects of EE. The thickness of the connecting lines reflects their strong associations with other researchers, showcasing their influence in shaping this field. Similarly, Gerd Kempermann and Hans G. Kuhn, central in the network and marked in red, illustrate strong interlinkages, primarily related to their work on adult neurogenesis and cognitive enhancement through EE. The red cluster illustrates a tightly knit group of researchers focusing on neuroplasticity and its applications, with robust internal connections that highlight frequent co-citation patterns. Michael T. Bardo and Anthony J. Hannan, shown in green, connect widely with multiple clusters, underscoring their interdisciplinary impacts on behavioral sciences and neurogenetic aspects of EE. The thick lines linking their nodes to other clusters emphasize their research cross-disciplinary relevance, as their work on behavioral outcomes and genetic models of EE is frequently co-cited with studies from various fields. Lamberto Maffei, highlighted in yellow, shows extensive connectivity, emphasizing his contributions to sensory plasticity and neuronal development studies within the context of EE. His position within the network suggests strong ties to other influential researchers, further demonstrating the breadth of his impact.
This analysis highlights the interconnected nature of research on EE. It shows that the original works by highly cited authors facilitate cross-disciplinary dialogues and advancements. This visualization depicts how the research on EE is interwoven, encompassing studies ranging from investigations of molecular mechanisms to therapeutic applications.
During the bibliometric analysis of global collaborations in EE research, contributions from 85 countries were identified. Out of these, at least five publications on EE were recorded from 42 countries, reflecting broad international interest in this field. Link strength in this context measures the degree of collaboration between countries, based on co-authorships across different nations. For the purposes of visualization, the focus was placed on the top 25 most active countries based on their publication output, citation count with other countries, and total link strengths (Table 5). A higher link strength between countries indicates frequent collaboration on shared research projects, showing how closely countries work together in EE research.
Table 5. Top 25 countries based on the number of documents, citations, and total link strength from collaboration work with other countries.
The United States led the field with 595 documents and a remarkable citation count of 29,975, highlighting its central role in EE research globally. The high link strength of the U.S. with other countries demonstrates its extensive collaborative efforts in driving EE studies forward, as many U.S. researchers frequently co-author with international colleagues. Other highly active countries included the United Kingdom and Brazil, which also showed strong link strengths with various international partners, illustrating their role in fostering global collaborations. Substantial contributions were also noted from countries like Canada, Spain, and Australia as well. The strong collaborative ties between these countries and others in the field have helped in the development and dissemination of EE-related knowledge. The link strength values help highlight the interconnectedness of the global research community, showing which countries have established strong international research partnerships and how these collaborations contribute to the advancement of EE studies.
The VOSviewer visualization shows global collaboration in EE research (Figure 7). Nodes in the visualization represent individual countries, with the size of each node reflecting the volume of publications produced by that country. Larger nodes, such as the United States, indicate that the country plays a central role in EE research, contributing a substantial number of publications and fostering international collaborations. The edges or lines between nodes represent collaborative relationships between countries, with the thickness of the edges indicating the strength of the collaboration. Thicker lines between two countries, such as the United States and Canada or the United Kingdom, demonstrate frequent co-authorship and strong bilateral research ties, showing how these nations collaborate closely in EE research. The color of the nodes represents different clusters, or groups of countries, that frequently collaborate within specific geographic or thematic areas of EE research.
Figure 7. Global collaboration network in EE research. The network visualization illustrates the global collaboration patterns in EE research, with each node representing a country and the edges representing collaborative ties between countries. The size of the nodes corresponds to the number of publications from each country, and the thickness of the edges reflects the strength of collaboration between countries.
The green cluster encompasses countries like the United States, China, Brazil, and Canada. This group represents a significant portion of EE research, with the United States acting as a hub that connects these countries through numerous collaborations. The blue cluster features countries like the United Kingdom, Sweden, and the Netherlands, highlighting a group of European nations that work closely together in EE research. The red cluster includes Germany, France, Italy, and Spain, showcasing strong intra-European collaborations. The dense network of connections between these countries indicates frequent research partnerships, suggesting that EE research within Europe is highly interconnected. The proximity of these nodes to one another and the thickness of the lines further underscore their robust research ties, highlighting Europe's role as a collaborative region within the global EE research community. Countries such as South Korea, Brazil, and Australia, represented in various colors, also demonstrate active involvement in EE research. Their strong connections with countries in other clusters, including the United States and China, reflect meaningful partnerships that enhance the global diversity of the research community. While their nodes may be slightly smaller, indicating fewer total publications, the thickness of their connecting lines emphasizes the significance of their collaborations. Additionally, nodes like Japan and India show connections with several key research hubs, indicating their growing participation in global EE research. These connections help integrate diverse geographical regions into the collaborative network, contributing to the advancement of EE studies worldwide.
This visualization illustrates the geographic diversity and interconnected nature of EE research. It highlights the central role of the United States as a global leader, while also showcasing the strong research networks within Europe and between various regions such as North America, Asia, and Latin America. By visualizing these collaborations, the map underscores how international partnerships contribute to the growth and dissemination of knowledge in the field of EE.
The results of the bibliometric analysis for the top 25 journals published articles on EE revealed a robust and diverse scholarly output (Table 6). Link strength in this context refers to the frequency of co-citation between journals, representing the degree to which journals are cited together within the same research articles. Journals with higher link strength are more frequently co-cited with others, indicating strong intellectual connections between the studies they publish.
Table 6. Top 25 scientific journals, determined by the number of documents, citations, and total link strength related to EE research.
The journal Applied Animal Behavior Science leads with the highest number of documents (100) and a significant citation count (6,311), highlighting its significant role in the dissemination of EE research, particularly in applied animal behavior contexts. The strong link strength between this journal and others in the network reflects the interdisciplinary nature of EE studies, as its research is often cited alongside work published in other fields. This is closely followed by Behavioral Brain Research, which has published 94 documents and received 4,559 citations, demonstrating its significant impact on behavioral studies of EE. The link strength between Behavioral Brain Research and other journals emphasizes its importance in connecting behavioral and neurological research, showing frequent co-citation with journals focused on neuroscience. The European Journal of Neuroscience and Journal of Neuroscience are also prominent in the field and maintain considerable citation impact and frequent co-citation with other journals, particularly in studies exploring neural plasticity and cognitive function related to EE. Physiology and Behavior and Neuroscience both contribute significantly as well, focusing on physiological responses to environmental factors and their link strengths indicate their role in bridging the gap between physiology and behavior in EE research.
PLoS One stands out for its broad scope as it supports interdisciplinary reach and open access to EE research. It has published 42 documents related to EE, facilitating wider dissemination and impact of work on EE. Specialized journals like Zoo Biology and Laboratory Animals also reflect the application of EE paradigm across fields like zoology and veterinary sciences, indicating the multidisciplinary nature of EE studies.
The journals Brain Research and Experimental Neurology show focused contributions to understanding the neurological underpinnings and experimental aspects of EE. These journals also have strong link strengths with others in the field, particularly those that focus on neuroplasticity and cognitive studies, indicating that their research is often cited together with key studies in EE. The presence of high-impact journals like Proceedings of the National Academy of Sciences of the United States of America (PNAS) with fewer documents but substantial citation numbers highlight key groundbreaking research contributions to the field of EE from the journal. The strong link strength of PNAS indicates that its publications on EE are frequently cited alongside foundational studies in the field, illustrating its influence in shaping major research trends.
The visualization of journal citation networks within the field of EE reveals a concentrated interaction among key scientific publications, each playing a significant role in disseminating research on this topic (Figure 8). Nodes in the visualization represent individual journals, and the size of each node corresponds to the number of citations that journal has received within the EE research network. Larger nodes, such as those representing Behavioural Brain Research and Neuroscience, indicate that these journals are central to EE research and frequently cited by others in the field, reflecting their prominence in publishing influential studies. Edges or lines between the nodes represent citation links between journals, where the thickness of the lines reflects the strength of the citation relationships. For example, the thicker lines between Behavioural Brain Research, Neuroscience, and Brain Research highlight their close citation relationships and their central role in advancing the neurobiological aspects of EE.
Figure 8. Journal citation network in EE research. The network visualization shows the citation relationships between journals publishing EE research. Each node represents a journal, with the size of the node corresponding to the number of articles published on EE, and the edges representing citation links between journals. The color of each node reflects clusters of closely related journals in terms of their citation patterns.
The color-coded clusters represent groups of journals that frequently cite each other, indicating thematic or disciplinary closeness. Journals such as Behavioural Brain Research, Neurobiology of Learning and Memory, and Neuroscience form a red cluster, highlighting their tight-knit focus on neurological and behavioral research in EE. This cluster demonstrates a strong focus on cognitive function, neural plasticity, and behavioral outcomes of EE, emphasizing their role in contributing foundational research in these areas. The green cluster, containing journals like Pharmacology Biochemistry and Behavior, Psychopharmacology, and Frontiers in Behavioral Neuroscience, reflects an emphasis on psychopharmacology and behavioral neuroscience, where EE research intersects with studies on drug effects and behavioral outcomes. The frequent citation patterns among these journals illustrate their shared interest in how environmental factors and pharmacological agents influence behavior and brain function.
Journals such as PLoS One and Physiology and Behavior serve as central connectors, with blue clusters representing their interdisciplinary impact. These journals bridge research areas across neuroscience, physiology, and behavioral sciences, as indicated by their multiple connections to other clusters. The diversity of research covered in these journals makes them key hubs for disseminating a wide range of EE-related studies, from neurobiology to behavior and physiology. Their position in the network underscores the interdisciplinary nature of EE research, which spans multiple fields, including psychoneuroendocrinology, as represented by journals like Psychoneuroendocrinology.
Additionally, specialized journals such as Applied Animal Behaviour Science and Zoo Biology form part of the light blue cluster, representing the extension of EE research into applied animal behavior and zoological studies. Their presence in the network highlights how EE research is not confined to laboratory neuroscience but also plays a role in understanding animal welfare and behavioral enrichment in applied settings, such as zoos and laboratories.
This network visualization highlights the interconnected nature of journal publications within EE research, demonstrating how journals from diverse disciplines contribute to the broader understanding of how environmental factors influence behavior and brain function. The various clusters and the thickness of the connecting lines emphasize the intellectual connections that shape the field and point to the interdisciplinary nature of EE research, spanning core neuroscience, behavior, pharmacology, and applied animal studies.
EE involves enhancing the living conditions of captive animals to promote their physical and psychological wellbeing (Singhal et al., 2014). Since the 1960s, research in this field has evolved significantly, reflecting shifts in scientific focus, technological advancements, and a deeper understanding of neurobiology and animal welfare. This manuscript examined the emerging patterns and trends in EE research from 1967 to 2024 utilizing robust software, such as R and VOSviewer, for bibliometric analysis and to generate co-occurrence networks.
In the late 1960s and 1970s, early research by Vogel et al. (1967, 1968), Walsh et al. (1972), and Gardner et al. (1975) primarily focused on comparing EE with deprivation. In 1967, keywords like EE, deprivation, and cognitive functioning indicate a focus on how enriched environments affect cognition when compared to deprived settings, signaling a foundational understanding that environmental factors significantly shape brain development and behavior (see Appendix 2 for keywords each year). However, by 1970, research began incorporating specific behavioral tests. Keywords such as open field, food competition behavior, mouse, and running wheel suggest that researchers started measuring the exploratory and feeding behavior in rodents in response to EE, while also suggesting an early interest in the relationship between physical exercise and brain function (Monosevitz, 1970). The use of rodents in these studies reflected a growing trend toward controlled measurable experiments that could provide deeper insights into the behavioral changes brought about by different environmental conditions.
In 1972 and 1973, terms like Hebb-Williams maze, defecation, exploration, and open-field activity further indicate the use of mazes and open-field tests to assess learning and anxiety-related behaviors in rodents reared in enriched vs. standard environments (Smith, 1972; Manosevitz and Montemayor, 1972; Manosevitz and Joel, 1973). Running wheel activity remained a common measure of enrichment. The keyword post-weaning enrichment underscores research exploring the effects of EE at developmental stages, with findings suggesting that EE may help mitigate the impact of early deprivation (Smith, 1972).
In 1975, studies investigated the effects of factors like cage size and environmental conditions on learning, memory, and physiological responses like body weight and water intake (Huck and Price, 1975; Manosevitz and Pryor, 1975). The impact of environmental impoverishment became a central focus, leading to a deeper understanding of how different degrees of environmental stimulation can influence cognitive and physical health. Studies on surface texture as an enrichment factor demonstrated that even seemingly minor changes in the environment could have profound effects on learning outcomes (Manosevitz and Pryor, 1975). By 1976, the scope broadened to examine the effects of environmental crowding on aggression and hormonal responses, highlighting a growing awareness of the social and hormonal dynamics influenced by EE (Hull et al., 1976). In 1977, a new avenue of research emerged, where EE was studied in the context of recovery from brain injuries or lesions. Researchers explored how brief periods of enrichment following post-weaning cerebral lesions could promote recovery of learning and memory in rodents, particularly in animals exposed to methamphetamine (Will, 1977). This year marked the beginning of using EE as a potential therapeutic tool for brain recovery, laying the groundwork for later studies on neuroplasticity and rehabilitation.
By 1978, studies began incorporating pharmacological compounds, as seen in the use of keywords like chlorpromazine and strychnine (Cummins et al., 1978). This prompted investigation on how EE interacts with neurochemicals related to arousal and activity. The exploration of drug-environment interactions indicated an emerging complexity in the understanding of how external stimuli, environmental and chemical, could combine to affect brain and behavior. Overall, the early period represents a transition in EE research, from behavioral observations to the exploration of social, developmental, and pharmacological factors, setting the stage for the more mechanistic and molecular investigations that emerged in the following decades.
The 1980s marked a shift toward exploring the neural effects of EE. In 1981, research began to explore how EE could influence neurodevelopment under challenging conditions. For example, studies looked at the effects of prenatal x-irradiation and microcephaly in conjunction with EE (Shibagaki et al., 1981; Kiyono et al., 1981). The focus on benzodiazepine receptors and tasks like the Hebb-Williams maze suggested that EE was being studied not only in relation to behavior but also in how it could impact specific neuroreceptors and cognitive outcomes in rodents. The combination of prenatal damage and EE interventions reflected an interest in how early-life environmental factors could shape later developmental trajectories. By 1982, the scope broadened to examine how EE influenced exploratory behavior, maze learning, and reactivity, particularly under conditions of environmental restriction and septal lesions (Engellenner et al., 1982). Researchers investigated how EE or its restriction affect both behavior and the brain structure, emphasizing the role of EE in mitigating the negative effects of brain lesions or environmental deficits. In 1983, a shift toward investigating the neurophysiological processes underlying the effects of EE was observed. Studies on cortical development, active sleep, and clonidine administration in infant rats indicated that EE was now being examined for its influence on developmental neuroplasticity and the interactions between environmental stimuli and pharmacological agents (Mirmiran and Uylings, 1983; Mirmiran et al., 1983).
By 1985, the keyword prenatal EE suggests that research had expanded to investigate how enrichment during prenatal development could influence outcomes like maze learning, pointing to an interest in the developmental origins of cognitive effects (Kiyono et al., 1985). In 1986, the use of terms like 6-hydroxydopamine and acetylcholine indicates that researchers began delving into specific neurochemical systems, such as dopamine and acetylcholine pathways, to understand how EE affects neurotransmitter functioning (Dunnett et al., 1986). The involvement of brain regions such as the fimbria-fornix, hippocampus, nucleus basalis magnocellularis, and septum indicated that EE was being investigated for its impact on learning, memory and attention-related pathways through these neurotransmitter systems.
In 1987 and 1988, the focus expanded to recovery from brain injuries. The use of keywords like compensation, contralateral neglect, hemidecorticate rat, and recovery by Rose et al. (1987, 1988) and Rose (1988) suggest their focus on investigating how EE helps in rehabilitating cognitive and motor functions after cerebral damage. The use of recovery and compensation as keywords suggests that EE was seen as a mechanism by which animals could adapt and recover functions that had been impaired by damage to the brain or nervous system. Behavioral assessments of learning and memory continued to play a central role, as indicated by the consistent use of the Hebb-Williams maze and discrimination reversal (Rose et al., 1988; Venable et al., 1988). This period also saw research on Macaca mulatta, signaling that the research had expanded beyond rodents to primates, further reinforcing the generalizability of findings related to EE across species (Reinhardt et al., 1988; Ross and Everitt, 1988).
By 1989, research became more focused on the cellular and anatomical effects of EE. Key terms such as basal dendrite, Golgi method, occipital cortex, and pyramidal neuron indicated that EE started being examined for its influence on dendritic morphology and neural connectivity (Venable et al., 1989). The emphasis on ethanol intake and its relationship to environmental conditions suggested that researchers were also beginning to explore how EE could interact with substance use and addictive behaviors (Rockman et al., 1989). Overall, the 1980s marked a crucial transition from examining behavioral outcomes to exploring the neural underpinnings of EE, particularly in the context of how enriched environments could affect brain structure, neurotransmitter systems, and compensatory mechanisms following neurological damage or deprivation. This decade laid the groundwork for the molecular and cellular studies of brain plasticity and repair that dominated EE research in the following years.
During the 1990s, there was increased emphasis on the interplay between stress, abnormal behaviors, and neurobiology in animal models. Early research in the 1990s primarily concentrated on the behavioral effects of EE, particularly in non-human primates, such as Macaca mulatta (Champoux et al., 1990; Line et al., 1990). Keywords such as abnormal, behavior, cortisol, serotonin, and stress reflected an increased focus on how EE influence the physiological stress response, particularly through the modulation of hormonal and neurotransmitter systems (Line et al., 1990; Widman et al., 1992; Carlstead and Shepherdson, 1994; Klein et al., 1994). Keywords from 1991, such as cage furnishings, further emphasize how environmental modifications like enriched cages affected animal wellbeing (Carlstead et al., 1991). Studies explored how EE could mitigate the negative effects of stress and promote better welfare for laboratory animals, as indicated by terms like single caging, object enrichment, and environment (Champoux et al., 1990). The studies in this early period also began to delve into cognitive recovery, with tasks like the Morris water maze being used to study learning and recovery of function, particularly in rodent models (Wainwright et al., 1994).
By the mid-1990s, there was a noticeable shift in the subjects of EE studies from primates to rodents. Researchers began to investigate the social aspect of EE. Keywords such as breeding colony, maternal behavior, aggression, and social restriction in 1995 highlighted a growing interest in the role of early experiences and social interactions in shaping behavior and development (Schapiro et al., 1995). Simultaneously, the neurophysiological mechanisms behind these behaviors were increasingly explored. Studies began to investigate corticosterone, testosterone, nerve growth factor, and fatty acid metabolism to understand how EE impacted brain structure and function (Barnard et al., 1996; Pham et al., 1999; Van de Weerd et al., 1997). Cognitive tasks like the Hebb-Williams maze and Lashley maze continued to feature prominently, emphasizing the growing recognition of the role of EE in enhancing cognitive performance and mitigating stress-related behaviors in laboratory animals (Boehm et al., 1996).
As EE research progressed into the late 1990s, the focus became more molecular, with an emphasis on understanding the neuroplastic and neurochemical mechanisms underlying the effects of enrichment. The keywords from 1998 and 1999 indicate a significant interest in glutamate receptors, NGF mRNA, and astrocytes, suggesting that researchers were increasingly investigating how EE affected brain plasticity at the cellular level (Torasdotter et al., 1998; Gagné et al., 1998; Soffi et al., 1999). At the same time, the species studied became more diverse, with EE research extending beyond rodents and primates to include dogs, turtles, guinea pigs, rabbits, and turkeys (Dean, 1999; Lidfors, 1997; Crowe and Forbes, 1999; Sherwin et al., 1999a,b; Burghardt et al., 1996). This expanded interest in applying EE paradigms across a variety of contexts and understanding its broader biological implications, such as the effects of EE on pecking behavior. Research on EE also started including other primates, such as apes and chimpanzees (Wood, 1998). There was also an increasing focus on welfare and ethical concerns, as terms like psychological wellbeing and regulatory toxicology became more prevalent in the literature (Dean, 1999; Burghardt et al., 1996; Wood, 1998; Boinski et al., 1999).
Overall, the decade from 1990 to 1999 saw a marked progression from addressing behavioral and stress responses in isolated or restricted environments to exploring the complex molecular, neurophysiological, and ethical dimensions of EE. The inclusion of some keywords, such as amphetamine, dopamine, and nucleus accumbens, by a group of scientists points to their focus on the neurochemical mechanisms underlying social behaviors and reward systems (Bowling et al., 1993; Bardo et al., 1995, 1999). The growing interest in neuroplasticity, neuronal health, and the molecular pathways that are influenced by EE helped researchers understand how enriched environments could promote better cognitive function, stress resilience, and overall wellbeing across various species. Overall, this decade saw a substantial shift toward understanding how EE influences both the social and neurochemical aspects of animal behavior, setting the stage for deeper molecular investigations in the 2000s, particularly in the context of mental health, neurobiological changes, and neurodevelopmental disorders, and thereby opened new avenues for both scientific inquiry and animal welfare practices.
In the decade between 2000 and 2009, EE research expanded significantly, with increasing focus on its effects on animal welfare, neuroplasticity, neurogenesis, neurodegeneration, behavior, and neurochemical processes across various animal models, including rodents, fish, pigs, rabbits, parrots, bats, and primates. Throughout the decade, keywords like neurotrophins, BDNF, and fibroblast growth factor indicate that studies were increasingly exploring how EE influences the expression of neurotrophic factors critical for synaptic plasticity and neuron growth (Ickes et al., 2000; Turner and Lewis, 2003; Will et al., 2004; Rossi et al., 2006; Angelucci et al., 2009).
At the beginning of the decade, research on EE placed a strong emphasis on animal welfare, particularly examining the effects of enriched environments on pigs. These studies highlighted improvements in learning, behavior, stress reduction, and circadian rhythm regulation under enriched condition (Beattie et al., 2000a,b; de Jong et al., 2000; Sneddon et al., 2000). Simultaneously, the cognitive and behavioral implications of EE were being explored extensively in rodent models, with a focus on enhanced memory, spatial learning, visual acuity, contextual processing, and stress resilience (Woodcock and Richardson, 2000; Varty et al., 2000; Prusky et al., 2000). This period also marked the initial investigations into the potential of EE to combat neurodegenerative diseases such as Alzheimer's and Parkinson's, with research demonstrating its role in reducing oxidative stress and improving cognitive performance. Key areas of focus included mitochondrial health and the impact of caloric intake on the progression of these diseases (Mattson et al., 2001).
As the decade progressed, studies delved deeper into the cellular mechanisms underlying the effects of EE, such as neuroplasticity and emotional reactivity, and addressed behaviors linked to addiction, social environments, and traumatic brain injury (Duffy et al., 2001; Hoplight et al., 2001; Passineau et al., 2001; Williams et al., 2001; Bardo et al., 2001). Rodent studies expanded to explore a wider range of behaviors, including anxiety, learning, novelty seeking, and exploration, while also underscoring the influence of EE on neurogenesis, dendritic spine density, and cellular markers like cytochrome oxidase (Fernández-Teruel et al., 2002a,b; Schrijver et al., 2002; Komitova et al., 2002; Johansson and Belichenko, 2002). EE research also began to include developmental and acquired conditions, such as traumatic brain injury and Down syndrome, suggesting its growing recognition as a potential therapeutic intervention for both genetic and environmental brain abnormalities (Hicks et al., 2002; Wagner et al., 2002; Martínez-Cué et al., 2002). The importance of social interaction was emphasized through comparisons of single and social housing models, illustrating how social components enhance the effects of EE (Schapiro, 2002). Similarly, the connection between EE and stress regulation became more prominent, with a focus on the modulation of the HPA axis and reductions in corticosterone levels. These findings emphasized the role of EE in mitigating physiological and behavioral stress responses (Belz et al., 2003; Morley-Fletcher et al., 2003). Additionally, research investigated how EE could influence immune functions, play behavior, and repetitive behaviors in transgenic mouse models, expanding understanding of its broad neuroendocrine and immunological impact (Marashi et al., 2003). Alzheimer's disease research remained a focal point, with studies revealing how EE reduces amyloid plaque buildup, enhances synaptic plasticity, and promotes neurogenesis through neurotrophins like BDNF, thereby supporting overall hippocampal health (Turner and Lewis, 2003; Jankowsky et al., 2003).
Around the middle of the decade, EE research placed more emphasis on neurotrophic factors like BDNF and fibroblast growth factor, linking them to synaptic plasticity and memory consolidation (Will et al., 2004). Researchers were increasingly interested in how enriched environments could modulate long-term potentiation, gene activation, and cellular processes like dendritic branching and synapse regulation (Keyvani et al., 2004; Cancedda et al., 2004; Johansson, 2004; Nithianantharajah et al., 2004). Researchers continued to focus on neurogenesis, synaptic plasticity, and the role of EE in conditions like traumatic brain injury and Alzheimer's disease (Bruel-Jungerman et al., 2005; Gaulke et al., 2005; Percaccio et al., 2005; Wagner et al., 2005; Jankowsky et al., 2005; Lambert et al., 2005). The introduction of auditory stimulation (Percaccio et al., 2005; Lutz and Novak, 2005) and epigenetic factors into EE research marked a new direction, indicating an interest in how environmental factors interact with sensory and genetic mechanisms to affect brain function (Friske and Gammie, 2005). EE research expanded further to include autism models (Schneider et al., 2006), and explored how enriched environments might influence behaviors related to stereotypy, anticipatory behaviors, and functional recovery after spinal cord injury (Schneider et al., 2006; Wood et al., 2006; Erschbamer et al., 2006). Researchers also examined how chronic stress impacted the effects of EE, particularly in relation to neurogenesis and HPA-axis regulation (Welberg et al., 2006). By 2007, the focus on brain plasticity became even more pronounced, with studies examining neurogenesis, synaptophysin levels, and the role of neurotransmitters like acetylcholine and dopamine in enriched environments (Mora et al., 2007; Niu et al., 2007; Fan et al., 2007; Leal-Galicia et al., 2007; Arco et al., 2007). The role of EE in recovery from brain injury, spinal cord injury, and ischemic stroke was a growing area of interest, highlighting its potential therapeutic applications (Berrocal et al., 2007; Pereira et al., 2007; Buchhold et al., 2007). Themes like behavioral synchronization (Scott et al., 2007) and anxiety-related behavior (Leal-Galicia et al., 2007; Iwata et al., 2007) were explored reflecting a broader consideration of welfare in enriched environments.
Toward the end of the decade, EE studies continued to focus on neurogenesis and synaptic transmission, with research incorporating neurotrophic factors, addiction models, and stress-related behaviors (Kondo et al., 2008; Parks et al., 2008; Rahman and Bardo, 2008; Brenes and Fornaguera, 2008; Qian et al., 2008; Segovia et al., 2008; Solinas et al., 2008). An increasing attention was given to the impact of EE on conditions like stroke (Plane et al., 2008; Wang et al., 2008), depression (Brenes and Fornaguera, 2008), Alzheimer's disease (Görtz et al., 2008; Herring et al., 2008), and schizophrenia (McOmish et al., 2008). Research also began to examine gene-environment interactions, exploring how EE could influence gene expression in response to stress, exercise, and neurodegenerative and neurodevelopmental conditions (Kondo et al., 2008; Herring et al., 2008; McOmish et al., 2008; Bernberg et al., 2008; Stam et al., 2008; Thiriet et al., 2008). There was also a growing interest in the role of EE in addiction, particularly how it could modulate drug-seeking behavior and relapse (Rahman and Bardo, 2008; Solinas et al., 2008; Smith et al., 2008; Grimm et al., 2008). By 2009, EE research had become highly diverse, exploring topics such as neurodegenerative diseases (Catlow et al., 2009; Herring et al., 2009; Mirochnic et al., 2009), addiction (Chauvet et al., 2009; El Rawas et al., 2009; Solinas et al., 2009; Thiel et al., 2009; Stairs and Bardo, 2009), and developmental plasticity (Cai et al., 2009; Caleo et al., 2009; Petrosini et al., 2009). Research emphasized the cellular effects of EE, focusing on dendritic spines (Goshen et al., 2009; Gelfo et al., 2009; Fréchette et al., 2009), synaptic reorganization (Caleo et al., 2009), and neuroplasticity (Darmopil et al., 2009). The role of EE in addiction models, including studies on drug self-administration and protracted abstinence, reflected an increasing interest in how environmental factors could modulate behavioral and physiological responses to drugs (Chauvet et al., 2009; Stairs and Bardo, 2009; Smith et al., 2009). The integration of advanced techniques like in vivo microdialysis (El Rawas et al., 2009), Magnetic Resonance Imaging (MRI) (Nag et al., 2009), and molecular biology methods, such as immunohistochemistry (Herring et al., 2009) provided a mechanistic understanding of how EE impacts neural circuits involved in various brain functions, offering new insights into its potential therapeutic applications.
Between 2010 and 2019, research on EE expanded significantly each year and focused on both the molecular and genetic mechanisms by which EE influences brain function. This underscores the importance of discussing the progression of EE research on a year-by-year basis.
In 2010, studies highlighted the roles of BDNF, cortisol, dopamine, and other neurochemicals, which are critical for neural plasticity, stress regulation, and emotional wellbeing, in relation to EE (Sun et al., 2010; Lonetti et al., 2010; Segovia et al., 2010; Munsterhjelm et al., 2010; Zajac et al., 2010). EE was shown to significantly improve spatial memory, reduce anxiety, and enhance cognition, with outcomes often linked to changes in neurotransmitter systems and neural circuit modulation (Sun et al., 2010; Nowakowska et al., 2010; Chen et al., 2010; Hughes and Collins, 2010; Sztainberg et al., 2010). The Morris Water Maze and Y-maze tasks assessed cognitive behavior, revealing substantial improvements in spatial learning and memory in enriched animals (Chen et al., 2010; Sparling et al., 2010). Additionally, the antidepressant effects of EE were explored using behavioral assays like the forced swim test, indicating its potential to alleviate depressive-like symptoms (Green et al., 2010). Notably, EE was explored in brain conditions such as Alzheimer's disease (Herring et al., 2010), drug addiction (Solinas et al., 2010), Rett syndrome (Lonetti et al., 2010; Kerr et al., 2010), and Huntington's disease (Wood et al., 2010; Benn et al., 2010). Rodent models remained central to this research, demonstrating how EE paradigms influence key brain areas like the hippocampus, nucleus accumbens, amygdala, frontal cortex, and striatum (Sun et al., 2010; Segovia et al., 2010; Zajac et al., 2010; Sztainberg et al., 2010; Mainardi et al., 2010). Advanced techniques such as gene expression profiling (including microarray and real-time RT-PCR), neuroimmune responses and neuroimaging were increasingly used to investigate the underlying molecular mechanisms that contribute to EE-induced changes in behaviors and brain plasticity (Kerr et al., 2010; Benn et al., 2010; Workman et al., 2010; Devonshire et al., 2010; Arranz et al., 2010). EE was also studied in non-rodent species, such as pigs and marsupials, to understand its impact on behavioral and physiological outcomes (Munsterhjelm et al., 2010; Oostindjer et al., 2010; Hogan et al., 2010). The interplay between EE, stress, and neural plasticity emerged as a critical theme (Hendriksen et al., 2010; Schloesser et al., 2010). The exploration of EE extended to complex neurobehavioral paradigms, such as incentive sensitization and conditioned place preference, which investigated its role in addiction and reward processing (Green et al., 2010). Techniques like in vivo imaging provided insights into how EE modulates neurogenesis, synaptogenesis, and neurotransmitter levels (Segovia et al., 2010; Mainardi et al., 2010). The growing use of transgenic and knockout mice, combined with a focus on transcriptional and epigenetic regulation, paved the way for a deeper understanding of how EE could be harnessed to develop therapeutic interventions for various neurological and psychiatric conditions (Zajac et al., 2010; Herring et al., 2010; Wood et al., 2010; Benn et al., 2010).
In 2011, EE research broadened considerably, emphasizing its effects on neurogenesis, neuroprotection, and recovery from neurological and psychiatric disorders, as well as stress-related conditions. Studies highlighted the role of EE in key brain regions, such as the hippocampus, amygdala, and prefrontal cortex, which are critical for learning, memory, executive functions, and emotional regulation (Munetsuna et al., 2011; Peng et al., 2011; Rawas et al., 2011; Wooters et al., 2011). EE interventions were shown to mitigate anxiety, aggression, and stereotypic behaviors by reshaping neural circuits (Kim and Sufka, 2011; Akre et al., 2011; Melotti et al., 2011). Research increasingly focused on neurodegenerative diseases, like Alzheimer's (Arranz et al., 2011; Rodríguez et al., 2011), and emphasized the ability of EE to alleviate prenatal stress (Peng et al., 2011), boost functional recovery (Zai et al., 2011), and promote social behavior (Workman et al., 2011) and neural resilience (Lehmann and Herkenham, 2011). EE also proved beneficial in neurodevelopmental disorders, with Down syndrome models, such as Ts65Dn mice, showing enhanced neural regeneration and cognitive improvement (Baamonde et al., 2011; Begenisic et al., 2011). Furthermore, the impact of EE on neuroinflammatory conditions was linked to decreased microglial activity (Lahiani-Cohen et al., 2011), and its influence on dopamine system underscored its effects on reward and motivational pathways (Wooters et al., 2011). Beyond rodents, EE improved welfare and cognitive function in zoo species. For instance, captive animals, including chimpanzees and parrots, exhibited reduced stress behaviors and heightened adaptability through the use of toys and structured spaces (de Andrade and de Azevedo, 2011; Zaragoza et al., 2011). Physical exercise integrated with EE was found to support neuronal survival and growth (Chakrabarti et al., 2011). On a molecular level, techniques like real-time RT-PCR (Munetsuna et al., 2011) and immunohistochemistry (Bednarek and Caroni, 2011; Lanosa et al., 2011) revealed that EE modulates neurogenesis and synaptogenesis markers, while epigenetic research confirmed its potential to induce lasting gene expression changes, providing a robust framework for long-term brain health and resilience (Lopez-Atalaya et al., 2011).
By 2012, EE research had evolved to incorporate a diverse range of molecular techniques, deepening the understanding of its neurobiological effects. Studies began to examine glial cell roles, specifically microglia and astrocytes, and the involvement of immune proteins, such as cytokines and chemokines (Williamson et al., 2012; Jurgens and Johnson, 2012). In Alzheimer's models, EE showed promising results in reducing amyloid-beta deposition and memory deficit (Maesako et al., 2012). Likewise, EE emerged as a potential intervention for traumatic brain injury, demonstrating efficacy in promoting functional recovery and reducing neuronal loss (Cheng et al., 2012; Kline et al., 2012). The research into stress hormones, including corticosterone and HPA axis regulation, explored the role of EE in alleviating physiological stress, with direct implications for treating anxiety and aggression (Du et al., 2012; Skwara et al., 2012; Hutchinson et al., 2012a,b). The hippocampus and prefrontal cortex remained a focal area, with findings that EE upregulates neurotrophins like BDNF, enhances synaptic connectivity, and improves learning and memory (Chourbaji et al., 2012; Rueda et al., 2012; Kumar et al., 2012; Zhang et al., 2012). EE was also linked to reductions in stereotypic and abnormal behaviors, underscoring its significance in improving animal wellbeing (Gross et al., 2012; Meagher and Mason, 2012). EE research expanded to explore aging, emphasizing its ability to combat cognitive decline and oxidative damage (Lee et al., 2012). EE also displayed potential in reducing drug-seeking behavior and preventing relapses, with research pointing to the modulation of dopamine system as central to these effects (Alvers et al., 2012; Gomez et al., 2012; Klaissle et al., 2012). Additionally, an in vivo study significantly advanced the understanding of how EE fosters brain plasticity and facilitates recovery processes (Buschler and Manahan-Vaughan, 2012).
In 2013, the role of EE on hippocampal neurogenesis was extensively studied, revealing how enriched conditions can stimulate the proliferation of neural progenitor cells and enhance dendritic spine density that translates into improved learning and memory (Pons-Espinal et al., 2013; Speisman et al., 2013; Tanti et al., 2013). EE was shown to mitigate age-related cognitive deficits, suggesting its potential to counteract the decline in neuroplasticity associated with aging and neurodegenerative diseases, such as Alzheimer's disease (Speisman et al., 2013; Barak et al., 2013; Beauquis et al., 2013). EE was shown to lower corticosterone levels (Garrido et al., 2013), modulate HPA axis (Sampedro-Piquero et al., 2013), and reduce anxiety-like behaviors in paradigms such as the elevated plus maze (Ravenelle et al., 2013) and elevated zero-maze (Sampedro-Piquero et al., 2013). Neurotransmitter systems, including dopamine, serotonin, and acetylcholine, were investigated to understand the role of EE in influencing emotional and cognitive processes. The regulation of dopamine receptors (Zeeb et al., 2013) and serotonin pathways (Renoir et al., 2013) contributed to the effects of EE on reward, motivation, and emotionality related behaviors. The role of cholinergic signaling in improving spatial learning and memory was also explored (Harati et al., 2013). EE was shown to mitigate symptoms and improve social and cognitive outcomes in models of autism (Reynolds et al., 2013) and research on genetic factors, such as Dyrk1a in Down syndrome models, provided insights into the gene-by-environment interactions that influence neurodevelopment and behavior (Pons-Espinal et al., 2013). EE influence on seasonal epigenetic regulation, specifically through histone variant H2A.Z, revealed a dynamic interplay between environmental factors and chromatin modifications that affect gene expression (Simonet et al., 2013). Furthermore, a systematic review introduced the concept of enviromimetics, or the use of environmental cues to mimic the benefits of physical exercise and cognitive stimulation (Pang and Hannan, 2013).
In 2014, the hippocampus remained a primary area of focus, given its critical role in learning, memory, and emotional regulation (Barichello et al., 2014; Morelli et al., 2014). The involvement of glucocorticoid receptors and the modulation of neuroimmune responses, such as reductions in cytokine levels, were explored to understand neuroprotective effects (Connors et al., 2014; Durairaj and Koilmani, 2014; Gurfein et al., 2014; Sampedro-Piquero et al., 2014). In Alzheimer's models like the App23 and 3 × Tg-AD mice, EE reduced amyloid-β accumulation and improved cognitive function, shedding light on the potential of non-pharmacological interventions to slow disease progression (Polito et al., 2014; Blázquez et al., 2014). The use of advanced techniques such as two-photon in vivo imaging and proteomics provided insights into the cellular and molecular changes induced by EE (Jung and Herms, 2014; Lichti et al., 2014). The continued study of neurotransmitter systems, including dopamine, serotonin, and acetylcholine, revealed how EE influences mood, motivation, and cognitive processes (Hofford et al., 2014; Batzina et al., 2014a,b; Lima et al., 2014). Models of drug addiction, including methamphetamine and nicotine sensitization, demonstrated that EE reduced drug-seeking behavior and altered neurochemical reactivity (Hofford et al., 2014; Hamilton et al., 2014). EE was shown to influence glial cell activation, promoting a shift from a pro-inflammatory to an anti-inflammatory phenotype. Microglial polarization, and by changes in cytokine expression such as TNF-α and IL-1β, contributed to a healthier neural environment (Pusic et al., 2014; Briones et al., 2014). Mechanisms such as DNA methylation were linked to experience-dependent plasticity, offering a deeper understanding of how environmental factors improve learning and memory in aged animals (Irier et al., 2014). The role of EE in autophagy and metabolism was also examined, with studies suggesting that enriched conditions may promote brain health through the regulation of cellular homeostasis and energy balance (Takahashi et al., 2014). Furthermore, the modulation of synaptic and structural plasticity in regions like the prefrontal cortex and nucleus accumbens was crucial for understanding the potential of EE in treating cognitive and emotional dysfunctions (Lichti et al., 2014; Fan et al., 2014; Mychasiuk et al., 2014). The use of EE in a model of epilepsy highlighted its impact on neuronal excitability and network stability, suggesting avenues for therapeutic applications (Morelli et al., 2014).
In 2015, the hippocampus remained a focal point, with studies highlighting the role of EE in enhancing neurogenesis, increasing synaptic plasticity, and improving learning and memory (Ahmadalipour et al., 2015; Morse et al., 2015; Soares et al., 2015; Valero-Aracama et al., 2015). BDNF upregulation was associated with increased hippocampal cell proliferation and dendritic spine density, supporting the idea that EE promotes brain plasticity and cognitive recovery (Ahmadalipour et al., 2015; Mosaferi et al., 2015; Novkovic et al., 2015). The modulation of signaling pathways, such as the extracellularly regulated kinase (ERK) and cAMP response element-binding protein (CREB), were found to link molecular changes to functional outcomes (Gomez et al., 2015; Matteucci et al., 2015). EE altered reward-related behaviors and reduced relapse rates in models of drug addiction, including methamphetamine and heroin dependence (Hajheidari et al., 2015a,b; Peck et al., 2015). Enrichment interventions, such as sensory and physical enrichment, were explored to reduce stereotypic behaviors like feather-pecking in birds (Clyvia et al., 2015; Hartcher et al., 2015). Studies on prenatal and early postnatal stress underscored the protective effects of EE, with enriched conditions mitigating the long-term impact of adverse experiences (Pascual et al., 2015; Zubedat et al., 2015). Similarly, sensory and social stimulation attenuated the age-related decline in the mRNA expression of hippocampal steroidogenic enzymes (Rossetti et al., 2015). The intersection of EE and physical activity was also explored, with studies demonstrating that combining exercise with cognitive enrichment amplifies the benefits on brain plasticity and overall wellbeing. This was particularly relevant for aging populations, where EE and exercise were shown to prevent cognitive decline and promote resilience against age-related diseases (Torres-Lista and Giménez-Llort, 2015). Model of social isolation highlighted the detrimental effects of deprivation, which could be reversed through enriched rearing conditions (Tanaś et al., 2015). Social interaction and positive reinforcement training in domesticated animals, such as cats and goats, were found to improve behavior and welfare (Oliveira et al., 2015; Rehnberg et al., 2015).
In 2016, the hippocampus and HPA axis continued to be the focal areas, as EE was shown to enhance neurogenesis, promote synaptic plasticity, reduce anxiety and improve memory and learning (Stein et al., 2016; Catuara-Solarz et al., 2016; Kapgal et al., 2016; Ronzoni et al., 2016). Studies examining early life stress and prenatal stress exposure found that EE could reverse or mitigate the negative effects on brain development and behavior, highlighting its therapeutic potential (do Prado et al., 2016; Rajesh et al., 2016). The use of paradigms like conditioned place preference provided insights into how EE can modulate reward pathways and prevent relapse (Mustroph et al., 2016). EE strategies like foraging enrichment were explored to improve welfare and reduce stereotypic behaviors (Coelho et al., 2016). A study showed that the benefits of EE could extend across generations, with offspring of enriched parents exhibiting better stress resilience, cognitive performance, and social behavior (Li et al., 2016). Dietary supplements, such as epigallocatechin-3-gallate and anthocyanins, were also studied in conjunction with EE, revealing potential synergistic effects on brain health and neuroprotection (Catuara-Solarz et al., 2016; Kreilaus et al., 2016). The use of techniques like high-throughput sequencing, transcriptomics, and proteomics provided insights into the molecular pathways involved in EE-induced recovery (Dowle et al., 2016; Zhang et al., 2016a,b).
In 2017, the hippocampus remained central to the EE research like previous years and the role of EE in alleviating anxiety, depression, and stress was extensively studied. Research into emotional wellbeing emphasized the role of glucocorticoid receptors and the epigenetic regulation of stress responses, with findings suggesting that EE could have long-term protective effects on mental health (Novaes et al., 2017; Shilpa et al., 2017; Yeshurun et al., 2017). In models of Alzheimer's disease, EE reduced amyloid accumulation and improved cognitive function (Salmin et al., 2017), while in models of traumatic brain injury, EE promoted functional recovery, and neural regeneration (Liu et al., 2017). Studies on addiction highlighted the role of EE in reducing drug-seeking behavior and craving, particularly for substances like cocaine, methamphetamine, and opioids (Gauthier et al., 2017; Hajheidari et al., 2017; Hofford et al., 2017). Paradigms such as cue-induced reinstatement provided insights into how EE can modulate reward pathways, offering potential therapeutic avenues for treating substance use disorders (Gauthier et al., 2017; Glueck et al., 2017). Studies on captive psittacines explored the use of enrichment objects and sensory stimulation to improve welfare and reduce stereotypic behaviors (Carvalho et al., 2017; Prathipa et al., 2017). EE was found to contribute to greater resilience against stress and psychiatric disorders like posttraumatic stress disorder (PTSD) (Novaes et al., 2017; Blanco et al., 2017; Nawaz et al., 2017). The intersection of EE with physical exercise was widely explored, as combining physical exercise with EE was shown to amplify neurogenic and neuroprotective effects (Rajab et al., 2017; Sakalem et al., 2017). The use of acetylcholinesterase inhibitors (de la Tremblaye et al., 2017), dopamine agonists (Glueck et al., 2017), and serotonin transporter (Rogers et al., 2017) in research provided insights into how EE affects synaptic transmission and receptor expression, offering new perspectives for treating psychiatric and neurodegenerative disorders.
In 2018, research on early-life stress, including maternal separation and juvenile isolation, revealed that EE could mitigate the long-term adverse effects on brain development and behavior, underscoring its potential as a non-pharmacological intervention for stress-related disorders (Dandi et al., 2018; Durán-Carabali et al., 2018; Borniger et al., 2018). EE was shown to counteract the negative effects of aging, and oxidative stress (Cintoli et al., 2018; Seo et al., 2018). Studies using paradigms such as conditioned place preference and self-administration showed that EE decreased drug-seeking behavior and craving for substances like alcohol, cocaine, and heroin (Freese et al., 2018; Imperio et al., 2018; Wang et al., 2018). The reduction in addictive behaviors was linked to changes in dopamine signaling and alterations in the nucleus accumbens, a key region involved in reward and motivation (Brown et al., 2018; Grimm et al., 2018). Studies on broiler chickens, pigs, and primates demonstrated that environmental stimulation, such as providing opportunities for natural behaviors, improved welfare and reduced harmful behaviors (Baxter et al., 2018; Chou et al., 2018; Costa et al., 2018). The influence of EE on epigenetic mechanisms and transgenerational inheritance continued to gain attention (Cintoli et al., 2018; Griñán-Ferr et al., 2018; Benito et al., 2018). EE was shown to induce changes in DNA methylation, histone modifications, and microRNA expression, which could be passed on to subsequent generations (Imperio et al., 2018; Benito et al., 2018). Neurodevelopment and early-life interventions were explored to understand the critical windows during which EE has the most significant impact (Durán-Carabali et al., 2018; Bator et al., 2018).
In 2019, research on EE continued to shed light on its substantial impact on behavior, brain function, and overall welfare, with a focus on both rodent models and practical applications for animals in agricultural and zoological settings. EE was shown to provide protection against the negative effects of chronic stress, adolescent stress, and early-life adversity, enhancing resilience and emotional regulation (González-Pardo et al., 2019a; Dixon and Hughes, 2019; Moradi-Kor et al., 2019). Research in rodent models showed significant reductions in anxiety- and depressive-like behaviors when exposed to EE, attributed to the upregulation of neurotrophic factors like BDNF and reductions in neuroinflammation (Singhal et al., 2019a,b; Wang et al., 2019). The role of glucocorticoids and serotonin in mediating the effects of EE on mood and behavior was also explored (Tatemoto et al., 2019; Lopes et al., 2019). The use of controlled cortical impact models demonstrated that EE could improve motor function, reduce brain tissue loss, and enhance synaptic connectivity (Besagar et al., 2019; Bleimeister et al., 2019; Lajud et al., 2019). In agricultural animals, such as broiler chickens, EE strategies that included straw provision, group housing, and sensory enrichment improved welfare, reduced stereotypic behaviors, and enhanced growth and production outcomes (Meyer et al., 2019; Vasdal et al., 2019). In zoo animals, enrichment items and structured activities were implemented to reduce stress, promote natural behaviors, and improve overall wellbeing (Fernandez and Timberlake, 2019; Regaiolli et al., 2019). The role of microglia in mediating neuroinflammatory responses to EE was also investigated, revealing that EE promotes an anti-inflammatory environment conducive to neuroprotection (Ali et al., 2019; Stuart et al., 2019). Studies on oxidative stress emphasized the role of antioxidant enzymes and dietary interventions, such as green tea extract, in enhancing the neuroprotective effects of EE (De Toma et al., 2019). The impact of EE on maternal separation highlighted its role in shaping wellbeing across the lifespan (Doreste-Mendez et al., 2019; González-Pardo et al., 2019b).
In conclusion, across 2010–2019, research into EE expanded significantly and focused on several key areas: neurotrophic factors and brain plasticity, neurogenesis and neuroprotection, stress and cortisol regulation, neurodegenerative diseases and cognitive decline, and spatial memory and learning. In the early part of the decade, keywords such as epigenetic, CREB, and transcription factors indicate a growing interest in how EE modulates gene expression and neural circuitry (Solinas et al., 2010; Lopez-Atalaya et al., 2011; Simonet et al., 2013; Matteucci et al., 2015; Xie et al., 2012). By the mid-2010s, the research expanded into deeper molecular explorations, with terms like microRNA (Ragu Varman et al., 2013; Pusic and Kraig, 2014; Gomez et al., 2016), DNA methylation (Rossetti et al., 2015), histone acetylation (Morse et al., 2015), and neuroinflammation (Jurgens and Johnson, 2012; Aranda et al., 2019) pointing to studies investigating how EE could influence genetic and inflammatory processes within the brain. Additionally, this decade saw an increased focus on psychiatric and neurological disorders, as reflected by increasing use of keywords such as depression, anxiety, addiction, and traumatic brain injury (Stein et al., 2016; Dandi et al., 2018; Singhal et al., 2019a; Rogers et al., 2017; Imperio et al., 2018; Wang et al., 2019; Ragu Varman et al., 2013; Stairs et al., 2016; Gong et al., 2018; Tomiga et al., 2016; Novaes et al., 2018; Alwis et al., 2016; Bondi and Kline, 2017). Behavioral assessments, such as the Morris water maze and elevated plus maze, continued to be used extensively to evaluate cognitive performance and anxiety-related behaviors in animals exposed to EE (Dandi et al., 2018; Rogers et al., 2017; Ragu Varman et al., 2013; Gong et al., 2018; Novaes et al., 2018). This period represents an important shift toward understanding how EE affects the brain on a genetic and molecular level, with growing implications for its use in treating neuropsychiatric and neurodegenerative conditions.
Analyzing the keywords from 2020 to 2024 reveals several emerging patterns and trends in EE research. This period saw ongoing research interest in brain plasticity, with terms like neurogenesis, synaptic plasticity, axon regeneration, and dentate gyrus increasingly becoming popular in EE studies (Khalil, 2024; Tang, 2020; de Oliveira et al., 2020; Yan et al., 2023). This trend highlights a growing interest in understanding how EE influences brain plasticity, particularly in diseases like Alzheimer's disease (Zhu et al., 2024), and anxiety and depression (Kimura et al., 2022; Wei et al., 2022). BDNF has been a consistent focal point, emphasizing research on how EE engages molecular pathways that promote neuroplasticity (Tan et al., 2021; Costa et al., 2023; Ismail et al., 2024). In particular, the BDNF-TrkB signaling pathway has been studied for its role in underpinning memory and cognition improvements associated with EE (Ismail et al., 2024). Moreover, neuroinflammation and oxidative stress are frequently featured, especially in studies investigating neuroprotection in affective disorders and neurodegenerative diseases (Kimura et al., 2022; Seo et al., 2020).
Behavioral and cognitive enhancements have also remained significant research themes. The role of EE in improving cognitive performance, learning, memory, and behavior is well-documented, with positive outcomes consistently measured through behavioral paradigms like the Barnes maze and elevated plus maze (Heimer-McGinn et al., 2020; Belo-Silva et al., 2024). By 2024, research focused on advanced cognitive functions, as indicated by keywords such as context, episodic memory, object recognition, pattern separation, and place cell (Pintori et al., 2022; Ventura et al., 2024; Riaz et al., 2021; Dickson et al., 2024). These terms suggest that studies were exploring how EE enhances higher-order cognitive processes, including memory and spatial learning. There has been a particular surge in research focused on adolescence and aging, suggesting that EE interventions might offer therapeutic potential across different stages of life, from age-related memory decline to enhancing emotional resilience and supporting developmental milestones (Song et al., 2021; Suárez et al., 2020; Dandi et al., 2022; Brancato et al., 2020).
Research has also expanded to investigate non-cognitive outcomes of EE, including immune functions and metabolic health. Keywords like inflammation, oxidative stress, and mitochondrial function highlight growing interest in the ways EE may improve overall physiological health (Seo et al., 2020; Lee et al., 2023; Durán-Carabali et al., 2024; Dwir et al., 2021; Réus et al., 2023). Emerging research into the gut-brain axis is also notable, as studies increasingly explore how EE could influence gut microbiota and immune responses, particularly in models of stress, aging, and neuroinflammation (Zhu et al., 2024, 2022; Orock et al., 2020; Colombino et al., 2021). The modulation of corticosterone and the regulation of the HPA axis continue to remain central themes, with EE often linked to reduced anxiety behaviors and improved emotional stability (Fan et al., 2021; Muthmainah et al., 2021). There is also a growing body of work on how EE can mitigate the negative impact of early life stress, especially in the context of emotional dysregulation (Borba et al., 2021; Rule et al., 2021; Xu et al., 2022).
The scope of EE research has broadened to include a wide range of species, extending beyond traditional rodent models to encompass swine, poultry, primates, sun bear, sea lions, zebrafish, turtles, dolphins, and marsupials. This expansion reflects an increasing recognition of the benefits of EE for animal welfare in agricultural and zoo settings. Keywords related to enrichment devices (Harvey-Carroll et al., 2023), social enrichment (Lauderdale and Miller, 2020), and physical enrichment (Brunet et al., 2023) are becoming more central, with research emphasizing the reduction of stereotypic behaviors and the overall enhancement of animal wellbeing (Donald et al., 2023; Abdul-Mawah et al., 2022). Furthermore, EE continues to be a prominent topic in studies on neurodevelopmental and neurodegenerative disorders, such as autism spectrum disorder (Queen et al., 2020; Favre et al., 2021) and Down syndrome (Toma et al., 2020; Alemany-González et al., 2022), as well as Alzheimer's disease (Wei et al., 2020; Nam et al., 2024) and Parkinson's disease (Seo et al., 2020; Kim et al., 2021). Research in this area focuses on how enriched environments can mitigate or reverse cognitive deficits, synaptic damage, and behavioral abnormalities, with the use of genetic models like APP/PS1 mice for Alzheimer's (Fulopova et al., 2021) and Ts65Dn mice for Down syndrome (Toma et al., 2020) underscoring the relevance of EE as a non-pharmacological intervention. Studies revealed sex-specific responses to EE (Dickson and Mittleman, 2021; Cosgrove et al., 2022; Marion et al., 2022; Monje-Reyna et al., 2022; Sabzalizadeh et al., 2022; Dandi et al., 2024; Weiner et al., 2024), emphasizing the need for tailored interventions.
Research on EE continued to investigate cellular and molecular pathways, while also expanded to explore more complex cognitive and social behaviors. Keywords such as PTEN, mTOR, and CREB-binding protein shows investigation on how EE affects intricate cellular mechanisms related to brain plasticity, neurogenesis, and synaptic function (Tang, 2020; Huang et al., 2020). The integration of novel technologies, like CRISPR-Cas9, expanded the understanding of EE associated molecular mechanisms (Sandoval-Quintana et al., 2023). Research on DNA methylation, histone acetylation, and microRNAs further reflects the trend of exploring how environmental factors like EE can induce lasting changes in gene expression (Wei et al., 2020; McKibben and Dwivedi, 2021; Arraes et al., 2024; Reiser et al., 2021; Lin et al., 2020).
Additionally, the therapeutic potential of EE has gained attention, particularly in the context of rehabilitation and functional recovery following stroke or traumatic brain injury (Lee et al., 2023; Lin et al., 2020; Zhu et al., 2021; de Boer et al., 2020). Terms like self-administration, addiction, and ventral tegmental area indicate an interest in how EE impacts reward systems and addiction-related behaviors (Nicolas et al., 2022; Galaj et al., 2023; Razavinasab et al., 2022). Simultaneously, the continued use of keywords like welfare, behavior, stress, anxiety, and social housing reflects a sustained commitment to improving animal wellbeing, with a focus on reducing stress and promoting natural behaviors. Overall, the current period features the integration of molecular, cognitive, and welfare facets in EE research, aiming to understand both the underlying biological mechanisms and the practical applications of EE in enhancing mental health and animal welfare.
Over the past five decades, research on EE has evolved from a simple focus on improving animal welfare in laboratory settings to a complex and multifaceted field. Initially aimed at understanding the effects of enriched environments on brain anatomy and behavior, the field has grown to include studies on neuroplasticity, gene-environment interactions, and the therapeutic potential of EE for neurological and psychiatric disorders. The stacked bar plot of the top 10 keywords in EE research from 1967 to August 2024, as shown in Figure 9, provide valuable insights into the evolving focus of the field. The Y-axis represents the frequency of keyword occurrences per year, with each bar section corresponding to one of the top keywords. This visualization highlights the increasing complexity and diversity of EE research topics over time. In earlier years, keywords such as behavior and welfare appear most prominently, indicating an initial focus on these aspects of EE. As research expanded, keywords like hippocampus, BDNF, and neurogenesis gained prominence, reflecting a shift toward understanding the neurobiological mechanisms of EE, particularly in terms of brain structure and plasticity. The notable occurrence of keywords such as aging, memory, and anxiety after 2010 indicates a growing interest in the therapeutic applications of EE for age-related cognitive decline, memory deficits, and mental health conditions over the past decade. Corticosterone, frequently studied alongside stress, underscores the focus on stress-related biomarkers and their modulation through EE. Overall, the stacked bar plot emphasizes the multidisciplinary nature of EE research, encompassing fields like neuroscience and animal welfare. This trend points toward an expanding scope of EE, with research increasingly focused on molecular mechanisms, neuroplasticity, and translational applications of EE for mental health and age-related brain disorders.
Figure 9. Top 10 Keywords in Environmental Enrichment Research (1967–August 2024). The figure presents a longitudinal analysis of the top 10 keywords associated with EE research from 1967 to August 2024.
As we move forward, EE research will likely continue to expand into new areas, including its applications for aging populations, neurodegenerative diseases, and mental health disorders. The growing focus on molecular mechanisms and epigenetics suggests that future studies will delve even deeper into how the environment shapes brain functions at the most fundamental levels. EE is not just about improving cognitive and emotional wellbeing in animals but is increasingly recognized as a powerful tool for promoting health across the lifespan, in both animals and humans.
Our bibliometric analysis shows significant contributions from a select group of researchers whose work has shaped the field of EE. These researchers represent diverse continents, highlighting the global interest in EE research. They have contributed to a broad spectrum of topics, ranging from behavioral sciences to neurorehabilitation and neuroplasticity. Notably, Anthony E. Kline emerged as the most central figure, with his extensive collaborative network and a substantial body of work that spans various aspects of EE, particularly in neurorehabilitation and neuroprotection (Bondi and Kline, 2017; Kline et al., 2007; Hoffman et al., 2008). Lamberto Maffei's work also stood out, particularly for his contributions to understanding neuroplasticity and changes in visual sensory processing induced by EE (Rossi et al., 2006; Cancedda et al., 2004; Baroncelli et al., 2013; Bartoletti et al., 2004). His high citation count reflects the substantial impact and relevance of his research in the field. Furthermore, the extensive collaboration network of Anthony J. Hannan indicates a strong focus on the neuropsychiatric implications of EE, linking genetic models with mental health outcomes (Singhal et al., 2019a,b, 2021, 2020). Gerd Kempermann's research, particularly on adult hippocampal neurogenesis (Fabel et al., 2009; Mirochnic et al., 2009; Kempermann et al., 2002), underscores the regenerative and organizational potential of the brain under enriched conditions. Similarly, others, such as Michael T. Bardo and Marcello Solinas, have highlighted the role of EE in addiction and reward system modulation through their research (El Rawas et al., 2009; Hofford et al., 2017; Green et al., 2002; Gipson et al., 2011; Nader et al., 2012).
These key authors not only advance the scientific understanding of EE but also play crucial roles in mentoring the next generation of researchers and shaping future research directions. Their extensive citations and collaborations highlight their influential positions within the scientific community, making their works pivotal in the ongoing research on the applications and benefits of EE.
The role of journals in disseminating EE research is critical. Applied Animal Behaviour Science and Behavioural Brain Research have been instrumental in bridging experimental research with clinical applications, emphasizing the translational aspect of EE studies. These journals not only lead in terms of document volume but also in influencing the research community, as seen in their citation impacts.
Journals like European Journal of Neuroscience and Journal of Neuroscience provide platforms for cutting-edge research that connects physiological findings with neurological implications of EE. Their focused contributions are pivotal in driving forward the understanding of how EE affects brain function and structure.
The presence of PLoS One highlights the role of open-access journals in enhancing the accessibility and impact of EE research across various scientific disciplines. This broadens the reach of research findings, facilitating cross-disciplinary research that enriches the field. There is also a strong presence of journals such as Physiology & Behavior and Psychopharmacology, which have contributed to understanding the role of enriched environments modulate hormonal responses, stress physiology, and behavioral outcomes. Additionally, journals like Developmental Psychobiology and Psychoneuroendocrinology have provided insights into how early-life environmental factors shape long-term neurobiological outcomes. Overall, these journals have collectively advanced the field, ensuring that findings reach a wide and varied scientific audience. This widespread dissemination fosters collaboration, innovation, and a comprehensive understanding of the role, benefits and mechanisms of EE across various species and contexts.
The analysis of contributions by country revealed a diverse and global effort in EE research. The United States leads in both publication volume and citations, reflecting its central role in the global EE research. However, the significant contributions from countries like Brazil, China, Australia and European nations underline a widespread international commitment to advancing EE research. Brazil's prominence in the network points to its active research community focused on animal welfare and neurobiology, while China's increasing contributions underscore its growing influence in neuroscience and behavioral studies. Australia's research efforts are closely related to translational studies that address both human and animal wellbeing. European countries such as the United Kingdom, Germany, Spain, France, and Italy also play major roles, with robust research networks and a strong focus on cross-disciplinary studies. These nations often collaborate with each other, creating a web of research network that facilitate knowledge exchange and innovation.
This geographic diversity is crucial as it introduces a variety of research perspectives and cultural contexts into EE studies, enhancing the generalizability and applicability of research findings. International collaborations, as seen in the co-authorship and citation networks, are vital for sharing resources, knowledge, and techniques, which facilitate advancements in research and practical implementations of EE principles.
The dynamic collaborations among key researchers, influential journals, and global contributions forms a robust framework that supports the continuous growth of EE research. This will ensure sustained progress and innovation in the field, helping to address new challenges and opportunities presented by evolving scientific and technological settings.
By continuing to build on the foundational work of leading authors and leveraging the strengths of top journals and diverse international contributions, the EE research community can enhance its impact on neuroscience, psychology, psychiatry and beyond. The integrative approach will not only advance the understanding of EE but also its practical applications in improving cognitive functions, mental health, and overall wellbeing in various populations.
Our bibliometric analysis of research on EE is extensive and informative, yet there are several limitations that require consideration. For instance, the study relied exclusively on data extracted from Scopus, which, despite its comprehensive coverage, may not include all relevant publications, especially those indexed in other significant databases like PubMed or Web of Science. While we acknowledge this limitation, we decided to rely solely on Scopus for several reasons. Scopus provides a wide range of high-quality peer-reviewed literature across various disciplines, including neuroscience, psychology, and behavioral sciences. This ensured that our analysis captured the core of EE research across multiple fields. Including additional databases, while potentially adding value, would have considerably increased the complexity of the data analysis without proportionally enhancing the quality or scope of the insights derived. By using a single, well-established database, consistency in the data curation process was maintained, avoiding potential redundancies that might arise from merging datasets from multiple sources. Expanding the analysis to multiple databases increases the risk of introducing duplicate entries and errors during data integration. This would require extensive data cleaning to remove duplicates and resolve inconsistencies, which could lead to potential inaccuracies and misinterpretation of the data. Bibliometric analyses rely heavily on the accurate extraction and analysis of metadata (e.g., titles, abstracts, keywords, and citations). Metadata formats can vary significantly across different databases, which could also lead to inconsistencies when merging datasets. Scopus provides well-structured metadata, ensuring uniformity and accuracy in the analysis process. Additionally, with nearly 1,850 articles included, we believe that our dataset is large and comprehensive enough to identify key trends, influential studies, and global contributions to EE research and can reflect patterns and trends on EE research with high accuracy.
It can be imagined that restricting the analysis to articles published in English could have excluded valuable research contributions available in other languages, however, it must be noted that 99.5 % (1,847 out of 1,856) of the indexed articles were published in English so the exclusion of non-English articles did not have any significant impact on the analysis.
We also recognize that citations are not always indicative of quality or scientific value, as they are influenced by various factors, including publication age and the network effect, where well-cited papers continue to attract more citations. Therefore, given the diversity of our dataset, we performed a broader analysis of publication patterns and research themes, which helped us understand the impact beyond mere citation metrics.
The decision to focus on the top 25 results in various categories for visualization purposes might have resulted in the exclusion of relevant but less cited or recently published authors, journals, and countries. It can be said that this approach could limit the representation of emerging trends and contributors who might be playing significant roles but have not yet gained extensive recognition. However, we believe that the primary purpose of bibliometric study is to highlight the most influential and active contributors, providing clear insights into the core areas of research and development, while still noting the potential for emerging trends in the broader discussion.
It is important to note that while our analysis highlights the global nature of EE research, the actual distribution might still be skewed toward institutions and countries with more resources and better access to large research databases. Since we targeted Scopus for our dataset, we could only focus on the publications that Scopus indexes.
This bibliometric analysis of research on EE provides a comprehensive overview of the significant contributions of key researchers, the crucial role of leading journals, and the global impact of studies across numerous countries. The findings underscore the importance of interdisciplinary approaches and international collaborations in advancing the understanding of the effects of EE on neural and cognitive outcomes.
From basic behavioral assessments in the late 1960s to complex molecular studies in the 2020s, EE research has significantly evolved, deepening the understanding of how enriched environments impact brain function and behavior. The study has demonstrated that EE research is not only a vibrant and dynamically growing area but also increasingly influential in shaping practices in neuro rehabilitation, animal welfare, and behavioral sciences. Future research is likely to continue exploring these molecular pathways and therapeutic applications, reinforcing the importance of EE in neuroscience and animal welfare studies.
The original contributions presented in the study are included in the article/Supplementary material, further inquiries can be directed to the corresponding author.
GS: Formal analysis, Writing – review & editing, Conceptualization, Data curation, Investigation, Methodology, Resources, Software, Visualization, Writing – original draft. BB: Formal analysis, Writing – review & editing, Funding acquisition, Project administration, Supervision, Validation.
The author(s) declare that no financial support was received for the research, authorship, and/or publication of this article.
The authors declare that the research was conducted in the absence of any commercial or financial relationships that could be construed as a potential conflict of interest.
The author(s) declared that they were an editorial board member of Frontiers, at the time of submission. This had no impact on the peer review process and the final decision.
All claims expressed in this article are solely those of the authors and do not necessarily represent those of their affiliated organizations, or those of the publisher, the editors and the reviewers. Any product that may be evaluated in this article, or claim that may be made by its manufacturer, is not guaranteed or endorsed by the publisher.
The Supplementary Material for this article can be found online at: https://www.frontiersin.org/articles/10.3389/fnbeh.2024.1501377/full#supplementary-material
Abdul-Mawah, S. S., Norma-Rashid, Y., and Ramli, R. (2022). The effect of environmental enrichment on sun bear (Herlarctos malayanus) behaviour in captivity. Sains Malays. 51, 1283–1291. doi: 10.17576/jsm-2022-5105-01
Aghighi Bidgoli, F., Salami, M., and Talaei, S. A. (2020). Environmental enrichment restores impaired spatial memory and synaptic plasticity in prenatally stress exposed rats: The role of GABAergic neurotransmission. Int. J. Dev. Neurosci. 80, 573–585. doi: 10.1002/jdn.10052
Ahmadalipour, A., Sadeghzadeh, J., Vafaei, A. A., Bandegi, A. R., Mohammadkhani, R., Rashidy-Pour, A., et al. (2015). Effects of environmental enrichment on behavioral deficits and alterations in hippocampal BDNF induced by prenatal exposure to morphine in juvenile rats. Neuroscience 305, 372–383. doi: 10.1016/j.neuroscience.2015.08.015
Akre, A. K., Bakken, M., Hovland, A. L., Palme, R., and Mason, G. (2011). Clustered environmental enrichments induce more aggression and stereotypic behaviour than do dispersed enrichments in female mice. Appl. Animal Behav. Sci. 131, 145–152. doi: 10.1016/j.applanim.2011.01.010
Alemany-González, M., Vilademunt, M., Gener, T., and Puig, M. V. (2022). Postnatal environmental enrichment enhances memory through distinct neural mechanisms in healthy and trisomic female mice. Neurobiol. Dis. 173:105841. doi: 10.1016/j.nbd.2022.105841
Ali, S., Liu, X., Queen, N. J., Patel, R. S., Wilkins, R. K., Mo, X., et al. (2019). Long-term environmental enrichment affects microglial morphology in middle age mice. Aging 11, 2388–2402. doi: 10.18632/aging.101923
Alvers, K. M., Marusich, J. A., Gipson, C. D., Beckmann, J. S., and Bardo, M. T. (2012). Environmental enrichment during development decreases intravenous self-administration of methylphenidate at low unit doses in rats. Behav. Pharmacol. 23, 650–657. doi: 10.1097/FBP.0b013e3283584765
Alwis, D. S., Yan, E. B., Johnstone, V., Carron, S., Hellewell, S., Morganti-Kossmann, M. C., et al. (2016). Environmental enrichment attenuates traumatic brain injury: Induced neuronal hyperexcitability in supragranular layers of sensory cortex. J. Neurotrauma 33, 1084–1101. doi: 10.1089/neu.2014.3774
Angelucci, F., De Bartolo, P., Gelfo, F., Foti, F., Cutuli, D., Boss,ù, P., et al. (2009). Increased concentrations of nerve growth factor and brain-derived neurotrophic factor in the rat cerebellum after exposure to environmental enrichment. Cerebellum 8, 499–506. doi: 10.1007/s12311-009-0129-1
Aranda, M. L., Fleitas, M. F. G., Dieguez, H. H., Milne, G. A., Devouassoux, J. D., Sarmiento, M. I. K., et al. (2019). Therapeutic benefit of environmental enrichment on optic neuritis. Neuropharmacology 145, 87–98. doi: 10.1016/j.neuropharm.2017.12.017
Arco, A. D., Segovia, G., Garrido, P., de Blas, M., and Mora, F. (2007). Stress, prefrontal cortex and environmental enrichment: Studies on dopamine and acetylcholine release and working memory performance in rats. Behav. Brain Res. 176, 267–273. doi: 10.1016/j.bbr.2006.10.006
Arraes, G. C., Barreto, F. S., Vasconcelos, G. S., Lima, C. N. d., da Silva, F. E. R., Ribeiro, W. L. C., et al. (2024). Long-term environmental enrichment normalizes schizophrenia-like abnormalities and promotes hippocampal slc6a4 promoter demethylation in mice submitted to a two-hit model. Neuroscience 551, 205–216. doi: 10.1016/j.neuroscience.2024.05.023
Arranz, L., De Castro, N. M., Baeza, I., Giménez-Llort, L., and Fuente, M. D. (2011). Effect of environmental enrichment on the immunoendocrine aging of male and female triple-transgenic 3xTg-AD mice for alzheimer's disease. J. Alzheimer's Dis. 25, 727–737. doi: 10.3233/JAD-2011-110236
Arranz, L., De Castro, N. M., Baeza, I., Maté, I., Viveros, M. P., Fuente, M. D., et al. (2010). Environmental enrichment improves age-related immune system impairment: Long-term exposure since adulthood increases life span in mice. Rejuvenation Res. 13, 415–428. doi: 10.1089/rej.2009.0989
Baamonde, C., Martínez-Cué, C., Flórez, J., and Dierssen, M. (2011). G-protein-associated signal transduction processes are restored after postweaning environmental enrichment in Ts65Dn, a down syndrome mouse model. Dev. Neurosci. 33, 442–450. doi: 10.1159/000329425
Barak, B., Shvarts-Serebro, I., Modai, S., Gilam, A., Okun, E., Michaelson, D. M., et al. (2013). Opposing actions of environmental enrichment and Alzheimer's disease on the expression of hippocampal microRNAs in mouse models. Transl. Psychiatry 3:e304. doi: 10.1038/tp.2013.77
Bardo, M., Klebaur, J., Valone, J., and Deaton, C. (2001). Environmental enrichment decreases intravenous self-administration of amphetamine in female and male rats. Psychopharmacology 155, 278–284. doi: 10.1007/s002130100720
Bardo, M. T., Bowling, S. L., Rowlett, J. K., Manderscheid, P., Buxton, S. T., Dwoskin, L. P., et al. (1995). Environmental enrichment attenuates locomotor sensitization, but not in vitro dopamine release, induced by amphetamine. Pharmacol. Biochem. Behav. 51, 397–405. doi: 10.1016/0091-3057(94)00413-D
Bardo, M. T., Valone, J. M., Robinet, P. M., Shaw, W. B., and Dwoskin, L. P. (1999). Environmental enrichment enhances the stimulant effect of intravenous amphetamine: search for a cellular mechanism in the nucleus accumbens. Psychobiology 27, 292–299. doi: 10.3758/BF03332123
Barichello, T., Fagundes, G. D., Generoso, J. S., Dagostin, C. S., Simões, L. R., Vilela, M. C., et al. (2014). Environmental enrichment restores cognitive deficits induced by experimental childhood meningitis. Rev. Brasil. Psiquiatr 36, 322–329. doi: 10.1590/1516-4446-2014-1443
Barnard, C. J., Behnke, J. M., and Sewell, J. (1996). Environmental enrichment, immunocompetence, resistance to Babesia microti in male mice. Physiol. Behav. 60, 1223–1231. doi: 10.1016/S0031-9384(96)00174-6
Baroncelli, L., Braschi, C., and Maffei, L. (2013). Visual depth perception in normal and deprived rats: Effects of environmental enrichment. Neuroscience 236, 313–319. doi: 10.1016/j.neuroscience.2013.01.036
Bartoletti, A., Medini, P., Berardi, N., and Maffei, L. (2004). Environmental enrichment prevents effects of dark-rearing in the rat visual cortex. Nat. Neurosci. 7, 215–216. doi: 10.1038/nn1201
Bator, E., Latusz, J., Wedzony, K., and Maćkowiak, M. (2018). Adolescent environmental enrichment prevents the emergence of schizophrenia-like abnormalities in a neurodevelopmental model of schizophrenia. Eur. Neuropsychopharmacol. 28, 97–108. doi: 10.1016/j.euroneuro.2017.11.013
Batzina, A., Dalla, C., Papadopoulou-Daifoti, Z., and Karakatsouli, N. (2014b). Effects of environmental enrichment on growth, aggressive behaviour and brain monoamines of gilthead seabream Sparus aurata reared under different social conditions. Compar. Biochem. Physiol. 169, 25–32. doi: 10.1016/j.cbpa.2013.12.001
Batzina, A., Dalla, C., Tsopelakos, A., Papadopoulou-Daifoti, Z., and Karakatsouli, N. (2014a). Environmental enrichment induces changes in brain monoamine levels in gilthead seabream Sparus aurata. Physiol. Behav. 130, 85–90. doi: 10.1016/j.physbeh.2014.03.023
Baumans, V. (2005). Environmental enrichment for laboratory rodents and rabbits: Requirements of rodents, rabbits, research. ILAR J. 46, 162–170. doi: 10.1093/ilar.46.2.162
Baxter, M., Bailie, C. L., and O'Connell, N. E. (2018). Evaluation of a dustbathing substrate and straw bales as environmental enrichments in commercial broiler housing. Appl. Anim. Behav. Sci. 200, 78–85. doi: 10.1016/j.applanim.2017.11.010
Beattie, V. E., O'connell, N. E., Kilpatrick, D. J., and Moss, B. W. (2000a). Influence of environmental enrichment on welfare-related behavioural and physiological parameters in growing pigs. Animal Sci. 70, 443–450. doi: 10.1017/S1357729800051791
Beattie, V. E., O'Connell, N. E., and Moss, B. W. (2000b). Influence of environmental enrichment on the behaviour, performance and meat quality of domestic pigs. Livestock Product. Sci. 65, 71–79. doi: 10.1016/S0301-6226(99)00179-7
Beauquis, J., Pavía, P., Pomilio, C., Vinuesa, A., Podlutskaya, N., Galvan, V., et al. (2013). Environmental enrichment prevents astroglial pathological changes in the hippocampus of APP transgenic mice, model of Alzheimer's disease. Exp. Neurol. 239, 28–37. doi: 10.1016/j.expneurol.2012.09.009
Beauquis, J., Roig, P., De Nicola, A. F., and Saravia, F. (2010). Short-term environmental enrichment enhances adult neurogenesis, vascular network and dendritic complexity in the hippocampus of type 1 diabetic mice. PLoS ONE 5. doi: 10.1371/journal.pone.0013993
Bednarek, E., and Caroni, P. (2011). β-adducin is required for stable assembly of new synapses and improved memory upon environmental enrichment. Neuron 69, 1132–1146. doi: 10.1016/j.neuron.2011.02.034
Begenisic, T., Spolidoro, M., Braschi, C., Baroncelli, L., Milanese, M., Pietra, G., et al. (2011). Environmental enrichment decreases gabaergic inhibition and improves cognitive abilities, synaptic plasticity and visual functions in a mouse model of down syndrome. Front. Cell. Neurosci. 5:29. doi: 10.3389/fncel.2011.00029
Belo-Silva, A. E., Silva, N. K. D. G. T., Marianno, P., de Araújo Costa, G., da Rovare, V. P., Bailey, A., et al. (2024). Effects of the combination of chronic unpredictable stress and environmental enrichment on anxiety-like behavior assessed using the elevated plus maze in Swiss male mice: hypothalamus-pituitary-adrenal axis-mediated mechanisms. Hormones Behav. 162:105538. doi: 10.1016/j.yhbeh.2024.105538
Belz, E. E., Kennell, J. S., Czambel, R. K., Rubin, R. T., and Rhodes, M. E. (2003). Environmental enrichment lowers stress-responsive hormones in singly housed male and female rats. Pharmacol. Biochem. Behav. 76, 481–486. doi: 10.1016/j.pbb.2003.09.005
Benito, E., Kerimoglu, C., Ramachandran, B., Pena-Centeno, T., Jain, G., Stilling, R. M., et al. (2018). RNA-dependent intergenerational inheritance of enhanced synaptic plasticity after environmental enrichment. Cell Rep. 23, 546–554. doi: 10.1016/j.celrep.2018.03.059
Benn, C. L., Luthi-Carter, R., Kuhn, A., Sadri-Vakili, G., Blankson, K. L., Dalai, S. C., et al. (2010). Environmental enrichment reduces neuronal intranuclear inclusion load but has no effect on messenger rna expression in a mouse model of huntington disease. J. Neuropathol. Exp. Neurol. 69, 817–827. doi: 10.1097/NEN.0b013e3181ea167f
Bernberg, E., Andersson, I. J., Gan, L.-m., Naylor, A. S., Johansson, M. E., and Bergström, G. (2008). Effects of social isolation and environmental enrichment on atherosclerosis in ApoE-/- mice. Stress 11, 381–389. doi: 10.1080/10253890701824051
Berrocal, Y., Pearse, D. D., Singh, A., Andrade, C. M., McBroom, J. S., Puentes, R., et al. (2007). Social and environmental enrichment improves sensory and motor recovery after severe contusive spinal cord injury in the rat. J. Neurotr. 24, 1761–1772. doi: 10.1089/neu.2007.0327
Besagar, S., Radabaugh, H. L., Bleimeister, I. H., Meyer, E. A., Niesman, P. J., Cheng, J. P., et al. (2019). Aripiprazole and environmental enrichment independently improve functional outcome after cortical impact injury in adult male rats, but their combination does not yield additional benefits. Exp. Neurol. 314, 67–73. doi: 10.1016/j.expneurol.2019.01.010
Birch, A. M., McGarry, N. B., and Kelly, A. M. (2013). Short-term environmental enrichment, in the absence of exercise, improves memory, and increases NGF concentration, early neuronal survival, and synaptogenesis in the dentate gyrus in a time-dependent manner. Hippocampus 23, 437–450. doi: 10.1002/hipo.22103
Blanco, M. B., De Souza, R. R., and Canto-De-souza, A. (2017). Effects of environmental enrichment in a mouse model of posttraumatic stress disorder. Psychol. Neurosci. 10, 225–242. doi: 10.1037/pne0000081
Blázquez, G., Cañete, T., Tobeña, A., Giménez-Llort, L., and Fernández-Teruel, A. (2014). Cognitive and emotional profiles of aged Alzheimer's disease (3 × TgAD) mice: Effects of environmental enrichment and sexual dimorphism. Behav. Brain Res. 268, 185–201. doi: 10.1016/j.bbr.2014.04.008
Bleimeister, I. H., Wolff, M., Lam, T. R., Brooks, D. M., Patel, R., Cheng, J. P., et al. (2019). Environmental enrichment and amantadine confer individual but nonadditive enhancements in motor and spatial learning after controlled cortical impact injury. Brain Res. 1714, 227–233. doi: 10.1016/j.brainres.2019.03.007
Boehm, G. W., Sherman, G. F., Hoplight, I. I. B. J., Hyde, L. A., Waters, N. S., Bradway, D. M., et al. (1996). Learning and memory in the autoimmune BXSB mouse: effects of neocortical ectopias and environmental enrichment. Brain Res. 726, 11–22. doi: 10.1016/0006-8993(96)00299-5
Boinski, S., Swing, S. P., Gross, T. S., and Davis, J. K. (1999). Environmental enrichment of brown capuchins (Cebus apella): behavioral and plasma and fecal cortisol measures of effectiveness. Am. J. Primatol. 48, 49–68. doi: 10.1002/(SICI)1098-2345(1999)48:1<49::AID-AJP4>3.0.CO;2-6
Bondi, C. O., and Kline, A. E. (2017). “Environmental enrichment: a preclinical model of neurorehabilitation for traumatic brain injury,” in Traumatic Brain Injury: Rehabilitation, Treatment, Case Management, Fourth Edition (CRC Press), 67–76. doi: 10.1201/9781315371351-5
Borba, L. A., Broseghini, L. D. R., Manosso, L. M., de Moura, A. B., Botelho, M. E. M., Arent, C. O., et al. (2021). Environmental enrichment improves lifelong persistent behavioral and epigenetic changes induced by early-life stress. J. Psychiatr. Res. 138, 107–116. doi: 10.1016/j.jpsychires.2021.04.008
Borniger, J. C., Ungerleider, K., Zhang, N., Karelina, K., Magalang, U. J., Weil, Z. M., et al. (2018). Repetitive brain injury of juvenile mice impairs environmental enrichment-induced modulation of REM sleep in adulthood. Neuroscience 375, 74–83. doi: 10.1016/j.neuroscience.2018.01.064
Bowling, S. L., Rowlett, J. K., and Bardo, M. T. (1993). The effect of environmental enrichment on amphetamine-stimulated locomotor activity, dopamine synthesis and dopamine release. Neuropharmacology 32, 885–893. doi: 10.1016/0028-3908(93)90144-R
Bramati, G., Stauffer, P., Nigri, M., Wolfer, D. P., and Amrein, I. (2023). Environmental enrichment improves hippocampus-dependent spatial learning in female C57BL/6 mice in novel IntelliCage sweet reward-based behavioral tests. Front. Behav. Neurosci. 17. doi: 10.3389/fnbeh.2023.1256744
Brancato, A., Castelli, V., Lavanco, G., and Cannizzaro, C. (2020). Environmental enrichment during adolescence mitigates cognitive deficits and alcohol vulnerability due to continuous and intermittent perinatal alcohol exposure in adult rats. Front. Behav. Neurosci. 14:583122. doi: 10.3389/fnbeh.2020.583122
Brenes, J. C., and Fornaguera, J. (2008). Effects of environmental enrichment and social isolation on sucrose consumption and preference: associations with depressive-like behavior and ventral striatum dopamine. Neurosci. Lett. 436, 278–282. doi: 10.1016/j.neulet.2008.03.045
Briones, T. L., Woods, J., and Rogozinska, M. (2014). Decreased neuroinflammation and increased brain energy homeostasis following environmental enrichment after mild traumatic brain injury is associated with improvement in cognitive function. Acta Neuropathol. Commun. 2, 1–10. doi: 10.1186/s40478-022-01380-1
Brown, R. W., Schlitt, M. A., Owens, A. S., DePreter, C. C., Cummins, E. D., Kirby, S. L., et al. (2018). Effects of environmental enrichment on nicotine sensitization in rats neonatally treated with quinpirole: analyses of glial cell line-derived neurotrophic factor and implications towards schizophrenia. Dev. Neurosci. 40, 64–72. doi: 10.1159/000486391
Bruel-Jungerman, E., Laroche, S., and Rampon, C. (2005). New neurons in the dentate gyrus are involved in the expression of enhanced long-term memory following environmental enrichment. Eur. J. Neurosci. 21, 513–521. doi: 10.1111/j.1460-9568.2005.03875.x
Brunet, V., Lafond, T., Kleiber, A., Lansade, L., Calandreau, L., Colson, V., et al. (2023). Environmental enrichment improves cognitive flexibility in rainbow trout in a visual discrimination task: first insights. Front. Veter. Sci. 10:1184296. doi: 10.3389/fvets.2023.1184296
Buchhold, B., Mogoanta, L., Suofu, Y., Hamm, A., Walker, L., Kessler, C., et al. (2007). Environmental enrichment improves functional and neuropathological indices following stroke in young and aged rats. Restor. Neurol. Neurosci. 25, 467–484.
Burghardt, G. M., Ward, B., and Rosscoe, R. (1996). Problem of reptile play: Environmental enrichment and play behavior in a captive Nile soft-shelled turtle, Trionyx triunguis. Zoo Biol. 15, 223–238. doi: 10.1002/(SICI)1098-2361(1996)15:3<223::AID-ZOO3>3.0.CO;2-D
Burnham, J. F. (2006). Scopus database: a review. Biomed. Digit. Libr. 3, 1–8. doi: 10.1186/1742-5581-3-1
Buschler, A., and Manahan-Vaughan, D. (2012). Brief environmental enrichment elicits metaplasticity of hippocampal synaptic potentiation in vivo. Front. Behav. Neurosci. 6:85. doi: 10.3389/fnbeh.2012.00085
Cabrera-Álvarez, M. J., Arechavala-Lopez, P., Mignucci, A., Oliveira, A. R., Soares, F., Saraiva, J. L., et al. (2024). Environmental enrichment reduces the effects of husbandry stressors in gilthead seabream broodstock. Aquaculture Rep. 37:102256. doi: 10.1016/j.aqrep.2024.102256
Cai, R., Guo, F., Zhang, J., Xu, J., Cui, Y., Sun, X., et al. (2009). Environmental enrichment improves behavioral performance and auditory spatial representation of primary auditory cortical neurons in rat. Neurobiol. Learn. Mem. 91, 366–376. doi: 10.1016/j.nlm.2009.01.005
Caleo, M., Tropea, D., Rossi, C., Gianfranceschi, L., and Maffei, L. (2009). Environmental enrichment promotes fiber sprouting after deafferentation of the superior colliculus in the adult rat brain. Exp. Neurol. 216, 515–519. doi: 10.1016/j.expneurol.2008.12.016
Cancedda, L., Putignano, E., Sale, A., Viegi, A., Berardi, N., Maffei, L., et al. (2004). Acceleration of visual system development by environmental enrichment. J. Neurosci. 24, 4840–4848. doi: 10.1523/JNEUROSCI.0845-04.2004
Carlstead, K., Seidensticker, J., and Baldwin, R. (1991). Environmental enrichment for zoo bears. Zoo Biol. 10, 3–16. doi: 10.1002/zoo.1430100103
Carlstead, K., and Shepherdson, D. (1994). Effects of environmental enrichment on reproduction. Zoo Biol. 13, 447–458. doi: 10.1002/zoo.1430130507
Carvalho, T. S. G., Saad, C. E. P., Alvarenga, R. R., Ferreira, W. M., Assis, V. D. L., Pereira, V. M. M. G., et al. (2017). Use of collard green stalks as environmental enrichment for cockatiels (Nymphicus hollandicus) kept in captivity. Arquivo Brasileiro de Medicina Veterinária e Zootecnia 69, 718–724. doi: 10.1590/1678-4162-8988
Catlow, B. J., Rowe, A. R., Clearwater, C. R., Mamcarz, M., Arendash, G. W., Sanchez-Ramos, J., et al. (2009). Effects of environmental enrichment and physical activity on neurogenesis in transgenic PS1/APP mice. Brain Res. 1256, 173–179. doi: 10.1016/j.brainres.2008.12.028
Catuara-Solarz, S., Espinosa-Carrasco, J., Erb, I., Langohr, K., Gonzalez, J. R., Notredame, C., et al. (2016). Combined treatment with environmental enrichment and (-)-epigallocatechin-3-gallate ameliorates learning deficits and hippocampal alterations in a mouse model of down syndrome. eNeuro 3:103. doi: 10.1523/ENEURO.0103-16.2016
Chakrabarti, L., Scafidi, J., Gallo, V., and Haydar, T. F. (2011). Environmental enrichment rescues postnatal neurogenesis defect in the male and female Ts65Dn mouse model of down syndrome. Dev. Neurosci. 33, 428–441. doi: 10.1159/000329423
Champoux, M., Digregorio, G., Schneider, M. L., and Suomi, S. J. (1990). Inanimate environmental enrichment for group-housed rhesus macaque infants. Am. J. Primatol. 22, 61–67. doi: 10.1002/ajp.1350220104
Chauvet, C., Lardeux, V., Goldberg, S. R., Jaber, M., and Solinas, M. (2009). Environmental enrichment reduces cocaine seeking and reinstatement induced by cues and stress but not by cocaine. Neuropsychopharmacology 34, 2767–2778. doi: 10.1038/npp.2009.127
Chen, Y., Mao, Y., Zhou, D., Hu, X., Wang, J., Ma, Y., et al. (2010). Environmental enrichment and chronic restraint stress in ICR mice: effects on prepulse inhibition of startle and Y-maze spatial recognition memory. Behav. Brain Res. 212, 49–55. doi: 10.1016/j.bbr.2010.03.033
Cheng, J. P., Shaw, K. E., Monaco, C. M., Hoffman, A. N., Sozda, C. N., Olsen, A. S., et al. (2012). A relatively brief exposure to environmental enrichment after experimental traumatic brain injury confers long-term cognitive benefits. J. Neurotrauma 29, 2684–2688. doi: 10.1089/neu.2012.2560
Chou, J. Y., D'Eath, R. B., Sandercock, D. A., Waran, N., Haigh, A., O'Driscoll, K., et al. (2018). Use of different wood types as environmental enrichment to manage tail biting in docked pigs in a commercial fully-slatted system. Livestock Sci. 213, 19–27. doi: 10.1016/j.livsci.2018.04.004
Chourbaji, S., Hörtnagl, H., Molteni, R., Riva, M. A., Gass, P., Hellweg, R., et al. (2012). The impact of environmental enrichment on sex-specific neurochemical circuitries - Effects on brain-derived neurotrophic factor and the serotonergic system. Neuroscience 220, 267–276. doi: 10.1016/j.neuroscience.2012.06.016
Cintoli, S., Cenni, M. C., Pinto, B., Morea, S., Sale, A., Maffei, L., et al. (2018). Environmental enrichment induces changes in long-term memory for social transmission of food preference in aged mice through a mechanism associated with epigenetic processes. Neural Plast. 2018:3725087. doi: 10.1155/2018/3725087
Clyvia, A., Faggioli, A. B., and Cipreste, C. F. (2015). Effects of environmental enrichment in a captive pair of golden parakeet (Guaruba guarouba, Psittacidae) with abnormal behaviors. Rev. Bras. Ornitol. 23, 309–314. doi: 10.1007/BF03544297
Coelho, C. M., de Azevedo, C. S., Guimarães, M. A. D. B. V., and Young, R. J. (2016). Environmental enrichment effect on fecal glucocorticoid metabolites and captive maned wolf (Chrysocyon brachyurus) behavior. J. Appl. Animal Welfare Sci. 19, 353–362. doi: 10.1080/10888705.2016.1161512
Colombino, E., Biasato, I., Ferrocino, I., Bellezza Oddon, S., Caimi, C., Gariglio, M., et al. (2021). Effect of insect live larvae as environmental enrichment on poultry gut health: gut mucin composition, microbiota and local immune response evaluation. Animals 11:2819. doi: 10.3390/ani11102819
Connors, E. J., Shaik, A. N., Migliore, M. M., and Kentner, A. C. (2014). Environmental enrichment mitigates the sex-specific effects of gestational inflammation on social engagement and the hypothalamic pituitary adrenal axis-feedback system. Brain, Behav. Immun. 42, 178–190. doi: 10.1016/j.bbi.2014.06.020
Cosgrove, J. A., Kelly, L. K., Kiffmeyer, E. A., and Kloth, A. D. (2022). Sex-dependent influence of postweaning environmental enrichment in Angelman syndrome model mice. Brain Behav. 12:e2468. doi: 10.1002/brb3.2468
Costa, G. A., Silva, NKdT., Marianno, P., Chivers, P., Bailey, A., and Camarini, R. (2023). Environmental enrichment increased bdnf transcripts in the prefrontal cortex: implications for an epigenetically controlled mechanism. Neuroscience 526, 277–289. doi: 10.1016/j.neuroscience.2023.07.001
Costa, R., Sousa, C., and Llorente, M. (2018). Assessment of environmental enrichment for different primate species under low budget: a case study. J. Appl. Animal Welf. Sci. 21, 185–199. doi: 10.1080/10888705.2017.1414606
Crowe, R., and Forbes, J. M. (1999). Effects of four different environmental enrichment treatments on pecking behaviour in turkeys. Br. Poult. Sci. 40, 11–12. doi: 10.1080/00071669986558
Cummins, R. A., Carlyon, T. N., and Walsh, R. N. (1978). Drug-modulated behavioural responses to environmental enrichment. Psychopharmacology 58, 197–199. doi: 10.1007/BF00426907
Dandi, E., Kalamari, A., Touloumi, O., Lagoudaki, R., Nousiopoulou, E., Simeonidou, C., et al. (2018). Beneficial effects of environmental enrichment on behavior, stress reactivity and synaptophysin/BDNF expression in hippocampus following early life stress. Int. J. Dev. Neurosci. 67, 19–32. doi: 10.1016/j.ijdevneu.2018.03.003
Dandi, E., Kesidou, E., Simeonidou, C., Spandou, E., Grigoriadis, N., Tata, D. A., et al. (2024). Sex-specific differences and the role of environmental enrichment in the expression of hippocampal CB1 receptors following chronic unpredictable stress. Brain Sci. 14:357. doi: 10.3390/brainsci14040357
Dandi, E., Spandou, E., and Tata, D. A. (2022). Investigating the role of environmental enrichment initiated in adolescence against the detrimental effects of chronic unpredictable stress in adulthood: Sex-specific differences in behavioral and neuroendocrinological findings. Behav. Proc. 200:104707. doi: 10.1016/j.beproc.2022.104707
Darmopil, S., Petanjek, Z., Mohammed, A. H., and Bogdanović, N. (2009). Environmental enrichment alters dentate granule cell morphology in oldest-old rat. J. Cell. Mol. Med. 13, 1845–1856. doi: 10.1111/j.1582-4934.2008.00560.x
Darna, M., Beckmann, J. S., Gipson, C. D., Bardo, M. T., and Dwoskin, L. P. (2015). Effect of environmental enrichment on dopamine and serotonin transporters and glutamate neurotransmission in medial prefrontal and orbitofrontal cortex. Brain Res. 1599, 115–125. doi: 10.1016/j.brainres.2014.12.034
de Andrade, A. A., and de Azevedo, C. S. (2011). Effects of environmental enrichment in the diminution of abnormal behaviours exhibited by captive bluefronted Amazon parrots (Amazona aestiva, Psittacidae). Rev. Bras. Ornitol. 19, 56–62.
de Boer, A., Storm, A., Gomez-Soler, M., Smolders, S., Rué, L., Poppe, L., et al. (2020). Environmental enrichment during the chronic phase after experimental stroke promotes functional recovery without synergistic effects of EphA4 targeted therapy. Hum. Mol. Genet. 29, 605–617. doi: 10.1093/hmg/ddz288
de Jong, I. C., Prelle, I. T., van de Burgwal, J. A., Lambooij, E., Korte, S. M., Blokhuis, H. J., et al. (2000). Effects of environmental enrichment on behavioral responses to novelty, learning, and memory, and the circadian rhythm in cortisol in growing pigs. Physiol. Behav. 68, 571–578. doi: 10.1016/S0031-9384(99)00212-7
de la Tremblaye, P. B., Bondi, C. O., Lajud, N., Cheng, J. P., Radabaugh, H. L., and Kline, A. E. (2017). Galantamine and environmental enrichment enhance cognitive recovery after experimental traumatic brain injury but do not confer additional benefits when combined. J. Neurotr. 34, 1610–1622. doi: 10.1089/neu.2016.4790
de Oliveira, T. C. G., Carvalho-Paulo, D., de Lima, C. M., de Oliveira, R. B., Filho, C. S., Diniz, D. G., et al. (2020). Long-term environmental enrichment reduces microglia morphological diversity of the molecular layer of dentate gyrus. Eur. J. Neurosci. 52, 4081–4099. doi: 10.1111/ejn.14920
De Toma, I., Ortega, M., Aloy, P., Sabido, E., and Dierssen, M. (2019). DYRK1A overexpression alters cognition and neural-related proteomic pathways in the hippocampus that are rescued by green tea extract and/or environmental enrichment. Front. Molec. Neurosci. 12:272. doi: 10.3389/fnmol.2019.00272
Dean, S. W. (1999). Environmental enrichment of laboratory animals used in regulatory toxicology studies. Lab. Anim. 33, 309–327. doi: 10.1258/002367799780487823
Derviş, H. (2019). Bibliometric analysis using bibliometrix an R package. J. Scientometr. Res. 8, 156–160. doi: 10.5530/jscires.8.3.32
Devonshire, I. M., Dommett, E. J., Grandy, T. H., Halliday, A. C., and Greenfield, S. A. (2010). Environmental enrichment differentially modifies specific components of sensory-evoked activity in rat barrel cortex as revealed by simultaneous electrophysiological recordings and optical imaging in vivo. Neuroscience 170, 662–669. doi: 10.1016/j.neuroscience.2010.07.029
Dickson, E. J., Monk, J. E., Lee, C., and Campbell, D. L. (2024). Environmental enrichment during yard weaning alters the performance of calves in an attention bias and a novel object recognition test. Front. Animal Sci. 5:1364259. doi: 10.3389/fanim.2024.1364259
Dickson, P. E., and Mittleman, G. (2021). Strain and sex dependent effects of isolation housing relative to environmental enrichment on operant sensation seeking in mice. Sci. Rep. 11:17826. doi: 10.1038/s41598-021-97252-0
Dixon, E. I., and Hughes, R. N. (2019). Treatment with 1-benzylpiperazine (BZP) during adolescence of male and female hooded rats exposed to environmental enrichment: subsequent behavioral outcomes. Int. J. Dev. Neurosci. 73, 32–40. doi: 10.1016/j.ijdevneu.2018.12.005
do Prado, C. H., Narahari, T., Holland, F. H., Lee, H. N., Murthy, S. K., and Brenhouse, H. C. (2016). Effects of early adolescent environmental enrichment on cognitive dysfunction, prefrontal cortex development, and inflammatory cytokines after early life stress. Dev. Psychobiol. 58, 482–491. doi: 10.1002/dev.21390
Donald, K., Benedetti, A., Goulart, V. D. L. R., Deming, A., Nollens, H., Stafford, G., et al. (2023). Environmental enrichment devices are safe and effective at reducing undesirable behaviors in California sea lions and northern elephant seals during rehabilitation. Animals 13:1222. doi: 10.3390/ani13071222
Donthu, N., Kumar, S., Mukherjee, D., Pandey, N., and Lim, W. M. (2021). How to conduct a bibliometric analysis: an overview and guidelines. J. Business Res. 133, 285–296. doi: 10.1016/j.jbusres.2021.04.070
Doreste-Mendez, R., Ríos-Ruiz, E. J., Rivera-López, L. L., Gutierrez, A., and Torres-Reveron, A. (2019). Effects of environmental enrichment in maternally separated rats: age and sex-specific outcomes. Front. Behav. Neurosci. 13:198. doi: 10.3389/fnbeh.2019.00198
Dowle, E. J., Pochon, X., Banks, J. C., Shearer, K., and Wood, S. A. (2016). Targeted gene enrichment and high-throughput sequencing for environmental biomonitoring: a case study using freshwater macroinvertebrates. Mol. Ecol. Resour. 16, 1240–1254. doi: 10.1111/1755-0998.12488
Du, X., Leang, L., Mustafa, T., Renoir, T., Pang, T. Y., and Hannan, A. J. (2012). Environmental enrichment rescues female-specific hyperactivity of the hypothalamic-pituitary-adrenal axis in a model of Huntington's disease. Transl. Psychiat. 2:e133. doi: 10.1038/tp.2012.58
Duffy, S. N., Craddock, K. J., Abel, T., and Nguyen, P. V. (2001). Environmental enrichment modifies the PKA-dependence of hippocampal LTP and improves hippocampus-dependent memory. Learn. Memory 8, 26–34. doi: 10.1101/lm.36301
Dunnett, S. B., Whishaw, I. Q., Bunch, S. T., and Fine, A. (1986). Acetylcholine-rich neuronal grafts in the forebrain of rats: effects of environmental enrichment, neonatal noradrenaline depletion, host transplantation site and regional source of embryonic donor cells on graft size and acetylcholinesterase-positive fibre outgrowth. Brain Res. 378, 357–373. doi: 10.1016/0006-8993(86)90939-X
Durairaj, R. V., and Koilmani, E. R. (2014). Environmental enrichment modulates glucocorticoid receptor expression and reduces anxiety in Indian field male mouse Mus booduga through up-regulation of microRNA-124a. Gen. Comp. Endocrinol. 199, 26–32. doi: 10.1016/j.ygcen.2014.01.005
Durán-Carabali, L. E., Arcego, D. M., Odorcyk, F. K., Reichert, L., Cordeiro, J. L., Sanches, E. F., et al. (2018). Prenatal and early postnatal environmental enrichment reduce acute cell death and prevent neurodevelopment and memory impairments in rats submitted to neonatal hypoxia ischemia. Mol. Neurobiol. 55, 3627–3641. doi: 10.1007/s12035-017-0604-5
Durán-Carabali, L. E., Odorcyk, F. K., Grun, L. K., Schmitz, F., Junior, O. R., de Oliveria, C. A., et al. (2024). Maternal environmental enrichment protects neonatal brains from hypoxic-ischemic challenge by mitigating brain energetic dysfunction and modulating glial cell responses. Exper. Neurol. 374:114713. doi: 10.1016/j.expneurol.2024.114713
Dwir, D., Cabungcal, J. H., Xin, L., Giangreco, B., Parietti, E., Cleusix, M., et al. (2021). Timely N-acetyl-cysteine and environmental enrichment rescue oxidative stress-induced parvalbumin interneuron impairments via MMP9/RAGE pathway: a translational approach for early intervention in psychosis. Schizophr. Bull. 47, 1782–1794. doi: 10.1093/schbul/sbab066
El Rawas, R., Thiriet, N., Lardeux, V., Jaber, M., and Solinas, M. (2009). Environmental enrichment decreases the rewarding but not the activating effects of heroin. Psychopharmacology 203, 561–570. doi: 10.1007/s00213-008-1402-6
Ellegaard, O., and Wallin, J. A. (2015). The bibliometric analysis of scholarly production: how great is the impact? Scientometrics 105, 1809–1831. doi: 10.1007/s11192-015-1645-z
Engellenner, W. J., Goodlett, C. R., Burright, R. G., and Donovick, P. J. (1982). Environmental enrichment and restriction: effects on reactivity, exploration and maze learning in mice with septal lesions. Physiol. Behav. 29, 885–893. doi: 10.1016/0031-9384(82)90339-0
Erschbamer, M. K., Pham, T. M., Zwart, M. C., Baumans, V., and Olson, L. (2006). Neither environmental enrichment nor voluntary wheel running enhances recovery from incomplete spinal cord injury in rats. Exp. Neurol. 201, 154–164. doi: 10.1016/j.expneurol.2006.04.003
Fabel, K., Wolf, S., Ehninger, D., Babu, H., Galicia, P., and Kempermann, G. (2009). Additive effects of physical exercise and environmental enrichment on adult hippocampal neurogenesis in mice. Front. Neurosci. 3:994. doi: 10.3389/neuro.22.002.2009
Fan, C., Zhang, M., Shang, L., Cynthia, N. A., Li, Z., Yang, Z., et al. (2014). Short-term environmental enrichment exposure induces proliferation and maturation of doublecortin-positive cells in the prefrontal cortex. Neural Regener. Res. 9, 318–328. doi: 10.4103/1673-5374.128231
Fan, Y., Liu, Z., Weinstein, P. R., Fike, J. R., and Liu, J. (2007). Environmental enrichment enhances neurogenesis and improves functional outcome after cranial irradiation. Eur. J. Neurosci. 25, 38–46. doi: 10.1111/j.1460-9568.2006.05269.x
Fan, Z., Chen, J., Li, L., Wang, H., Gong, X., Xu, H., et al. (2021). Environmental enrichment modulates HPA axis reprogramming in adult male rats exposed to early adolescent stress. Neurosci. Res. 172, 63–72. doi: 10.1016/j.neures.2021.04.007
Favre, M. R., Mendola, D. L., Meystre, J., Christodoulou, D., Cochrane, M. J., Markram, H., et al. (2021). The effect of environmental enrichment with autistic-like behavior symptoms on a rattus norvegicus model. Open Access Maced. J. Med. Sci. 9, 78–81. doi: 10.3889/oamjms.2021.5616
Fernandez, E. J., and Timberlake, W. (2019). Selecting and testing environmental enrichment in lemurs. Front. Psychol. 10:2119. doi: 10.3389/fpsyg.2019.02119
Fernández-Teruel, A., Driscoll, P., Gil, L., Aguilar, R., Tobeña, A., Escorihuela, R. M., et al. (2002a). Enduring effects of environmental enrichment on novelty seeking, saccharin and ethanol intake in two rat lines (RHA/Verh and RLA/Verh) differing in incentive-seeking behavior. Pharmacol. Biochem. Behav. 73, 225–231. doi: 10.1016/S0091-3057(02)00784-0
Fernández-Teruel, A., Giménez-Llort, L., Escorihuela, R. M., Gil, L., Aguilar, R., Steimer, T., et al. (2002b). Early-life handling stimulation and environmental enrichment: Are some of their effects mediated by similar neural mechanisms? Pharmacol. Biochem. Behav. 73, 233–245. doi: 10.1016/S0091-3057(02)00787-6
Fréchette, M., Rennie, K., and Pappas, B. A. (2009). Developmental forebrain cholinergic lesion and environmental enrichment: behaviour, CA1 cytoarchitecture and neurogenesis. Brain Res. 1252, 172–182. doi: 10.1016/j.brainres.2008.11.082
Freese, L., Almeida, F. B., Heidrich, N., Hansen, A. W., Steffens, L., Steinmetz, A., et al. (2018). Environmental enrichment reduces cocaine neurotoxicity during cocaine-conditioned place preference in male rats. Pharmacol. Biochem. Behav. 169, 10–15. doi: 10.1016/j.pbb.2018.04.001
Friske, J. E., and Gammie, S. C. (2005). Environmental enrichment alters plus maze, but not maternal defense performance in mice. Physiol. Behav. 85, 187–194. doi: 10.1016/j.physbeh.2005.03.022
Fulopova, B., Stuart, K. E., Bennett, W., Bindoff, A., King, A. E., Vickers, J. C., et al. (2021). Regional differences in beta amyloid plaque deposition and variable response to midlife environmental enrichment in the cortex of APP/PS1 mice. J. Compar. Neurol. 529, 1849–1862. doi: 10.1002/cne.25060
Gagné, J., Gélinas, S., Martinoli, M. G., Foster, T. C., Ohayon, M., Thompson, R. F., et al. (1998). AMPA receptor properties in adult rat hippocampus following environmental enrichment. Brain Res. 799, 16–25. doi: 10.1016/S0006-8993(98)00451-X
Galaj, E., Barrera, E. D., Persaud, K., Nisanov, R., Vashisht, A., Goldberg, H., et al. (2023). The impact of heroin self-administration and environmental enrichment on ventral tegmental CRF1 receptor expression. Int. J. Neuropsychopharmacol. 26, 828–839. doi: 10.1093/ijnp/pyad060
Gardner, E. B., Boitano, J. J., Mancino, N. S., D'Amico, D. P., and Gardner, E. L. (1975). Environmental enrichment and deprivation: effects on learning, memory and exploration. Physiol. Behav. 14, 321–327. doi: 10.1016/0031-9384(75)90040-2
Garrido, P., De Blas, M., Ronzoni, G., Cordero, I., Antón, M., Giné, E., et al. (2013). Differential effects of environmental enrichment and isolation housing on the hormonal and neurochemical responses to stress in the prefrontal cortex of the adult rat: relationship to working and emotional memories. J. Neural Transm. 120, 829–843. doi: 10.1007/s00702-012-0935-3
Gaulke, L. J., Horner, P. J., Fink, A. J., McNamara, C. L., and Hicks, R. R. (2005). Environmental enrichment increases progenitor cell survival in the dentate gyrus following lateral fluid percussion injury. Molec. Brain Res. 141, 138–150. doi: 10.1016/j.molbrainres.2005.08.011
Gauthier, J. M., Lin, A., Dhonnchadha, B. Á. N., Spealman, R. D., Man, H.-Y., Kantak, K. M., et al. (2017). Environmental enrichment facilitates cocaine-cue extinction, deters reacquisition of cocaine self-administration and alters AMPAR GluA1 expression and phosphorylation. Addict. Biol. 22, 152–162. doi: 10.1111/adb.12313
Gelfo, F., Bartolo, D. E., Giovine, P., Petrosini, A., and Leggio, L. M. G. (2009). Layer and regional effects of environmental enrichment on the pyramidal neuron morphology of the rat. Neurobiol. Learning Memory 91, 353–365. doi: 10.1016/j.nlm.2009.01.010
Gipson, C. D., Beckmann, J. S., El-Maraghi, S., Marusich, J. A., and Bardo, M. T. (2011). Effect of environmental enrichment on escalation of cocaine self-administration in rats. Psychopharmacology 214, 557–566. doi: 10.1007/s00213-010-2060-z
Glueck, E., Ginder, D., Hyde, J., North, K., and Grimm, J. W. (2017). Effects of dopamine D1 and D2 receptor agonists on environmental enrichment attenuated sucrose cue reactivity in rats. Psychopharmacology 234, 815–825. doi: 10.1007/s00213-016-4516-2
Gomez, A. M., Altomare, D., Sun, W.-L., Midde, N. M., Ji, H., Shtutman, M., et al. (2016). Prefrontal microRNA-221 mediates environmental enrichment-induced increase of locomotor sensitivity to nicotine. Int. J. Neuropsychopharmacol. 19, 1–12. doi: 10.1093/ijnp/pyv090
Gomez, A. M., Midde, N. M., Mactutus, C. F., Booze, R. M., and Zhu, J. (2012). Environmental enrichment alters nicotine-mediated locomotor sensitization and phosphorylation of DARPP-32 and CREB in rat prefrontal cortex. PLoS ONE 7:e44149. doi: 10.1371/journal.pone.0044149
Gomez, A. M., Sun, W. L., Midde, N. M., Harrod, S. B., and Zhu, J. (2015). Effects of environmental enrichment on ERK 1/2 phosphorylation in the rat prefrontal cortex following nicotine-induced sensitization or nicotine self-administration. Eur. J. Neurosci. 41, 109–119. doi: 10.1111/ejn.12758
Gong, X., Chen, Y., Chang, J., Huang, Y., Cai, M., Zhang, M., et al. (2018). Environmental enrichment reduces adolescent anxiety-and depression-like behaviors of rats subjected to infant nerve injury. J. Neuroinflam. 15, 1–13. doi: 10.1186/s12974-018-1301-7
González-Pardo, H., Arias, J. L., Vallejo, G., and Conejo, N. M. (2019a). Environmental enrichment effects after early stress on behavior and functional brain networks in adult rats. PLoS ONE 14:e0226377. doi: 10.1371/journal.pone.0226377
González-Pardo, H., Arias, J. L., Vallejo, G., and Conejo, N. M. (2019b). Influence of environmental enrichment on the volume of brain regions sensitive to early life stress by maternal separation in rats. Psicothema 31, 46–52. doi: 10.7334/psicothema2018.290
Görtz, N., Lewejohann, L., Tomm, M., Ambrée, O., Keyvani, K., Paulus, W., et al. (2008). Effects of environmental enrichment on exploration, anxiety, memory in female TgCRND8 Alzheimer mice. Behav. Brain Res. 191, 43–48. doi: 10.1016/j.bbr.2008.03.006
Goshen, I., Avital, A., Kreisel, T., Licht, T., Segal, M., Yirmiya, R., et al. (2009). Environmental enrichment restores memory functioning in mice with impaired IL-1 signaling via reinstatement of long-term potentiation and spine size enlargement. J. Neurosci. 29, 3395–3403. doi: 10.1523/JNEUROSCI.5352-08.2009
Green, T. A., Alibhai, I. N., Roybal, C. N., Winstanley, C. A., Theobald, D. E. H., Birnbaum, S. G., et al. (2010). Environmental enrichment produces a behavioral phenotype mediated by low cyclic adenosine monophosphate response element binding (CREB) activity in the nucleus accumbens. Biol. Psychiatry 67, 28–35. doi: 10.1016/j.biopsych.2009.06.022
Green, T. A., Gehrke, B. J., and Bardo, M. T. (2002). Environmental enrichment decreases intravenous amphetamine self-administration in rats: Dose-response functions for fixed- and progressive-ratio schedules. Psychopharmacology 162, 373–378. doi: 10.1007/s00213-002-1134-y
Grimm, J. W., Glueck, E., Ginder, D., Hyde, J., North, K., and Jiganti, K. (2018). Sucrose abstinence and environmental enrichment effects on mesocorticolimbic DARPP32 in rats. Sci. Rep. 8:13174. doi: 10.1038/s41598-018-29625-x
Grimm, J. W., Osincup, D., Wells, B., Manaois, M., Fyall, A., Buse, C., et al. (2008). Environmental enrichment attenuates cue-induced reinstatement of sucrose seeking in rats. Behav. Pharmacol. 19, 777–785. doi: 10.1097/FBP.0b013e32831c3b18
Griñán-Ferr,é, C., Izquierdo, V., Otero, E., Puigoriol-Illamola, D., Corpas, R., Sanfeliu, C., et al. (2018). Environmental enrichment improves cognitive deficits, AD hallmarks and epigenetic alterations presented in 5xFAD mouse model. Front. Cellular Neurosci. 12:224. doi: 10.3389/fncel.2018.00224
Gross, A. N., Richter, S. H., Engel, A. K. J., and Würbel, H. (2012). Cage-induced stereotypies, perseveration and the effects of environmental enrichment in laboratory mice. Behav. Brain Res. 234, 61–68. doi: 10.1016/j.bbr.2012.06.007
Gurfein, B. T., Davidenko, O., Premenko-Lanier, M., Milush, J. M., Acree, M., Dallman, M. F., et al. (2014). Environmental enrichment alters splenic immune cell composition and enhances secondary influenza vaccine responses in Mice. Molec. Med. 20, 179–190. doi: 10.2119/molmed.2013.00158
Hajheidari, S., Miladi-Gorji, H., and Bigdeli, I. (2015a). Effect of the environmental enrichment on the severity of psychological dependence and voluntary methamphetamine consumption in methamphetamine withdrawn rats. Neurosci. Lett. 584, 151–155. doi: 10.1016/j.neulet.2014.10.017
Hajheidari, S., Miladi-gorji, H., and Bigdeli, I. (2015b). Effects of environmental enrichment during induction of methamphetamine dependence on the behavioral withdrawal symptoms in rats. Neurosci. Lett. 605, 39–43. doi: 10.1016/j.neulet.2015.08.010
Hajheidari, S., Miladi-Gorji, H., and Bigdeli, I. (2017). Environmental enrichment prevents methamphetamine-induced spatial memory deficits and obsessive-compulsive behavior in rats. Iran. J. Psychiatry 12, 8–14.
Hamilton, K. R., Elliott, B. M., Berger, S. S., and Grunberg, N. E. (2014). Environmental enrichment attenuates nicotine behavioral sensitization in male and female rats. Exp. Clin. Psychopharmacol. 22, 356–363. doi: 10.1037/a0037205
Harati, H., Barbelivien, A., Herbeaux, K., Muller, M.-A., Engeln, M., Kelche, C., et al. (2013). Lifelong environmental enrichment in rats: Impact on emotional behavior, spatial memory vividness, and cholinergic neurons over the lifespan. Age 35, 1027–1043. doi: 10.1007/s11357-012-9424-8
Hartcher, K. M., Tran, K. T. N., Wilkinson, S. J., Hemsworth, P. H., Thomson, P. C., Cronin, G. M., et al. (2015). The effects of environmental enrichment and beak-trimming during the rearing period on subsequent feather damage due to feather-pecking in laying hens. Poult. Sci. 94, 852–859. doi: 10.3382/ps/pev061
Harvey-Carroll, J., Crespo-Picazo, J. L., Saubidet, M., Robinson, N. J., García-Párraga, D., March, D., et al. (2023). Brushes and shelters as low-cost environmental enrichment devices for loggerhead turtles (Caretta caretta) during rehabilitation. Chelonian Conserv. Biol. 22, 213–219. doi: 10.2744/CCB-1596.1
Heimer-McGinn, V. R., Wise, T. B., Hemmer, B. M., Dayaw, J. N., and Templer, V. L. (2020). Social housing enhances acquisition of task set independently of environmental enrichment: a longitudinal study in the Barnes maze. Learn. Behav. 48, 322–334. doi: 10.3758/s13420-020-00418-5
Hendriksen, H., Prins, J., Olivier, B., and Oosting, R. S. (2010). Environmental enrichment induces behavioral recovery and enhanced hippocampal cell proliferation in an antidepressant-resistant animal model for PTSD. PLoS ONE 5:e11943. doi: 10.1371/journal.pone.0011943
Herring, A., Ambrée, O., Tomm, M., Habermann, H., Sachser, N., Paulus, W., et al. (2009). Environmental enrichment enhances cellular plasticity in transgenic mice with Alzheimer-like pathology. Exp. Neurol. 216, 184–192. doi: 10.1016/j.expneurol.2008.11.027
Herring, A., Blome, M., Ambrée, O., Sachser, N., Paulus, W., Keyvani, K., et al. (2010). Reduction of cerebral oxidative stress following environmental enrichment in mice with alzheimer-like pathology. Brain Pathol. 20, 166–175. doi: 10.1111/j.1750-3639.2008.00257.x
Herring, A., Yasin, H., Ambrée, O., Sachser, N., Paulus, W., Keyvani, K., et al. (2008). Environmental enrichment counteracts Alzheimer's neurovascular dysfunction in TgCRND8 mice. Brain Pathol. 18, 32–39. doi: 10.1111/j.1750-3639.2007.00094.x
Hicks, R. R., Zhang, L., Atkinson, A., Stevenon, M., Veneracion, M., Seroogy, K. B., et al. (2002). Environmental enrichment attenuates cognitive deficits, but does not alter neurotrophin gene expression in the hippocampus following lateral fluid percussion brain injury. Neuroscience 112, 631–637. doi: 10.1016/S0306-4522(02)00104-5
Hoffman, A. N., Malena, R. R., Westergom, B. P., Luthra, P., Cheng, J. P., Aslam, H. A., et al. (2008). Environmental enrichment-mediated functional improvement after experimental traumatic brain injury is contingent on task-specific neurobehavioral experience. Neurosci. Lett. 431, 226–230. doi: 10.1016/j.neulet.2007.11.042
Hofford, R. S., Chow, J. J., Beckmann, J. S., and Bardo, M. T. (2017). Effects of environmental enrichment on self-administration of the short-acting opioid remifentanil in male rats. Psychopharmacology 234, 3499–3506. doi: 10.1007/s00213-017-4734-2
Hofford, R. S., Darna, M., Wilmouth, C. E., Dwoskin, L. P., and Bardo, M. T. (2014). Environmental enrichment reduces methamphetamine cue-induced reinstatement but does not alter methamphetamine reward or VMAT2 function. Behav. Brain Res. 270, 151–158. doi: 10.1016/j.bbr.2014.05.007
Hogan, L. A., Johnston, S. D., Lisle, A., Horsup, A. B., Janssen, T., Phillips, C. J., et al. (2010). Stereotypies and environmental enrichment in captive southern hairy-nosed wombats, Lasiorhinus latifrons. Appl. Animal Behav. Sci. 126, 85–95. doi: 10.1016/j.applanim.2010.05.009
Hoplight, B. J., Sherman, G. F., Hyde, L. A., and Denenberg, V. H. (2001). Effects of neocortical ectopias and environmental enrichment on Hebb-Williams maze learning in BXSB mice. Neurobiol. Lear. Memory 76, 33–45. doi: 10.1006/nlme.2000.3980
Huang, W., Queen, N. J., McMurphy, T. B., Ali, S., Wilkins, R. K., Appana, B., et al. (2020). Adipose PTEN acts as a downstream mediator of a brain-fat axis in environmental enrichment. Compr. Psychoneuroendocrinol. 4:100013. doi: 10.1016/j.cpnec.2020.100013
Huck, U. W., and Price, E. O. (1975). Differential effects of environmental enrichment on the open-field behavior of wild and domestic Norway rats. J. Comp. Physiol. Psychol. 89, 892–898. doi: 10.1037/h0077160
Hughes, R. N., and Collins, M. A. (2010). Enhanced habituation and decreased anxiety by environmental enrichment and possible attenuation of these effects by chronic α-tocopherol (vitamin E) in aging male and female rats. Pharmacol. Biochem. Behav. 94, 534–542. doi: 10.1016/j.pbb.2009.11.008
Hull, E. M., Kastaniotis, C., L'hommedieu, G., and Franz, J. (1976). Environmental enrichment and crowding: behavioral and hormonal effects. Physiol. Behav. 17, 735–741. doi: 10.1016/0031-9384(76)90032-9
Hutchinson, E. K., Avery, A. C., and VandeWoude, S. (2012a). Environmental enrichment during rearing alters corticosterone levels, thymocyte numbers, aggression in female BALB/c mice. J. Am. Assoc. Labor. Animal Sci. 51, 18–24.
Hutchinson, K. M., McLaughlin, K. J., Wright, R. L., Ortiz, J. B., Anouti, D. P., Mika, A., et al. (2012b). Environmental enrichment protects against the effects of chronic stress on cognitive and morphological measures of hippocampal integrity. Neurobiol. Learn. Mem. 97, 250–260. doi: 10.1016/j.nlm.2012.01.003
Ickes, B. R., Pham, T. M., Sanders, L. A., Albeck, D. S., Mohammed, A. H., Granholm, A. C., et al. (2000). Long-term environmental enrichment leads to regional increases in neurotrophin levels in rat brain. Exp. Neurol. 164, 45–52. doi: 10.1006/exnr.2000.7415
Imperio, C. G., McFalls, A. J., Hadad, N., Blanco-Berdugo, L., Masser, D. R., Colechio, E. M., et al. (2018). Exposure to environmental enrichment attenuates addiction-like behavior and alters molecular effects of heroin self-administration in rats. Neuropharmacology 139, 26–40. doi: 10.1016/j.neuropharm.2018.06.037
Irier, H., Street, R. C., Dave, R., Lin, L., Cai, C., Davis, T. H., et al. (2014). Environmental enrichment modulates 5-hydroxymethylcytosine dynamics in hippocampus. Genomics 104, 376–382. doi: 10.1016/j.ygeno.2014.08.019
Ismail, T. R., Yap, C. G., Naidu, R., Shri, L., and Pamidi, N. (2024). Environmental enrichment and the combined interventions of EE and metformin enhance hippocampal neuron survival and hippocampal-dependent memory in type 2 diabetic rats under stress through the BDNF-TrkB signaling pathways. Biomed. Pharmacother. 175:116729. doi: 10.1016/j.biopha.2024.116729
Iwata, E., Kikusui, T., Takeuchi, Y., and Mori, Y. (2007). Fostering and environmental enrichment ameliorate anxious behavior induced by early weaning in Balb/c mice. Physiol. Behav. 91, 318–324. doi: 10.1016/j.physbeh.2007.03.013
Jankowsky, J. L., Melnikova, T., Fadale, D. J., Xu, G. M., Slunt, H. H., Gonzales, V., et al. (2005). Environmental enrichment mitigates cognitive deficits in a mouse model of Alzheimer's disease. J. Neurosci. 25, 5217–5224. doi: 10.1523/JNEUROSCI.5080-04.2005
Jankowsky, J. L., Xu, G., Fromholt, D., Gonzales, V., and Borchelt, D. R. (2003). Environmental enrichment exacerbates amyloid plaque formation in a transgenic mouse model of Alzheimer disease. J. Neuropathol. Exp. Neurol. 62, 1220–1227. doi: 10.1093/jnen/62.12.1220
Johansson, B. B. (2004). Functional and cellular effects of environmental enrichment after experimental brain infarcts. Restor. Neurol. Neurosci. 22, 163–174.
Johansson, B. B., and Belichenko, P. V. (2002). Neuronal plasticity and dendritic spines: effect of environmental enrichment on intact and postischemic rat brain. J. Cerebral Blood Flow Metabol. 22, 89–96. doi: 10.1097/00004647-200201000-00011
Jung, C. K. E., and Herms, J. (2014). Structural dynamics of dendritic spines are influenced by an environmental enrichment: an in vivo imaging study. Cerebral Cortex 24, 377–384. doi: 10.1093/cercor/bhs317
Jurgens, H. A., and Johnson, R. W. (2012). Environmental enrichment attenuates hippocampal neuroinflammation and improves cognitive function during influenza infection. Brain, Behav. Immun. 26, 1006–1016. doi: 10.1016/j.bbi.2012.05.015
Kapgal, V., Prem, N., Hegde, P., Laxmi, T. R., and Kutty, B. M. (2016). Long term exposure to combination paradigm of environmental enrichment, physical exercise and diet reverses the spatial memory deficits and restores hippocampal neurogenesis in ventral subicular lesioned rats. Neurobiol. Learn. Mem. 130, 61–70. doi: 10.1016/j.nlm.2016.01.013
Kempermann, G., Gast, D., and Gage, F. H. (2002). Neuroplasticity in old age: Sustained fivefold induction of hippocampal neurogenesis by long-term environmental enrichment. Ann. Neurol. 52, 135–143. doi: 10.1002/ana.10262
Kerr, B., Silva, P. A., Walz, K., and Young, J. I. (2010). Unconventional transcriptional response to environmental enrichment in a mouse model of Rett syndrome. PLoS ONE 5:e11534. doi: 10.1371/journal.pone.0011534
Keyvani, K., Sachser, N., Witte, O. W., and Paulus, W. (2004). Gene expression profiling in the intact and injured brain following environmental enrichment. J. Neuropathol. Exp. Neurol. 63, 598–609. doi: 10.1093/jnen/63.6.598
Khalil, M. H. (2024). Environmental enrichment: a systematic review on the effect of a changing spatial complexity on hippocampal neurogenesis and plasticity in rodents, with considerations for translation to urban and built environments for humans. Front. Neurosci. 18:1368411. doi: 10.3389/fnins.2024.1368411
Kim, E. H., and Sufka, K. J. (2011). The effects of environmental enrichment in the chick anxiety-depression model. Behav. Brain Res. 221, 276–281. doi: 10.1016/j.bbr.2011.03.013
Kim, K., Wi, S., Seo, J. H., Pyo, S., and Cho, S. R. (2021). Reduced interaction of aggregated α-synuclein and VAMP2 by environmental enrichment alleviates hyperactivity and anxiety in a model of Parkinson's disease. Genes 12:392. doi: 10.3390/genes12030392
Kim, M.-.S., Yu, J. H., Kim, C. H., Choi, J. Y., Seo, J. H., Lee, M.-Y., et al. (2016). Environmental enrichment enhances synaptic plasticity by internalization of striatal dopamine transporters. J. Cerebr. Blood Flow Metabol. 36, 2122–2133. doi: 10.1177/0271678X15613525
Kimura, L. F., Novaes, L. S., Picolo, G., Munhoz, C. D., Cheung, C. W., Camarini, R., et al. (2022). How environmental enrichment balances out neuroinflammation in chronic pain and comorbid depression and anxiety disorders. Br. J. Pharmacol. 179, 1640–1660. doi: 10.1111/bph.15584
Kiyono, S., Seo, M., and Shibagaki, M. (1981). Effects of environmental enrichment upon maze performance in rats with microcephaly induced by prenatal x-irradiation. Jpn. J. Physiol. 31, 769–773. doi: 10.2170/jjphysiol.31.769
Kiyono, S., Seo, M. L., Shibagaki, M., and Inouye, M. (1985). Facilitative effects of maternal environmental enrichment on maze learning in rat offspring. Physiol. Behav. 34, 431–435. doi: 10.1016/0031-9384(85)90207-0
Klaissle, P., Lesemann, A., Huehnchen, P., Hermann, A., Storch, A., Steiner, B., et al. (2012). Physical activity and environmental enrichment regulate the generation of neural precursors in the adult mouse substantia nigra in a dopamine-dependent manner. BMC Neurosci. 13, 1–15. doi: 10.1186/1471-2202-13-132
Klein, S. L., Lambert, K. G., Durr, D., Schaefer, T., and Waring, R. E. (1994). Influence of environmental enrichment and sex on predator stress response in rats. Physiol. Behav. 56, 291–297. doi: 10.1016/0031-9384(94)90197-X
Kline, A. E., Olsen, A. S., Sozda, C. N., Hoffman, A. N., and Cheng, J. P. (2012). Evaluation of a combined treatment paradigm consisting of environmental enrichment and the 5-HT1A receptor agonist buspirone after experimental traumatic brain injury. J. Neurotrauma 29, 1960–1969. doi: 10.1089/neu.2012.2385
Kline, A. E., Wagner, A. K., Westergom, B. P., Malena, R. R., Zafonte, R. D., Olsen, A. S., et al. (2007). Acute treatment with the 5-HT1A receptor agonist 8-OH-DPAT and chronic environmental enrichment confer neurobehavioral benefit after experimental brain trauma. Behav. Brain Res. 177, 186–194. doi: 10.1016/j.bbr.2006.11.036
Koh, S., Magid, R., Chung, H., Stine, C. D., and Wilson, D. N. (2007). Depressive behavior and selective downregulation of serotonin receptor expression after early-life seizures: reversal by environmental enrichment. Epilepsy Behav. 10, 26–31. doi: 10.1016/j.yebeh.2006.11.008
Komitova, M., Perfilieva, E., Mattsson, B., Eriksson, P. S., and Johansson, B. B. (2002). Effects of cortical ischemia and postischemic environmental enrichment on hippocampal cell genesis and differentiation in the adult rat. J. Cerebr. Blood Flow Metabol. 22, 852–860. doi: 10.1097/00004647-200207000-00010
Kondo, M., Gray, L. J., Pelka, G. J., Christodoulou, J., Tam, P. P. L., Hannan, A. J., et al. (2008). Environmental enrichment ameliorates a motor coordination deficit in a mouse model of Rett syndrome - Mecp2 gene dosage effects and BDNF expression. Eur. J. Neurosci. 27, 3342–3350. doi: 10.1111/j.1460-9568.2008.06305.x
Kreilaus, F., Spiro, A. S., Hannan, A. J., Garner, B., and Jenner, A. M. (2016). Therapeutic effects of anthocyanins and environmental enrichment in R6/1 huntington's disease mice. J. Huntington's Dis. 5, 285–296. doi: 10.3233/JHD-160204
Kumar, A., Rani, A., Tchigranova, O., Lee, W. H., and Foster, T. C. (2012). Influence of late-life exposure to environmental enrichment or exercise on hippocampal function and CA1 senescent physiology. Neurobiol. Aging 33, 828–e1. doi: 10.1016/j.neurobiolaging.2011.06.023
Lahiani-Cohen, I., Lourbopoulos, A., Haber, E., Rozenstein-Tsalkovich, L., Abramsky, O., Grigoriadis, N., et al. (2011). Moderate environmental enrichment mitigates tauopathy in a neurofibrillary tangle mouse model. J. Neuropathol. Exp. Neurol. 70, 610–621. doi: 10.1097/NEN.0b013e318221bfab
Lajud, N., Díaz-Chávez, A., Radabaugh, H. L., Cheng, J. P., Rojo-Soto, G., Valdéz-Alarcón, J. J., et al. (2019). Delayed and abbreviated environmental enrichment after brain trauma promotes motor and cognitive recovery that is not contingent on increased neurogenesis. J. Neurotrauma 36, 756–767. doi: 10.1089/neu.2018.5866
Lambert, T. J., Fernandez, S. M., and Frick, K. M. (2005). Different types of environmental enrichment have discrepant effects on spatial memory and synaptophysin levels in female mice. Neurobiol. Learn. Mem. 83, 206–216. doi: 10.1016/j.nlm.2004.12.001
Lanosa, X. A., Santacroce, I., and Colombo, J. A. (2011). Exposure to environmental enrichment prior to a cerebral cortex stab wound attenuates the postlesional astroglia response in rats. Neuron. Glia Biol. 7, 163–175. doi: 10.1017/S1740925X12000099
Lauderdale, L. K., and Miller, L. J. (2020). Efficacy of an interactive apparatus as environmental enrichment for common bottlenose dolphins (Tursiops truncatus). Animal Welfare 29, 379–386. doi: 10.7120/09627286.29.4.379
Leal-Galicia, P., Saldívar-González, A., Morimoto, S., and Arias, C. (2007). Exposure to environmental enrichment elicits differential hippocampal cell proliferation: role of individual responsiveness to anxiety. Dev. Neurobiol. 67, 395–405. doi: 10.1002/dneu.20322
Lee, H. Y., Song, S. Y., Hwang, J., Baek, A., Baek, D., Kim, S. H., et al. (2023). Very early environmental enrichment protects against apoptosis and improves functional recovery from hypoxic–ischemic brain injury. Front. Molec. Neurosci. 15:1019173. doi: 10.3389/fnmol.2022.1019173
Lee, S., Doulames, V., Donnelly, M., Levasseaur, J., and Shea, T. B. (2012). Environmental enrichment can prevent cognitive decline induced by dietary oxidative challenge. J. Alzheimer's Dis. 28, 497–501. doi: 10.3233/JAD-2011-111562
Leggio, M. G., Mandolesi, L., Federico, F., Spirito, F., Ricci, B., Gelfo, F., et al. (2005). Environmental enrichment promotes improved spatial abilities and enhanced dendritic growth in the rat. Behav. Brain Res. 163, 78–90. doi: 10.1016/j.bbr.2005.04.009
Lehmann, M. L., and Herkenham, M. (2011). Environmental enrichment confers stress resiliency to social defeat through an infralimbic cortex-dependent neuroanatomical pathway. J. Neurosci. 31, 6159–6173. doi: 10.1523/JNEUROSCI.0577-11.2011
Li, K. A., Lund, E. T., and Voigt, J. P. W. (2016). The impact of early postnatal environmental enrichment on maternal care and offspring behaviour following weaning. Behav. Proc. 122, 51–58. doi: 10.1016/j.beproc.2015.11.008
Lichti, C. F., Fan, X., English, R. D., Zhang, Y., Li, D., Kong, F., et al. (2014). Environmental enrichment alters protein expression as well as the proteomic response to cocaine in rat nucleus accumbens. Front. Behav. Neurosci. 8:246. doi: 10.3389/fnbeh.2014.00246
Lidfors, L. (1997). Behavioural effects of environmental enrichment for individually caged rabbits. Appl. Anim. Behav. Sci. 52, 157–169. doi: 10.1016/S0168-1591(96)01141-0
Lima, A. P. A. S., Silva, K., Padovan, C. M., Almeida, S. S., and Fukuda, M. T. H. (2014). Memory, learning, participation of the cholinergic system in young rats exposed to environmental enrichment. Behav. Brain Res. 259, 247–252. doi: 10.1016/j.bbr.2013.10.046
Lin, Y. H., Yao, M. C., Wu, H. Y., Dong, J., Ni, H. Y., Kou, X. L., et al. (2020). HDAC2 (Histone deacetylase 2): a critical factor in environmental enrichment-mediated stroke recovery. J. Neurochem. 155, 679–696. doi: 10.1111/jnc.15043
Line, S. W., Clarke, A. S., Markowitz, H., and Ellman, G. (1990). Responses of female rhesus macaques to an environmental enrichment apparatus. Lab. Anim. 24, 213–220. doi: 10.1258/002367790780866245
Liu, X., Qiu, J., Alcon, S., Hashim, J., Meehan III, W. P., and Mannix, R. (2017). Environmental enrichment mitigates deficits after repetitive mild traumatic brain injury. J. Neurotr. 34, 2445–2455. doi: 10.1089/neu.2016.4823
Lonetti, G., Angelucci, A., Morando, L., Boggio, E. M., Giustetto, M., Pizzorusso, T., et al. (2010). Early environmental enrichment moderates the behavioral and synaptic phenotype of MeCP2 null mice. Biol. Psychiatry 67, 657–665. doi: 10.1016/j.biopsych.2009.12.022
Lopes, D. A., Souza, T. M. O., de Andrade, J. S., Silva, M. F. S., Antunes, H. K. M., Maluf, L. L. S., et al. (2019). Anxiolytic and panicolytic-like effects of environmental enrichment seem to be modulated by serotonin neurons located in the dorsal subnucleus of the dorsal raphe. Brain Res. Bull. 150, 272–280. doi: 10.1016/j.brainresbull.2019.06.012
Lopez-Atalaya, J. P., Ciccarelli, A., Viosca, J., Valor, L. M., Jimenez-Minchan, M., Canals, S., et al. (2011). CBP is required for environmental enrichment-induced neurogenesis and cognitive enhancement. EMBO J. 30, 4287–4298. doi: 10.1038/emboj.2011.299
Lutz, C. K., and Novak, M. A. (2005). Environmental enrichment for nonhuman primates: theory and application. ILAR J. 46, 178–191. doi: 10.1093/ilar.46.2.178
Maesako, M., Uemura, K., Kubota, M., Kuzuya, A., Sasaki, K., Asada, M., et al. (2012). Environmental enrichment ameliorated high-fat diet-induced Aβ deposition and memory deficit in APP transgenic mice. Neurobiol. Aging 33, 1011.e11–1011.e23. doi: 10.1016/j.neurobiolaging.2011.10.028
Mainardi, M., Landi, S., Gianfranceschi, L., Baldini, S., De Pasquale, R., Berardi, N., et al. (2010). Environmental enrichment potentiates thalamocortical transmission and plasticity in the adult rat visual cortex. J. Neurosci. Res. 88, 3048–3059. doi: 10.1002/jnr.22461
Manosevitz, M., and Joel, U. (1973). Behavioral effects of environmental enrichment in randomly bred mice. J. Comp. Physiol. Psychol. 85, 373–382. doi: 10.1037/h0035041
Manosevitz, M., and Montemayor, R. J. (1972). Interaction of environmental enrichment and genotype. J. Comp. Physiol. Psychol. 79, 67–76. doi: 10.1037/h0032547
Manosevitz, M., and Pryor, J. B. (1975). Cage size as a factor in environmental enrichment. J. Comp. Physiol. Psychol. 89, 648–654. doi: 10.1037/h0077426
Marashi, V., Barnekow, A., Ossendorf, E., and Sachser, N. (2003). Effects of different forms of environmental enrichment on behavioral, endocrinological, and immunological parameters in male mice. Hormones Behav. 43, 281–292. doi: 10.1016/S0018-506X(03)00002-3
Marion, M., Hamilton, J., Richardson, B., Roeder, N., Figueiredo, A., Nubelo, A. P. K., et al. (2022). Environmental enrichment sex-dependently rescues memory impairment in FABP5 KO mice not mediated by brain-derived neurotrophic factor. Behav. Brain Res. 425:113814. doi: 10.1016/j.bbr.2022.113814
Martínez-Cué, C., Baamonde, C., Lumbreras, M., Paz, J., Davisson, M. T., Schmidt, C., et al. (2002). Differential effects of environmental enrichment on behavior and learning of male and female Ts65Dn mice, a model for Down syndrome. Behav. Brain Res. 134, 185–200. doi: 10.1016/S0166-4328(02)00026-8
Matteucci, A., Ceci, C., Mallozzi, C., Macrì, S., Malchiodi-Albedi, F., Laviola, G., et al. (2015). Effects of neonatal corticosterone and environmental enrichment on retinal ERK1/2 and CREB phosphorylation in adult mice. Exp. Eye Res. 128, 109–113. doi: 10.1016/j.exer.2014.08.007
Mattson, M. P., Duan, W., Lee, J., and Guo, Z. (2001). Suppression of brain aging and neurodegenerative disorders by dietary restriction and environmental enrichment: Molecular mechanisms. Mech. Ageing Dev. 122, 757–778. doi: 10.1016/S0047-6374(01)00226-3
McAllister, J. T., Lennertz, L., and Atencio Mojica, Z. (2022). Mapping a discipline: a guide to using VOSviewer for bibliometric and visual analysis. Sci. Technol. Libr. 41, 319–348. doi: 10.1080/0194262X.2021.1991547
McKibben, L. A., and Dwivedi, Y. (2021). Early life and adult stress promote sex dependent changes in hypothalamic miRNAs and environmental enrichment prevents stress-induced miRNA and gene expression changes in rats. BMC Genom. 22, 1–24. doi: 10.1186/s12864-021-08003-4
McOmish, C. E., Burrows, E., Howard, M., Scarr, E., Kim, D., Shin, H.-S., et al. (2008). Phospholipase C-β1 knockout mice exhibit endophenotypes modeling schizophrenia which are rescued by environmental enrichment and clozapine administration. Mol. Psychiatry 13, 661–672. doi: 10.1038/sj.mp.4002046
Meagher, R. K., and Mason, G. J. (2012). Environmental enrichment reduces signs of boredom in caged mink. PLoS ONE 7:e49180. doi: 10.1371/journal.pone.0049180
Melotti, L., Oostindjer, M., Bolhuis, J. E., Held, S., and Mendl, M. (2011). Coping personality type and environmental enrichment affect aggression at weaning in pigs. Appl. Animal Behav. Sci. 133, 144–153. doi: 10.1016/j.applanim.2011.05.018
Meyer, M. M., Johnson, A. K., and Bobeck, E. A. (2019). A novel environmental enrichment device improved broiler performance without sacrificing bird physiological or environmental quality measures. Poult. Sci. 98, 5247–5256. doi: 10.3382/ps/pez417
Mirmiran, M., and Uylings, H. B. M. (1983). The environmental enrichment effect upon cortical growth is neutralized by concomitant pharmacological suppression of active sleep in female rats. Brain Res. 261, 331–334. doi: 10.1016/0006-8993(83)90639-X
Mirmiran, M., Uylings, H. B. M., and Corner, M. A. (1983). Pharmacological suppression of REM sleep prior to weaning counteracts the effectiveness of subsequent environmental enrichment on cortical growth in rats. Dev. Brain Res. 7, 102–105. doi: 10.1016/0165-3806(83)90086-X
Mirochnic, S., Wolf, S., Staufenbiel, M., and Kempermann, G. (2009). Age effects on the regulation of adult hippocampal neurogenesis by physical activity and environmental enrichment in the APP23 mouse model of Alzheimer disease. Hippocampus 19, 1008–1018. doi: 10.1002/hipo.20560
Monje-Reyna, D., Manzo Denes, J., and Santamaria, F. (2022). Effects of environmental enrichment and sexual dimorphism on the expression of cerebellar receptors in C57BL/6 and BTBR+ Itpr3tf/J mice. BMC Res. Notes, 15:175. doi: 10.1186/s13104-022-06062-8
Monosevitz, M. (1970). Early environmental enrichment and mouse behavior. J. Comp. Physiol. Psychol. 71, 459–466. doi: 10.1037/h0029141
Mora, F., Segovia, G., and del Arco, A. (2007). Aging, plasticity and environmental enrichment: Structural changes and neurotransmitter dynamics in several areas of the brain. Brain Res. Rev. 55, 78–88. doi: 10.1016/j.brainresrev.2007.03.011
Moradi-Kor, N., Ghanbari, A., Rashidipour, H., Yousefi, B., Bandegi, A. R., Rashidy-Pour, A., et al. (2019). Beneficial effects of Spirulina platensis, voluntary exercise and environmental enrichment against adolescent stress induced deficits in cognitive functions, hippocampal BDNF and morphological remolding in adult female rats. Horm. Behav. 112, 20–31. doi: 10.1016/j.yhbeh.2019.03.004
Morelli, E., Ghiglieri, V., Pendolino, V., Bagetta, V., Pignataro, A., Fejtova, A., et al. (2014). Environmental enrichment restores CA1 hippocampal LTP and reduces severity of seizures in epileptic mice. Exp. Neurol. 261, 320–327. doi: 10.1016/j.expneurol.2014.05.010
Morley-Fletcher, S., Rea, M., Maccari, S., and Laviola, G. (2003). Environmental enrichment during adolescence reverses the effects of prenatal stress on play behaviour and HPA axis reactivity in rats. Eur. J. Neurosci. 18, 3367–3374. doi: 10.1111/j.1460-9568.2003.03070.x
Morse, S. J., Butler, A. A., Davis, R. L., Soller, I. J., and Lubin, F. D. (2015). Environmental enrichment reverses histone methylation changes in the aged hippocampus and restores age-related memory deficits. Biology 4, 298–313. doi: 10.3390/biology4020298
Mosaferi, B., Babri, S., Mohaddes, G., Khamnei, S., and Mesgari, M. (2015). Post-weaning environmental enrichment improves BDNF response of adult male rats. Int. J. Dev. Neurosci. 46, 108–114. doi: 10.1016/j.ijdevneu.2015.07.008
Munetsuna, E., Hattori, M., Sakimoto, Y., Ishida, A., Sakata, S., Hojo, Y., et al. (2011). Environmental enrichment alters gene expression of steroidogenic enzymes in the rat hippocampus. Gen. Comp. Endocrinol. 171, 28–32. doi: 10.1016/j.ygcen.2010.12.007
Munsterhjelm, C., Valros, A., Heinonen, M., Hälli, O., Siljander-Rasi, H., Peltoniemi, O. A. T., et al. (2010). Environmental enrichment in early life affects cortisol patterns in growing pigs. Animal 4, 242–249. doi: 10.1017/S1751731109990814
Mustroph, M. L., Pinardo, H., Merritt, J. R., and Rhodes, J. S. (2016). Parameters for abolishing conditioned place preference for cocaine from running and environmental enrichment in male C57BL/6J mice. Behav. Brain Res. 312, 366–373. doi: 10.1016/j.bbr.2016.06.049
Muthmainah, M., Sari, W. A., Wiyono, N., Ghazali, D. A., Yudhani, R. D., Wasita, B., et al. (2021). Environmental enrichment ameliorates anxiety-like behavior in rats without altering plasma corticosterone level. Open Access Maced. J. Med. Sci. 9, 1074–1080. doi: 10.3889/oamjms.2021.6396
Mychasiuk, R., Muhammad, A., and Kolb, B. (2014). Environmental enrichment alters structural plasticity of the adolescent brain but does not remediate the effects of prenatal nicotine exposure. Synapse 68, 293–305. doi: 10.1002/syn.21737
Nader, J., Claudia, C., Rawas, R. E., Favot, L., Jaber, M., Thiriet, N., et al. (2012). Loss of environmental enrichment increases vulnerability to cocaine addiction. Neuropsychopharmacology 37, 1579–1587. doi: 10.1038/npp.2012.2
Nag, N., Moriuchi, J. M., Peitzman, C. G. K., Ward, B. C., Kolodny, N. H., Berger-Sweeney, J. E., et al. (2009). Environmental enrichment alters locomotor behaviour and ventricular volume in Mecp21lox mice. Behav. Brain Res. 196, 44–48. doi: 10.1016/j.bbr.2008.07.008
Nam, Y., Kim, S., Park, Y. H., Kim, B.-H., Shin, S. J., Leem, S. H., et al. (2024). Investigating the impact of environmental enrichment on proteome and neurotransmitter-related profiles in an animal model of Alzheimer's disease. Aging Cell 2024:e14231. doi: 10.1111/acel.14231
Nawaz, A., Batool, Z., Ahmed, S., Khaliq, S., Sajid, I., Anis, L., et al. (2017). Attenuation of restraint stress-induced behavioral deficits by environmental enrichment in male rats. Pakistan Veter. J. 37, 435–439.
Nicolas, C., Hofford, R. S., Dugast, E., Lardeux, V., Belujon, P., Solinas, M., et al. (2022). Prevention of relapse to methamphetamine self-administration by environmental enrichment: involvement of glucocorticoid receptors. Psychopharmacology 239, 1009–1018. doi: 10.1007/s00213-021-05770-6
Nithianantharajah, J., Levis, H. J., and Murphy, M. (2004). Environmental enrichment results in cortical and subcortical changes in levels of synaptophysin and PSD-95 proteins. Neurobiol. Learn. Mem. 81, 200–210. doi: 10.1016/j.nlm.2004.02.002
Niu, X., Tahera, Y., and Canlon, B. (2007). Environmental enrichment to sound activates dopaminergic pathways in the auditory system. Physiol. Behav. 92, 34–39. doi: 10.1016/j.physbeh.2007.05.020
Novaes, L. S., Dos Santos, N. B., Batalhote, R. F. P., Malta, M. B., Camarini, R., Scavone, C., et al. (2017). Environmental enrichment protects against stress-induced anxiety: Role of glucocorticoid receptor, ERK, CREB signaling in the basolateral amygdala. Neuropharmacology 113, 457–466. doi: 10.1016/j.neuropharm.2016.10.026
Novaes, L. S., Dos Santos, N. B., Perfetto, J. G., Goosens, K. A., and Munhoz, C. D. (2018). Environmental enrichment prevents acute restraint stress-induced anxiety-related behavior but not changes in basolateral amygdala spine density. Psychoneuroendocrinology 98, 6–10. doi: 10.1016/j.psyneuen.2018.07.031
Novkovic, T., Mittmann, T., and Manahan-Vaughan, D. (2015). BDNF contributes to the facilitation of hippocampal synaptic plasticity and learning enabled by environmental enrichment. Hippocampus 25, 1–15. doi: 10.1002/hipo.22342
Nowakowska, E., Czubak, A., Kus, K., Metelska, J., Burda, K., Nowakowska, A., et al. (2010). Effect of lamotrigine and environmental enrichment on spatial memory and other behavioral functions in rats. Arzneimittel-Forschung/Drug Res. 60, 307–314. doi: 10.1055/s-0031-1296292
Oliveira, A. P. G., Costa, W. M., da Costa, W. M., Nunes, R. D. A., Dias, N. C. D. S., and Madella-Oliveira, A. D. F. (2015). Influence of environmental enrichment in patterns of social behaviour and abnormal of goats in containment. Arch. Veter. Sci. 20, 1–6.
Oostindjer, M., Bolhuis, J. E., Mendl, M., Held, S., Gerrits, W., van den Brand, H., et al. (2010). Effects of environmental enrichment and loose housing of lactating sows on piglet performance before and after weaning. J. Anim. Sci. 88, 3554–3562. doi: 10.2527/jas.2010-2940
Orock, A., Louwies, T., Yuan, T., and Greenwood-Van Meerveld, B. (2020). Environmental enrichment prevents chronic stress-induced brain-gut axis dysfunction through a GR-mediated mechanism in the central nucleus of the amygdala. Neurogastroenterol. Motil. 32:e13826. doi: 10.1111/nmo.13826
Pang, T. Y. C., and Hannan, A. J. (2013). Enhancement of cognitive function in models of brain disease through environmental enrichment and physical activity. Neuropharmacology 64, 515–528. doi: 10.1016/j.neuropharm.2012.06.029
Parks, E. A., McMechan, A. P., Hannigan, J. H., and Berman, R. F. (2008). Environmental enrichment alters neurotrophin levels after fetal alcohol exposure in rats. Alcoholism 32, 1741–1751. doi: 10.1111/j.1530-0277.2008.00759.x
Pascual, R., Valencia, M., and Bustamante, C. (2015). Purkinje cell dendritic atrophy induced by prenatal stress is mitigated by early environmental enrichment. Neuropediatrics 46, 37–43. doi: 10.1055/s-0034-1395344
Passineau, M. J., Green, E. J., and Dietrich, W. D. (2001). Therapeutic effects of environmental enrichment on cognitive function and tissue integrity following severe traumatic brain injury in rats. Exp. Neurol. 168, 373–384. doi: 10.1006/exnr.2000.7623
Peck, J. A., Galaj, E., Eshak, S., Newman, K. L., and Ranaldi, R. (2015). Environmental enrichment induces early heroin abstinence in an animal conflict model. Pharmacol. Biochem. Behav. 138, 20–25. doi: 10.1016/j.pbb.2015.09.009
Peng, Y., Jian, X., Liu, L., Tong, J., and Lei, D. (2011). Influence of environmental enrichment on hippocampal synapses in adolescent offspring of mothers exposed to prenatal stress. Neural Regener. Res. 6, 378–382.
Percaccio, C. R., Engineer, N. D., Pruette, A. L., Pandya, P. K., Moucha, R., Rathbun, D. L., et al. (2005). Environmental enrichment increases paired-pulse depression in rat auditory cortex. J. Neurophysiol. 94, 3590–3600. doi: 10.1152/jn.00433.2005
Pereira, L. O., Arteni, N. S., Petersen, R. C., Rocha, d. a., Achaval, A. P., Netto, M. C. A., et al. (2007). Effects of daily environmental enrichment on memory deficits and brain injury following neonatal hypoxia-ischemia in the rat. Neurobiol. Lear. Memory 87, 101–108. doi: 10.1016/j.nlm.2006.07.003
Petrosini, L., De Bartolo, P., Foti, F., Gelfo, F., Cutuli, D., Leggio, M. G., et al. (2009). On whether the environmental enrichment may provide cognitive and brain reserves. Brain Res. Rev. 61, 221–239. doi: 10.1016/j.brainresrev.2009.07.002
Pham, T. M., Ickes, B., Albeck, D., Söderström, S., Granholm, A. C., Mohammed, A. H., et al. (1999). Changes in brain nerve growth factor levels and nerve growth factor receptors in rats exposed to environmental enrichment for one year. Neuroscience 94, 279–286. doi: 10.1016/S0306-4522(99)00316-4
Pintori, N., Piva, A., Guardiani, V., Decimo, I., and Chiamulera, C. (2022). Brief environmental enrichment exposure enhances contextual-induced sucrose-seeking with and without memory reactivation in rats. Behav. Brain Res. 416:113556. doi: 10.1016/j.bbr.2021.113556
Plane, J. M., Whitney, J. T., Schallert, T., and Parent, J. M. (2008). Retinoic acid and environmental enrichment alter subventricular zone and striatal neurogenesis after stroke. Exp. Neurol. 214, 125–134. doi: 10.1016/j.expneurol.2008.08.006
Polito, L., Chierchia, A., Tunesi, M., Bouybayoune, I., Kehoe, P. G., Albani, D., et al. (2014). Environmental enrichment lessens cognitive decline in APP23 mice without affecting brain sirtuin expression. J. Alzheimer's Dis. 42, 851–864. doi: 10.3233/JAD-131430
Pons-Espinal, M., de Lagran, M. M., and Dierssen, M. (2013). Environmental enrichment rescues DYRK1A activity and hippocampal adult neurogenesis in TgDyrk1A. Neurobiol. Dis. 60, 18–31. doi: 10.1016/j.nbd.2013.08.008
Prathipa, A., Gomathinayagam, S., Thangavelu, A., Thirumurugan, R., Sarupriya, M. S., Jayatahnagaraj, M. G., et al. (2017). Environmental enrichment of captive psittacine birds enclosure at Arignar Anna Zoological Park, Vandalur. Indian Veter. J. 94, 20–23.
Prusky, G. T., Reidel, C., and Douglas, R. M. (2000). Environmental enrichment from birth enhances visual acuity but not place learning in mice. Behav. Brain Res. 114, 11–15. doi: 10.1016/S0166-4328(00)00186-8
Pusic, A. D., and Kraig, R. P. (2014). Youth and environmental enrichment generate serum exosomes containing miR-219 that promote CNS myelination. Glia 62, 284–299. doi: 10.1002/glia.22606
Pusic, K. M., Pusic, A. D., Kemme, J., and Kraig, R. P. (2014). Spreading depression requires microglia and is decreased by their M2a polarization from environmental enrichment. Glia 62, 1176–1194. doi: 10.1002/glia.22672
Qian, J., Zhou, D., Pan, F., Liu, C.- X., and Wang, Y.-W. (2008). Effect of environmental enrichment on fearful behavior and gastrin-releasing peptide receptor expression in the amygdala of prenatal stressed rats. J. Neurosci. Res. 86, 3011–3017. doi: 10.1002/jnr.21736
Queen, N. J., Boardman, A. A., Patel, R. S., Siu, J. J., Mo, X., Cao, L., et al. (2020). Environmental enrichment improves metabolic and behavioral health in the BTBR mouse model of autism. Psychoneuroendocrinology 111:104476. doi: 10.1016/j.psyneuen.2019.104476
Ragu Varman, D., Marimuthu, G., and Emmanuvel Rajan, K. (2013). Environmental enrichment upregulates micro-RNA-183 and alters acetylcholinesterase splice variants to reduce anxiety-like behavior in the little Indian field mouse (Mus booduga). J. Neurosci. Res. 91, 426–435. doi: 10.1002/jnr.23165
Rahman, S., and Bardo, M. T. (2008). Environmental enrichment increases amphetamine-induced glutamate neurotransmission in the nucleus accumbens: a neurochemical study. Brain Res. 1197, 40–46. doi: 10.1016/j.brainres.2007.12.052
Rajab, E., Al-Kafaji, G., Al Enazi, H., Al Qassab, N., Sakusic, A., Kamal, A., et al. (2017). Additive benefits of environmental enrichment and voluntary exercise on cognition and motor coordination in diabetic mice. Bahrain Med. Bull. 39, 11–16. doi: 10.12816/0047434
Rajesh, T., Tantradi, R. R., Rai, K. S., Gopalakrishnan, S., Babu, M. R., Adiga, M., et al. (2016). Prenatal inflammation induced alterations in spatial learning and memory abilities in adult offspring: mitigated by physical exercise and environmental enrichment. Res. J. Pharm. 7, 1681–1688.
Ravenelle, R., Byrnes, E. M., Byrnes, J. J., McInnis, C., Park, J. H., Donaldson, S. T., et al. (2013). Environmental enrichment effects on the neurobehavioral profile of selective outbred trait anxiety rats. Behav. Brain Res. 252, 49–57. doi: 10.1016/j.bbr.2013.05.041
Rawas, R. E., Thiriet, N., Nader, J., Lardeux, V., Jaber, M., Solinas, M., et al. (2011). Early exposure to environmental enrichment alters the expression of genes of the endocannabinoid system. Brain Res. 1390, 80–89. doi: 10.1016/j.brainres.2011.03.025
Razavinasab, M., Parsania, S., Nikootalab, M., Khaleghi, M., Saleki, K., Banazadeh, M., et al. (2022). Early environmental enrichment prevents cognitive impairments and developing addictive behaviours in a mouse model of prenatal psychological and physical stress. Int. J. Dev. Neurosci. 82, 72–84. doi: 10.1002/jdn.10161
Regaiolli, B., Rizzo, A., Ottolini, G., Petrazzini, M. E. M., Spiezio, C., Agrillo, C., et al. (2019). Motion illusions as environmental enrichment for zoo animals: a preliminary investigation on lions (Panthera leo). Front. Psychol. 10:2220. doi: 10.3389/fpsyg.2019.02220
Rehnberg, L. K., Robert, K. A., Watson, S. J., and Peters, R. A. (2015). The effects of social interaction and environmental enrichment on the space use, behaviour and stress of owned housecats facing a novel environment. Appl. Animal Behav. Sci. 169, 51–61. doi: 10.1016/j.applanim.2015.06.002
Reinhardt, V., Houser, D., Eisele, S., Cowley, D., and Vertein, R. (1988). Behavioral responses of unrelated rhesus monkey females paired for the purpose of environmental enrichment. Am. J. Primatol. 14, 135–140. doi: 10.1002/ajp.1350140204
Reiser, S., Pohlmann, D. M., Blancke, T., Koops, U., and Trautner, J. (2021). Environmental enrichment during early rearing provokes epigenetic changes in the brain of a salmonid fish. Compar. Biochem. Physiol. Part D 39:100838. doi: 10.1016/j.cbd.2021.100838
Renoir, T., Pang, T. Y. C., Mo, C., Chan, G., Chevarin, C., Lanfumey, L., et al. (2013). Differential effects of early environmental enrichment on emotionality related behaviours in Huntington's disease transgenic mice. J. Physiol. 591, 41–55. doi: 10.1113/jphysiol.2012.239798
Réus, G. Z., Abitante, M. S., Manosso, L. M., de Moura, A. B., Borba, L. A., Botelho, M. E. M., et al. (2023). Environmental enrichment rescues oxidative stress and behavioral impairments induced by maternal care deprivation: sex- and developmental-dependent differences. Mol. Neurobiol. 60, 6757–6773. doi: 10.1007/s12035-021-02588-3
Reynolds, S., Urruela, M., and Devine, D. P. (2013). Effects of environmental enrichment on repetitive behaviors in the btbr t+tf/j mouse model of autism. Autism Research 6, 337–343. doi: 10.1002/aur.1298
Riaz, W., Khan, Z. Y., Jawaid, A., and Shahid, S. (2021). Virtual reality (VR)-based environmental enrichment in older adults with mild cognitive impairment (MCI) and mild dementia. Brain Sci. 11:1103. doi: 10.3390/brainsci11081103
Rizzi, S., Bianchi, P., Guidi, S., Ciani, E., and Bartesaghi, R. (2011). Impact of environmental enrichment on neurogenesis in the dentate gyrus during the early postnatal period. Brain Res. 1415, 23–33. doi: 10.1016/j.brainres.2011.08.007
Rockman, G. E., Gibson, J. E. M., and Benarroch, A. (1989). Effects of environmental enrichment on voluntary ethanol intake in rats. Pharmacol. Biochem. Behav. 34, 487–490. doi: 10.1016/0091-3057(89)90545-5
Rodríguez, J. J., Noristani, H. N., Olabarria, M., Fletcher, J., Somerville, T. D. D., Yeh, C. Y., et al. (2011). Voluntary running and environmental enrichment restores impaired hippocampal neurogenesis in a triple transgenic mouse model of Alzheimer's disease. Curr. Alzheimer Res. 8, 707–717. doi: 10.2174/156720511797633214
Rogers, J., Li, S., Lanfumey, L., Hannan, A. J., and Renoir, T. (2017). Environmental enrichment reduces innate anxiety with no effect on depression-like behaviour in mice lacking the serotonin transporter. Behav. Brain Res. 332, 355–361. doi: 10.1016/j.bbr.2017.06.009
Rojas, J. J., Deniz, B. F., Miguel, P. M., and Diaz, R. (2013). Hermel EdE-S, Achaval M, et al. Effects of daily environmental enrichment on behavior and dendritic spine density in hippocampus following neonatal hypoxia-ischemia in the rat. Exp. Neurol. 241, 25–33. doi: 10.1016/j.expneurol.2012.11.026
Ronzoni, G., Antón, M., Mora, F., Segovia, G., and Arco, A. D. (2016). Infralimbic cortex controls the activity of the hypothalamus-pituitary-adrenal axis and the formation of aversive memory: effects of environmental enrichment. Behav. Brain Res. 297, 338–344. doi: 10.1016/j.bbr.2015.10.037
Rose, F. D. (1988). Environmental enrichment and recovery of function following brain damage in the rat. Med. Sci. Res. 16, 257–263.
Rose, F. D., Davey, M. J., Love, S., and Dell, P. A. (1987). Environmental enrichment and recovery from contralateral sensory neglect in rats with large unilateral neocortical lesions. Behav. Brain Res. 24, 195–202. doi: 10.1016/0166-4328(87)90057-X
Rose, F. D., Dell, P. A., Love, S., and Davey, M. J. (1988). Environmental enrichment and recovery from a complex go/no-go reversal deficit in rats following large unilateral neocortical lesions. Behav. Brain Res. 31, 37–45. doi: 10.1016/0166-4328(88)90156-8
Ross, P. W., and Everitt, J. I. (1988). A nylon ball device for primate environmental enrichment. Lab. Anim. Sci. 38, 481–483.
Rossetti, M. F., Varayoud, J., Moreno-Piovano, G. S., Luque, E. H., and Ramos, J. G. (2015). Environmental enrichment attenuates the age-related decline in the mRNA expression of steroidogenic enzymes and reduces the methylation state of the steroid 5α-reductase type 1 gene in the rat hippocampus. Mol. Cell. Endocrinol. 412, 330–338. doi: 10.1016/j.mce.2015.05.024
Rossi, C., Angelucci, A., Costantin, L., Braschi, C., Mazzantini, M., Babbini, F. M., et al. (2006). Brain-derived neurotrophic factor (BDNF) is required for the enhancement of hippocampal neurogenesis following environmental enrichment. Eur. J. Neurosci. 24, 1850–1856. doi: 10.1111/j.1460-9568.2006.05059.x
Rueda, A. V. L., Teixeira, A. M. A., Yonamine, M., and Camarini, R. (2012). Environmental enrichment blocks ethanol-induced locomotor sensitization and decreases BDNF levels in the prefrontal cortex in mice. Addict. Biol. 17, 736–745. doi: 10.1111/j.1369-1600.2011.00408.x
Rule, L., Yang, J., Watkin, H., Hall, J., and Brydges, N. M. (2021). Environmental enrichment rescues survival and function of adult-born neurons following early life stress. Mol. Psychiatry 26, 1898–1908. doi: 10.1038/s41380-020-0718-4
Sabzalizadeh, M., Mollashahi, M., Afarinesh, M. R., Mafi, F., Joushy, S., Sheibani, V., et al. (2022). Sex difference in cognitive behavioral alterations and barrel cortex neuronal responses in rats exposed prenatally to valproic acid under continuous environmental enrichment. Int. J. Dev. Neurosci. 82, 513–527. doi: 10.1002/jdn.10206
Sahini, S. N. M., Hazalin, N. A. M. N., Srikumar, B. N., Chellammal, H. S. J., and Singh, G. K. S. (2024). Environmental enrichment improves cognitive function, learning, memory and anxiety-related behaviours in rodent models of dementia: Implications for future study. Neurobiol. Learn. Mem. 208:107880. doi: 10.1016/j.nlm.2023.107880
Sakalem, M. E., Seidenbecher, T., Zhang, M., Saffari, R., Kravchenko, M., Wördemann, S., et al. (2017). Environmental enrichment and physical exercise revert behavioral and electrophysiological impairments caused by reduced adult neurogenesis. Hippocampus 27, 36–51. doi: 10.1002/hipo.22669
Salmin, V. V., Komleva, Y. K., Kuvacheva, N. V., Morgun, A. V., Khilazheva, E. D., Lopatina, O. L., et al. (2017). Differential roles of environmental enrichment in Alzheimer's type of neurodegeneration and physiological aging. Front. Aging Neurosci. 9:245. doi: 10.3389/fnagi.2017.00245
Sampedro-Piquero, P., Begega, A., and Arias, J. L. (2014). Increase of glucocorticoid receptor expression after environmental enrichment: relations to spatial memory, exploration and anxiety-related behaviors. Physiol. Behav. 129, 118–129. doi: 10.1016/j.physbeh.2014.02.048
Sampedro-Piquero, P., Zancada-Menendez, C., Begega, A., Rubio, S., and Arias, J. L. (2013). Effects of environmental enrichment on anxiety responses, spatial memory and cytochrome c oxidase activity in adult rats. Brain Res. Bull. 98, 1–9. doi: 10.1016/j.brainresbull.2013.06.006
Sandoval-Quintana, E., Stangl, C., Huang, L., Renkens, I., Duran, R., van Haaften, G., et al. (2023). CRISPR-Cas9 enrichment, a new strategy in microbial metagenomics to investigate complex genomic regions: the case of an environmental integron. Mol. Ecol. Resour. 23, 1288–1298. doi: 10.1111/1755-0998.13798
Schapiro, S. J. (2002). Effects of social manipulations and environmental enrichment on behavior and cell-mediated immune responses in rhesus macaques. Pharmacol. Biochem. Behav. 73, 271–278. doi: 10.1016/S0091-3057(02)00779-7
Schapiro, S. J., Bloomsmith, M. A., Suarez, S. A., and Porter, L. M. (1995). Maternal behavior of primiparous rhesus monkeys: effects of limited social restriction and inanimate environmental enrichment. Appl. Animal Behav. Sci. 45, 139–149. doi: 10.1016/0168-1591(95)00587-I
Schloesser, R. J., Lehmann, M., Martinowich, K., Manji, H. K., and Herkenham, M. (2010). Environmental enrichment requires adult neurogenesis to facilitate the recovery from psychosocial stress. Mol. Psychiatry 15, 1152–1163. doi: 10.1038/mp.2010.34
Schneider, T., Turczak, J., and Przewłocki, R. (2006). Environmental enrichment reverses behavioral alterations in rats prenatally exposed to valproic acid: Issues for a therapeutic approach in autism. Neuropsychopharmacology 31, 36–46. doi: 10.1038/sj.npp.1300767
Schrijver, N. C., Bahr, N. I., Weiss, I. C., and Würbel, H. (2002). Dissociable effects of isolation rearing and environmental enrichment on exploration, spatial learning and HPA activity in adult rats. Pharmacol. Biochem. Behav. 73, 209–224. doi: 10.1016/S0091-3057(02)00790-6
Scott, K., Taylor, L., Gill, B. P., and Edwards, S. A. (2007). Influence of different types of environmental enrichment on the behaviour of finishing pigs in two different housing systems: 2. Ratio of pigs to enrichment. Appl. Animal Behav. Sci. 105, 51–58. doi: 10.1016/j.applanim.2006.05.042
Segovia, G., Arco, A. D., De Blas, M., Garrido, P., and Mora, F. (2010). Environmental enrichment increases the in vivo extracellular concentration of dopamine in the nucleus accumbens: a microdialysis study. J. Neural Transm. 117, 1123–1130. doi: 10.1007/s00702-010-0447-y
Segovia, G., Arco, A. D., Garrido, P., de Blas, M., and Mora, F. (2008). Environmental enrichment reduces the response to stress of the cholinergic system in the prefrontal cortex during aging. Neurochem. Int. 52, 1198–1203. doi: 10.1016/j.neuint.2007.12.007
Seo, J. H., Kang, S.- W., Kim, K., Wi, S., Lee, J. W., Cho, S. R., et al. (2020). Environmental enrichment attenuates oxidative stress and alters detoxifying enzymes in an a53t α-synuclein transgenic mouse model of Parkinson's disease. Antioxidants 9, 1–17. doi: 10.3390/antiox9100928
Seo, J. H., Pyo, S., Shin, Y.-K., Nam, B.-G., Kang, J. W., Kim, K. P., et al. (2018). The effect of environmental enrichment on glutathione-mediated xenobiotic metabolism and antioxidation in normal adult mice. Front. Neurol. 9:425. doi: 10.3389/fneur.2018.00425
Sherwin, C. M., Lewis, P. D., and Perry, G. C. (1999a). The effects of environmental enrichment and intermittent lighting on the behaviour and welfare of male domestic turkeys. Appl. Anim. Behav. Sci. 62, 319–333. doi: 10.1016/S0168-1591(98)00215-9
Sherwin, C. M., Lewis, P. D., and Perry, G. C. (1999b). Effects of environmental enrichment, fluorescent and intermittent lighting on injurious pecking amongst male turkey poults. Br. Poult. Sci. 40, 592–598. doi: 10.1080/00071669986954
Shibagaki, M., Seo, M., Asano, T., and Kiyono, S. (1981). Environmental enrichment to alleviate maze performance deficits in rats with microcephaly induced by X-irradiation. Physiol. Behav. 27, 797–802. doi: 10.1016/0031-9384(81)90045-7
Shilpa, B. M., Bhagya, V., Harish, G., Bharath, M. M. S., and Rao, B. S. S. (2017). Environmental enrichment ameliorates chronic immobilisation stress-induced spatial learning deficits and restores the expression of BDNF, VEGF, GFAP and glucocorticoid receptors. Progr. Neuro-Psychopharmacol. Biol. Psychiat. 76, 88–100. doi: 10.1016/j.pnpbp.2017.02.025
Simonet, N. G., Reyes, M., Nardocci, G., Molina, A., and Alvarez, M. (2013). Epigenetic regulation of the ribosomal cistron seasonally modulates enrichment of H2A.Z and H2A.Zub in response to different environmental inputs in carp (Cyprinus carpio). Epigenet. Chrom. 6, 1–13. doi: 10.1186/1756-8935-6-22
Singhal, G., Jaehne, E. J., Corrigan, F., and Baune, B. T. (2014). Cellular and molecular mechanisms of immunomodulation in the brain through environmental enrichment. Front. Cell. Neurosci. 8:97. doi: 10.3389/fncel.2014.00097
Singhal, G., Morgan, J., Corrigan, F., Toben, C., Jawahar, M. C., Jaehne, E. J., et al. (2021). Short-term environmental enrichment is a stronger modulator of brain glial cells and cervical lymph node t cell subtypes than exercise or combined exercise and enrichment. Cell. Molec. Neurobiol. 41, 469–486. doi: 10.1007/s10571-020-00862-x
Singhal, G., Morgan, J., Jawahar, M. C., Corrigan, F., Jaehne, E. J., Toben, C., et al. (2019a). The effects of short-term and long-term environmental enrichment on locomotion, mood-like behavior, cognition and hippocampal gene expression. Behav. Brain Res. 368:111917. doi: 10.1016/j.bbr.2019.111917
Singhal, G., Morgan, J., Jawahar, M. C., Corrigan, F., Jaehne, E. J., Toben, C., et al. (2019b). Short-term environmental enrichment, and not physical exercise, alleviate cognitive decline and anxiety from middle age onwards without affecting hippocampal gene expression. Cogn. Affect. Behav. Neurosci. 19, 1143–1169. doi: 10.3758/s13415-019-00743-x
Singhal, G., Morgan, J., Jawahar, M. C., Corrigan, F., Jaehne, E. J., Toben, C., et al. (2020). Duration of environmental enrichment determines astrocyte number and cervical lymph node T lymphocyte proportions but not the microglial number in middle-aged C57BL/6 mice. Front. Cell. Neurosci. 14:57. doi: 10.3389/fncel.2020.00057
Skwara, A. J., Karwoski, T. E., Czambel, R. K., Rubin, R. T., and Rhodes, M. E. (2012). Influence of environmental enrichment on hypothalamic-pituitary-adrenal (HPA) responses to single-dose nicotine, continuous nicotine by osmotic mini-pumps, nicotine withdrawal by mecamylamine in male and female rats. Behav. Brain Res. 234, 1–10. doi: 10.1016/j.bbr.2012.06.003
Smith, H. V. (1972). Effects of environmental enrichment on open-field activity and Hebb-Williams problem solving in rats. J. Comp. Physiol. Psychol. 80, 163–168. doi: 10.1037/h0032841
Smith, M. A., Cole, K. T., Gergans, S. R., Iordanou, J. C., Lyle, M. A., Schmidt, K. T., et al. (2008). Effects of environmental enrichment on sensitivity to mu, kappa, mixed-action opioids in female rats. Physiol. Behav. 94, 563–568. doi: 10.1016/j.physbeh.2008.03.015
Smith, M. A., Iordanou, J. C., Cohen, M. B., Cole, K. T., Gergans, S. R., Lyle, M. A., et al. (2009). Effects of environmental enrichment on sensitivity to cocaine in female rats: Importance of control rates of behavior. Behav. Pharmacol. 20, 312–321. doi: 10.1097/FBP.0b013e32832ec568
Sneddon, I. A., Beattie, V. E., Dunne, L., and Neil, W. (2000). The effect of environmental enrichment on learning in pigs. Animal Welfare, 9, 373–383. doi: 10.1017/S096272860002296X
Soares, R. O., Rorato, R. C., Padovan, D., Lachat, J.-J., Antunes-Rodrigues, J., Elias, L. L. K., et al. (2015). Environmental enrichment reverses reduction in glucocorticoid receptor expression in the hippocampus of and improves behavioral responses of anxiety in early malnourished rats. Brain Res. 1600, 32–41. doi: 10.1016/j.brainres.2014.12.047
Soffi,é, M., Hahn, K., Terao, E., and Eclancher, F. (1999). Behavioural and glial changes in old rats following environmental enrichment. Behav. Brain Res. 101, 37–49. doi: 10.1016/S0166-4328(98)00139-9
Solinas, M., Chauvet, C., Thiriet, N., El Rawas, R., and Jaber, M. (2008). Reversal of cocaine addiction by environmental enrichment. Proc. Natl. Acad. Sci. 105, 17145–17150. doi: 10.1073/pnas.0806889105
Solinas, M., Thiriet, N., Chauvet, C., and Jaber, M. (2010). Prevention and treatment of drug addiction by environmental enrichment. Progr. Neurobiol. 92, 572–592. doi: 10.1016/j.pneurobio.2010.08.002
Solinas, M., Thiriet, N., Rawas, R. E., Lardeux, V., and Jaber, M. (2009). Environmental enrichment during early stages of life reduces the behavioral, neurochemical, molecular effects of cocaine. Neuropsychopharmacology 34, 1102–1111. doi: 10.1038/npp.2008.51
Song, M. K., Kim, Y. J., Kim, S. H., Yeo, S. G., and Kim, Y. J. (2021). Environmental enrichment modulates silent information regulator 1 (SIRT1) activity to attenuate central presbycusis in a rat model of normal aging. Exper. Gerontol. 155:111552. doi: 10.1016/j.exger.2021.111552
Sparling, J. E., Mahoney, M., Baker, S., and Bielajew, C. (2010). The effects of gestational and postpartum environmental enrichment on the mother rat: a preliminary investigation. Behav. Brain Res. 208, 213–223. doi: 10.1016/j.bbr.2009.11.041
Speisman, R. B., Kumar, A., Rani, A., Pastoriza, J. M., Severance, J. E., Foster, T. C., et al. (2013). Environmental enrichment restores neurogenesis and rapid acquisition in aged rats. Neurobiol. Aging 34, 263–274. doi: 10.1016/j.neurobiolaging.2012.05.023
Stairs, D. J., and Bardo, M. T. (2009). Neurobehavioral effects of environmental enrichment and drug abuse vulnerability. Pharmacol. Biochem. Behav. 92, 377–382. doi: 10.1016/j.pbb.2009.01.016
Stairs, D. J., Kangiser, M., Hickle, T., and Bockman, C. S. (2016). “Effects of environmental enrichment on nicotine addiction,” in Neuropathology of Drug Addictions and Substance Misuse Volume 1: Foundations of Understanding, Tobacco, Alcohol, Cannabinoids and Opioids (Elsevier), 246–253. doi: 10.1016/B978-0-12-800213-1.00023-7
Stam, N. C., Nithianantharajah, J., Howard, M. L., Atkin, J. D., Cheema, S. S., Hannan, A. J., et al. (2008). Sex-specific behavioural effects of environmental enrichment in a transgenic mouse model of amyotrophic lateral sclerosis. Eur. J. Neurosci. 28, 717–723. doi: 10.1111/j.1460-9568.2008.06374.x
Stein, L. R., O'Dell, K. A., Funatsu, M., Zorumski, C. F., and Izumi, Y. (2016). Short-term environmental enrichment enhances synaptic plasticity in hippocampal slices from aged rats. Neuroscience 329, 294–305. doi: 10.1016/j.neuroscience.2016.05.020
Stuart, K. E., King, A. E., King, N. E., Collins, J. M., Vickers, J. C., Ziebell, J. M., et al. (2019). Late-life environmental enrichment preserves short-term memory and may attenuate microglia in male APP/PS1 mice. Neuroscience 408, 282–292. doi: 10.1016/j.neuroscience.2019.04.015
Suárez, A., Fabio, M. C., Bellia, F., Fernández, M. S., and Pautassi, R. M. (2020). Environmental enrichment during adolescence heightens ethanol intake in female, but not male, adolescent rats that are selectively bred for high and low ethanol intake during adolescence. Am. J. Drug Alcohol Abuse 46, 553–564. doi: 10.1080/00952990.2020.1770778
Sun, H., Zhang, J., Zhang, L., Liu, H., Zhu, H., Yang, Y., et al. (2010). Environmental enrichment influences BDNF and NR1 levels in the hippocampus and restores cognitive impairment in chronic cerebral hypoperfused rats. Curr. Neurovasc. Res. 7, 268–280. doi: 10.2174/156720210793180819
Sztainberg, Y., Kuperman, Y., Tsoory, M., Lebow, M., and Chen, A. (2010). The anxiolytic effect of environmental enrichment is mediated via amygdalar CRF receptor type 1. Mol. Psychiatry 15, 905–917. doi: 10.1038/mp.2009.151
Takahashi, T., Shimizu, K., Shimazaki, K., Toda, H., and Nibuya, M. (2014). Environmental enrichment enhances autophagy signaling in the rat hippocampus. Brain Res. 1592, 113–123. doi: 10.1016/j.brainres.2014.10.026
Tan, X. D., Liu, B., Jiang, Y., Yu, H. J., and Li, C. Q. (2021). Gadd45b mediates environmental enrichment-induced neurogenesis in the SVZ of rats following ischemia stroke via BDNF. Neurosci. Lett. 745:135616. doi: 10.1016/j.neulet.2020.135616
Tanaś, Ł., Ostaszewski, P., and Iwan, A. (2015). Effects of post-weaning social isolation and environmental enrichment on exploratory behavior and anxiety in Wistar rats. Acta Neurobiol. Exp. 75, 72–79. doi: 10.55782/ane-2015-2017
Tang, B. (2020). Axon regeneration induced by environmental enrichment- epigenetic mechanisms. Neural Regener. Res. 15, 10–15. doi: 10.4103/1673-5374.264440
Tanti, A., Westphal, W.-P., Girault, V., Brizard, B., Devers, S., Leguisquet, A.-M., et al. (2013). Region-dependent and stage-specific effects of stress, environmental enrichment, antidepressant treatment on hippocampal neurogenesis. Hippocampus 23, 797–811. doi: 10.1002/hipo.22134
Tatemoto, P., Bernardino, T., Alves, L., Oliveira, S.ouza, d. e., Palme, A. C., Zanella, R. A. J., et al. (2019). Environmental enrichment for pregnant sows modulates HPA-axis and behavior in the offspring. Appl. Animal Behav. Sci. 220:104854. doi: 10.1016/j.applanim.2019.104854
Thelwall, M., and Sud, P. (2022). Scopus 1900–2020: Growth in articles, abstracts, countries, fields, journals. Quantit. Sci. Stud. 3, 37–50. doi: 10.1162/qss_a_00177
Thiel, K. J., Sanabria, F., Pentkowski, N. S., and Neisewander, J. L. (2009). Anti-craving effects of environmental enrichment. Int. J. Neuropsychopharmacol. 12, 1151–1156. doi: 10.1017/S1461145709990472
Thiriet, N., Amar, L., Toussay, X., Lardeux, V., Ladenheim, B., Becker, K. G., et al. (2008). Environmental enrichment during adolescence regulates gene expression in the striatum of mice. Brain Res. 1222, 31–41. doi: 10.1016/j.brainres.2008.05.030
Toma, D. E., Ortega, I., Catuara-Solarz, M., Sierra, S., Sabidó, C., Dierssen, E., et al. (2020). Re-establishment of the epigenetic state and rescue of kinome deregulation in Ts65Dn mice upon treatment with green tea extract and environmental enrichment. Sci. Rep. 10:16023. doi: 10.1038/s41598-020-72625-z
Tomiga, Y., Ito, A., Sudo, M., Ando, S., Maruyama, A., Nakashima, S., et al. (2016). Effects of environmental enrichment in aged mice on anxiety-like behaviors and neuronal nitric oxide synthase expression in the brain. Biochem. Biophys. Res. Commun. 476, 635–640. doi: 10.1016/j.bbrc.2016.06.010
Torasdotter, M., Metsis, M., Henriksson, B. G., Winblad, B., and Mohammed, A. H. (1998). Environmental enrichment results in higher levels of nerve growth factor mRNA in the rat visual cortex and hippocampus. Behav. Brain Res. 93, 83–90. doi: 10.1016/S0166-4328(97)00142-3
Torres-Lista, V., and Giménez-Llort, L. (2015). Early postnatal handling and environmental enrichment improve the behavioral responses of 17-month-old 3xTg-AD and non-transgenic mice in the Forced Swim Test in a gender-dependent manner. Behav. Proc. 120, 120–127. doi: 10.1016/j.beproc.2015.09.011
Turner, C. A., and Lewis, M. H. (2003). Environmental enrichment: effects on stereotyped behavior and neurotrophin levels. Physiol. Behav. 80, 259–266. doi: 10.1016/j.physbeh.2003.07.008
Valero-Aracama, M. J., Sauvage, M. M., and Yoshida, M. (2015). Environmental enrichment modulates intrinsic cellular excitability of hippocampal CA1 pyramidal cells in a housing duration and anatomical location-dependent manner. Behav. Brain Res. 292, 209–218. doi: 10.1016/j.bbr.2015.05.032
Van de Weerd, H. A., Van Loo, P. L., Van Zutphen, L. F., Koolhaas, J. M., and Baumans, V. (1997). Nesting material as environmental enrichment has no adverse effects on behavior and physiology of laboratory mice. Physiol. Behav. 62, 1019–1028. doi: 10.1016/S0031-9384(97)00232-1
Varty, G. B., Paulus, M. P., Braff, D. L., and Geyer, M. A. (2000). Environmental enrichment and isolation rearing in the rat: effects on locomotor behavior and startle response plasticity. Biol. Psychiatry 47, 864–873. doi: 10.1016/S0006-3223(99)00269-3
Vasdal, G., Vas, J., Newberry, R. C., and Moe, R. O. (2019). Effects of environmental enrichment on activity and lameness in commercial broiler production. J. Appl. Animal Welfare Sci. 22, 197–205. doi: 10.1080/10888705.2018.1456339
Venable, N., Fernández, V., Díaz, E., and Pinto-Hamuy, T. (1989). Effects of preweaning environmental enrichment on basilar dendrites of pyramidal neurons in occipital cortex: a Golgi study. Dev. Brain Res. 49, 140–144. doi: 10.1016/0165-3806(89)90068-0
Venable, N., Pinto-Hamuy, T., Arraztoa, J. A., Contador, M. T., Chellew, A., Perán, C., et al. (1988). Greater efficacy of preweaning than postweaning environmental enrichment on maze learning in adult rats. Behav. Brain Res. 31, 89–92. doi: 10.1016/0166-4328(88)90162-3
Ventura, S., Duncan, S., and Ainge, J. A. (2024). Increased flexibility of CA3 memory representations following environmental enrichment. Curr. Biol. 34, 2011–2019.e7. doi: 10.1016/j.cub.2024.03.054
Vogel, W., Kun, K. J., and Meshorer, E. (1967). Effects of environmental enrichment and environmental deprivation on cognitive functioning in institutionalized retardates. J. Consult. Psychol. 31, 570–576. doi: 10.1037/h0025166
Vogel, W., Kun, K. J., and Meshorer, E. (1968). Changes in adaptive behavior in institutionalized retardates in response to environmental enrichment or deprivation. J. Consult. Clin. Psychol. 32, 76–82. doi: 10.1037/h0025454
Wagner, A. K., Chen, X., Kline, A. E., Li, Y., Zafonte, R. D., Dixon, C. E., et al. (2005). Gender and environmental enrichment impact dopamine transporter expression after experimental traumatic brain injury. Exp. Neurol. 195, 475–483. doi: 10.1016/j.expneurol.2005.06.009
Wagner, A. K., Kline, A. E., Sokoloski, J., Zafonte, R. D., Capulong, E., Dixon, C. E., et al. (2002). Intervention with environmental enrichment after experimental brain trauma enhances cognitive recovery in male but not female rats. Neurosci. Lett. 334, 165–168. doi: 10.1016/S0304-3940(02)01103-5
Wainwright, P. E., Huang, Y. S., Bulman-Fleming, B., Lévesque, S., and McCutcheon, D. (1994). The effects of dietary fatty acid composition combined with environmental enrichment on brain and behavior in mice. Behav. Brain Res. 60, 125–136. doi: 10.1016/0166-4328(94)90139-2
Walsh, R. N., Cummins, R. A., Budtz-Olsen, O. E., and Torok, A. (1972). Effects of environmental enrichment and deprivation on rat frontal cortex. Int. J. Neurosci. 4, 239–242. doi: 10.3109/00207457209149780
Wang, R., Hausknecht, K. A., Shen, Y.-L., Haj-Dahmane, S., Vezina, P., Shen, R.-Y., et al. (2018). Environmental enrichment reverses increased addiction risk caused by prenatal ethanol exposure. Drug Alcohol Depend. 191, 343–347. doi: 10.1016/j.drugalcdep.2018.07.013
Wang, X.- M., Zhang, G.- F., Jia, M., Xie, Z.- M., Yang, J.- J., Shen, J. C., et al. (2019). Environmental enrichment improves pain sensitivity, depression-like phenotype, memory deficit in mice with neuropathic pain: role of NPAS4. Psychopharmacology 236, 1999–2014. doi: 10.1007/s00213-019-5187-6
Wang, Y., Bontempi, B., Hong, S. M., Mehta, K., Weinstein, P. R., Abrams, G. M., et al. (2008). A comprehensive analysis of gait impairment after experimental stroke and the therapeutic effect of environmental enrichment in rats. J. Cerebr. Blood Flow Metabol. 28, 1936–1950. doi: 10.1038/jcbfm.2008.82
Wei, F., Xian, D., He, Y., Yan, Z., Deng, X., Chen, Y. Y., et al. (2022). Effects of maternal deprivation and environmental enrichment on anxiety-like and depression-like behaviors correlate with oxytocin system and CRH level in the medial-lateral habenula. Peptides 158:170882. doi: 10.1016/j.peptides.2022.170882
Wei, Z., Meng, X., El Fatimy, R., Sun, B., Mai, D., Zhang, J. S., et al. (2020). Environmental enrichment prevents Aβ oligomer-induced synaptic dysfunction through mirna-132 and hdac3 signaling pathways. Neurobiol. Dis. 134:104617. doi: 10.1016/j.nbd.2019.104617
Weiner, S. P., Vasquez, C., Song, S., Zhao, K., Ali, O., Rosenkilde, D., et al. (2024). Sex difference in the effect of environmental enrichment on food restriction-induced persistence of cocaine conditioned place preference and mechanistic underpinnings. Addict. Neurosci. 10:100142. doi: 10.1016/j.addicn.2024.100142
Welberg, L., Thrivikraman, K. V., and Plotsky, P. M. (2006). Combined pre- and postnatal environmental enrichment programs the HPA axis differentially in male and female rats. Psychoneuroendocrinology 31, 553–564. doi: 10.1016/j.psyneuen.2005.11.011
Widman, D. R., Abrahamsen, G. C., and Rosellini, R. A. (1992). Environmental enrichment: The influences of restricted daily exposure and subsequent exposure to uncontrollable stress. Physiol. Behav. 51, 309–318. doi: 10.1016/0031-9384(92)90146-S
Will, B., Galani, R., Kelche, C., and Rosenzweig, M. R. (2004). Recovery from brain injury in animals: relative efficacy of environmental enrichment, physical exercise or formal training (1990–2002). Progr. Neurobiol. 72, 167–182. doi: 10.1016/j.pneurobio.2004.03.001
Will, B. E. (1977). Relatively brief environmental enrichment aids recovery of learning capacity and alters brain measures after postweaning brain lesions in rats. J. Comp. Physiol. Psychol. 91, 33–50. doi: 10.1037/h0077306
Williams, B. M., Luo, Y., Ward, C., Redd, K., Gibson, R., Kuczaj, S. A., et al. (2001). Environmental enrichment: effects on spatial memory and hippocampal CREB immunoreactivity. Physiol. Behav. 73, 649–658. doi: 10.1016/S0031-9384(01)00543-1
Williamson, L. L., Chao, A., and Bilbo, S. D. (2012). Environmental enrichment alters glial antigen expression and neuroimmune function in the adult rat hippocampus. Brain, Behav. Immun. 26, 500–510. doi: 10.1016/j.bbi.2012.01.003
Wood, D. A., Siegel, A. K., and Rebec, G. V. (2006). Environmental enrichment reduces impulsivity during appetitive conditioning. Physiol. Behav. 88, 132–137. doi: 10.1016/j.physbeh.2006.03.024
Wood, N. I., Carta, V., Milde, S., Skillings, E. A., McAllister, C. J., Ang, Y. M., et al. (2010). Responses to environmental enrichment differ with sex and genotype in a transgenic mouse model of Huntington's disease. PLoS ONE 5:e9077. doi: 10.1371/journal.pone.0009077
Wood, W. (1998). Interactions among environmental enrichment, viewing crowds, and zoo chimpanzees (Pan troglodytes). Zoo Biol. 17, 211–230. doi: 10.1002/(SICI)1098-2361(1998)17:3<211::AID-ZOO5>3.0.CO;2-C
Woodcock, E. A., and Richardson, R. (2000). Effects of environmental enrichment on rate of contextual processing and discriminative ability in adult rats. Neurobiol. Learn. Mem. 73, 1–10. doi: 10.1006/nlme.1999.3911
Wooters, T. E., Bardo, M. T., Dwoskin, L. P., Midde, N. M., Gomez, A. M., Mactutus, C. F., et al. (2011). Effect of environmental enrichment on methylphenidate-induced locomotion and dopamine transporter dynamics. Behav. Brain Res. 219, 98–107. doi: 10.1016/j.bbr.2011.01.001
Workman, J. L., DeWitt, S. J., Fonken, L. K., and Nelson, R. J. (2010). Environmental enrichment enhances delayed-type hypersensitivity in both short- and long-day Siberian hamsters. Physiol. Behav. 99, 638–643. doi: 10.1016/j.physbeh.2010.01.029
Workman, J. L., Fonken, L. K., Gusfa, J., Kassouf, K. M., and Nelson, R. J. (2011). Post-weaning environmental enrichment alters affective responses and interacts with behavioral testing to alter nNOS immunoreactivity. Pharmacol. Biochem. Behav. 100, 25–32. doi: 10.1016/j.pbb.2011.07.008
Xie, T., Wang, W. P., Jia, L. J., Mao, Z. F., Qu, Z. Z., Luan, S. Q., et al. (2012). Environmental enrichment restores cognitive deficits induced by prenatal maternal seizure. Brain Res. 1470, 80–88. doi: 10.1016/j.brainres.2012.06.034
Xu, H., Li, B., Li, L., Fan, Z., Gong, X., Wu, L., et al. (2022). Environmental enrichment mitigates PTSD-like behaviors in adult male rats exposed to early life stress by regulating histone acetylation in the hippocampus and amygdala. J. Psychiatr. Res. 155, 120–136. doi: 10.1016/j.jpsychires.2022.07.067
Yan, J., Liu, Y., Zheng, F., Lv, D., and Jin, D. (2023). Environmental enrichment enhanced neurogenesis and behavioral recovery after stroke in aged rats. Aging 15:9453. doi: 10.18632/aging.205010
Yeshurun, S., Short, A. K., Bredy, T. W., Pang, T. Y., and Hannan, A. J. (2017). Paternal environmental enrichment transgenerationally alters affective behavioral and neuroendocrine phenotypes. Psychoneuroendocrinology 77, 225–235. doi: 10.1016/j.psyneuen.2016.11.013
Zai, L., Ferrari, C., Dice, C., Subbaiah, S., Havton, L. A., Coppola, G., et al. (2011). Inosine augments the effects of a Nogo receptor blocker and of environmental enrichment to restore skilled forelimb use after stroke. J. Neurosci. 31, 5977–5988. doi: 10.1523/JNEUROSCI.4498-10.2011
Zajac, M. S., Pang, T. Y. C., Wong, N., Weinrich, B., Leang, L. S. K., Craig, J. M., et al. (2010). Wheel running and environmental enrichment differentially modify exon-specific BDNF expression in the hippocampus of wild-type and pre-motor symptomatic male and female Huntington's disease mice. Hippocampus 20, 621–636. doi: 10.1002/hipo.20658
Zaragoza, F., Ibañez, M., Mas, B., Laiglesia, S., and Anzola, B. (2011). Influence of environmental enrichment in captive chimpanzees (Pan troglodytes spp.) and gorillas (Gorilla gorilla gorilla): behavior and faecal cortisol levels. Rev. Científica 21, 447–456.
Zeeb, F. D., Wong, A. C., and Winstanley, C. A. (2013). Differential effects of environmental enrichment, social-housing, isolation-rearing on a rat gambling task: Dissociations between impulsive action and risky decision-making. Psychopharmacology 225, 381–395. doi: 10.1007/s00213-012-2822-x
Zentall, T. R. (2021). Effect of environmental enrichment on the brain and on learning and cognition by animals. Animals 11:973. doi: 10.3390/ani11040973
Zhang, Y., Crofton, E. J., Fan, X., Li, D., Kong, F., Sinha, M., et al. (2016a). Convergent transcriptomics and proteomics of environmental enrichment and cocaine identifies novel therapeutic strategies for addiction. Neuroscience 339, 254–266. doi: 10.1016/j.neuroscience.2016.09.051
Zhang, Y., Kong, F., Crofton, E. J., Dragosljvich, S. N., Sinha, M., Li, D., et al. (2016b). Transcriptomics of environmental enrichment reveals a role for retinoic acid signaling in addiction. Front. Mol. Neurosci. 9:119. doi: 10.3389/fnmol.2016.00119
Zhang, Z., Zhang, H., Du, B., and Chen, Z. (2012). Neonatal handling and environmental enrichment increase the expression of GAP-43 in the hippocampus and promote cognitive abilities in prenatally stressed rat offspring. Neurosci. Lett. 522, 1–5. doi: 10.1016/j.neulet.2012.05.039
Zhu, G., Guo, M., Zhao, J., Zhang, H., Wang, G., Chen, W., et al. (2022). Bifidobacterium breve intervention combined with environmental enrichment alleviates cognitive impairment by regulating the gut microbiota and microbial metabolites in Alzheimer's disease mice. Front. Immunol. 13:1013664. doi: 10.3389/fimmu.2022.1013664
Zhu, G., Guo, M., Zhao, J., Zhang, H., Wang, G., Chen, W., et al. (2024). Environmental enrichment in combination with Bifidobacterium breve HNXY26M4 intervention amplifies neuroprotective benefits in a mouse model of Alzheimer's disease by modulating glutamine metabolism of the gut microbiome. Food Sci. Hum. Wellness 13, 982–992. doi: 10.26599/FSHW.2022.9250084
Zhu, Y. T., Zhang, Q., Xie, H. Y., Yu, K. W., Xu, G. J., Li, S. Y., et al. (2021). Environmental enrichment combined with fasudil promotes motor function recovery and axonal regeneration after stroke. Neural Regener. Res. 16, 2512–2520. doi: 10.4103/1673-5374.313048
Keywords: environmental enrichment, brain, rodents, hippocampus, learning, memory, behavior, cognition
Citation: Singhal G and Baune BT (2024) A bibliometric analysis of studies on environmental enrichment spanning 1967–2024: patterns and trends over the years. Front. Behav. Neurosci. 18:1501377. doi: 10.3389/fnbeh.2024.1501377
Received: 24 September 2024; Accepted: 11 November 2024;
Published: 04 December 2024.
Edited by:
Kai Diederich, German Federal Institute for Risk Assessment, GermanyReviewed by:
Paul Mieske, Federal Institute for Risk Assessment (BfR), GermanyCopyright © 2024 Singhal and Baune. This is an open-access article distributed under the terms of the Creative Commons Attribution License (CC BY). The use, distribution or reproduction in other forums is permitted, provided the original author(s) and the copyright owner(s) are credited and that the original publication in this journal is cited, in accordance with accepted academic practice. No use, distribution or reproduction is permitted which does not comply with these terms.
*Correspondence: Bernhard T. Baune, YmVybmhhcmQuYmF1bmVAdW5pLW11ZW5zdGVyLmRl
Disclaimer: All claims expressed in this article are solely those of the authors and do not necessarily represent those of their affiliated organizations, or those of the publisher, the editors and the reviewers. Any product that may be evaluated in this article or claim that may be made by its manufacturer is not guaranteed or endorsed by the publisher.
Research integrity at Frontiers
Learn more about the work of our research integrity team to safeguard the quality of each article we publish.